- 1National Institute for Earth Physics (INFP), Magurele, Romania
- 2Department of Reinforced Concrete Structures, Technical University of Civil Engineering Bucharest (UTCB), Bucharest, Romania
- 3National Institute for Research and Development in Construction, Urban Planning and Sustainable Spatial Development, URBAN-INCERC, Bucharest, Romania
- 4Department of Environment and Land Reclamation, University of Agronomic Sciences and Veterinary Medicine of Bucharest, Bucharest, Romania
The seismic instrumentation of structures in order to assess their condition and to track it over long periods or after representative events has proven to be a topic of large interest, under continuous development at international level. The seismic hazard of Romania poses one of the most dangerous threats for the country, in terms of potential physical and socio-economic losses. In recent years, taking advantage of the new scientific and technological advances, among which the exponential growth in computational resources, significant improvements have been made in extending the seismic networks for structural monitoring and using the data as input for products and services addressed not only to the research community but also to stakeholders. The paper covers focused aspects of the topic for Romania, referring to past developments of the most important institutions and seismic networks in the country and the current status, including the research and regulatory gaps. Currently, three main research and academic institutions perform structural health monitoring of twenty-two buildings in Romania. As the number of monitored buildings grows and new actors in the private sector start to get involved in the process, the need for data standardization and a regulatory framework increases. Ongoing national and international projects (PREVENT, SETTING, TURNkey) address these issues and outline the roadmap for future actions of the main institutions responsible for seismic risk reduction, including authorities, research and academia.
Introduction
An essential activity for seismic countries is the monitoring and tracking of the condition of the building stock, aiming to ensure the safety of the population and quick recovery after extreme events. This endeavor has proved important not only for preparedness, mitigation and decision-making in emergency situations, but also for opening and supporting a wide range of multi-disciplinary research approaches. The condition assessment of aging structures and infrastructures is becoming a more and more critical issue, especially when developing life extension and replacement strategies. A cost-effective maintenance strategy should aim for minimizing the total life-cycle cost of a structure, considering the costs for preventive maintenance, inspection, monitoring, repair, and failure losses (Bergmeister et al., 2003). The importance and the benefits were analyzed, by assessing the Value of Information (VoI) for structural health monitoring (SHM) systems, by Pozzi and Der Kiureghian (2011) and Kamariotis et al. (2022).
The main objectives of SHM are to assess the structural condition and to rapidly detect the changes that could reveal damage occurrence, based on vibration recordings. The research in the field of SHM was initiated with a special focus on the aerospace, nuclear power and gas exploration industries (Doebling et al., 1996; Sohn et al., 2004). The following decades witnessed a large and diversified development of SHM approaches and methods, supported by the progress of sensing technology, computer hardware and software and leveraged by the need of integration of SHM in earthquake early-warning (EEW) systems (Cosenza et al., 2010; Wu and Beck, 2012; Su et al., 2020; Iaccarino et al., 2021; Sivasuriyan et al., 2021).
In Romania, a country affected by recurring earthquakes originating from various shallow and intermediate-depth sources (Radulian et al., 2000), a large percentage of the building stock dates from before 1963 (Lungu et al., 2008; Pavel et al., 2016), the year of the enforcement of the first mandatory seismic design code, with many of them being highly vulnerable. According to data from the latest National Census (2011), more than 40% of the residential building stock in the whole country and more than 44% in the capital city Bucharest were erected before 1963. The significant losses generated by the 1977 Vrancea earthquake (when almost 33,000 buildings were partially or completely damaged), highlighted the need for an improved seismic design of buildings and for extending seismic instrumentation.
The extensive implementation of SHM systems and rapid damage assessment tools is nowadays essential for assisting decision-makers to set up strategies for the retrofit of the vulnerable building stock. Several countries have already elaborated specific guidelines and standards for the seismic instrumentation of buildings (Çelebi, 2000) and SHM (ISIS Canada, 2001; Mufti, 2002—Canada; Teshigawara et al., 2004—Japan; Moreu et al., 2018; Yang et al., 2017—China; Porter et al., 2004; Rücker et al., 2006). At present, no detailed regulations for SHM exist in Romania, even though several buildings and infrastructures are monitored and several research projects in the field have been completed or are in progress.
The article presents an overview of the evolution and current status of the seismic instrumentation of building structures in Romania, with reference to the international research and regulatory framework and to the national implementation. It covers a broad perspective, from long-term SHM under operational conditions to seismic monitoring of structures under weak-to-moderate Vrancea earthquakes. The current research gaps regarding the seismic instrumentation of structures in Romania are discussed, as well as potential future actions to overcome these issues, including the improvement of the national legislation in the field.
Evolution of the Seismic Instrumentation of Building Structures in Romania
In Romania, seismic monitoring of buildings started in the 1960’s, when buildings in several cities were instrumented, mainly for scientific purposes, by the National Institute for Building Research, INCERC1 (Georgescu et al., 2010). By the time the MW 7.4, 4 March 1977, Vrancea earthquake occurred, four accelerographs were installed at the top and in the basement of two reinforced concrete (RC) buildings, located in the cities of Bucharest (RC shear walls, 11 stories) and Galati (RC frames, 12-story) (Berg et al., 1980; Balan et al., 1982). The first reference also mentions partially instrumented multistory buildings, with accelerographs installed, at that time, either in the basement or near the top, located in Bucharest (RC frames, 13 stories), Bacau (RC shear walls) and Focsani (masonry, 3 stories). In addition, in the years before the 1977 earthquake, an extensive campaign was conducted to determine the dynamic characteristics of various buildings, by ambient vibrations measurements. The database compiled from these measurements was used, after the earthquake, as a reference to assess modifications of natural periods for 47 residential buildings in Bucharest, with various structural systems and numbers of stories ranging from 8 to 18 (Balan et al., 1982). The availability of the reference values was crucial for later seismic vulnerability assessments, given that a large part of the mentioned buildings was based on standardized designs. It was shown that an increase of the natural period of vibration of the buildings with less than 20–25% was associated with low damage, percentages of 25–50% corresponded to light damage, while multiple, systematic or local and significant damage was observed for percentages higher than 50%.
The seismic network of INCERC evolved significantly after the 1977 earthquake, when new strong motion accelerographs were used for the instrumentation of multistory residential buildings, hotels, public and administrative buildings (Craifaleanu et al., 2011). The height of the monitored buildings ranged between 4 and 11 stories, with the recording equipment typically placed in the basement and at the top floor. In 2010, the seismic network of URBAN-INCERC consisted of over 100 stations, with 11 instrumented buildings (Georgescu et al., 2010). A database of seismic records obtained on buildings instrumented by INCERC during strong earthquakes (MW 7.1, 30 August 1986; MW 6.9, 30 May 1990, and MW 6.4, 31 May 1990), was compiled (Borcia et al., 2013, 2014, 2015; Craifaleanu and Borcia, 2015). The seismic data recorded in buildings were analyzed by Popescu and Demetriu (1994, 1994b, 1996) and by Demetriu and Borcia (Demetriu and Borcia, 2001; Demetriu, 2002). For the data recorded on a RC building during the 1986 earthquake, Popescu and Demetriu (1994) identified five vibration modes on each direction by running a system identification algorithm based on fitting of multivariate autoregressive model (MAR), assuming a multi-input single-output system. Popescu and Demetriu (1994) reported the initial (35–40 s) nonlinear behavior of a 12-story RC building during the 1986 earthquake, based on recorded acceleration components.
Two buildings in the Bucharest area and an experimental building at INCERC were instrumented in 1996–1998 in the framework of the Collaborative Research Center “Strong Earthquakes: A Challenge for Geosciences and Civil Engineering” project SFB 461 (Wenzel, 1997), with the National Institute for Earth Physics (INFP), the Technical University of Civil Engineering Bucharest (UTCB) and INCERC as partners (Aldea et al., 2004b). In 2003, for 3 months, one pair of strong motion instruments was deployed in a 11-story RC building, headquarters of the Institute of Atomic Physics (TURN), to study the influence of the building structure on the seismic waveforms. The monitoring was conducted within the framework of the Urban Seismology (URS) project (Ritter et al., 2005), having as partners the University of Karlsruhe and INFP.
Another structure of interest, instrumented by National Centre for Seismic Risk Reduction (NCSRR2), was the Faculty of Civil, Industrial and Agricultural Buildings (FCCIA) of UTCB, a RC frame, low-code building. The experimental data recorded during ambient vibration monitoring campaigns were used to validate its numerical model. In addition, the soil-structure interaction (SSI) analysis revealed slight interaction effects, however with no significant numerical impact (Demetriu et al., 2012).
The progress in the seismic instrumentation of buildings occurred in the broader context of the general development of the seismic networks in Romania. In addition, it should be mentioned that distinct monitoring is performed, by other organizations, for dams, bridges or for the subway lines in Bucharest. These construction categories are, however, beyond the scope of this paper.
Current Status of Structural Health Monitoring for Buildings in Romania. Recent Projects
With the enforcement of the 2006 and 2013 editions of the Romanian seismic design codes, P100-1/2006 (UTCB, 2006) and P100-1/2013 (UTCB, 2013), both drafted by UTCB, the seismic monitoring of structures has gained additional momentum. The in force code state mandatory instrumentation for importance-exposure class I buildings, as well as for buildings higher than 45 m above ground level, located in areas with peak ground design acceleration values equal or greater than 0.25 g. In addition, since 2005, a Ministerial Order (OMTCT/OMAI No. 1995/1160 from 2005/2006) requires all the public and private buildings to be instrumented, if they have more than 16 stories (or are more than 50 m-high) or have a developed area larger than 7,500 m2. At present, INFP, URBAN-INCERC and UTCB monitor twenty-two buildings in Romania (Figure 1 and Table 1). Information on instrument types and representative photos are provided in the Supplementary Material.
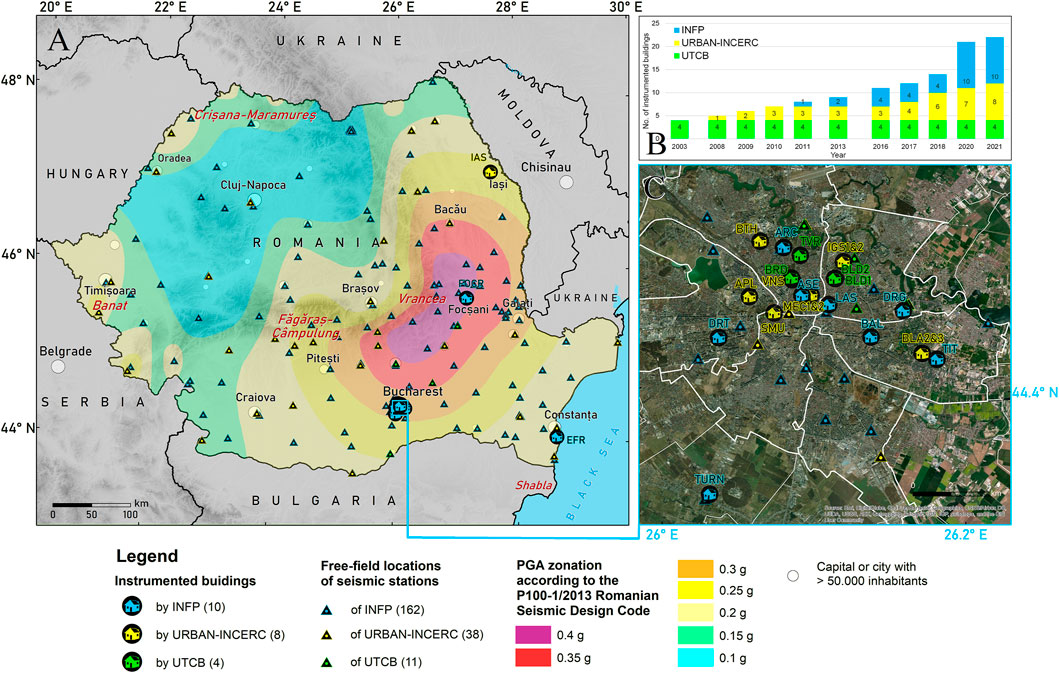
FIGURE 1. Map of the seismic stations installed in buildings, by INFP (10 buildings, 36 sensors), URBAN-INCERC (8 buildings, 20 sensors) and UTCB (4 buildings, 13 sensors) and free-field, and the seismic zonation from the Romanian seismic design code P100-1/2013, indicating the main seismic sources (red text) (A), the timeline of the installation year for the 22 currently instrumented structures, for each institution (B), and a detailed map of Bucharest with the instrumented structures and free-field stations (C).
At URBAN-INCERC, the National Network for the Seismic Monitoring and Protection of Building Stock is the department in charge of the operation of the seismic network, including the instrumented buildings (Dragomir et al., 2015a; Dragomir et al., 2015b; Dragomir et al., 2016; Dragomir et al., 2021). Currently, URBAN-INCERC monitors, mainly for research purposes, eight buildings with various functions and occupancies, located in Bucharest (7) and Iasi (1); six of these are connected online to the Data Center of the Institute. The instrumentation of these buildings consists of at least two sensors (ground floor/basement and top); two of them also have sensors close to the building, in free-field conditions. Other buildings have at present only ground-level sensors installed, complete instrumentation being envisaged in the future. In addition, short-term building vibration monitoring is being conducted, generally focused on actions induced by industrial or transportation activities. In a study conducted by Dragomir et al. (2017b), the fundamental period (0.18 s) of the Biotechnology Faculty building (BTH) was experimentally determined based on the Fourier Spectra (FS) of several recordings and validated with the values from the design code (0.15 s) and by using the Operational Modal Analysis tool of the ARTeMIS Modal Pro software3 (0.19 s). Dragomir et al. (2017a) estimated the fundamental frequency for two other buildings, a 10-story RC shear walls apartment block (BLA) and a 15-story RC shear walls office building (APL), using noise and earthquake data. Applying the FS, they found fundamental frequencies of fx = 1.73 Hz and fy = 2.05 Hz for the first building, and fx = 1.5 Hz and fy = 1.3 Hz for the second building, respectively. Moreover, there is an ongoing experimental project for real-time damage detection in buildings (Dragomir et al., 2019; Dragomir et al., 2020) using ARTeMIS and an extensive campaign, in the framework of the ECOSMARTCONS project, for the seismic instrumentation of the premises of national research institutes all over the country. Starting with 2022, the Data Center of URBAN-INCERC has implemented SeisComP4.
Significant progress in seismic instrumentation was made within the Japan International Cooperation Agency (JICA) Technical Cooperation Project “Reduction of Seismic Risk for Buildings and Structures”, in which the NCSRR instrumented four representative buildings in Bucharest (Aldea et al., 2004a; Aldea et al., 2007a; Aldea et al., 2007b): the Romanian National Television (TVR), the BRD-SG Tower (BRD), and two residential multistory buildings (BLD1 and BLD2). Several detailed analyses of the modal frequencies, based on ambient vibration and earthquake data, were performed on the BRD-SG Tower, a newly constructed RC office building (Demetriu and Aldea, 2006). The SSI effect was investigated based on free-field and borehole data by Aldea et al. (2007c). For the same building, Perrault et al. (2013) proposed a methodology to reduce the uncertainty of the single-building fragility curve using experimental data. First, a linear MDOF model was adjusted for experimental modal analysis using a Timoshenko beam model (Boutin et al., 2005) and based on Anderson’s criteria (Anderson, 2004). Then, the structure’s response to a large set of accelerograms simulated by the SIMQKE software (Gasparini and Vanmarcke, 1976) was computed and, for the final step, the fragility curves were constructed by comparing numerical inter-story drift with the threshold criteria provided by the Hazus methodology (FEMA, 2003) for the slight damage state. Recent research on SHM, performed by UTCB, widened the scope of previous studies, approaching heritage buildings, such as the minaret of the Royal Mosque in Constanta (Aldea et al., 2018), and traditional Romanian timber framed masonry houses (Aldea et al., 2020).
In 2011, a heritage building of the University of Economic Studies (ASE), located in Bucharest, was retrofitted using seismic isolators and viscous dampers, the first action of this kind in Romania. INFP was in charge of SHM and of the efficiency assessment of this innovative solution, by placing accelerometers under and above the seismic isolators. Data recorded during two seismic events (MW 5.5, 28 October 2018 and MW 4.8, 31 January 2020) revealed a reduction of the acceleration amplitude by a factor ranging from 2.0 to 3.8, for the two horizontal components. The same promising results were reported for the same earthquakes on another heritage structure equipped with earthquake-protection system in Bucharest, the Arch of Triumph (ARC), with reductions of acceleration amplitude by a factor up to 4.5 (Balan et al., 2020).
INFP is currently monitoring 10 buildings, with 36 sensors (Table 1). The instrumentation setup consists of strong motion sensors located mainly at the ground (or basement) level, at an intermediate floor and at the roof level. New low-cost sensors (Raspberry Shake5 RS3D and RS4D) are tested to extend the building monitoring network in the framework of the TURNkey6 and PREVENT7 projects. Low-cost sensors (Micro-electromechanical systems - MEMS accelerometers) have proven useful and provided promising results when used for early-warning systems (Nof et al., 2019), small local earthquake detection (Cascone et al., 2021) or even initial ground-motion assessment (Holmgren and Werner, 2021). However, their usability and reliability for SHM has not yet been extensively studied. The very high level of digital noise is masking any type of low-amplitude ambient vibrations. This type of sensors should be of paramount importance in case of earthquakes with MW > 6.0, given the amount of data they can provide from a larger number of instrumented structures, when compared to professional equipment, within the same monitoring expenses.
The data from all stations are transmitted in real-time to the Romanian National Data Center (RONDC) of INFP. For data acquisition, quality control and recording, real-time data processing and exchange, network status monitoring, automatic and interactive event detection and location, waveform archiving and distribution, INFP has run, since 2008, SeisComP, in parallel with Kinemetrics Antelope8 (Marmureanu et al., 2021).
Recently, Tiganescu et al. (2020) analyzed the dynamic characteristics (fundamental period and damping ratio) of the three representative high-rise buildings from Bucharest, based on ambient vibration data recorded during a two-day measurement campaign. The fundamental periods obtained using FS analysis, Random Decrement Technique (Cole, 1973) and Transfer Function were validated against results computed using empirical formulas from the design code corresponding to each building. The values were consistent for both the fundamental period and the damping ratio of the buildings, regardless of the method and of the measurement day. However, small diurnal and weekly variations were reported for the two parameters, due to small differences in atmospheric conditions and building occupancy at different moments of data acquisition.
Preliminary analysis of earthquake data recorded on structures during the latest moderate magnitude Vrancea seismic event (MW 5.5, 28 October 2018) highlighted different behaviors and trends, depending on the structural characteristics and of the existence of earthquake-protection system. Amplification and reduction of motion on different frequency ranges were revealed, with clear peaks corresponding to the dynamic characteristics of the buildings (Tiganescu et al., 2019).
The Bighorn module, an extension of the Antelope package, is also used at INFP to perform seismic monitoring of structures. The system computes near real-time response spectra and issues alarms, depending of the level of exceedance of a preset limit spectra. This procedure was tested for Bucharest using the 28 October 2018 earthquake data (Balan et al., 2019). The reporting service is currently performed in an offline environment, on request. The permanent seismic stations installed in buildings were used in a recent study conducted by Grecu et al. (2021) to assess the effect of the COVID-19 related restrictions on the level of high-frequency content of the ambient vibrations generated by human activity. Significant noise reductions (40–80%) on the 15–40 Hz frequency range for stations in and near buildings were associated to the mobility restrictions of people working inside the office buildings and with the shift to online classes for educational units.
In the context of other studies highlighting the influence of atmospheric conditions on the dynamic parameters of structures (Clinton et al., 2006; Herak and Herak, 2009; Mikael et al., 2013; Guéguen and Tiganescu, 2018), a case-study building (TURN) was instrumented with both seismic sensors and a meteorological station (Tiganescu et al., 2021a), in the framework of the PREVENT project. A fundamental frequency variation analysis was conducted on a 72-h dataset of ambient vibration and earthquake data, using the Frequency Domain Decomposition method (Brincker et al., 2001). Small variations of the fundamental frequency were observed in the ambient vibration regime, while for the forced vibrations (earthquake) the variation was larger (drop of 10%) and followed by a recovery. Moreover, correlation of the atmospheric and environmental conditions (mainly air temperature, relative humidity and wind speed) with the building’s natural frequency was tested, but with no sharp conclusions due to limited timespan.
Discussion
A large number of buildings, representing different typologies (construction period, structural system, material, height, exposure to earthquakes, vulnerability) were previously and are currently instrumented in Romania, as a need for acquiring pre-, during and post-event vibration data. From the point of view of the coverage of areas of interest considering seismic hazard levels and building exposure, there is still a need to instrument and monitor structures that could be affected by crustal earthquakes (Figure 1A), in seismic zones such as Banat, Fagaras-Campulung, Crisana-Maramures or nearby Shabla, Bulgaria.
The effort is ongoing by means of national and international projects involving seismic instrumentation of structures and the development of web platforms for data and metadata inventory (SETTING9 or TURNkey) and waveform acquisition, processing and visualization (PREVENT). Data standardization for easy integration in international infrastructures such as European Plate Observing System - EPOS (Luzi et al., 2016; Astorga et al., 2020) and for use in international research projects is another objective that the Romanian research and engineering community working on SHM is envisaging.
In addition, the low and narrow-band frequency content of the ground motion, observed in Bucharest for large-magnitude Vrancea earthquakes (Lungu and Cornea, 1988; Lungu et al., 1992; Craifaleanu, 2011), and its effect on different building typologies (Ambraseys 1977), needs further investigations. Outcomes of the seismic monitoring of structures can also significantly help as input for refined rapid seismic loss estimates, using already available systems such as SeisDaRo (Toma-Danila and Armas, 2017).
There is also a crucial need to continuously develop and upgrade the national guidelines regarding SHM, including clear requirements for modern digital sensors, standard installation procedures, data acquisition and processing. Currently, there are no specific procedures for the elaboration, checking and approval of the seismic instrumentation plan. A better definition of the technical specifications of the digital accelerometers is needed, regarding their minimum sensitivity, the maximum amplitude that can be recorded, the frequency sampling, the storage, and the time precision. Moreover, online access should be mandatory ensured for easy maintenance and periodical checks on the system operational status. The data processing and results interpretation should be performed by specialists, using well-established routines and algorithms, to obtain reliable results and to avoid any artefact errors or uncertainties that can arise and propagate during the signal processing stage.
In the recent years, the collaboration between Romanian institutions involved in the health monitoring of structures (URBAN-INCERC, UTCB and INFP) has been enforced by joint research projects and publications (Tiganescu et al., 2021a; Tiganescu et al., 2021b; Tiganescu et al., 2021c; Marmureanu et al., 2021). A system integrating URBAN-INCERC’s SHM system and INFP’s EEW was proposed by Dragomir et al. (2016). The SETTING project, as well as the Romanian consortium10 contributing in the EPOS research infrastructure11 aim to provide a national research platform consisting of a standardized inventory of organizations which could provide data, products, and services relevant for the field of Earth Sciences—including SHM relevant categories. The platform will be designed to meet the needs of various user communities (research, academia, industry and general public). The effort to strengthen the collaboration with local and central authorities has gained momentum, as well, as several researchers from the three institutions are participating in the elaboration of a national strategy for the seismic risk reduction of the building stock, and in the development of a national emergency procedure in case of a strong earthquake. A special SHM section will be held at the third European Conference on Earthquake Engineering and Seismology (Bucharest, 2022), as a step towards bringing together the significant actors in the field and bridging the gap between research, academia and industry.
Author Contributions
AT and I-GC designed the paper, and all the authors contributed to the manuscript. AA and RV contributed to the part referring to UTCB network, I-GC and C-SD contributed to the part referring to the URBAN-INCERC network, AT, BG, DT-D, and S-FB contributed to the part referring to the INFP network. DT-D designed Figure 1. All the authors contributed to the Discussion section.
Funding
Part of the developments received support through the following INFP Projects PREVENT (Open system for integrated civil structures monitoring; no. PN-III-P2-2.1-PED-2019-0832) and Pre-Quake (Preparing for the next major earthquake: exploring new scientific opportunities; No. PN-III-P1-1.1-PD-2019-0969) supported grants from the Romanian Ministry of Research, Innovation and Digitalization, CNCS–UEFISCDI within PNCDI III, as well as the SETTING Project (Integrated thematic services in the field of Earth observation: a national platform for innovation; No. 108206) cofinanced from the Regional Development European Fund (FEDR) through the Operational Competitivity Programme 2014–2020; TURNkey (Towards more earthquake-resilient urban societies through a multisensor-based information systemenabling earthquake forecasting, early warning and rapid response actions, available at https://earthquake-turnkey.eu/, GA 821046), funded by the European Commission (EC); The paper refers also some of the results obtained at INCD URBAN-INCERC within the projects PN 18-35 01 01, “Research for the development of an instrumental detection system for structural damage caused by seismic or non-seismic sources”, and PN 19-33 01 01, “Research on the implementation of an integrated system for ensuring the security of the constructed space, with semi-automatic generation of PGA maps provided by seismic actions or other vibratory sources and quick evaluation of vulnerability of instrumented buildings”, funded in the framework of the “Nucleu” Programmes CONCRET and ECOSMARTCONS by the Romanian Ministry of Research, Innovation and Digitalization.
Conflict of Interest
The authors declare that the research was conducted in the absence of any commercial or financial relationships that could be construed as a potential conflict of interest.
Publisher’s Note
All claims expressed in this article are solely those of the authors and do not necessarily represent those of their affiliated organizations, or those of the publisher, the editors and the reviewers. Any product that may be evaluated in this article, or claim that may be made by its manufacturer, is not guaranteed or endorsed by the publisher.
Acknowledgments
Japan International Cooperation Agency (JICA) is gratefully acknowledged for the donation of equipment for seismic instrumentation in the frame of the Technical Cooperation Project “Reduction of Seismic Risk for Buildings and Structures” Ref.#7241011E0, equipment operated at present by UTCB. The authors also thank to Editor DP and two independent reviewers for their helpful and constructive reviews, which lead to an improvement of the manuscript.
Supplementary Material
The Supplementary Material for this article can be found online at: https://www.frontiersin.org/articles/10.3389/feart.2022.819153/full#supplementary-material
Footnotes
1Today a branch of the National Institute for Research and Development in Constructions, Urban Planning and Sustainable Spatial Development, URBAN-INCERC
2NCRRS functioned between 2003 and 2010. The seismic instrumentation installed by NCRRS continued to be operated by URBAN-INCERC and at present by UTCB
6https://earthquake-turnkey.eu
8The Boulder Real-Time Technologies, Inc. (BRTT) https://brtt.com
References
Aldea, A., Albota, E., Demetriu, S., and Kashima, T. (2007a). “Seismic Response of a Low-Code High-Rise RC Building in Bucharest,” in Proc. of the International Symposium on Strong Vrancea Earthquakes and Risk Mitigation, Bucharest, Romania.
Aldea, A., Demetriu, S., Albota, E., and Kashima, T. (2007b). “Instrumental Response of Buildings. Studies within JICA Project in Romania,” in Proc. of the International Symposium on Seismic Risk Reduction. The JICA Technical Cooperation Project in Romania, Vacareanu. Editor R. Hiroto and, 157–170.
Aldea, A., Dutu, A., Demetriu, S., and Dima, D. I. (2020). Dynamic Properties Identification for a Timber Framed Masonry House. Constructii 21 (1), 3–12.
Aldea, A., Iiba, M., Demetriu, S., and Kashima, T. (2007c). “Evidence of Soil-Structure Interaction from Earthquake Records at a High-Rise Building Site in Bucharest,” in Proc. of the 4th International Conference on Earthquake Geotechnical Engineering, Thessaloniki, Greece, 1523.
Aldea, A., Kashima, T., Lungu, D., Vacareanu, R., Koyama, S., and Arion, C. (2004a). “Modern Urban Seismic Network in Bucharest, Romania,” in Proc. of the First International Conference on Urban Earthquake Engineering, Tokyo, Japan.
Aldea, A., Lungu, D., Vacareanu, R., and Arion, C. (2004b1800). “Development of Strong Ground Motion Network in Romania and Bucharest Instrumentation for Site Effects Assessment,” in Proc. of the 13th World Conference on Earthquake Engineering, Vancouver (Canada).
Aldea, A., Neagu, C., Lozinca, E., Demetriu, S., El-Amine Bourdim, S. M., and Turano, F. (2018). “Toward the Seismic Evaluation of "Carol I" Royal Mosque in Constanța,” in Seismic Hazard and Risk Assessment. Editors R. Vacareanu, and C. Ionescu (Springer), 345–359. doi:10.1007/978-3-319-74724-8_23
Ambraseys, N. N. (1977). Long-period Effects in the Romanian Earthquake of March 1977. Nature 268, 324–325. doi:10.1038/268324a0
Anderson, J. G. (2004). “Quantitative Measure of the Goodness-Of-Fit of Synthetic Seismograms,” in Proc. of the 13th World Conference on Earthquake Engineering, Vancouver (Canada), 243.
Astorga, A., Guéguen, P., Ghimire, S., and Kashima, T. (2020). NDE1.0: a New Database of Earthquake Data Recordings from Buildings for Engineering Applications. Bull. Earthquake Eng. 18 (4), 1321–1344. doi:10.1007/s10518-019-00746-6
Balan, S., Capatina, D., Cornea, I., Cristescu, V., Dumitrescu, D., Enescu, D., et al. (1982). Cutremurul de pamint din Romania de la 4 martie 1977 (The 1977 March 4, Earthquake in Romania). Bucharest: The Publishing House of the Romanian Academy. (in Romanian).
Balan, S. F., Tiganescu, A., Apostol, B. F., and Danet, A. (2019). Post-earthquake Warning for Vrancea Seismic Source Based on Code Spectral Acceleration Exceedance. Earthq. Struct. 17 (4), 365–372. doi:10.12989/eas.2019.17.4.365
Balan, S. F., Tiganescu, A., and Apostol, B. F. (2020). Structure Response Analysis of the Seismic Isolated Buildings in Bucharest City. IOP Conf. Ser. Earth Environ. Sci. 609, 012080. doi:10.1088/1755-1315/609/1/012080
Berg, G. V., Bolt, B. A., Sozen, M. A., and Rojahn, C. (1980). Earthquake in Romania, March 4, 1977. An Engineering Report. Washington, DC: The National Academies Press. doi:10.17226/19783
Bergmeister, K., Aktan, A. E., Bucher, C., Dorfmann, L., Fehling, E., Frey, R. P., et al. (2003). Monitoring and Safety Evaluation of Existing concrete Structures: State-Of-Art Report. Lausannef:ib Bulletin 22. doi:10.35789/fib.BULL.0022
Borcia, I. S., Craifaleanu, I. G., Calarasu, E. A., Tanase, N. F., and Praun, I. C. (2015). SM-ROM-GL (Strong Motion ROMania Ground Level) Database. Constructii 16 (1), 3–10.
Borcia, I. S., Craifaleanu, I. G., Georgescu, E. S., and Praun, I. C. (2014). “The National strong-motion Network of URBAN-INCERC: Recent Developments,” in Proc. of the 2nd European Conference on Earthquake Engineering and Seismology, Turkey (Istanbul), 3354.
Borcia, I. S., Craifaleanu, I. G., Praun, I. C., and Tanase, N. F. (2013). Banca de date SP VRANCEA NT - Prelucrari ale inregistrarilor cutremurelor vrancene (The SP VRANCEA NT Databank - Processing of Vrancea earthquakes records). Urbanism. Arhitectura. Constructii. 4 (1), 71–78. (in Romanian).
Boutin, C., Hans, S., Ibraim, E., and Roussillon, P. (2005). In Situ experiments and Seismic Analysis of Existing Buildings. Part II: Seismic Integrity Threshold. Earthquake Engng Struct. Dyn. 34 (12), 1531–1546. doi:10.1002/eqe.503
Brincker, R., Zhang, L., and Andersen, P. (2001). Modal Identification of Output-Only Systems Using Frequency Domain Decomposition. Smart Mater. Struct. 10 (3), 441–445. doi:10.1088/0964-1726/10/3/303
Cascone, V., Boaga, J., and Cassiani, G. (2021). Small Local Earthquake Detection Using Low-Cost MEMS Accelerometers: Examples in Northern and Central Italy. Seismic Rec. 1 (1), 20–26. doi:10.1785/0320210007
Çelebi, M. (2000). Seismic Instrumentation of Buildings. Open-File Report 2000-157. Menlo Park, CA: US Geological Survey. doi:10.3133/ofr00157
Clinton, J. F., Bradford, S. C., Heaton, T. H., and Favela, J. (2006). The Observed Wander of the Natural Frequencies in a Structure. Bull. Seismological Soc. America 96 (1), 237–257. doi:10.1785/0120050052
Cole, H. A. (1973). On-line Failure Detection and Damping Measurement of Aerospace Structures by Random Decrement signatures Report NASA CR-2205. Washington, DC: National Aeronautics and Space Administration.
Cosenza, E., Galasso, C., and Iervolino, I. (2010). “Ongoing Applications of EEW and SHM at the University of Naples Federico II,” in Proc. of the 5th European Workshop on Structural Health Monitoring. Editors F. Casciati, and M. Giordano (DEStech Publications), 25–31.
Craifaleanu, I. G., Borcia, I. S., and Praun, I. C. (2011). “Strong-motion Networks in Romania and Their Efficient Use in the Structural Engineering Applications,” in Earthquake Data in Engineering Seismology: Predictive Models, Data Management and Networks. Editors S. Akkar, P. Gülkan, and T. van Eck (Springer), 247–259. doi:10.1007/978-94-007-0152-6_17
Craifaleanu, I. G., and Borcia, I. S. (2015). Reconsideration of Ground-Level Seismic Records Obtained in Instrumented Buildings: a Preliminary Study for strong Vrancea Earthquakes. Constructii 16 (2), 35–44.
Craifaleanu, I. G. (2011). “Investigation of the Frequency Content of Ground Motions Recorded during strong Vrancea Earthquakes, Based on Deterministic and Stochastic Indices,” in Proc. of the 8th International Conference on Structural Dynamics EURODYN. Editors G. De Roeck, G. Degrande, G. Lombaert, and G. Muller, 2893–2898.
Demetriu, S., and Aldea, A. (2006). “Recorded Seismic Response of an Instrumented High-Rise Reinforced-concrete Building in Bucharest,” in Proc. of the First European Conf. on Earthquake Engineering and Seismology, Geneva, Switzerland, 777.
Demetriu, S., Aldea, A., and Udrea, A. (2012). “Modal Parameters of RC Frame Structure Identified from Ambient Vibration Measurements,” in Proc. of the 15th World Conference on Earthquake Engineering, Lisbon, Portugal.
Demetriu, S., and Borcia, I. S. (2001). Seismic Response of Instrumented Buildings during Vrancea Earthquakes, 2. Bulletin of the Technical University of Civil Engineering, Structural Mechanics and Structural Engineering, 1–12.
Demetriu, S. (2002). Modal Identification of Instrumented Buildings from Vrancea Earthquake Records, 2. Bulletin of the Technical University of Civil Engineering, Structural Mechanics and Structural Engineering, 13–21.
Doebling, S. W., Farrar, C. R., Prime, M. B., and Shevitz, D. W. (1996). Damage Identification and Health Monitoring of Structural and Mechanical Systems from Changes in Their Vibration Characteristics: A Literature Review. Los Alamos National Laboratory Report LA-13070-MS. doi:10.2172/249299
Dragomir, C.-S., Craifaleanu, I.-G., Dobre, D., and Georgescu, E.-S. (2019). Prospective Studies for the Implementation of a Remote Access Earthquake Damage Detection System for High-Rise Buildings in Romania. IOP Conf. Ser. Earth Environ. Sci. 221, 012036. doi:10.1088/1755-1315/221/1/012036
Dragomir, C.-S., Meita, V., Dobre, D., Georgescu, E.-S., and Borcia, I.-S. (2015b). A Holistic and Integrative Concept for strong-motion Records on Constructions of the URBAN-INCERC National Seismic Network. Present Environ. Sustain. Dev. 9 (2), 113–124. doi:10.1515/pesd-2015-0029
Dragomir, C. S., Craifaleanu, I.-G., Dobre, D., and Georgescu, E. S. (2016). “An Experimental Investigation on the Health Monitoring of the new City Hall Building in Bucharest Based on Real-Time Data Transmission,” in Life-Cycle of Engineering Systems: Emphasis on Sustainable Civil Infrastructure. Editors J. Bakker, D. M. Frangopol, and K. Van Breugel (CRC Press), 239–244. doi:10.1201/9781315375175-24
Dragomir, C. S., Craifaleanu, I. G., Meiţă, V., Georgescu, E. S., Dobre, D., Sandu, M., et al. (2021). Insights from the Management of a Large Research Infrastructure in Romania: National Network for the Seismic Monitoring and Protection of Building Stock at NIRD URBAN-INCERC. IOP Conf. Ser. Mater. Sci. Eng. 1138, 012021. doi:10.1088/1757-899X/1138/1/012021
Dragomir, C. S., Dobre, D., Craifaleanu, I., and Georgescu, E.-S. (2017a). On-line Data Transmission, as Part of the Seismic Evaluation Process in the Buildings Field. IOP Conf. Ser. Earth Environ. Sci. 95, 022004. doi:10.1088/1755-1315/95/2/022004
Dragomir, C. S., Dobre, D., Craifaleanu, I. G., and Georgescu, E. S. (2020). An Integrated National System for Assuring the Quick Evaluation of the Vulnerability of All Instrumented Buildings after an Earthquake. Recent Developments. Scientific Papers-Series E-Land Reclamation Earth Observation Surv. Environ. Eng. 9, 94–97.
Dragomir, C. S., Dobre, D., Craifaleanu, I. G., and Georgescu, E. S. (2017b). Ex-ante and Ex-post Instrumental Diagnosis of Buildings Structural Health. An Approach at the Level of the National Seismic Network, URBAN-INCERC. Scientific Papers-Series E-Land Reclamation Earth Observation Surv. Environ. Eng. 6, 77–80.
Dragomir, C. S., Dobre, D., and Georgescu, E. S. (2015a). “Seismic Instrumentation on Vibrations to Determine the Dynamic Characteristics of Buildings,” in Proc. of the 15th International Multidisciplinary Scientific GeoConference SGEM, Albena, Bulgaria, 957–964.
Gasparini, D. A., and Vanmarcke, E. H. (1976). Simulated Earthquake Motions Compatible with Prescribed Response Spectra. Research Report, R76-4. Cambridge, MA: MIT Department of Civil Engineering, Massachusetts Institute of Technology.
Georgescu, E. S., Borcia, I. S., Praun, I. C., and Dragomir, C. S. (2010). “State of the Art of Structural Health Monitoring in Seismic Zones of Romania,” in Proc. Of the MEMSCON Workshop (Bucharest, Romania, 89–93.
Grecu, B., Borleanu, F., Tiganescu, A., Poiata, N., Dinescu, R., and Tataru, D. (2021). The Effect of 2020 COVID-19 Lockdown Measures on Seismic Noise Recorded in Romania. Solid Earth 12, 2351–2368. doi:10.5194/se-12-2351-2021
Guéguen, P., and Tiganescu, A. (2018). Consideration of the Effects of Air Temperature on Structural Health Monitoring through Traffic Light-Based Decision-Making Tools. Shock and Vibration 2018, 1–12. doi:10.1155/2018/9258675
Hefford, R. T., Tyler, R. G., and Skinner, R. I. (1980). The M02A Strong-Motion Accelerograph. Bnzsee 13 (4), 374–379. doi:10.5459/bnzsee.13.4.374-379
Herak, M., and Herak, D. (2009). Continuous Monitoring of Dynamic Parameters of the DGFSM Building (Zagreb, Croatia). Bull. Earthquake Eng. 8 (3), 657–669. doi:10.1007/s10518-009-9112-y
Holmgren, J. M., and Werner, M. J. (2021). Raspberry Shake Instruments Provide Initial Ground-Motion Assessment of the Induced Seismicity at the United Downs Deep Geothermal Power Project in Cornwall, United Kingdom. Seismic Rec. 1 (1), 27–34. doi:10.1785/0320210010
Iaccarino, A. G., Gueguen, P., Picozzi, M., and Ghimire, S. (2021). Earthquake Early Warning System for Structural Drift Prediction Using Machine Learning and Linear Regressors. Front. Earth Sci. 9, 666444. doi:10.3389/feart.2021.666444
ISIS Canada (2001). Guidelines for Structural Health Monitoring – Design Manual No. 2. Winnipeg: Intelligent Sensing for Innovative Structures (ISIS) Canada.
Kamariotis, A., Chatzi, E., and Straub, D. (2022). Value of Information from Vibration-Based Structural Health Monitoring Extracted via Bayesian Model Updating. Mech. Syst. Signal Process. 166, 108465. doi:10.1016/j.ymssp.2021.108465
Lungu, D., Aldea, A., and Arion, C. (2008). “Romania'S Seismicity and Seismic Hazard: From Historical Records to Design Codes,” in Harmonization of Seismic Hazard in Vrancea Zone. Editors A. Zaicenco, I. G. Craifaleanu, and I. Paskaleva (Dordrecht): Springer, 1–16. doi:10.1007/978-1-4020-9242-8_1
Lungu, D., Cornea, T., and Demetriu, S. (1992). “Frequency Bandwidth of Vrancea Earthquakes and the 1991 Edition of Seismic Code in Romania,” in Proc. of the 10th Conf. on Earthquake Engineering, Madrid, Spain, 5633–5638.10
Lungu, D., and Cornea, T. (1988). “Power Spectra in Bucharest for Vrancea Earthquakes,” in Proc. of the Symposium on reliability-based design in civil engineering, Lausanne, Switzerland, 17–24.
Luzi, L., Puglia, R., Russo, E., D'Amico, M., Felicetta, C., Pacor, F., et al. (2016). The Engineering Strong‐Motion Database: A Platform to Access Pan‐European Accelerometric Data. Seismological Res. Lett. 87 (4), 987–997. doi:10.1785/0220150278
Mărmureanu, A., Ionescu, C., Grecu, B., Toma-Danila, D., Tiganescu, A., Neagoe, C., et al. (2021). From National to Transnational Seismic Monitoring Products and Services in the Republic of Bulgaria, Republic of Moldova, Romania, and Ukraine. Seismol. Res. Lett. 92 (3), 1685–1703. doi:10.1785/0220200393
Mikael, A., Gueguen, P., Bard, P. Y., Roux, P., and Langlais, M. (2013). The Analysis of Long‐Term Frequency and Damping Wandering in Buildings Using the Random Decrement Technique. Bull. Seismological Soc. America 103 (1), 236–246. doi:10.1785/0120120048
Moreu, F., Li, X., Li, S., and Zhang, D. (2018). Technical Specifications of Structural Health Monitoring for Highway Bridges: New Chinese Structural Health Monitoring Code. Front. Built Environ. 4, 10. doi:10.3389/fbuil.2018.00010
Mufti, A. A. (2002). Structural Health Monitoring of Innovative Canadian Civil Engineering Structures. Struct. Health Monit. 1 (1), 89–103. doi:10.1177/147592170200100106
Nof, R. N., Chung, A. I., Rademacher, H., Dengler, L., and Allen, R. M. (2019). MEMS Accelerometer Mini-Array (MAMA): A Low-Cost Implementation for Earthquake Early Warning Enhancement. Earthquake Spectra 35 (1), 21–38. doi:10.1193/021218EQS036M
Pavel, F., Vacareanu, R., Douglas, J., Radulian, M., Cioflan, C., and Barbat, A. (2016). An Updated Probabilistic Seismic hazard Assessment for Romania and Comparison with the Approach and Outcomes of the SHARE Project. Pure Appl. Geophys. 173 (6), 1881–1905. doi:10.1007/s00024-015-1223-6
Perrault, M., Gueguen, P., Aldea, A., and Demetriu, S. (2013). Using Experimental Data to Reduce the Single-Building Sigma of Fragility Curves: Case Study of the BRD tower in Bucharest, Romania. Earthq. Eng. Eng. Vib. 12 (4), 643–658. doi:10.1007/s11803-013-0203-z
Popescu, T. D., and Demetriu, S. (1994a). Change Detection in Dynamic Characteristics of a Structural System. Proc. 1994 Am. Control. Conf. - ACC 941, 937–941. doi:10.1109/ACC.1994.751881
Popescu, T. D., and Demetriu, S. (1996). Dynamic Characteristics Identification of a Structural System from Earthquake Records. Syst. Anal. Model. Sim. 23 (3), 223–236.
Popescu, T. H. D., and Demetriu, S. (1994b). Identification of a Multi-story Structure from Earthquake Records. IFAC Proc. Volumes 27 (8), 707–712. doi:10.1016/S1474-6670(17)47792-5
Porter, K. A., Beck, J. L., Ching, J. Y., Mitrani-Reiser, J., Miyamura, M., Kusaka, A., et al. (2004). Real-time Loss Estimation for Instrumented BuildingsReport No. EERL 2004-08. Pasadena, CA: Earthquake Engineering Research Laboratory, California Institute of Technology.
Pozzi, M., and Der Kiureghian, A. (2011). “Assessing the Value of Information for Long-Term Structural Health Monitoring,” in Proc. Of SPIE, Health Monitoring of Structural and Biological Systems. Editor T. Kundu, 7984. 79842W. doi:10.1117/12.881918
Radulian, M., Vaccari, F., Mândrescu, N., Panza, G. F., and Moldoveanu, C. L. (2000). Seismic Hazard of Romania: Deterministic Approach. Pure Appl. Geophys. 157, 221–247. doi:10.1007/PL00001096
Ritter, J. R. R., Balan, S. F., Bonjer, K.-P., Diehl, T., Forbriger, T., Marmureanu, G., et al. (2005). Broadband Urban Seismology in the Bucharest Metropolitan Area. Seismological Res. Lett. 76 (5), 574–580. doi:10.1785/gssrl.76.5.574
Rücker, W., Hille, F., and Rohrmann, R. (2006). Guideline for Structural Health Monitoring. European Association on Structural Assessment Monitoring and Control SAMCO.
Sivasuriyan, A., Vijayan, D. S., Górski, W., Wodzyński, Ł., Vaverková, M. D., and Koda, E. (2021). Practical Implementation of Structural Health Monitoring in Multi-Story Buildings. Buildings 11 (6), 263. doi:10.3390/buildings11060263
Sohn, H., Farrar, C. R., Hemez, F. M., Shunk, D. D., Stinemates, D. W., Nadler, B. R., et al. (2004). A Review of Structural Health Monitoring Literature: 1996-2001. Los Alamos National Laboratory Report LA-13976-MS.
Su, J., Xia, Y., and Weng, S. (2020). Review on Field Monitoring of High‐rise Structures. Struct. Control Health Monit. 27, e2629. doi:10.1002/stc.2629
Teshigawara, M., Isoda, H., Morita, K., Kitagawa, Y., Mita, A., Nakai, S., et al. (2004). “Technology Utilization Guidelines of Structural Health Monitoring for Buildings and its Application,” in Proc. of the 13th World Conference on Earthquake Engineering, Vancouver (Canada), 2221.
Tiganescu, A., Balan, S. F., Toma-Danila, D., and Apostol, B. F. (2019). “Preliminary Analysis of Data Recorded on Instrumented Buildings from Bucharest Area during the 28Th October 2018 Vrancea Earthquake,” in Proc. of the 19th International Multidisciplinary Scientific GeoConference SGEM, Albena, Bulgaria, 897–904. doi:10.5593/sgem2019/1.1/S05.111
Tiganescu, A., Craifaleanu, I. G., and Balan, S. F. (2021b). Dynamic Parameters of a RC Building Extracted from Earthquake Data Using the Random Decrement Technique. IOP Conf. Ser. Earth Environ. Sci. 664, 012088. doi:10.1088/1755-1315/664/1/012088
Tiganescu, A., Craifaleanu, I. G., and Balan, S. F. (2021a). Short-term Evolution of Dynamic Characteristics of a RC Building under Seismic and Ambient Vibrations. IOP Conf. Ser. Earth Environ. Sci. 664, 012105. doi:10.1088/1755-1315/664/1/012105
Tiganescu, A., Grecu, B., and Craifaleanu, I. G. (2020). Dynamic Identification for Representative Building Typologies: Three Case Studies from Bucharest Area. Civ Eng. J. 6 (3), 418–430. doi:10.28991/cej-2020-03091480
Tiganescu, A., Toma-Danila, D., Grecu, B., Craifaleanu, I.-G., Florin Balan, S., and Sorin Dragomir, C. (2021c). “Current Status and Perspectives on Seismic Monitoring of Structures and Rapid Seismic Loss Estimation in Romania,” in Proc. of the 1st Croatian Conference on Earthquake Engineering, 1CroCEE, Zagreb, Croatia. Editors S. Lakušić, and J. Atalić, 81–91. doi:10.5592/CO/1CroCEE.2021.120
Toma-Danila, D., and Armaş, I. (2017). Insights into the Possible Seismic Damage of Residential Buildings in Bucharest, Romania, at Neighborhood Resolution. Bull. Earthquake Eng. 15 (3), 1161–1184. doi:10.1007/s10518-016-9997-1
UTCB (2006). Cod de proiectare seismica – partea I –Prevederi de proiectare pentru cladiri P 100-1/2006 (Seismic design code - part I: Design prescriptions for buildings P 100-1/2006). Bucharest: Monitorul Oficial. Part I, No. 803bis of 25.09 (In Romanian).
UTCB (2013). Cod de proiectare seismica – partea I –Prevederi de proiectare pentru cladiri P 100-1/2013 (Seismic design code - part I: Design prescriptions for buildings P 100-1/2013). Bucharest: Monitorul Oficial. Part I, No. 558bis of 03.09.2013 (In Romanian).
Wenzel, F. (1997). Strong Earthquakes: A Challenge for Geosciences and Civil Engineering--A New Collaborative Research Center in Germany. Seismological Res. Lett. 68 (3), 438–443. doi:10.1785/gssrl.68.3.438
Wu, S., and Beck, J. L. (2012). Synergistic Combination of Systems for Structural Health Monitoring and Earthquake Early Warning for Structural Health Prognosis and Diagnosis. ProcSPIE 8348, 83481Z. doi:10.1117/12.914996
Keywords: seismic monitoring, structural health monitoring, building structures, seismic instrumentation, Vrancea earthquakes
Citation: Tiganescu A, Craifaleanu I-G, Aldea A, Grecu B, Vacareanu R, Toma-Danila D, Balan S-F and Dragomir C-S (2022) Evolution, Recent Progress and Perspectives of the Seismic Monitoring of Building Structures in Romania. Front. Earth Sci. 10:819153. doi: 10.3389/feart.2022.819153
Received: 20 November 2021; Accepted: 04 March 2022;
Published: 07 April 2022.
Edited by:
Dimitris Pitilakis, Aristotle University of Thessaloniki, GreeceReviewed by:
Sotirios Argyroudis, Brunel University London, United KingdomKosmas Dragos, Hamburg University of Technology, Germany
Copyright © 2022 Tiganescu, Craifaleanu, Aldea, Grecu, Vacareanu, Toma-Danila, Balan and Dragomir. This is an open-access article distributed under the terms of the Creative Commons Attribution License (CC BY). The use, distribution or reproduction in other forums is permitted, provided the original author(s) and the copyright owner(s) are credited and that the original publication in this journal is cited, in accordance with accepted academic practice. No use, distribution or reproduction is permitted which does not comply with these terms.
*Correspondence: Alexandru Tiganescu, YWxleGFuZHJ1LnRpZ2FuZXNjdUBpbmZwLnJv