- 1School of Highway, Chang’an University, Xi’an, China
- 2Jiangsu Zhongshe Group Co.,Ltd., Wuxi, China
On the Qinghai–Tibet Plateau area, the permafrost and the wetlands are interdependent to form a symbiotic system, called permafrost wetlands (PWs). Due to the extremely complex hydrothermal conditions, the PWs greatly impact road stability. Thus, it is necessary to classify PWs in terms of engineering characteristics and evaluate their engineering stability. In this study, the typical diseases of subgrade in permafrost wetland areas are analyzed based on field investigation. Then, the permafrost type, waterlogged area ratio, and meadow development degree are used as the main indicators for classifying PWs by a three-level division method. Finally, a scheme for the engineering stability evaluation of PWs was established based on the fuzzy comprehensive theory and used for the pre-evaluation of the engineering stability for the proposed Qinghai 224 highway. The results indicate that the longitudinal cracks and uneven deformation are the main road diseases in this area, caused by the combined effect of permafrost, waterlogged areas, and meadow development. The PWs are divided into 15 types according to the engineering characteristics. Waterlogged area ratio and meadow development degree are proven to represent the development of underlying permafrost. The influencing factors include climatic environment, permafrost property, and wetland conditions, which have decreased influence on the engineering stability of PWs. The engineering stability of k230 + 100 and k255 + 400 is evaluated as basically stable and less stable, and the corresponding measures are adopted. At present, no significant damage occurred on the two road sections. The results suggest that the evaluation model in this article can be used to pre-evaluate the engineering stability of PWs.
Introduction
The Qinghai–Tibet Plateau is a typical region of high-altitude permafrost distribution in China and even the world. Due to the water blocking effect of the permafrost layer, rainfall, snowmelt, and surface runoff cannot infiltrate into the permafrost, resulting in the accumulation of water on the surface to form wetlands. The natural environment, vegetation characteristics, and permafrost combine to create the environmental sensitivity of permafrost wetlands (Bai et al., 2004; Wang et al., 2015), which significantly affects the stability of roads (Wang et al., 2004; Chai et al., 2017). On the Qinghai–Tibet Plateau, the road pavement instead of the original vegetation has disrupted the original hydrothermal balance of the wetlands and blocked surface runoff, thus exacerbating the degradation of the permafrost, which has led to a series of road disease problems, especially uneven settlement and longitudinal cracks (Wen et al., 2009; Mu et al., 2014; Li et al., 2015; Peng et al., 2015; Chang et al., 2016; Chai et al., 2017; Yang et al., 2019). Some scholars also pointed out that the thaw settlement of the permafrost beneath the roadway is one of the sources of the subgrade settlement (Qi et al., 2012; Yu et al., 2013). In addition, the wetlands features are also conducive to the development of road diseases. The accumulation of water in the soil layer leads to water migration to the subgrade, causing road diseases with seasonal freezing and thawing (Mao et al., 2010). Meantime, the low temperature of PWs promotes the formation of a peat layer with high compressibility. The settlement caused by the compression of the peat layer is also part of the subgrade settlement. It can be found that these road diseases are closely related to the type and stability of the permafrost and wetlands conditions. The permafrost state is not only influenced by road constructions but also closely related to the wetland conditions (Liu et al., 2009; Chang et al., 2012). It can be seen from the above analysis that the permafrost and wetlands both interact and contribute to each other, and together they affect road stability. Thus, the engineering characteristics of PWs on the Qinghai–Tibet Plateau require attention.
In order to better understand the characteristics of wetlands on the Qinghai–Tibet Plateau, many researchers proposed the classification system in terms of causes or features (Ni et al., 1998; Sun, 2003). Meantime, several scholars proposed classification systems at different spatial scales. Li et al. (1998) classified the wetlands in the Three-River Source region into moor, lake, river, and riverbed. Zhao (1999) classified the alpine wetlands into peat marshes and submerged marshes based on the presence or absence of peat accumulation and then divided them into 11 marsh subtypes based on geomorphological conditions and water recharge types. Sun (2003) classified the wetlands of the Qinghai–Tibet Plateau into grass wetlands, forested wetlands, river wetlands, and lake wetlands according to the Ramsar Convention. Chen et al. (2002) added artificial wetlands on the basis of 3 basic natural wetland types—lake wetland, swamp wetland, and river wetland—according to the basic hydrological, biological, and soil characteristics of plateau wetlands. Zhang et al. (2010) proposed a revised 4-class classification system of alpine wetlands based on remote sensing and classified the wetlands into 13 subcategories, such as river, weedy wet meadow, hippophae rhamnoides, beach gravel, lake, and Phragmites australis marsh. Although the classification systems considering the causes, features, and space of wetlands on the Qinghai–Tibet Plateau have been widely documented, little information exists paying attention to the engineering characteristics of wetlands.
The engineering characteristics of wetlands significantly affect the stability of permafrost wetlands. The traditional road safety assessment system mainly adopts the safety factor as the evaluation criterion. The evaluation results have great inaccuracy due to the uncertainty of geotechnical parameters. In order to overcome this problem, Qi et al. (2006) proposed a calculation method to calculate the reliability index and failure probability based on a genetic algorithm for the thawing settlement of the permafrost subgrade. However, road stability is related to geological and climatic conditions, regulation measures, and so on. Huo et al. (2010) selected the annual ground temperature, permafrost type, artificial permafrost table, drainage condition of subgrade, and method of special adjustment as the key factors and established the linguistic variables and fuzzy membership function to describe the relationship between the factors and the road diseases. Meanwhile, Qi et al. (2007) and Miao et al. (2018) established a prediction model of subgrade deformation considering ground temperature, subgrade height, and permafrost table using a BP neural network. For the special engineering measures for subgrade in permafrost areas, the treatment effect was evaluated using fuzzy theory and catastrophe progression method considering the natural environment, frozen soil properties, and engineering measures (Cao et al., 2015; Zhao et al., 2019; Luo et al., 2021). It can be seen that the current research mostly focuses on the evaluation of subgrade stability in permafrost areas and rarely considers the impact of permafrost wetlands on road stability. Therefore, it is necessary to evaluate the engineering stability of the PWs in order to take appropriate treatment measures to strengthen the wetland foundation.
This study proposes the classification system of permafrost wetlands according to engineering characteristics. Furthermore, the engineering stability of permafrost wetlands is evaluated based on the fuzzy comprehensive evaluation model. The results provide an important reference to understanding the role of permafrost wetlands in engineering construction.
Permafrost Wetlands and Typical Diseases of Subgrade in Study Area
The study area is located in the center of the Qinghai–Tibet Plateau and the Midwest of Yushu, with an average altitude of 4179.1 m, belonging to a typical alpine climate. The road traverses many wetlands with meadows covering the surface, waterlogged areas, and permafrost existing underground, which can be called permafrost wetland (PWs), as shown in Figure 1. The developed modern glaciers and glacial landforms, dense rivers and lakes, abundant ice-snow meltwater, alpine climate, and the prevalence of the permafrost have contributed to the formation and development of wetlands on the Qinghai–Tibet Plateau.
Based on field investigation, the strata of PWs are composed of the grass layer with grass roots, peat layer with an inadequate decomposition of plant residues, powdered clay interspersed with gravel, and permafrost layer, as shown in Figure 2. The wetlands and the permafrost underneath constitute a symbiotic system (Sun, 2000; Sun et al., 2008), which is mainly manifested in two aspects: the protection and enhancement of peat and grass layer to permafrost and the promotion of permafrost to peat and grass layer. Vegetation has been found to strongly protect frozen soil from incoming radiation, thereby insulating the soil from ambient conditions (Kudelyacev et al., 1977; Kelly et al., 2004; Cannone and Guglielmin, 2009; Anderson et al., 2019; Fedorov et al., 2019). Moreover, the grass-root layer can reduce the surface heat transfer into a deep stratum due to the poor thermal conductivity. Meantime, the role of ground-hugging vegetation and plant roots in water absorption and retention enables the frozen soil to reach a material-energy balance and ecological equilibrium (Niu et al., 2019). When the temperature rises, the peat layer consumes heat by evaporating water to slow down the transmission of the cold storage of permafrost to the surface. When the temperature drops to negative temperature, the peat layer in a frozen state, due to its higher thermal conductivity, can transfer the cold energy deeper into the soil to promote cold storage of the permafrost (Sun, 2000). The permafrost layer can effectively block water infiltration, allowing snowmelt, precipitation, and river water to accumulate on the surface, forming a waterlogging area, which facilitates the continuity of the soil swampy process. Instead, when the surface vegetation is destroyed, its functions of heat insulation, heat preservation, and water storage are weakened or disappear (Iwahana et al., 2003; Wang et al., 2008), causing heat and water to enter directly into the subsurface, which is manifested by enhanced vertical transport of water and heat. Eventually, the underlying permafrost degrades or disappears, which affects the existence of wetlands leading to the degradation or disappearance (He et al., 2009; Luo et al., 2012). The symbiotic mechanism existing between wetlands and permafrost is a key factor in forming the special geological environment of the Tibetan Plateau. It was found that permafrost on the Qinghai–Tibet Plateau is degrading year by year due to climate warming and construction of ground engineering (Luo et al., 2012; Han et al., 2018; Sheng et al., 2020), which in turn affects the development of wetlands (Wang et al., 2010; Liu et al., 2019).
Based on the above analysis, it is clear that the complex characteristics of permafrost wetlands are the main challenge to be overcome for road construction, which leads to a series of road disease problems. In this study area, the longitudinal cracks and the uneven deformation are the main forms of road diseases according to the field investigation (Figure 3). The black asphalt pavement breaks the original hydrothermal equilibrium of the wetlands, and the input of a large amount of heat energy makes the permafrost under the subgrade in a state of degradation. The thawing of the permafrost layer leads to the uneven compression deformation of the subgrade, which is the main internal cause of the uneven settlement and longitudinal cracks. In addition, the developed permafrost wetlands have lush grass, and the soil is wet or overwet all year-round, which leads to the active migration of moisture to the subgrade. The surface water infiltrated by the road shoulder and slope foot carries much heat, resulting in a relative decrease of the permafrost table. Furthermore, water gathers near the permafrost table to form a thick ice layer under the road shoulder (Mao et al., 2010). Finally, the longitudinal cracks and uneven settlement are generated in the subgrade under the coupling action of heat and water. It indicates that the combination of the permafrost, waterlogged area, and meadow development contributes to road diseases.
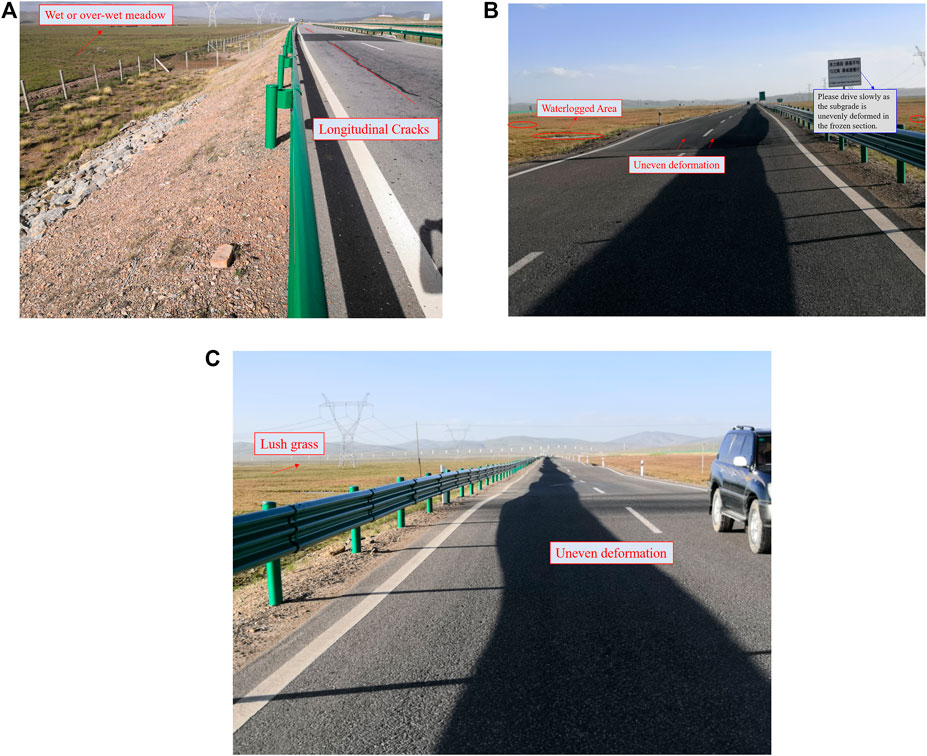
FIGURE 3. Diseases of the road in the permafrost wetland area. (A) Longitudinal cracks. (B) Uneven deformation in frozen section. (C) Uneven deformation in developed meadow areas.
Type Classification of Permafrost Wetlands
Determination of Classification Index
For the actual engineering project, the classification of PWs should comprehensively consider and analyze the various elements that affect road stability. Based on a field investigation, the permafrost type, waterlogged area ratio, and meadow development degree were selected to be the classification indexes, which considered the effects of permafrost, meadow, and water on the project construction. The permafrost type was identified by the field drilling method. The waterlogged area ratio was obtained by the aerial survey and micro-geomorphology extraction technology. The thickness of the vegetation layer was determined by field measurement.
Permafrost Type
The types of permafrost are the products of the comprehensive action of various natural conditions, reflecting the characteristics of seasonal thaw layer and permafrost, which are the main factor for subgrade design in the permafrost area. Some scholars take permafrost type and ground temperature as the main zoning index (Cheng and Wang, 1982). In addition, the stability of the subgrade in the permafrost region depends on the ice content of the permafrost under the roadway (Wen et al., 2009; Xu, 2014). In China, “Specifications for Design of Highway Subgrades” classifies permafrost according to the volume ice content, which can be divided into ice-poor soil, icy soil, ice-rich soil, saturated frozen soil, and ice layer interspersed with soil, as shown in Table 1.
Waterlogged Area Ratio
In the permafrost area, the permafrost layer hinders the infiltration of surface water. Combined with the low-lying terrain and poor drainage conditions, this often increases the water in the soil and forms the waterlogged areas in severe cases. However, the water on both sides of the road infiltrates into the subgrade, and with the freeze-thaw process, it is easy to form a thick pure ice layer inside the subgrade leading to disease problems (Mao et al., 2010). Furthermore, the upper soil layer in the waterlogged area is in an oversaturated state, which can lead to a reduction in its bearing capacity. The consolidation of the upper soil layer is a key factor causing the subgrade settlement. Therefore, it is necessary to pay attention to water accumulation along the road during the road design, construction, and operation and set up drainage facilities or intercept facilities in advance.
Figure 4 shows different waterlogging conditions of wetlands along the road in the study area. It can be seen that the road crosses PWs with different waterlogging conditions. Moreover, the percentage of waterlogged areas is positively correlated with vegetation development; that is, the larger the percentage, the more developed the vegetation. The reason is that water can promote vegetation growth, which shows that the occurrence and development of surface vegetation, water, and permafrost are interdependent and mutually influential relationships. The waterlogging condition of the PWs was evaluated according to the percentage of waterlogged area ns: almost no waterlogging (ns <5%), less waterlogging (5% ≤ ns <25%), more waterlogging (25% ≤ ns <55%), and severe waterlogging (ns ≥ 55%).
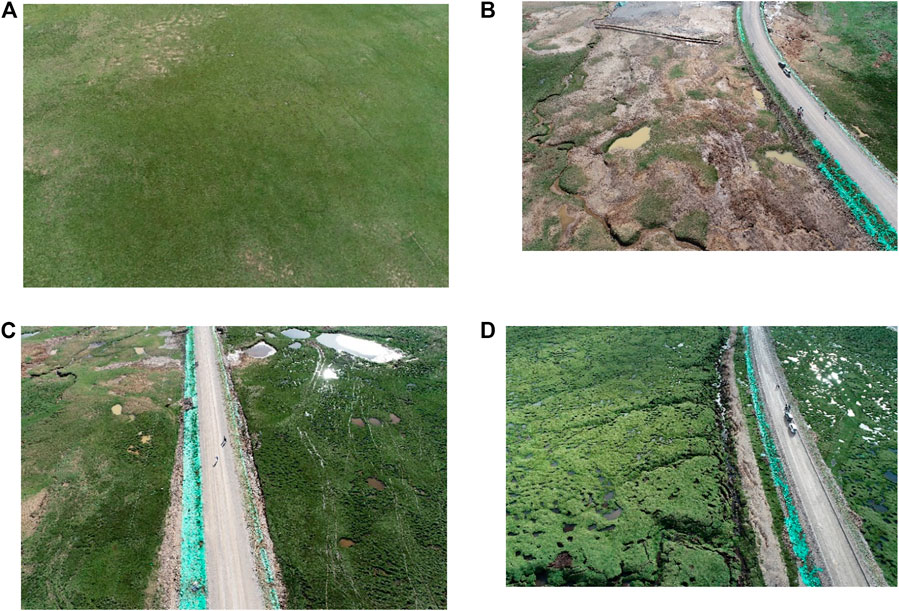
FIGURE 4. Different waterlogged areas in permafrost wetlands along the road. (A) Almost no waterlogging. (B) Less waterlogging. (C) More waterlogging. (D) Severe waterlogging.
Meadow Development Degree
Vegetation has a significant influence on the hydrothermal processes of permafrost (Wu et al., 2003; Kelly et al., 2004), although the permafrost restricts the growth of some plants owing to low temperature, water isolation, and other restricts. For a long time, an ecological balance has been established between vegetation and permafrost, and vegetation is often used as an indicator of underlying permafrost and thawing soil (Chang et al., 2012). In this study, the meadow development degree is expressed by the thickness of vegetation h, measured from the top of vegetation to the bottom of grass root. According to the field survey findings, the meadow development degree can be divided into four cases: undeveloped (h < 8 cm), basically developed (8 cm ≤ h < 15 cm), developed (15 cm ≤ h < 40 cm), and fairly developed (h ≥ 40 cm), as shown in Figure 5. It can be seen that the more developed the meadow, the higher the ice content of the permafrost. This result indicates that the meadow development degree can be used as an indicator of the permafrost type on the Qinghai–Tibet Plateau wetlands.
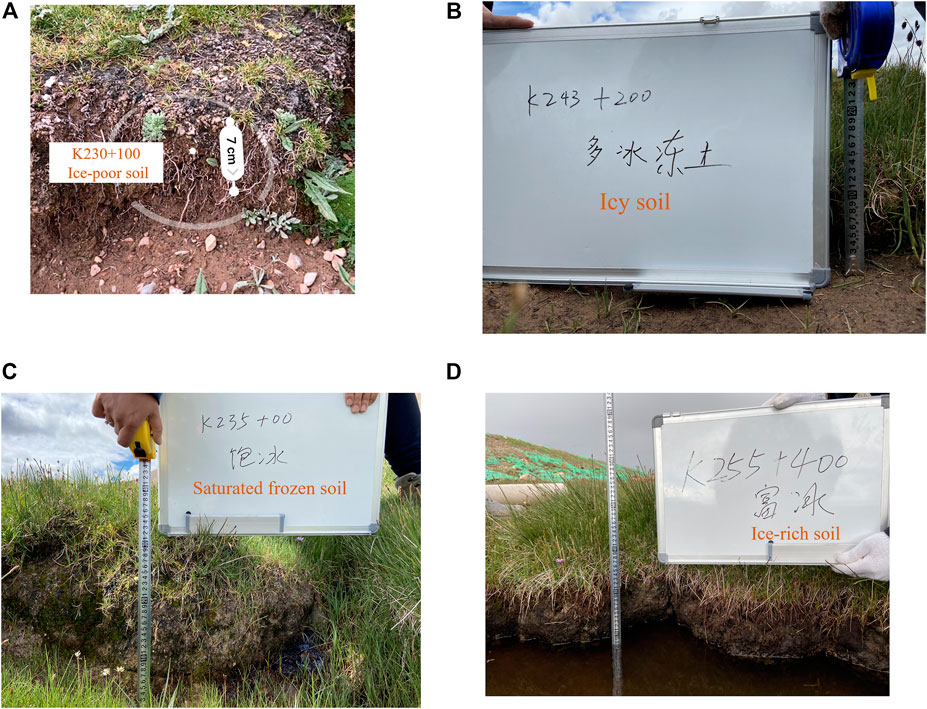
FIGURE 5. Meadow development degree of different areas along the road. (A) Undeveloped (h = 7 cm). (B) Basically developed (h = 21 cm). (C) Developed (h = 39 cm). (D) Fairly developed (h = 55 cm).
Extraction of Waterlogged Areas
Based on the field survey and exploration data, the typical road sections with different permafrost types and waterlogged areas were selected. Then, aerial surveys are conducted on typical road sections to extract the waterlogged areas of specific areas.
The unmanned aerial vehicle (UAV) used for this study is a DJI Genie 4 pro v2.0 with a 20-megapixel FC6310 digital camera. The topographic data of the wetlands along the road are collected by shooting at an altitude of 60 m above the study area. The aerial photography area was a rectangle of 150 m on each side perpendicular to the roadbed centerline and 300 m along the roadbed centerline. In the flight, the ground resolution (GSD) of 1.64 cm/pixel was applied according to the requirements of 1:500 mapping scale. In order to get better results during the flight, the fore-and-aft overlap was set to 80% and the side overlap was set to 70% to facilitate image stitching and generation of elevation points.
The orthophotos taken by the UAV were calibrated by Global Mapper and then imported into Image-Pro Plus for micro-geomorphology extraction, as shown in Figure 6. The waterlogged area ratio ns can be computed by the following equation:
where si is the area of a single waterlogged area, i = 1, 2, … , n; n is the number of identified waterlogged areas; s is the total area of the selected area, m2; sw is the waterlogged area of the selected area, m2.
The calculation of ns is mainly done by extracting the micro-geomorphology from the actual captured 2D model images, identifying the waterlogged area in the specified area, and then counting the proportion of waterlogged area in the specific area. According to the image recognition results, the waterlogged area ratio near the typical road sections was calculated, as shown in Table 2. The analysis found a spatial correlation between the waterlogged area ratio and the type of permafrost. The larger the waterlogged area ratio, the higher the ice content of the underlying permafrost, which indicates that the waterlogged area ratio can be used as an indicator of different permafrost types.
Classification of Permafrost Wetlands
This classification system belonging to the small-scale classification model integrates the effect of permafrost, moisture, and vegetation on the engineering characteristics of permafrost wetlands. The permafrost wetlands are divided into 15 types using the three-level division, composed of the permafrost type, waterlogged area ratio, and meadow development degree, as shown in Table 3. The permafrost wetlands' characteristics are quantitatively reflected by a combination of letters and numbers. The first letter represents the classfication index, such as W represents the waterlogged area ratio, P represents the permafrost type, and M represents the meadow development degree. The numbers represent the development degree of each index. For waterlogged area ratio, 1∼4 represent ns<5%, 5%≤ns <25%, 25%≤ns<55% and ns≥55%, respectively. For permafrost type, 1∼5 represent ice-poor soil, icy soil, ice-rich soil, saturated frozen soil, and ice layer interspersed with soils, respectively. For meadow development degree, 1∼4 represent h<8 cm, 8 cm≤ h<15 cm, 15 cm≤h<40 cm, and h≥ 40 cm, respectively. For example, W3 means the proportion of the waterlogged area is 25∼55%, P4 means the permafrost type is saturated frozen soil, and M3 means the degree of meadow development is 15∼40 cm. Thus, W3-P4-M3 represents the permafrost wetlands having more waterlogging and the developed meadows, and the underlying permafrost is saturated frozen soil. The larger number indicates the greater degree of influence of a factor on the wetland's formation. Correspondingly, the more susceptible the permafrost wetlands are to weakness and less carrying capacity.
Evaluation of Engineering Stability of Permafrost Wetlands Based on Fuzzy Theory
For evaluating the engineering stability of permafrost wetlands, the analytic hierarchy process (APH) and fuzzy comprehensive evaluation are applied to appropriately reduce the randomness of subjective judgment and improve the reliability of the evaluation results.
Construction of Index System of the Foundation Stability
The construction of the index system needs to meet the systematic, scientific, operable, and practical principles. Firstly, the index system can accurately and fully reflect the situation of the evaluation object through qualitative or quantitative methods. In addition, the difficulty of quantifying the indicators should be considered. It is required that the scoring methods and standards should be clear and easy to operate while ensuring that the evaluation results are as objective, comprehensive, and fair as possible. Based on the above analysis of factors affecting PWs formation, the hierarchical substructure model for PWs stability is established, as shown in Figure 7. This hierarchical substructure model consists of three levels: object hierarchy, rule hierarchy, and factor hierarchy. Rule hierarchy includes climatic environment, permafrost property, and wetlands conditions, controlled by the elements in factor hierarchy. The elements in the factor hierarchy are the evaluation indexes of the engineering stability for PWs.
Establishment of Fuzzy Comprehensive Evaluation Model
Determination of Weight
The reasonableness of weight determination directly affects the accuracy and scientificity of the evaluation results. Firstly, the judgment matrix should be established, which is the evaluation for objective factors. Each value in the matrix was marked by a number from 1 to 9 or its reciprocal and characterized the relative importance of each element. The larger the number, the more important this factor is relative to other factors. Assume that Ai denotes the kth element in the rule hierarchy (i = 1, 2, … , n), and the element in factor hierarchical is Bij (j = 1, 2, … , m). The relative importance of elements Bi1, Bi2, … , Bim is determined by pairwise comparison and is quantified as blk (l = 1, 2, … , m; k = 1, 2, … , m). The relative importance of each element is determined by an expert questionnaire survey. The judgment matrix can be expressed as
where blk represents the importance of factor Bil relative to Bik and blk is determined as
The judgment matrix is built to make the judgment process and conclusion mathematical and quantifiable. Then, the weight value of each factor is calculated based on the judgment matrix. The calculation steps are as follows:
First, the judgment matrix Bm is normalized to obtain matrix
And then, each line of the normalized matrix
The vector W is normalized to obtain the eigenvector vector
Finally, each value in the eigenvector vector
Consistency Checking of the Judgment Matrix
The consistency checking of the judgment matrix is performed to ensure the rationality of weight value. The consistency index of the judgment matrix can be written as
where
where RI is the mean random consistency index, as shown in Table 4. When CR < 0.1, it indicates that the degree of inconsistency of judgment matrix Bm is within the allowed range and the weight value is reasonable. Thus, the weight value of factors can be applied in the stability evaluation calculations.
Establishment of Multi-Level Fuzzy Comprehensive Evaluation Model
The fuzzy comprehensive evaluation method belongs to the category of fuzzy mathematics. Based on the principle of affiliation of fuzzy mathematics, the qualitative problem is transformed into a quantitative problem to make an overall evaluation of the complex system. The steps are as follows:
(1) Determine the influencing factor set and evaluation set.
Assume that
(2) Construct the single factor evaluation matrix
The membership degree
In this study, the value limit of each factor evaluation can be uniformly expressed as < v1, v1∼ v2, v2∼ v3, > v4, and the membership functions are shown in the following equations:
(3) Determine the weight vector of evaluation factors.
In fuzzy evaluation, the weight vector
(4) Single-level fuzzy comprehensive evaluation.
For the weight vector W and the single-factor judgment matrix R, the fuzzy operation
(5) Multi-level fuzzy comprehensive evaluation.
In this study, the index system has three levels: the object set U, the rule set Ui (
Evaluation of Engineering Stability of Permafrost Wetlands
The evaluation set V = {stable, basically stable, less stable, unstable} is established based on the engineering stability of permafrost wetlands in alpine and high-altitude areas. Further, quantify the evaluation set and assign the value to
Based on the field investigation, many references, and experts’ opinions, the evaluation standards for the grading of each evaluation index in engineering stability of PWs are given in Table 6.
Case Study
The k230 + 100 and k255 + 400 are selected from the study’s road—the Qinghai 224 highway—for the engineering stability evaluation of the permafrost wetlands. In this area, precipitation is mainly concentrated from late May to late September, accounting for more than 80% of the total annual precipitation. The annual average precipitation is 409 mm, the annual average evaporation is 1401.8 mm, and the annual solar radiation is about 6200 MJ/m2 (Wang et al., 2015; Bao et al., 2017). The engineering stability of the wetlands passed through by the selected sections is evaluated. Meantime, the corresponding treatment measures are proposed. The type of wetlands and the property of permafrost are shown in Table 7.
The fuzzy evaluation method is used to evaluate the factors affecting the engineering stability of PWs. According to the above survey results and the calculation method for membership degree, the single factor evaluation matrix for each factor set is determined, as shown in Table 8.
The judgment matrix for each set can be determined by expert questionnaire survey and reference; refer to Tables 9–12. The weight value can be obtained by a judgment matrix to indicate the relative importance of each factor. The weight values are given in Table 13. According to the results obtained by the AHP method, the weight values of the climatic environment, permafrost property, and wetlands conditions are 0.1638, 0.2973, and 0.5389, respectively. In the factor set, the relative weights of waterlogged area ratio, meadow development degree, mean annual ground temperature, mean annual precipitation, and permafrost type are 0.3593, 0.1796, 0.1602, 0.0913, and 0.0884, respectively, and the impact on the engineering stability of PWs is reduced in turn.
Through the fuzzy comprehensive evaluation model, the evaluation results of PWs near the two stake numbers are obtained, as shown in Table 14. According to the stability index, the engineering stability of PWs near stake numbers k230 + 100 and k255 + 400 is basically stable and less stable, respectively. The area near stake number k230 + 100 belongs to the ice-poor soil area. Foundation soil has weak frost heaving and weak thawing characteristics. The surface was directly impacted by the impact compaction roller without removing the vegetation. Then, backfill the sand gravel to the original surface elevation. After settlement stabilization, fill the sand gravel to the bottom of the pavement layer. The area near stake number k255 + 400 belongs to the ice-rich soil area, with a wet and soft layer thickness of about 0.5 m. Due to its poor bearing characteristics, this layer was reinforced by riprap with a thickness of 0.5 m. Then, the impact compaction technology was applied to squeeze the silt out. Finally, the gravel with 1.0 m thickness was filled with heavy rolling. The engineering stability of the foundation has been enhanced after the treatments. Therefore, the fuzzy comprehensive evaluation model used to evaluate the engineering stability of permafrost wetlands has certain reliability.
Conclusion
In this study, the permafrost type, waterlogged area ratio, and meadow development degree were selected to quantify the engineering characteristics of permafrost wetlands. A three-level classification system was applied to classify the types of permafrost wetlands. Based on these analyses and field investigation, a fuzzy comprehensive evaluation model for engineering stability of permafrost wetlands was established, and a case study was conducted to verify the rationality of the evaluation model. The following conclusions can be drawn:
(1) Field investigation indicates that the permafrost layer and the wetlands constitute a symbiotic system on the Qinghai–Tibet Plateau. The permafrost layer facilitates the formation and development of wetlands due to the water isolation effect of the permafrost layer. Under the combined effect of permafrost, waterlogged area, and meadow development, the longitudinal cracks and the uneven deformation are the main forms of road diseases.
(2) The permafrost wetlands can be divided into 15 types using a three-level division considering the engineering characteristics. The permafrost type, meadow development degree, and waterlogged area ratio were selected as the classification indexes.
(3) According to the results obtained by the AHP method, the degree of impact on wetland stability is wetlands conditions > permafrost property > climatic environment, and the waterlogged area ratio, meadow development degree, mean annual ground temperature, mean annual precipitation, and permafrost type are the main factors that affect wetland stability.
(4) The method of combining AHP and fuzzy comprehensive evaluation can effectively evaluate the engineering stability of the permafrost wetlands and can be used to pre-evaluate the engineering stability of permafrost wetlands.
Data Availability Statement
The raw data supporting the conclusions of this article will be made available by the authors without undue reservation.
Author Contributions
XM and QW conceived this research. XM and LH contributed to conception and field investigation. WH and YZ contributed to data analysis and methodology. YZ wrote the first draft of the manuscript. All authors contributed to manuscript revision and read and approved the submitted version.
Funding
This research was supported by the reconstruction project of Qinghai Provincial Highway 224, the Annual Science and Technology Plan of Inner Mongolia Transportation Department–Research on Key Technologies of Island Frozen Soil Composite Foundation at High-Latitude and Low-Altitude, and the Science and Technology Project of Shaanxi Province (2021JQ-244).
Conflict of Interest
LH was employed by the company Jiangsu Zhongshe Group Co., Ltd.
The remaining authors declare that the research was conducted in the absence of any commercial or financial relationships that could be construed as a potential conflict of interest.
Publisher’s Note
All claims expressed in this article are solely those of the authors and do not necessarily represent those of their affiliated organizations or those of the publisher, the editors, and the reviewers. Any product that may be evaluated in this article, or claim that may be made by its manufacturer, is not guaranteed or endorsed by the publisher.
Reference
Anderson, J. E., Douglas, T. A., Barbato, R. A., Saari, S., Edwards, J. D., and Jones, R. M. (2019). Linking Vegetation Cover and Seasonal Thaw Depths in interior Alaska Permafrost Terrains Using Remote Sensing. Remote Sensing Environ. 233, 111363. doi:10.1016/j.rse.2019.111363
Bai, J. H., Ouyang, H., Xu, H. F., Zhou, C. P., and Gao, J. Q. (2004). Advances in Studies of Wetlands in Qinghai-Tibet Plateau. Prog. Geogr. 23 (04), 1–9. doi:10.11820/dlkxjz.2004.04.001
Bao, G. Y., Zhang, J., Zhou, D., Ma, S. C., and Liu, W. (2017). Analysis of the Spatiotemporal Characteristics of Solar Radiation Intensity in Qinghai Province. J. Glaciology Geocryology 03, 563–571. doi:10.7522/j.issn.1000-0240.2017.0063
Cannone, N., and Guglielmin, M. (2009). Influence of Vegetation on the Ground thermal Regime in continental Antarctica. Geoderma 151 (3-4), 215–223. doi:10.1016/j.geoderma.2009.04.007
Cao, W., Chen, J., Zhang, B., Wu, J. C., Li, J., and Sheng, Y. (2015). Fuzzy Evaluation of the Effect of Rubble Roadbed Engineering in Permafrost Regions along the Chaidaer-Muli Railway in Qinghai Province. J. Glaciology Geocryology 37 (6), 1555–1562. doi:10.7522/j.isnn.1000-0240.2015.0172
Chai, M. T., Zhang, J. M., Mu, Y. H., Liu, G., and Zhou, G. Q. (2017). Probability Model for Subgrade Hazards Susceptibility of Qinghai-Tibet Highway in Permafrost Regions. J. Chang’an Univ. (Natural Sci. Edition) 37 (04), 76–83. doi:10.19721/j.cnki.1671-8879.2017.04.010
Chang, X. L., Jin, H. J., Wang, Y. P., Zhou, G. Y., Che, F. Q., and Zhao, Y. M. (2012). Influences of Vegetation on Permafrost: a Review. Acta Eco Sin 32 (24), 7981–7990. doi:10.5846/stxb201202120181
Chang, Y. T., Chen, Z. D., Zhang, Z., and Chen, D. G. (2016). Analysis on Influencing Factors of Typical Subgrade Diseases of Qinghai Tibet Highway. J. China Foreign Highw. 01, 19–22. doi:10.14048/j.issn.1671-2579.2016.01.005
Chen, G. C., Huang, Z. W., Lu, X. F., and Peng, M. (2002). Characteristics of Wetland and its Conservation in the Qinghai Plateau. J. Glaciology Geocryology 03, 254–259. doi:10.3969/j.issn.1000-0240.2002.03.005
Cheng, G. D., and Wang, S. L. (1982). On the Zonation of High-Altitude Permafrost in China. J. Glaciology Geocryology 02, 1–17. http://www.bcdt.ac.cn/CN/Y1982/V4/I2/1.
Fedorov, A. N., Konstantinov, P. Y., Vasilyev, N. F., and Shestakova, A. A. (2019). The Influence of Boreal forest Dynamics on the Current State of Permafrost in Central Yakutia. Polar Sci. 22, 100483. doi:10.1016/j.polar.2019.100483
Guo, D. X., Ma, S. M., Ding, D. W., and Liu, J. R. (1992). Translated. Lanzhou, China: Lanzhou University Press, 96–131.
Han, L. W., Yang, Y. H., Dai, L. S., Zhang, F., and Cheng, J. (2018). Variation of Permafrost on Fill Embankments along Qinghai-Tibet Railway. China Saf. Sci. J. S2, 6–10. doi:10.16265/j.cnki.issn1003-3033.2018.S2.002
He, R. X., Jin, H. J., Lv, L. Z., Yu, S. P., Chang, X. L., et al. (2009). Recent Changes of Permafrost and Cold Regions Environments in the Northern Part of Northeastern China. J. Glaciology Geocryology 31 (03), 525–531. http://www.bcdt.ac.cn/CN/Y2009/V31/I3/524.
Huo, M., Chen, J. B., Zhu, D. P., and Zhang, J. Z. (2010). Study of Early Warning on Roadbed Diseases of Qinghai-Tibet Highway in Permafrost Regions. Rock Soil Mech. 31 (01), 331–336. doi:10.16285/j.rsm.2010.01.012
Iwahana, G., Fukuda, M., Kobayashi, Y., and Fedorow, A. N. (2003). “A Comparative Study of the Surface and Active Layer Conditions at Disturbed forest Site Near Yakutsk,” in Proceedings of the 8th International Conference on Permafrost, London, June 16, 2003 (Taylor and Francis Group publication), 483–488.Vol. 1
Kelley, A. M., Epstein, H. E., and Walker, D. A. (2004). Role of Vegetation and Climate in Permafrost Active Layer Depth in Arctic Tundra of Northern Alaska and Canada. J. Glaciology Geocryology (s1), 269–274.
Kudelyacev, V. A., Garagulya, L. S., and Melamed, V. (1977). Fundamentals of Frost Forecasting in Geological Engineering Investigations. Nauka, Moscow: CRREL, Hanover, NH, 489.
Li, J. P., Wang, Z., Zhang, Y., and Yuan, K. (2015). Cause Analysis on Uneven Deformation of Embankment in Permafrost Regions. China J. Highw. Transport 28 (12), 78–85+91. doi:10.19721/j.cnki.1001-7372.2015.12.011
Li, L. X., Zhou, X. M., Wang, Q. J., and Zhou, Z. J. (1998). Jiangheyuan diqu de shidi jiqi zhuyao shengwu leiqun. J. Qinghai Environ. 8 (3), 108–114.
Liu, G. S., Wang, G. X., Hu, H. C., Li, T. B., Wang, J. F., et al. (2009). Influence of Vegetation Coverage on Water and Heat Processes of the Active Layer in Permafrost Regions of the Tibetan Plateau. J. Glaciology Geocryology 31 (001), 89–095. http://www.bcdt.ac.cn/CN/Y2009/V31/I1/89.
Liu, Z. W., Li, S. N., Wei, W., and Song, X. J. (2019). Research Progress on Alpine Wetland Changes and Driving Forces in Qinghai-Tibet Plateau during the Last Three Decades. Chin. J. Ecol. 03, 856–862. doi:10.13292/j.1000-4890.201903.002
Luo, D. L., Jin, H. J., Lin, L., He, R. X., Yang, S. Z., and Chang, X. L. (2012). Degradation of Permafrost and Cold-Environments on the Interior and Eastern Qinghai Plateau. J. Glaciology Geocryology 03, 538–546. http://www.bcdt.ac.cn/CN/Y2012/V34/I3/538.
Luo, X. -x., Ma, Q. -g., and Jiang, H. -q. (2021). Pre-evaluation on Stability of Proposed Expressway Embankment with Existing Geothermal Regulation Measures in Permafrost Regions. J. Cent. South. Univ. 28 (01), 264–283. doi:10.1007/S11771-021-4601-5
Mao, X. S., Hou, Z. J., Wang, W. N., and Lu, L. (2010). Formation Mechanism and Numerical Simulation of Longitudinal Cracks in Wetland Section of Qinghai-Tibet Highway. Chin. J. Rock Mech. Eng. 09, 1915–1921. http://rockmech.whrsm.ac.cn/CN/abstract/abstract26688.shtml.
Miao, J. L., Chen, X., Lv, Y. N., and Wang, D. Y. (2018). Prediction on Deformation and Reliability of Subgrade of Qinghai-Tibet Railway Based on BP Neural Network Method. J. Nat. Disasters 27 (04), 81–87. doi:10.13577/j.jnd.2018.0411
Mu, Y. H., Ma, W., Niu, F. J., Liu, G., and Zhang, Q. L. (2014). Study on Geotechnical Hazards to Roadway Engineering in Permafrost Regions. J. Disaster Prev. Mitigation Eng. 03, 259–267. doi:10.13409/j.cnki.jdpme.2014.03.005
Ni, J. R., Yin, K. Q., and Zhao, Z. J. (1998). Comprehensive Classification for Wetlands I. Classification. J. od Nat. Resour. 03, 22–29.
Niu, F., Gao, Z., Lin, Z., Luo, J., and Fan, X. (2019). Vegetation Influence on the Soil Hydrological Regime in Permafrost Regions of the Qinghai-Tibet Plateau, China. Geoderma 354, 113892. doi:10.1016/j.geoderma.2019.113892
Peng, H., Ma, W., Mu, Y. H., Jin, L., and Zhu, D. P. (2015). Analysis of Disease Investigation and Long-Term Deformation Characteristics of Common Fill Embankment of the Qinghai-Tibet Highway. Rock Soil Mech. 36 (07), 2049–2056. doi:10.16285/j.rsm.2015.07.029
Qi, C. Q., Wu, Q. B., Shi, B., and Tang, C. S. (2006). Reliability Analysis of Thawing Settlement of Permafrost Subgrade Based on Genetic Algorithm. Rock Soil Mech. 27 (08), 1429–1432+1436. doi:10.16285/j.rsm.2006.08.042
Qi, C. Q., Wu, Q. B., Shi, B., Wu, J. M., and Yu, J. (2007). Application of BP Neural Network on Deformation Prediction for Permafrost Embankment. Hydrogeology Eng. Geology. 34 (04), 27–30. doi:10.3969/j.issn.1000-3665.2007.04.008
Qi, J., Yao, X., Yu, F., and Liu, Y. (2012). Study on Thaw Consolidation of Permafrost under Roadway Embankment. Cold Regions Sci. Technol. 81, 48–54. doi:10.1016/j.coldregions.2012.04.007
Sheng, Y., Wu, J. C., Cao, W., Fang, J. H., Xu, A. H., and Peng, E. X. (2020). Characteristics and Changes of Permafrost along the Engineering Corridor of National Highway 214 in the Eastern Qinghai-Tibet Plateau. Sci. Cold Arid Regions 12 (6), 503–516. doi:10.3724/SP.J.1226.2020.00503
Sun, G. Y. (2000). Discussion on the Symbiotic Mechanisms of Swampy with Permafrost-Taking Da-Xiao Hinggan Mountains as Examples. J. Glaciology Geocryology 04, 309–316. doi:10.3969/j.issn.1000-0240.2000.04.003
Sun, G. Y., Jin, H. J., and Yu, S. P. (2008). The Symbiotic Models of Marshes and Permafrost – A Case Study in Daxing’an and Xiaoxing’an Mountain in Range. Wetland Sci. 04, 479–485. doi:10.13248/j.cnki.wetlandsci.2008.04.009
Sun, S. Y. (2003). Characteristic of Ecological Environment and its Protection along the Qinghai-Tibet Railway. J. Glaciology Geocryology S1, 181–185. doi:10.3969/j.issn.1000-0240.2003.z1.042
The Professional Standards Compilation Group of People’s Republic of China (2015). Specifications for Design of Highway Subgrades JTG D30-2015. Beijing: People's Communications Press.
Wang, C. L., Zhang, Y. L., Wang, Z. F., and Bai, W. Q. (2010). Changes of Wetland Ecosystem Service Value in the Lhasa River Basin of Tibetan Plateau. Resour. Sci. 10, 2038–2044. http://www.resci.cn/CN/Y2010/V32/I10/2036.
Wang, C. T., Wang, Q. L., Jing, Z. C., Feng, B. F., Du, Y. G., et al. (2008). Vegetation Roots and Soil Physical and Chemical Characteristic Changes in Kobresia Pygmaca Meadow Under Different Grazing Gradients. Acta Prataculturae Siniva 17 (5), 9–15. doi:10.11686/cyxb20080502
Wang, S. J., Huo, M., and Zhou, W. J. (2004). Subgrade Failure of Qinghai-Tibet Highway in Permafrost Area. Highway 49 (05), 22–26. doi:10.3969/j.issn.0451-0712.2004.05.006
Wang, S. J., Wang, Z., Yuan, K., and Zhao, Y. G. (2015). Qinghai-Tibet Highway Engineering Geology in Permafrost Regions: Review and Prospect. China J. Highw. Transport 28 (12), 1–8+32. doi:10.19721/j.cnki.1001-7372.2015.12.001
Wang, Z. H., Huang, Z. F., Chen, Y., Xiao, H. B., and Guan, Q. (2015). Research on Variation of Solar Radiation over Yushu Region of Qinghai Province from 1961 to 2010. J. Glaciology Geocryology 03, 692–700. doi:10.4236/wjet.2015.33c010
Wen, Z., Sheng, Y., Wu, Q. B., Huang, B., Xu, A. H., and Li, H. Q. (2009). Ground Temperature and Deformation Laws of Highway Embankments in Degenerative Permafrost Regions. Chin. J. Rock Mech. Eng. 28 (07), 1477–1483. doi:10.3321/j.issn:1000-6915.2009.07.022
Wu, Q. B., Shen, Y. P., and Shi, B. (2003). Relationship between Frozen Soil Together with its Water-Heat Process and Ecological Environment in the Tibetan Plateau. J. Glaciology Geocryology 25 (03), 250–255. doi:10.3969/j.issn.1000-0240.2003.03.002
Xu, A. H. (2014). Analysis of the Sensitivity of Highway Diseases in Permafrost Regions to Ground Temperatures and Ice Contents. J. Glaciology Geocryology 36 (03), 622–625. doi:10.7522/j.issn.1000-0240.2014.007
Yang, K. F., Mu, Y. H., Bi, G. Q., Li, G. Y., and Chen, T. (2019). Recent Research Progress and Prospect of the South-And North-facing Slope Effect in the Roadway Engineering along the Qinghai-Tibet Engineering Corridor. J. Disaster Prev. Mitigation Eng. 39 (01), 180–190. doi:10.13409/j.cnki.jdpme.2019.01.02
Yu, F., Qi, J., Yao, X., and Liu, Y. (2013). In-situ Monitoring of Settlement at Different Layers under Embankments in Permafrost Regions on the Qinghai-Tibet Plateau. Eng. Geology. 160, 44–53. doi:10.1016/j.enggeo.2013.04.002
Zhang, Y., Wang, C., Bai, W., Wang, Z., Tu, Y., and Yangjaen, D. G. (2010). Alpine Wetlands in the Lhasa River Basin, China. J. Geogr. Sci. 20 (03), 375–388. doi:10.1007/s11442-010-0375-7
Zhao, H. T., Wu, Q. B., Zhang, Z. Q., and Huo, Y. D. (2019). Assessment of Cooling Effect of the Crushed Rock Embankment Based on Catastrophe Progression Method. Chin. J. Rock Mech. Eng. 38 (08), 1686–1695. doi:10.13722/j.cnki.jrme.2018.1273
Keywords: permafrost wetlands, typical diseases, type classification, engineering stability, evaluation model
Citation: Mao X, Zhao Y, Wu Q, Huang W and Han L (2022) Type Classification and Engineering Stability Evaluation of Permafrost Wetlands on the Qinghai–Tibet Plateau. Front. Earth Sci. 10:837062. doi: 10.3389/feart.2022.837062
Received: 16 December 2021; Accepted: 18 January 2022;
Published: 11 March 2022.
Edited by:
Dongliang Luo, Northwest Institute of Eco-Environment and Resources (CAS), ChinaReviewed by:
Yanhui You, Northwest Institute of Eco-Environment and Resources (CAS), ChinaYao Xiaoliang, Xi’an University of Technology, China
Zhaohui Yang, University of Alaska Anchorage, United States
Copyright © 2022 Mao, Zhao, Wu, Huang and Han. This is an open-access article distributed under the terms of the Creative Commons Attribution License (CC BY). The use, distribution or reproduction in other forums is permitted, provided the original author(s) and the copyright owner(s) are credited and that the original publication in this journal is cited, in accordance with accepted academic practice. No use, distribution or reproduction is permitted which does not comply with these terms.
*Correspondence: Ying Zhao, 2017021007@chd.edu.cn