- 1School of Traffic and Transportation Engineering, Changsha University of Science and Technology, Changsha, China
- 2National Engineering Laboratory of Highway Maintenance Technology, Changsha University of Science and Technology, Changsha, China
- 3Zhaotong Zhaoyang Ring Highway Investment and Development Corporation Ltd., Zhaotong, China
Rainfall is the main factor leading to the landslide of expansive soil slope. To deeply understand the instability mechanism of expansive soil slope under rainfall conditions, it is necessary to clarify the strength attenuation mechanism of expansive soil caused by water absorption and swelling. Therefore, the improved constant volume direct shear test was used in this study to compare with the conventional test, focusing on the variation of effective shear strength parameters with dry density (volume). The micromechanism of expansive soil strength attenuation is further studied, and the influence of expansive soil on slope stability is analyzed by numerical calculation. The results show that the improved direct shear apparatus and method can keep the volume of expansive soil specimens unchanged before and after shear and obtain the real effective shear strength parameter of soil. Under the same conditions, the shear strength parameter of the improved direct shear test is lower than that of the conventional direct shear test. The rapid thickening of the lamellar structure of montmorillonite in expansive soil will lead to the fragmentation and dispersion of clay particles, which is one of the fundamental reasons for the strength difference between expansive soil and ordinary clay. In slope stability analysis, the effect of swelling on the shear strength of slope cannot be ignored and should be considered in engineering design.
Introduction
Under the influence of hygrothermal environment and geological conditions, landslide disasters occur frequently in expansive soil areas (Hou et al., 2013; Dai et al., 2021; Liang et al., 2021). Rainfall infiltration is an important inducement factor of slope collapse (Qi and Vanapalli, 2018; Qi et al., 2019). Some slopes will slide in the rainy season when the slope ratio is 1:3 or even 1:5 (Xiao et al., 2018; Zhai and Cai, 2018; Abeykoon and Trofimovs, 2021). Therefore, only by deeply understanding the state change of expansive soil caused by humidification and clarifying the strength attenuation mechanism of expansive soil, target economic and reasonable engineering measures can be taken.
Studies have shown that the shear strength variation of expansive soil is mainly affected by the stress state, matric suction, dry–wet cycle, crack, and moisture content (Li et al., 2013; Al Haj and Standing, 2015; Zhang et al., 2016; Dong et al., 2018a; Huang et al., 2018). The strength envelope of expansive soil has non-linear characteristics, and there is a certain gap between the parameters obtained by linear fitting and the real shear strength parameter (Lade, 2010; He et al., 2017). In the low-stress range (0–50 kPa), the cohesion is only a few kPa, but the internal friction angle is as high as 30°–40°. In the high-stress range (greater than 50 kPa), the cohesion obtained by fitting is more than 20 kPa, and the internal friction angle is about 20°, in the stress range of conventional strength tests, the cohesion and internal friction angles obtained by fitting are not low (Xiao et al., 2017). Many slope failures still occur when strength parameters are obtained by the conventional direct shear test and can be kept stable by calculation (Zhang et al., 2020a). Therefore, the actual shear strength parameter value of expansive soil is lower than that measured by conventional tests. The variation of matric suction can express the strength variation of general clay from unsaturated to saturated. However, for expansive soil, the saturated strength of specimens under different initial suction states varies greatly, so the unsaturated strength model cannot be effectively used for calculation and analysis. The dry–wet cycles and cracks mainly affect the soil structure (Burton et al., 2015; Julina and Thyagaraj, 2018; Huang et al., 2021), but the number of dry–wet cycles and the development of cracks are highly subjective, and they are difficult to apply quantitatively in slope stability analysis. The change of moisture content leads to the change of matric suction of soil, and it can affect the state of water retention in soil (Kayadelen et al., 2007; Çokça and Tilgen, 2010). The essential difference between expansive soil and general clay soil is that it is rich in swelling minerals such as montmorillonite and exhibits remarkable expansibility after humidification (Zhang et al., 2020b). For non-expansive soil, the change of soil moisture content only affects the volume of pore gas and pore water, while the volume of the soil skeleton remains constant. However, with the increase of soil skeleton volume, the dry density of expansive soil decreases, and the influence of humidifying swelling on the effective stress shear strength parameter cannot be ignored (Mašín and Khalili, 2016).
The expansive clay mineral particles expand with the increase of moisture content and compress with the increase of overburden, thus changing the microstructure and average particle size of soil (Katti and Shanmugasundaram, 2001). To reflect the effect of pore ratio on the shear strength of clay and its parameter, scholars proposed true strength theory and true strength parameter based on experiments. It was suggested that saturated clay should be obtained with different effective stresses but the same pore ratio by loading and unloading and then sheared to obtain the true strength parameter (Hvorslev, 1961). At present, the shear strength parameter of expansive soil is obtained by a triaxial and a direct shear apparatus (Ye et al., 2010; Dong et al., 2018b), but there are no specific measures and methods to ensure that the specimen volume (dry density) remains unchanged before and after shear. The reason is that, in the conventional test, the specimen will be saturated with water after applying different confining pressure or overburden loads, and the specimen will have different degrees of swelling or compression, which leads to the change of the dry density of the specimen before shear. This makes it difficult to obtain the real shear strength parameter of expansive soil under specific dry density conditions at present and also leads to the landslide failure of many expansive soil slopes that can be kept stable by design calculation.
This study aims to investigate the effect of swelling deformation on the effective shear strength parameter of expansive soil and reveal the attenuation mechanism of expansive soil strength caused by water absorption and swelling. Based on the true strength theory, direct shear tests of expansive soil at constant dry density under different initial saturation conditions were carried out by using the improved direct shear test apparatus, and the effective cohesion and effective internal friction angle of expansive soil at this dry density state were measured. Then, several groups of improved direct shear tests under different dry densities were carried out, and the results were compared with those of conventional direct shear tests, to obtain the variation rule of effective shear strength parameter with the decrease of dry density. A scanning electron microscope (SEM) and particle analyzer were used to obtain the changes of the microstructure of the specimens before and after shear and to reveal the attenuation mechanism of the saturated strength of expansive soil. Through numerical analysis, the influence of swelling on slope stability is studied, and the results can provide a reference for the instability mechanism analysis and support structure design of expansive soil slopes.
Selected Soil Sample
The soil sample used in the laboratory study is a brown–yellow expansive soil taken from the cutting slope of Anxiao Road, Zhijiang, Hubei Province, China. It was obtained from three different test pits at a depth of 0.5, 1.5, and 2.5 m below the ground surface and transferred to the laboratory for full geotechnical characterization. The natural moisture content of the samples is 20.8%. The block samples were protected from loss of moisture using a plastic membrane and sealed metal box. The sampling process is shown in Figure 1.
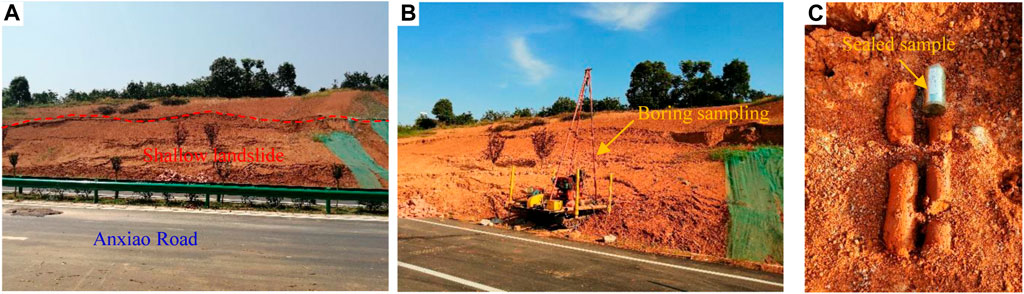
FIGURE 1. Scene investigation and sampling. (A) Anxiao Road cutting landslide. (B) Field sampling. (C) Sealed sample storage.
Laboratory tests shall be carried out immediately after the soil samples are brought back. The particle-size distribution shows that the soil has 52.1% silt and 47.9% clay, and the liquid limit and plastic limit are 54.3 and 29.9%, respectively. Hence, this soil can be described as clay with intermediate plasticity. The predominant clay minerals in the soil, as determined by X-ray diffractometry, are smectite (18.6%). The values of one-dimensional free swelling are approximately 11% for the natural and compacted specimens, respectively. Table 1 shows the index properties of the soil.
Improved Direct Shear Apparatus and Method for Expansive Soils
Improved Direct Shear Apparatus
To keep the volume of expansive soil unchanged before and after shearing, an improved direct shear apparatus is used to test the shear strength of expansive soil. Compared with the conventional direct shear apparatus, the major improvements include increasing the top diameter of the loading plate so that it is at least 5 mm larger than the inner diameter of the shear box, adding a ring gasket with a limit function between the loading plate and the shear box, and adding grooves at the bottom of the loading plate and placing pressure sensors. The pressure sensor is used to measure the vertical swelling pressure of expansive soil specimens in the process of saturation. After the above improvement, the ring gasket can control the loading plate to only slightly contact the porous stone at the top of the specimen before the specimen is soaked, and the load applied is all borne before the specimen swells, to ensure that the volume of the expansive soil specimen is not compressed before the specimen is soaked. After the specimen is immersed in water, the loading plate with a pressure sensor can read the vertical swelling pressure of the specimen from the initial moisture content to the process of saturation. Before shearing, as long as the initial load is slightly greater than the maximum vertical swelling pressure under the initial moisture content of the specimen, the specimen volume can be guaranteed to remain unchanged during shearing. The section of the improved direct shear apparatus is shown in Figure 2.
Improved Direct Shear Test Method
Swelling of expansive soil is a process of dry density reduction. If expansive soil swells before and after shearing, the dry density of the actual shear specimen will be less than the set dry density, which cannot effectively represent the strength parameter of a specific dry density. Considering that compared with the triaxial apparatus, the direct shear apparatus is easier to realize the saturated condition and control the dry density of the sample before shear, and the conventional direct shear apparatus is used for the test. However, by changing the loading mode of the conventional direct shear test and using the suction unloading mode, the dry density of specimens before shear remains unchanged, and the specimens are guaranteed to be subjected to different effective stresses. The swelling test with load was carried out before the direct shear test to obtain the relationship between dry density and swelling deformation of the compacted specimen after soaking in water in the initial wet dense state. The lower limit of dry density control for subsequent improved direct shear test specimens was determined by referring to the dry density after no-charge swelling. The steps of the improved direct shear test are as follows:
1) The specimens were prepared using the static compaction method. At a predetermined dry density and saturation (45–90%), four soil specimens with the same dry density and different initial moisture content were prepared at a time.
2) The specimens were put into the direct shear box and soaked (suction unloading), using the balance compression method to control the overburden and keep the height (dry density) of the specimen in the soaking process unchanged.
3) When the overburden load did not change, the slow shear test was carried out to obtain the critical strength and peak strength by a shear displacement of 7 mm under the overburden load. The moisture content of the specimen after shearing was determined, and the saturation was calculated.
4) Repeat steps 2) and 3) for other specimens to obtain the peak strength and ultimate strength corresponding to different overburden loads and then obtain the peak and ultimate effective shear strength parameters by fitting.
5) Direct shear tests with different initial dry densities were carried out according to steps 1) to 4), and then the peak effective shear strength parameter and ultimate effective shear strength parameter were obtained with the decrease of dry density (regarded as the swelling process).
Testing Program
The test scheme for this laboratory study is presented in Table 2. Both improved direct shear tests and conventional direct shear tests were carried out on expansive soil slope in Zhijiang City, Hubei Province.
The Improved Direct Shear Test Program
Five groups of specimens with different dry densities were selected within the range of the initial dry density of 1.6 g/cm3 and the minimum dry density of 1.44 g/cm3 after saturated swelling. The moisture content of the specimen was determined according to the saturation of expansive soil of 45 ∼ 90%, as shown in Table 2. One-dimensional unloading swelling tests were carried out on the specimens with different initial dry densities under different initial saturation conditions to determine the vertical swelling pressure generated when the test reached saturation. The variation of swelling deformation and dry density with vertical pressure is shown in Figure 3. Then, the swelling pressure value was taken as the vertical load value in the direct shear process to conduct the direct shear test. The effective internal friction angle and cohesion of expansive soil at constant dry density were obtained.
There is a one-to-one relationship between the swelling rate and dry density, so the decrease of dry density can indicate swelling of expansive soil. The dry density of expansive soil samples decreased from 1.60 g/cm3 to 1.43 g/cm3, and the swelling rate increased by 11%. Considering that the actual swelling of expansive soil slope includes not only vertical swelling but also lateral swelling, the lower limit of dry density is set as 1.40 g/cm3 in the test.
Conventional Direct Shear Test Program
Combined with the direct shear test method under low-stress conditions, the load classification was added based on the conventional direct shear test. Conventional tests in this paper adopted eight different vertical loads of 12.5, 25, 37.5, 50, 100, 150, 200, and 250 kPa. The conventional direct shear tests with different densities of 1.4 g/cm3, 1.5 g/cm3, and 1.6 g/cm3 were carried out under the same moisture content.
Experimental Results and Analysis
Improved Direct Shear Test Results
As shown in Table 2, the improved direct shear test was divided into five groups (MD1–MD5) according to different dry densities, and each group was equipped with four specimens with different moisture content according to 45, 60, 75, and 90% saturation. For the improved direct shear test, the water content of saturated samples under different dry densities is different, and the water content given in Table 2 is saturated water content. For the conventional direct shear test, the water content refers to the initial water content of the specimens. However, the overburden load is uniformly set according to the maximum swelling pressure obtained by the one-dimensional loaded swelling test, which is set here as 250 kPa. The values of state parameters of the improved direct shear test are shown in Table 3.
As shown in Table 3, the vertical stress, peak strength, and critical strength of specimens with different dry densities increase with the decrease of initial saturation. This is because the specimen with lower saturation has lower corresponding moisture content. In the process of saturation, the soil will absorb more water, thus releasing greater swelling force. Under the vertical load on the top of the specimen, the vertical deformation is constrained, and the swelling force will act on the soil skeleton in the form of vertical stress. A larger swelling force corresponds to a larger effective stress, thus obtaining a higher strength value. The small difference of moisture content after saturation indicates that the pore ratio of specimens with different initial saturations is the same after saturation, which avoids the influence of pore ratio change on soil structure and strength.
Conventional Direct Shear Test Results
Conventional direct shear tests were carried out on three specimens with different dry densities. To compare with the improved direct shear specimen under constant volume conditions, the maximum vertical load was set at 250 kPa. As the expansive soil slope usually has the characteristics of the shallow slide, the shear test under low-stress conditions is added according to the practice of Xiao et al. (2017), and the test results are shown in Table 4.
As shown in Table 4, after saturation, the dry density of the same specimen changes with different vertical pressure. The specimen with a dry density of 1.4 g/cm3 swells greatly under low-stress conditions, and the maximum vertical swelling rate is 3.21%. The moisture content (saturation) of the specimen decreases with the increase of the dry density of the specimen, indicating that the compaction expansive soil under the loading condition is not the only saturated state but also affected by the vertical pressure. Under the same dry density after consolidation, the dry density of the specimen after swelling increases with the increase of vertical effective stress, which is caused by the swelling of expansive soil.
Effects of Dry Density on Shear Strength for Expansive Soil
As shown in Figure 4, the variation of shear strength with normal pressure of three kinds of dry density specimens in the conventional direct shear test shows that the peak strength and critical strength of Zhijiang expansive soil increase with the increase of dry density. Under low-stress conditions, the measured values are mostly below the fitting line. The main reason is that the expansive soil has swelling properties. The smaller the overburden pressure is, the larger the volume swelling of the specimen with the same initial dry density is.
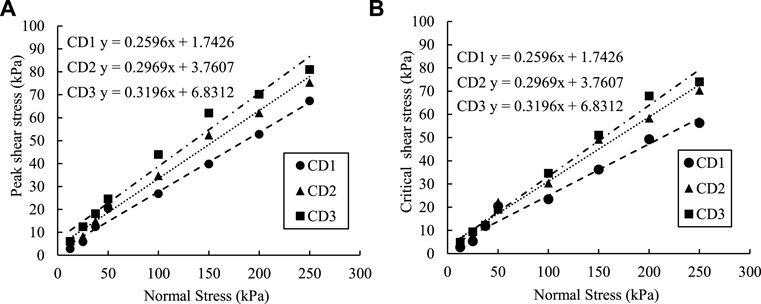
FIGURE 4. Conventional direct shear test results of expansive soil. (A) Variation of peak shear strength with normal stress. (B) Variation of critical shear strength with normal stress.
Figure 4 shows a comparison of shear strength and its parameter between the different dry density specimens. Figure 4 is constructed by plotting the peak values of shear strength from Table 4 against the corresponding dry density. As discussed previously, the peak value increases with an increase in the applied normal stress for different dry density compacted specimens. For a given density, the peak shear strength of the higher dry density specimens is always greater than that of the lower dry density specimens.
The test values and fitting lines of the improved direct shear test of five expansive soil samples with different dry densities are shown in Figure 5. By comparing Figures 5A,B, it is seen that the values of peak strength and ultimate strength of expansive soil with different dry densities have a little difference under test-stress conditions. This is because the value of saturated shear strength can be determined by two shear strength parameters, cohesion and internal friction angle, regardless of peak strength or ultimate strength. Under the condition of low stress, the true cohesion between saturated soil particles without cementation is very small, and the contribution of vertical stress to strength is also low, resulting in a little difference in strength values. The internal friction angle of expansive soil with high dry density is larger, which is more significantly affected by vertical stress. With the increase of vertical stress, the strength difference increases. The comparison between Figures 4, 5 shows that, under the same stress state, the strength difference obtained by the conventional direct shear test is larger than that obtained by the improved direct shear test. This is because the improved direct shear test keeps the volume constant during the whole direct shear process, and the dry density does not change during the shear process. However, the strength of conventional direct shear specimens is lower under low stress and higher under high stress due to volume change.
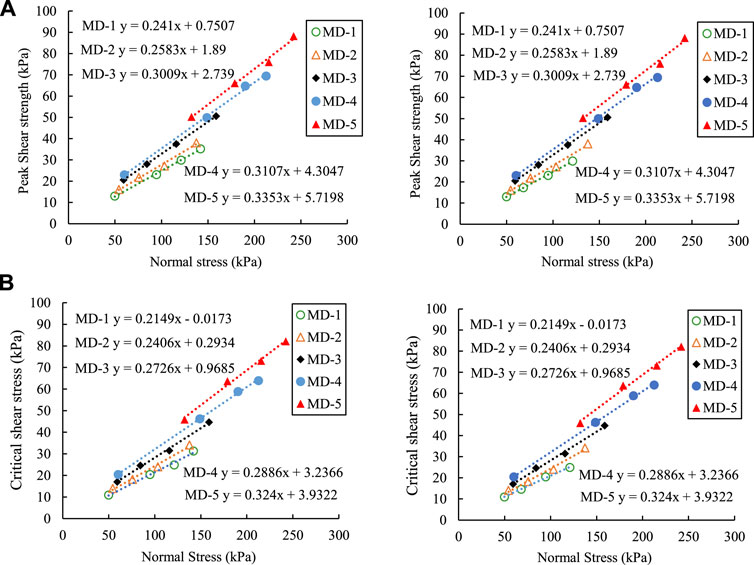
FIGURE 5. Improved direct shear test results of expansive soil. (A) Variation of peak shear strength with normal stress. (B) Variation of critical shear strength with normal stress.
There was a good linear correlation between the peak shear strength and the ultimate shear strength obtained by the improved direct shear test method and the vertical loading. This method not only ensures the consistency of the dry density of specimens under different vertical loadings but also ensures the consistency of the structure of specimens before shearing as far as possible. Therefore, the obtained shear strength parameters can represent the true cohesive and frictional properties of expansive soil under the condition of dry density.
As shown in Figure 6, when the initial dry density is 1.6 g/cm3, the effective cohesive of expansive soil is only about 4 kPa, and the effective friction angle is 18.1°. The friction strength mainly comes from the interaction between particles on the contact surface. The peak shear strength and residual shear strength decrease with the decrease of dry density. The effective internal friction angle of saturated compacted expansive soil is only 12.1° and the cohesion is close to 0 when the dry density is 1.4 g/cm3. Under the condition of low stress, the shear strength parameter of expansive soil measured by the improved direct shear test is 8 ∼ 36% higher than that measured by the conventional direct shear test. Some scholars classify this situation as the weakening of bond strength caused by the increase of expansive soil volume and the thickening of the granular water film. The clay particles dispersed gradually, and the adsorption weakened. The test results show that the true shear strength of expansive soil is very low.
The influence of different dry densities on expansion deformation of expansive soil was referred (Zhang et al., 2020b). To establish the relationship between dry density and saturated shear strength parameters, the following equations are used to fit them:
where c′ is the effective cohesion, ρd is the dry density, φ′ is the effective internal friction angle, e is the void ratio, and a1, b1, k1, a2, b2, k2 are the fitting parameters.
The fitting results are as follows: a1 = 4.86, b1 = 5.34, k1 = 18.37, a2 = 18.86, b2 = 8.73, k2 = 13.37, and the correlation coefficients are, respectively, 0.974 and 0.990.
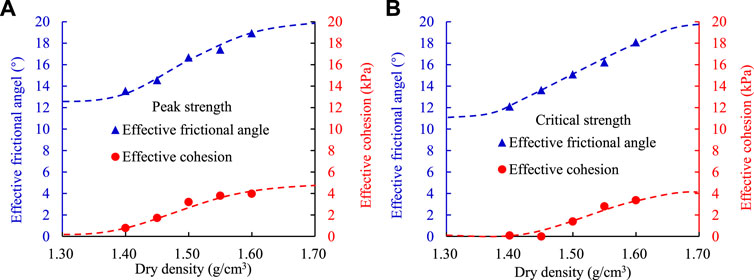
FIGURE 6. Variation of the effective shear strength parameter with dry density. (A) Variation of the peak shear strength parameter with dry density. (B) Variation of the critical shear strength parameter with dry density.
Influence of Microstructure Change on Shear Strength Parameter
For expansive soils, swelling not only changes the macroscopic volume but also changes the microstructure (Lin and Cerato, 2014). To understand the actual effect of humidification and swelling on the microstructure of the specimen, the saturated specimen before and after swelling was analyzed by an SEM. Three expansive soil specimens with the same initial saturation were prepared at the dry densities of 1.4 g/cm3, 1.5 g/cm3, and 1.6 g/cm3, respectively, and saturated by the improved direct shear method. As the SEM scanning process needs to be carried out in a dry environment, a vacuum freeze-drying method is needed to dry the specimen. After saturation, specimens with different dry densities were taken out of the ring knife and cut into small soil blocks of 1.0 × 1.0 × 1.0 cm. Then, the specimens were quickly put into liquid nitrogen and frozen for 15 min, so that the liquid in the specimens became amorphous ice without swelling. Finally, at −50°C, the amorphous ice in the soil specimen was sublimated by a vacuum freeze dryer for more than 8 h, to maintain the structure of the soil skeleton and saturated soil particles.
It can be seen from Figure 7 that, with the decrease of dry density, the original particles of expansive soil become more broken and become smaller flaky structures stacked upon each other. At the same time, the fragmented lamellar structure leads to the relative sliding of the lamellar in the humidification process, and the reduction of interlamellar friction makes the lamellar dislocation relatively easy which is also the factor leading to the reduction of the internal friction angle of the soil on the macro level. This is consistent with the relationship between shear strength and dry density found in macroscopic tests.
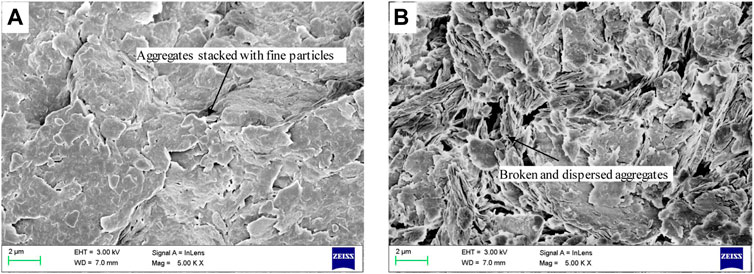
FIGURE 7. Microstructure changes during swelling (5,000 times larger): (A) before swelling, ρd = 1.6 g/cm3; (B) after swelling, ρd = 1.45 g/cm3.
To verify the particle fragmentation of the specimen after swelling, a particle analyzer was used to measure the particle content of the specimen with 1.6 g/cm3 dry density before and after swelling, and the cumulative curve of particle distribution was obtained, as shown in Figure 8. After swelling, the particle size below 0.1 mm increased significantly, and the smaller the particle size, the greater the change of cumulative content. The particles in the range of 0.1∼2 mm are reduced correspondingly, which also proves from the side that the large particles of soil will be broken and dispersed after swelling and the large particles will be replaced by more small particles. However, under constant volume conditions, the swelling is limited, the particle size changes little, and the swelling potential energy is mainly released in the form of swelling force, and the influence of microstructure on soil strength is relatively small.
As shown in Figure 9A, when unsaturated expansive soil is humidified, the crystal layer of montmorillonite mineral grains expands significantly due to water absorption, which results in a change in the volume of the soil skeleton. Figure 9B shows montmorillonite clay particles will expand significantly from the inside after absorbing water, leading to the gradual dispersion of aggregates and local fragmentation. The contact morphology of particles is affected, which results in partial contact between silt particles and silt particles into silt particles and clay particles. The change of contact surface shape will change the friction force between each other, and the effective internal friction angle will decrease. However, the general shear strength theory of saturated–unsaturated soil does not consider the swelling effect of soil particles in the soil, and the contact surface of soil particles does not change in the process of humidification, so the effective internal friction angle and cohesion remain unchanged.
Engineering Application
Numerical simulation is an important means for slope collapse analysis and prediction (Zheng et al., 2019). In order to have a deeper understanding of the long-term stability of expansive soil slope affected by swelling, taking the Zhijiang typical expansive soil cutting slope as the research object, the influence of swelling (decrease of dry density) on slope stability is analyzed. The height of the expansive soil slope is 12 m, and the slope ratio is 1:1.5. Under the long-term atmospheric humid and hot environment, cracks are continuously connected and developed in a large number within 2 m of the slope. The local stagnant water zone is easily formed after rainfall, which makes the soil in the region reach the saturation state. According to the field test data, the average dry density of soil within 1 m depth is 1.56 g/cm3, and the average dry density of soil below 1 m depth is 1.60 g/cm3. Therefore, the expansive soil slope can be roughly divided into three areas, as shown in Figure 10. The average dry density of layer A soil is 1.56 g/cm3 which is in the range of significant influence of the atmospheric dry–wet cycle, and the soil can reach saturation after rainfall. The average dry density of layer B is 1.60 g/cm3, but it is within the range of significant influence of the atmospheric dry–wet cycle. Layer C is not affected by the atmospheric environment, and its average dry density (1.60 g/cm3) generally does not change during rainfall. For Zhijiang expansive soil with an initial dry density of 1.56 g/cm3 and 1.6 g/cm3 and initial moisture content of 17.9%, the loaded swelling test was carried out, respectively, and the maximum saturated swelling was 6.16 and 2.56% under the condition of overburden load of 10 kPa (the overlying soil layer is about 0.5 m) and 30 kPa (the overlying soil layer is about 1.5 m). Then, the dry density of expansive soil could be obtained. The test results are shown in Table 5.
Since the moisture content of shallow soil is close to saturation after rain, it can be assumed that layer A soil of expansive soil slope can be completely saturated under continuous rainfall conditions. According to Table 5, the dry density of soil in this region decreases from 1.56 g/cm3 to 1.47 g/cm3 under saturation, decreasing by 5.77%. The dry density of layer B soil decreased from 1.60 g/cm3 to 1.56 g/cm3, reduced by 2.50%. In layer C, the dry density remains constant due to the little change in humidity. It can be seen from the above that the decrease of dry density caused by swelling will directly affect the effective shear strength parameter of soil. The dry density values after attenuation of soil in layers A and B are substituted into Eqs 1, 2 for calculation, to obtain the real effective cohesion and effective internal friction angle after swelling. Then, the shear strength parameter is used to calculate the slope stability, and the safety factor of expansive soil slope under the condition of strength attenuation is obtained.
Under the influence of atmospheric wetting and drying cycle, soil in the region will swell after rainfall. Calculating the slope stability after the rain, not only should the intensity of the attenuation caused by the loss of matric suction be considered but also the effect of swelling on effective shear strength parameters should be considered. As shown in Figure 11, when calculating the slope safety factor, the other parameters are set in the same way, and only the influence of shallow soil swelling on shear strength is considered. The safety factor of slope considering swelling is much smaller than that calculated by the conventional method. To understand the stability state of an expansive soil slope in a larger density range after rain, the Fs, Fsw parameters of shear strength before and after swelling obtained by different test methods were used for stability analysis under the condition that other working conditions remained unchanged. The calculation results are shown in Figure 12.
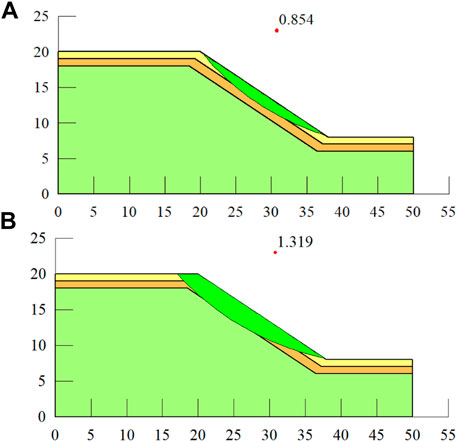
FIGURE 11. Safety factors and the potential sliding surface of the cutting slope. (A) Before swelling. (B) After swelling.
As shown in Figure 12, the safety factor (Fsw) of slope considering the swelling effect is 28–33% lower than that (Fs) without swelling effect. The higher the dry density of expansive soil is, the greater the saturated swelling deformation is, and the slope safety factor decreases more obviously under the influence of swelling. Therefore, in the treatment of expansive soil slope, special attention should be paid to the prevention and drainage measures of high dry density expansive soil slope; at the same time, attention should also be paid to not keep the moisture content of backfill expansive soil too low and to avoid excessive attenuation of strength caused by soil swelling, and the influence of swelling on slope stability cannot be ignored.
Conclusions
1) The linear correlation between peak and critical strength and overlying load obtained by the improved direct shear test method is excellent. This method ensures the consistency of the dry density of the specimens before shearing and the consistency of the structure of the specimens before shearing. Therefore, the obtained shear strength parameters can represent the real cohesive and frictional properties of the expansive soil under the dry density condition.
2) Under the condition of low stress, the shear strength of expansive soil measured by the improved direct shear test is 8 ∼ 36% higher than that measured by the conventional direct shear test. With the decrease of dry density, the peak and ultimate shear strength parameters decrease. Under different densities, the fitting formula related to dry density can be used to characterize the shear strength parameters.
3) The decrease of cohesion is due to the increase of interparticle spacing and the decrease of intermolecular forces. The decrease of internal friction angle is because the large particles of soil are broken into fine particles due to swelling. The contact between large particles and large particles is replaced by the contact between large particles and small particles, and the friction decreases gradually.
4) When analyzing the stability of expansive soil slope under rainfall conditions, it is suggested to determine the dry density state of the shallow soil after the swelling of the slope and then calculate the effective shear strength parameter under the dry density state. Under the condition of the maximum dry density of 1.40 g/cm3 ∼ 1.60 g/cm3, the safety factor calculated by using the improved direct shear test data is 28–33% lower than that obtained by the conventional direct shear test. The influence of dry density change caused by swelling on the shear strength of expansive soil cannot be ignored.
Data Availability Statement
The original contributions presented in the study are included in the article/supplementary material, and further inquiries can be directed to the corresponding author.
Author Contributions
All authors listed have made a substantial, direct, and intellectual contribution to the work and approved it for publication.
Funding
This work was supported by the National Natural Science Foundation of China (Grant No. 51978085) and the Highway Industry Standard Compilation Project of Ministry of Transportation, China (Grant No. JTG-201507).
Conflict of Interest
Author BY and XM were employed by Zhaotong Zhaoyang Ring Highway Investment and Development Corporation Ltd.
The remaining authors declare that the research was conducted in the absence of any commercial or financial relationships that could be construed as a potential conflict of interest.
Publisher’s Note
All claims expressed in this article are solely those of the authors and do not necessarily represent those of their affiliated organizations, or those of the publisher, the editors, and the reviewers. Any product that may be evaluated in this article, or claim that may be made by its manufacturer, is not guaranteed or endorsed by the publisher.
References
Abeykoon, T., and Trofimovs, J. (2021). Shear Strength of Unsaturated Expansive Soils from Queensland Natural Slopes. Geomate 20 (20), 53–60. doi:10.21660/2021.82.Gx353
Al Haj, K. M. A., and Standing, J. R. (2015). Mechanical Properties of Two Expansive clay Soils from Sudan. Géotechnique 65 (4), 258–273. doi:10.1680/geot.14.p.139
Burton, G. J., Pineda, J. A., Sheng, D., and Airey, D. (2015). Microstructural Changes of an Undisturbed, Reconstituted and Compacted High Plasticity clay Subjected to Wetting and Drying. Eng. Geology. 193, 363–373. doi:10.1016/j.enggeo.2015.05.010
Çokça, E., and Tilgen, H. P. (2010). Shear Strength-Suction Relationship of Compacted Ankara clay. Appl. Clay Sci. 49 (4), 400–404. doi:10.1016/j.clay.2009.08.028
Dai, Z., Zhang, C., Wang, L., Fu, Y., and Zhang, Y. (2021). Interpreting the Influence of Rainfall and Reservoir Water Level on a Large-Scale Expansive Soil Landslide in the Danjiangkou Reservoir Region, China. Eng. Geology. 288, 106110. doi:10.1016/j.enggeo.2021.106110
Dong, J.-g., Lv, H.-b., and Wu, W. (2018a). Development and Application of an Instrument for Simulating Wetting-Drying Cycles of Expansive Soils under Loads. J. Mt. Sci. 15 (11), 2552–2560. doi:10.1007/s11629-018-4934-1
Dong, J.-g., Xu, G.-y., Lv, H.-b., and Yang, J.-y. (2018b). Prediction of Expansive Soil Strength Based on Micro-scale Properties. Geotech Geol. Eng. 37, 869–882. doi:10.1007/s10706-018-0657-x
He, P., Li, S.-c., Xiao, J., Zhang, Q.-q., Xu, F., and Zhang, J. (2017). Shallow Sliding Failure Prediction Model of Expansive Soil Slope Based on Gaussian Process Theory and its Engineering Application. KSCE J. Civ Eng. 22 (5), 1709–1719. doi:10.1007/s12205-017-1934-6
Hou, T.-s., Xu, G.-l., Shen, Y.-j., Wu, Z.-z., Zhang, N.-n., and Wang, R. (2013). Formation Mechanism and Stability Analysis of the Houba Expansive Soil Landslide. Eng. Geology. 161, 34–43. doi:10.1016/j.enggeo.2013.04.010
Huang, Z., Wei, B., Zhang, L., Chen, W., and Peng, Z. (2018). Surface Crack Development Rules and Shear Strength of Compacted Expansive Soil Due to Dry-Wet Cycles. Geotech Geol. Eng. 37, 2647–2657. doi:10.1007/s10706-018-00784-y
Huang, Z., Zhang, H., Liu, B., Wei, B., and Wang, H. (2021). Using CT to Test the Damage Characteristics of the Internal Structure of Expansive Soil Induced by Dry-Wet Cycles. AIP Adv. 11 (7), 075305. doi:10.1063/5.0057450
Hvorslev, M. J. (1961). Physical Components of the Shear Strength of Saturated Clays. Fort Collins, United States: American Society of Civil Engineering.
Julina, M., and Thyagaraj, T. (2018). Determination of Volumetric Shrinkage of an Expansive Soil Using Digital Camera Images. Int. J. Geotechnical Eng. 15, 1–9. doi:10.1080/19386362.2018.1460961
Katti, D. R., and Shanmugasundaram, V. (2001). Influence of Swelling on the Microstructure of Expansive Clays. Can. Geotech. J. 38 (1), 175–182. doi:10.1139/t00-079
Kayadelen, C., Tekinsoy, M. A., and Taşkıran, T. (2007). Influence of Matric Suction on Shear Strength Behavior of a Residual Clayey Soil. Environ. Geol. 53 (4), 891–901. doi:10.1007/s00254-007-0701-2
Lade, P. V. (2010). The Mechanics of Surficial Failure in Soil Slopes. Eng. Geology. 114 (1-2), 57–64. doi:10.1016/j.enggeo.2010.04.003
Li, Z., Tang, C., Hu, R., and Zhou, Y. (2013). Research on Model Fitting and Strength Characteristics of Critical State for Expansive Soil. J. Civil Eng. Management 19 (1), 9–15. doi:10.3846/13923730.2012.734857
Liang, C., Wu, Z., Liu, X., Xiong, Z., and Li, T. (2021). Analysis of Shallow Landslide Mechanism of Expansive Soil Slope under Rainfall: a Case Study. Arab J. Geosci. 14 (7). doi:10.1007/s12517-021-06829-6
Lin, B., and Cerato, A. B. (2014). Applications of SEM and ESEM in Microstructural Investigation of Shale-Weathered Expansive Soils along Swelling-Shrinkage Cycles. Eng. Geology. 177, 66–74. doi:10.1016/j.enggeo.2014.05.006
Mašín, D., and Khalili, N. (2016). Swelling Phenomena and Effective Stress in Compacted Expansive Clays. Can. Geotech. J. 53 (1), 134–147. doi:10.1139/cgj-2014-0479
Qi, S., and Vanapalli, S. K. (2018). Simulating Hydraulic and Mechanical Responses of Unsaturated Expansive Soil Slope to Rainfall: Case Study. Int. J. Geomech. 18 (6), 1–17. 05018002. doi:10.1061/(asce)gm.1943-5622.0001106
Qi, S., Vanapalli, S., Yang, X., Zhou, J., and Lu, G. (2019). Stability Analysis of an Unsaturated Expansive Soil Slope Subjected to Rainfall Infiltration. Int. J. Geomech. 1. doi:10.12989/gae.2019.19.1.001
Xiao, J., Yang, H.-p., Zhang, J.-h., and Tang, X.-y. (2018). Surficial Failure of Expansive Soil Cutting Slope and its Flexible Support Treatment Technology. Adv. Civil Eng. 2018, 1–13. doi:10.1155/2018/1609608
Xiao, J., Yang, H., Zhang, J., and Tang, X. (2017). Properties of Drained Shear Strength of Expansive Soil Considering Low Stresses and its Influencing Factors. Int. J. Civil Eng. 16. doi:10.1007/s40999-017-0268-6
Ye, W., Zhang, Y., Chen, B., Zhou, X., and Xie, Q. (2010). Shear Strength of an Unsaturated Weakly Expansive Soil. J. Rock Mech. Geotechnical Eng. 2 (2), 155–161. doi:10.3724/sp.j.1235.2010.00155
Zhai, J.-y., and Cai, X.-y. (2018). Strength Characteristics and Slope Stability of Expansive Soil from Pingdingshan, China. Adv. Mater. Sci. Eng. 2018, 1–7. doi:10.1155/2018/3293619
Zhang, J., Niu, G., Li, X., and Sun, D. a. (2019). Hydro-mechanical Behavior of Expansive Soils with Different Dry Densities over a Wide Suction Range. Acta Geotech. 15, 265–278. doi:10.1007/s11440-019-00874-y
Zhang, J., Sun, D. a., Zhou, A., and Jiang, T. (2016). Hydromechanical Behaviour of Expansive Soils with Different Suctions and Suction Histories. Can. Geotech. J. 53 (1), 1–13. doi:10.1139/cgj-2014-0366
Zhang, R., Liu, Z., Zheng, J., and Zhang, J. (2020a). Experimental Evaluation of Lateral Swelling Pressure of Expansive Soil Fill behind a Retaining Wall. J. Mater. Civ. Eng. 32 (2), 04019360. doi:10.1061/(asce)mt.1943-5533.0003032
Zhang, R., Long, M.-x., Lan, T., Zheng, J.-l., and Geoff, C. (2020b). Stability Analysis Method of Geogrid Reinforced Expansive Soil Slopes and its Engineering Application. J. Cent. South. Univ. 27 (7), 1965–1980. doi:10.1007/s11771-020-4423-x
Keywords: expansive soil, dry density, improved direct shear test, slope stability, shear strength
Citation: Lan T, Zhang R, Yang B and Meng X (2022) Influence of Swelling on Shear Strength of Expansive Soil and Slope Stability. Front. Earth Sci. 10:849046. doi: 10.3389/feart.2022.849046
Received: 05 January 2022; Accepted: 10 March 2022;
Published: 06 April 2022.
Edited by:
Yun Zheng, Institute of Rock and Soil Mechanics (CAS), ChinaReviewed by:
Shunchao Qi, Sichuan University, ChinaMohammadhossein Sadeghiamirshahidi, Montana Technological University, United States
Copyright © 2022 Lan, Zhang, Yang and Meng. This is an open-access article distributed under the terms of the Creative Commons Attribution License (CC BY). The use, distribution or reproduction in other forums is permitted, provided the original author(s) and the copyright owner(s) are credited and that the original publication in this journal is cited, in accordance with accepted academic practice. No use, distribution or reproduction is permitted which does not comply with these terms.
*Correspondence: Rui Zhang, enJAY3N1c3QuZWR1LmNu