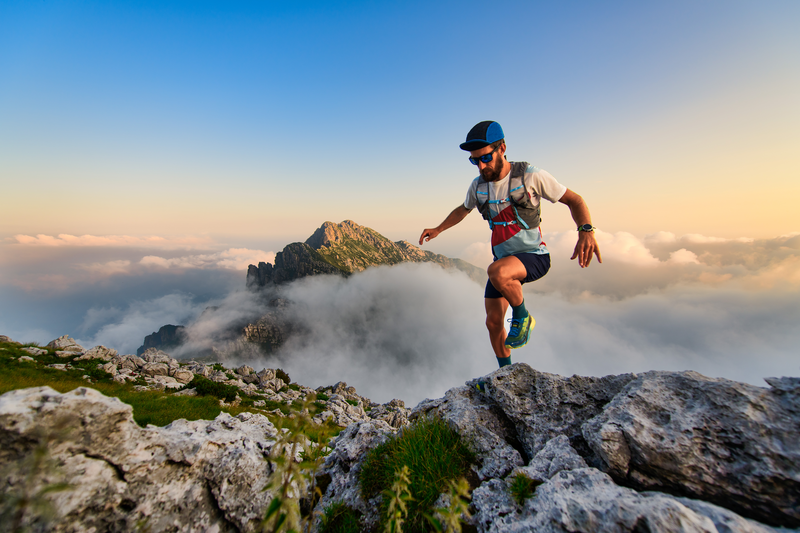
95% of researchers rate our articles as excellent or good
Learn more about the work of our research integrity team to safeguard the quality of each article we publish.
Find out more
ORIGINAL RESEARCH article
Front. Earth Sci. , 15 February 2022
Sec. Structural Geology and Tectonics
Volume 10 - 2022 | https://doi.org/10.3389/feart.2022.818171
This article is part of the Research Topic Tectono-Magmatism, Metallogenesis, and Sedimentation at Convergent Margins View all 13 articles
Post-subduction dextral faulting was restored to evaluate the spatial distribution of units of the Franciscan subduction complex of California that formed as a result of subduction accretion. The Franciscan and related rocks of western California exhibit significant along-strike variation in its recording of subduction-accretion processes. Most notably, two segments 830 km apart record subduction erosion associated with low-angle subduction events that took place at ca. 120 Ma and ca. 80–70 Ma in the north and south, respectively. This spatial relationship is not affected by restoration of post-subduction dextral slip because none of the slip passes between the two inboard tectonic windows. Between these segments the subduction complex records net accretion from ca.175 Ma to 12 Ma, but includes horizons recording non-accretion. None of the accreted units of the subduction complex are preserved over the entire length of the subduction complex. One unit, however, correlated on the basis of its structural level in the subduction complex and distinctive detrital zircon age spectra, accreted at about 80–83 Ma extends a strike length of 580 km, an amount increased to 850 km with restoration of post-subduction dextral faulting. The long-strike length of accretion of this unit demonstrates that detrital zircon age populations of subduction complex clastic rocks are poor indicators of strike-slip displacement. Some reaches of the subduction complex include schistose blueschist facies rocks (most Franciscan blueschist facies rocks are not schistose), whereas others do not, and some reaches lack blueschist facies rocks altogether. The significant along-strike variation in the Franciscan and related rock units reflects temporal and spatial differences in history of accretion, non-accretion, subduction erosion, and probably subduction dip. Similar time and space variation in processes and resultant geologic record should be expected for other subduction complexes of the world.
Modern subduction zones exhibit along-strike variation from subduction-accretion (subduction fault slices into downgoing plate; referred to as “accretion” in this paper), non-accretion (subduction fault does not cut into either upper plate or downgoing plate), and subduction erosion (subduction fault cuts into upper plate) (e.g., von Huene, 1986; von Huene and Scholl, 1991; Clift and Vannucchi, 2004). Recognizing the details of along-strike variation of accretion, non-accretion, and subduction erosion along exhumed paleo subduction zones is difficult because of the need to restore post-subduction deformation and the challenges posed in identifying subduction erosion and non-accretionary horizons along paleo-subduction interfaces exhumed from depth ranges that are commonly >10 km beneath the sea floor (e.g., Dumitru et al., 2010; Wakabayashi, 2015, Wakabayashi, 2021a; Chapman et al., 2016). The difficulty in recognizing non-accretionary or eroding paleo subduction interfaces in such exhumed rocks is because the depth of exposure/exhumation is well below that directly observed in sea floor studies, including drilling.
This paper restores post-subduction strike-slip and related faulting to examine the spatial relationships of subduction complex rocks of the Franciscan Complex of California that formed by sporadic subduction-accretion from ca. 175 to 12 Ma prior to disruption by the post-subduction San Andreas fault system (Hamilton, 1969; Ernst, 1970; Maxwell, 1974; Blake et al., 1988; McLaughlin et al., 1996; Ernst et al., 2009; Dumitru et al., 2010; Snow et al., 2010; Dumitru et al., 2013; Dumitru et al., 2015; Dumitru et al., 2018; Raymond, 2015; Raymond and Bero, 2015; Wakabayashi, 2015; Mulcahy et al., 2018; Raymond, 2018; Apen et al., 2021; Wakabayashi, 2021a) (Figure 1 current map view, Figure 2 current cross sections, restored map view Figure 3, cross sections of restored geology Figure 4; lists current compared to restored spatial relationships). The map view and inferred cross sectional relationships of Franciscan Complex units are then evaluated in the context of the history of accretion, non-accretion, and subduction erosion in space and time. This paper presents a largely descriptive view of the three-dimensional relationship of Franciscan rocks and does not attempt to speculate on mechanisms that caused the along-strike variation recorded in the subduction complex.
FIGURE 1. Map showing the Franciscan Complex and related rocks in coastal California as well post-subduction dextral faults of the San Andreas fault system, post-subduction volcanic rocks, and some details of pre-Franciscan and upper plate rocks. Revised from Wakabayashi (2015), Chapman et al. (2016), Kuiper and Wakabayashi (2018) and Chapman et al. (2021). Post-subduction dextral fault offsets (revised from Wakabayashi, 1999) used in the restoration are shown.
FIGURE 2. Cross sections of present-day geologic relationships. Subsurface interpretations are partly based on Godfrey et al. (1997), Langenheim et al. (2013), and Wakabayashi (2015). Colors are the same as those used in Figure 1 except where specifically noted. Note that arc and ophiolitic rocks correlative to the Great Valley ophiolite that may crop out in the western Sierra Nevada are not colored in Figure 1, but are shown with the same color as Coast Range ophiolite volcanic and plutonic rocks on Figure 2.
FIGURE 3. Map showing Franciscan Complex rocks restoring dextral faulting of the San Andreas fault system largely following Wakabayashi (1999) and Chapman et al. (2016) for the southern areas. See text for details of the restoration method.
FIGURE 4. Cross sections of Franciscan Complex and related rocks with post-subduction dextral faulting restored. See text for details. Colors are the same as those used in Figures 1–3 except where directly labeled.
Western California includes outcrops of the Franciscan subduction complex, as well as the components of the “upper plate” of the subduction system (never part of downgoing plate) that comprises the Coast Range ophiolite and the Great Valley Group forearc basin deposits that depositionally overlie the ophiolite (Figures 1, 2) (e.g., Hamilton, 1969; Ernst, 1970, Dickinson, 1970, Hopson et al., 1981; Hopson et al., 2008). The upper plate rocks structurally overlie the Franciscan subduction complex (Hamilton, 1969; Dickinson, 1970; Ernst, 1970) along a fault known as the Coast Range fault, which records differential exhumation of the Franciscan relative to the upper plate (e.g., Suppe, 1973; Platt, 1986; Jayko et al., 1987; Harms et al., 1992; Ring and Brandon, 1994; Unruh et al., 2007). The upper plate rocks display neglible burial metamorphism and record comparatively little deformation compared to the Franciscan (Dickinson et al., 1969; Hopson et al., 1981; Evarts and Schiffman, 1983; Dumitru, 1988; Hopson et al., 2008; Ring, 2008).
Franciscan rocks consist primarily of clastic sedimentary rocks with volumetrically smaller amounts of mafic volcanic rocks, chert, serpentinite, and very rare limestone (e.g., Berkland et al., 1972; Maxwell, 1974; Blake et al., 1988; Wakabayashi, 2015; Raymond, 2018). Note that on Figure 1 areas rich in mafic volcanic rocks with chert or limestone are delimited by “vf” but even in those areas have a significant fraction of clastic sedimentary rocks (see detailed maps two of the most volcanic and chert rich areas in the Franciscan: the Marin Headlands of Wahrhaftig, 1984, and Mt. Diablo of Wakabayashi, 2021b). The Franciscan is well-known for having high-pressure/low-temperature (HP-LT) metamorphic rocks that resulted from subduction (Ernst, 1970) but HP-LT metamorphic rocks of lawsonite-albite or blueschist facies or higher grade (blue and dark blue on Figure 1) make up only about a fourth of Franciscan rocks (Blake et al., 1984; Blake et al., 1988; Ernst, 1993, Terabayashi and Maruyama, 1998; Ernst and McLaughlin, 2012). A relatively small fraction of these HP-LT rocks (dark blue on Figure 1) are schistose and completely or nearly completely recrystallized (Blake et al., 1988; Wakabayashi, 2015). The Franciscan consists of block-in-matrix mélange and non-mélange “coherent” horizons, with the proportion of mélange varying significantly from one area to another (Berkland et al., 1972; Blake et al., 1984; Blake et al., 1988; Cloos and Shreve, 1988a; Cloos and Shreve, 1988b; Wakabayashi, 2015; Raymond, 2018).
Different researchers define Franciscan units differently (e.g., Blake et al., 1982, 1984, 1988; Raymond, 2015; Wakabayashi, 2015; Ernst, 2017; Wakabayashi, 2021a; Raymond et al., 2019; Apen et al., 2021) so it is useful to specify how units are defined in this paper. This paper follows the approach of Wakabayashi, 2015; Wakabayashi, 2021a). Units are delimited by age of accretion, estimated by radioisotopic ages of metamorphism, or by depositional age of clastic rocks, constrained by biostratigraphy and/or maximum depositional ages from U-Pb age determinations of detrital zircons and/or specific age-probability distributions of detrital zircon ages. Many individual outcrops lack geochronologic data, so for such rocks correlations are made to dated rocks on the basis of similarity in relative structural position within the Franciscan and similarities in lithologic character. It is beyond the scope of this paper to debate merits of alternative unit nomenclature schemes. The pronounced along-strike variation in the subduction complex is apparent regardless of the unit definitions used.
A slice of continental magmatic arc rocks, called the Salinian block, correlative to rocks of the southern Sierra Nevada magmatic arc and the related rocks to the south, crops out west of the main belt of Franciscan Complex exposures and east of the subduction complex rocks known as the Nacimiento belt (Figure 1) (Chapman et al., 2016). The rocks associated the paleo trench-forearc system (Franciscan, Coast Range ophiolite, Great Valley Group) are unconformably overlain by post-subduction volcanic and sedimentary deposits associated with the post-subduction dextral plate boundary, represented by the San Andreas fault system (only post-subduction volcanic deposits shown on Figure 1) (e.g., Page, 1981).
Although Franciscan, Great Valley Group and Coast Range ophiolite units dip steeply in most outcrops, the regional dips of unit boundaries are low-angle, as demonstrated by the traces of contacts over topography, so that the general architecture is that of folded low-angle sheets (Langenheim et al., 2013; Wakabayashi, 2015). Most regional-scale folds of the Franciscan are west-vergent and overturned, so easterly dips predominate (Wakabayashi, 2015) (shown somewhat schematically in Figure 2). Where post-subduction deposits overlap Franciscan and related rocks, most of the folding of the Franciscan rocks appears to predate this overlap, whereas some older folds have been tightened by post-subduction shortening (examples in Figures 7, 8 of Wakabayashi, 2021a). The Coast Range fault and overlying Great Valley Group has also experienced folding prior to subduction termination, as illustrated by the overlap of this fault by deposits that date from early in the post-subduction regime (for example, see maps of Blake et al., 2000; 2002). Internal imbrication of Franciscan units as well as some of their folding predates syn-subduction movement along the Coast Range fault as illustrated by the truncation of internal Franciscan structure by the fault (Wakabayashi, 2021b) (Figure 2).
Post-subduction dextral faulting has significantly impacted the map-view spatial relationships of Franciscan units (e.g., Page, 1981; McLaughlin et al., 1996; Wakabayashi, 1999). In contrast, the amount of post-exhumation has been <3 km for most of exposed Franciscan rocks, so post-subduction metamorphic overprinting is lacking (Dumitru, 1989; Unruh et al., 2007). At deeper levels in the California Coast Ranges metamorphic overprinted and intrusion of post-subduction plutons is expected (Figure 2) from post-subduction increase in geothermal gradients and magmatism related to slab window development (Furlong, 1984; Liu and Furlong, 1992; Wakabayashi, 1996; Kuiper and Wakabayashi, 2018). This post-subduction metamorphism is recorded in some xenoliths in post-subduction volcanic rocks (Stimac, 1993; Metzger et al., 2005) but not in exposed rocks, owing to the lack of sufficient exhumation.
The deformation associated with the dextral San Andreas fault system that has replaced east-dipping subduction associated with Franciscan subduction has been primarily accommodated by dextral faults, with subordinate local shortening or extension (Atwater, 1970; Argus and Gordon, 1991; Atwater and Stock, 1998; Argus and Gordon, 2001). In addition to slight discordance between the azimuth of relative plate motion and the Pacific-North American plate boundary that may have been recorded by small convergent or divergent components along the otherwise dextral plate margin (e.g., Argus and Gordon, 1991; Atwater and Stock, 1998; Argus and Gordon, 2001), local transpression and transtension has been associated with the development of step-overs and bends along the dextral faults (Aydin and Page, 1984; Wakabayashi et al., 2004; Wakabayashi, 2007; Unruh et al., 2007).
Transform fault system deformation and slip has been restored by the following procedure: First, displacements along the various dextral faults of the San Andreas fault system were restored following the slip assignments of Wakabayashi (1999) (main displacements shown on Figure 1). Because many of the faults have bends and step-overs, gaps and minor overlaps result from dextral fault restoration. In addition, there are many faults that cut Franciscan rocks that have been proposed to be slip-transfer faults associated with fault step-overs (e.g., Wakabayashi et al., 2004; Wakabayashi, 2007; Kuiper and Wakabayashi, 2018). Accordingly, Franciscan units have been restored across various mapped dextral faults of the San Andreas fault system, but with additional smaller-scale slivering. The areas labeled with “distributed” deformation on Figure 1 (between 38° and 39.5°N latitude) are examples. Other areas restored with distributed slip include region NW of the northern tip of the West Napa fault, and the region between the Healdsburg-Rodgers Creek-Hayward faults and the San Andreas fault. Gaps and overlaps are removed in the restored map relationships.
Surface erosion since the termination of subduction has changed the outcrop pattern, but the details of this erosion are poorly known. Because of the uncertainty in restoring erosion, and the qualitative nature of accommodation of distributed deformation and step-overs and bends (removing gaps and overlaps), the restored Franciscan geology is shown with much less detail in Figure 3 compared that of the present-day Figure 1. For the southernmost part of the Franciscan Complex, represented by the Nacimiento Belt, the restoration of inboard interpreted tectonic windows (including the Sierra de Salinas, Rand, and Pelona schists) largely follows Chapman et al. (2016) with the minor adjustment of including ∼55 km of sinistral slip on the Garlock Fault (Hatem and Dolan, 2018).
The restoration leaves the northern end of the Salinian block projecting about 175 km northwest of the southernmost inboard Franciscan exposures (Figure 3). This may reflect 175 km of syn-subduction dextral slip along a “proto San Andreas fault” (e.g., Page, 1981) or the west-directed low-angle faulting of a relatively thin crystalline sheets or sheets derived from the southernmost Sierra Nevada and regions to the south by west-directed low-angle faulting associated with earliest Paleogene thrusting (Hall, 1991) or latest Cretaceous extensional collapse (Chapman et al., 2012). If the eastern contact of the northern Salinian block is a syn-subduction dextral fault (Page 1981), Nacimiento Belt exposures restore an additional 175 km further southeastward relative to the inboard main Franciscan outcrop belt than the position shown in Figure 3. The cross sections of Figures 2, 4 have been constructed following a model of a thin Salinian block originally emplaced by westward-directed low-angle faulting (e.g., Hall, 1991; Chapman et al., 2012) whereas the model of Page (1981) would result in a thicker Salinian block bounded by a high-angle fault on its east. It is beyond the scope of this paper to discuss the relative strengths of the two types of proposals for Salinian block emplacement, and these different models to not impact the interpretations of this study about along-strike variation of Franciscan rocks with the exception of the relative position between the Nacimiento Belt and inboard main Franciscan outcrop belt.
There have been alternative schemes presented for slip distribution on the San Andreas fault system, such as Powell (1993) and McLaughlin et al., 1982; for the eastern part of the fault system). Units east of all of the strands of San Andreas fault system are unaffected by different models of slip distribution on the various dextral strands. The unaffected units include the relative position tectonic windows of the Condrey Mtn. inner unit and Rand schists (830 km between them), as well as various features along the eastern margin of the Coast Ranges such as the South Fork Mtn. schist and related units.
The positions of other Franciscan units relative to one another are also largely unaffected by the choice of slip distribution between the two alternatives noted above and Wakabayashi (1999). The primary difference is that Powell (1993) and McLaughlin et al., 1996 restore the southernmost limit of Franciscan exposures east of the Salinian block to a position more than 100 km north of the position proposed by Wakabayashi (1999). Accordingly, the general pattern of along-strike variation of Franciscan rocks is not significantly different between different restoration schemes.
The restoration of San Andreas fault slip lengthens Franciscan Complex main exposure belt (excluding Nacimiento Belt) from 830 km to a restored length of about 980 km (Figures 1, 3). If the Nacimiento Belt is included, the outcrop belt currently has a total length of 980 km and a restored length of about 1,300 km.
The amount of post-subduction dextral slip cutting Franciscan exposures decreases northward, reflecting the progressive northward lengthening of the transform fault system and progressive propagation of the eastern faults of the system (e.g., Wakabayashi, 1999; Wakabayashi et al., 2004; Wakabayashi 2007). For this reason, the Franciscan units of the northern (north of ∼39°N on Figure 1) Coast Ranges (Fig. 4ab) are relatively unaffected by restoration of such dextral faulting (Figures 1, 3). As apparent in past analyses of Franciscan accreted units, such as those of Wakabayashi (2015), there are significant differences in the tectonic stack of units along strike, with no single unit extending the length of the belt (Figure 3).
The most extensive unit in the Franciscan in terms of along-strike extent occupies one of the lowest structural positions among prehnite-pumpellyite facies units in the main Franciscan outcrop belt, as well as constituting part of the Nacimiento Belt, has distinctive detrital zircon age spectra, and apparently was deposited and accreted about 80–83 Ma (Type E unit of Dumitru et al., 2016 in the Franciscan and Nacimiento Belt; Wakabayashi, 2015 for structural context). This clastic-dominated unit crops out along a strike distance of 580 km in present-day exposures and 830 km with restoration of post-subduction dextral faulting (northern and southern limits labeled as E(N) and E(S) respectively on Figures 1, 3). Although mapping and sampling to confirm correlations of this unit are far from exhaustive, published mapping and field observations suggest that this unit forms a reasonably continuous belt of exposures with the exception of the gap between the Nacimiento Belt and main Franciscan exposure belt (Wakabayashi, 2015; Dumitru et al., 2016). Structural thickness of the unit within the main Franciscan exposure belt reaches 3 km (Wakabayashi, 2015; Wakabayashi, 2021a).
The next most extensive unit that forms a close to continuous belt of outcrops is that of the South Fork Mtn. schist and correlative units (Blake et al., 1988; Dumitru et al., 2010; Chapman et al., 2021). This unit of schistose blueschist and greenschist facies rocks was subducted, accreted, and metamorphosed at about 121 Ma (Ar-Ar phengite, Dumitru et al., 2010), and makes up the dark blue units on Figure 1 in the northern Coast Ranges north of ∼39°N but exclusive of the Condrey Mtn schist inner unit. These rocks crop out over a distance of about 420 km and a restored distance of about 450 km (Figures 1, 3), and attain a structural thickness of up to about 5 km (Worrall, 1981; Schmidt and Platt, 2018).
The comparative continuity of the extensive ca. 80–83 Ma unit and the South Fork Mtn. schist contrasts markedly with the high-grade (amphibolite, garnet-amphibolite, eclogite, with varying degrees of blueschist overprint) coherent sheets that locally make up the structurally highest and oldest units in the Franciscan and have the same metamorphic ages and metamorphic assemblages as blocks-in-mélange (commonly referred to as “high-grade blocks”) (e.g., Ernst et al., 1970; Raymond, 1973; Suppe and Foland, 1978; Wakabayashi et al., 2010; Wakabayashi, 2015). Peak metamorphic ages of the high-grade Franciscan rocks, that are interpreted to reflect subduction and accretion range from about 155 to 176 Ma (Ross and Sharp, 1988; Ancziewicz et al., 2004; Wakabayashi and Dumitru 2007; Mulcahy et al., 2018; Rutte et al., 2020). The coherent high-grade sheets crop out over a distance of 330 km along the eastern margin of the Coast Ranges (Goat Mtn at the northern limit and “csch (S)” the southern limit on Figure 1, but “Ward Creek csch (S)" for the southern limit on Figure 3), and possibly 500 km if a structurally high amphibolite body in the Stanley Mtn window of the Nacimiento Belt is a coherent slab instead of a block-in-mélange (Brown, 1968) (“SMcsch(S)" on Figures 1, 3). These sheets are small (all but two <2 km in long dimension, with thicknesses of hundreds of m) and rare (widely scattered). If the Stanley Mtn window exposure is such a slab then the restored along-strike distance of high-grade slab outcrops is about 800 km, whereas without the Stanley Mtn area exposure, whereas the other restored outcrops span an along strike distance of about 350 km (Figure 3).
The Marin Headlands terrane, a unit rich in basalt and chert that includes the oldest oceanic crust preserved in Franciscan units (e.g., Murchey and Jones, 1984) currently extends a distance of about 220 km, and this distance is increased to 420 km with restoration of post-subduction faulting (“MH(N)” and “MH(S)” on Figures 1, 3). The remnants of the Marin Headlands terrane do not form a continuous belt of outcrops whereas they are more voluminous and less scattered than the high-grade coherent sheets; locally the unit attains a maximum structural thickness of about 2.5 km (Wahrhaftig, 1984).
Some units, such as the limestone-bearing, basalt-rich Permanente terrane (Blake et al., 1984; McLaughlin et al., 1996) are reduced in outcrop length by restoration (“P(N)” and “P(S)” on Figures 1, 3). The current outcrop length of Permanente terrane is 260 km, and this collapses to about 110 km with restoration of dextral faults; the Permanente terrane forms a fairly continuous belt of exposures with a structural thickness of up to about 3 km (Wakabayashi, 2015; Wakabayashi, 2021a).
The Skaggs Springs, a completely recrystallized (in most exposures) glaucophane-lawsonite-quartz metaclastic schist (Wakabayashi, 1999) that has yielded phengite Ar-Ar ages of ca. 132 Ma (Wakabayashi and Dumitru, 2007), also forms a fairly continuous belt of exposures. This unit attains a structural thickness of up to 2 km (Wakabayashi, 2021a) and spans a distance of 330 km currently, but only about 100 km with restoration of dextral faulting (“SSS” thin Dark blue unit between ∼38.5 and 39°N and parts of very small remnants between ∼36.5 and 37°N on Figure 1).
Whereas the Permanente terrane limestones and Laytonville limestone of the Franciscan are of approximately the same age (e.g, Sliter, 1984; Sliter and McGann, 1992), their paleolatitude of deposition in the open ocean differs with the Permanente terrane limestones apparently having formed north of the equator (Tarduno et al., 1985), whereas the Laytonville limestone (“L(N)” and “L(S)” on Figures 1, 3) formed south of the equator (Alvarez et al., 1980; Tarduno et al., 1990). It may be potentially useful, however, to examine the along strike extent of these units collectively as they reflect accretion of possible oceanic highs (seamounts, oceanic plateau) of about the same age. They crop out over a distance of 500 km currently and 670 km with dextral faulting restored (From “L(N)” to “P(S)” on Figures 1, 3).
Note that whereas the Franciscan is well known for its high-pressure/low-temperature blueschist facies and lawsonite-albite facies metamorphic rocks (e.g., Ernst 1970; 1975), some restored reaches lack such rocks (Figure 3). Schistose blueschist facies units of regional extent, the Skaggs Springs schist and the South Fork Mtn. schist are absent over larger reaches of the restored subduction complex.
As noted previously, the low-angle regional contacts between various Franciscan units are unaffected by restoration of post-subduction dextral faulting. Because of this, the general pattern of accretionary ages that young structurally downward (Ernst et al., 2009; Dumitru et al., 2010; Snow et al., 2010; Wakabayashi, 2015; Wakabayashi, 2021b) is also unchanged by restoration of post-subduction faulting.
FEART_feart-2022-818171_wc_f1The most significant along-strike variation in the Franciscan and related rocks, the distance between subduction complex units and coeval arc plutons, is not impacted by restoration of post-subduction dextral slip. The distance between Franciscan units and coeval arc plutons is especially short adjacent to the Klamath Mtns and to the south (Transverse Ranges and southward) and it associated with correlative inboard tectonic windows of high-pressure metamorphic rocks (Chapman et al., 2016; Chapman et al., 2021) (Figures 1, 3). These relationships reflect low-angle subduction and subduction erosion that took place at ca. 121 Ma to the north (Chapman et al., 2021) and ca. 80–70 Ma to the south (Chapman et al., 2016) with about 800 km between the two reaches (Figures 1, 3). The length of subduction zone associated with the southern subduction erosion reach may be estimated by the 500 km along-strike extent of the restored position of the tectonic windows (Chapman et al., 2016; note the southernmost windows restore well south of the limit of Figure 3). The length of the northern reach that accommodated subduction erosion is difficult to evaluate because only one tectonic window into subduction complex rocks has been found; it is possible that other tectonic windows are buried beneath Cenozoic volcanic rocks of the Cascade arc (Chapman et al., 2021).
In addition to the different time of subduction and metamorphism for the two apparent low-angle subduction segments, there are other differences in spatial relationships and metamorphism that may reflect different tectonic mechanisms. The South Fork Mtn. schist is about 75 km across-strike from the correlative inner Condrey Mtn schist unit. By comparison, the most proximal windows of the Schist de Salinas-Rand and related schists are about 40 km from the correlative outboard subduction complex rocks. The blueschist and greenschist facies metamorphism of the outboard South Fork Mtn schist and related units is reasonably similar to the blueschist and greenschist facies metamorphism of the Inner Condrey Mtn schist (Chapman et al., 2021). In contrast, the outboard (Nacimiento Belt), zeolite, prehnite-pumpellyite, and lawsonite-albite rocks have correlative rocks in various windows metamorphosed at amphibolite grade (Chapman et al., 2016).
Although accretion took place along both the northern and southern reaches described above, beginning with the rocks now exposed in the windows and including rocks structurally below outboard correlatives, these reaches of the paleosubduction system record net subduction erosion in contrast to the net accretion of the 800 km reach between that records sporadic accretion from ca. 175 Ma to 12 Ma (McLaughlin et al., 1998; Mulcahy et al., 2014; Wakabayashi, 2015; 2021a). Non-accretion is recorded by gaps in time of accretion between adjacent units (Dumitru et al., 2010; Wakabayashi 2021a). The reach of net accretion includes at least one horizon that records prolonged non-accretion of tens of My (Dumitru et al., 2010), but without net subduction erosion, owing to the preservation of the rare high-grade sheets above this horizon that are interpreted to have had limited original structural thickness (≤500 m) (Wakabayashi, 2015). Other horizons may reflect varying periods of non-accretion and the possibility of subduction erosion of previously accreted material (Wakabayashi, 2015; Wakabayashi, 2021a).
Details of accretion, accretion history, and possibly exhumation are reflected in the smaller-scale differences between the subduction complex tectonic stack at various positions along the restored paleo convergent margin (Figures 3, 4). These differences reflect the fact that no accreted unit has been preserved along the entire length of the restored subduction complex, including high-pressure metamorphic units.
Whereas no unit accreted or was preserved from along the entire length of the subduction complex, some accreted along distances of several hundred km. The most extensive such unit is the 80–82 Ma Type E unit of (Dumitru et al., 2016) that accreted over a strike length of about 830 km (restored length) (Figure 3). Such a strike length apparently reflects a long distance of trench-parallel clastic sediment transport (Dumitru et al., 2016). This unit is the most extreme example of other Franciscan units (all of which contain clastic components) of hundreds of km in along-strike extent, illustrating that detrital zircon age populations of subduction complex rocks are poor piercing points for estimating along-strike post-depositional tectonic transport. The Franciscan units with this detrital zircon age population appear to represent trench deposition and accretion at ca. 80–82 Ma, whereas forearc basin (Great Valley Group and correlatives) rocks with this detrital zircon age population appear to represent two separate depositional events (80–82 Ma and 71–75 Ma) (Surpless, 2015; Dumitru et al., 2016). Taken collectively, the Great Valley Group and correlative rocks and Franciscan rocks with this detrital zircon age population crop out over an along-strike distance of about 1,130 km (restored; present distance is about 860 km). Along strike distances between zircon sources and deposits may be further increased if fluvial or aeolian transport of zircon-bearing sediment had a significant along-strike component; the additional along-strike distance between zircon sources and deposits may exceed 500 km (e.g., Dumitru et al., 2013; 2016).
The deeper parts of the cross sections of Figures 2, 4 are speculative owing to the comparative lack of subsurface information. Whereas the low-angle nature of regional contacts and steep local dips, apparent in present-day map-view contact geometry, is retained in the restored relationships, the deeper subsurface geology is poorly constrained. For those sections in the net accreting reach of the paleo subduction system (Figures 4B–E), the deeper subsurface interpretations are based partly on seismic and potential field data interpretations of Godfrey et al. (1997), as well as interpretations presented in Wakabayashi and Unruh (1995) and Wakabayashi (2015).
The sections with the inboard tectonic windows associated with net tectonic erosion (Figures 4A,F,G) present particularly daunting cross-sectional reference frame issues associated with the apparent steepening of subduction dip that took place after the subduction erosion events. This steepening of subduction dip was reflected by the westward migration of arc magmatism to a position similar to where it was prior to shut off of Sierra Nevada arc magmatism associated with the shallow slab/subduction erosion event (Busby et al., 2008). The steepening dip of the subducting slab resulted in a large vertical distance between inboard accreted units and the position of the subducted slab. Whereas an alternative is to fill this volume with accreted material, this is an immense thickness of accreted material (>50 km) and this is well in excess of the structural thickness of any exhumed subduction complex exposed in orogenic belts of the world. Steepening of slab dip is essentially slab rollback and this may have resulted in the trenchward flow of upper plate mantle beneath previously-accreted rocks of the tectonic windows. However, no examples exist in orogenic belts of large slabs of mantle (kms to tens of km thick) structurally intermediate between subduction complex rocks or below subduction complex rocks. On the other hand, such mantle material beneath subduction complexes may not have been exhumed in any of the world’s orogenic belts and the density of such mantle material may preclude exhumation.
In an attempt to address the structural-tectonic condundrum posed above, I have tentatively proposed arc-vergent thrusting, associated with “tectonic wedging”, as has been interpreted for the net-accretionary reach in the past (Wentworth et al., 1984; Unruh et al., 1991: Wakabayashi and Unruh, 1995), for the northern window (Condrey Mtn. schist inner unit) (Figure 4A). This east-vergent thrusting is interpreted to have taken place after syn-subduction extensional exhumation of the tectonic windows, similar to the scenario for the net-accretionary reaches as proposed by Wakabayashi and Unruh (1995). A potential problem with the tectonic wedging mechanism for the northern tectonic window is that it requires a far greater amount of shortening than that proposed for the net accretionary reach (Figure 4A compared to Figures 4B–E). Some researchers argued against the presence of syn-subduction tectonic wedge structures along the net accretionary reach (e.g., Costenius et al., 2000; Dickinson, 2002), whereas seismically-active tectonic wedge structures are not disputed (e.g., Wentworth et al., 1984; Namson and Davis, 1988; Unruh and Moores, 1992) (Figure 2).
The potential amount of shortening associated with hypothetical wedge structures would be even greater for the southern California tectonic windows (Figures 4F,G) that would require hundreds of km of shortening by tectonic wedging to place the most inboard windows (east of those shown in the cross sections) on the upper plate of such a thrust system. Accordingly, a compromise solution was attempted for the southern California cross sections that interprets significant accretion (30 km thick) before steepening of the slab and flow of mantle westward. This is a far higher rate of accretion than any exposed Franciscan section, so this may be unrealistic. Thus, the tentative cross sectional interpretations presented for the tectonic window reaches (Figures 4A,F,G) should be considered speculative, likely unrealistic, and they point to the need for detailed subsurface (seismic and potential field) data.
The Franciscan subduction complex exhibits significant along-strike variation, reflecting along-strike differences in the history of accretion, non-accretion, and subduction erosion, and likely slab dip. This along-strike difference is apparent, whether examining present-day relationships or those with post-subduction dextral faulting restored. Whereas different models have been presented for the distribution of post-subduction dextral slip, the use of these models to restore dextral slip has a minimal impact on the apparent along-strike variation in subduction complex rocks.
The original contributions presented in the study are included in the article/Supplementary Material, further inquiries can be directed to the corresponding author.
JW conducted the research and wrote the paper.
Some of the foundational research for this paper was supported by National Science Foundation Grants EAR-0635767, EAR-0948676, EAR-1032156.
The author declares that the research was conducted in the absence of any commercial or financial relationships that could be construed as a potential conflict of interest.
All claims expressed in this article are solely those of the authors and do not necessarily represent those of their affiliated organizations, or those of the publisher, the editors and the reviewers. Any product that may be evaluated in this article, or claim that may be made by its manufacturer, is not guaranteed or endorsed by the publisher.
I thank reviewers for their thorough and thoughtful comments on the manuscript.
Alvarez, W., Kent, D. V., Premoli Silva, I., Schweickert, R. A., and Larson, R. A. (1980). Franciscan Complex limestone Deposited at 17° South Paleolatitude. Geol. Soc. America Bull. 91, 476–484. doi:10.1130/0016-7606(1980)91<476:fcldas>2.0.co;2
Anczkiewicz, R., Platt, J. P., Thirlwall, M. F., and Wakabayashi, J. (2004). Franciscan Subduction off to a Slow Start: Evidence from High-Precision Lu-Hf Garnet Ages on High Grade-Blocks. Earth Planet. Sci. Lett. 225, 147–161. doi:10.1016/j.epsl.2004.06.003
Apen, F., Wakabayashi, J., Day, H., Roeske, S., Souders, A. K., and Dumitru, T. (2021). Regional-scale Correlations of Accreted Units in the Franciscan Complex: A Record of Long-Lived, Episodic Subduction Accretion. Geol. Soc. America 552. doi:10.1130/2021.2552(11)
Argus, D. F., and Gordon, R. G. (1991). Current Sierra Nevada-North America Motion from Very Long Baseline interferometry:Implications for the Kinematics of the Western United States. Geol 19, 1085–1088. doi:10.1130/0091-7613(1991)019<1085:csnnam>2.3.co;2
Argus, D. F., and Gordon, R. G. (2001). Present Tectonic Motion across the Coast Ranges and San Andreas Fault System in central California. Geol. Soc. America Bull. 113, 1580–1592. doi:10.1130/0016-7606(2001)113<1580:ptmatc>2.0.co;2
Atwater, T. (1970). Implications of Plate Tectonics for the Cenozoic Tectonic Evolution of Western North America. Geol. Soc. America Bull. 81, 3513–3536. doi:10.1130/0016-7606(1970)81[3513:ioptft]2.0.co;2
Atwater, T., and Stock, J. (1998). Pacific-North America Plate Tectonics of the Neogene Southwestern United States: An Update. Int. Geology. Rev. 40, 375–402. doi:10.1080/00206819809465216
Aydin, A., and Page, B. M. (1984). Diverse Pliocene-Quaternary Tectonics in a Transform Environment, San Francisco Bay Region, California. Geol. Soc. America Bull. 95, 303–1317. doi:10.1130/0016-7606(1984)95<1303:dptiat>2.0.co;2
Berkland, J. O., Raymond, L. A., Kramer, J. C., Moores, E. M., and O'Day, M. (1972). What Is Franciscan. Am. Assoc. Pet. Geologists Bull. 56, 2295–2302. doi:10.1306/819a421a-16c5-11d7-8645000102c1865d
Blake, M. C., Graymer, R. W., and Jones, D. L. (2000). Geologic Map and Database of Parts of Marin, San Francisco, Contra Costa, and Sonoma Counties, 2337. California: U.S. Geological Survey Miscellaneous Field Studies Map MF.
Blake, M. C., Graymer, R. W., and Stamski, R. E. (2002). Geologic Map and Map Database of Western Sonoma, Northernmost Marin and Southernmost Mendocino Counties. California: US Geological Survey Miscellaneous Field Studies Map MF, 2402.
Blake, M. C., Howell, D. G., and Jayko, A. S. (1984). Tectonostratigraphic Terranes of the San Francisco Bay Region. Soc. Econ. Paleontologists Mineralogists 43, 5–22.
Blake, M. C., Howell, D. G., and Jones, D. L. (1982). Preliminary Tectonostratigraphic Terrane Map of California, U.S.A: Geological Survey Open File Report, 82–593.
Blake, M. C., Jayko, A. S., McLaughlin, R. J., and Underwood, M. B. (1988). “Metamorphic and Tectonic Evolution of the Franciscan Complex, Northern California,” in Metamorphism and Crustal Evolution of the Western United States. Editor W. G. Ernst (Englewood Cliffs, New Jersey: Prentice-Hall. Rubey Volume VII), 1035–1060.
Brown, J. A.. (1968)Thrust Contact Between Franciscan Group and Great Valley Sequence. Northeast Santa Maria, CA: Ph.D. Dissertation, Los Angeles, University of Southern California, 234.
Busby, C. J., Hagan, J. C., Putirka, K., Pluhar, C. J., Gans, P. B., Wagner, D. L., et al. (2008). The Ancestral Cascades Arc: Cenozoic Evolution of the central Sierra Nevada (California) and the Birth of the New Plate boundaryThe Ancestral Cascades Arc: Implications for the Development of the Sierran Microplate and Tectonic Significance of High K2O Volcanism. Geol. Soc. America Spec. Paper 438, 331–378. doi:10.1130/2008.2438(12)
Chapman, A. D., Jacobson, C. E., Ernst, W. G., Grove, M., Dumitru, T., Hourigan, J., et al. (2016). Assembling the World's Type Shallow Subduction Complex: Detrital Zircon Geochronologic Constraints on the Origin of the Nacimiento Block, central California Coast Ranges. Geosphere 12, 533–557. doi:10.1130/GES01257.1
Chapman, A. D., Saleeby, J. B., Wood, D. J., Piasecki, A., Kidder, S., Ducea, M. N., et al. (2012). Late Cretaceous Gravitational Collapse of the Southern Sierra Nevada Batholith, California. Geosphere 8 (2), 314–341.doi:10.1130/GES00740.1
Chapman*, A. D., Yule, D., Schmidt, W., and LaMaskin, T. (2021). Middle Jurassic to Early Cretaceous Tectonic Evolution of the Western Klamath Mountains and Outboard Franciscan Assemblages, Northern California-southern Oregon, USA. Geol. Soc. America Field Guide 62, 73–130. doi:10.1130/2021.0062(04)
Clift, P., and Vannucchi, P. (2004). Controls on Tectonic Accretion versus Erosion in Subduction Zones: Implications for the Origin and Recycling of the continental Crust. Rev. Geophys. 42, RG2001. doi:10.1029/2003RG000127
Cloos, M., and Shreve, R. L. (1988a). Subduction-channel Model of Prism Accretion, Melange Formation, Sediment Subduction, and Subduction Erosion at Convergent Plate Margins: 1. Background and Description. Pageoph 128, 455–500. doi:10.1007/bf00874548
Cloos, M., and Shreve, R. L. (1988b). Subduction-channel Model of Prism Accretion, Melange Formation, Sediment Subduction, and Subduction Erosion at Convergent Plate Margins: 2. Implications and Discussion. Pageoph 128, 501–545. doi:10.1007/bf00874549
Costenius, K. N., Johnson, R. A., Dickinson, W. R., and Williams, T. A. (2000). Tectonic Evolution of the Jurassic-Cretaceous Great Valley Forearc, California: Implications for the Franciscan Thrust-Wedge Hypothesis. Geol. Soc. America Bull. 112, 1703–1723. doi:10.1130/0016-7606(2000)112<1703:TEOTJC>2.0.CO;2
Dickinson, W. R., Ojakangas, R. W., and Stewart, R. J. (1969). Burial Metamorphism of the Late Mesozoic Great Valley Sequence, Cache Creek, California. Geol. Soc. America Bull. 80, 519–526. doi:10.1130/0016-7606(1969)80[519:bmotlm]2.0.co;2
Dickinson, W. R. (2002). Reappraisal of Hypothetical Franciscan Thrust Wedging at Coalinga: Implications for Tectonic Relations along the Great Valley Flank of the California Coast Ranges. Tectonics 21, 1039. doi:10.1029/2001/TC00131510.1029/2001tc001315
Dickinson, W. R. (1970). Relations of Andesites, Granites, and Derivative Sandstones to Arc-Trench Tectonics. Rev. Geophys. 8, 813–860. doi:10.1029/rg008i004p00813
Dumitru, T. A. (1989). Constraints on Uplift in the Franciscan Subduction Complex from Apatite Fission Track Analysis. Tectonics 8, 197–220. doi:10.1029/tc008i002p00197
Dumitru, T. A., Elder, W. P., Hourigan, J. K., Chapman, A. D., Graham, S. A., and Wakabayashi, J. (2016). Four Cordilleran Paleorivers that Connected Sevier Thrust Zones in Idaho to Depocenters in California, Washington, Wyoming, and, Indirectly, Alaska. Geology 44, 75–78. doi:10.1130/g37286.1
Dumitru, T. A., Ernst, W. G., Hourigan, J. K., and McLaughlin, R. J. (2015). Detrital Zircon U-Pb Reconnaissance of the Franciscan Subduction Complex in Northwestern California. Int. Geology. Rev. 57, 767–800. doi:10.1080/00206814.2015.1008060
Dumitru, T. A., Ernst, W. G., Wright, J. E., Wooden, J. L., Wells, R. E., Farmer, L. P., et al. (2013). Eocene Extension in Idaho Generated Massive Sediment Floods into the Franciscan Trench and into the Tyee, Great Valley, and Green River Basins. Green. River Basins: Geology. 41, 187–190. doi:10.1130/G33746.1
Dumitru, T. A., Hourigan, J. K., Elder, W. P., Ernst, W. G., and Joesten, R. (2018). New, Much Younger Ages for the Yolla Bolly Terrane and a Revised Time Line for Accretion in the Franciscan Subduction Complex, California, Geol. Soc. America Spec. Paper 540, 339–366. doi:10.1130/2018.2540(15)
Dumitru, T. A. (1988). Subnormal Geothermal Gradients in the Great Valley Forearc basin, California, during Franciscan Subduction: A Fission Track Study. Tectonics 7, 1201–1221. doi:10.1029/tc007i006p01201
Dumitru, T. A., Wakabayashi, J., Wright, J. E., and Wooden, J. L. (2010). Early Cretaceous Transition from Nonaccretionary Behavior to Strongly Accretionary Behavior within the Franciscan Subduction Complex. Tectonics 29, a–n. doi:10.1029/2009TC002542
Ernst, W. G. (2017). Geologic Evolution of a Cretaceous Tectonometamorphic Unit in the Franciscan Complex, Western California. Int. Geology. Rev. 59, 563–576. doi:10.1080/00206814.2016.1201440
Ernst, W. G., Martens, U., and Valencia, V. (2009). U-pb Ages of Detrital Zircons in Pacheco Pass Metagraywackes: Sierran-Klamath Source of Mid-cretaceous and Late Cretaceous Franciscan Deposition and Underplating. Tectonics 28, TC6011. doi:10.2019/2008TC00235210.1029/2008tc002352
Ernst, W. G., and McLaughlin, R. J. (2012). Mineral Parageneses, Regional Architecture, and Tectonic Evolution of Franciscan Metagraywackes, Cape Mendocino-Garberville-Covelo 30′ × 60′ Quadrangles, Northwest California. Tectonics 31, a–n. doi:10.1029/2011TC002987
Ernst, W. G. (1993). Metamorphism of Franciscan Tectonostratigraphic Assemblage, Pacheco Pass Area, East-central Diablo Range, California Coast Ranges. Geol. Soc. America Bull. 105, 618–636. doi:10.1130/0016-7606(1993)105<0618:moftap>2.3.co;2
Ernst, W. G., Seki, Y., Onuki, H., and Gilbert, M. C. (1970). Comparative Study of Low-Grade Metamorphism in the California Coast Ranges and the Outer Metamorphic belt of Japan. Geol. Soc. America Memoir 124, 276.
Ernst, W. G. (1975). Systematics of Large-Scale Tectonics and Age Progressions in Alpine and Circum-Pacific Blueschist Belts. Tectonophysics 26, 229–246. doi:10.1016/0040-1951(75)90092-x
Ernst, W. G. (1970). Tectonic Contact between the Franciscan Mélange and the Great Valley Sequence-Crustal Expression of a Late Mesozoic Benioff Zone. J. Geophys. Res. 75, 886–901. doi:10.1029/jb075i005p00886
Evarts, R. C., and Schiffman, P. (1983). Submarine Hydrothermal Metamorphism of the Del Puerto Ophiolite, California. Am. J. Sci. 283, 289–340. doi:10.2475/ajs.283.4.289
Furlong, K. P. (1984). Lithospheric Behavior with Triple junction Migration: An Example Based on the Mendocino Triple junction. Phys. Earth Planet. Interiors 36, 213–223. doi:10.1016/0031-9201(84)90047-5
Godfrey, N. J., Beaudoin, B. C., and Klemperer, S. L. (1997). Ophiolitic Basement to the Great Valley Forearc basin, California, from Seismic and Gravity Data: Implications for Crustal Growth at the North American continental Margin. Geol. Soc. America Bull. 108 (12), 1536–2156. doi:10.1130/0016-7606(1997)109<1536:OBTTGV>2.3.CO;2
Hall, C. A. (1991). Geology of the Point Sur-Lopez Point Region, Coast Ranges, California: A Part of the Southern California Allochthon. Geol. Soc. America Spec. Paper 266, 40.
Hamilton, W. (1969). Mesozoic California and the Underflow of Pacific Mantle. Geol. Soc. America Bull. 80, 2409–2430. doi:10.1130/0016-7606(1969)80[2409:mcatuo]2.0.co;2
Harms, T. A., Jayko, A. S., and Blake, M. C. (1992). Kinematic Evidence for Extensional Unroofing of the Franciscan Complex along the Coast Range Fault, Northern Diablo Range, California. Tectonics 11, 228–241. doi:10.1029/91tc01880
Hatem, A. E., and Dolan, J. F. (2018). A Model for the Initiation, Evolution, and Controls on Seismic Behavior of the Garlock Fault, California. Geochem. Geophys. Geosyst. 19, 2166–2178. doi:10.1029/2017gc007349
Hopson, C. A., Mattinson, J. M., and Pessagno, E. A. (1981). “Coast Range Ophiolite, Western California,” in Geotectonic Development of California. Rubey. (Englewood Cliffs, New Jersey: Prentice-Hall), Volume I 418–510.
Hopson, C. A., Mattinson, J. M., Pessagno, E. A., and Luyendyk, B. P. (2008). California Coast Range Ophiolite: Composite Middle and Late Jurassic Oceanic Lithosphere. Geol. Soc. America Spec. Paper 483, 1–101. doi:10.1130/2008.2438(01)
Jayko, A. S., Blake, M. C., and Harms, T. (1987). Attenuation of the Coast Range Ophiolite by Extensional Faulting, and Nature of the Coast Range "Thrust," California. Tectonics 6, 475–488. doi:10.1029/tc006i004p00475
Kuiper, Y. D., and Wakabayashi, J. (2018). A Comparison between Mid-paleozoic New England, USA, and the Modern Western USA: Subduction of an Oceanic ridge-transform Fault System. Tectonophysics 745, 278–292. doi:10.1016/j.tecto.2018.08.020
Langenheim, V. E., Jachens, R. C., Wentworth, C. M., and McLaughlin, R. J. (2013). Previously Unrecognized Regional Structure of the Coastal Belt of the Franciscan Complex, Northern California, Revealed by Magnetic Data. Geosphere 9 (6), 1514–1529. doi:10.1130/GES00942.1
Liu, M., and Furlong, K. P. (1992). Cenozoic Volcanism in the California Coast Ranges: Numerical Solutions. J. Geophys. Res. 97, 4941–4951. doi:10.1029/92jb00193
Maxwell, J. C. (1974). Anatomy of an Orogen. Geol. Soc. America Bull. 85, 1195–1204. doi:10.1130/0016-7606(1974)85<1195:aoao>2.0.co;2
McLaughlin, R. J., Blake, M. C., Griscom, A., Blome, C. D., and Murchey, B. (1988). Tectonics of Formation, Translation, and Dispersal of the Coast Range Ophiolite of California. Tectonics 7, 1033–1056. doi:10.1029/tc007i005p01033
McLaughlin, R. J., Kling, S. A., Poore, R. Z., McDougall, K., and Beutner, E. C. (1982). Post-middle Miocene Accretion of Franciscan Rocks, Northwestern California. Geol. Soc. America Bull. 93, 595–605. doi:10.1130/0016-7606(1982)93<595:pmaofr>2.0.co;2
McLaughlin, R. J., Sliter, W. V., Sorg, D. H., Russell, P. C., and Sarna-Wojcicki, A. M. (1996). Large-scale Right-Slip Displacement on the East San Francisco Bay Region Fault System, California: Implications for Location of Late Miocene to Pliocene Pacific Plate Boundary. Tectonics 15, 1–18. doi:10.1029/95tc02347
Metzger, E. P., Ernst, W. G., and Sorg, D. (2005). Aluminous Xenoliths in Miocene Andesite, Central California Coast Ranges: Magma-Crust Interaction in a Subduction-Transform Transitional Setting. Int. Geology. Rev. 47, 573–590. doi:10.2747/0020-6814.47.6.573
Mulcahy, S. R., Starnes, J. K., Day, H. W., Coble, M. A., and Vervoort, J. D. (2018). Early Onset of Franciscan Subduction. Tectonics 37, 1194–1209. doi:10.1029/2017TC004753
Mulcahy, S. R., Vervoort, J. D., and Renne, P. R. (2014). Dating Subduction-Zone Metamorphism with Combined Garnet and Lawsonite Lu-Hf Geochronology. J. Meta. Geol. 32, 515–533. doi:10.1111/jmg.12092
Murchey, B. M., and Jones, D. L. (1984). Age and Significance of Chert in the Franciscan Complex of the San Francisco Bay Region. Franciscan Geology of Northern California. Pac. Section Soc. Econ. Paleontologists Mineralogists (Sepm) 43, 23–30.
Namsom, J. S., and Davis, T. L. (1988). Seismically Active Fold and Thrust belt in the San Joaquin Valley, California. Geol. Soc. America Bull. 100, 257–273. doi:10.1130/0016-7606(1988)100<0257:SAFATB>2.3.CO;2
Page, B. M. (1981). “The Southern Coast Ranges,” in The Geotectonic Development of California, Rubey Volume I. Editor W. G. Ernst (Englewood Cliffs, New Jersey: Prentice-Hall), 329–417.
Platt, J. P. (1986). Dynamics of Orogenic Wedges and the Uplift of High-Pressure Metamorphic Rocks. Geol. Soc. America Bull. 97, 1037–1053. doi:10.1130/0016-7606(1986)97<1037:doowat>2.0.co;2
Powell, R. E. (1993). Chapter 1: Balanced Palinspastic Reconstruction of Pre-late Cenozoic Paleogeology, Southern California: Geologic and Kinematic Constraints on Evolution of the San Andreas Fault System. Geol. Soc. America Memoir 178, 1–106. doi:10.1130/mem178-p1
Raymond, L. A., and Bero, D. A. (2015). Sandstone-matrix Mélanges, Architectural Subdivision, and Geologic History of Accretionary Complexes: A Sedimentological and Structural Perspective from the Franciscan Complex of Sonoma and Marin Counties, California, USA. Geosphere 11, 1077–1110. doi:10.1130/GES01137.1
Raymond, L. A. (2015). Designating Tectonostratigraphic Terranesversusmapping Rock Units in Subduction Complexes: Perspectives from the Franciscan Complex of California, USA. Int. Geology. Rev. 57, 801–823. doi:10.1080/00206814.2014.911124
Raymond, L. A. (1973). Franciscan Geology of the Mount Oso Area. CaliforniaDavis: University of California, 180. Ph.D. dissertation.
Raymond, L. A., Ogawa, Y., and Maddock, M. E. (2019). Accretionary Unit Formats in Subduction Complexes: Examples from the Miura-Bosa and Franciscan Complexes. Int. Geology. Rev. 62, 1581–1609. doi:10.1080/00206814.2019.1667881
Raymond, L. A. (2018). What Is Franciscan?: Revisited. Int. Geology. Rev. 60, 1968–2030. doi:10.1080/00206814.2017.1396933
Ring, U., and Brandon, M. T. (1994). Kinematic Data for the Coast Range Fault and Implications for Exhumation of the Franciscan Subduction Complex. Geol 22, 735–738. doi:10.1130/0091-7613(1994)022<0735:kdftcr>2.3.co;2
Ring, U. (2008). Deformation and Exhumation at Convergent Margins: The Franciscan Subduction Complex. Boulder, CO: Geological Society of America Special Paper 445, 61.
Ross, J. A., and Sharp, W. D. (1988) The Effects of Sub-Blocking Temperature Metamorphism on the K/Ar Systematics of Hornblendes: 40Ar/39Ar Dating of Polymetamorphic Garnet Amphibolite From the Franciscan Complex, California, Contributions to Mineralogy and Petrology. 100, 213–221
Rutte, D., Garber, J., Kylander-Clark, A., and Renne, P. R. (2020) An Exhumation Pulse From the Nascent Franciscan Subduction Zone (California, USA), Tectonics. 39 (10), e2020TC006305. doi:10.1029/2020TC006305
Schmidt, W. L., and Platt, J. P. (2018) Subduction, Accretion, and Exhumation of Coherent Franciscan Blueschist-Facies Rocks, Northern Coast Ranges, California, Lithosphere. 10, 301–326. doi:10.1130/l697.1
Sliter, R. V., and McGann, M. L. (1992). Age and Correlation of the Calera Limestone in the Permanente Terrane of Northern California: U.S. Washington, D.C: United States Government Printing Office, 27p.
Sliter, W. V. (1984). “Foraminifers from Cretaceous limestone of the Franciscan Complex, Northern California, Pac. Section Soc. Econ. Paleontologists Mineral. 43, 5–22.
Snow, C. A., Wakabayashi, J., Ernst, W. G., and Wooden, J. L. (2010). Detrital Zircon Evidence for Progressive Underthrusting in Franciscan Metagraywackes, West-central California. Geol. Soc. America Bull. 122, 282–291. doi:10.1130/b26399.1
Stimac, J. A. (1993). The Origin and Significance of High-Grade Metamorphic Xenoliths, Clear Lake Volcanics, California. Soc. Econ. Paleontologists Mineralogists Guidebook 16, 171–189.
Suppe, J., and Foland, K. A. (1978). “The Goat Mountain Schists and Pacific Ridge Complex: A Redeformed but Still-Intact Late Mesozoic Franciscan Schuppen Complex,” in Mesozoic Paleogeography of the Western United States. Pacific Coast Paleogeography Symposium 2. Editors D. G. Howell, and K. A. McDougall (Los Angeles, California: Society of Economic Paleontologists and Mineralogists, Pacific Section), 431–451.
Suppe, J. (1973). Geology of the Leech Lake Mountain-Ball Mountain Region, California. University of California Publications Geological Sciences, 107, 1–81.
Surpless, K. D. (2015). Hornbrook Formation, Oregon and California: A Sedimentary Record of the Late Cretaceous Sierran Magmatic Flare-Up Event. Geosphere 11, 1770–1789. doi:10.1130/GES01186.1
Tarduno, J. A., McWillams, M., and Sleep, N. (1990). Fast Instantaneous Oceanic Plate Velocities Recorded by the Cretaceous Laytonville limestone: Paleomagnetic Analysis and Kinematic Implications. J. Geophys. Res. 95, 15503–15527. doi:10.1029/jb095ib10p15503
Tarduno, J. A., McWilliams, M., Debiche, M. G., Sliter, W. V., and Blake, M. C. (1985). Franciscan Complex Calera Limestones: Accreted Remnants of Farallon Plate Oceanic Plateaus. Nature 317, 345–347. doi:10.1038/317345a0
Terabayashi, M., and Maruyama, S. (1998). Large Pressure Gap Between the Coastal and Central Belts, Northern and Central California. Tectonophysics 285, 87–101.
Unruh, J. R., Dumitru, T. A., and Sawyer, T. L. (2007). Coupling of Early Tertiary Extension in the Great Valley Forearc basin with Blueschist Exhumation in the Underlying Franciscan Accretionary Wedge at Mount Diablo, California. Geol. Soc. America Bull. 119, 1347–1367. doi:10.1130/b26057.1
Unruh, J. R., and Moores, E. M. (1992). Quaternary Blind Thrusting in the Southwestern Sacramento Valley, California. Tectonics 11, 192–203. doi:10.1029/91tc02494
Unruh, J. R., Ramirez, V. R., Phipps, S. P., and Moores, E. M. (1991). Tectonic Wedging beneath Forearc Basins: Ancient and Modern Examples from California and the Lesser Antilles. GSA Today 1, 185–190.
von Huene, R., and Scholl, D. W. (1991). Observations at Convergent Margins Concerning Sediment Subduction, Subduction Erosion, and the Growth of continental Crust. Rev. Geophys. 29, 279–316. doi:10.1029/91rg00969
von Huene, R. (1986). To Accrete or Not Accrete, that Is the Question. Geol. Rundsch 75, 1–15. doi:10.1007/bf01770175
Wahrhaftig, C. W. (1984). Structure of the Marin Headlands Block, California: A Progress Report. Pac. Section Soc. Econ. Paleontologists Mineralogists (Sepm) 43, 31–50.
Wakabayashi, J. (2015). Anatomy of a Subduction Complex: Architecture of the Franciscan Complex, California, at Multiple Length and Time Scales. Int. Geology. Rev. 57, 669–746. doi:10.1080/00206814.2014.998728
Wakabayashi, J. (1999). Distribution of Displacement on and Evolution of a Young Transform Fault System: The Northern San Andreas Fault System, California. Tectonics 18, 1245–1274. doi:10.1029/1999tc900049
Wakabayashi, J., and Dumitru, T. A. (2007). 40Ar/39Ar Ages from Coherent, High-Pressure Metamorphic Rocks of the Franciscan Complex, California: Revisiting the Timing of Metamorphism of the World's Type Subduction Complex. Int. Geology. Rev. 49, 873–906. doi:10.2747/0020-6814.49.10.873
Wakabayashi, J. (2021b). Field and Petrographic Reconnaissance of Franciscan Complex Rocks of Mount Diablo, California: Imbricated Ocean Floor Stratigraphy with a Roof Exhumation Fault System. Geol. Soc. America Memoir 217, 155–178. doi:10.1130/2021.1217(09)
Wakabayashi, J., Ghatak, A., and Basu, A. R. (2010). Suprasubduction-zone Ophiolite Generation, Emplacement, and Initiation of Subduction: A Perspective from Geochemistry, Metamorphism, Geochronology, and Regional Geology. Geol. Soc. America Bull. 122, 1548–1568. doi:10.1130/b30017.1
Wakabayashi, J., Hengesh, J. V., and Sawyer, T. L. (2004). Four-dimensional Transform Fault Processes: Progressive Evolution of Step-Overs and Bends. Tectonophysics 392, 279–301. doi:10.1016/j.tecto.2004.04.013
Wakabayashi, J. (2007). Stepovers that Migrate with Respect to Affected Deposits: Field Characteristics and Speculation on Some Details of Their Evolution. Geol. Soc. Lond. Spec. Publications 290, 169–188. doi:10.1144/sp290.4
Wakabayashi, J. (2021a). “Subduction and Exhumation Slip Accommodation at Depths of 10-80 Km Inferred from Field Geology of Exhumed Rocks: Evidence for Temporal-Spatial Localization of Slip, Geol. Soc. America Spec. Paper 552, 257–296. doi:10.1130/2021.2552(12)
Wakabayashi, J. (1996). Tectono-metamorphic Impact of a Subduction-Transform Transition and Implications for Interpretation of Orogenic Belts. Int. Geology. Rev. 38, 979–994. doi:10.1080/00206819709465376
Wakabayashi, J., and Unruh, J. R. (1995). Tectonic Wedging, Blueschist Metamorphism, and Exposure of Blueschists: Are They Compatible. Geol 23, 85–88. doi:10.1130/0091-7613(1995)023<0085:twbmae>2.3.co;2
Wentworth, C. M., Blake, M. C., Jones, D. L., Walter, A. W., and Zoback, M. D. (1984). Tectonic Wedging Associated with Emplacement of the Franciscan Assemblage, California Coast Ranges. Soc. Econ. Paleontologists Mineralogists 43, 163–173.
Keywords: subduction accretion, subduction erosion, along-strike variation, subduction tectonics, post-subduction deformation restoration
Citation: Wakabayashi J (2022) Along-Strike Variation in Accretion, Non-accretion, and Subduction Erosion Recorded in Rocks of the Jurassic-Neogene Convergent Plate Margin of California. Front. Earth Sci. 10:818171. doi: 10.3389/feart.2022.818171
Received: 19 November 2021; Accepted: 24 January 2022;
Published: 15 February 2022.
Edited by:
Andrea Festa, University of Turin, ItalyReviewed by:
Andrea Zanchi, University of Milano-Bicocca, ItalyCopyright © 2022 Wakabayashi. This is an open-access article distributed under the terms of the Creative Commons Attribution License (CC BY). The use, distribution or reproduction in other forums is permitted, provided the original author(s) and the copyright owner(s) are credited and that the original publication in this journal is cited, in accordance with accepted academic practice. No use, distribution or reproduction is permitted which does not comply with these terms.
*Correspondence: John Wakabayashi, andha2FiYXlhc2hpQGNzdWZyZXNuby5lZHU=
Disclaimer: All claims expressed in this article are solely those of the authors and do not necessarily represent those of their affiliated organizations, or those of the publisher, the editors and the reviewers. Any product that may be evaluated in this article or claim that may be made by its manufacturer is not guaranteed or endorsed by the publisher.
Research integrity at Frontiers
Learn more about the work of our research integrity team to safeguard the quality of each article we publish.