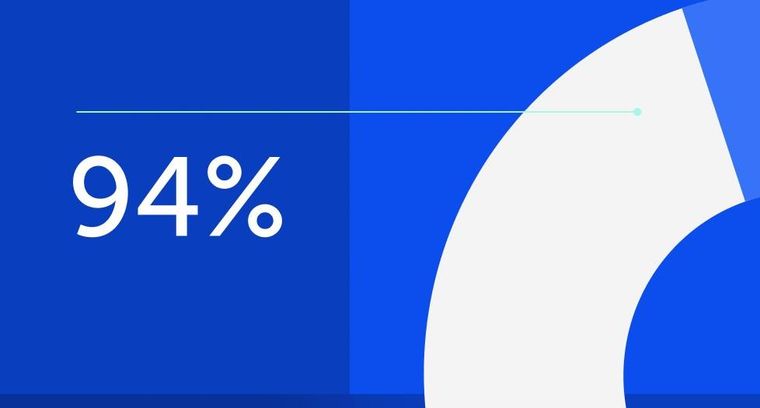
94% of researchers rate our articles as excellent or good
Learn more about the work of our research integrity team to safeguard the quality of each article we publish.
Find out more
ORIGINAL RESEARCH article
Front. Earth Sci., 24 March 2022
Sec. Quaternary Science, Geomorphology and Paleoenvironment
Volume 10 - 2022 | https://doi.org/10.3389/feart.2022.847358
This article is part of the Research TopicHuman Behavior, Cognition, and Environmental Interactions for the Lower PaleolithicView all 10 articles
The life cycle of a successful technological innovation usually follows a well-known path: a slow inception, gradual assimilation of the technology, an increase in its frequency up to a certain peak, and then a decline. These different phases are characterized not only by varying frequency of use but also by degree of standardization and distinguishability. The Levallois method, a sophisticated Middle Paleolithic technology aimed at producing desired stone items of predetermined morphology, is one such innovation. It has been repeatedly suggested that the Levallois method originated within earlier Lower Paleolithic Acheulian industries, and this work contributes to this discussion. We analyze the reduction trajectory of prepared cores and predetermined blanks from the late Acheulian sites of Jaljulia and Revadim, adding important new evidence for the Lower Paleolithic origins of the Levallois method and its adoption and assimilation in the human stone-tool repertoire of this period in the Levant. Revadim and Jaljulia also provide a rare opportunity to study patterns in the early assimilation of technological innovations. These sites yielded rich lithic assemblages typical of the late Acheulian in the Levant. The assemblages include handaxes but are mostly dominated by flake production technologies and flake-tools. The early appearance of prepared cores at both sites signals, in our view, the inception of concepts related to the Levallois method, termed here proto-Levallois, in the late Acheulian Levant. Through a detailed analysis of prepared cores and their products, we are able to characterize the early stages of assimilation of this method, using it as a case study in a broader discussion of the adoption and assimilation of technological innovations during Lower Paleolithic times.
LEVALLOIS. Sometime a term becomes embedded with so much meaning that a single word can stand alone to serve as a code for a set of behaviors and capabilities that characterize early hominin adaptation. It is no wonder, then, that the origins of the Levallois method remain a central and controversial topic in Paleolithic archaeology.
The invention, introduction and assimilation of the Levallois method reflect a significant change in the cognitive and technological capabilities of Middle Pleistocene hominins (e.g., Bordes, 1971; Ambrose, 2001; Wynn and Coolidge, 2010; Stout, 2011; Eren and Lycett, 2012; Cole, 2015; Stout, et al., 2015; Wynn and Coolidge, 2016; Muller et al., 2017). This method played a significant role in human adaptation over a vast time span of at least 200,000 years, before being replaced by blade production technologies at the advent of the Upper Paleolithic period, some 45/47,000 years ago (e.g., Boaretto et al., 2021).
Following central paradigms in Paleolithic research, it is generally accepted that Early-Middle Pleistocene technological concepts of stone-tool production and use are roughly correlated with the two major cultural complexes known as the Lower Paleolithic Acheulian and the Middle Paleolithic Mousterian (sometimes referred to as Mode 2 and Mode 3 technologies, e.g., Clark, 1969; Ambrose, 2001; Stout, 2010). The Lower Paleolithic Acheulian Cultural Complex (henceforth Acheulian) is characterized by the production of small, medium and large (over 10 cm) flakes, the manufacture of bifaces, usually known as handaxes or Large Cutting Tools, and sets of core-tools (e.g., chopping tools, cleavers, spheroids/polyhedrons) and flake-tools (Sharon, 2007; Lycett and Gowlet, 2009; Machin, 2009; Sharon, 2009; Tryon and Potts, 2011; Agam et al., 2015; Shimelmitz, 2015; Sharon et al., 2011; Sharon, 2014; Agam and Barkai, 2018a; Finkel and Barkai, 2018; Goren-Inbar et al., 2018). The Acheulian handaxe appears in a vast geographical range in Africa, Europe and west and east Asia starting at around 1.8 million years ago (Bar-Yosef and Belmaker, 2010; Bar-Yosef and Belfer-Cohen, 2011; Dennel, 2011; Jiménez-Arenas et al., 2011; Lepre et al., 2011; Pappu et al., 2011). While in Europe biface manufacture and use continued into the Middle Paleolithic Mousterian (roughly 200-40/30 kya), bifaces disappear altogether from post-Acheulian, Middle Paleolithic Mousterian industries in the Levant (Hovers and Belfer-Cohen, 2013; Sharon, 2014; Hérisson and Soriano, 2020; Mathias et al., 2020) and Africa (Tryon and Faith, 2013; Richter et al. ,2017). However, some scholars point out substantial differences between Acheulian and Mousterian bifaces in Europe (e.g., Soressi, 2004; Claud, 2008; White and Pettit, 2016).
Middle Paleolithic (MP) assemblages in the Levant do not contain any “core-tools” (handaxes, cleavers and spheroids), while blanks (flakes, blades and points) were produced using various techniques. Flake-tools predominate. The Levantine MP is most prominently characterized by the use of the Levallois method, a well-studied technological system aimed at the production of well-planned and predetermined items (Boëda et al., 1995; Schlanger, 1996; Chazan, 1997; Eren and Lycett, 2012; Hovers and Belfer-Cohen, 2013; Shea, 2013). Other reduction strategies were used elsewhere alongside the Levallois (e.g., Meignen, 2000, Meignen, 2002), but in the Levant, the Levallois prevailed.
The late Acheulian sites of Revadim and Jaljulia, both in central Israel, provide a rare opportunity to study the patterns of assimilation of a technological innovation within the lithic assemblages of the Levantine Late Lower Paleolithic. The assemblages, typical of the late Acheulian Levant, are mostly dominated by flake production and flake-tools but also include handaxes (for Revadim, see, e.g., Malinsky-Buller et al., 2011; Marder et al., 2011; Rabinovich et al., 2012; Agam and Barkai, 2018a; Zupancich et al., 2018; Rosenberg-Yefet and Barkai, 2019; for Jaljulia, see Shemer et al., 2018; Rosenberg-Yefet et al., 2021; Zupancich et al., 2021; Shemer et al., submitted).
Revadim and Jaljulia contribute important new data in support of the early Lower Paleolithic origins of the Levallois method in the Levant. Our findings show that these sites might represent an early stage in the assimilation of technologies that resemble the Levallois and were rather widespread within late Acheulian archaeological sites in this region. In this paper we will present the reduction sequence of the proto-Levallois method as represented at both sites and characterize what we believe to be the early stages of its assimilation, using it as a case study to discuss broader issues of the adoption and assimilation of technological innovations during Lower Paleolithic times.
The Levallois method is a distinctive blank production method for manufacturing flakes/blades of predetermined shape and size. Careful preparation of the core in a series of removals creates the necessary core convexities and dictates the size and shape of the desired end products (Boëda et al., 1995; Schlanger, 1996; Chazan, 1997; Eren and Lycett, 2012). Examples of this widely distributed method, typically associated with Homo heidelbergensis, Homo neanderthalensis, Homo sapiens, Denisovans and other MP human groups, can be found in sites in Africa, Europe and Asia (e.g., Eren and Lycett, 2012; Adler et al., 2014; Akhilesh et al., 2018; Jacobs et al., 2019; Hu et al., 2019; Centi and Zaidner, 2021; Hershkovitz et al., 2021). As we will later show, the appearance of the Levallois method at pre-Middle Paleolithic sites indicates that it was most probably invented and assimilated by the ancestors of Middle Paleolithic hominins in the Levant.
Levallois cores are characterized by two asymmetrical platforms, separated by a plane of intersection. The lower platform has a larger volume and serves as the striking platform, while the upper platform serves as the production surface. The hierarchical relationship between the two platforms means that they cannot be switched during the reduction sequence. The Levallois concept can also be thought of as a specific organization of the volume from which a mass of material will be reduced while preserving the convexities of the platforms (Boëda et al., 1995; Van Peer, 1995). Levallois cores that can produce one flake are termed lineal\preferential cores, and those that can produce a series of flakes are termed recurrent cores. These cores are further categorized as unidirectional, bidirectional, or centripetal, in accordance with the direction of the flaking on the production surface (Inizan, 1999:61–68; Shea 2013:84–93).
Many definitions of the Levallois method have been proposed. The early definitions, influenced by Bordes’s (1971) typological approach, were morphological, focused mainly on the form of the blanks. But this approach ignored the process itself: core preparation, reduction, and maintenance (Van Peer, 1995; Chazan, 1997). In the 1990s, with advances in the study of the Levallois method, a group of French archaeologists proposed a more precise definition of this technology, to which they assigned six major characteristics (Boëda et al., 1995): 1) the volume of the core is bifacial, with two distinct surfaces that intersect at the core’s margin, thereby forming a “plane of intersection”; 2) the two surfaces are hierarchically related, one constituting the striking platform and the other the primary production surface; 3) the flaking surface is shaped to possess both distal and lateral convexities; 4) the blanks are removed parallel to the plane of intersection; 5) the intersection of the striking platform surface and the flaking surface is perpendicular to the flaking axis of the blank; 6) direct hard hammer percussion is used to remove the blanks.
By thus reducing the core, the knapper was able to produce two types of items: predetermined items, which are the target items (or end products), and predetermining items, which were detached during core design and maintenance. This division facilitates technological understanding but also illuminates the intention and preplanning of the knapper, with all their cognitive implications (Schlanger, 1996). The Levallois is viewed as more cognitively sophisticated than earlier methods of flint knapping practiced by Lower Paleolithic Acheulian hominins (e.g., Ambrose, 2001; Pelegrin, 2005; Haidle, 2010; Stout, 2011; Eren and Lycett, 2012; Stout et al., 2015; Wynn and Coolidge, 2016). It is thus frequently regarded as a landmark in cognitive human evolution, indicative of working memory capacity and expertise, among other qualities (Ambrose, 2001; Pelegrin, 2005; Haidle, 2010; Wynn and Coolidge, 2010; Stout, 2011; Eren and Lycett, 2012; Cole, 2015; Stout et al., 2015; Wynn and Coolidge, 2016). However, the diversity of complex prepared core methods which precedes the emergence of the fully fledged Mousterian Levallois method weakens any claims for a cognitive “jump” (Chazan, 2020).
A reconstruction of the reduction sequence reveals how these cores reached their final shape, the one usually found at archaeological sites (sometimes nicknamed “tortoise-shape” cores). The knapper focused first on the shaping of the striking platform. Most preparations were initiated at the distal and proximal ends. In some cases, the lateral edges remained almost unshaped. As the reduction process continued, the knapper focused on the proximal end of the core rather than the distal one. In the case of recurrent Levallois cores, most preparations were carried out during the first stage of core design, while later in the process, preparations were only minimal (Van Peer, 1992). The preparation stage is clearly the most important, and hence the preplanning ascribed to the method.
Humans in the Old World produced sharp-edged stone items as early as the Lomekwian and Oldowan stone tool industries in Africa (Harmand et al., 2015; Toth and Schick, 2018). Why, then, after more than two million years of flake production, did Middle Pleistocene early humans begin to produce such items by means of a complicated and sophisticated technological procedure such as Levallois (Režek et al., 2018)? Once considered a relatively wasteful production method in terms of volume removed to achieve the desired end product, the Levallois is now seen in a different light (Lycett and Eren, 2013; Shimelmitz and Kuhn, 2018). Studies over the last two decades argue that the need for certain functional advantages might have motivated people to produce items in a new way. These studies suggest that the Levallois method is in fact more efficient in terms of both stone utilization and controlling the shape and size of the end-product (Brantingham and Kuhn, 2001; Eren and Lycett, 2012; Lycett and Eren, 2013; Muller et al., 2017). A further advantage of the Levallois method is that it produces a wide and rather thin flake that extends across most of the core production surface but is also light enough for easy transport and use. Another possible advantage is in the obtuse angle between the two faces of the end product, which makes these items more resistant and durable (Eren and Lycett, 2016) while also facilitating improved handling and hafting. The relative standardization of the end products might be seen as an advantage as well (Schlanger, 1996), in terms of knapping economy and ease of hafting, key factors according to some scholars (Hérisson and Soriano, 2020). The use of technology that allows better control over the final product reduced the need for further retouch of the items after flake production (Moncel et al., 2020).
While the Levallois method was once widely viewed as a Middle Paleolithic innovation, the idea that it was practiced in the Levantine Acheulian, and especially the late Acheulian assemblages, is no longer outside the mainstream. Its roots in the Lower Paleolithic Acheulian have been demonstrated by a plethora of studies during the last four decades in sites in Africa (Rolland, 1995; Tryon, 2006; de la Torre, 2010; Wilkins et al., 2010), Europe (Villa, 2009; Despriée et al., 2010; Nowell and White, 2010; Moncel et al., 2011; Moncel et al., 2011; Rodríguez et al., 2011; Ollé et al., 2013; Picin et al., 2013; Moncel et al., 2015; Hérisson et al., 2016), the Levant (Gilead and Ronen, 1977; Goren, 1979; Ronen et al., 1980; Goren-Inbar, 1985; Chazan, 2000; DeBono and Goren-Inbar, 2001; Goren-Inbar, 2011; Shimelmitz et al., 2016; Zaidner and Weinstein-Evron, 2016; Goren-Inbar et al., 2018; Chazan 2020), and the Caucasus (Adler et al., 2014). Additionally, recent publications demonstrate that the Levallois method appears earlier than previously thought in India (Akhilesh et al., 2018) and perhaps even in China (Hu et al., 2019, although see Li et al., 2019 for reservations). We do note, however, that some researchers see the Levallois as having arrived in the Levant from outside the region, probably from Africa, linking its beginnings to the appearance of Homo sapiens. In this approach, its appearance in the Middle Paleolithic is thus seen as a completely new phenomenon, and not a gradual local development (Zaidner and Weinstein-Evron, 2020), as we argue in this paper.
The Levallois method also varies widely from region to region, as reflected, for example, in Levallois variants such as the Nubian and the Aduma of the Khormusan in Africa (Goder-Goldberger, 2013; Usik et al., 2013). In addition to the well-defined variants themselves, inter- and intra-site variability is common (e.g., Meignen, 1995; Tuffreau, 1995; Hovers and Belfer-Cohen, 2013; Hérisson et al., 2016; Carmignani et al., 2017; Prévost and Zaidner, 2020; Centi and Zaidner, 2021). Variability (regional or not) raises an important question regarding the ability to identify technologies at different stages of adoption. Variants at the periphery of the mainstream of the technology might be misinterpreted as stages of discovery and/or adoption. This challenging issue is, however, beyond the scope of this paper, which focus on the late Acheulian in the Levant, before the fully fledged Levallois method was practiced. Thus, the identified variants could not be mistaken for anything but early stages of adoption or assimilation.
Despite the growing consensus that the Levallois had Acheulian roots, its practice in the later terminal Lower Paleolithic Acheulo-Yabrudian cultural complex is still unresolved. The definition of the Acheulo-Yabrudian cultural complex did not originally include the use of the Levallois method as an integral part of the technological repertoire and adaptation strategy of these early humans (Barkai and Gopher, 2013; Adler et al., 2014; Gopher et al., 2005). However, some scholars have argued for the presence of some characteristics of the Levallois method at Acheulo-Yabrudian sites as well (Bar Yosef and Belmaker, 2010; Shimelmitz et al., 2016; Zaidner and Weinstein-Evron, 2016). At the Acheulo-Yabrudian site of Qesem Cave, in any case, the 200,000 years of human occupation of the site (Barkai and Gopher, 2013) show no evidence of Levallois practice, despite the very close proximity of the cave to the late Acheulian site of Jaljulia, for which we will present evidence that Levallois concepts were practiced to some extent. Therefore, although technological continuity from the terminal Lower Paleolithic late Acheulian to the Acheuleo-Yabrudian and to the Middle Paleolithic has not yet been established, the presence of Levallois concepts during this time interval remains an intriguing scenario that awaits further data and analyses.
Pre-Middle Paleolithic cores displaying Levallois characteristics are defined differently for the different sites, seriously impeding any effort to clearly portray the chrono-geographic distribution of these items. The various definitions include, for example, prepared cores, centripetal cores, mode 3 technology, hierarchical cores, radial cores, proto-Levallois cores, and more (White and Ashton, 2003; Wilkins et al., 2010; Moncel et al., 2015; Picin, 2017; Leader et al., 2018, to name but a few). To try and mitigate the confusion arising from the multiplicity of terms used to describe these items, we will consider all of them to be prepared cores, sharing new concepts of flake production technology, as will be described in detail later.
The actual technological origins of the Levallois are also controversial. Some see earlier core technologies as a source for the emergence of the Levallois method. The Victoria West and Tabelbala-Tachengit giant core technologies have been cited in support of this approach (Sharon, 2009), as have as the cores of the Kapthurin Formation (Tryon et al., 2005; Johnson and McBrearty, 2012). Similarly, Adler et al. (2014) see the development of Levallois method as a local evolutionary process, suggesting that “Levallois technology is an inherent property of the Acheulian that evolves out of the existing.” Others see the Acheulian handaxes as the technological source of origin. Handaxes characterized by a later preferential flake scar might offer evidence of a link to Levallois technologies (DeBono and Goren-Inbar, 2001; Marder et al., 2006; Goren-Inbar, 2011; Shimelmitz, 2015; Rosenberg-Yefet et al., 2021). The knapper used a bifacial flaking strategy that took advantage of the convexities on both faces of the handaxe, a concept similar to the Levallois method. Rolland (1995), however, takes the view that the Levallois method might have been discovered by accident, as a result of knapping mistakes. He suggests that the skill involved in the production of handaxes, and particularly the final stage of handaxe trimming by the detachment of thinning flakes, could have led to this “accidental” discovery. Shipton et al. (2013), on the other hand, suggest that “Levallois technology appears to have arisen out of adapting aspects of handaxe knapping, including shaping of surfaces, the utilization of two inter-dependent surfaces, and the striking of invasive thinning flakes”. Moreover, White et al. (2011) see the combination of bifacial flaking and predetermined blank production as two elements that together provide the technological background for the invention and appearance of the Levallois method. In this same context, a recent paper (Rosenberg-Yefet et al., 2021) questioned the association of a single technological origin with the emergence of the Levallois method. The authors argued that human culture is an amalgamation of many different cultural traits as well as many innovation events. In what is known as “cumulative cultural evolution” or “the ratchet effect” (Tomasello, 1999:37; Tennie et al., 2009; Mesoudi, 2011), a technological innovation can be a combined outcome of several different technological trajectories. Hence, the Levallois method could have been invented and assimilated as the result of both handaxe technology as well as Acheulian core technologies. The existence of a “pull of knowledge” was suggested for the emergence of prepared core technologies during the early Middle Paleolithic at the site of Payre, France, where different methods (Levallois, discoid and Quina) were used in tandem and might imply a combination of different trajectories (Baena et al., 2017).
Two distribution models are generally considered in the study of the emergence and spread of significant innovations: cultural transmission versus convergent cultural evolution. The first model posits invention in one core-area that becomes widespread through social learning (Fontana et al., 2013; Groucutt et al., 2015; Lycett et al., 2016). The second model posits the invention of a technology independently in different areas, through individual learning (White et al., 2011; Wilkins and Chazan, 2012; Ollé et al., 2013; Adler et al., 2014; Shipton, 2016). The gradual, local development of a technological innovation was shown recently through the Orgnac 3 sequence for the European case (Bahain, et al., 2022). This important issue will be addressed in depth in a separate paper.
In the Levant, the adoption of prepared core technologies might also have been influenced by environmental conditions. Unlike Europe, the Levant was not heavily influenced by glacial and inter-glacial cycles (however, it is evident that other environmental conditions, related not to climate but to the availability of prey animals, changed dramatically at the end of the Lowe Plaeolithic period. This change is reflected in the transition from the consumption of very large animals in the Lower Paleolithic Acheulian (megaherbivores, specifically proboscideans) to medium and small animals in the terminal Lower Paleolithic (the Acheuleo-Yabrudian Cultural Complex, or AYCC) and the Middle Paleolithic of the southern Levant. A recent study examining faunal remains from 58 Pleistocene sites from the Levant demonstrates this dramatic decline in animal body mass but suggests that climate change had little, if any, effect on that decline (Dembitzer et al., 2022).
Mega herbivores, and elephants in particular, provided a unique combination of fat and meat, making them the “ideal” caloric package for early humans (Guil-Guerrero et al., 2014; Guil-Guerrero et al., 2018). In addition to their high fat content, high energetic return, and significant biomass, it is suggested that early human were capable and skillful in hunting megaherbivores (Bunn and Pickering, 2010; Bunn and Gurtov, 2014; Domínguez-Rodrigo et al., 2017; Agam and Barkai, 2018b; Bunn, 2019; Ben-Dor and Barkai, 2020; Ben-Dor et al., 2021a; Ben‐Dor et al., 2021b; Ben-Dor and Barkai, 2021). Proboscidean remains are indeed found at many Lower Paleolithic Acheulian sites in the Levant and beyond (e.g.,: Goren-Inbar et al., 1994; Solodenko et al., 2015; Zutovski and Barkai, 2016; Ben-Dor and Barkai ,2020a; Barkai, 2021; Konidaris and Tourloukis, 2021).
However, it appears that proboscideans played a role in Lower Paleolithic human life and cosmology well beyond their dietary importance. Archaeological and ethnographic research supports the idea that early humans in the Old and New Worlds shared habitats with other animals while perceiving them as both essential food sources and equal other-than-human entities (Tanner, 2014; Barkai, 2019; Barkai, 2021). In hunter-gatherer societies, some animals are perceived as equal partners in a relationship defined by reciprocity (Ingold, 2000; Tanner, 2014; Halfon and Barkai, 2020), although not all hunter-gatherers perceive these relationships in exactly the same way (Lewis, 2021; Tanner, 2021).
The nexus between faunal and technological transformations and/or stability during Pleistocene times has led some of us to posit a link between the hunted animals, the technology used to hunt and process them, and the cosmological perceptions of the hunters (Finkel and Barkai, 2018; Halfon and Barkai, 2020; Finkel and Barkai, 2021). In this view, early humans during Lower Paleolithic times adopted technologies suited to the hunting and processing of large herbivores. Their reasons for doing were, of course, practical, but were motivated also by cosmology (e.g., Finkel and Barkai, 2021; Dembitzer et al., 2022), reflected, for example, in the manipulation of elephant bones for bot nutritional and non-nutritional purposes, such as the use of bones for the production of bifaces (Mussi, 2005; Finkel and Barkai, 2018; Boschian et al., 2019; Barkai, 2021). When these prey animals were no longer available, the hunters adopted new technology suited to the acquisition and processing of smaller animals. Elephants disappeared from post-Acheulian sites in the Levant and so did handaxes, perhaps paving the way for new technological changes to come.
Changes in human culture as a response to changes in the environment have been proposed as a driver of technological change that might also have led to the invention and assimilation of the Levallois. In studies of east Africa, for example (Potts and Faith, 2015; Owen et al., 2018; Potts, et al., 2018), analyses of sediments from Lake Magadi (Kenya) reveal significant environmental changes around 525 to 400 ka, mainly increasing aridity, accompanied by a significant change in the fauna, mainly the local extinction of several large-bodied mammals and their replacement by smaller, related species, leading in turn to the need for new toolkits (Owen et al., 2018). Environmental changes such as these are known to create pressure on the population, forcing humans into greater mobility and therefore greater interaction between groups. That scenario can also motivate technological changes and facilitate the spread of technological knowledge (Potts et al., 2018). A study by Picin (2017) also links the invention of the Levallois method to environmental and ecological changes. According to this hypothesis, the Levallois method was a response to the changing faunal conditions, bringing early humans to increase their foraging radius and change their mobility patterns, which in turn motivated changes in core technology.
Whatever its origins, we can expect the Levallois life cycle to follow a pattern that typifies many other innovations: gradual emergence and early ascent, more widespread assimilation, and eventual domination (Henrich, 2001). We thus expect the early Levallois technology (senso lato) at the two late Acheulian sites of Revadim and Jaljulia to differ from its mature, full-fledged Middle Paleolithic version in terms of standardization, technological distinctiveness, and frequency of appearance. The present paper aims at capturing an intriguing phase in the adoption and spread of this technological innovation during late Acheulian times in the Levant.
Revadim is a multi-layered late Acheulian site located on the southern coastal plain of Israel (Figure 1A). Paleomagnetism results for the sequence exposed in the quarry showed normal polarity, indicating that the sequence is younger than 780,000 BP. Uranium-thorium analysis of carbonate covering flint items dated it to between 500,000 and 300,000 BP. During four excavation seasons, four main areas were excavated (A–D) as well as two trenches which stratigraphically connect Areas C and B. Altogether, an area of 250 m2 was excavated, 170 m2 were exposed in Areas A–D, and ca. 80 m2 in Trenches 12 and 23. The analysis of the flint and faunal assemblages indicates a late Acheulian assignment of all layers of the site (see details in Gvirtzman et al., 1999; Marder et al., 1999; Marder et al., 2011; Rabinovich et al., 2012; Solodenko et al., 2015; Agam and Barkai, 2018a; Zupancich et al., 2018; Rosenberg-Yefet and Barkai, 2019; Rosenberg-Yefet et al., 2021). The rich faunal assemblage includes Palaeoloxodon antiquus, Bos primigenius, Gazella gazelle, Capra cf. aegagrus, and Dama cf. mesopotamica (Gvirtzman et al., 1999; Rabinovich et al., 2012).
FIGURE 1. (A) Location of the sites discussed in the text; (B) Revadim site areas of excavation; (C) A close-up at the archaeological horizon of Area B, Jaljulia. Note the high density of the flint items; (D) Jaljulia site areas of excavation.
Previous analysis of the Revadim site revealed a varied core technology that includes three main reduction sequences: 1) The production of large and medium flakes from single, double or multiple striking platform cores, 2) the production of predetermined items from prepared cores and discoid cores, and 3) the production of very small flakes by recycling of existing items as cores on flakes (Malinsky-Buller et al., 2011; Agam et al., 2015; Solodenko et al., 2015; Rosenberg-Yefet, 2016; Agam and Barkai, 2018a; Venditti et al., 2019a; Venditti et al., 2019b).
The blanks produced by these methods were sometimes further modified in different ways and constitute the largest group of tools, termed flake-tools, alongside a wide variety of bifaces and other tool types (e.g., handaxes and chopping tools) (Marder et al., 2006; Solodenko, 2010; Malinsky-Buller et al., 2011; Agam et al., 2015; Solodenko et al., 2015; Malinsky-Buller, 2016; Cohen, 2018; Rosenberg-Yefet and Barkai, 2019).
The sample chosen for this study includes prepared cores and items detached from prepared cores, originating from four different contexts of the site, representing the four lithic assemblages studied thus far. These are layers B2 and C5 (Figure 1B), which are the oldest contexts of the site and assumed to be rather chrono-stratigraphically contemporaneous, and layers C3 and B1, which are stratigraphically younger. All four assemblages have been initially sorted and analyzed (Supplementary Table S1).
Core trimming elements (CTEs), which are the products of shaping and maintaining prepared cores and originated from the studied layers (C3 and C5), were also included. These items were separated from the rest of the CTE assemblage during preliminary classification.
Jaljulia is a late Acheulian site located on the central part of the coastal plain of Israel (Figure 1A), some 6 km south of the late Acheulian site of Eyal and 6 km north-west of the Acheulo-Yabrudian site of Qesem Cave (Ronen and Winter 1997; Gopher et al., 2005). Approximately 80 m2 of late Acheulian deposits were excavated during 2016–2017 in the framework of a salvage excavation by the Israel Antiquities Authority in collaboration with the Department of Archaeology at Tel-Aviv University (Shemer et al., 2018). Lithic assemblages are rich (Figure 1C) but faunal preservation is poor. Lithic analysis is currently in process, and therefore only preliminary observations are currently available (Supplementary Table S2). The assemblages are mostly dominated by flake production and flake-tools, although all include handaxes. Core technology varies and includes three main reduction sequences, very much similar to those described above for Revadim. The lithic assemblage chosen for this study, currently at the final stages of lithic analysis, was retrieved from an area of 6 m2 (area B, Figure 1D).
A total of 72 prepared cores from Revadim (out of a total of 1407 cores from the four assemblages, 5.1% of all cores) and a total of 105 prepared cores, including 13 core fragments, (out of a total of 602 cores, 17.4% of all cores) from Jaljulia were included in the current study, with a further subdivision into proto-Levallois cores, discoid cores, and prepared cores (general) (Supplementary Figure S1) for those that could not be assigned to the Levallois/discoid categories. See Supplementary Table S3 for details.
Characterizing prepared cores is difficult due to the variety of definitions used in the literature and the lack of uniformity. While some scholars use the term “prepared cores” to describe cores similar to Levallois cores (e.g., Wilkins et al., 2010; Leader et al., 2018), we use the term more generally to include proto-Levallois cores but also other core methods. Our definition is in the spirit of Debénath and Dibble (1994:23), who defined prepared cores as “a number of technologies…in which the core was intentionally shaped or prepared in such a way as to predetermine the shapes of flakes taken from it”. All cores under that definition are fully or partially centripetal in their design, in contrast to one, two or multi-platform cores. Therefore, all cores are characterized by two surfaces and a plane of intersection, although their exact role in the reduction sequence varies between sub-methods, as does the presence or absence of hierarchy between them.
Cores originating from pre-Middle Paleolithic contexts but bearing conceptual resemblance to Levallois cores are defined in very different ways by different scholars. We follow here the definition proposed by Picin (2017), whereby proto-Levallois cores are cores in which the volume is divided into two hierarchical surfaces, a striking platform and a flaking surface, with a plane of intersection separating the two surfaces. The plane of intersection is delineated by a partial or complete bifacial ridge. Striking platforms are usually roughly prepared, and lateral and distal convexities of the flaking surface are roughly configured by either débordants flakes or by preparational flaking of the core circumference (Picin 2017). In this study we did not define cores as fully fledged Levallois, though some of the cores do represent a more developed stage of the technology’s adoption and some do meet all Boëda’s criteria. The main technological difference concentrates on less strict application of the criteria. For instance, core circumference is not always prepared fully and lateral and distal convexities of the flaking surface are sometimes only roughly designed in Proto-Levallois cores.
The discoid method was the most common core technology within the prepared core category from the Lower Paleolithic onwards, although it was recognized and defined as Mousterian debitage at earlier stages of research (González-Molina et al., 2020).
On the basis of definitions by both Boëda in the mid-1990s and Terradas (2003), the criteria for identifying discoid cores were recently summarized by González-Molina et al. (2020): 1) the volume of the core is conceived in two asymmetric, secant and convex surfaces that delimit an intersection plane. 2) There is no hierarchization between the two surfaces of the core: one is conceived as a flaking surface and the other as striking platform, but with the possibility of role inversion during one operational sequence. 3) The exploitation or debitage surface is designed with a peripheral convexity that controls the knapping of each extraction. 4) The flaking axis of predetermined removals is perpendicular to the striking platform employed to obtain it. 5) The striking surface is oriented so that the intersection between both surfaces is perpendicular to the edge of the core. 6) The technique is direct percussion using a hard hammer.
Because the discoid method shares four of the six criteria described by Boëda (1993) (detailed in the introduction), further clarification is required to distinguish the two methods. The discovery and analysis of variety of discoid cores, both chronologically and geographically distributed, as part of a collection of studies by Peresani (2003), have helped researchers to resolve some of the ambiguities by distinguishing between the sensu stricto and sensu lato definitions of discoid cores (Picin and Vaquero, 2016). The term discoid sensu lato describes better cores that do exhibit hierarchy between the two surfaces, whereas sensu stricto describes cores that differ from the Levallois in the lack of hierarchy between their surfaces and in the direction of production, which is secant to the plane of intersection of the two surfaces (Picin and Vaquero, 2016). For a recent study on the distinctions between these two methods, see González-Molina et al. (2020)
Our definition of discoid cores follows the guidelines set out by Terradas, who sees the discoid flaking method as consisting of “the core exploitation in order to obtain various numbers of flakes by centripetal, recurrent and usually bifacial organization of removals. This exploitation proceeds by maintaining a strong stability of the volumetric concept of the core, which requires little specific preparation of its striking platforms and flaking surfaces. The core resulting from this sort of exploitation has an oval shape and a biconvex asymmetric section” (Terradas, 2003). These cores comply with the sensu lato definition, since the term refers to cores that do exhibit hierarchy between the two surfaces, which in our case are also switchable.
We analyzed the cores by weight (light, 1–49 g, medium, 50–99 g, and heavy, above 100 g), flint quality (homogeneous, fairly homogeneous, and heterogeneous), and texture (fine-grained, medium-grained, and coarse). Determining flint quality and its effect on toolmaking is challenging. While microscopic material evaluations are gaining a foothold, this is out of the scope of the current research. Therefore, here we determined flint quality and texture as visible to the naked eye. Stone quality, although a subjective criterion, was determined in this study by the homogeneity of the material and by the presence or absence of disturbances in the flint. Homogeneous flint refers to flint with no disturbances or cracks. Fairly homogeneous flint will show one or two disturbances or cracks, and heterogeneous flint will show several. A detailed analysis of prepared core flint types from Jaljulia appears in Agam et al. (submitted).
Blank types include nodules, pebbles, old cores\handaxes, flakes, and undetermined. Handaxes that were recycled as cores while undergoing some modifications were included here, whereas handaxes with a preferential flake scar having minimal preparations were not included in the core category and are not part of the current study (see Rosenberg-Yefet et al., 2021 for a detailed description of these items). The presence of cortex on the striking platform and on the production surface was also noted, as was the presence of flaked patinated surfaces as an indication for the use of old flaked items as cores. Scar characteristics include the number of shaping scars on the production surface, the number of shaping scars on the striking platform, and the number of unsuccessful removal scars on each surface. Unsuccessful removals were determined by scars with hinge or step termination, scars indicating an angle which damaged core morphology, or scars that differ from the others in shape, depth or thickness. Scars that cross the midline of the production surface were also indicated. The minimum and maximum angles between the two platforms of the cores were measured. Core convexities were estimated (% of the core circumference). The presence of éclats débordants scars was noted, as were indications of striking platform preparation following the detachment of predetermined blanks. (By this we mean preparations that appear to have been made in the process of producing another item but the core was abandoned before that item was produced.) The core exploitation method (preferential, recurrent centripetal, recurrent unidirectional, or recurrent bidirectional) was also indicated. The detailed criteria are listed in Table 1, Table 2, and Table 3.
TABLE 1. Major attributes of proto-Levallois cores from both sites. Prefer. (Preferential); Rec. (Recurrent); centri. (centripetal); uni. (unidirectional); bidi. (bidirectional) Homoge. (Homogeneous); Hetero. (Heterogeneous) Undeter. (Undetermined).
TABLE 2. Major attributes of discoid cores from both sites. Alter. (Alternate); Switch. (Switchable hierarchy); Undeter. (Undetermined); Prefer. (Preferential); Rec. (Recurrent); centri. (centripetal); uni. (unidirectional); bidi. (bidirectional) Homoge. (Homogeneous); Hetero. (Heterogeneous).
TABLE 3. Major attributes of prepared cores (general) from both sites. Prefer. (Preferential); Rec. (Recurrent); centri. (centripetal); uni. (unidirectional); bidi. (bidirectional); Homoge. (Homogeneous); Hetero. (Heterogeneous) Undeter. (Undetermined).
CTEs were categorized as either CTEs removed from prepared cores or éclats débordants, with the latter category further subdivided into éclats débordants, éclats débordants a dos limité, and éclats outrepassés. CTES removed from prepared cores are items for which part of the intersecting ridge between the two platforms was removed, most probably in an effort to maintain core convexities throughout the reduction sequence. These items do not fully conform to the definition of the specific CTEs detailed below.
The éclats débordants items are defined as follows:
Éclats débordants (Supplementary Figure S2A): The terminology is drawn from similar items used in the application of the Middle Paleolithic Levallois method (Beyries and Boëda, 1983; Geneste, 1985:230; Meignen, 1995:280). It describes items removed from the lateral edge of a core during the shaping and maintenance of the production surface, mostly between production phases. Part of the bifacially shaped plane of intersection that separated the production surface from the striking platform was also removed (Boëda, 1993). Such removals took place during relatively advanced stages of the Levallois reduction sequence (Hovers, 2009:82).
Éclats débordants a dos limité (Supplementary Figure S2B), also termed pseudo-Levallois flakes: These items were produced during advanced stages of Levallois core reduction (Boëda et al., 1990:68; Hovers, 2009:82) and were removed from the core in order to correct irregularities in the morphology of the production surface, thus contributing to the maintenance of lateral convexities during the different phases of the Levallois reduction sequence (Boëda et al., 1990).
Éclats outrepassés (Supplementary Figure S2C). “These were detached only when intensive use of both the striking platform and the flaking surface of the core had distorted the geometry of the flaking surface. Rejuvenation of the lateral and (mainly) distal convexities required removal of the exploited surface and extensive rearrangement of the flaking surface. Such items are expected to show a large number of dorsal face scars and a complex dorsal face scar pattern” (Hovers, 2009:82).
Similarities between CTEs removed from prepared cores and items removed from handaxes during their different manufacturing stages are also noteworthy. These similarities, mainly in dorsal scar pattern and the removal of parts of the bifacial ridge, make it difficult to distinguish between these two categories (Copeland, 1995; Goren-Inbar et al., 2018).
CTEs were analyzed according to the following parameters: metric measurements (maximum length and width), scar pattern (centripetal, unidirectional, or bidirectional) and number of scars, platform preparation type (plain, punctiform, faceted, dihedral, linear, or undetermined), interior and exterior platform angle (IPA and EPA, respectively), ridge angle, cross section type (obtuse triangle, isosceles trapezoid, parallelogram, equilateral triangle, right triangle), and end termination (feather, overshot, hinge, retouched, or unclear).
For the platform angles, we followed Dibble and Whittaker (1981: 286), who define IPA as “the angle formed between the platform surface and a line through the point of percussion to the base of the bulb” and EPA as “measured at the intersection of the platform surface and the exterior surface of the core” (Supplementary Figure S3).
Blanks produced from prepared cores are predetermined items that were detached from prepared cores. It seems that these blanks were not produced for the purpose of shaping or maintaining the core; we thus view them as the desired end-products of the knapping process. They usually exhibit a larger IPA than the regular flakes, and their striking platforms are usually shaped, faceted, or dihedral. Their dorsal scar pattern varies, but it is either centripetal or unidirectional. If unidirectional, it differs from the scar pattern that we see in these sites for the regular flakes. These items were retrieved from all other categories (shaped and unshaped items) following the identification of particular characteristics (shaped striking platform, scar pattern, curvature, and so forth). Items detached from prepared cores were analyzed according to the following parameters: metric measurements (including thicknesses in the bulb area, middle and end of the flake), scar pattern and number of scars, platform preparation type, IPA and EPA, and end termination.
(Supplementary Figure S4): The major attributes and metrics of the proto-Levallois cores are presented in Table 1. All cores exhibit full/partial bifacial shaping of their circumference, delineating the plane of intersection, and a clear hierarchy between the striking platform and the production surface. Scars on the production surface reflect blanks removed in parallel to the plane of intersection. Cores were exploited mostly in the centripetal (Figures 2A–C; Figures 3A–F) or preferential (Figures 2D–E; Figures 3G–H) methods and less frequently unidirectionally or bidirectionally. Core convexities were maintained either by removing éclats débordants items or, alternatively, by shaping the entire circumference of the core or part of it. Scars indicating éclats débordants removals were observed on half of the cores (Figures 2A,D,E; Figures 3C,E,F,H). Scars indicating shaping of the entire circumference were observed on some of the Revadim and Jaljulia cores, and scars indicating shaping of part of the circumference were observed on most of the cores in both sites. Removals originating from the production surface towards the striking platform following the production of desired end products are evident in quarter of the Revadim proto-Levallois cores and one-third of the Jaljulia cores (Figure 3E). This phenomenon is not, by strict Levallois definition, an integral part of the method. We believe these removals could reflect some degree of striking platform maintenance between core production cycles and/or an attempt to produce additional blanks after production of the predetermined blanks. In any case, this subsequent stage of flake detachment distinguishes these late Acheulian proto-Levallois cores from MP Levallois cores.
FIGURE 2. Proto-Levallois centripetal (A–C) and preferential (D,E) cores from Revadim. Note the scars on the production surfaces indicating débordant removals (A,D,E). (E) Note the extremely small size of the core. The striking platform is shown in the left part of each figure.
FIGURE 3. Proto-Levallois centripetal (A–F) and preferential (G–H) cores from Jaljulia. Note the scars on the production surfaces indicating débordant removals (C,E,F,H). The striking platform is shown in the left part of each figure.
The cores were shaped from nodules, pebbles/cobbles, old cores\handaxes, flakes, or undetermined items (Table 1). Cases where the blank was defined as a former core\handaxe reflect the recycling of old items into cores. The knappers of both sites occasionally selected and collected old items, in these cases old cores or handaxes covered with patina, and used them as blanks for proto-Levallois cores. This was determined based on patina differences between the two stages of use, differences in scar morphology between the stages, or both.
The Revadim proto-Levallois cores show an average of eight removal scars on the production surface and nine on the striking platform, whereas the Jaljulia cores show an average of 11 removal scars on the production surface and 13 on the striking platform. Failed removals are visible both on the production surface and on the striking platform. Scars on the production surface that cross the midline are visible on most coresin both sites. Cortex is rarely present and is restricted mostly to the striking platform (Table 1). Only one Revadim core and four Jaljulia cores show some cortex on the production surface.
Flint quality for these cores varied at both sites. A significant number in both sites are made of homogeneous flint and the rest are fairly homogeneous or heterogeneous. As for texture, some of the cores are made from fine-grained flint, while the rest are medium- or coarse. Nevertheless, obstacles visible in the flint that might have been the reason for core abandonment are evident in only two of the Revadim cores and four of the Jaljulia cores.
Metric measurements show that the Jaljulia proto-Levallois cores are larger and produced larger items than the Revadim cores (Supplementary Table S4). The extent of exploitation of the production surface of the cores is quite similar for both sites.
Discoid cores: The major attributes and metrics of the discoid cores from Revadim and Jaljulia are presented in Table 2. These cores either exhibit full\partial shaping of the circumference with no hierarchy between the two core platforms, also termed alternate cores (Figure 4; Figures 5A–F), or they exhibit a switchable hierarchy (Figure 5F). Most of the cores are the former type: the removals were alternated between the two surfaces in the course of the reduction sequence. The remaining cores were the latter type, exploited by switching between the platforms, as a striking platform became a production surface and vice versa. Core convexities were maintained by shaping the entire circumference of the core, or part of it. Éclats débordants a dos limité scars are visible on some of the cores (Figures 5A,F). These flakes were removed from the core in order to correct irregularities in the production surface morphology (Boëda et al., 1990). Cores were made of nodules, pebbles/cobbles, old cores\handaxes (Supplementary Figure S5), flakes, or undetermined items (Table 2).
The Revadim cores show an average of seven to eight removal scars on each surface and nine to ten for the Jaljulia cores. Failed removals (as defined above) are visible on both surfaces. Unlike classic MP discoid cores, some cores bear scars that cross the midline of the production surface. When cortex is present on the cores, it is found mostly on the striking platform. Some cores bear no cortex, some bear 1–24% cortex, and some 25–50% cortex. Flaked patinated surfaces, indicating a time lag between the two use cycles of the core, are visible on most of the Revadim cores but are rather rare at Jaljulia. Flint quality varied for both sites: some of the cores are made from homogeneous flint, while the rest are fairly homogeneous or heterogeneous in about half of the cores. As for texture, some of the cores are made of fine-grained flint, and the rest are medium-grained, in about half of the cores, or coarse. Nevertheless, disturbances in the flint that might have been the reason for core abandonment were observed in only four (16.6%) cases at Revadim and one case at Jaljulia.
The metric data in Supplementary Table S4 shows that the discoid cores are symmetrical, to some extent, in length and width, a property that results from the convexities having been treated around the periphery of the core. Discoid cores bear fewer éclats débordants a dos limité scars than the proto-Levallois cores (in which the débordant scars are of the type shown in Supplementary Figure S2A). In addition, as expected, discoid cores produced smaller items than the proto-Levallois cores and exploit the surface to a lesser degree. The degree of exploitation of discoid cores is similar for both sites (Revadim: 54%; Jaljulia: 55%).
The major attributes and metrics of these prepared cores are presented in Table 3 and Supplementary Table S4. These cores do not fully conform to the abovementioned proto-Levallois and discoid definitions, although they do exhibit two platforms, with or without hierarchy (Revadim: hierarchy, 100%; Jaljulia: hierarchy, 50%; without hierarchy, 50%), and a bifacially shaped ridge intersecting the platforms to a certain degree (usually not along the entire core circumference). These cores were reduced centripetally in half of the cores (Figure 6), preferentially, unidirectionally, bidirectionally, or in an unclear manner. Core convexities were maintained by shaping the entire circumference of the core, or part of it. Éclats débordants a dos limité scars are visible on one-third of the Revadim cores and half of the Jaljulia cores.
Cores show an average of eight removal scars on the production surface and seven on the striking platform in the Revadim case, and an average of eight removal scars on the production surface and 12.6 on the striking platform for Jaljulia. Failed removals are visible both on the production surface and on the striking platform. Almost half of the cores in Revadim and most of the cores in Jaljulia exhibit scars on the production surface that cross the midline of the surface. Cortex is rather rare and is present mainly on the striking platform. The Jaljulia cores bear less cortex than the Revadim cores (Table 3).
Flaked patinated surfaces, indicating a time lag between the two use cycles of the core, are rather rare.
Flint quality varied for both sites: about half of the cores are made from homogeneous materials, while the rest are fairly homogeneous or heterogeneous. As for texture, the majority of cores at both sites are made of finely textured flint while the rest are almost equally divided into medium or coarse texture (Table 3). However, it should be noted that flint quality appears to be the reason for abandonment in only one case at Revadim and one case at Jaljulia.
We additionally defined three roughout items from the Revadim assemblage as prepared cores: these are cores that might have been in the process of being shaped but were abandoned during quite early stages of the reduction sequence. For one item the reason for abandonment is not clear, while the other two exhibit scars resulting from unsuccessful removals that likely resulted in their abandonment. These cores bear a high percentage of cortex on both platforms and were not included in the analysis presented here. However, their presence supports our contention that prepared cores were indeed shaped and reduced on-site.
An analysis of the length and width of the production surfaces revealed no significant differences for the three core groups at Revadim. The length and width of the Jaljulia core production surfaces differ slightly, as proto-Levallois cores are a bit larger than discoid and prepared cores (general). The Revadim cores are slightly smaller than the Jaljulia cores, and all three core groups seem to represent the same size distribution (calculated by comparing the standard deviation to the average; see Supplementary Table S4) The picture for Jaljulia is more complex, as proto-Levallois cores are somewhat less standard in size (as can be seen by the broader statistical distribution) than the two other core groups in the assemblage.
The angle between the two core platforms displayed by the proto-Levallois cores from both sites is more acute than for the discoid cores (Figure 7), as expected from the core definitions. In addition, the angle between the two platforms is generally more acute for the Jaljulia than the Revadim cores. This might indicate more stringent technological standards when applying the Levallois concepts and mastery of the skills required. However, we believe that the skill level manifested in the different lithic trajectories most probably reflects decision-making for site-specific tasks rather than a more general level of capabilities and knowhow. This conclusion is based on preliminary analysis of bifaces from both sites, which revealed a heavier investment in workmanship at Revadim as opposed to Jaljulia (Zupancich et al., 2021). The much smaller difference between the minimum and maximum angles between the two surfaces, for all three core groups in the Jaljulia assemblage (Figure 7), might indicate high knapping skill level at the site for this line of production. We can reasonably assume that a small difference between the minimum and maximum angles is indicative of a well-prepared core that will promote fewer knapping mistakes. And vice versa, the larger difference between the minimum and maximum angles at Revadim might indicate that knapping was less meticulous, as reflected in flakes removed at unsuitable angles. However, in the case of the proto-Levallois cores, the asymmetrical angles between the two platforms at the lateral and proximal edges, mostly when débordant removals were applied, might complicate our ability to fully interpret these angle differences.
FIGURE 7. Distribution of minimal and maximal angles between two core platforms for each method at both sites.
Regarding the proto-Levallois cores, at Jaljulia a greater number were made of undetermined blanks than at Revadim (Table 1), a difference possibly related to the rock types available in the vicinity of the two sites, which are characterized by different topographies.
The proto-Levallois cores from both assemblages were produced from more homogeneous and more finely textured flint than the discoid cores. However, the Jaljulia proto-Levallois cores show higher percentages of finely textured material than do those from Revadim, and the discoid cores from Jaljulia were produced from more homogeneous and finely textured flint than were the Revadim discoid cores. Thus, the Jaljulia knappers might have placed greater emphasis on material selectivity.
When low quality flint bearing visible disturbances was used, attempts were made to overcome these obstacles, as can be seen on some of the cores and CTEs. For example, some CTEs bear signs of flint disturbances on the dorsal face of the flake, suggesting that it was removed to get rid of the obstacle and allow further knapping (e.g., Figure 9C). A detailed study, including petrographic analysis of prepared core flint types from Jaljulia, further supports the trend we identified here that homogeneous flint was more common in the proto-Levallois cores than in the discoid cores (Agam et al., submitted).
To evaluate patterns of flint selectivity, we further compared the proto-Levallois cores from Revadim to a sample of ∼300 regular one/two striking platform cores from Revadim layer C3 (Table 4). Flint type analysis of the Jaljulia regular cores is not yet available, so this analysis was conducted for the Revadim assemblage only at this stage. The results indicate a preference for coarse-grained flint for both the proto-Levallois and discoid cores, in contrast to the sample of regular cores, where the preference is for finely textured, and medium textured flint. Whether the Revadim knappers preferred specific characteristics of flint homogeneity and texture for the production of prepared cores will be further evaluated in the future using a larger sample from both sites.
TABLE 4. Distribution of flint quality for one and two striking platform cores at Revadim layer C3 as compared to prepared cores from the Revadim assemblages used in our study.
While the Jaljulia cores very infrequently exhibit patinated surfaces, the Revadim cores were more frequently produced from “old” patinated cores/blanks in more than one life cycle, for all core groups. This phenomenon was recently documented for the site of Gesher Benot Ya’aqov as well (Goren-Inbar et al., 2018: 231). Although we did identify the presence of these two life cycles, we could not further ascertain whether these items represented a former handaxe or a former core. In any case, whether their original function changed (from handaxe to core) or remained the same (old core to new core), this phenomenon indicates recycling of old items (Vaquero et al., 2015; Lemorini et al., 2016; Agam and Barkai, 2018a; Efrati et al., 2019; Venditti et al., 2019a).
The small number of patinated cores in our Jaljulia sample is also striking when compared to the general sample of cores of all categories from area B at Jaljulia (Bar Efrati, personal communication). In the general sample (n = 503), patinated cores are well represented (22.07%; n = 111), in sharp contrast to the prepared core category, where patination was observed on only 2.2% of the proto-Levallois cores and 11.4% of the discoid cores. The difficulty of shaping a prepared core from an old core might explain this phenomenon, whereas simple flake removal could have been executed with much less effort from an old patinated core.
Moreover, the rather meticulous shaping process required to produce a prepared core would necessitate the use of a fairly large nodule/chunk in order to allow the removal of a significant number of shaping flakes. An old shaped core would probably not have been suitable for the initiation of this shaping process. The exception to this rule is the use of “old”, sometime patinated, handaxes as blanks for the production of predetermined blanks. As has been previously demonstrated, the knappers of Revadim and Jaljulia took advantage of handaxe convexities as a “shortcut” in the reduction sequence, enabling the detachment of predetermined blanks with minimal preparatory steps (Rosenberg-Yefet et al., 2021) while simultaneously shaping prepared cores anew from fresh nodules/chunks. This flexibility on the part of the knappers further highlights the importance of this technological trajectory and the level of knowhow and intentionality practiced by early humans during late Acheulian times in the Levant. We wish to emphasize that systematic lithic recycling in the late Lower Paleolithic has been demonstrated time and again for the production of small sharp flakes from “parent” flakes (Barkai et al., 2010; Agam et al., 2015; Lemorini et al., 2015; Parush et al., 2015; Agam and Barkai, 2018a; Venditti et al., 2019a; Venditti et al., 2019b), as well as the transformation of handaxes into cores (DeBono and Goren-Inbar, 2001; Marder et al., 2006; Shimelmitz, 2015; Akhilesh et al., 2018; Rosenberg-Yefet and Barkavi, 2019), and the use of old patinated flaked items for the production of new items such as cores and tools (Vaquero et al., 2015; Lemorini et al., 2016; Agam and Barkai, 2018a; Efrati et al., 2019). The evidence presented here thus adds to our growing knowledge regarding the role of lithic recycling in late Lower Paleolithic assemblages while also emphasizing inter site variability, as the Jaljulia prepared cores were not part of this intriguing behavioral pattern.
The major attributes and metrics of the CTE assemblages from Revadim and Jaljulia are presented in Table 5 and Supplementary Table S5.
TABLE 5. Major attributes of the two CTE types from both sites. Prefer. (Preferential); Rec. (Recurrent); centri. (centripetal); uni. (unidirectional); bidi. (bidirectional); Undeter. (Undetermined).
Éclats débordants (Revadim: n = 11; Jaljulia: n = 20): This group was further divided into éclats débordants (Figures 8A–D; Figures 9A,B), éclats débordants a dos limité (Figure 9D), and outrepassés (Figure 8E; Figure 9C). Due to the small sample size of each group, the analysis below will be presented for all sub-types together. Most items are characterized by a centripetal scar pattern on the dorsal face while the minority are unidirectional or bidirectional, with an average of seven scars on the dorsal face for Revadim and 5.3 for Jaljulia. The items have quite similar average EPA (Revadim: 82°; Jaljulia: 83.1°), reflecting the angle between the two platforms of the core from which they were removed, and an average IPA of 112° for Revadim and 105.6° for Jaljulia, indicating the angle of removal of the items. Variability in striking platform preparation was also noted, as some are plain, punctiform, faceted, dihedral, linear, or undetermined. At Revadim, only one item was removed from the right lateral part of the core, while the rest were removed from the left lateral part of the core (Figure 10A). At Jaljulia, most items were removed from the right lateral part of the core, while only a few were removed from the left lateral part of the core (Figure 10B). The average angle of the ridge removed from the core is quite similar for both sites (Revadim: 62.7°; Jaljulia: 67.7°). The EPA and ridge angle both mirror the original core circumference at different locations on the core. The difference between the EPA and the ridge angle is relatively large (about 20°), which might indicate a calculated decision on the part of the knapper as to where to strike the blow, even for centripetal cores that seem at first glance to be rounded and symmetrical. The cross sections of most items are obtuse triangles, while the rest are isosceles trapezoids, right triangles, parallelograms, or undetermined. The end terminations of the items vary between overshot (Figure 8E; Figure 9C), feather (Figures 8A,C; Figure 9A), hinge (Figures 8B,D), and broken\undetermined. (See Figure 9D).
FIGURE 8. Éclats débordants CTEs from Jaljulia. The bulb of percussion is highlighted by a blue line. (A–D) Éclats débordants; (E) Outrepassé. Note that for this item, not only has the lateral ridge of the core been removed but the distal one as well.
FIGURE 9. (A,B) Éclats débordants CTEs from Revadim. (C) Outrepassé CTE from Revadim. Note that this item may have been removed from the core in order to overcome obstacles in the flint; (D) Éclats débordants a dos limité. The bulb of percussion is highlighted by a black line.
FIGURE 10. Prepared core CTEs and débordant items from Revadim (A) and Jaljulia (B), showing the location of removal in relation to the core circumference. Note that the core is shown for illustrative purposes only.
Prepared core CTEs (Revadim: n = 10; Jaljulia: n = 40): Most items bear a centripetal scar pattern on the dorsal face while the minority are unidirectional or bidirectional, with an average of six scars on the dorsal face for Revadim and 5.6 for Jaljulia. The items have quite similar average EPA (Revadim: 73°; Jaljulia: 78.7°), reflecting the angle between the two platforms of the core from which they were removed, and an average IPA of 111° for Revadim and 110.1° for Jaljulia, indicating the angle of removal of the items. Variability in striking platform preparation was also noted, as some are plain, punctiform, faceted, dihedral, linear (or undetermined. At Revadim, half of the items were removed from the left lateral part of the core and 40% (n = 4) from the right lateral part of the core (Figure 10A); the remaining 10% were removed from the proximal end. Similarly, at Jaljulia, about half of the items were removed from the left lateral part of the core, and the other half from the right lateral part of the core (Figure 10B); the remaining 5% were removed from the proximal end. The average angle of the ridge removed from the core is quite similar for both sites (Revadim: 70°; Jaljulia: 66.1°). Here, too, the large difference between the EPA and the ridge angle might indicate a deliberate decision as to where to strike the core, as explained above. Most items have an obtuse triangular cross section, while the rest consist of a few different cross section types (Table 5). The end terminations of the items vary, but the most common are overshot, and hinge (Table 5).
The frequency of blanks produced from prepared cores differs significantly for the two sites (Jaljulia, n = 99; Revadim, n = 8). Since the number of items from Revadim is very small, quantitative analysis is not possible and thus the data will be presented separately for each site.
The majority of items exhibit a centripetal dorsal scar pattern (n = 6) while two of the items exhibit a unidirectional scar pattern, all having 7–8 scars on the dorsal face. The flakes have plain (n = 5), faceted (n = 2) or dihedral (n = 1) striking platforms with an average IPA of ca. 110° and EPA of ca. 83.7°. Flake terminations are feather (n = 3), overshot (n = 1), hinge (n = 1), retouch (n = 1), or undetermined (n = 2). Flakes are 4 cm long, 3.2 cm wide, and 0.5 cm thick on average.
FIGURE 11. (A,B) Prepared cores flakes from Revadim. The bulb of percussion is highlighted by a blue line. Jaljulia blanks produced from prepared cores (n = 99).
The major attributes and metrics of blanks produced from prepared cores from Jaljulia are presented in Table 6, Supplementary Table S6, and Figure 12.
TABLE 6. Major attributes of blanks produced from prepared cores, from Jaljulia. Prefer. (Preferential); Rec. (Recurrent); Centri. (centripetal); Uni. (unidirectional); Bidi. (bidirectional); Undeter. (Undetermined); En chapeau. (En chapeau de gendarme).
FIGURE 12. (A–D) Blanks produced from prepared cores, from Jaljulia. The bulb of percussion is highlighted by a blue line.
Items usually exhibit a centripetal dorsal scar pattern (62.6%, n = 62) or otherwise unidirectional (31.3%, n = 31), with an average of 6.1 scars on the dorsal face. Flakes are characterized by an IPA of ca. 112.3°. Most flakes have a faceted striking platform, while the rest are plain, dihedral or less frequently represented, and their average EPA is ca. 79.3°. The end terminations of the items vary between feather, hinge, retouched, and undetermined. Half of the flakes are uniform in thickness, while 40% are irregular. Of those, all are thicker at the base and thinner at the distal end. The rest of the flakes are moderately uniform or broken.
We analyzed the techno-typological characteristics of prepared cores and blanks originating from four layers of Revadim and one excavation area from Jaljulia, including all observable aspects of the core reduction sequence. Three sub-types of prepared cores were defined at Revadim and Jaljulia: prepared cores (general), discoid cores, and proto-Levallois cores. Proto-Levallois and prepared cores (general) adhere to concepts characteristic of the Levallois method. We thus believe that the production of predetermined blanks in the late Acheulian should be viewed as a precursor of the fully-fledged Levallois method of the Middle Paleolithic Mousterian.
Proto-Levallois and Mousterian Levallois cores bear many similarities, the most important of which is the shaping into two hierarchical surfaces. Additionally, both relied on the preparation of core convexities to produce predetermined blanks, on the creation of a ridge intersecting the two core surfaces acting as a striking platform, and on the removal of predetermined blanks in parallel with the plane of intersection. Moreover, in both cases, the cores were maintained by systematically removing éclats dèbordants flakes in order to control core convexities. This evidence suggests that the conceptualization of predetermined blank production—what has come to be known as the Levallois reduction concept—first emerged during late Acheulian times in the Levant.
Discoid cores are considered here as part of the parcel of prepared core technologies and are mostly characterized by a lack of hierarchy between core platforms (or having a switchable hierarchy), and by the removals of flakes at a typical obtuse angle. The discoid cores represented here differ somewhat from the classic Middle Paleolithic discoid cores, as a significant number of the scars cross the core midline, a feature not typical of later discoid cores. Prepared core CTEs, not commonly represented in Lower Paleolithic sites in the Levant, further add to our understanding of the complete reduction sequence. Prepared cores (general) reflect, in our view, a less distinctive technological procedure than the proto-Levallois and discoid. The technological analysis presented above suggests that this reduction sequence is predetermined, albeit less systematically, than the other two methods. A thin line separates this group of cores from the proto-Levallois ones. It is possible that cores defined by us as prepared cores (general) actually represent less successful attempts to execute the proto-Levallois method. Moreover, many of the CTEs defined by us as prepared core CTEs resemble débordant items, and were not classified as such only because they do not precisely fit the definition. With regard to the previously discussed difficulty of correctly identifying a variant that is on the periphery of a particular technology, the definition of this group as distinct from the proto-Levallois and discoid cores is intended as a possible methodological solution for such cases. We thus argue that proto-Levallois and prepared cores (general) should be viewed as a technological and conceptual continuum, reflecting early stages of the assimilation and adoption of predetermined blank production in the late Lower Paleolithic Levant.
The Revadim and Jaljulia cores were meticulously designed by both preparation removals and débordant removals, intended to facilitate the later production of desired predetermined items. The proto-Levallois reduction sequence is generally similar at both sites, excluding some differences that will be mentioned below. Notably, the proto-Levallois reduction sequence we defined here has been documented in other late Acheulian sites in the Levant and beyond (Gilead and Ronen, 1977; Goren, 1979; Ronen et al., 1980; Goren-Inbar, 1985; Chazan, 2000; DeBono and Goren- Inbar, 2001; Goren-Inbar, 2011; Shimelmitz et al., 2016; Zaidner and Weinstein-Evron, 2016; Goren-Inbar et al., 2018), and it thus can be seen as a hallmark of the late Acheulian lithic repertoire. In this paper we present the first comprehensive and detailed analysis of this intriguing phenomenon.
Although prepared cores and blanks represent a small percentage of the core and blank assemblages at both sites (Revadim: Agam et al., 2015; Solodenko 2010; Rosenberg-Yefet and Barkai 2019), we believe that their ubiquitous presence in every lithic assemblage at these two late Acheulian sites reflects the habitual use of prepared core technologies during this period in the Levant.
Proto-Levallois cores were more intensively shaped than discoid and prepared cores (general), as can be construed from the number of scars, scars representing débordant items, and cortex coverage. Discoid cores, however, were more systematically shaped around the circumference of the cores than the proto-Levallois cores, a difference that might be explained by their having been reduced along the entire core circumference, whereas reduction in proto-Levallois cores tended to be more restricted. The larger number of éclats débordants removals observed on the proto-Levallois cores might also explain this difference, as they might have spared the knapper the need to shape the entire circumference by means of bifacial flaking. Shaping the convexities of proto-Levallois cores by éclats débordants removals requires fewer bifacial blows at the lateral edges of the cores, as is common in Mousterian assemblages as well (Beyries and Boëda, 1983; Geneste, 1985:230; Meignen, 1995:280; Hovers, 2009:82; Shimelmitz and Kuhn, 2013; Hu et al., 2019). The use of débordant removals in order to design and maintain prepared cores in late Acheulian assemblages requires both expertise and an understanding of the blow direction, angle of striking, and precise use of force. The appearance of this technique as early as the late Acheulian demonstrates that knappers had the cognitive capabilities to master this distinctive and complex maintenance procedure long before its widespread adoption during Middle Paleolithic times.
The CTEs, included as part of our analysis of the entire reduction sequence and all its components, provide a glimpse into the initial shaping and maintenance stages of prepared cores: pre-planned core design and the strict adherence to concepts related to core volume and convexities. The presence of éclats débordants clearly demonstrates similarities in core design and maintenance with the fully-fledged Middle Paleolithic Levallois method, while éclats débordants a dos limité can be assigned, in the assemblages analyzed in this paper, to either the Levallois or the discoid methods.
Given the data presented above, the entire reduction sequence of prepared cores appears to be better represented in the Jaljulia sample than in the Revadim sample. This is reflected in the more frequent occurrence of both CTEs and blanks related to these core methods. Nevertheless, we have little doubt that the CTE items associated with prepared cores at Revadim also attest to the execution of core preparation and maintenance related to the Levallois concept. The high frequency of items characterized as éclats débordants in layer C3 East at Revadim, an assemblage analyzed by Malinsky-Buller et al. (2011), is noteworthy in this regard. The assemblage includes 209 éclats débordants and 56 outrepassés. Prepared cores (n = 61), or, in the authors’ terminology—cores with two surfaces perpendicular to each other with hierarchy—comprise 13% of all the cores in area C East. Intra-site variability in the application and intensity of prepared core technologies is of course to be expected, but nor can one disregard the presence of prepared core technologies at every single archaeological context at Revadim. We hope to gain a clearer understanding of intra-site variability once detailed analysis of all the areas at Jaljulia is complete.
The ridge angles of the éclats débordants items (Revadim: 62°; Jaljulia: 67°) differ significantly from the EPA angles (Revadim: 82°; Jaljulia: 83°). These angular differences reflect the asymmetry in the two platforms along the core circumference, a feature that generally characterizes proto-Levallois rather than discoid cores. Éclats débordants might thus have been detached mostly from the former type.
Inter-site variability can also be observed in the striking platforms of the éclats débordants items from the two sites. Whereas the Jaljulia items frequently exhibit faceted and dihedral striking platforms (éclats débordants: faceted, 45%; dihedral, 15%), these features are less frequent at Revadim (eclats débordants: faceted, 18%; dihedral, none). Even fewer of these faceted striking platforms (14.5%) were observed for the éclats débordants items in layer C3 East (Malinsky-Buller et al., 2011). In Mousterian sites, faceted or dihedral striking platforms are usually better represented (for example at Tabun; see Shimelmitz and Kuhn, 2013). The greater number of faceted striking platforms at Jaljulia is correlated with the more intensive treatment of convexities along the proto-Levallois core circumference (Tables 1, 4). The larger number of CTE items as well as the more intensive shaping of their striking platforms at Jaljulia might indicate greater assimilation of the Levallois concept there than at Revadim. The removal of these items was not symmetrical, as éclats débordants were repeatedly struck from one edge of the core: 81.6% were removed from the left lateral side at Revadim (Figure 10A) and 65% from the right lateral side at Jaljulia (Figure 10B). This asymmetrical treatment of core convexities is also common in other Middle Paleolithic Levallois assemblages in the Levant (Shimelmitz and Kuhn, 2013) and might reflect some technological continuity between late Acheulian proto-Levallois to the Middle Paleolithic Levallois.
As for blanks produced from prepared cores, the small number of these items at Revadim precludes a comparison between the two sites. Their infrequency is perhaps due simply to the difficulty in identifying these items. In this study, no distinction was made between proto Levallois flakes, discoid flakes and prepared cores (general) flakes. We are currently developing the methodology required to distinguish between these categories, to be published in future study. In a recent paper, González-Molina et al. (2020) used machine learning algorithms to address the challenges of distinguishing between discoid and Levallois flakes. Width at 50 and 75% of the flake length, inner angle, and maximum width were found to be the best parameters for accurate classification. The large number of faceted platforms in the Jaljulia assemblage is documented in many other Levallois assemblages, for example, at the Early Middle Paleolithic site of Misliya Cave, where convex faceted and “chapeau de gendarme” striking platforms are seen as diagnostic features of the Levallois method (Zaidner and Weinstein-Evron, 2020). These along with other factors will be considered in the future.
Changes in the Levantine habitat towards the end of the Lower Paleolithic might have played a role in the invention, adoption and assimilation of the Levallois method. The disappearance of elephants from the Levantine post-Acheulian landscape, marking the end of the Acheulian mode of adaptation, was probably a slow process, but one that must have had severe consequences for the Acheulian groups, who had lived in their presence for hundreds of thousands of years. The strong spiritual bond between early humans and elephants might be expressed in the production of replicas of the iconic handaxes from elephant bones, which seems to have accelerated towards the end of the Acheulian (Mussi, 2005; Costa, 2010; Zutovski and Barkai, 2016; Barkai, 2019; Barkai, 2021).
We suggest grouping together these separate lines of evidence into what seems to all be part of the same story: the assimilation and adoption of the Levallois method has its roots in the late Lower Paleolithic and might also have been driven by the disappearance of elephants from the Levantine habitat. This major habitat change may have created a “cultural storm” in which the adoption and assimilation of the Levallois method began in the late Acheulian, before the final extinction of the elephants, and became much more pronounced during the Middle Paleolithic. The AYCC, with its transition to the hunting and consumption of smaller prey, demonstrated a different kind of human adaptation and might well be part of the same story (e.g. Barkai et al., 2017).
The study of prepared core technologies at Revadim and Jaljulia provides an interesting observation point regarding the adoption and assimilation of technological innovations during Lower Paleolithic times. Technological innovations often involve a long and multi-participant process as the idea progresses and develops in what is known as “cumulative culture” (Tomasello, 1999; Hoppitt and Laland, 2013), also sometimes termed the ratchet effect (Tomasello, 1999; Tennie et al., 2009; Mesoudi, 2011). It has been argued that this accumulation of knowledge requires high-fidelity social learning mechanisms in order to allow the transmission of innovative ideas (Boyd and Richerson, 1988; Acerbi and Mesoudi, 2015; Tennie et al., 2016; Tennie et al., 2017). Some of us have previously argued that evidence for the existence of cumulative culture appears as early as the end of the Lower Paleolithic (Rosenberg-Yefet et al., 2021).
When considering the life cycle of a technological innovation, a few steps are generally expected: a slow inception, gradual assimilation of the technology, an increase in its frequency up to a certain peak, and then a decline, in an S curve representation, created by differences among individuals in their degree of “innovativeness” (although not all adoption curves are S-shaped) (Henrich, 2001). Five adopter categories are usually considered: innovators, early adopters, early majority, later majority, and laggards (Valente, 1996; Henrich, 2001). These differences between early and late adopters can create inter-individual variability regarding some technologies (Roux et al., 2018). This variability is expressed both in inter-group variability between individuals (Rogers, 2003; Correa, 2016; Assaf, 2021; Gandon et al., 2021) and, if we view the bigger picture in lower resolution, in inter-site variability along the chrono-geographical range. Many factors determine how innovative ideas and technologies are disseminated, including scales of mobility, population size, connectivity and networks, population stability, the presence of “weak ties”, defined as occasionally accessed connections (as opposed to “strong ties” such as family/kin), and environmental richness (Kuhn, 2012; Collar et al., 2015).
Given these high-resolution and low-resolution views of technological variability, we can see why a technology might be expected to differ in its initial versus peak phases. These differences will be expressed in the level of standardization and in the distinctiveness of the technology. Hence, we expect that some traits of a technology will be less standardized during the initial phases of adoption.
The Revadim and Jaljulia proto-Levallois assemblages reflect, in our opinion, an initial phase of technological adoption and assimilation. The partial correspondence of the proto-Levallois cores, CTEs, and prepared core end products to the Mousterian Levallois definition reveals the conceptual principles that will later define the technology at its peak phase.
The percentage of prepared cores among the total number of cores at Acheulian sites varies but remains a noteworthy phenomenon. At Berekhat Ram, for example, 64.8% of the cores are prepared (sample size is, however, restricted) (Goren-Inbar, 1985). At Holon, while only 3.2% of the cores are prepared, this percentage increases to 19.3% for cores with hierarchy, which might correspond to our definition of prepared cores) (Malinsky-Buller, 2014). At the different layers of Gesher Benot Yaʻaqov, 5.2–17.3% of the cores are prepared (Goren- Inbar et al., 2018). These are but a few of many examples of assemblages that might reflect early stages of adoption of the technology, as suggested for the Revadim and Jaljulia cases, and therefore can be seen as part of the chrono-geographical range of inter-site variability, mentioned earlier.
The early appearance of prepared cores at both sites signals, in our view, the inception of concepts related to the Levallois method, termed here proto-Levallois, in the late Acheulian Levant. The technological and conceptual characterization of the initial stage presented here reflect a broader behavioral model of adoption and assimilation of technological innovations during Lower Paleolithic times.
Scholars agree that the Levallois method reflects high technological proficiency, depth of planning, and structured cultural norms that were shared by Neanderthals, modern humans, and other human groups of the Middle Paleolithic period. In this study we showed that these capabilities were also within the reach of late Acheulian early humans, and that techniques for predetermined blank production, later to be associated with the Levallois, were invented, developed, and geographically dispersed during late Lower Paleolithic times in the Levant.
The prepared core assemblages from Revadim and Jaljulia reflect a significant step forward in terms of knapping organization, depth of planning, and successful predetermination of the items produced, considered by many to be unparalleled until the Middle Paleolithic, when the Levallois method took central stage. The Jaljulia area B assemblage represents a more advanced stage in the assimilation of the Levallois concept than the Revadim assemblage, as attested to by the cores, CTEs and blanks that we analyzed in our study, possible inter- and intra-site variability notwithstanding. Preliminary chronological data places area B at Jaljulia relatively late in the late Acheulian (Shemer et al., submitted) so chronology might also explain the more advanced implementation of predetermined production at this site. These assumptions will be further investigated in the future, as more absolute dates are determined for the two sites.
Our analysis of cores, CTEs, and end products quite clearly demonstrates that the early appearance of predetermined blank production via the Levallois method (referred to here as proto-Levallois) reflects a stage in the adoption, spread and assimilation of a new and significant technological innovation in the Old World, one that would be developed later into the Mousterian Levallois. The late Acheulian adoption of Levallois concepts is one of the most significant developments in the cultural evolution of Paleolithic knapping systems and, as such, a remarkable milestone in human cultural evolution.
The original contributions presented in the study are included in the article/Supplementary Material, further inquiries can be directed to the corresponding author.
TR-Y analyzed all lithic material, organized and analyzed database and wrote the manuscript. RB participated in data analysis and manuscript writing. MS excavated the Jaljulia site and participated in manuscript writing. All authors contributed to manuscript revision, read, and approved the submitted version.
This work was supported by Israel Science Foundation grant 321/19 entitled “Late Acheulean Jaljulia—A lithic perspective” and by the joint UGC–ISF Research Grant (Israel-India program) entitled “The First Global Culture: Lower Paleolithic Acheulean Adaptations at the Two Ends of Asia” 2712/16.
The authors declare that the research was conducted in the absence of any commercial or financial relationships that could be construed as a potential conflict of interest.
All claims expressed in this article are solely those of the authors and do not necessarily represent those of their affiliated organizations, or those of the publisher, the editors and the reviewers. Any product that may be evaluated in this article, or claim that may be made by its manufacturer, is not guaranteed or endorsed by the publisher.
We would like to express gratitude to our editor, Sharon Kessler, for providing useful comments to improve the paper. We also wish to thank Sasha Flit (TAU) for the photographs used in this article and Leore Grosman and Gadi Herzlinger for the 3D scans. We would like to specially thank Liliane Meignen for her help in guiding us through the lithic analysis of the Levallois-related assemblages.
The Supplementary Material for this article can be found online at: https://www.frontiersin.org/articles/10.3389/feart.2022.847358/full#supplementary-material
Acerbi, A., and Mesoudi, S. H. (2015). If We Are All Cultural Darwinians What’s the Fuss about? Clarifying Recent Disagreements in the Field of Cultural Evolution. Geophys. Res. Lett. 30 (4), 481–503. doi:10.1007/s10539-015-9490-2
Adler, I. V., Wilkinson, G. E., Blockley, S., Mark, D. F., Pinhasi, R., Schmidt-Magee, B. A., et al. (2014). Early Levallois Technology and the Lower to Middle Paleolithic Transition in the Southern Caucasus. Science. 153-154 (6204), 1609–1613. doi:10.1126/science.1256484
Agam, A., and Barkai, R. (2018a). Small Flake Acheulian: Further Insights into Lithic Recycling at Late 46 Acheulian Revadim, Israel. Tel Aviv. 45 (2), 170–192. doi:10.1080/03344355.2018.1494783
Agam, A., and Barkai, R. (2018b). Elephant and mammoth Hunting during the Paleolithic: a Review of the Relevant Archaeological, Ethnographic and Ethno-Historical Records. Quaternary. 1 (1), 3. doi:10.3390/quat1010003
Agam, A., Marder, O., and Barkai, R. (2015). Small flake production and lithic recycling at Late Acheulian Revadim, Israel. Quaternary International. 361, 46–60. doi:10.1016/j.quaint.2014.06.070
Akhilesh, K., Pappu, S., Rajapara, H. M., Gunnell, Y., Shukla, A. D., and Singhvi, A. K. (2018). Early Middle Palaeolithic Culture in India Around 385–172 Ka Reframes Out of Africa Models. Nature. 554 (7690), 9–101. doi:10.1038/nature25444
Ambrose, S. (2001). Paleolithic Technology and Human Evolution. Science. 291, 1748–1753. doi:10.1126/science.1059487
Assaf, E. (2021). Dawn of a New Day: The Role of Children in the Assimilation of New Technologies throughout the Lower Paleolithic. L'Anthropologie. 125 (1), 102836. doi:10.1016/j.anthro.2021.102836
Baena, S. X., Moncel, M. H., Cuartero, F., Navarro, M. G. C., and Rubio, D. (2017). Late Middle Pleistocene Genesis of Neanderthal Technology in Western Europe: The Case of Payre Site (South-east France). Heat Mass. Transfer. 436, 212–238. doi:10.1016/j.quaint.2014.08.031
Bahain, Y., Mercier, C., Valladas, H., Falguères, C., Masaoudi, H., Joron, J. L., et al. (2022). Reappraisal of the Chronology of Orgnac 3 Lower-To Lower to Middle Paleolithic Site (Ardèche, France), a Regional Key Sequence for the Middle Pleistocene of Southern France. J. Hum. Evol. 162, 103092. doi:10.1016/j.jhevol.2021.103092
Bar-Yosef, O., and Belfer-Cohen, A. (2013). Following Pleistocene Road Signs of Human Dispersals across Eurasia. Quat. Int. 285, 30–43. doi:10.1016/j.quaint.2011.07.043
Bar-Yosef, O., and Belmaker, M. (2010). Early and Middle Pleistocene Faunal and Hominins Dispersals through Southwestern Asia. Quat. Sci. Rev. 30, 1318–1337. doi:10.1016/j.quascirev.2010.02.016
Barkai, R. (2019). “An Elephant to Share: Rethinking the Origins of Meat and Fat Sharing in Paleolithic Societies,” in Towards a Broader View of Hunter Gatherer Sharing. Editors N. Lavi,, and D. E. Friesem (Cambridge: McDonald Institute for Archaeological Research), 153–167.
Barkai, R., and Gopher, A. (2013). “Cultural and Biological Transformations in the Middle Pleistocene Levant: a View from Qesem Cave, Israel,” in Dynamics of Learning in Neanderthals and Modern Humans. EditorsT. Akazawa, Y. Nishiaki, and K. Aok (Tokyo: Springer), 1, 115–137. doi:10.1007/978-4-431-54511-8_7
Barkai, R., Lemorini, C., and Gopher, A. (2010). Palaeolithic Cutlery 400 000–200 000 Years Ago: Tiny Meat-Cutting Tools from Qesem Cave, Israel. Antiquity. 84 (325).
Barkai, R., Rosell, J., Blasco, R., and Gopher, A. (2017). Fire for a Reason. Curr. Anthropol. 58 (S16), S314–S328. doi:10.1086/691211
Barkai, R. (2021). The elephant in the handaxe: Lower Palaeolithic ontologies and representations. Cambridge Archaeological Journal, 31 (2), 349–361. doi:10.1017/S0959774320000360
Ben-Dor, M., Sirtoli, G. R., and Barkai, S. C. (2021a). The Evolution of the Human Trophic Level during the Pleistocene. Am. J. Phys. Anthropol. 175, 27–56. doi:10.1002/ajpa.24247
Ben-Dor, G. S., Barkai, Z. Y., Konidaris, X. Z., Barkai, C. F., Tourloukis, J. C., and Hvarti, L. W. (2021b). Supersize Does Matter: The Importance of Large Prey in Palaeolithic Subsistence and a Method for Measuring its Significance in Zooarchaeological Assemblages. Tübingen: Tübingen University Press, 323–348.
Ben-Dor, M., and Barkai, H. (2021). Prey Size Decline as a Unifying Ecological Selecting Agent in Pleistocene Human Evolution. Quaternary. 4(1), 7.doi:10.3390/quat4010007
Ben-Dor, M., and Barkai, R. (2020). The Importance of Large Prey Animals during the Pleistocene and the Implications of Their Extinction on the Use of Dietary Ethnographic Analogies. J. Anthropological Archaeology. 59, 101192. doi:10.1016/j.jaa.2020.101192
Beyries, S., and Boëda, E. (1983). Étude technologique et traces d'utilisation des éclats débordants de Corbehem (Pas-de-Calais). Bull. de la Société préhistorique française. 80-9, 275–279. https://www.jstor.org/stable/27918716
Boaretto, E., Hernandez, W. J., Goder-Goldberger, J. W., Aldeias, V., Regev, L., Caracuta, V., et al. (2021). The Absolute Chronology of Boker Tachtit (Israel) and Implications for the Middle to Upper Paleolithic Transition in the Levant. Proc. Natl. Acad. Sci. 118 (25), 86. doi:10.1073/pnas.2014657118
Boëda, E. (1993). Le débitage discoïde et le débitage Levallois récurrent centripède. Bull. de la Société préhistorique française. 90 (6), 392–404. doi:10.3406/bspf.1993.9669
Boëda, E. (1995). “Levallois: a Volumetric Construction, Methods, a Technique,” in The Definition and Interpretation of Levallois Technology. Editer. H. L. Dibble, and O. Bar-Yosef. (Madison: Prehistory Press), 41–65.
Boëda, E., Geneste, J. M., and Meignen, L. (1990). Identification de chaînes opératoires lithiques du Paléolithique ancien et moyen. Paléo. 2 (1), 43–80. doi:10.3406/pal.1990.988
Bordes, F. (1971). Physical Evolution and Technological Evolution in Man: a Parallelism. World archaeology. 3 (1), 1–5. https://www.jstor.org/stable/124199
Boschian, G., Caramella, D., Saccà, D., and Barkai, R. (2019). Are there marrow cavities in Pleistocene elephant limb bones, and was marrow available to early humans? New CT scan results from the site of Castel di Guido (Italy). Adv. Geo-energy Res. 215, 86–97. doi:10.1016/j.quascirev.2019.05.010
Boyd, R., and Richerson, P. J. (1988). The Evolution of Reciprocity in Sizable Groups. J. Theor. Biol. 132 (3), 337–356. doi:10.1016/s0022-5193(88)80219-4
Brantingham, J., and Khun, S. (2001). Constraints on Levallois Core Technology: A Mathematical Model. J. Archaeological Sci. 28, 747–761. doi:10.1006/jasc.2000.0594
Bunn, H. T., and Gurtov, A. N. (2014). Prey Mortality Profiles Indicate that Early Pleistocene Homo at Olduvai Was an Ambush Predator. Quat. Int. 322-323, 44–53. doi:10.1016/j.quaint.2013.11.002
Bunn, H. T. (2019). Large Ungulate Mortality Profiles and Ambush Hunting by Acheulean-Age Hominins at Elandsfontein, Western Cape Province, South Africa. J. Archaeological Sci. 107, 40–49. doi:10.1016/j.jas.2019.04.002
Bunn, H. T., and Pickering, T. R. (2010). Bovid Mortality Profiles in Paleoecological Context Falsify Hypotheses of Endurance Running-Hunting and Passive Scavenging by Early Pleistocene Hominins. Quat. Res. 74, 395–404. doi:10.1016/j.yqres.2010.07.012
Carmignani, L., Moncel, M. H., Fernandes, P., and Wilson, L. (2017). Technological variability during the Early Middle Palaeolithic in Western Europe. Reduction systems and predetermined products at the Bau de l'Aubesier and Payre (South-East France). PLoS One. 12 (6), e0178550. doi:10.1371/journal.pone.0178550
Centi, T., and Zaidner, S. (2021). The Levallois Flaking System in Nesher Ramla Upper Sequence. J. Paleolithic Archaeology. 4 (2), 1–42. doi:10.1007/s41982-021-00088-3
Chazan, M. (1997). “Review of Le Concept Levallois: Variabilite des Methodes. by Boëda, E., The Levallois Reduction Strategy by P. van Peer, and The Definition and Interpretation of Levallois Technology,” in Journal of Human Evolution. Editors H. Dibble, and O. Bar Yosef, Vol. 33, 719–735. doi:10.1006/jhev.1997.0167
Chazan, M. (2000). Typological Analysis of the Lower Paleolithic Site of Holon, Israel. J. Israel Prehist. Soc. 30, 7–32.
Chazan, M. (2020). “Levallois,” in Oxford Research Encyclopedia of Anthropology. doi:10.1093/acrefore/9780190854584.013.28
Claud, E. (2008). Le statut fonctionnel des bifaces au Paléolithique moyen récent dans le Sud-Ouest de la France. Étude tracéologique intégrée des outillages des sites de La Graulet, La Conne de Bergerac, Combe Brune 2, Fonseigner et Chez-Pinaud/Jonzac. [Ph.D. Dissertation. Université Sciences et Technologies-Bordeaux I
Cohen, A. (2018). The Handaxes from Late Acheulian Revadim Quarry: Similarities and Differences in Time and Space. Tel-Aviv: University of Tel-Aviv. [MA dissertation].
Cole, J. (2015). Examining the Presence of Symmetry within Acheulean Handaxes: a Case Study in the British Palaeolithic. Cambridge Archaeological J. 25 (04), 713–732. doi:10.1017/S0959774315000141
Collar, A., Coward, T. S., Brughmans, T., and Mills, B. J. (2015). Networks in Archaeology: Phenomena, Abstraction, Representation. IEEE Trans. Power Deliv. 22 (1), 1–32. doi:10.1007/s10816-014-9235-6
Copeland, L. (1995). “Are Levallois Flakes in the Acheulean the Results of Biface Preparation?” in The Definition and Interpretation of Levallois Technology. Editors H. L. Dibble, and O. Bar-Yosef (Madison, Wisconsin: Prehistory Press), 171–183.
Correa, T. (2016). Acquiring a New Technology at home: A Parent-Child Study about Youths’ Influence on Digital media Adoption in a Family. J. Broadcasting Electron. Media. 60 (1), 123–139. doi:10.1080/08838151.2015.1127238
Costa, A. G. (2010). A geometric morphometric assessment of plan shape in bone and stone Acheulean bifaces from the Middle Pleistocene site of Castel di Guido, Latium, Italy. In New perspectives on old stones (pp. 23–41). Springer, New York, NY. doi:10.1007/978-1-4419-6861-6_2
de la Torre, I. (2010). “Insights on the Technological Competence of the Early Oldowan,” in Stone Tools and the Evolution of Human Cognition. Editors A. Nowell, and I. Davidson (University Press of Colorado).
Debénath, A., and Dibble, H. L. (1994). Handbook of Paleolithic Typology: Lower and Middle Paleolithic of Europe, 1. Philadelphia: University of Pennsylvania Museum of Archaeology and Anthropology, 23.
DeBono, H. N., and Goren-Inbar, N. (2001). Note: a Link between Acheulean Handaxes and the Levallois Method. Geophys. Res. Lett. 31, 9–23.
Dembitzer, B. Q., Barkai, Z. H., Ben-Dor, M., and Meiri, C. Y. (2022). Levantine Overkill: 1.5 Million Years of Hunting Down the Body Size Distribution. Int. J. Heat Mass Transfer. 276, 107316. doi:10.1016/j.quascirev.2021.107316
Dennel, R. (2011). An Earlier Acheulian Arrival in South Asia. Science. 331, 1532–1533. doi:10.1126/science.1203806
Despriée, H., Voinchet, Z., Tissoux, H., Moncel, M. H., Arzarello, M., Robin, S., et al. (2010). Lower and Middle Pleistocene Human Settlements in the Middle Loire River basin, Centre Region, France. Quat. Int. 223, 345–359. doi:10.1016/j.quaint.2009.07.019
Dibble, H. L., and Whittaker, J. C. (1981). New Experimental Evidence on the Relation between Percussion Flaking and Flake Variation. J. Archaeological Sci. 8 (3), 283–296. doi:10.1016/0305-4403(81)90004-2
Domínguez-Rodrigo, M., and Pickering, T. R. (2017). The Meat of the Matter: An Evolutionary Perspective on Human Carnivory. Azania: Archaeological Res. Africa. 52 (1), 4–32. doi:10.1080/0067270X.2016.1252066
Efrati, B., Parush, Y., Ackerfeld, D., Gopher, A., and Barkai, R. (2019). Seeing Colors. Mitekufat Haeven: J. Isr. Prehistoric Society 49, 32–62.
Eren, M. I., and Lycett, S. J. (2012). Why Levallois? A Morphometric Comparison of Experimental “preferential” Levallois Flakes versus Debitage Flakes. PLoS One. 7 (1), e29273. doi:10.1371/journal.pone.0029273
Eren, M. I., and Lycett, S. J. (2016). A Statistical Examination of Flake Edge Angles Produced During Experimental Lineal Levallois Reductions and Consideration of Their Functional Implications. J. Archaeological Method Theor., 1–20. doi:10.1007/s10816-015-9245-z
Finkel, M., and Barkai, R. (2021). Technological Persistency Following Faunal Stability during the Pleistocene: A Model for Reconstructing Paleolithic Adaptation Strategies Based on Mosaic Evolution. L'Anthropologie. 125 (1), 102839. doi:10.1016/j.anthro.2021.102839
Finkel, M., and Barkai, R. (2018). Acheulean handaxe technological persistence: a case of preferred cultural conservatism? In Proceedings of the Prehistoric Society 84, 1–19. Cambridge University Press. doi:10.1017/ppr.2018.2
Fontana, B., Moncel, J., Nenzioni, M., Onorevoli, G., Peretto, C., and Combier, J. (2013). Widespread Diffusion of Technical Innovations Around 300,000 Years Ago in Europe as a Reflection of Anthropological and Social Transformations? New Comparative Data from the Western Mediterranean Sites of Orgnac (France) and Cave dall’Olio (Italy). J. Anthropological Archaeology. 32 (4), 478–498. doi:10.1016/j.jaa.2013.08.003
Gandon, B., Nonaka, T., Coyle, T., Coyle, E., Sonabend, R., and Ogbonnaya, C. (2021). Cultural Transmission and Perception of Vessel Shapes Among Hebron Potters. J. Anthropological Archaeology. 63, 101334. doi:10.1016/j.jaa.2021.101334
Geneste, B. (1985). Analyse lithique d'industries moustériennes du Périgord: une approche du comportement des groupes humains au paléolithique moyen, 1. Bordeaux: Doctoral dissertation.
Gilead, B., and Ronen, J. (1977). Acheulian Industries from Evron on the Western Galilee Coastal plain. Eretz- Isr. 13, 56–86.
Goder-Goldberger, M. (2013). The Khormusan: Evidence for an MSA East African Industry in Nubia. Quat. Int. 300, 182–194. doi:10.1016/j.quaint.2012.11.031
González-Molina, I., Jiménez-García, B., Maíllo-Fernández, J. M., Baquedano, E., and Domínguez-Rodrigo, M. (2020). Distinguishing Discoidal and Centripetal Levallois Methods through Machine Learning. PLoS One 15 (12), e0244288. doi:10.1371/journal.pone.0244288
Gopher, A., Barkai, R., Shimelmitz, R., Khalaily, M., Lemorini, C., Heshkovitz, I., et al. (2005). Qesem Cave: an Amudian Site in central Israel. Mitekufat Haeven: J. Isr. Prehistoric Society 35, 69–92. https://www.jstor.org/stable/23383554
Goren-Inbar, N., Alperson-Afil, N., Sharon, G., and Herzlinger, G. (2018). The Acheulian Site of Gesher Benot Ya’aqov Volume IV: The Lithic Assemblages. New-York: Springer.
Goren-Inbar, N. (2011). “Behavioral and Cultural Origins of Neanderthals: A Levantine Perspective,” in Continuity and Discontinuity in the Peopling of Europe. One Hundred Fifty Years of Neanderthal Study. Editors S. Condemi, and G. C. Weniger (Springer Netherlands), 89–100. doi:10.1007/978-94-007-0492-3_8
Goren-Inbar, N., Lister, A., Werker, E., and Chech, M. (1994). A Butchered Elephant Skull and Associated Artifacts from the Acheulian Site of Gesher Benot Ya'aqov, Israel. paleo. 20, 99–112. doi:10.3406/paleo.1994.4604
Goren-Inbar, N. (1985). The Lithic Assemblage of the Berekhat Ram Acheulian Site, Golan Heights. paleo. 11 (1), 7–28. doi:10.3406/paleo.1985.4358
Groucutt, H. S., Scerri, E. M. L., Lewis, L., Clark-Balzan, L., Blinkhorn, J., Jennings, R. P., et al. (2015). Stone Tool Assemblages and Models for the Dispersal of Homo sapiens Out of Africa. Quat. Int. 382, 8–30. doi:10.1016/j.quaint.2015.01.039
Guil-Guerrero, J. L., Tikhonov, A., Ramos‐Bueno, R. P., Grigoriev, S., Protopopov, A., Savvinov, G., et al. (2018). Mammoth Resources for Hominins: from omega‐3 Fatty Acids to Cultural Objects. J. Quat. Sci. 33 (4), 455–463.
Guil-Guerrero, J. L., Tikhonov, A., Rodríguez-García, I., Protopopov, A., Grigoriev, S., and Ramos-Bueno, R. P. (2014). The Fat from Frozen Mammals Reveals Sources of Essential Fatty Acids Suitable for Palaeolithic and Neolithic Humans. PloS one. 9 (1), e84480. doi:10.1371/journal.pone.0084480
Gvirtzman, G., Wieder, M., Marder, O., Khalaily, H., Rabinovichm, R., and Ron, H. (1999). Geological and Pedological Aspects of an Early-Paleolithic Site: Revadim, central Coastal plain, Israel. Geoarchaeology. 14 (2), 101–126.
Haidle, M. N. (2010). Working‐Memory Capacity and the Evolution of Modern Cognitive Potential. Curr. Anthropol. 51 (S1), S149–S166. doi:10.1086/650295
Halfon, E., and Barkai, R. (2020). The Material and Mental Effects of Animal Disappearance on Indigenous hunter-gatherers, Past and Present. Time and Mind. 13 (1), 5–33. doi:10.1080/1751696X.2020.1718309
Harmand, S., Lewis, J. E., Feibel, C. S., Lepre, C. J., Prat, S., Lenoble, A., et al. (2015). 3.3-million-year-old Stone Tools from Lomekwi 3, West Turkana, Kenya. Nature. 521 (7552), 310–315. doi:10.1038/nature14464
Henrich, J. (2001). Cultural Transmission and the Diffusion of Innovations: Adoption Dynamics Indicate that Biased Cultural Transmission Is the Predominate Force in Behavioral Change. Am. Anthropologist. 103 (4), 992–1013. doi:10.1525/aa.2001.103.4.992
Hérisson, D., Brenet, M., Cliquet, D., Moncel, M. H., Richter, J., Scott, B., et al. (2016). The Emergence of the Middle Palaeolithic in north-western Europe and its Southern Fringes. Quat. Int. 411, 233–283.
Hérisson, D., and Soriano, S. (2020). A View of the Lower to Middle Paleolithic Boundary from Northern France, Far from the Near East? J. Hum. Evol. 145, 102814. doi:10.1016/j.jhevol.2020.102814
Hershkovitz, I., May, H., Sarig, R., Pokhojaev, A., Grimaud-Hervé, D., Bruner, E., et al. (2021). A Middle Pleistocene Homo from Nesher Ramla, Israel. Science. 372 (6549), 1424–1428. doi:10.1126/science.abh3169
Hoppitt, W., and Laland, K. N. (2013). Social Learning: An Introduction to Mechanisms, Methods, and Models. Princeton, NJ: Princeton University Press.
Hovers, E., and Belfer-Cohen, A. (2013). On Variability and Complexity. Curr. Anthropol. 54 (S8), S337–S357. doi:10.1086/673880
Hu, Y., Marwick, B., Zhang, J.-F., Rui, X., Hou, Y.-M., Yue, J.-P., et al. (2019). Late Middle Pleistocene Levallois Stone-Tool Technology in Southwest China. Nature. 565, 82–85. doi:10.1038/s41586-018-0710-1
Ingold, T. (2000). The Perception of the Environment. Essays in Livelihood, Dwelling and Skill. London: Routledge.
Jacobs, Z., Li, B., Shunkov, M. V., Kozlikin, M. B., Bolikhovskaya, N. S., Agadjanian, A. K., et al. (2019). Timing of Archaic Hominin Occupation of Denisova Cave in Southern Siberia. Nature. 565 (7741), 594–599. doi:10.1038/s41586-018-0843-2
Jiménez-Arenas, J. M., Santonja, M., and BotellaPalmqvist, M. P. (2011). The Oldest Handaxes in Europe: Fact or Artefact? J. Archaeological Sci. 38 (12), 3340–3349. doi:10.1016/j.jas.2011.07.020
Johnson, C. R., and McBrearty, S. (2012). Archaeology of Middle Pleistocene Lacustrine and spring Paleoenvironments in the Kapthurin Formation, Kenya. J. Anthropological Archaeology. 31 (4), 485–499. doi:10.1016/j.jaa.2012.05.001
Konidaris, G. E., and Tourloukis, V. (2021). “Proboscidea-Homo Interactions in Open-Air Localities during the Early and Middle Pleistocene of Western Eurasia: A Palaeontological and Archaeological Perspective,”. Editors G. E. Konidaris, R. Barkai, V. Tourloukis, and K. Harvati (Tübingen University Press: Tübingen), 67.
Kuhn, S. L. (2012). Emergent Patterns of Creativity and Innovation in Early Technologies. Dev. Quat. Sci. 16, 69–87. doi:10.1016/B978-0-444-53821-5.00006-3
Leader, G. M., Kuman, K., Gibbon, R. J., and Granger, D. E. (2018). Early Acheulean Organised Core Knapping Strategies Ca. 1.3 Ma at Rietputs 15, Northern Cape Province, South Africa. Quat. Int. 480, 16–28. doi:10.1016/j.quaint.2016.08.046
Lemorini, C., Bourguignon, L., Zupancich, A., Gopher, A., and Barkai, R. (2016). A Scraper's Life History: Morpho-Techno-Functional and Use-Wear Analysis of Quina and Demi-Quina Scrapers from Qesem Cave, Israel. Quat. Int. 398, 86–93. doi:10.1016/j.quaint.2015.05.013
Lemorini, C., Venditti, F., Assaf, E., Parush, Y., Barkai, R., and Gopher, A. (2015). The Function of Recycled Lithic Items at Late Lower Paleolithic Qesem Cave, Israel: an Overview of the Use-Wear Data. Quat. Int. 361, 103–112. doi:10.1016/j.quaint.2014.07.032
Lepre, C. J., Roche, H., Kent, D. V., Harmand, S., Quinn, R. L., Brugal, J.-P., et al. (2011). An Earlier Origin for the Acheulian. Nature. 477, 82–85. doi:10.1038/nature10372
Lewis, J. (2021). “BaYaka Elephant Hunting in Congo: the Importance of Ritual and Technique,”. Editors G. E. Konidaris, R. Barkai, V. Tourloukis, and K. Harvati (Tübingen: Tübingen University Press), 439–454.
Li, Y., Boëda, E., Forestier, H., and Zhou, Y. (2019). Lithic Technology, Typology and Cross-Regional Comparison of Pleistocene Lithic Industries: Comment on the Earliest Evidence of Levallois in East Asia. L'Anthropologie, 102728.
Lycett, S. J., and Eren, M. I. (2013). Levallois Economics: an Examination of "waste” Production in Experimentally Produced Levallois Reduction Sequences. J. Archaeological Sci. 40, 238. doi:10.1016/j.jas.2013.01.016
Lycett, S. J., and Gowlett, J. A. (2009). On Questions Surrounding the Acheulean ‘tradition. World Archaeology 40 (3), 295–315. doi:10.1080/00438240802260970
Lycett, S. J., von Cramon-Taubadel, N., and Eren, M. I. (2016). Levallois: Potential Implications for Learning and Cultural Transmission Capacities. Lithic Technology. 41 (1), 19–38. doi:10.1179/2051618515y.0000000012
Machin, A. (2009). The Role of the Individual Agent in Acheulean Biface Variability. J. Soc. Archaeology. 9 (1), 35–58. doi:10.1177/1469605308099370
Malinsky-Buller, A., Grosman, L., and Marder, O. (2011). A Case of Techno-Typological Lithic Variability & Continuity in the Late Lower Palaeolithic. Before Farming. 2011, 1–32. doi:10.3828/bfarm.2011.1.3
Malinsky-Buller, A. (2014). Contextualizing Curational Strategies at the Late Lower Paleolithic Site of Holon, Israel. PaleoAnthropology, 483,504. doi:10.4207/PA.2014.ART87
Malinsky-Buller, A. (2016). The Muddle in the Middle Pleistocene: The Lower-Middle Paleolithic Transition from the Levantine Perspective. J. World Prehist. 29 (1), 1–78. doi:10.1007/s10963-016-9092-1
Marder, O., Gvirtzman, G., Ron, H., Khalaily, H., Wieder, M., Bankirer, R., et al. (1999). The Lower Paleolithic Site Revadim Quarry, Preliminary Finds. J. Isr. Prehistoric Soc. 28, 21–53.
Marder, O. I., Milevski, A., and Matskevich, Z. (2006). “The Handaxes of Revadim Quarry: Typo-Technological Considerations and Aspects of Intra-site Variability,” in Axe Age Acheulian Tool-Making from Quarry to Discard. Editors N. Goren-Inbar,, and G. Sharon (London: Equinox), 223–242.
Marder, O., Malinsky-Buller, A., Shahack-Gross, R., Ackermann, O., Ayalon, A., Bar-Matthews, M., et al. (2011). Archaeological Horizons and Fluvial Processes at the Lower Paleolithic Open-Air Site of Revadim (Israel)☆. J. Hum. Evol. 60, 508–522. doi:10.1016/j.jhevol.2010.01.007
Mathias, C., Bourguignon, L., Brenet, M., Grégoire, S., and Moncel, M. H. (2020). Between New and Inherited Technical Behaviours: a Case Study from the Early Middle Palaeolithic of Southern France. Archaeological Anthropological Sci. 12 (7), 1–39. doi:10.1007/s12520-020-01114-1
Meignen, L. (2000). Early Middle Palaeolithic Blade Technology in Southwestern Asia. Acta Anthropologica Sinica. 19, 158–168.
Meignen, L. (2002). “Hayonim Cave Lithic Assemblages in the Context of the Near Eastern Middle Paleolithic,” in Neandertals and Modern Humans in Western Asia (Boston: Springer), 165–180.
Meignen, L. (1995). Levallois Lithic Production Systems in the Middle Paleolithic of the Near East: the Case of the Unidirectional Method. definition interpretation Levallois Technol. 23, 361–380.
Mesoudi, A. (2011). Variable Cultural Acquisition Costs Constrain Cumulative Cultural Evolution. PLoS One. 6 (3), e18239. doi:10.1371/journal.pone.0018239
Moncel, M.-H., Ashton, N., Arzarello, M., Fontana, F., Lamotte, A., Scott, B., et al. (2020). Early Levallois Core Technology between marine Isotope Stage 12 and 9 in Western Europe. J. Hum. Evol. 139, 102735. doi:10.1016/j.jhevol.2019.102735
Moncel, M.-H., Ashton, N., Lamotte, A., Tuffreau, A., Cliquet, D., and Despriée, J. (2015). The Early Acheulian of north-western Europe. J. Anthropological Archaeology. 40, 302–331. doi:10.1016/j.jaa.2015.09.005
Moncel, M.-H., Moigne, A.-M., and Sam, Y. (2011). “Technological Behavior and Mobility of Human Groups Deduced from Lithic Assemblages in the Late Middle and Early Late Pleistocene of the Middle Rhône Valley (France),” in Neanderthal Lifeways, Subsistence and Technology (London and New York: Springer), 261–287.
Muller, A., Clarkson, C., and Shipton, C. (2017). Measuring Behavioural and Cognitive Complexity in Lithic Technology throughout Human Evolution. J. Anthropological Archaeology. 48, 166–180. doi:10.1016/j.jaa.2017.07.006
Mussi, M. (2005). Hombres y Elefantes en las latitudes medias: una larga convivencia. Zona arqueológica. (5), 396–417.
Nowell, A., and White, M. J. (2010). “Growing Up in the Middle Pleistocene: Life History Strategies and Their Relationship to Acheulian Industries,” in Stone Tools and the Evolution of Human Cognition. Editors A. Nowell,, and I. Davidson (University of Colorado Press), 67–82.
Ollé, A., Mosquera, M., Rodríguez, X. P., de Lombera-Hermida, A., García-Antón, M. D., García-Medrano, P., et al. (2013). The Early and Middle Pleistocene technological record from Sierra de Atapuerca (Burgos, Spain). Quat. Int. 295, 138–167. doi:10.1016/j.quaint.2011.11.009
Owen, R. B., Muiruri, V. M., Lowenstein, T. K., Renaut, R. W., Rabideaux, N., Luo, S., et al. (2018). Progressive Aridification in East Africa over the Last Half Million Years and Implications for Human Evolution. Proc. Natl. Acad. Sci. USA. 115 (44), 11174–11179. doi:10.1073/pnas.1801357115
Pappu, S., Gunnell, Y., Akhilesh, K., Braucher, R., Taieb, M., Demory, F., et al. (2011). Early Pleistocene Presence of Acheulian Hominins in South India. Science. 331, 1596–1599. doi:10.1126/science.1200183
Parush, Y., Assaf, E., Slon, V., Gopher, A., and Barkai, R. (2015). Looking for Sharp Edges: Modes of flint Recycling at Middle Pleistocene Qesem Cave, Israel. Quat. Int. 361, 61–87. doi:10.1016/j.quaint.2014.07.057
Pelegrin, J. (2005). “Remarks about Archaeological Techniques and Methods of Knapping: Elements of a Cognitive Approach to Stone Knapping,” in Stone Knapping: The Necessary Conditions for a Uniquely Human Behavior. Editors V. Roux,, and B. Bril (Cambridge: McDonald Institute for Archaeological Research), 23–34.
Peresani, M. (2003). Discoid Lithic Technolog. British Archaeological Reports International Series 1120. Oxford: Archaeopress.
Picin, A., Peresani, M., Falguères, C., Gruppioni, G., and Bahain, J.-J. (2013). San Bernardino Cave (Italy) and the Appearance of Levallois Technology in Europe: Results of a Radiometric and Technological Reassessment. PLoS One. 8 (10), e76182. doi:10.1371/journal.pone.0076182
Picin, A. (2017). Technological Adaptation and the Emergence of Levallois in Central Europe: New Insight from the Markkleeberg and Zwochau Open-Air Sites in Germany. J. Quat. Sci. 33 (3), 300–312. doi:10.1002/jqs.2978
Picin, A., and Vaquero, M. (2016). Flake Productivity in the Levallois Recurrent Centripetal and Discoid Technologies: New Insights from Experimental and Archaeological Lithic Series. J. Archaeological Sci. Rep. 8, 70–81. doi:10.1016/j.jasrep.2016.05.062
Potts, R., Behrensmeyer, A. K., Faith, J. T., Tryon, C. A., Brooks, A. S., Yellen, J. E., et al. (2018). Environmental Dynamics during the Onset of the Middle Stone Age in Eastern Africa. Science. 360 (6384), 86–90. doi:10.1126/science.aao2200
Potts, R., and Faith, J. T. (2015). Alternating High and Low Climate Variability: The Context of Natural Selection and Speciation in Plio-Pleistocene Hominin Evolution. J. Hum. Evol. 87, 5–20. doi:10.1016/j.jhevol.2015.06.014
Prévost, M., and Zaidner, Y. (2020). New Insights into Early MIS 5 Lithic Technological Behavior in the Levant: Nesher Ramla, Israel as a Case Study. PLoS One 15 (4), e0231109. doi:10.1371/journal.pone.0231109
Rabinovich, R., Ackermann, O., Aladjem, E., Barkai, R., Biton, R., Milevski, I., et al. (2012). Elephants at the Middle Pleistocene Acheulian Open-Air Site of Revadim Quarry, Israel. Quat. Int. 276-277, 183–197. doi:10.1016/j.quaint.2012.05.009
Režek, Ž., Dibble, H. L., McPherron, S. P., Braun, D. R., and Lin, S. C. (2018). Two Million Years of Flaking Stone and the Evolutionary Efficiency of Stone Tool Technology. Nat. Ecol. Evol. 2 (4), 628–633. doi:10.1038/s41559-018-0488-4
Richter, D., Grün, R., Joannes-Boyau, R., Steele, T. E., Amani, F., Rué, M., et al. (2017). The Age of the Hominin Fossils from Jebel Irhoud, Morocco, and the Origins of the Middle Stone Age. Nature. 546 (7657), 293–296. doi:10.1038/nature22335
Rodríguez, J., Burjachs, F., Cuenca-Bescós, G., García, N., Van der Made, J., González, A. P., et al. (2011). One Million Years of Cultural Evolution in a Stable Environment at Atapuerca (Burgos, Spain). Quat. Sci. Rev. 30 (11-12), 1396–1412. doi:10.1016/j.quascirev.2010.02.021
Rolland, N. (1995). “Levallois Technique Emergence: Single or Multiple? A Review of the Euro-African Record,” in The Definition and Interpretation of Levallois Technology. Editors H. L. Dibble, and O. Bar-Yosef (Madison: Prehistory Press), 333–359.
Ronen, A., Ohel, M. Y., and LamdanAssaf, M. A. (1980). Acheulean Artifacts from Two Trenches at Ma’ayan Barukh. Isr. Exploration J. 30, 17–33. https://www.jstor.org/stable/27925739
Ronen, A., and Winter, Y. (1997). Eyal 23: a Lower Palaeolithic Site in the Eastern Sharon, Israel. Quartär. 47, 177–188.
Rosenberg-Yefet, T., and Barkai, R. (2019). Early in the Late Acheulian. Mitekufat Haeven: J. Isr. Prehistoric Society 49, 31–36. https://www.jstor.org/stable/26913197
Rosenberg-Yefet, T., Shemer, M., and Barkai, R. (2021). Acheulian Shortcuts: Cumulative Culture and the Use of Handaxes as Cores for the Production of Predetermined Blanks. J. Archaeological Sci. Rep. 36, 102822. doi:10.1016/j.jasrep.2021.102822
Rosenberg-Yefet, T. (2016). The Origins of the Levallois Method and Implications for its Distribution Model. [M.A. Thesis]. Israel: Tel Aviv University. (in Hebrew).
Roux, V., Bril, B., and Karasik, A. (2018). Weak Ties and Expertise: Crossing Technological Boundaries. J. Archaeol Method Theor. 25 (4), 1024–1050. doi:10.1007/s10816-018-9397-8
Schlanger, N. (1996). Understanding Levallois: Lithic Technology and Cognitive Archaeology. Caj. 6 (2), 231–254. doi:10.1017/s0959774300001724
Sharon, G. (2009). Acheulian Giant‐Core Technology. Curr. Anthropol. 50 (3), 335–367. doi:10.1086/598849
Sharon, G. (2007). Acheulian Large Flake Industries: Technology, Chronology, and Significance. University in Oxford, England: BAR International Series 1701. Archaeopress.
Sharon, G., Alperson-Afil, N., and Goren-Inbar, N. (2011). Cultural Conservatism and Variability in the Acheulian Sequence of Gesher Benot Ya'aqov☆. J. Hum. Evol. 60, 387–397. doi:10.1016/j.jhevol.2009.11.012
Sharon, G. (2014). “The Early Prehistory of West and Central Asia,” in The Cambridge World Prehistory. Editors C. Renfrew, and P. Bahn (Cambridge: Cambridge University Press), 1357–1378.
Shea, J. J. (2013). Stone Tools in the Paleolithic and Neolithic Near East: A Guide. New York: Cambridge University Press.
Shemer, M., Brailovsky-Rokser, L., Solodenko, N., Agam, A., Greenbaum, N., Porat, N., et al. (2018). Introducing Jaljulia: A Late Acheulian Mega-Site in central Israel. Paper Presented at the Annual UISPP. Paris, France.
Shimelmitz, R., and Kuhn, S. L. (2013). Early Mousterian Levallois Technology in Unit IX of Tabun Cave. PaleoAnthropology. 2013, 1–27. doi:10.4207/PA.2013.ART77
Shimelmitz, R., and Kuhn, S. L. (2018). The Toolkit in the Core: There Is More to Levallois Production Than Predetermination. Quat. Int. 464, 81–91. doi:10.1016/j.quaint.2017.08.011
Shimelmitz, R. (2015). The Recycling of flint throughout the Lower and Middle Paleolithic Sequence of Tabun Cave, Israel. Quat. Int. 361, 34–45. doi:10.1016/j.quaint.2014.08.033
Shimelmitz, R., Weinstein-Evron, M., Ronen, A., and Kuhn, S. L. (2016). The Lower to Middle Paleolithic Transition and the Diversification of Levallois Technology in the Southern Levant: Evidence from Tabun Cave, Israel. Quat. Int. 409, 23–40. doi:10.1016/j.quaint.2015.12.036
Shipton, C., Clarkson, C., Pal, J. N., Jones, S. C., Roberts, R. G., Harris, C., et al. (2013). Generativity, Hierarchical Action and Recursion in the Technology of the Acheulean to Middle Palaeolithic Transition: A Perspective from Patpara, the Son Valley, India. J. Hum. Evol. 65 (2), 93–108. doi:10.1016/j.jhevol.2013.03.007
Shipton, C. (2016). Hierarchical Organization in the Acheulean to Middle Palaeolithic Transition at Bhimbetka, India. Caj. 26 (4), 601–618. doi:10.1017/s095977431600041x
Solodenko, N. (2010). On Tools and Elephant: An Analysis of a Lithic Assemblage from Area B of the Late Acheulian Site Revadim Quarry. [MA Dissertation]. Tel-Aviv: University of Tel-Aviv.
Solodenko, N., Zupancich, A., Cesaro, S. N., Marder, O., Lemorini, C., and Barkai, R. (2015). Fat Residue and Use-Wear Found on Acheulian Biface and Scraper Associated with Butchered Elephant Remains at the Site of Revadim, Israel. PloS one. 10 (3), e0118572. doi:10.1371/journal.pone.0118572
Soressi, M. (2004). From the Mousterian of Acheulian Tradition Type A to Type B: A Change in Technical Tradition, Raw Material, Task, or Settlement Dynamics. Settlement Dyn. Middle Paleolithic Middle Stone Age. 2, 343–366.
Stout, D., Hecht, E., Khreisheh, N., Bradley, B., and Chaminade, T. (2015). Cognitive Demands of Lower Paleolithic Toolmaking. PLoS One. 10 (4), e0121804. doi:10.1371/journal.pone.0121804
Stout, D. (2010). “Possible Relations between Language and Technology in Human Evolution,” in Stone Tools and the Evolution of Human Cognition. Editors A. Nowell, and I. Davidson (Boulder: University Press of Colorado), 159–184.
Stout, D. (2011). Stone Toolmaking and the Evolution of Human Culture and Cognition. Phil. Trans. R. Soc. B. 366, 1050–1059. doi:10.1098/rstb.2010.0369
Tanner, A. (2021). An Embarrassment of Riches: The Ontological Aspect of Meat and Fat Harvesting Among Subarctic hunters. Editors G. E. Konidaris, R. Barkai, V. Tourloukis, and K. Harvati (Tübingen): Tübingen University Press), 23–46.
Tanner, A. (2014). Bringing home Animals: Mistissini hunters of Northern Quebec. Newfoundland: ISER Books.
Tennie, C., Braun, D. R., PremoMcPherron, L. S. S. P., and McPherron, S. P. (2016). “The Island Test for Cumulative Culture in the Paleolithic,”. Editors M. Haidle, N. Conard, and M. Bolus (Springer), 121–133. doi:10.1007/978-94-017-7426-0_11
Tennie, C., Call, J., and Tomasello, M. (2009). Ratcheting up the Ratchet: on the Evolution of Cumulative Culture. Phil. Trans. R. Soc. B. 364 (1528), 2405–2415. doi:10.1098/rstb.2009.0052
Tennie, C., Premo, L. S., Braun, D. R., and McPherron, S. P. (2017). Early Stone Tools and Cultural Transmission: Resetting the Null Hypothesis. Curr. Anthropol. 58 (5), 652–672. doi:10.1086/693846
Terradas, X. (2003). Discoid Flaking Method: conception and Technological Variability. Bar Int. Ser. 1120, 19–32.
Tomasello, M. (1999). The Cultural Origins of Human Cognition. Cambridge, MA: Harvard University Press.
Toth, N., and Schick, K. (2018). An Overview of the Cognitive Implications of the Oldowan Industrial Complex. Azania: Archaeological Res. Africa. 53 (1), 3–39. doi:10.1080/0067270x.2018.1439558
Tryon, C. A. (2006). “Early” Middle Stone Age Lithic Technology of the Kapthurin Formation (Kenya). Curr. Anthropol. 47, 367–375. doi:10.1086/503066
Tryon, C. A., and Faith, J. T. (2013). Variability in the Middle Stone Age of Eastern Africa. Curr. Anthropol. 54 (S8), S234–S254. doi:10.1086/673752
Tryon, C. A., McBrearty, S., and Texier, P. J. (2005). Levallois Lithic Technology from the Kapthurin Formation, Kenya: Acheulian Origin and Middle Stone Age Diversity. Afr. Archaeological Rev. 22 (4), 199–229. doi:10.1007/s10437-006-9002-5
Tryon, C. A., and Potts, R. (2011). Reduction Sequence, Chaîne Opératoire, and Other Methods: The Epistemologies of Different Approaches to Lithic Analysis Approaches for Understanding Flake Production in the African Acheulean. PaleoAnthropology, 376–389. doi:10.4207/PA.2011.ART65
Tuffreau, A. (1995). “The Variability of Levallois Technology in Northern France and Neighbouring Areas,” in The Definition and Interpretation of Levallois Technology. Editors H. L. Dibble, and O. Bar Yosef (Madison: Prehistory Press), 413–427.
Usik, V. I., Rose, J. I., Hilbert, Y. H., Van Peer, P., and Marks, A. E. (2013). Nubian Complex Reduction Strategies in Dhofar, Southern Oman. Quat. Int. 300, 244–266. doi:10.1016/j.quaint.2012.08.2111
Valente, T. W. (1996). Social Network Thresholds in the Diffusion of Innovations. Social networks. 18 (1), 69–89.
Van Peer, P. (1995). “The Levallois Problem: Present Issues,” in The Definition and Interpretation of Levallois Technology. Editors H. L. Dibble, and O. Bar Yosef (Madison: Prehistory Press), 1–10.
Vaquero, M., Bargalló, A., Chacón, M. G., Romagnoli, F., and Sañudo, P. (2015). Lithic Recycling in a Middle Paleolithic Expedient Context: Evidence from the Abric Romaní (Capellades, Spain). Quat. Int. 361, 212–228. doi:10.1016/j.quaint.2014.05.055
Venditti, F., Cristiani, E., Nunziante-Cesaro, S., Agam, A., Lemorini, C., and Barkai, R. (2019a). Animal Residues Found on Tiny Lower Paleolithic Tools Reveal Their Use in Butchery. Sci. Rep. 9 (1), 13031–13114. . doi:10.1038/s41598-019-49650-8
Venditti, F., Agam, A., and Barkai, R. (2019b). Techno-functional Analysis of Small Recycled Flakes from Late Acheulian Revadim (Israel) Demonstrates a Link between Morphology and Function. J. Archaeological Sci. Rep. 28, 102039. doi:10.1016/j.jasrep.2019.102039
Villa, P. (2009). DISCUSSION 3: The Lower to Middle Paleolithic Transition eds: M. Camps, and P. R. Chauhan, Sourcebook of Paleolithic Transitions: Methods, Theories, and Interpretations, 265–270.doi:10.1007/978-0-387-76487-0_17
White, M., and Ashton, N. (2003). Lower Palaeolithic Core Technology and the Origins of the Levallois Method in North‐Western Europe. Curr. Anthropol. 44, 598–609. doi:10.1086/377653
White, M., Ashton, N., and Scott, B. (2011). The Emergence, Diversity and Significance of Mode 3 (Prepared Core) Technologies. Dev. Quat. Sci. 14, 53–65. doi:10.1016/b978-0-444-53597-9.00005-4
White, M., and Pettitt, P. (2016). Technology of Early Palaeolithic Western Europe: an Heuristic Framework. Lithics–The J. Lithic Stud. Soc. (16), 27–40.
Wilkins, J., and Chazan, M. (2012). Blade Production ∼500 Thousand Years Ago at Kathu Pan 1, South Africa: Support for a Multiple Origins Hypothesis for Early Middle Pleistocene Blade Technologies. J. Archaeological Sci. 39 (6), 1883–1900. doi:10.1016/j.jas.2012.01.031
Wilkins, J., Pollarolo, L., and Kuman, K. (2010). Prepared Core Reduction at the Site of Kudu Koppie in Northern South Africa: Temporal Patterns across the Earlier and Middle Stone Age Boundary. J. Archaeological Sci. 37 (6), 1279–1292. doi:10.1016/j.jas.2009.12.031
Wynn, T., and Coolidge, F. L. (2016). Archeological Insights into Hominin Cognitive Evolution. Evol. Anthropol. 25 (4), 200–213. doi:10.1002/evan.21496
Wynn, T., and Coolidge, F. L. (2010). “How Levallois Reduction Is Similar to, and Not Similar to, Playing Chess,” in Stone Tools and the Evolution of Human Cognition. Editors A. Nowell, and I. Davidson (Boulder, CO: University Press of Colorado), 83–103.
Zaidner, Y., and Weinstein-Evron, M. (2020). The Emergence of the Levallois Technology in the Levant: A View from the Early Middle Paleolithic Site of Misliya Cave, Israel. J. Hum. Evol. 144, 102785. doi:10.1016/j.jhevol.2020.102785
Zaidner, Y., and Weinstein-Evron, M. (2016). The End of the Lower Paleolithic in the Levant: the Acheulo-Yabrudian Lithic Technology at Misliya Cave, Israel. Quat. Int. 409, 9–22. doi:10.1016/j.quaint.2015.12.080
Zupancich, A., Shemer, M., and Barkai, R. (2021). Biface Use in the Lower Paleolithic Levant: First Insights from Late Acheulean Revadim and Jaljulia (Israel). J. Archaeological Sci. Rep. 36, 102877. doi:10.1016/j.jasrep.2021.102877
Zupancich, A., Solodenko, N., Rosenberg-Yefet, T., and Barkai, R. (2018). On the Function of Late Acheulean Stone Tools: New Data from Three Specific Archaeological Contexts at the Lower Palaeolithic Site of Revadim, Israel. Lithic Technology 43 (4), 255–268. doi:10.1080/01977261.2018.1523098
Keywords: Levallois, prepared cores, Late Acheulian, technological innovation, core technology
Citation: Rosenberg-Yefet T, Shemer M and Barkai R (2022) Lower Paleolithic Winds of Change: Prepared Core Technologies and the Onset of the Levallois Method in the Levantine Late Acheulian. Front. Earth Sci. 10:847358. doi: 10.3389/feart.2022.847358
Received: 02 January 2022; Accepted: 07 February 2022;
Published: 24 March 2022.
Edited by:
Marie-Hélène Moncel, Director of research CNRS-MNHN, FranceReviewed by:
Javier Baena, Autonomous University of Madrid, SpainCopyright © 2022 Rosenberg-Yefet, Shemer and Barkai. This is an open-access article distributed under the terms of the Creative Commons Attribution License (CC BY). The use, distribution or reproduction in other forums is permitted, provided the original author(s) and the copyright owner(s) are credited and that the original publication in this journal is cited, in accordance with accepted academic practice. No use, distribution or reproduction is permitted which does not comply with these terms.
*Correspondence: T. Rosenberg-Yefet, dGFtYXJyb3N5QGdtYWlsLmNvbQ==
Disclaimer: All claims expressed in this article are solely those of the authors and do not necessarily represent those of their affiliated organizations, or those of the publisher, the editors and the reviewers. Any product that may be evaluated in this article or claim that may be made by its manufacturer is not guaranteed or endorsed by the publisher.
Research integrity at Frontiers
Learn more about the work of our research integrity team to safeguard the quality of each article we publish.