- 1Instituto de Geociências, Brasília, Brazil
- 2Programa de Pós-Graduação, Universidade de Brasília, Campus Darcy Ribeiro, Brasília, Brazil
The exceptional development of lateritic profiles make the Amazon one of the best places to conduct a refined study on the effects of long-term tropical climate on the Earth's surface rocks. Concentrations of Nd and Sr isotopes, as well as rare earth elements (REE), Sr, and other trace elements were determined in six profiles in order to shed new light into element behavior and into the processes controlling the geochemistry and the transfer of these elements among the different compartments of the critical zone. Our investigation indicates natural isotope regional pulse transformations in the Oxisol at the top of all profiles. The ƐNd(t=0) values of the parent rock, mottled horizon, and lateritic duricrust are radiogenic similar to the Amazon craton signature. The thick Oxisol has ƐNd(t=0) values which are much more radiogenic and less variable, whereas it has Zr, Th, Y, and REE in higher concentrations. These issues highlight a complex process for the Nd system, and the following possibilities are proposed to explain this behavior: formation of authigenic cerianite and/or kaolinite, penetration of low-temperature weathering solutions along zircon fractures, and some vegetation uptake. These possibilities allow keeping the more radiogenic Nd isotopes, whereas the less radiogenic Nd is released especially in the Oxisol after REE mineral(s) weathering. The Sr system contrary to that of Nd became less radiogenic along the profiles and relatively to the parent rocks content, because nearly all Sr was removed after K-Rb feldspar and mica weathering. However, a mixture return of less radiogenic Sr from plant, biogenic aerosol, and rainwater may have helped let the weathering profiles become less radiogenic. These characteristics let the Oxisol even less Sr radiogenic but still permitting to identify the general parent rocks signature. In contrast, Nd is highly fractionated in the Oxisol relative to the parent rock. Thus, the REE and Sr behavior, similar in the six profiles, does not necessarily reflect only the parent rock geochemistry, but it also depends on the multiple surficial processes typical of the critical zone: weathering, pedogenesis, plant root activity, and rainwater composition which change the inherited primary minerals isotope signatures.
1 Introduction
Up to 30% of the continental surface in low relief landscapes in tropical regions have developed deep weathering lateritic profiles from the basement rock (Tardy and Roquin 1998). The formation of lateritic profiles causes chemical elements and solution transfer, residual minerals accumulation (e.g. zircon and rutile), and supergene phases formation. This generates clays (e.g., kaolinite), Al and Fe oxi-hydroxides (e.g., gibbsite, hematite, and goethite), and Ti and Mn-K oxides (anatase and cryptomelane) from the parent rock to the weathering horizons (Nahon, 1991). The lateritic profile consists (from the base to the top) of a saprolite developing over the basement rock and a mottled horizon capped with a Fe-duricrust or bauxite crust. Oxisol can develop on top of the duricrust (e.g., Lucas 1997; Horbe and Costa 1999; Costa et al., 2014). Those deep weathering profiles reflect the combination of intense chemical weathering and slow physical erosion (Retallack, 2010).
During the weathering process, elements react differently, and their contents change according the parent rock, time, and weathering intensity. For instance, the rare earth elements (REE) fractionation may be controlled by different processes, such as weathering of REE-bearing minerals, physicochemical conditions, provenance differentiation, and hydraulic sorting (e.g., Henderson, 1986; Wood, 1990; Su et al., 2017; Jonell et al., 2018).
Most REE in rocks (∼70–90%) are present in accessory minerals (e.g., allanite, apatite, titanite, monazite, zircon), whereas the remainders are distributed among the rock-forming minerals (Braun et al., 1993; Harlavan et al., 2009). The REE are commonly mobilized and released by weathering when hosted in phosphates (e.g., apatite) and silicates (e.g., allanite, biotite, amphibole, and plagioclase) (e.g., Hoskin and Schaltegger, 2003; Pereira et al., 2005; Laveuf and Cornu, 2009). In contrast, they accumulate when associated with residual minerals such as zircon, xenotime, monazite, thorite, and titanite (e.g., Horbe and Costa, 1999; Velbel, 1999).
The REE migrate according to their relative mobility and solubility (Laveuf and Cornu, 2009). They can be either incorporated or adsorbed into authigenic amorphous or crystalline phases (clay minerals, Fe-Mn oxyhydroxides, Al-phosphates) and organic compounds or leached out as solutes (cerianite) (Braun et al., 1993; Minarik et al., 1998; Bayon et al., 2002; Tyler, 2004; Zaitsev et al., 2011; Braun et al., 2017). Low pH and reducing conditions favor REE release from the soil and REE fractionation (Cao et al., 2001), as well as canopy interactions (Braun et al., 2017). This REE mobilization vs. accumulation behavior controls the depletion or enrichment relative to the basement rock and the formation of Ce positive anomaly (Braun et al., 1990; Ohlander et al., 1996; Tyler, 2004).
Although the weathering effect on the REE concentrations is relatively well understood, the knowledge about the effects on Nd isotope variations is not yet fully understood. For example, MacFarlane et al. (1994), McDaniel et al. (1994), Ohlander et al. (2000), Negrel et al. (2000), Viers and Wasserburg (2004), and Tripathy et al. (2011) suggest that in the natural environment, the isotope compositions are controlled by chemical and isotope signatures of REE-bearing minerals and the parent rocks. For Ohlander et al. (2000) the ƐNd values increase with increasing Sm/Nd ratios and with preferential adsorption of Sm relative to Nd. Ma et al. (2010) propose a Nd fractionation with preferential 143Nd removal compared to 144Nd. On the other hand, Liu et al. (2013), Chabaux et al. (2013), Thieblemont et al. (2014), Babechuk et al. (2015), and Moragues-Quiroga et al. (2017) relate the isotopic changes to source, atmospheric deposition, and seasonal water saturation dynamics, whereas Jonell et al. (2018) associate the changes to the grain size fractionation and mineralogy distribution. Finally, plants uptake in the upper humus-richer portion of soil profiles can fractionate REE changing the Sm-Nd isotope ratio in the soils (Andersson et al., 2001; Aubert et al., 2001).
Relative to Nd isotopes, there are much more investigations on the Sr isotopes behavior during progressive rocks weathering (e.g., Capo et al., 1998; Stewart et al., 1998; Souza et al., 2010; Wei et al., 2018). Since Sr is mostly associated with weatherable minerals (biotite, K feldspar, plagioclase, hornblende, calcite, and apatite), it is easier leached than REE and may be redistributed throughout the soil–vegetation system via cation exchange (e.g., Blum and Erel, 1997; Capo et al., 1998; Stewart et al., 1998). Therefore, the Sr isotope fractionation is more perceptible than Nd isotope fractionation, although no fractionation is expected for both in the natural environment due to their relative high atomic number and small relative mass difference (Tripathy et al., 2011). Thus, the study of Nd and Sr appears to be an extremely powerful approach for understanding the evolution of the polygenetic regolith systems (Moragues-Quiroga et al., 2017). With this goal, the present study combines mineralogical, major and trace elements, and Nd and Sr isotope analyses in order to identify the behavior and the processes controlling the REE and Sr systems in the critical zone. These information will help to better understand the transfer of elements between the different weathering lateritic profiles compartments, and to find tracers of weathering processes and element cycling. For this purpose, six lateritic profiles in the Amazon (Brazil) have been selected for study (Figure 1).
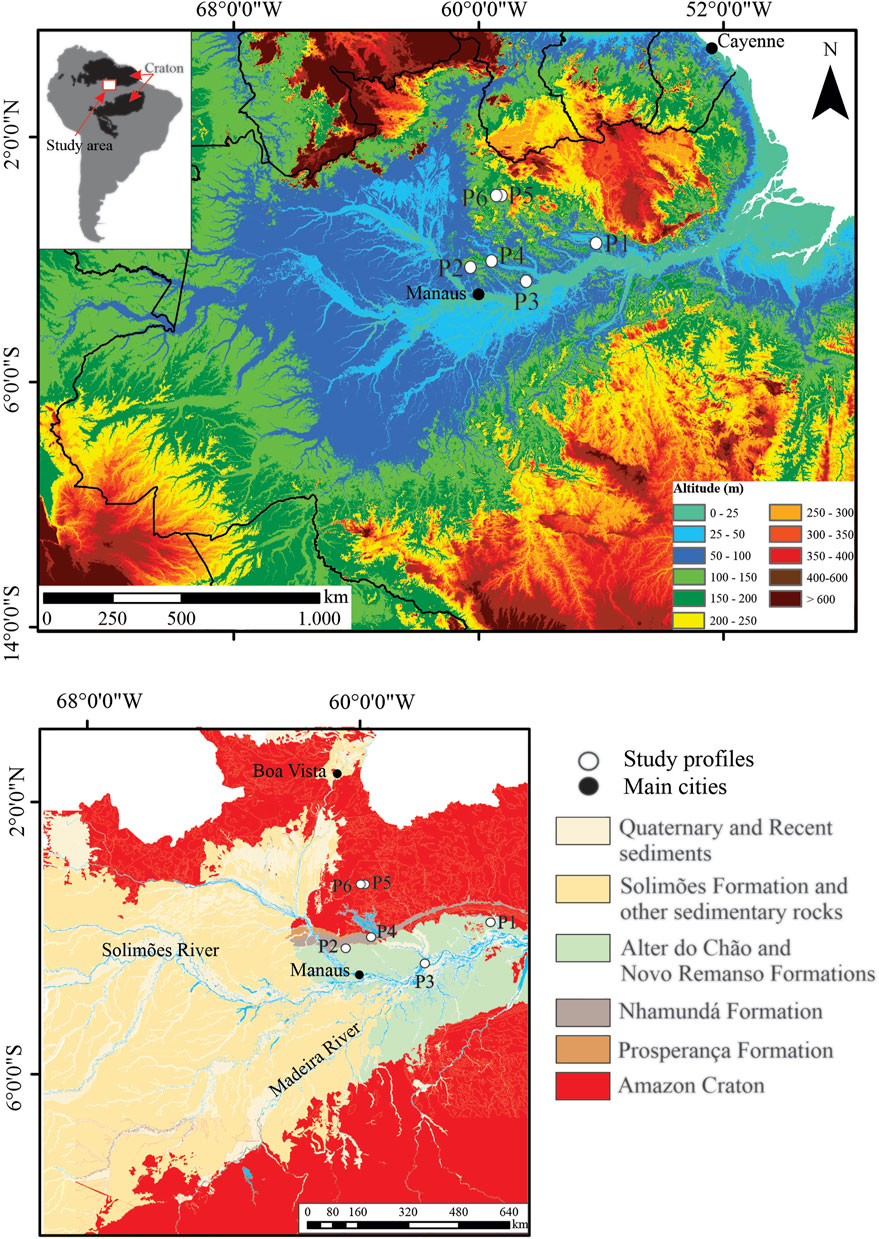
FIGURE 1. Geographical and geological landscape of the studied region and location of the sampled profiles.
2 Materials and Methods
2.1 Sampling Strategy
Six lateritic profiles, with the associated soils developed above the lateritic Fe-duricrust or Al-duricrust (bauxite), have been sampled in Central Amazon (Brazil) (Figure 1). These six profiles represent the effect of the critical zone on the main groups of rocks in the Amazon. Profile 1 was sampled in one open pit (Trombetas bauxite Mine), whereas profiles 2, 3, and 4 were sampled in road outcrops and 5 and 6 were sampled in boreholes (Pitinga Tin Mine) (Figure 2). In profiles 1, 2, and 3, developed on the same sedimentary rocks (Cretaceous Alter do Chão Formation), were sampled the weathering horizons as well as 11 samples of the parent rocks in outcrops next to the weathering profiles. In the other three profiles, only the upper part was sampled because the parent rocks were not cropping out. These latest profiles allowed the complementary geochemical behavior between the lateritic duricrust and the Oxisol. Their coordinates and details about geology, geomorphology conditions, and sampled horizons are in Table 1.
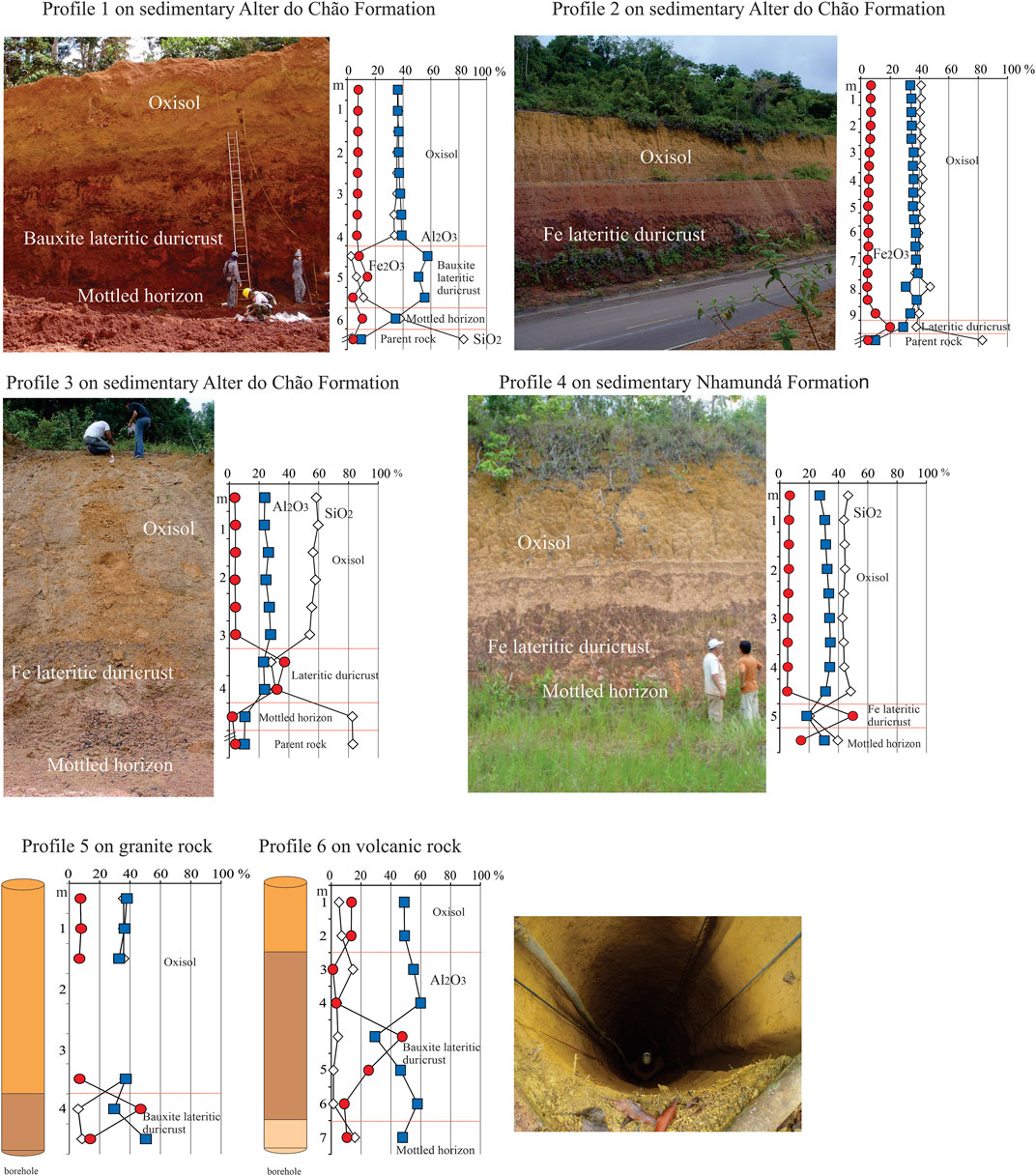
FIGURE 2. Profile structure showing the sampled horizons and the respective plot of SiO2, Fe2O3, and Al2O3 contents expressed as weight % along the horizons.
The region has a humid tropical climate with temperatures averaging 26°C and rainfall of up to 2,100 mm/year, occurring mainly from November to May. All profiles are in areas well preserved, close to or under the dense forest vegetation, with low anthropic effect.
2.2 Profile Characteristics
Profiles 1, 2, and 3 were developed on Cretaceous sedimentary rocks (Alter do Chão Formation; Daemon and Contreiras, 1971; Daemon, 1975; Cunha et al., 1994; Silva et al., 2003) (Figure 1), and profile 4 on Early Silurian sedimentary rock (Cunha et al., 1994). Profiles 5 and 6 were developed on granite and felsic volcanic rocks, respectively, of the Tapajós-Parima province (2.04–1.86 Ga) from the Amazon craton (Santos et al., 2000; Santos, 2003).
The sampled horizons of the profiles are as follows (Figure 2): profile 1—the mottled horizon, 2 m of bauxite lateritic duricrust covered by 3.5 m of Oxisol; profile 2—the 0.5 m of ferruginous lateritic duricrust covered by 9 m of Oxisol; profiles 3 and 4—a mottled horizon, 1 and 2 m of ferruginous lateritic duricrust covered by 3 and 4.5 m of Oxisol, respectively; profile 5—at least 1.5 m of bauxite lateritic duricrust covered by 3.5 m of Oxisol; profile 6—a mottled horizon, the thickest bauxite lateritic crust (4 m) covered only by 2 m of Oxisol.
The mottled horizon (profiles 1, 3, 4, and 6) consists of a friable white to yellow clayey material composed mainly of kaolinite with less goethite, hematite, and variable quartz content. The lateritic duricrusts of the profiles are hard and porous with some friable and clayey portions; often it is massive to pisolith with micro- to cryptocrystalline texture and colored red to pink when bauxite, and red to brown when ferruginous (Figure 2). The lateritic duricrust of profiles 1, 5, and 6 is bauxitic formed mainly of gibbsite, while those of profiles 2 to 4 are ferruginous formed of hematite, goethite, quartz, and less gibbsite and anatase. Profile 2 shows some gibbsite pockets in the ferruginous duricrust.
The Oxisol covering the bauxite and the ferruginous lateritic duricrusts in all six profiles, between 2 and 9 m thick, is very homogeneous with clayey to sandy–clayey texture and no visible stratification and, sometimes, accumulates some loose bauxite and ferruginous pisoliths depending on whether they are covering bauxite and/or ferruginous lateritic duricrust. The silt and clay fractions, composed mainly of kaolinite, have minor amounts of gibbsite (profiles 1, 2, 5, and 6). The sand fraction is a mix of quartz grains, Fe oxi-hydroxide ooliths, and hard kaolinite fragments. The Oxisol in profiles 1, 5, and 6 on the bauxitic laterite duricrust has more gibbsite than the other profiles. More details about mineralogy and chemistry of profiles 1, 5, and 6 can be found in Boulangé and Carvalho, (1997), Lucas (1997), Horbe and Costa (1999), Horbe (2011), and Horbe and Anand (2011).
2.3 Analytical Procedures
Sixty-seven samples were collected from the profiles at approximately 0.5-m intervals and 11 samples from the parent rocks of profiles 1 to 3. Following air-drying and powdering, each bulk sample was subsequently submitted to chemical and isotopic analyses. For the chemical analysis, a sub-sample of 0.2 g from each sample was fused at 1,000°C with lithium meta and tetraborate for 1 h, followed by digestion with HNO3. The resulting solution was analyzed by ICP-AES (inductively coupled plasma atomic emission spectrometer) to obtain total SiO2, Al2O3, Fe2O3, TiO2, and P2O5. Another 0.2 g sub-sample was digested in two stages with HCl, HClO4, and HF for determining Hf, Nb, Rb, Sr, Ta, Th, Y, Zr, and REE with ICP-MS (inductively coupled plasma mass spectrometer). The analyses were performed at the Acme Analytical Laboratories LTD, in Canada.
A second sampling was conducted in profiles 1 and 2 to collect zircon grains from the parent rock, the lateritic duricrust, and the Oxisol due to the possible relationship between zircon, REE, and Nd isotope composition. Electron microprobe analysis (EMPA, JEOL JXA 8230) was performed at Universidade de Brasília, in Brazil in 61 zircons grains, after carbon metallization.
The isotope analyses following two procedures were performed at Laboratório de Estudos Geodinâmicos e Geocronológicos of Universidade de Brasília, in Brazil. In the first procedure, a 40–60 mg sub-sample from each sample was totally digested in two steps in the clean room environment, using a mixture of ultrapure HF/HNO3 and 6 N HCl on a hot plate according to Gioia and Pimentel (2000). In the second procedure, a 40–60 mg sub-sample from profile 1 was digested in two steps in a clean room environment using aqua regia on a hot plate (Santoro et al., 2017). This leaching procedure digestion using aqua regia was done to determine the REE isotope composition of the exchangeable ions, colloidal, iron oxyhydroxides, and organic compounds in the leaching solution of the samples relative to the isotopes signature of the totally digested samples composed mainly of Si-Al and residual minerals.
The AG 50W-X8 cation-exchange resin was used to concentrate and separate REE. Neodymium and Sm were further separated from the REE fraction on a second AG50-X2 cation-exchange column as NH4+ using α-hydroxyisobutyric acid (α-HIBA) buffered at pH 4.5. Also, we used an Eichrom Sr-Spec® cation-exchange resin to separate the Sr isotopes. The Nd concentrates were loaded on double rhenium filaments with H3PO4 (0.1 M) and Sr concentrates on a tungsten filament using a TaF-solution; both were measured as metal on a Thermal Ionization Mass Spectrometer (TRITON), following Gioia and Pimentel (2000). The blank level was 100 pg, and the reproducibility was verified using the NBS 987 Sr standard (0.71028 ± 2). The measured 143Nd/144Nd ratios are presented as fractional deviations in parts per 104 (units) from 143Nd/144Nd in a chondritic uniform reservoir (CHUR) as measured at the present day: ƐNd(t=0) = [(143Nd/144Nd)S/ICHUR(0) − 1] × 04 where (143Nd/144Nd)S is the present-day ratio measured in the sample, and ICHUR(0) is the 143Nd/144Nd ratio in the CHUR reference reservoir at the present (ICHUR(0) = 0.512638; Jacobsen and Wasserburg, 1980). The 143Nd/144Nd ratios were normalized to 146Nd/144Nd of 0.7219 for a decay constant (λ) of 6.54 × 10−12 year−1.
3 Results
3.1 Bulk Geochemistry
In general, the parent rock, the mottled horizon, and the Oxisol show low Fe2O3 compared to the lateritic duricrust (Figure 2; Table 2). The lateritic duricrusts may have either high Fe2O3 or high Al2O3, depending on whether they are more ferruginous (profiles 2, 3, and 4) or bauxitic (profiles 1, 5, and 6). The similar SiO2 and Al2O3 contents in the Oxisol in profiles 1, 3, and 5 (Figure 2) are related to the coexistence of quartz, kaolinite, and gibbsite; the highest Al2O3 content in profile 6 is related to the predominance of kaolinite in the mottled horizon and of gibbsite in the Oxisol, and the highest SiO2 content in profiles 3 and 4 is related to the predominance of quartz along the profiles.
In all profiles, the Hf, Nb, Ta, Th, TiO2, Y, and Zr concentrations are higher in the Oxisol (Table 2). There are some chemical variabilities among the parent rock, the mottled horizon, and the lateritic duricrust. For example, in the high Th and Fe2O3 in samples 3.5 and 5 m of profiles 3 and 4, respectively, the Th/Nb and Th/Y ratios are also high. There is also high SiO2, Zr, and Y content in sample 8 m of profile 2. Despite these variabilities, the Th/Nb, Nb/Y, Zr/Th, Zr/Y, and Th/Y ratios along the profiles are very similar, and the Zr/Hf and Nb/Ta ratios of profiles 1, 2, and 3 are in the range of the parent rock variability (Figure 3).
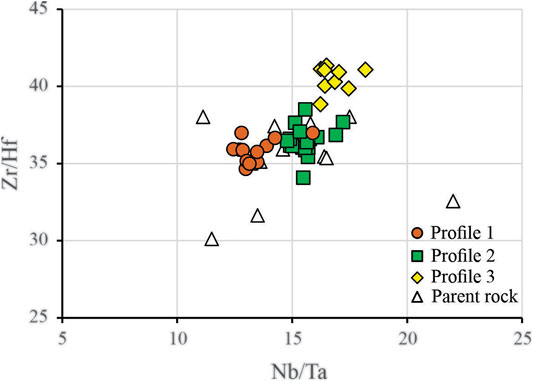
FIGURE 3. Plot of Zr/Hf vs. Nb/Ta ratios of the bulk samples showing the range among the parent rock and the horizons of profiles 1 and 2.
The REE concentrations are higher in the parent rock (profiles 1, 2, and 3) and in the mottled horizon (profile 6) relative to the upward horizons of the respective profiles (Table 3). On the other hand, the REE in the mottled horizon of profiles 5 and 6 and in most samples of the lateritic duricrust and Oxisol of all profiles follow the Zr and TiO2 concentrations (Tables 2, 3) that are higher in the Oxisol than in the downward horizons. The P2O5 content along the profiles follow the REE concentration only in the parent rock of profiles 1 and 2 and in the mottled horizon of profile 6.
3.2 Normalization Relative to the Upper Continental Crust and Lateritic Duricrust
The REE concentrations in the samples were normalized to the upper continental crust (UCC, Taylor and McLenann, 1985) and to the average of the respective lateritic duricrust (Figures 4, 5) of the profiles to seek the weathering effect. The UCC normalized profile 5 (on granite rock) shows enrichment of heavy rare earth elements (HREE) relative to light rare earth elements (LREE) and negative Eu anomaly (Figure 4). Among the profiles, the UCC normalized profile 6 (on felsic volcanic rock) is the most enriched in REE, and profiles 1 to 4 (on sedimentary rocks) show slight enrichment in HREE relative to the LREE. In general, the lateritic duricrusts are less enriched in REE than the Oxisol, the mottled horizon, and the parent rock, except for the LREE of the lateritic duricrust of profiles 2 and 3 whose REE fractionation ratios are similar to the Oxisol (Figure 4). The parent rock of profiles 1, 2, and 3 (Alter do Chão Formation) is more enriched in LREE than most of the weathering horizon samples.
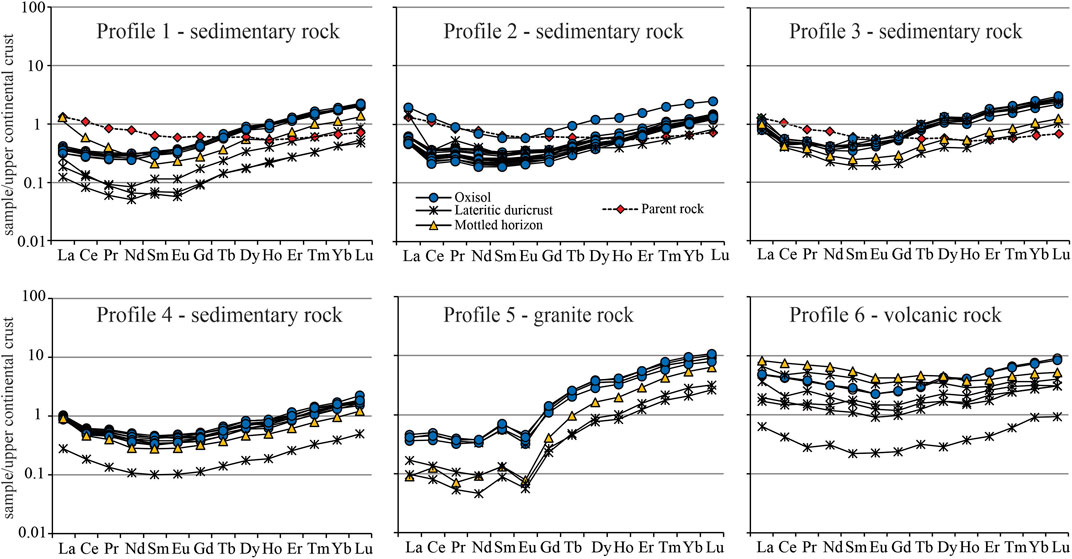
FIGURE 4. Plot of REE expressed as ppm normalized to the estimated average composition of upper continental crust (UCC) of Taylor and McLenann (1985). In general, the lateritic duricrusts are less enriched in REE than the Oxisol, the mottled horizon, and the parent rock.
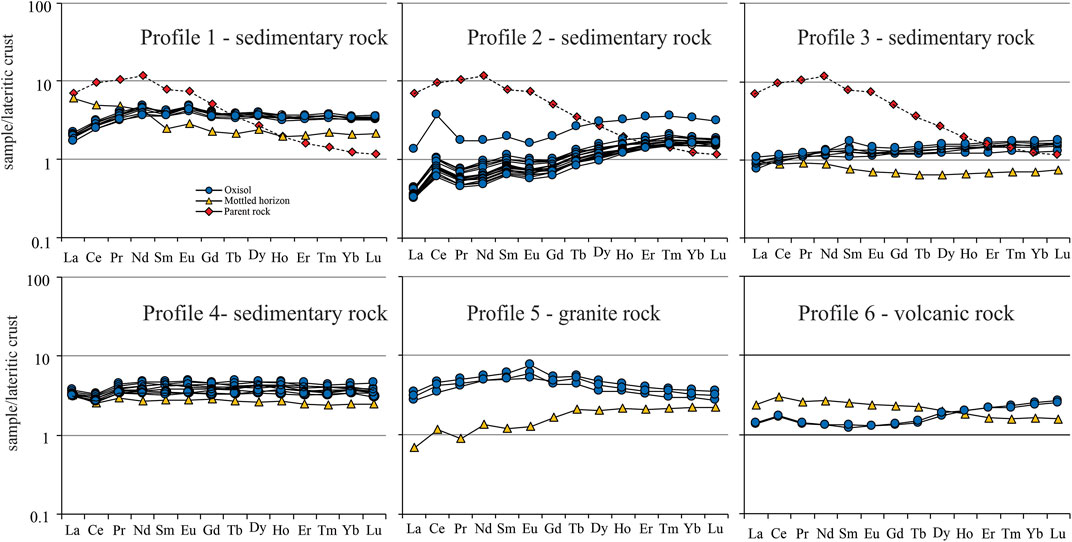
FIGURE 5. Plot of REE expressed as ppm normalized to the average composition of the duricrust. The Oxisol is enriched in REE and depleted in LREE, except the LREE in profiles 4. Profile 4 also shows a slight positive Ce anomaly.
The REE patterns normalized to the lateritic duricrust indicate that the Oxisol is REE enriched (Figure 5), except in profile 2, in which most samples are depleted in LREE.
3.3 Chemistry of Zircon Grains of Profiles 1 and 2
Profiles 1 and 2 were chosen for a more detailed study since they are almost 500 km apart in the east-to-west direction and can provide information about some atmospheric deposition. The zircon grain shapes from these profiles varied greatly, changing from prismatic to sub-round and round, homogenous to zoned, and colored from white and translucent to gray and opaque. The zircon grains from bauxite, Fe duricrust, and Oxisol have reddish stains, while in the parent rock, 90% of the zircon grains are translucent. Several grains show fractures where low-temperature weathering solutions could penetrate (Figure 6).
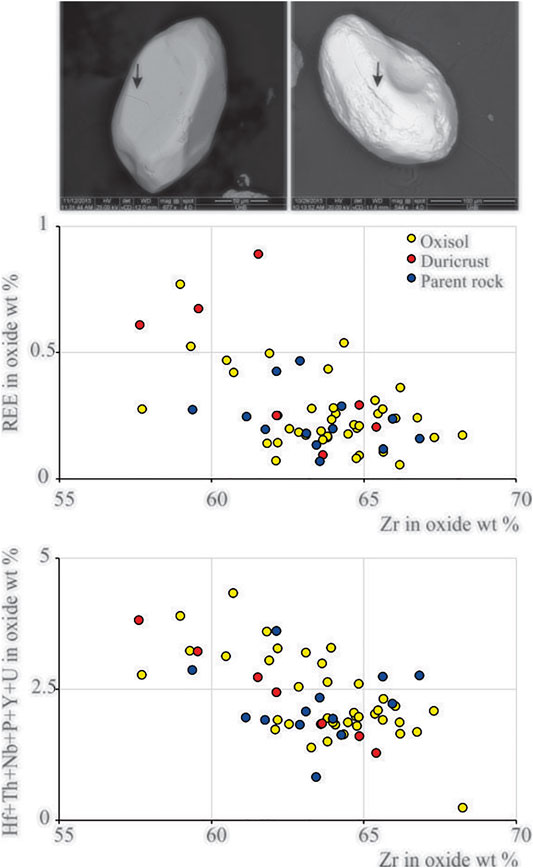
FIGURE 6. Scanning electron microscope (SEM) images of two zircon grains showing fractures where low-temperature weathering solutions can penetrate changing the Nd system. Plots of REE and Si + Hf + Y + Th + U + Nb + P vs. Zr from EMPA (all elements in oxides concentration and expressed as weight %) of zircon grains of profiles 1 and 2 on the sedimentary Alter do Chão Formation showing the range between the parent rock and the horizons.
The zircon grains have 57.63–68.25% of ZrO2, 30 to 35 wt% of SiO2, up to 0.9 wt% of REE2O3, and less than 4.3 wt% of other elements (Hf + Y + Th + U + Nb + P, all of them as oxides wt%) (Figure 6). There are some zircon samples out of the parent rock range because of high UO2 and high REE as well.
3.6 Sm/Nd and Nd Isotopes
The Sm/Nd ratios are higher in the parent rock of profiles 1 to 3 and do not follow the ƐNd(t=0) that is usually less radiogenic in the parent rock, mottled horizon, and lateritic duricrust compared to the much more radiogenic (a drift of 1–7 ƐNd(t=0) units, Figure 9) and less variable Oxisol (Figure 7; Tables 3, 4). However, some peculiarities should be highlighted. Sample 3 m of profile 2 shows a pulse to high radiogenic ratio (ƐNd(t=0) −12) relative to the other samples; profile 5 (from granite) is the most radiogenic (ƐNd(t=0) −13 to −9), while the other profiles are isotopically similar (ƐNd(t=0) −22 to −13); profile 6 (from felsic volcanic rocks), which has the thickest lateritic duricrust, became more radiogenic toward the top (ƐNd(t=0) −22 to −17). There is a slight displacement to more radiogenic ƐNd(t=0) in the Oxisol upper meter in profiles 1, 3, 4, and 5 (Figure 7).
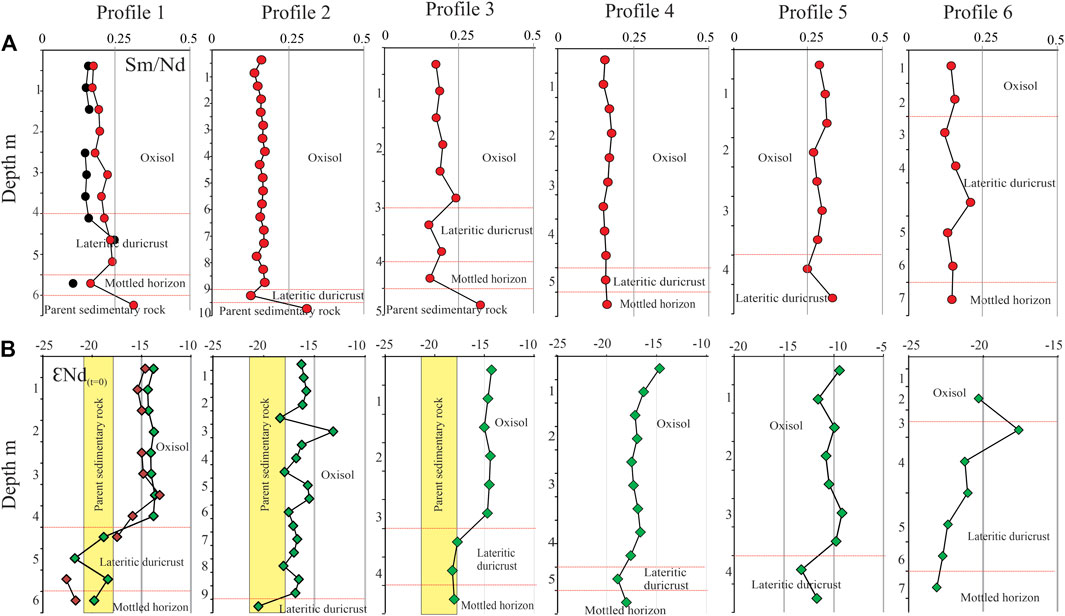
FIGURE 7. (A): Plot of Sm/Nd ratios in red dots color and (B): Plot of ƐNd(t=0) in green diamond color in the bulk samples (triacid leaching). In profile 1 the black dots color show the Sm/Nd ratios and the brown diamond color the ƐNd(t=0)) in the aqua regia leaching. The ƐNd(t=0) of the parent rocks of profiles 1, 2 and 3 are also shown in yellow rectangle. The Sm/Nd ratios are higher in the parent rock of profiles 1–3 do not following the ƐNd(t=0) that is usually less radiogenic in the parent rock, mottled horizon and lateritic duricrust compared to the much more radiogenic and less variable Oxisol.
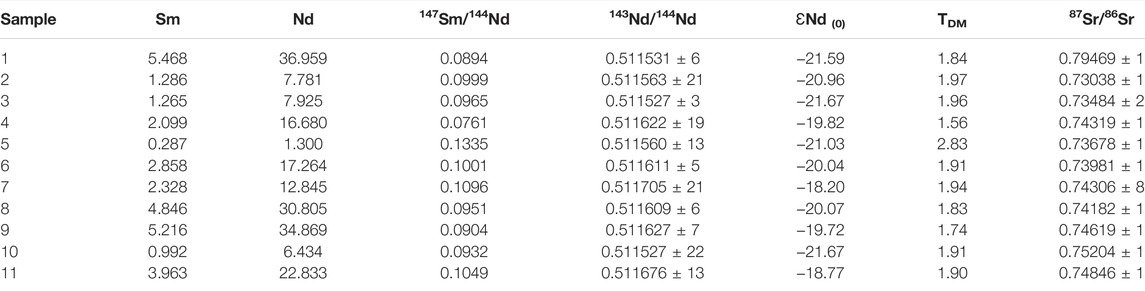
TABLE 4. Sm and Nd concentrations expressed as ppm and Nd and Sr isotopes ratios of profiles 1, 2, and 3 parent rock.
The ƐNd(t=0) of almost all profile 1 samples, digested using aqua regia to determine the REE isotope composition of the exchangeable ions, colloidal, iron oxyhydroxides, and organic compounds relative to the isotopes signature of the totally digested samples composed mainly of Si-Al and residual minerals, are radiogenic and similar to the respective bulk sample (Figure 7).
3.7 Sr and Rb Concentrations and Sr Isotopes
The Sr and Rb concentrations decreased from the parent rock and/or the mottled horizon to the lateritic duricrust (Table 2). Toward the top of the Oxisol Rb is close detection limit (<0.1 ppm), although sample 8 m of profile 2 and sample 2.5 m of profile 4 show some anomalously high concentrations causing variabilities in the Rb/Sr ratios (Figure 8). In all profiles Sr increases slightly from the lateritic duricrust to the Oxisol and reaches an anomalously high concentration in sample 8 m of profile 2.
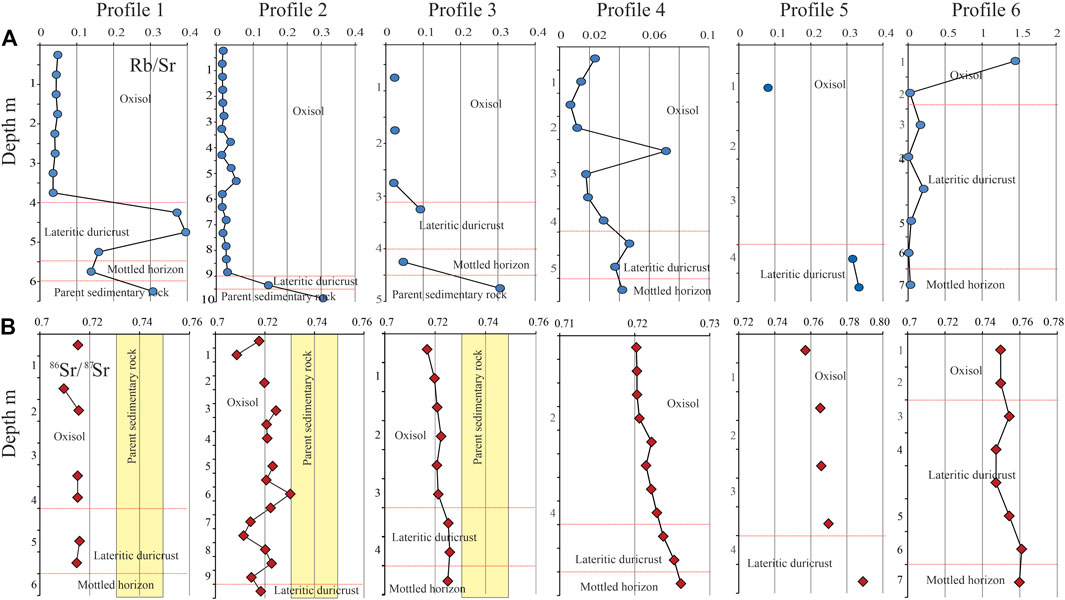
FIGURE 8. (A): Plot of Rb/Sr in blue dots color and (B): plot of 86Sr/87Sr ratios in red diamond color. The 86Sr/87Sr values of the parent rocks of profiles 1, 2 and 3 are also shown (yellow rectangle). The Rb/Sr ratios decrease from the parent rock and/or the mottled horizon to the lateritic duricrust. There is a trend to less radiogenic 86Sr/87Sr ratios toward the top of the profiles 3–6, whereas in the profiles 1 and 2 the 87Sr/86Sr isotope ratios making the horizons isotopically indistinguishable.
The horizons of profiles 1, 2, and 3 are less radiogenic than the parent rock (Figure 8), and most of the samples of profiles 5 and 6 are more radiogenic (87Sr/86Sr = 0.74–0.76) than the other profiles samples (87Sr/86Sr 0.715–0.73). Profiles 1 and 2 have highly variable 87Sr/86Sr isotope ratios, making the horizons isotopically indistinguishable; however, profiles 3 to 6 show less radiogenic 87Sr/86Sr trend toward the top.
4 Discussion
Since Nd and Sr isotope systems vary along the weathering profiles according to the REE and Rb-Sr-bearing mineral composition (e.g., Tripathy et al., 2011 and Jonell et al., 2018), biogeochemistry (e.g., Capo et al., 1998), and atmospheric contributions (e.g., Chabaux et al., 2013; Babechuk et al., 2015), it is necessary to establish well the chemical and mineral compositions relationship among the horizons.
4.1 The Geochemical Signatures of the Weathering Process
The similar SiO2 and Al2O3 contents in the Oxisol in profiles 1, 2, and 5 (Figure 2) indicate the coexistence of quartz, kaolinite, and gibbsite; the highest SiO2 content in profiles 3 and 4 is due to quartz predominance over kaolinite along the profiles, and the highest Al2O3 content in profile 6 is a consequence of kaolinite abundance in the mottled horizon and of gibbsite in the Oxisol. These changes in the mineral composition are a consequence of parent rock and the intensity of weathering. In the tropical harsh weathering there is more gibbsite and less quartz.
The Hf, Nb, Ta, Th, TiO2, Y, Zr, and REE concentrations follow the SiO2 and/or Al2O3 contents across the profiles. Slight variabilities in the Th/Nb, Nb/Y, Zr/Th, Zr/Y, and Th/Y ratios (Tables 2, 3), the Zr/Hf and Nb/Ta ratios of profiles 1, 2, and 3 in the range of the parent rock (Figure 3), the ƐNd(t=0) in the range of Amazon Craton province (Figure 9), and Abouchami et al. (2013) ruling out a Saara dust contribution in the study region indicate a progressive in situ weathering transformation to all horizons of the profiles. This transformation accumulating residual elements in a long and complex history that can take millions of years (e.g., Chabaux et al., 2013; Guinoiseau et al., 2021) is the typical tropical process that recycled the parent rock developing thick lateritic profiles. This reduced the primary mineral diversity through progressive dissolution and leaching of mobile elements of the mineral species, forming Fe and Al lateritic duricrust and concentrating residual minerals (e.g., Nahon 1991; Horbe and Costa 1999; Retallack, 2010). The in situ kaolinite Oxisol accumulation in the top of the profiles was produced by lateritic duricrust pedogenic transformation related to the modern humid conditions and intense Amazon forest plant root activity (e.g., Lucas, 2001; Horbe and Costa, 2005; Costa et al., 2014).
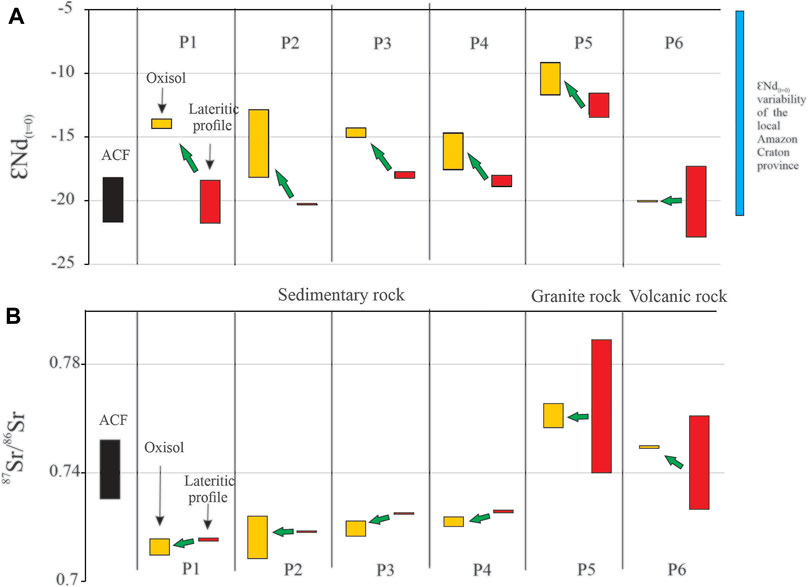
FIGURE 9. Plot of ƐNd(t=0) and 86Sr/87Sr ratios comparing the variabilities (maximum and minimum values) between the mottled horizon and the lateritic duricrust in red rectangle relative to the Oxisol in orange rectangle in profiles 1 to 6 (P1 to P6). ƐNd(t=0 parent rock variabilities from profiles 1, 2, and 3 in black rectangle. (A) Profiles 1 to 3 have similar parent rock, mottled horizon, and lateritic duricrust radiogenic ƐNd(t=0), and there is an increase in the radiogenic ƐNd(t=0) to the Oxisols except in P6. (B) Profiles 1 to 3 have decreased radiogenic86Sr/87Sr from the parent rock (AFC) to the Oxisol and profile 4 from the mottled horizon and lateritic duricrust to the Oxisol. Profiles 5 and 6 have less variabilities in the Oxisol relative to the mottled horizon and lateritic duricrust.
The increase of TiO2, Zr, and REE concentrations to the top of the profiles (Tables 2 and 3) are mostly in rutile, zircon, and REE minerals that are the typical residual minerals in lateritic profiles. Zircon is a REE-hosted mineral (Belousova et al., 2002; Meyer et al., 2011), since the zircon chemical composition of profiles 1 and 2 indicated up to 0.9% of REE concentration (Figure 6) and Zr reached between 1,107 and 3,757 ppm in the Oxisol (Table 2), most of the REE content of the profiles could be in the zircon grains. Other possible REE-residual hosted minerals are monazite, xenotime, and florencite, which are stable weathering phosphatic minerals. However, P2O5 follows the REE behavior, only between the parent rock and the mottled horizon of the profiles (Tables 2, 3), indicating they are probably related to weatherable apatite and not to residual REE phosphates-bearing minerals. However, small amount of Al phosphate may occur.
Since the ƐNd(t=0) is related to REE minerals, there are the following possibilities to explain the displacement of REE concentrations and the radiogenic ƐNd(t=0), especially in the Oxisol (Figure 9): 1) authigenic La-cerianite as indicated by the higher REE concentration in the Oxisol and the oxidant weathering condition that allowed the formation of REE oxides after apatite or other REE weathered mineral dissolution (Table 3, e.g., Viers and Wasserburg 2004; Zaitsev et al., 2011), 2) higher crystallographic disorder kaolinite in the Oxisol (Guinoiseau et al., 2021) that can sorbed REE (e.g., Yang et al., 2019), and 3) zircon accumulation in consequence of the weathering process (Horbe and Costa, 1999; Jonell et al., 2018). Although cerianite formation is a potential link to Ce/Ce* positive anomaly, it can occur even in samples where Ce anomaly is negative (Ram et al., 2019).
The bauxite and ferruginous lateritic duricrusts that are the most leached horizons of weathering profiles and have lower REE concentration than the other horizons, discard the REE be complexed or adsorbed into Al and Fe inorganic ligands (e.g., Ohlander et al., 1996; Ma et al., 2010; Yang et al., 2019) in these horizons after apatite or other REE weathered mineral dissolution.
4.2 Cerianite, Kaolinite, and Zircon as Controller of REE and Nd Isotope Signatures in the Lateritic Profiles
The probable authigenic cerianite after apatite or other REE weathered mineral dissolution and the formation of disordered kolinite in the acid and oxidant conditions typical of tropical weathering profiles, highlight the possibility that these minerals host the more radiogenic Nd isotopes in the Oxisol after the less radiogenic Nd fraction release (drift of 1–7 ƐNd(t=0) units, Figure 9) in consequence of pedogenic water interaction. Although Nd isotope fractionation in low temperature conditions is not expected, it is reported, for example, in basalt weathering profiles (Ma et al., 2010) and in river water (Andersson et al., 2001), just as it happens in other heavy elements as U (Stirling et al., 2007). On the other hand, the slight drift to further radiogenic ƐNd(t=0) in the topmost meter of the Oxisol (Figure 7, profiles 1, 3, 4, and 5), similar to the drift between the lateritic duricrust and the Oxisol, might indicate superficial REE mobilization effects related to pedogenic solution and vegetation uptake (e.g., Andersson et al., 2001; Aubert et al., 2001; Braun et al., 2017) under the modern humid conditions. Six to eight million years of this process, as indicated (Balan et al., 2005) for Oxisol formation in the region, could cause the radiogenic ƐNd(t=0) signature of the Oxisol.
Zircon is a residual resistant mineral that retains the low ƐNd(t=0) ratios of the parent rock and therefore the local province signature of the Amazon craton (Santos et al., 2000; Santos, 2003). The Amazon Craton parent rocks of profiles 5 and 6, and the source of the Paleozoic sedimentary parent rock of profiles 1, 2, 3, and 4 (Figure 1). Penetration of low-temperature weathering solutions along fractures, similar to those shown in the studied zircon grains (Figure 6), can change U-Pb systems in euhedral zircon grains as reported by Lee and Tromp (1995) and Pidgeon et al. (2019). If these solutions also affected the Nd system together the high Zr concentration in the Oxisol, it would be possible for zircon to become a potential source of the further radiogenic ƐNd(t=0) in the Oxisol after the less radiogenic fraction leaching. The penetrating weathering solutions could also explain the chemical composition of some zircon samples out of the parent rock range in profiles 1 and 2 (Figure 6).
Although details of the process that change the Nd system are not well known yet, our investigation indicates a similar effective fractionation degree in the Nd isotopes in the Oxisol profiles (Figure 9) related to surficial weathering/pedogenetic process where parent rock composition, atmospheric deposition, or seasonal water saturation dynamics have lower impact. Profile 6 does not follow this behavior very likely because in only one Oxisol sample it was possible to get ƐNd(t=0) values.
4.3 The Sr Isotope Signatures in the Lateritic Processes
Although there is variability of Sr isotopes especially along profile 2, profiles 3 to 6 show that there is a remarkable progressive transformation caused by the lateritic weathering in the 87Sr/86Sr system (Figure 8). On the other hand, the 87Sr/86Sr ratios higher than >0.74 allow highlighting the influence of K feldspar and mica that are common minerals in the granite and felsic volcanics in determining the more radiogenic isotopic signature of profiles 5 and 6. The weatherability of these minerals and the low capacity to be preserved in sedimentary environment explain the low 87Sr/86Sr ratios in the other profiles (Figure 8; Tables 2, 3).
The higher capacity of Sr to be hosted in kaolinite (Innocent et al., 1997) explains the Sr concentration in the Oxisol (Table 2). However, the decreasing behavior of Rb/Sr and in 87Sr/86Sr ratios along most of the profiles highlights two processes. The first one is the leached Sr and Rb making the lower horizons less radiogenic than the parent rocks and the second one is the probable Sr plant returns that increase the Sr concentration in the Oxisol. However, these plant returns that can assimilate the lighter isotopes of Sr (Souza et al., 2010) and the less radiogenic Sr inputs from biogenic aerosol, as well as the lower sea influence in the rainwater of the region (Artaxo et al., 2005; Honório et al., 2010) (Allègre et al., 1996), could explain the further decrease in the 87Sr/86Sr ratios. Although Sr and Rb are easily released in the lateritic environment affecting the 87Sr/86Sr ratios, the influence of the inherited parent rock minerals is partially retained in the profiles allowing to identify the parent rock signature. Remains and different amounts of Sr weatherable minerals with distinct 87Sr/86Sr ratios like biotite, K feldspar, plagioclase, hornblende, and apatite can also explain the variability in the radiogenic ratios across profile 2.
5 Conclusion
The progressive dissolution and leaching of the mobile elements under the harsh tropical chemical weathering, and especially in the Oxisol formed in consequence of the modern pedogenetic humid conditions, not only reduce the primary mineral diversity by concentrating Al and/or Fe oxi-hydroxides, kaolinite, and residual minerals but also change the inherited isotope signatures of primary minerals. However, Nd and Sr systems have distinct behavior. The Sr and Rb leaching makes the weathering profiles progressively less radiogenic, while the in situ upward Oxisol formation produces radiogenic Nd fractionation. Four possibilities are indicated together or separately as responsible for the Nd isotopes changes in the Oxisol: cerianite and/or disorder kaolinite authigenic minerals, penetration of low-temperature weathering solutions along zircon fractures, and some superficial REE mobilization related to vegetation uptake. All these possibilities store the radiogenic Nd isotopes fraction relative to the lower horizons. These similar behaviors identified in the six profiles, four from sedimentary rocks and two from igneous rocks, indicate that the radiogenic Nd of the Oxisols was produced in a natural process not related to parent rock, atmospheric deposition, and seasonal water saturation dynamics but related to water interaction. However, the degree of Sr and Nd radiogenie is controlled by the parent rock signature.
Data Availability Statement
The raw data supporting the conclusion of this article will be made available by the authors, without undue reservation.
Author Contributions
AH collected the samples and prepared the first draft. ED did the isotopes chemical analysis. MA prepared the samples for microprobe analysis. All the authors discussed the results and participated in the organization of the manuscript.
Funding
This research was supported by the CNPq (Conselho Nacional de Desenvolvimento Científico e Tecnológico, grants 620003/2006-8, 150132/2012-3, and 302.618/2016-3) and CLIMAZON (grant 295091).
Conflict of Interest
The authors declare that the research was conducted in the absence of any commercial or financial relationships that could be construed as a potential conflict of interest.
Publisher’s Note
All claims expressed in this article are solely those of the authors and do not necessarily represent those of their affiliated organizations or those of the publisher, the editors and the reviewers. Any product that may be evaluated in this article, or claim that may be made by its manufacturer, is not guaranteed or endorsed by the publisher.
References
Abouchami, W., Näthe, K., Kumar, A., Galer, S. J. G., Jochum, K. P., Williams, E., et al. (2013). Geochemical and Isotopic Characterization of the Bodélé Depression Dust Source and Implications for Transatlantic Dust Transport to the Amazon Basin. Earth Planet. Sci. Lett. 380, 112–123. doi:10.1016/j.epsl.2013.08.028
Allègre, C. J., Dupré, B., Négrel, P., and Gaillardet, J. (1996). Sr-Nd-Pb Isotopes Systematics in Amazon and Congo River Systems: Constrain about Erosion Processes. Chem. Geology. 131, 93–112. doi:10.1016/0009-2541(96)00028-9
Andersson, P. S., Dahlqvist, R., Ingri, J., and Gustafsson, O. R. (2001). The Isotopic Composition of Nd in a Boreal River: A Reflection of Selective Weathering and Colloidal Transport. Geochimica et Cosmochimica Acta 65 (4), 521–527. doi:10.1016/s0016-7037(00)00535-4
Artaxo, P., Gatti, L. V., Leal, A. M. C., Longo, C. M., Freitas, S. R., Lara, L. L., et al. (2005). Química atmosférica na Amazônia: A floresta e as emissões de queimadas controlando a composição da atmosfera amazônica. Acta Amazônica 35, 185–196. doi:10.1590/s0044-59672005000200008
Aubert, D., Stille, P., and Probst, A. (2001). REE Fractionation during Granite Weathering and Removal by Waters and Suspended Loads: Sr and Nd Isotopic Evidence. Geochimica et Cosmochimica Acta 65, 387–406. doi:10.1016/s0016-7037(00)00546-9
Babechuk, M. G., Widdowson, M., Murphy, M., and Kamber, B. S. (2015). A Combined Y/Ho, High Field Strength Element (HFSE) and Nd Isotope Perspective on basalt Weathering, Deccan Traps, India. Chem. Geology. 396, 25–41. doi:10.1016/j.chemgeo.2014.12.017
Balan, E., Allard, T., Fritsch, E., Sélo, M., Falguères, C., Chabaux, F., et al. (2005). Formation and Evolution of Lateritic Profiles in the Middle Amazon basin: Insights from Radiation-Induced Defects in Kaolinite. Geochimica et Cosmochimica Acta 69, 2193–2204. doi:10.1016/j.gca.2004.10.028
Bayon, G., German, C. R., Boella, R. M., Milton, J. A., Taylor, R. N., and Nesbitt, R. W. (2002). An Improved Method for Extracting marine Sediment Fractions and its Application to Sr and Nd Isotopic Analysis. Chem. Geology. 187, 179–199. doi:10.1016/s0009-2541(01)00416-8
Belousova, E. A., Griffin, W. L., O'Reilly, S. Y., and Fisher N, N. I. (2002). Igneous Zircon: Trace Element Composition as an Indicator of Source Rock Type. Contrib. Mineralogy Petrol. 143, 602–622. doi:10.1007/s00410-002-0364-7
Blum, J. D., and Erel, Y. (1997). Rb-Sr Isotope Systematics of a Granite Soil Chronosequences: The Importance of Biotite Weathering. Geochemica et Cosmochemica Acta 61, 3193–3204. doi:10.1016/s0016-7037(97)00148-8
Boulangé, B., and Carvalho, A. (1997). “The bauxite of Porto Trombetas,” in Brazilian Bauxites, USP/FAPESP/ORSTOM, Brazil. Editors A. Carvalho, B. Boulangé, A. J. Melfi, and Y. Lucas, 55–73.
Braun, J. J., Pagel, M., Herbillon, A., and Rosins, C. (1993). Mobilization and Redistribution of REE and Thorium in a Syenitic Lateritic Profile: A Mass Balance Study. Geochimica et Cosmochimica Acta 51, 4419–4434. doi:10.1016/0016-7037(93)90492-f
Braun, J. J., Pagel, M., Muller, J. P., Bilong, A. M., and Guillet, B. (1990). Cerium Anomalies in Lateritic Profiles. Geochimica et Cosmochimica Acta 54, 781–795. doi:10.1016/0016-7037(90)90373-s
Braun, J. J., Riotte, J., Battacharya, S., Violette, A., Prunier, J., Bouvier, V., et al. (2017). REY-Th-U Solute Dynamics in the Critical Zone: Combined Influence of Chemical Weathering, Atmospheric Deposit Leaching, and Vegetation Cycling (Mule Hole Watershed, South India). Geochem. Geophys. Geosystems 18, 4409–4425. doi:10.1002/2017gc007158
Cao, X., Chen, Y., Wang, X., and Deng, X. (2001). Effects of Redox Potential and pH Value on the Release of Rare Earth Elements from Soil. Chemosphere 44, 655–661. doi:10.1016/s0045-6535(00)00492-6
Capo, R. C., Stewart, B. W., and Chadwick, O. A. (1998). Strontium Isotopes as Tracers of Ecosystem Process: Theory and Methods. Geoderma 82, 197–225. doi:10.1016/s0016-7061(97)00102-x
Chabaux, F., Blaes, E., Stille, P., Roupert, R. C., Pelt, E., Dosseto, A., et al. (2013). Regolith Formation Rate from U-Series Nuclides: Implications from the Study of a Spheroidal Weathering Profile in the Rio Icacos Watershed (Puerto Rico). Geochimica et Cosmochimica Acta 100, 73–95. doi:10.1016/j.gca.2012.09.037
Costa, M. L., Cruz, G. S., Almeida, H. D. F., and Poellmann, H. (2014). On the Geology, Mineralogy and Geochemistry of the bauxite-bearing Regolith in the Lower Amazon basin: Evidence of Genetic Relationships. J. Geochemical Exploration 146, 58–74. doi:10.1016/j.gexplo.2014.07.021
Cunha, P. R. C., Gonzaga, F. G., Coutinho, L. F. C., and Feijó, F. J. (1994). Bacia Do Amazonas. Boletim Geociências da Petrobras 8, 47–55.
Daemon, R. F., and Contreiras, C. J. A. (1971). “Zoneamento palinológico da bacia Do Amazonas,” in Congresso Brasileiro de Geologia, 25o, São Paulo, 1971 (Anais. São Paulo: Sociedade Brasileira de Geologia), 3, 79–88.
Daemon, R. F. (1975). Contribuição à datação da Formacäo Alter do Chäo, bacia do Amazonas, 5. Rio de Janeiro: Revista Brasileira de Geociências, 58–84.
Gioia, S. M. C. L., and Pimentel, M. M. (2000). The Sm–Nd Isotopic Method in the Geochronology Laboratory of the University of Brasília. Anais da Academia Brasileira de Ciências 72, 219–245. doi:10.1590/s0001-37652000000200009
Guinoiseau, D., Fekiacova, Z., Allard, T., Druhan, J. L., Balan, E., and Bouchez, J. (2021). Tropical Weathering History Recorded in the Silicon Isotopes of Lateritic Weathering Profiles. Geophys. Res. Lett. 48, e2021GL092957. doi:10.1029/2021gl092957
Harlavan, Y., Erel, Y., and Blum, J. D. (2009). The Coupled Release of REE and Pb to the Soil Labile Pool with Time by Weathering of Accessory Phases, Wind River Mountains, WY. Geochimica and Cosmochimica Acta 73, 320–336. doi:10.1016/j.gca.2008.11.002
Honório, B. A. D., Horbe, A. M. C., and Seyler, P. (2010). Chemical Composition of Rainwater in Western Amazon — Brazil. Atmos. Res. 98, 416–425. doi:10.1016/j.atmosres.2010.08.001
Horbe, A. M. C., and Anand, R. (2011). Bauxite on Igneous Rocks from Amazon and Southwestern of Australia: Implication for Weathering Process. J. Geochemical Exploration 111, 1–12. doi:10.1016/j.gexplo.2011.06.003
Horbe, A. M. C., and Costa, M. L. (1999). Geochemical Evolution of Lateritic Sn. Zr. Th. Nb. Y and REE - Bearing Ore Body Derived from Apogranite: the Case of Pitinga. Amazonas - Brazil. J. Geochemical Exploration 66, 339–351. doi:10.1016/s0375-6742(99)00002-3
Horbe, A. M. C., and Costa, M. L. (2005). Lateritic Crusts and Related Oxisols in Eastern Brazilian Amazon. Geoderma 126, 225–239. doi:10.1016/j.geoderma.2004.09.011
Horbe, A. M. C. (2011). Oxygen and Hydrogen Isotopes in Pedogenic Minerals – Implications for Paleoclimate Evolution in Amazon during the Cenozoic. Geoderma 163, 178–184. doi:10.1016/j.geoderma.2011.03.017
Hoskin, P. W. O., and Schaltegger, U. (2003). The Composition of Zircon and Igneous and Metamorphic Petrogenesis. Rev. Mineralogy Geochem. 53, 27–62. doi:10.1515/9781501509322-005
Innocent, C., Michard, A., Malengreau, N., Loubet, M., Noack, Y., Benedetti, M., et al. (1997). Sr Isotopic Evidence for Ion-Exchange Buffering in Tropical Laterites from the Paraná, Brazil. Chem. Geology. 136, 219–232. doi:10.1016/s0009-2541(96)00145-3
Jacobsen, S. B., and Wasserburg, G. J. (1980). Sm–Nd Isotopic Evolution of Chondrites. Earth Planet. Sci. Lett. 50, 139–155. doi:10.1016/0012-821x(80)90125-9
Jonell, T. N., Li, Y., Blusztajn, J., Giosan, L., and Clift, P. D. (2018). Signal or Noise? Isolating Grain Size Effects on Nd and Sr Isotope Variability in Indus delta Sediment Provenance. Chem. Geology. 485, 56–73. doi:10.1016/j.chemgeo.2018.03.036
Laveuf, C., and Cornu, S. (2009). A Review on the Potentiality of Rare Earth Elements to Trace Pedogenetic Processes. Geoderma 154, 1–12. doi:10.1016/j.geoderma.2009.10.002
Lee, J. K. W., and Tromp, J. (1995). Self-induced Fracture Generation in Zircon. J. Geophys. Res. 100 (B917), 753-17–770. doi:10.1029/95jb01682
Liu, X-M., Rudnick, R. L., McDonough, W. F., and Cummings, M. L. (2013). Influence of Chemical Weathering on the Composition of the continental Crust: Insights from Li and Nd Isotopes in bauxite Profiles Developed on Columbia River Basalts. Geochimica et Cosmochimica Acta 115, 73–91. doi:10.1016/j.gca.2013.03.043
Lucas, Y. (1997). in The bauxite of Juriti. Editors A. Carvalho, B. Boulangé, A. J. Melfi, and Y. B. Lucasbauxites (USP/FAPESP/ORSTOM. Brazil), 107–136. doi:10.1097/00042307-199703000-00010
Lucas, Y. (2001). The Role of Plants in Controlling Rates and Products of Weathering: Importance of Biological Pumping. Annu. Rev. Earth Planet. Sci. 29, 135–163. doi:10.1146/annurev.earth.29.1.135
Ma, J., Wei, G., Xu, Y., and Long, W. (2010). Variations of Sr-Nd-Hf Isotopic Systematics in basalt during Intensive Weathering. Chem. Geology. 269, 376–385. doi:10.1016/j.chemgeo.2009.10.012
MacFarlane, A. W., Danielson, A., Holland, H. D., and Jacobsen, S. B. (1994). REE Chemistry and Sm-Nd Systematics of Late Archean Weathering Profiles in the Fortescue Group, Western Australia. Geochimica et Cosmochimica Acta 58, 1777–1794. doi:10.1016/0016-7037(94)90536-3
McDaniel, D. K., Hemming, S. R., McLennan, S. M., and Hanson, G. N. (1994). Resetting of Neodymium Isotopes and Redistribution of REE during Sedimentary Processes: The Early Proterozoic Chelmsford Formation, Sudbury Basin, Ontario, Canada. Geochimica et Cosmochimica Acta 58, 931–941. doi:10.1016/0016-7037(94)90516-9
Meyer, M., John, T., Brandt, S., and Klemd, R. (2011). Trace Element Composition of Rutile and the Application of Zr-In-Rutile Thermometry to UHT Metamorphism (Epupa Complex, NW Namibia). Lithos 126, 388–401. doi:10.1016/j.lithos.2011.07.013
Minarik, L., Anna, Z., Bendlb, J., Skrivan, P., and St'astn, M. (1998). The Behaviour of Rare-Earth Elements and Y during the Rock Weathering and Soil Formation in the Rıcany Granite Massif, Central Bohemia. Sci. Total Environ. 215, 101–111.
Moragues-Quiroga, C., Juilleret, J., Gourdol, L., Pelt, E., Perrone, T., Aubert, A., et al. (2017). Genesis and Evolution of Regoliths: Evidence from Trace and Major Elements and Sr-Nd-Pb-U Isotopes. Catena 149, 185–198. doi:10.1016/j.catena.2016.09.015
Nahon, D. B. (1991). Self-organization in Chemical Lateritic Weathering. Geoderma 51, 5–13. doi:10.1016/0016-7061(91)90063-y
Negrel, Ph., Guerrot, C., Cocherie, A., Azaroual, M., Brach, M., and Fouillac, C. (2000). Rare Earth Elements, Neodymium and Strontium Isotopic Systematics in mineral Waters: Evidence from the Massif Central, France. Appl. Geochem. 15, 1345–1367. doi:10.1016/s0883-2927(00)00008-1
Ohlander, B., Ingri, J., Land, M., and Schoberg, H. (2000). Change of Sm-Nd Isotope Composition during Weathering of till. Geochemical et Cosmochemical Acta 64, 813–820. doi:10.1016/s0016-7037(99)00365-8
Ohlander, B., Land, M., Ingri, J., and Widerlund, A. (1996). Mobility of Rare Earth Elements during Weathering of till in Northern Sweden. Appl. Geochem. 1, 93–99. doi:10.1016/0883-2927(95)00044-5
Pereira, V. P., Conceição, R. V., Formoso, M. L. L., and Pires, A. C. (2005). Alteration of Perovskite to Anatase in Silica-Under Saturated Rocks of the Catalão-I Carbonatite Complex, Brazil: a Raman Study. Revista Brasileira de Geociências 35, 239–244. doi:10.25249/0375-7536.2005352239244
Pidgeon, R. T., Nemchin, A. A., Roberts, M. P., Whitehouse, M. J., and Bellucci, j. J. (2019). The Accumulation of Non-formula Elements in Zircons during Weathering: Ancient Zircons from the Jack Hills, Western Australia. Chem. Geology. 530, 119310. doi:10.1016/j.chemgeo.2019.119310
Ram, R., Becker, M., Brugger, J., Etschmann, B., Burcher-Jones, C., Howard, D., et al. (2019). Characterisation of a Rare Earth Element- and Zirconium-Bearing Ion Adsorption clay deposit in Madagascar. Chem. Geology. 522, 93–107. doi:10.1016/j.chemgeo.2019.05.011
Retallack, G. J. (2010). Lateritization and Bauxitization Events. Econ. Geology. 105, 655–667. doi:10.2113/gsecongeo.105.3.655
Santoro, A.., Held, A., Linsinger, T. P. J., and Ricci, A. P. M. (2017). Comparison of Total and Aqua Regia Extractability of Heavy Metals in Sewage Sludge: The Case Study of a Certified Reference Material. Trends Anal. Chem. 89, 34–40. doi:10.1016/j.trac.2017.01.010
Santos, J. O. S., Hartmann, L. A., Gaudette, H. E., Groves, D. I., Mc Naughton, N. J., and Fletcher, I. R. (2000). A New Understanding of the Provinces of the Amazon Craton Based on Integration of Field Mapping and U-Pb and Sm-Nd Geochronology. Gondwana Res. 3, 453–488. doi:10.1016/s1342-937x(05)70755-3
Santos, J. O. S. (2003). “Geotectonics of the Guyana and Central Brazilian Shields,” in Geology, Tectonics, and Mineral Resources of Brazil, Text, Maps and GIS: Brasília, Brazil, Companhia de Pesquisa de Recursos Minerais. Editors L. A. Bizzi, C. Schobbenhaus, R. M. Vidotti, and J. H. Gonçalves, 169–226. ISBN 85-230-0790-3.
Santos, R. V., Sondag, F., Cochonneau, G., Lagane, C., Brunet, P., Hattingh, K., et al. (2014). “Source Area and Seasonal 87Sr/86Sr Variations in Rivers of the Amazon basin,” in Hydrological Process. (Published online in Wiley Online Library).
Silva, A. J. P., Lopes, R. C., Vasconcelos, A. M., and Bahia, R. B. C. (20032003). “Bacias Sedimentares Paleozóicas e Meso-Cenozóicas Interiores,” in Geologia, Tectônica e Recursos Minerais do Brasil, Capítulo II. Editors L. A. Bizzi, C. Schobbenhaus, R. M. Vidotti, and J. H. Gonçalves (Brasília: CPRM), 55–85. ISBN 85-230-0790-3, 674).
Souza, G. F., Reynolds, B. C., Kiczka, M., and Bourdon, B. (2010). Evidence for Mass-dependent Isotopic Fractionation of Strontium in a Glaciated Granitic Watershed. Geochimica et Cosmochimica Acta 74, 2996–2614. doi:10.1016/j.gca.2010.02.012
Stewart, B. W., Capo, R. C., and Chadwick, O. A. (1998). Quantitative Strontium Isotope Models for Weathering, Pedogenesis and Biogeochemical Cycling. Geoderma 82, 173–195. doi:10.1016/s0016-7061(97)00101-8
Stirling, C. H., Andersen, M. B., Potter, E-K., and Halliday, A. N. (2007). Low-temperature Isotopic Fractionation of Uranium. Earth Planet. Sci. Lett. 264, 208–225. doi:10.1016/j.epsl.2007.09.019
Su, N., Yang, S., Guo1, Y., Yue, W., Wang, X., Yin, P., et al. (2017). Revisit of Rare Earth Element Fractionation during Chemical Weathering and River Sediment Transport. Geochem. Geophys. Geosystems 18, 935–955. doi:10.1002/2016gc006659
Tardy, Y., and Roquin, C. (1998). Dérive des continents Paléoclimats at alterations tropicales. Orléans, FranceBRGM, 473.
Taylor, S. R., and McLenann, S. M. (1985). The Continental Crust its Composition and Evolution. Oxford: Blackwell Scientific Publications, 312.
Thieblemont, D., Guerrot, C., Negrel, Ph., Braucher, R., Bourles, D. L., and Thieblemont, R. (2014). Nd-isotope Evidence for the Distal Provenance of the Historical (C. <3000 BP) Lateritic Surface Cover Underlying the Equatorial forest. Gabon (Western Africa) 15, 177–192. doi:10.1016/j.aeolia.2014.06.002
Tripathy, G. R., Singh, S. K., and Krishnaswami, M., S. (2011). “Sr and Nd Isotopes as Tracers of Chemical and Physical Erosion Baskaran,” in Handbook of Environmental Isotope Geochemistry, Advances in Isotope Geochemistry (Springer-Verlag Berlin Heidelberg), 521–552. doi:10.1007/978-3-642-10637-8_26
Tyler, G. (2004). Rare Earth Elements in Oxisol and Plant Systems – A Review. Plant and Oxisol 267, 191–206. doi:10.1007/s11104-005-4888-2
Velbel, M. (1999). Bond Strength and the Relative Weathering Rates of Simple Orthosilicates. Am. J. Sci. 299, 679–696. doi:10.2475/ajs.299.7-9.679
Viers, J., and Wasserburg, G. J. (2004). Behavior of Sm and Nd in a Lateritic Soil Profile. Geochimica et Cosmochimica Acta 68, 2043–2054. doi:10.1016/j.gca.2003.10.034
Wei, X., Wang, S., Ji, H., and Shi, Z. (2018). Strontium Isotopes Reveal Weathering Processes in Lateritic Covers in Southern China with Implications for Paleogeographic Reconstructions. PLoS ONE 13, e0191780. doi:10.1371/journal.pone.0191780
West, A. J., Galy, A., and Bickle, M. (2005). Tectonic and Climatic Controls on Silicate Weathering. Earth Planet. Sci. Lett. 235, 211–228. doi:10.1016/j.epsl.2005.03.020
Wood, S. A. (1990). The Aqueous Geochemistry of the Rare-Earth Elements and Yttrium 1. Review of Available Low-Temperature Data for Inorganic Complexes and the Inorganic REE Speciation of Natural Waters. Chem. Geology. 82, 159–186. doi:10.1016/0009-2541(90)90080-q
Yang, M., Liang, X., Ma, L., Huang, J., He, H., and Zhu, J. (2019). Adsorption of REEs on Kaolinite and Halloysite: A Link to the REE Distribution on Clays in the Weathering Crust of Granite. Chem. Geology. 525, 210–217. doi:10.1016/j.chemgeo.2019.07.024
Zaitsev, A. N., Chakhmouradian, A. R., Siidra, O. I., Spratt, J., Williams, C. T., Stanley, C. J., et al. (2011). Fluorine-, Yttrium- and Lanthanide-Rich cerianite-(Ce) from Carbonatitic Rocks of the Kerimasi Volcano and Surrounding Explosion Craters, Gregory Rift, Northern Tanzania. Mineralogical Mag. 75, 2813–2822. doi:10.1180/minmag.2011.075.6.2813
Keywords: lateritic regolith, Oxisol, cerianite-(Ce), zircon, disorder kaolinite, rare earth element (REE)
Citation: Horbe AMC, Albuquerque MFDS and Dantas EL (2022) Nd and Sr Isotopes and REE Investigation in Tropical Weathering Profiles of Amazon Region. Front. Earth Sci. 10:845224. doi: 10.3389/feart.2022.845224
Received: 29 December 2021; Accepted: 21 February 2022;
Published: 25 March 2022.
Edited by:
Fabiano N. Pupim, Federal University of São Paulo, BrazilReviewed by:
Francisco Ladeira, State University of Campinas, BrazilIlya Bindeman, University of Oregon, United States
Copyright © 2022 Horbe, Albuquerque and Dantas. This is an open-access article distributed under the terms of the Creative Commons Attribution License (CC BY). The use, distribution or reproduction in other forums is permitted, provided the original author(s) and the copyright owner(s) are credited and that the original publication in this journal is cited, in accordance with accepted academic practice. No use, distribution or reproduction is permitted which does not comply with these terms.
*Correspondence: Adriana Maria Coimbra Horbe, YWhvcmJlQHVuYi5icg==