- 1Chengdu Institute of Biology, Chinese Academy of Sciences, Chengdu, China
- 2Zoîgé Wetland Ecosystem Research Station, Chinese Academy of Sciences, Aba, China
The Qinghai– Tibet Plateau (QTP), which embodies the largest area of permafrost at mid–low altitudes of the world, has been experiencing rapid permafrost degradation and changes in freeze–thaw processes for the past decades. However, the responses and potential feedbacks of the methane flux from peatlands on the QTP to changing freeze–thaw cycles (FTCs) remain unknown. In this study, we collected peat soils from the Zoîgé peatlands, the largest peatland complex on the QTP, to examine methane emissions under simulated diurnal FTC scenarios. In incubation experiments of 15 days, two freeze–thaw temperature ranges of −5 to 4°C (mild) and −15 to 4°C (intense) were applied to two sets of peat soil samples, and each of them was characterized by 100% or 80% maximum water holding capacity (MWHC). The results showed that the peak of methane emission from the peat soil occurred after the first freeze–thaw cycle (FTC1), with the highest reaching a value of 0.103 mg kg soil−1·h−1. Generally, the cumulative methane emissions were elevated by FTCs, and relative higher rates of methane emissions were found for the 2nd FTC to the 15th FTC, compared with those from low-altitude peatlands. Methane emissions were significantly correlated to the export of dissolved organic carbon (DOC) and the activities of β-D-cellobiosidase and phenol oxidase in various freeze–thaw conditions. This study highlights the importance of FTCs in stimulating methane emissions and implies that methane emissions during FTCs from high-altitude peatlands would increase under a warmer climate in the future.
Introduction
Since the Last Glacial Maximum, peatlands have accumulated more than 600 Pg organic carbon (Yu et al., 2010). Peatlands are an important source of atmospheric methane, accounting for about 10% of the global methane budget (Roulet, 2000; Frolking et al., 2011). However, methane emissions from peatlands are sensitive and vulnerable to environmental variations, and larger methane emissions when peatlands are subject to higher temperatures (Schuur et al., 2015) as the soil organic carbon decomposition would be accelerated (Natali et al., 2011).
Most of the peatlands are distributed in high latitude and high-altitude areas where the seasonal freeze–thaw is a typical surface process (Zhang and Armstrong, 2001). During the seasonal freeze–thaw periods, autumn freeze and spring thaw, with intensive diurnal freeze–thaw cycles (FTCs), were known as the “shoulder season” (Arndt et al., 2019; Pirket al., 2015). Recent studies from the northern region have reported considerable methane emissions during the periods of spring thaw (e.g., Song et al., 2012; Tokida et al., 2007) and autumn freeze (Bao et al., 2021; Raz-Yaseef et al., 2017; Pirk et al., 2015; Mastepanov et al., 2008). A significant increase of methane emissions during freeze–thaw periods has been observed from a peatland of the Sanjiang Plain, Northeast China (Yang W. et al., 2006). A large amount of methane was found to be released during the spring thaw period with great spatiotemporal heterogeneity from a peatland in Hokkaido, Japan (Tokida et al., 2007). One study even found that methane emissions during spring thaw accounted for 80% of the annual budget (Song et al., 2012). On the contrary, three to four times higher contributions of methane emissions during the autumn freeze than that of the spring thaw were observed from a tundra ecosystem in Alaska, United States (Bao et al., 2021). Another study found a methane emission burst in the early winter from tundra regions as well (Mastepanov et al., 2008). The field monitoring on a peatland of the Qinghai–Tibet Plateau (QTP) showed that methane emissions during FTCs accounted for 11 to 27% of the annual budget, and methane emissions of the autumn freeze period were higher than those of the spring thaw period (Chen et al., 2021), with the underlying mechanism remaining unknown.
The average thickness of peat in the northern region is 2.3 m (Gorham, 1991), and FTCs mainly occur in the surface soil (Jin et al., 1999; Song et al., 2006). Studies show that the FTC primarily changes the physical structure of soil by shifting the thermal and hydrological conditions, and then affects the related biological processes, including changes in plant root activities, soil enzyme activities, and microbial community composition, which consequently affect the methane production and emission (Freeman et al., 2001; Herrmann and Witter, 2002; Wang et al., 2007; Sorensen et al., 2018). Cellulose and lignin are the two most abundant soil organic compounds, and the microbial-mediated decomposition of these substances provides the main substrates for soil respiration. Among them, the hydrolysis of cellulose and hemicellulose is mainly catalyzed by cellulose, such as β-D-cellobiosidase, and the oxidation and degradation of phenolic recalcitrant-like compounds are catalyzed by ligninase, such as phenoloxidase (Chen et al., 2018).
In high altitude regions, due to the stronger diurnal temperature variations in the cold season (Dong and Huang, 2015), the seasonal freeze–thaw processes are characterized by a short period of freeze-up and long shoulder seasons of ground freezing and thawing than their counterparts in high latitudes (Yang M. et al., 2006). Under such conditions, FTCs are expected to have a similar or even stronger impact on methane emissions (Xiang et al., 2009; Wang et al., 2011). Despite the large areal extent of peatlands in the mountain regions of the world (Xu et al., 2018), there have been limited attempts to quantify their methane emissions during FTCs. Therefore, in this study we aimed to test 1) whether FTCs will enhance methane emissions from high-altitude peatlands; 2) the relationships between methane emissions and influencing factors of FTCs.
Materials and Methods
Study Site and Sample Collection
We selected the Zoîgé peatlands on the northeastern QTP as our study site. With an area extent of 4.6 × 103 km2, the Zoîgé peatlands are the largest high-altitude peatlands of the world and account for about 44% of the total peatland area of China (Liu et al., 2012; Mei Wang et al., 2014). The sampling plot was located at a peatland developed on a fluvial–pluvial fan in the interior of the Zoîgé peatlands (33o04′06″N, 102o34′31″E; 3428 m a.s.l.) (Figure 1). The thickness of the peat of the sampling plot was 2–3 m.
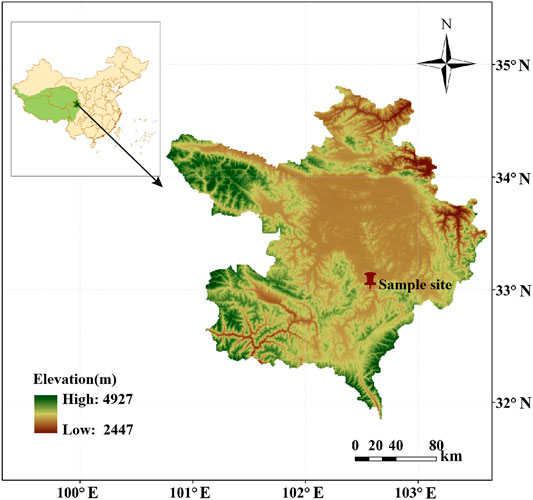
FIGURE 1. Map of the Zoîgé Plateau with the sample site location in the Ruokeba peatland. The green part of China map is QTP.
The climate in the Zoîgé peatlands belongs to cold temperate continental climate with alternating summer and winter monsoons. During the period of 2013 to 2017, the highest monthly mean air temperature ranged from 9.1 to 11.4°C in July, while the lowest one ranged from −10.9 to −8.2°C in January (Liu, 2021). The range of mean annual precipitation was approximately 656 mm, 86% of which occurred between April and October. According to field observation at 5 cm in soil (Liu, 2021), spring ground surface thawing occurred between February to April, whereas autumn ground surface freezing occurred between September to December. During the autumn ground surface freezing periods, the mean soil freezing temperature was around −5°C, while spring ground surface thawing temperature was around 4°C. Moreover, the minimum daily mean soil temperature was around −15°C. The vegetation plant species of the sampling plot were dominated by Kobresia tibetica and Carex muliensis.
We collected 20 cylindrical peat blocks (diameter, 9 cm; height, 20 cm) from the surface layer (0–20 cm) in October, 2018. Each peat soil sample was divided into two halves: one was analyzed for physical and chemical properties, while the other for incubation experiments.
Determination of Peat Samples
The pH was measured with an electronic pH meter (Sartorius P10). Total carbon and nitrogen were measured with an element analyzer (Isoprime 100, Elementar). The total organic carbon (TOC) of the soil was measured with a TOC analyzer (Vario TOC). The maximum water holding were calculated according to the procedures described in Rey et al. (2005).
Incubation Experiments
Two freeze–thaw intensities (freezing at −5°C for 12 h, then thawing at 4°C for 12 h, and freezing at −15°C for 12 h, then thawing at 4°C for 12 h. Named as mild FTCs and intense FTCs hereafter, respectively) were introduced into the incubation and two water content gradients, maximum water holding capacity of 100% (100% MWHC) for one half of the soil sample and the other half to 80% (80% MWHC). A set of control samples was incubated at 4°C (control) for reference. Therefore, the 20 peat soil samples that were collected from the field were divided into five groups, i.e., mild+100% MWHC, mild+80% MWHC, intense +100% MWHC, intense+80% MWHC and control, with four replicates in each group. For each replicate, air-dried peat soil (55 g) was placed in 250 mL flasks, all samples were kept at 4°C for 5 days until they were incubated in an adjustable temperature freezer.
The methane flux measurement for headspace gas samples was collected at 10 min intervals after 0, 1st, 3rd, 5th, 10th, and 15th FTCs (FTC0, FTC1, FTC3, FTC5, FTC10, and FTC15, respectively). The gas samples were analyzed for methane concentrations by flame ionization detection (FID) on a gas chromatograph (GC, PE Clarus 500, PerkinElmer, Inc., United States), operating at 350°C and a 2 m porapak 80-100 Q column. The column oven temperature was 35°C and the carrier gas was N2 with a flow rate of 20 cm3 min−1. The minimum detectable concentration was 1 × 10−3 μL L−1 (ppb) standard with a concentration of 4.9 μL L−1 (China National Research Center for Certified Reference Materials, Beijing).
The formula for the gas emission rate is (Liu et al., 2016)
where F represents the gas emission rate (mg kg soil−1·h−1); dc/dt (mol h−1) represents the rate of change in gas concentration; M (mg mol−1) represents the molar mass of the gas; P (Pa) represents the atmospheric pressure at the sampling site; T (K) represents the absolute room temperature during the sampling time; V (m3) represents the headspace volume of the culture flask; m (kg) indicates the dry weight of the soil sample; and V0 (m3), P0 (Pa), and T0 (K) represent the molar volume, atmospheric pressure, and absolute temperature under standard conditions, respectively.
The formula for cumulative gas emissions is (Wang, 2014)
where CE represents the cumulative gas emission (mg kg soil−1); F stands for the gas emission rate (mg kg soil−1·h−1); i represents the times of gas collection; Ti +1-Ti represents the interval between two adjacent gas collections; n represents the total number of gas collections during the observation period; and 24 means 24 h in 1 day.
Dissolved organic carbon (DOC) measurement fresh peat soil (5 g) was passed through a 100-mesh sieve and was placed in a centrifuge tube. 50 ml of deionized water was added and shaken for 30 min. The supernatant was taken and passed through a 0.45- μm organic filter membrane. The concentration was determined with a TOC analyzer. We adopted the procedures of Zhang et al. (2006).
The formula for cumulative DOC output is (Wang, 2014)
where CD represents the cumulative output of DOC (g kg soil−1); D represents the output of DOC (g kg soil−1); i represents the number of FTCs; Ti+1-Ti represents the time interval between two measurements; and n represents the total number of measurements in the observation period.
β-D-cellobiosidase and phenoloxidase activity measurement fresh peat soil (2 g) was placed in a 250 ml beaker, and 200 ml of acetic acid buffer was added to make a suspension. A fluorescence plate was used for the determination of the β-D-cellobiosidase activity (with 4-MUB-β-d-glucoside and 4-MUB-β-d-cellobiosid as substrates), and a white board was used for the phenoloxidase activity (with L-DOPA as substrates). β-D-cellobiosidase was reacted for four h and the phenoloxidase substrate was reacted for 20 h at 25°C. Finally, 20 μL of sodium hydroxide was added to the fluorescent plate for comparing the color at the absorbance of 365 nm/450 nm and at the absorbance of 460 nm for the white plate (Blagodatskaya et al., 2016; Dunn and Freeman, 2018; Sorensen et al., 2018).
Statistical Analysis
The variations of the methane emission rate and methane cumulative emission, DOC, and enzyme activity under different ground surface freezing and thawing conditions were examined by a one-way analysis of variance (ANOVA). The correlation between DOC, enzyme activity, and the methane emission rate were examined by the Pearson correlation. The effects of the number of FTCs, freeze–thaw intensity, peat water content, and their interactions with the methane emission rate, DOC, and enzyme activity were tested by a multi-factor analysis of variance (MANOVA). The significant difference and extremely significant difference were indicated by p < 0.05 and p < 0.01, respectively. Statistical analyses were conducted using SPSS 17.0.
Results
Methane Emission
The methane emission rate showed significant peaks (p < 0.05) after the first freeze–thaw cycle (FTC1) in all the treatments. In terms of the emission rate of FTC1, the sequence was mild +100% MWHC > intense +100% MWHC > mild +80% MWHC > intense +80% MWHC, with their rates ranged from 0.060 to 0.103 mg kg soil−1·h−1, and mild +100% MWHC was approximately 2.5 times as control, yet FTC0, 3, 5, 10, and 15 showed no significant differences with control (Figure 2).
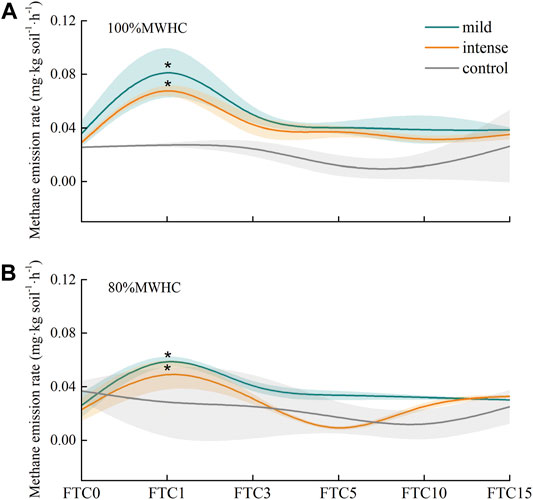
FIGURE 2. Methane emission rate in freeze–thaw cycles. (A) The methane emission rates of 100%MWHC peat; (B) the methane emission rates of 80%MWHC peat. Mild means freeze–thaw cycle at −5∼4°C; intense means freeze–thaw cycle at −15–4°C; *, there is a significant difference between freeze–thaw peat and control group (p < 0.05).
The cumulative methane emissions increased after all the FTCs compared with the control (△> 0). Similar to the emission rate of FTC1, the sequence of cumulative methane emissions was mild +100%MWHC > intense +100%MWHC > mild +80%MWHC > intense +80%MWHC, with their amounts ranged from 8.407 to 14.671 mg kg soil−1. Notably, under the 80% MWHC condition, the difference between mild and intense FTCs was significant (p = 0.031). The cumulative methane emission under 100% MWHC is higher than that under 80% MWHC, regardless of the freeze–thaw intensity (intense, p = 0.032; mild, p = 0.206) (Figure 3).
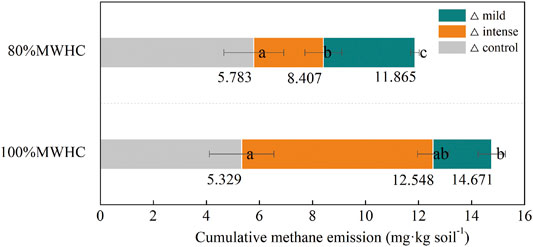
FIGURE 3. Cumulative methane emission. Mild means freeze–thaw cycle at −5∼4°C; intense means freeze–thaw cycle at −15–4°C. Triangles (△) indicate the difference of cumulative methane emission between the target setting condition and next sequence setting condition.
DOC Output
The DOC content reached its peak at FTC1in all the four treatments. Intense +80% MWHC and mild +100% MWHC showed a significant peak (p < 0.05) after FTC1. However, no significant differences were observed among the other two FTC treatments and control groups (p > 0.05). Furthermore, the DOC content of mild+80%MWHC decreased after FTC1 (Figure 4).
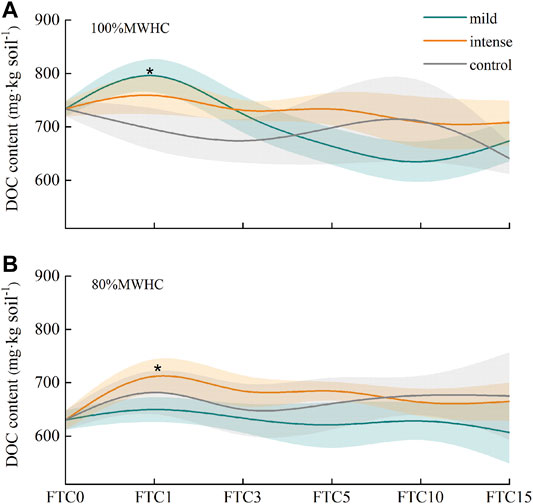
FIGURE 4. DOC content in freeze–thaw cycles. (A) The DOC outputs of 100%MWHC peat; (B) the DOC outputs of 80%MWHC peat. Mild means freeze–thaw cycle at −5∼4°C; intense means freeze–thaw cycle at −15–4°C; *, there is a significant difference between the freeze–thaw peat and control group (p < 0.05).
Under intense FTCs, the cumulative DOC output increased by 0.257 and 0.337 g kg soil−1 in 80% MWHC and 100% MWHC, respectively (△>0). Under mild FTCs, the cumulative DOC decreased by 0.603 and 0.399 g kg soil−1 in 80% MWHC and 100% MWHC, respectively (△<0). The cumulative DOC output under 100% MWHC was higher than that of 80% MWHC, and this was significant under the mild FTC (p = 0.029) (Figure 5).
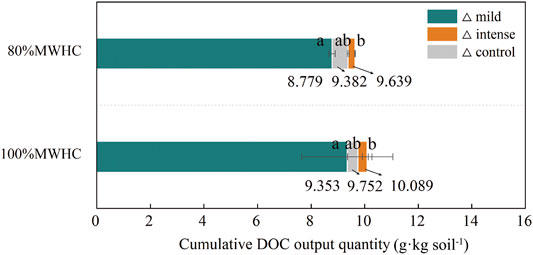
FIGURE 5. Cumulative DOC output quantity. Mild means freeze–thaw cycle at −5∼4°C; intense means freeze–thaw cycle at −15–4°C. Triangles (△) indicate the difference of cumulative DOC output quantity between the target setting condition and next sequence setting condition.
Enzyme Activity
The β-D-cellobiosidase activity decreased significantly after FTC1 and remained stable until the end of 15 FTCs under mild freeze–thaw conditions, the mild +80% MWHC decreased significantly compared with FTC0 (Figure 6A), and the mild +100%MWHC increased significantly at FTC1 (Figure 6B) (p < 0.05). Moreover, the β-D-cellobiosidase activity fluctuated and reached its minimum in FTC5 under intense FTC (Figure 6).
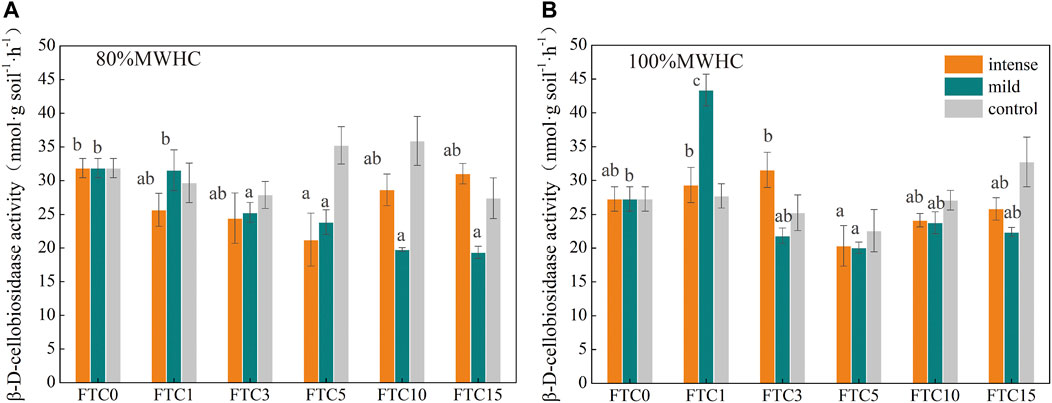
FIGURE 6. β-D-cellobiosidase activity changes during the freeze–thaw cycles. (A) the β-D-cellobiosidase activity of 80%MWHC peat; (B) the β-D-cellobiosidase activity of 100%MWHC peat; mild means freeze–thaw cycle at −5∼4°C; intense means freeze–thaw cycle at −15∼4°C.
Under intense +80% MWHC, the phenol oxidase activity decreased significantly after FTC1, and dropped to the lowest value after FTC3, whereas it remained stable in mild +80%MWHC (Figure 7A). Under intense +100% MWHC, the phenol oxidase activity was the highest in FTC1 while it increased significantly after FTC1 and FTC3, and was the highest after FTC3 under mild +100%MWHC (Figure 7B).
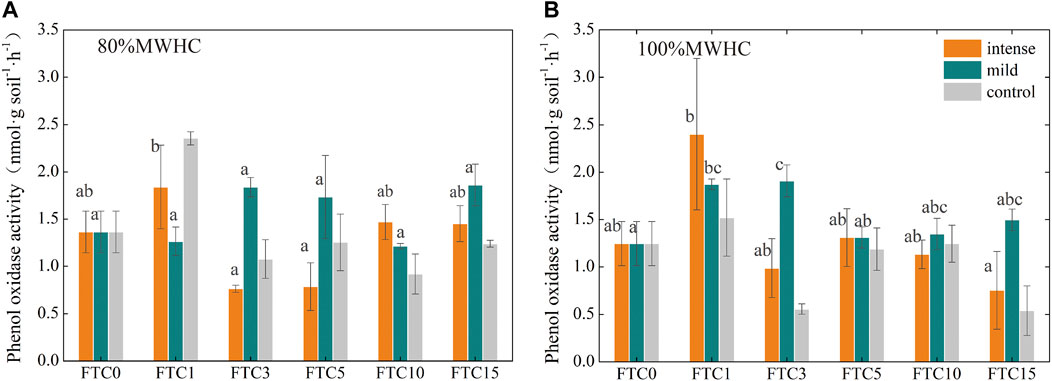
FIGURE 7. Phenol oxidase activity changes during the freeze–thaw cycles. (A) The phenol oxidase activity of 80%MWHC peat; (B) the phenol oxidase activity of 100%MWHC peat. Mild means freeze–thaw cycle at −5∼4°C; intense means freeze–thaw cycle at −15∼4°C.
Effects of FTCs on Methane Release, DOC Output, and Enzyme Activity
The methane emission rate was significantly affected by FTCs in different freeze–thaw patterns. Under 80% MWHC condition, the number and intensity of FTCs and the interaction between the two significantly affected the methane emission rate (p = 0.003, 0.000, 0.003), while the number of FTCs significantly affected the methane emission rate under 100% MWHC condition (p = 0.000). Furthermore, the number of FTCs and its interaction with water content significantly affected the methane emission rate (p < 0.05) under a mild FTC condition, while the number of FTCs and water content had extremely significant impacts under the intense FTC condition (p = 0.001, 0.000).
The intensity of FTCs affected the DOC under 100% MWHC condition (p = 0.000), and the number of FTCs had a significant effect on the DOC (p = 0.003). Under the mild FTC condition, the number of FTCs, water content, and the interaction between the two significantly affected the DOC concentration (p < 0.05).
The β-D-cellobiosidase activity was significantly affected by the number of FTCs and their interaction with intensity under 100% MWHC condition (p = 0.000; p = 0.000). Both the β-D-cellobiosidase (p = 0.015) and phenoloxidase (p = 0.025) activities were significantly affected by the interactions between the number of FTCs and intensity under 80%MWHC condition. Moreover, the number of FTCs and their interaction with water content significantly affected the β-D-cellobiosidase activity (p = 0.000; p = 0.001). However, the intense FTC condition did not show obvious effects on the DOC, β-D-cellobiosidase activity, and phenoloxidase activity (p > 0.05) (Table 1).
Relationship Between Methane Emission Rate and Variables
The methane emission rate was correlated significantly to the DOC content under 100% MWHC condition (p = 0.002), whereas no significant correlation was observed under 80%MWHC condition (p = 0.410). Moreover, the DOC content was found to have significant correlations with the methane emission rate under both mild (p = 0.000) and intense (p = 0.023) FTCs under 100%MWHC condition. Generally, the methane emission rate was significantly correlated with β-D-cellobiosidase and phenoloxidase activities, regardless of the freeze–thaw intensity and soil water content (Table 2).
Discussion
Methane Emissions During the FTCs
It has already been shown that methane emissions are more significant at the beginning of continuous FTCs (Wang, 2014; Kurganova et al., 2007). As the number of FTCs increases, methane emission rate tends to decrease (Wang, 2010; Wang, 2015; Hao et al., 2007; Prieme and Christensen, 2001). Our result was consistent with the aforementioned studies. When soil freezing and thawing occurs, the water phase changes, ice crystal grows, and water migrates on soil particles and pores, thus soil structure is damaged (Xie et al., 2015; Wang et al., 2013), and gas trapped in soil gaps is squeezed out and contributes to the increasing methane emission (Shindell et al., 2009). As the soil temperature decreased during the autumn freeze period, the thermal conductivity of peat soil increased by 13 times in the process of changing from dryness and positive temperature to ice saturation and freezing (Zhou et al., 2000), resulting in the change in the heat capacity of the soil and the heat exchange flux with the atmosphere. Accompanied by the release of a biological activity compound (Tan et al., 2012) and carbon dioxide (McLain et al., 2002), with the impact of organic carbon (Tveit et al., 2012; Wu et al., 2019) and enzymes (Jiaoyue Wang et al., 2014; Kuttim et al., 2017; Chen et al., 2018) on gas emissions, the methane emissions could be enhanced. In the field observation adjacent to our sampling plot, higher methane emissions during the autumn freeze period than those in the spring thaw period was found with the emission peaks during the early autumn freezing period as well (Chen et al., 2021). Although our study only conducted simulation experiments, it could be helpful for understanding the effects of the intensity and number of FTCs on in situ high methane emissions in the early autumn freezing period.
In terms of the source strength of the methane emission induced by FTCs, our results were much lower than those found from a low-altitude peatland, where the methane emission peak ranged from 13.40 to 55.02 mg m−2·h−1, and it was 4–19 times of the control (Wang, 2014). The incubation of peat soil from the low latitude area set the FTCs as freezing at −6°C for 2 days and thawing at 6°C for 5 days, resulting in high emissions due to longer-term ground surface freezing and thawing processes. Our incubation followed the FTCs in the field and thus has more frequent daily FTCs (Chen et al., 2021). Therefore, lower methane emission rates from this study could be attributed to the different experimental settings.
Our study also showed the impact of the number of FTCs on methane emissions in various freeze–thaw treatments. Generally, the gas emission tends to decrease after the initial emission peak (Prieme and Christensen, 2001; Hao et al., 2007; Wang, 2010, 2015). In our study, the cumulative methane emission under mild+100%MWHC condition was 2.469 mg kg soil−1 during FTC1 and 12.202 mg kg soil−1 from FTC2 to FTC15, which were 3.74 and 2.61 times their counterparts in the control group, respectively. Even under intense +80%MWHC condition, the lowest increments among the four FTC conditions, the cumulative methane emission was 1.430 mg kg soil−1 during FTC1 and 6.977 mg kg soil−1 from FTC2 to FTC15, which were 2.21 and 1.36 times of their counterparts in the control group, respectively. In summary, under a variety of freeze–thaw environmental conditions, the methane emission induced by the whole FTCs was 1.45–2.75 times of that without FTCs; The cumulative effect of the number of FTCs in our study was stronger to that of the low-altitude areas (Wang, 2014; Zhang et al., 2018). Jiaoyue Wang et al. (2014) found that the cumulative methane emission was about 1.35 times of that without FTCs under mild FTCs (−6∼6°C), whereas Zhang et al. (2018) found that it was about 1.19 times and 2.85 times under mild FTCs (−5∼5°C) and intense FTCs (−15–5°C), respectively. Therefore, our finding highlights the profound effect of the number or duration of FTCs on methane emissions on the peatlands, at least during the autumn freezing period at the high altitudes. Furthermore, it indicates higher methane emissions during shoulder seasons from those high-altitude peatlands in the context of warmer climate and longer shoulder seasons.
In contrast to other studies (e.g., Zhang et al., 2018; Wang, 2014; Goldberg et al., 2008; Gao et al., 2015), this study found the greater cumulative methane emission under mild FTC than that under intense FTCs. Although a large amount of organic carbon is released from the aggregates because of freezing damage to the soil structure and more greenhouse gases will be released in a more intense freezing state, soil biological changes that induced by a higher temperature were more effective for enhancing methane emissions in the mild freezing-thawing mode. Furthermore, as plant roots have degenerated during the autumn freeze period, substrates retained in the soil for methanogenesis, whereas ammonium and nitrate components were accumulated in the soil, inhibiting methane oxidation (Groffman et al., 2006), resulting in greater methane emissions. This indicated a potential higher methane emission from high-altitude peatlands under the mild FTCs in future climate scenarios.
Effect of DOC Content and Enzyme Activity on Methane Emission
The DOC content first increased and then decreased throughout the FTCs. Similar dynamics have also been presented in other studies (Hao et al., 2007; Zhou et al., 2008; Wang, 2014). In addition, the methane emission rate was significantly positively correlated with the DOC in a variety of freeze–thaw environments. The microbial community composition can adjust the carbon utilization efficiency to the freeze–thaw processes (Herrmann and Witter, 2002; Chen et al., 2018). At FTC1, the microbial community composition changed significantly, and then gradually returned to the state before FTCs. Moreover, the active organic carbon in the aggregates was gradually released at the beginning of FTCs (Groffman et al., 2001; Bechmann et al., 2005; Wang et al., 2013), whereas the original DOC in the soil was constantly being decomposed and consumed by living microorganisms. Therefore, the DOC content decreased after multiple FTCs (Herrmann and Witter, 2002; Wang et al., 2011), and this process is compatible with the methane production.
Our study showed that the number of FTCs had significantly impact on the β-D-cellobiosidase activity, which was consistent with the results from an analysis on a low-altitude peatland (Li et al., 2019). As a catalytic enzyme in the final stage of glucose production, the activity of β-D-cellobiosidase was greatly affected by the concentration of the substrate from cellulose decomposition (Brouns et al., 2016). Moreover, in the early stage of organic matter decomposition, cellulose with a higher content was decomposed by FTCs preferentially, producing more biodegradable substrates for eutrophic bacteria, such as Pedobacter, a predominant bacterium in eutrophic water (Zheng et al., 2015). Therefore, the β-D-cellobiosidase activity was higher at the early stage of FTCs.
Phenol oxidase was the limiting factor for the decomposition of soil organic carbon in peat soil (Chen et al., 2018). The activity of phenol oxidase in peatlands is found to be related to carbon storage and DOC release (Freeman et al., 2001). The increase of phenolic compounds will inhibit the decomposition of organic carbon in soil, thus reduce the amount of substrates for methane production. Therefore, the accumulation of phenolic compounds inhibited the decomposition of DOC, resulting in the increased cumulative release of DOC this ultimately leaded to a declined methane emission, particularly under intense FTCs. As the phenol oxidase activity is thought to be enhanced under warmer climate in the future (Chen et al., 2018), the effect of altered FTCs on methane emissions from peatlands would be complicated. Therefore, more insights are needed to obtain a better understanding on methane emissions during FTCs.
Conclusion
Increased methane emissions were found after the simulated FTCs from peat soils of a high-altitude peatland on the QTP. Similar to other studies, the methane emission peak occurred after the first FTC. The longer duration of the FTCs seems to have a profound effect on elevating methane emissions in our study compared with those from low-altitude peatlands. The incubated soils with mild freeze–thaw intensity and higher water content had a higher cumulative methane emission than that of their counterparts. Furthermore, DOC, β-D-cellobiosidase, and phenol oxidase were significantly correlated to methane emissions in a variety of freeze–thaw environments. These results have suggested a higher methane emission during the seasonal freeze–thaw process from high-altitude peatlands under the projected future climate scenarios, as both longer and warmer shoulder seasons could be expected.
Data Availability Statement
The original contributions presented in the study are included in the article/Supplementary Material, further inquiries can be directed to the corresponding author.
Author Contributions
Conceptualization: DZ. Methodology: ZY, XL, LL, and DZ. Investigation: ZY, XL, and DZ. Funding acquisition: DZ and HC. Writing—original draft: DZ, ZY, and HC.
Funding
This study was partially supported by the National Natural Science Foundation of China (41571220), West Light Foundation of the Chinese Academy of Sciences (2021XBZG_XBQNXZ_A_008), and Sichuan Science and Technology Program (2019YFH0041).
Conflict of Interest
The authors declare that the research was conducted in the absence of any commercial or financial relationships that could be construed as a potential conflict of interest.
Publisher’s Note
All claims expressed in this article are solely those of the authors and do not necessarily represent those of their affiliated organizations, or those of the publisher, the editors, and the reviewers. Any product that may be evaluated in this article, or claim that may be made by its manufacturer, is not guaranteed or endorsed by the publisher.
Acknowledgments
Our sincere thanks are due to Sunita Chaudhary for her great efforts on improving the manuscript.
References
Arndt, K. A., Oechel, W. C., Goodrich, J. P., Bailey, B. A., Kalhori, A., Hashemi, J., et al. (2019). Sensitivity of Methane Emissions to Later Soil Freezing in Arctic Tundra Ecosystems. J. Geophys. Res. Biogeosci. 124 (8), 2595–2609. doi:10.1029/2019jg005242
Bao, T., Xu, X., Jia, G., Billesbach, D. P., and Sullivan, R. C. (2021). Much Stronger Tundra Methane Emissions during Autumn Freeze Than spring Thaw. Glob. Change Biol. 27, 376–387. doi:10.1111/gcb.15421
Bechmann, M. E., Kleinman, P. J. A., Sharpley, A. N., and Saporito, L. S. (2005). Freeze-Thaw Effects on Phosphorus Loss in Runoff from Manured and Catch-Cropped Soils. J. Environ. Qual. 34, 2301–2309. doi:10.2134/jeq2004.0415
Blagodatskaya, Е., Blagodatsky, S., Khomyakov, N., Myachina, O., and Kuzyakov, Y. (2016). Temperature Sensitivity and Enzymatic Mechanisms of Soil Organic Matter Decomposition along an Altitudinal Gradient on Mount Kilimanjaro. Sci. Rep. 6 (1), 22240–22311. doi:10.1038/srep22240
Brouns, K., Keuskamp, J. A., Potkamp, G., Verhoeven, J. T. A., and Hefting, M. M. (2016). Peat Origin and Land Use Effects on Microbial Activity, Respiration Dynamics and Exo-Enzyme Activities in Drained Peat Soils in the Netherlands. Soil Biol. Biochem. 95, 144–155. doi:10.1016/j.soilbio.2015.11.018
Chen, H., Liu, X. W., Xue, D., Zhu, D., Zhan, W., Li, W., et al. (2021). Methane Emissions during Different Freezing-Thawing Periods from a Fen on the Qinghai-Tibetan Plateau: Four Years of Measurements. Agric. For. Meteorology 297, 1–13. doi:10.1016/j.agrformet.2020.108279
Chen, J., Luo, Y., García-Palacios, P., Cao, J., Dacal, M., Zhou, X., et al. (2018). Differential Responses of Carbon-Degrading Enzyme Activities to Warming: Implications for Soil Respiration. Glob. Change Biol. 24, 4816–4826. doi:10.1111/gcb.14394
Dong, D. H., and Huang, G. (2015). Relationship between Altitude and Variation Characteristics of the Maximum Temperature, Minimum Temperature, and Diurnal Temperature Range in China. Chin. J. Atmos. Sci. 39 (05), 1011–1024. (in Chinese). doi:10.3878/j.issn.1006-9895.1501.14291
Dunn, C., and Freeman, C. (2018). The Role of Molecular Weight in the Enzyme-Inhibiting Effect of Phenolics: the Significance in Peatland Carbon Sequestration. Ecol. Eng. 114, 162–166. doi:10.1016/j.ecoleng.2017.06.036
Freeman, C., Evans, C. D., Monteith, D. T., Reynolds, B., and Fenner, N. (2001). Export of Organic Carbon from Peat Soils. Nature 412, 785. doi:10.1038/35090628
Frolking, S., Talbot, J., Jones, M. C., Treat, C. C., Kauffman, J. B., Tuittila, E.-S., et al. (2011). Peatlands in the Earth's 21st century Climate System. Environ. Rev. 19, 371–396. doi:10.1139/a11-014
Gao, Y., Zeng, X., Xie, Q., and Ma, X. (2015). Release of Carbon and Nitrogen from Alpine Soils during Thawing Periods in the Eastern Qinghai-Tibet Plateau. Water Air Soil Pollut. 226 (7), 209–218. doi:10.1007/s11270-015-2479-2
Goldberg, S. D., Muhr, J., Borken, W., and Gebauer, G. (2008). Fluxes of Climate-Relevant Trace Gases between a Norway spruce forest Soil and Atmosphere during Repeated Freeze-Thaw Cycles in Mesocosms. Z. Pflanzenernähr. Bodenk. 171, 729–739. doi:10.1002/jpln.200700317
Gorham, E. (1991). Northern Peatlands: Role in the Carbon Cycle and Probable Responses to Climatic Warming. Ecol. Appl. 1, 182–195. doi:10.2307/1941811
Groffman, P. M., Driscoll, C. T., Fahey, T. J., Hardy, J. P., Fitzhugh, R. D., and Tierney, G. L. (2001). Effects of Mild winter Freezing on Soil Nitrogen and Carbon Dynamics in a Northern Hardwood forest. Biogeochemistry 56, 191–213. doi:10.1023/a:1013024603959
Groffman, P. M., Hardy, J. P., Driscoll, C. T., and Fahey, T. J. (2006). Snow Depth, Soil Freezing, and Fluxes of Carbon Dioxide, Nitrous Oxide and Methane in a Northern Hardwood forest. Glob. Change Biol. 12 (9), 1748–1760. doi:10.1111/j.1365-2486.2006.01194.x
Hao, R. J., Li, Z. P., and Che, Y. P. (2007). Effect of Freeze-Thaw Alternation on Water-Soluble Organic Carbon Content and Organic Carbon Mineralization in Paddy Soil. Soil Bull. 38, 1052–1057. doi:10.19336/j.cnki.trtb.2007.06.003
Herrmann, A., and Witter, E. (2002). Sources of C and N Contributing to the Flush in Mineralization upon Freeze-Thaw Cycles in Soils. Soil Biol. Biochem. 34, 1495–1505. doi:10.1016/s0038-0717(02)00121-9
Jiaoyue Wang, J., Song, C., Hou, A., Miao, Y., Yang, G., and Zhang, J. (2014). Effects of Freezing-Thawing Cycle on Peatland Active Organic Carbon Fractions and Enzyme Activities in the Da Xing'anling Mountains, Northeast China. Environ. Earth Sci. 72, 1853–1860. doi:10.1007/s12665-014-3094-z
Jin, H., Wu, J., Cheng, G., Nakano, T., and Sun, G. (1999). Methane Emissions from Wetlands on the Qinghai-Tibet Plateau. Chin.Sci.Bull. 44 (24), 2282–2286. doi:10.1007/bf02885940
Kurganova, I., Teepe, R., and Loftfield, N. (2007). Influence of Freeze-Thaw Events on Carbon Dioxide Emission from Soils at Different Moisture and Land Use. Carbon Balance Manage 2, 2–11. doi:10.1186/1750-0680-2-2
Kuttim, M., Hofsommer, M. L., Robroek, B. J. M., Signarbieux, C., Jassey, V. E. J., Laine, A. M., et al. (2017). Freeze-thaw Cycles Simultaneously Decrease Peatland Photosynthetic Carbon Uptake and Ecosystem Respiration. Boreal Environ. Res. 22, 267–276.
Li, F., Zang, S. Y., Liu, Y. N., Wu, X. W., and Ni, H. W. (2019). Effects of Freezing and Thawing on Soil Active Organic Carbon and Enzyme Activity in the Sanjiang Plain Wetlands. Chin. J. Ecol. 39 (21), 7938–7949. (in Chinese). doi:10.5846/stxb201809121962
Liu, L. F., Chen, H., Peng, C. H., Zhu, Q. A., and Li, B. X. (2016). CH4 Release from Peat at Different Depths on the Zoîgé Plateau under Increasing Temperature. Chin. J. Appl. Environ. Biol. 22 (1), 1–7. doi:10.3724/SP.J.1145.2015.05022
Liu, Z., Wang, M., and Ma, X. (2012). Estimation of Storage and Density of Organic Carbon in Peatlands of China. Chin. Geogr. Sci. 22, 637–646. doi:10.1007/s11769-012-0572-7
Liu, X. W. (2021). Research on Carbon Dynamics and Model Simulation of Typical Peatland in Qinghai-Tibet Plateau. dissertation. Beijing, China: The University of Chinese Academy of Sciences. (in Chinese).
Mastepanov, M., Sigsgaard, C., Dlugokencky, E. J., Houweling, S., Ström, L., Tamstorf, M. P., et al. (2008). Large Tundra Methane Burst during Onset of Freezing. Nature 456, 628–630. doi:10.1038/nature07464
McLain, J. E. T., Kepler, T. B., and Ahmann, D. M. (2002). Belowground Factors Mediating Changes in Methane Consumption in a forest Soil under Elevated CO2. Glob. Biogeochem. Cycles 16 (3), 2301–2314. doi:10.1029/2001gb001439
Mei Wang, M., Chen, H., Wu, N., Peng, C., Zhu, Q., Zhu, D., et al. (2014). Carbon Dynamics of Peatlands in China during the Holocene. Quat. Sci. Rev. 99, 34–41. doi:10.1016/j.quascirev.2014.06.004
Natali, S. M., Schuur, E. A. G., Trucco, C., Hicks Pries, C. E., Crummer, K. G., and Baron Lopez, A. F. (2011). Effects of Experimental Warming of Air, Soil and Permafrost on Carbon Balance in Alaskan Tundra. Glob. Change Biol. 17 (3), 1394–1407. doi:10.1111/j.1365-2486.2010.02303.x
Pirk, N., Santos, T., Gustafson, C., Johansson, A. J., Tufvesson, F., Parmentier, F. J. W., et al. (2015). Methane Emission Bursts from Permafrost Environments during Autumn Freeze-In: New Insights from Ground-Penetrating Radar. Geophys. Res. Lett. 42 (16), 6732–6738. doi:10.1002/2015gl065034
Priemé, A., and Christensen, S. (2001). Natural Perturbations, Drying-Wetting and Freezing-Thawing Cycles, and the Emission of Nitrous Oxide, Carbon Dioxide and Methane from Farmed Organic Soils. Soil Biol. Biochem. 33, 2083–2091. doi:10.1016/s0038-0717(01)00140-7
Raz-Yaseef, N., Torn, M. S., Wu, Y., Billesbach, D. P., Liljedahl, A. K., Kneafsey, T. J., et al. (2017). Large CO2 and CH4 Emissions from Polygonal Tundra during spring Thaw in Northern Alaska. Geophys. Res. Lett. 44, 504–513. doi:10.1002/2016gl071220
Rey, A., Petsikos, C., Jarvis, P. G., and Grace, J. (2005). Effect of Temperature and Moisture on Rates of Carbon Mineralization in a Mediterranean oak forest Soil under Controlled and Field Conditions. Eur. J. Soil Sci. 56, 589–599. doi:10.1111/j.1365-2389.2004.00699.x
Roulet, N. T. (2000). Peatlands, Carbon Storage, Greenhouse Gases, and the Kyoto Protocol: Prospects and Significance for Canada. Wetlands 20, 605–615. doi:10.1672/0277-5212(2000)020[0605:pcsgga]2.0.co;2
Schuur, E. A. G., McGuire, A. D., Schädel, C., Grosse, G., Harden, J. W., Hayes, D. J., et al. (2015). Climate Change and the Permafrost Carbon Feedback. Nature 520, 171–179. doi:10.1038/nature14338
Shindell, D. T., Faluvegi, G., Koch, D. M., Schmidt, G. A., Unger, N., and Bauer, S. E. (2009). Improved Attribution of Climate Forcing to Emissions. Science 326 (5953), 716–718. doi:10.1126/science.1174760
Song, C., Wang, Y., Wang, Y., and Zhao, Z. (2006). Emission of CO2, CH4 and N2O from Freshwater Marsh during Freeze-Thaw Period in Northeast of China. Atmos. Environ. 40 (35), 6879–6885. doi:10.1016/j.atmosenv.2005.08.028
Song, C. C., Xu, X. F., Sun, X. X., Tian, H. Q., Sun, L., MiaoY, Q., et al. (2012). Large Methane Emission upon spring Thaw from Natural Wetlands in the Northern Permafrost Region. Environ. Res. Lett. 7 (3), 1–8. doi:10.1088/1748-9326/7/3/034009
Sorensen, P. O., Finzi, A. C., Giasson, M.-A., Reinmann, A. B., Sanders-DeMott, R., and Templer, P. H. (2018). Winter Soil Freeze-Thaw Cycles lead to Reductions in Soil Microbial Biomass and Activity Not Compensated for by Soil Warming. Soil Biol. Biochem. 116, 39–47. doi:10.1016/j.soilbio.2017.09.026
Tan, B., Wu, F. Z., Yang, W. Q., Wang, A., and Yang, Y. L. (2012). Soil Biochemical Dynamics at Three Elevations during the Soil Thawing Period, Eastern Tibetan Plateau: Nutrient Availabilities, Microbial Properties and Enzyme Activities. Afr. J. Microbiol. Res. 6, 4712–4721. doi:10.5897/ajmr11.1537
Tokida, T., Mizoguchi, M., Miyazaki, T., Kagemoto, A., Nagata, O., and Hatano, R. (2007). Episodic Release of Methane Bubbles from Peatland during spring Thaw. Chemosphere 70, 165–171. doi:10.1016/j.chemosphere.2007.06.042
Tveit, A., Schwacke, R., Svenning, M. M., and Urich, T. (2012). Organic Carbon Transformations in High-Arctic Peat Soils: Key Functions and Microorganisms. ISME J. 7 (2), 299–311. doi:10.1038/ismej.2012.99
Wang, X. W. (2010). Analysis of Freezing and Thawing Laws and Simulation of Hydrological Characteristics of Seasonal Frozen Soil in Northern China. Heilongjiang, China: Northeastern Agricultural University. (in Chinese).
Wang, J. Y. (2014). Effects of Freezing and Thawing on Soil Organic Carbon of Peatland in Permafrost Region of Da Xing' Anling Mountains. dissertation. Beijing, China: The University of Chinese Academy of Sciences. (in Chinese).
Wang, X. L. (2015). Research on Methane Emission Characteristics of Zoîgé Alpine Peat Wetland. Yangling, Shanxi, China: Northwest A & F University. (in Chinese).
Wang, Y., Liu, J. S., Wang, G. P., and Zhou, W. M. (2007). Study on the Relationship between Freeze-Thaw Effects and Soil Physical and Chemical Effects. Geographical Inf. Sci. 2, 91–96. (in Chinese). doi:10.16258/j.cnki.1674-5906.2013.07.012
Wang, J. Y., Song, C. C., Wang, X. W., and Wang, L. L. (2011). Research Progress in the Studies of Effects of Freezing and Thawing on Soil Organic Carbon Pool and Microorganisms. J. Glaciology Geocryology 33, 442–452. (in Chinese).
Wang, Y., Liu, J. S., and Wang, Q. Y. (2013). The Effects of Freezing and Thawing on Soil Aggregates and Organic Carbon Components. J. Ecol. Environ. 22, 1269–1274. (in Chinese).
Wu, H., Xingkai, X., Cheng, W., and Lin, H. (2019). Dissolved Organic Matter and Inorganic N Jointly Regulate Greenhouse Gases Fluxes from forest Soils with Different Moistures during a Freeze-Thaw Period. Soil Sci. Plant Nutr. 66 (1), 163–176. doi:10.1080/00380768.2019.1667212
Xiang, S., Guo, R., Wu, N., and Sun, S. (2009). Current Status and Future Prospects of Zoige Marsh in Eastern Qinghai-Tibet Plateau. Ecol. Eng. 35, 553–562. doi:10.1016/j.ecoleng.2008.02.016
Xie, S.-b., Jian-jun, Q., Yuan-ming, L., Zhi-wei, Z., and Xiang-tian, X. (2015). Effects of Freeze-Thaw Cycles on Soil Mechanical and Physical Properties in the Qinghai-Tibet Plateau. J. Mt. Sci. 12, 999–1009. doi:10.1007/s11629-014-3384-7
Xu, J., Morris, P. J., Liu, J., and Holden, J. (2018). PEATMAP: Refining Estimates of Global Peatland Distribution Based on a Meta-Analysis. Catena 160, 134–140. doi:10.1016/j.catena.2017.09.010
Yang, M. X., Ya, T. D., Hirose, N., and Hideyuki, F. (2006a). The Daily Freeze-Thaw Cycle of the Surface Soil on the Qinghai-Tibet Plateau. Chin. Sci. Bull. 51, 1974–1976.
Yang, W., Song, C., and Zhang, J. (2006b). Dynamics of Methane Emissions from a Freshwater Marsh of Northeast China. Sci. total Environ. 371, 286–292. doi:10.1016/j.scitotenv.2006.08.034
Yu, R., Kampschreur, M. J., Loosdrecht, M. C. M. v., and Chandran, K. (2010). Mechanisms and Specific Directionality of Autotrophic Nitrous Oxide and Nitric Oxide Generation during Transient Anoxia. Environ. Sci. Technol. 44, 1313–1319. doi:10.1021/es902794a
Zhang, J. B., Song, C. C., and Yang, W. Y. (2006). Land Use Effects on the Distribution of Labile Organic Carbon Fractions through Soil Profiles. Soil Sci. Soc. America J. 70, 660–667. doi:10.2136/sssaj2005.0007
Zhang, C. F., Sheng, L. X., Gong, C., He, C. G., and Zhang, J. (2018). Effects of Freezing and Thawing on Soil Carbon Emissions and Soil Microbes in Wetlands in Northeast China. Chin. J. Ecol. 37, 304–311. (in Chinese). doi:10.13292/j.1000-4890.201802.035
Zhang, T., and Armstrong, R. L. (2001). Soil Freeze/thaw Cycles over Snow-free Land Detected by Passive Microwave Remote Sensing. Geophys. Res. Lett. 28, 763–766. doi:10.1029/2000gl011952
Zheng, Y. K., Liu, K., Xiong, Z. J., Miao, C. P., Chen, Y. W., Xu, L. H., et al. (2015). Effect of Large-Scale Planting Water Hyacinth on Cultivable Bacterial Community Structure in the Eutrophic lake. Microbiol. China 42 (1), 42–53. (in Chinese). doi:10.13344/j.microbiol.china.140385
Zhou, Y. W., Guo, D. X., and Cheng, G. D. (2000). Geocryology in China. Beijing: Science Press. (in Chinese).
Keywords: methane emissions, high-altitude peatlands, freeze–thaw cycles, dissolved organic carbon, enzyme activity
Citation: Yang Z, Zhu D, Liu L, Liu X and Chen H (2022) The Effects of Freeze–Thaw Cycles on Methane Emissions From Peat Soils of a High-Altitude Peatland. Front. Earth Sci. 10:850220. doi: 10.3389/feart.2022.850220
Received: 07 January 2022; Accepted: 07 March 2022;
Published: 31 March 2022.
Edited by:
Huijun Jin, Northeast Forestry University, ChinaReviewed by:
Xinhou Zhang, Nanjing Normal University, ChinaZiming Yang, Oakland University, United States
Copyright © 2022 Yang, Zhu, Liu, Liu and Chen. This is an open-access article distributed under the terms of the Creative Commons Attribution License (CC BY). The use, distribution or reproduction in other forums is permitted, provided the original author(s) and the copyright owner(s) are credited and that the original publication in this journal is cited, in accordance with accepted academic practice. No use, distribution or reproduction is permitted which does not comply with these terms.
*Correspondence: Dan Zhu, zhudan_m@cib.ac.cn