- 1Consiglio Nazionale Delle Ricerche, Istituto di Geoscienze e Georisorse, Firenze, Italy
- 2Dipartimento di Scienze Della Terra, Università di Firenze, Firenze, Italy
In the Main Ethiopian Rift (East Africa) a complex tectonic history preceded Tertiary rifting creating pre-existing discontinuities that influenced extension-related deformation. Therefore, this area offers the opportunity to analyze the control exerted by pre-existing structures on continental rifting at different scales. In this paper we present an overview of such an influence. We show that at a large scale (up to ∼800–1,000 km) rift localization has been controlled by a lithospheric-scale inherited heterogeneity corresponding to a Precambrian suture zone, separating two different lithospheric domains beneath the plateaus surrounding the rift. The inherited rheological differences between these two lithospheric domains, as well as the presence of pre-existing lithospheric-scale transversal structures, largely controlled the along-axis segmentation and symmetry/asymmetry of different, ∼80–100 km-long rift segments. Inherited transversal structures also controlled the development of off-axis volcano tectonic activity in the plateaus surrounding the rift. At a more local scale (<80 km), inherited fabrics controlled the geometry of normal faults and the distribution and characteristics of rift-related volcanism. These observations document a strong control exerted by pre-existing structures on continental rifting at all different scales.
Introduction
Continental rift systems normally develop within a previously deformed lithosphere in which the distribution, architecture and evolution of deformation may be strongly influenced by pre-existing structures. Indeed, inherited mechanical heterogeneities (from the lithospheric scale, e.g., suture zones, to the upper crustal scale, e.g., foliations, shear zones, folds, faults, dykes) either weaker or stronger than the surrounding material, are able to significantly influence the pattern, propagation and overall evolution of continental rifts (e.g., Rosendahl, 1987; Dunbar and Sawyer, 1989; Smith and Mosley, 1993; Vauchez et al., 1998; Morley, 1999; Corti, 2012; Buiter and Torsvik, 2014; Purcell, 2017; Will and Frimmel, 2017).
Many extensional settings suggest such a control, including the North Sea (Bell et al., 2014; Phillips et al., 2019), the Rhine graben system (e.g., Schumacher, 2002; Michon and Sokoutis, 2005; Edel et al., 2007), the Tertiary rifts of Thailand (Morley et al., 2004; Pongwapee et al., 2019) and, finally, the East African Rift System (Chorowicz, 2005; Vétel and Le Gall, 2006; Corti et al., 2007; Brune et al., 2017). Furthermore, several works show that many rifts developed in different, successive extensional phases (Whipp et al., 2014; Deng et al., 2017; Phillips et al., 2019; Wu et al., 2020), with normal faults formed during initial extensional events exerting a strong control on the structural architecture developed during the later rifting phases (Bell et al., 2014; Duffy et al., 2015; Henstra et al., 2015, 2019; Deng et al., 2018; Wang et al., 2021). Such a control exerted by early normal faults on structures formed during later extension is also supported by crustal and lithospheric scale analogue models (e.g., Corti et al., 2007; Sokoutis et al., 2007; Autin et al., 2013; Molnar et al., 2017, 2019, 2020; Zwaan and Schreurs, 2017; Maestrelli et al., 2020; Wang et al., 2021; Zwaan et al., 2021a).
The Main Ethiopian Rift (MER), in the East African Rift System (EARS), offers the possibility to analyse the control at different scales of pre-existing structures on rifting. Ethiopia has been indeed affected by several tectonic events that preceded Cenozoic rifting, from complex Precambrian phases of collision to extension during the Mesozoic (e.g., Korme et al., 2004; Chorowicz et al., 1994; Abbate et al., 2015 and references therein). This long tectonic history created pre-existing structures that controlled the development of the Ethiopian sector of the EARS from a regional to a local scale; the control of these mechanical heterogeneities (which are defined at different scales in the following sections) is the focus of this review paper.
Tectonic Setting
The MER is the northernmost portion of the EARS, extending from the Afar region in the north, to the Turkana depression in the south (Figure 1). This region of rifting results from active extension between the Nubia and Somalia Plates (e.g., Ebinger, 2005; Corti, 2009), with geodetic measurements indicating that extension is currently occurring in a roughly E-W direction at rates of ∼4–6 mm/yr (e.g., Bendick et al., 2006; Saria et al., 2014; Birhanu et al., 2016). Extension in the northern EARS, Gulf of Aden and the Red Sea occurred contemporaneously with, and after, an intense phase of flood basalt volcanism at ∼32–30 Ma (Wolfenden et al., 2004), which possibly resulted from upwelling of the African Superplume (e.g., Ritsema et al., 1999), although more complex scenarios have been recently proposed (e.g., Chang et al., 2020).
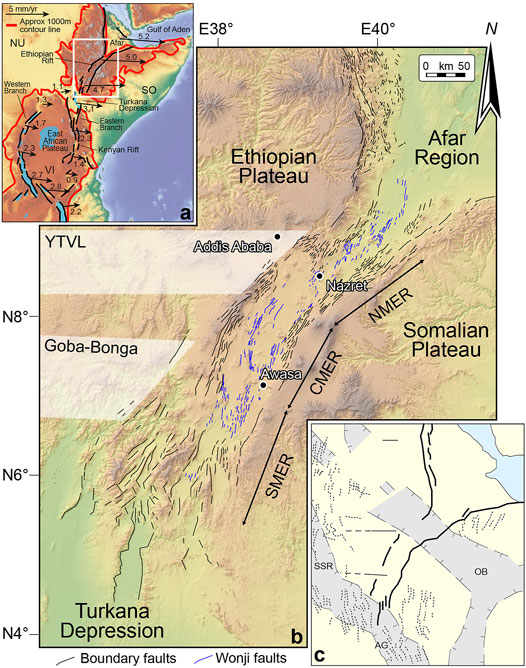
FIGURE 1. Tectonic setting of the Main Ethiopian Rift. (A) Present-day plate kinematics of the East African Rift (modified from Corti et al., 2019). Black arrows show relative motions with respect to a stable Nubian reference frame; values besides arrows indicate motion in mm/yr. NU: Nubian plate; SO: Somalian plate; VI: Victoria microplate. (B) Main rift-related faults differentiated in Boundary and Wonji (axial) faults; indicated is the subdivision in the different segments: Northern MER (NMER), Central MER (CMER), Southern MER (SMER). Whitish pattern indicates the transversal lineaments of Yerer-Tullu Wellel (YTVL) and Goba-Bonga. (C) Inherited structures in Ethiopia (modified from Korme et al., 2004; Gani et al., 2009). Dashed lines indicate NE-SW- to N-S-trending basement foliations; solid lines illustrate E-W structures, corresponding to the main transversal lineaments affecting the rift; greyish pattern represents NW-SE-trending structures corresponding to sedimentary Mesozoic basins (AG: Anza graben; OB: Ogaden basin; SSR: South Sudan rifts).
The MER is subdivided into three main sectors: Northern MER (NMER), Central MER (CMER) and Southern MER (SMER) (Figure 1). These sectors differ in terms of age and pattern of deformation, volcanic activity and lithospheric characteristics (e.g., Mohr, 1983; WoldeGabriel et al., 1990; Hayward and Ebinger, 1996; Bonini et al., 2005; Corti, 2009).
In the NMER, significant tectonic activity is localised at the rift axis along a belt of Pleistocene-Holocene volcano-tectonic structures, the so-called Wonji Fault Belt (WFB, e.g., Mohr, 1962; Boccaletti et al., 1998; Ebinger and Casey, 2001). The WFB is characterized by swarms of short, closely-spaced faults with minor vertical displacement, with associated focused volcanic activity and magma intrusion (e.g., Boccaletti et al., 1998; Tommasi and Vauchez, 2001). Large-offset boundary faults defining prominent marginal escarpments in this rift sector are interpreted to have accommodated deformation in the Mio-Pliocene, but they have been deactivated during the Pleistocene (e.g., Wolfenden et al., 2004; Casey et al., 2006; Keir et al., 2006). Voluminous magma intrusion beneath the axial WFB since 2 Ma caused important modifications of the composition, thermal structure and rheology of the crust/lithosphere in this rift sector (e.g., Beutel et al., 2010; Daniels et al., 2014). As a result, a combination of magma intrusion and normal faulting accommodates extension in this rift sector (e.g., Keranen et al., 2004; Dugda et al., 2005; Mackenzie et al., 2005; Keir et al., 2006; Bastow et al., 2010).
In the CMER and SMER, the large boundary faults are well developed and less eroded than in the Northern MER, and geological, geodetical and seismicity data indicate that they still accommodate significant extension (Gouin, 1979; Keir et al., 2006; Pizzi et al., 2006; Agostini et al., 2011; Kogan et al., 2012; Molin and Corti, 2015; Corti et al., 2020). This occurs together with a decrease in the amount of extension taken up by axial Wonji faults, which are considered to be in an incipient stage of development in the CMER, but almost absent in the SMER (e.g., Ebinger et al., 2000; Agostini et al., 2011). Similarly, the volume of Quaternary volcanism decreases southwards and the volcano-tectonic activity within the rift is sparse in the SMER and limited to the rift margins.
All these variations of the distribution and characteristics of the tectonic and magmatic activity along the rift axis have been interpreted to reflect a transition from initial rifting in the SMER, with marginal deformation and rift morphology dominated by faulting, to more advanced rifting stages in the NMER, where prominent axial intrusion, dyking and related normal faulting testify a phase of magma-assisted rifting that precedes continental break-up (e.g., Kendall et al., 2005).
The several tectonic events that affected Ethiopia since the Precambrian created different sets of inherited structures that exerted an important control on Cenozoic rifting and can be classified into three main groups (Figure 1C; e.g., Korme et al., 2004): 1) Rift-parallel or subparallel structures (NE-SW- to N-S-trending) are mainly related to deformation connected to the closure of the Mozambique Ocean during the Proterozoic and to a suture zone which formed in relation to this event. 2) Roughly E-W structures correspond to Neoproterozoic weaknesses (e.g., faults, fractures) sub-parallel to the trend of the Gulf of Aden. 3) NW-SE-trending structures correspond to sedimentary basins (e.g., Ogaden basin, Anza graben, South Sudan rifts) and associated normal faults formed during Mesozoic extension, which in turn likely reactivated pre-existing Precambrian major crustal weakness zones.
Control of Pre-existing Lithospheric Weaknesses on Rift Localisation
Along with other processes (e.g., magma intrusion), the presence of large-scale zones of weakness such as ancient suture zones (e.g., Buiter and Torsvik, 2014), whose rheology is different from the surrounding regions, facilitates deformation of a continental lithosphere which would be otherwise too strong to be deformed by the available tectonic forces (e.g., Buck, 2004). This is why rift structures typically localise within pre-existing zones of weakness at a lithospheric scale, which tend to strongly favour extensional deformation (e.g., Dunbar and Sawyer, 1989; Buiter and Torsvik, 2014).
Previous studies in the MER based on geophysical data suggest that initial rift location has been controlled by a lithospheric-scale, up to ∼800-1000 km-long, pre-existing Precambrian suture (Figure 2; e.g., Gashawbeza et al., 2004; Bastow et al., 2005; Daly et al., 2008; Keranen and Klemperer, 2008; Keranen et al., 2009; Cornwell et al., 2010; Purcell, 2017), resulting from the closure of the Mozambique Ocean and accretion of East to West Gondwana. Ophiolites composing this suture zone have been dated between 880 and 690 Ma (Kroner et al., 1992; Claesson et al., 1984; Pallister et al., 1988). The existence of such a suture zone, which puts in contact 870 Ma Neo-Proterozoic juvenile crust with 2 Ga Archean basement (Kazmin et al., 1978; Vail, 1983; Berhe, 1990; Stern et al., 1990; Stern, 1994, 2002; Abdelsalam and Stern, 1996), is supported by differences in crustal/mantle properties between the Ethiopian and Somalian plateaus surrounding the rift. Several geophysical data (crustal thickness and bulk crustal Vp/Vs ratios, resistivity from magnetotelluric data, arrival-time body-wave tomographic models and effective elastic plate thickness) indicate indeed a strong and homogenous Somalian plateau, which contrasts with the more heterogeneous Ethiopian plateau composed of a strong and thick northern portion and a thinner and weaker southern portion (Figure 2; e.g., Corti et al., 2018a and references therein). The reactivation of this weakness zone is interpreted to have occurred at the eastern margin of the upwelling mantle plume, not above its center (e.g., Bastow et al., 2008), as documented in other flood basalt provinces (e.g., Deccan, Greenland, Parana, Central Atlantic; Courtillot et al., 1999). Magmatic activity and melt upraising through the upper mantle and crust may have promoted thermal and mechanical weakening of the continental lithosphere, possibly contributing its extension-related deformation (e.g., Díaz-Alvarado et al., 2021). The curved plan-view geometry of this inherited weakness significantly influenced the trend of the Cenozoic faulting (e.g., Mohr, 1962; Kazmin et al., 1980), with a variation from roughly N-S-trending in the south to roughly NE-SW-trending in the North. This resulted in an along-axis variation of the kinematics of rifting, from orthogonal in the southern MER to moderately oblique in the northern MER (Keir et al., 2015; Erbello et al., 2016), which had a major control on the segmentation and structural architecture of the rift (Corti, 2008). Notably, the reactivation of an inherited weak zone oblique to the plate motion vector is able to impose a local reorientation of the extension direction at the rift margins, as illustrated in below Section 6.
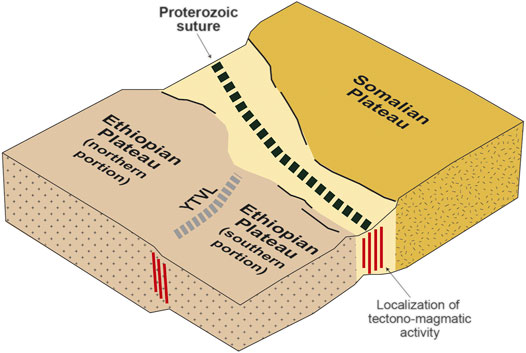
FIGURE 2. Pre-rift lithospheric domains and location of Cenozoic volcano-tectonic activity in the MER (modified from Keranen and Klemperer, 2008). Cenozoic extension follows the rheological boundaries between two distinct Proterozoic basement terranes, underlying the Ethiopian and Somalian plateaus; this lithospheric weakness zone corresponds to an ancient suture zone (see text for details).
Control of Large-Scale Transversal Structures on Off-Axis Deformation, Rift Interaction and Linkage
Transversal Lineaments and Off-Axis Deformation
The MER is characterised by the occurrence of major transversal lineaments, which affect the rift floor and the plateaus surrounding the rift valley (Figure 1; e.g., Abebe Adhana, 2014 and references therein). Specifically, the main structures correspond to the roughly E-W to WNW-ESE-trending Yerer-Tullu Wellel and Goba Bonga volcano-tectonic lineaments (Figure 1, Figure 3), causing deformation and volcanic activity to extend for hundreds of kilometres into the southern portion of the Ethiopian plateau and marking the transition between the different MER sectors. At a regional scale, these transversal structures have been interpreted to reflect the reactivation of inherited Neoproterozoic weaknesses roughly parallel to the trend of the Gulf of Aden (e.g., Abbate and Sagri, 1980; Abebe et al., 1998; Korme et al., 2004; Abebe Adhana, 2014; Corti et al., 2018b). As explained below, geophysical data support indeed the influence of inheritance on the development of these structures. The Yerer-Tullu Wellel lineament corresponds to a significant gradient in the thickness of the crust and marks the boundary between the northern and southern portions of the Ethiopian Plateau (Figure 2; Keranen and Klemperer, 2008). The P wave velocity model of Bastow et al. (2008) in its 75 km depth slice shows discrete low velocity anomalies that extend from the rift valley into the Ethiopian Plateau, beneath the Yerer-Tullu Wellel and Goba Bonga lineaments (see their Figure 7). A combination of a thermal anomaly and partial melting in response to asthenospheric upwelling and decompression, in turn related to localized lithospheric extension and thinning, best explains these low velocity anomalies beneath the two transversal lineaments (e.g., Bastow et al., 2010; Gallacher et al., 2016). The Goba Bonga lineament is also characterised by lower values of the elastic thickness of the lithosphere (Te; Pérez-Gussinyé et al., 2009), which shows a decrease from values of up to 40 km beneath the Ethiopian plateau to values of 10–15 km (Pérez-Gussinyé et al., 2009) in a narrow E-W domain corresponding to the transversal structure (see Figure 3). These zones of thinned crust or lithosphere beneath the Yerer-Tullu Wellel and Goba Bonga lineaments were possibly caused by either inherited lithospheric thinned regions or by syn-rift extension exploiting pre-rift weakness zones. Overall, this indicates a pre-rift, structural control on the current structure of the lithosphere (Corti et al., 2018a).
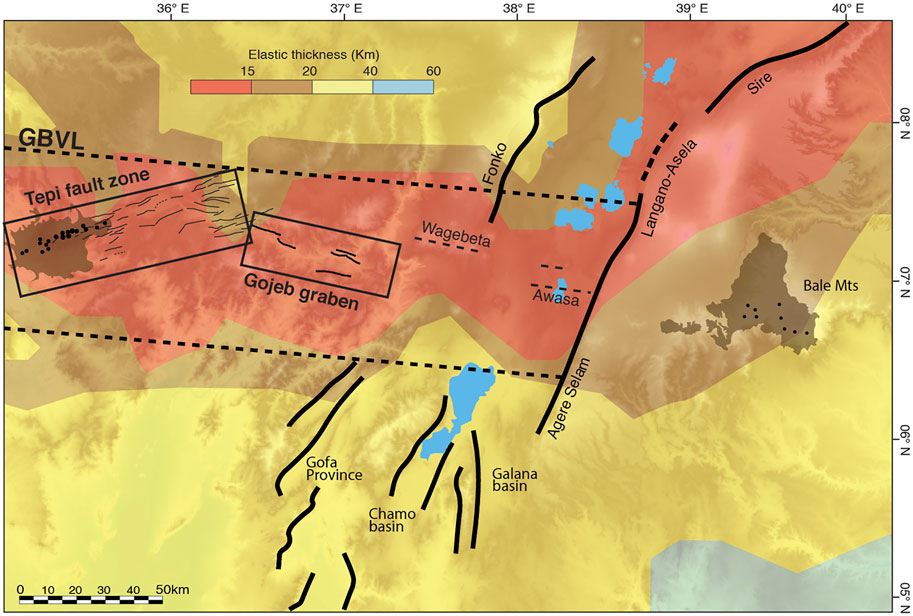
FIGURE 3. Structural scheme of the Goba Bonga lineament (from Corti et al., 2018b) superimposed on a map of the elastic thickness of the lithosphere (Te), modified after Pérez-Gussinyé et al. (2009). Low Te values (<10–15 km) are observed in a narrow region extending in a roughly E-W direction in the region corresponding to the extent of the Goba Bonga lineament.
Rift Interaction and Linkage
At its southern termination, the MER interacts with the Kenya Rift within the Turkana depression, a low-land where deformation, seismic activity and Pleistocene-Holocene volcanism are distributed over a width of more than 450 km (Figure 4; e.g., Ebinger et al., 2000). Within this anomalously wide region of ongoing tectonic and magmatic activity, extension is accommodated by numerous small normal faults with limited vertical displacement. This is in striking contrast with the narrow rift valleys to the north and south, characterized by a typical rift valley morphology dominated by large fault escarpments and boundary faults with large vertical displacement. Previous studies have suggested an influence of the Mesozoic-Early Paleogene tectonic phase on the later extensional deformation related to the EARS (e.g., Brune et al., 2017; Corti et al., 2019; Emishaw and Abdelsalam, 2019): the location of the diffuse Cenozoic tectono-magmatic activity has been likely controlled by the presence of a wide region of thinned crust, trending NW-SE and resulting from Mesozoic extension (Anza graben, South Sudan rifts; Figure 4). More specifically, the anomalously wide rift zone is likely caused by the N-S direction of propagation of the Main Ethiopian and Kenya rift systems into this NW-SE-trending region of thinned crust and stronger mantle lithosphere (e.g., Brune et al., 2017). In this region, the Kenyan and Ethiopian rift valleys are left-laterally deflected away from one another, avoiding a direct linkage to form a thoroughgoing N-S depression (Brune et al., 2017). Therefore, the presence of pre-existing transversal structures has a strong influence on the interaction and linkage of major rift segments.
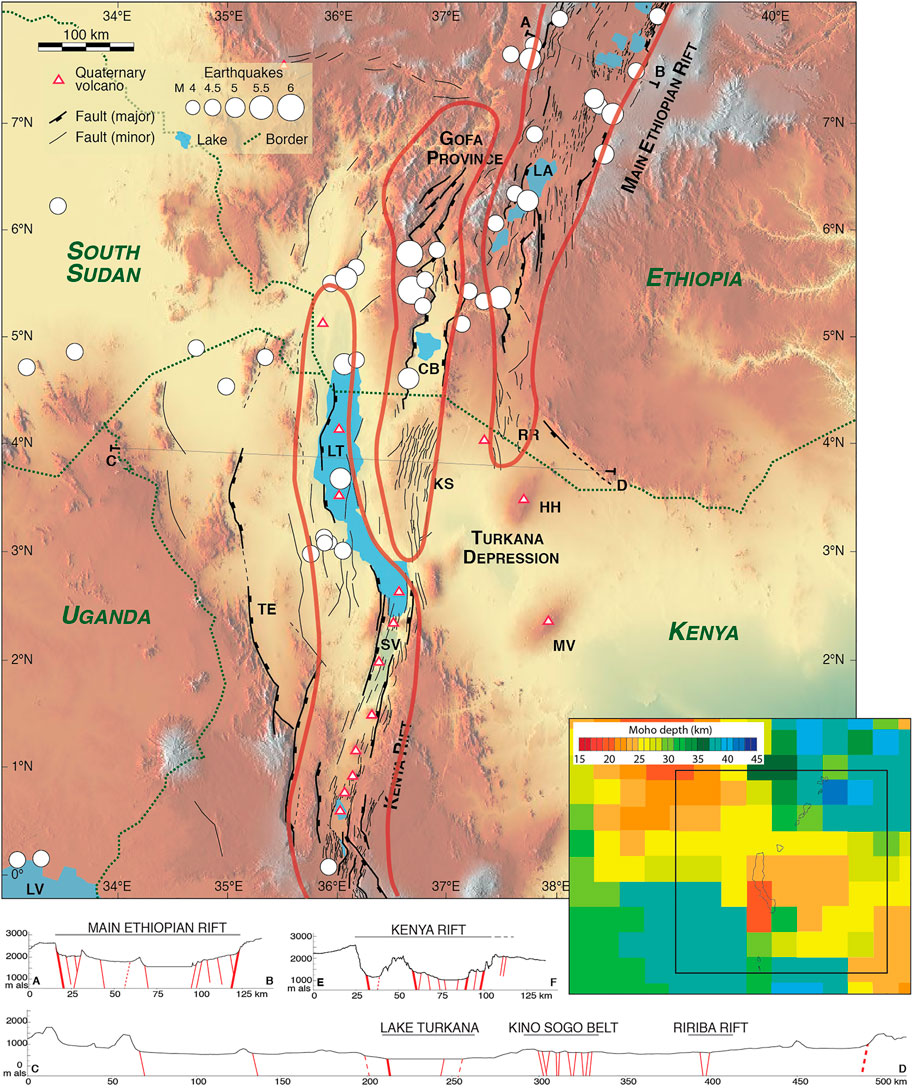
FIGURE 4. Tectonic setting of the Turkana depression and surrounding regions, with Quaternary faults, seismicity and Quaternary volcanoes superimposed on a SRTM (Nasa Shuttle Radar Topography Mission, 30 m resolution) digital elevation model (modified from Brune et al., 2017). CB: Chew Bahir basin; HH: Hurri Hills; KS: Kino Sogo belt; LA: Lake Abaya; LT: Lake Turkana; LV: Lake Victoria; MV: Marsabit volcano; RR: Ririba Rift; SV: Suguta Valley; TE: Turkwell Escarpment. Areas encircled by red lines are the main deformation domains. Inset in the bottom right shows the crustal thickness in the region (from Benoit et al., 2006). Also illustrated are simplified cross sections highlighting the different architecture and distribution of deformation in the Turkana depression with respect to the Kenyan and Ethiopian rifts.
Control of Pre-existing Structures on Rift Segmentation and Symmetry
Recent studies in the MER (e.g., Corti et al., 2018a) have shown that the pre-rift structure of the Ethiopian and Somalian plateaus at two sides of the reactivated ancient suture zone has a major control on rift architecture and segmentation.
The eastern margin of the MER, where the lithosphere beneath the Somalian Plateau is strong and homogeneous (e.g., Keranen and Klemperer, 2008; Corti et al., 2018a), is characterised by the presence of a more or less continuous system of large boundary faults (Figures 1, 5). Conversely, the western margin shows significant along-axis variations, with segments marked by large boundary faults (Ankober, Fonko-Guraghe, Gamo-Gidole) alternating with sectors characterised by flexures, with gentle monoclines dipping towards the rift axis (Figures 1, 5). This has been interpreted to reflect a more heterogeneous and complex structure of the Ethiopian plateau lithosphere than the Somalian, with the Ethiopian plateau characterised by a strong northern portion and a southern portion with a thinner and weaker crust (Figure 5; e.g., Keranen and Klemperer, 2008). This southern portion is marked by the presence of the E-W Yerer-Tullu Wellel and Goba Bonga volcano-tectonic lineaments (Figure 5). Where these pre-existing weaknesses intersect the rift at a high angle, major boundary faults are absent from the western margin and are instead replaced by gentle flexures; this, together with well-developed faults on the eastern side, gives rise to an overall asymmetry of the rift (Figure 5). Instead, where large boundary faults characterise the western margin, the rift is symmetric (Figure 5). Notably, southeast of Addis Ababa, the eastern rift margin is characterised by a prominent shift to the East, which occurs in spatial coincidence with the Yerer-Tullu Wellel volcano-tectonic lineament (Figures 1, 5). This coincidence has been interpreted to reflect an influence exerted by the E-W-trending pre-existing weakness on the plan-view geometry of the rift valley at this latitude (e.g., Korme et al., 2004).
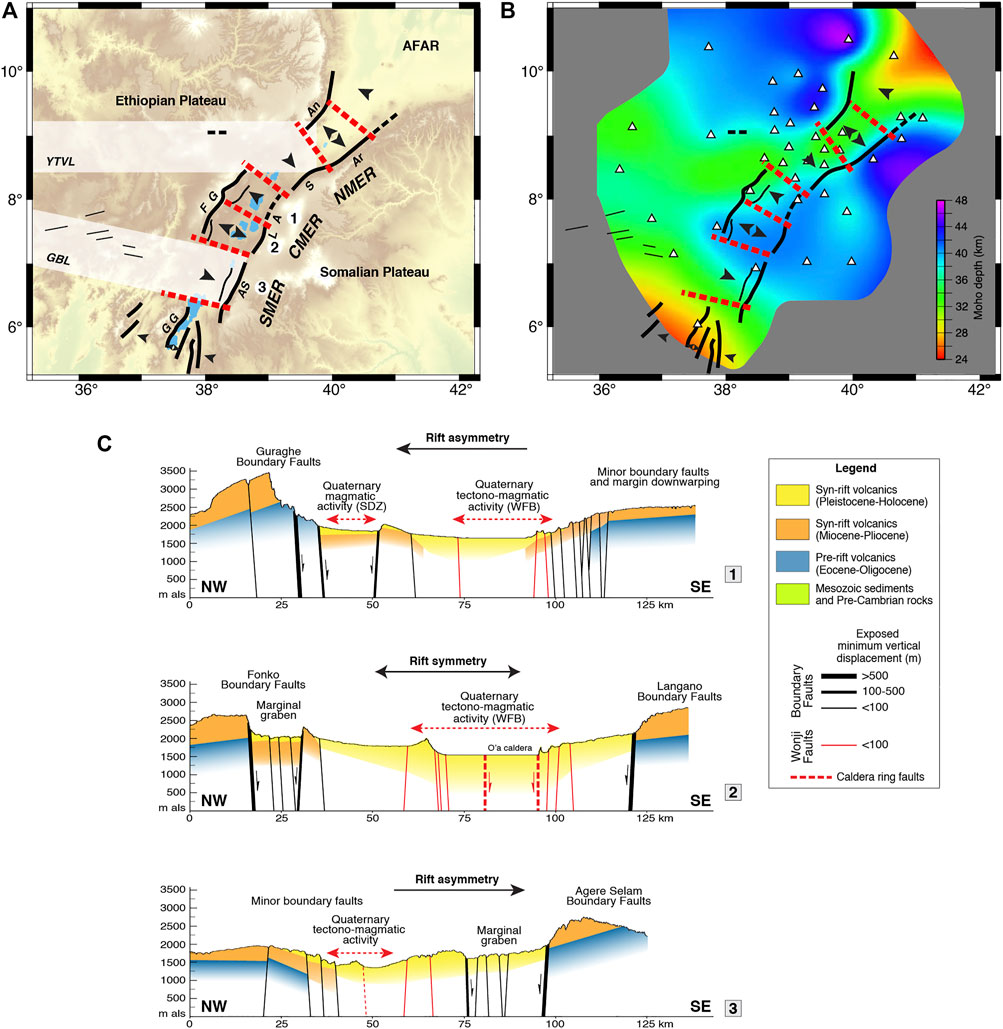
FIGURE 5. Along-axis variations in the structure of the Main Ethiopian Rift (modified from Corti et al., 2018a). (A) Interpreted variations in rift symmetry and polarity: single arrows indicate the polarity of the different rift sectors; double arrows indicate symmetry of rifting. Whitish boxes indicate the hypothesised plan-view extent of the transversal structures: Yerer-Tullu Wellel (YTVL) and Goba-Bonga (GBL) volcano-tectonic lineaments. Rift segments labelled as NMER: Northern MER; CMER: Central MER, SMER: Southern MER. Other labels indicate the main rift escarpments as follows, An: Ankober; Ar: Arboye; AS: Agere Selam; FG: Fonko-Guraghe; GG: Gamo Gidole; LA: Langano Asela; S: Sire. (B) Map of depth of the Moho in the MER, modified after Keranen et al. (2009). (C) Simplified geological profiles across different sectors of the MER, with vertical exaggeration of x10. WFB: Wonji Fault Belt; SDZ: Silti Debre Zeit volcanic belt.
In summary, the above observations indicate that the MER is characterized by a segmentation strongly controlled by the inherited lithospheric structure, which results in 80–100 km-long rift segments with alternating symmetric/asymmetric basins.
Control of Pre-existing Structures on Local-Scale Fault Geometry and Architecture
Pre-existing structures have a significant influence on the geometry and segmentation of extension-related normal faults at a more local (<80 km) and shallower scale. The architecture and kinematics of boundary faults at the margins of the CMER and NMER are strongly influenced by the oblique inherited weakness described in Section 3. The boundary faults are at surface en-echelon arranged and oblique to both the pre-existing weakness (i.e., the rift trend) and the orthogonal to the regional plate motion vector; they trend orthogonal to the local direction of extension (Figure 6; Corti et al., 2013; Philippon et al., 2015). Inversion of fault-slip data and detailed analysis of fault kinematics in analogue models of oblique rifting indicate indeed a pure dip-slip motion on these faults, in which (given the oblique orientation with respect to the extension direction) a strike-slip component of motion could be expected (Corti et al., 2013; Philippon et al., 2015). This documents a reorientation in the extension direction at the margins of the rift, where the local extension direction does not correspond to the regional plate divergence, resulting in a pure dip-slip motion in an overall oblique kinematics (e.g., Morley, 2010; Corti et al., 2013; Figure 6).
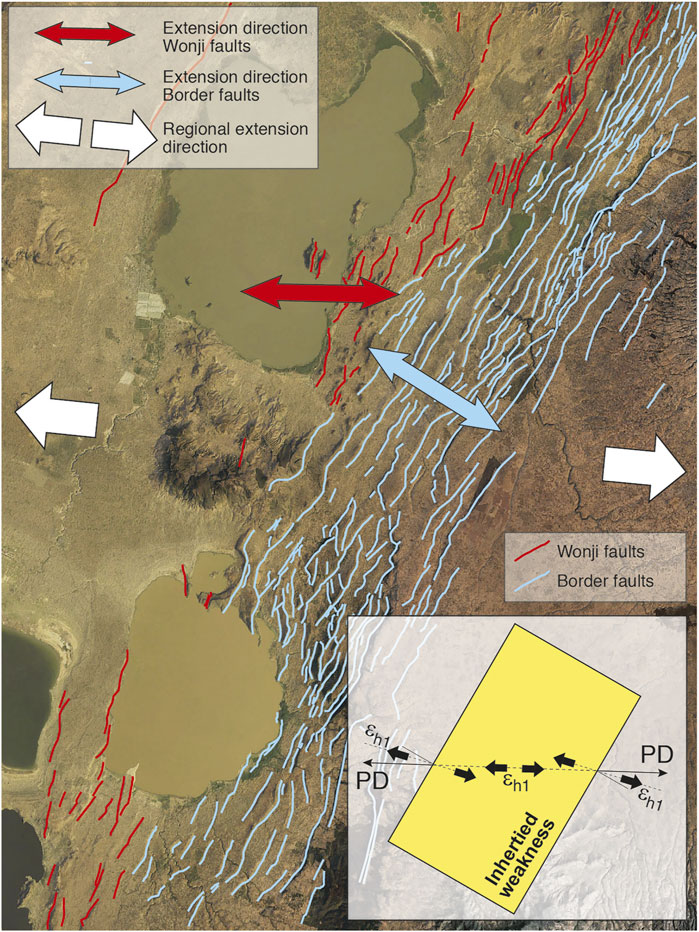
FIGURE 6. Reorientation of the extension direction at the Asela-Langano margin. The local direction of extension at boundary and internal faults is indicated with light blue and red arrows, respectively; the regional direction of extension is illustrated with the big white arrows. Inset in the bottom right shows the relationship between the rift trend (or its perpendicular), the regional direction of extension (or plate divergence, PD) and the trend of the greatest horizontal principal strain (εh1) in the different portions of the oblique rift (modified from Corti et al., 2020).
Examples of an important control of inherited structures like fractures, faults, foliations, and dikes on the geometry of individual faults or fault segments are evident in the Gofa Basin and Range and in the Chew Bahir basin, in southern Ethiopia (Figures 7, 8). In these regions, large boundary faults are typically highly segmented, with many short interacting segments characterised by sharp changes in orientation giving rise to zig-zag geometries and angular patterns (e.g., Moore and Davidson, 1978; Vétel et al., 2005; Vétel and Le Gall, 2006). Typically, the orientation of the boundary fault segments mimics the trend of foliations or mylonite zones indicating a strong control exerted by pre-existing basement structures (Figures 7, 8; Moore and Davidson, 1978). Faults parallel to the extension direction are related to reactivation of basement fabrics rather than to recent transcurrent faults (Moore and Davidson, 1978; Philippon et al., 2014).
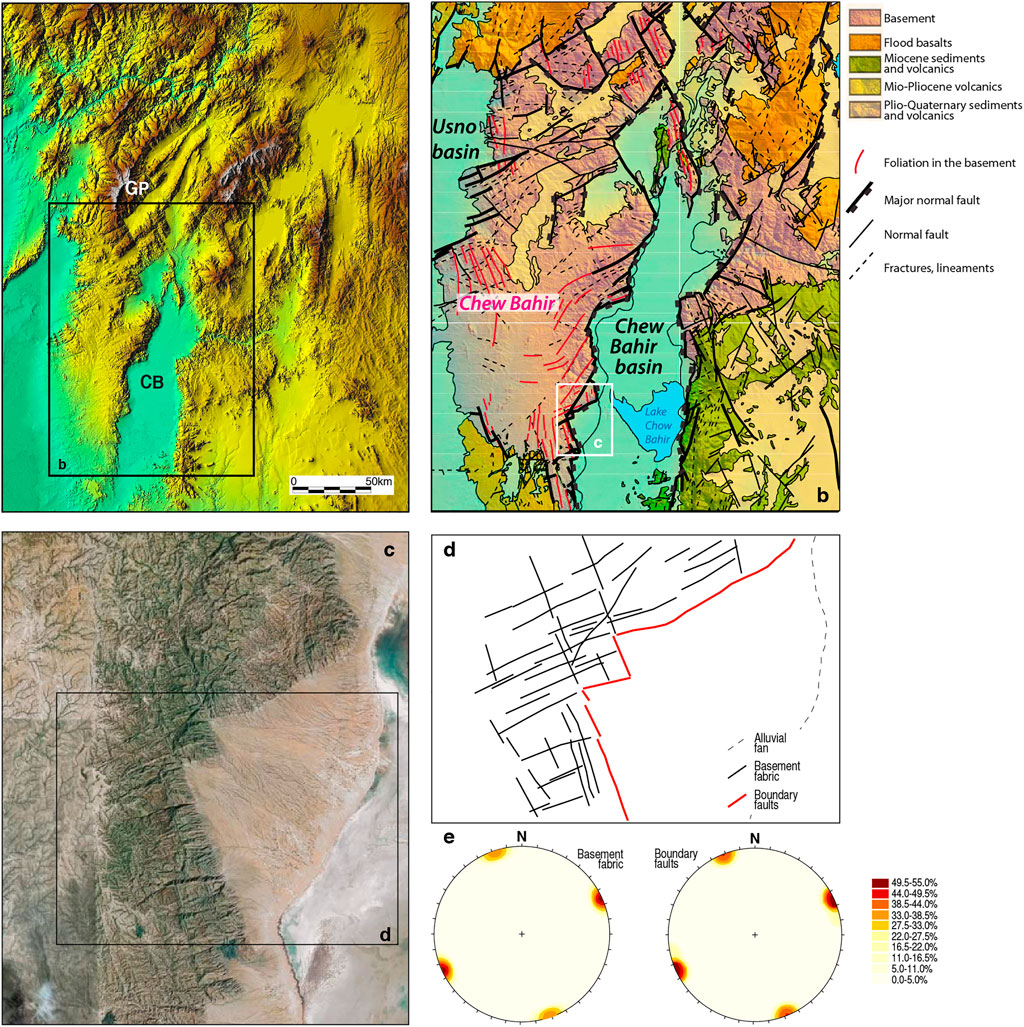
FIGURE 7. (A) Digital elevation model (NASA-SRTM) of southern Ethiopia. CB: Chew Bahir; GP: Gofa province. (B) Geological map of the Chew Bahir basin and surrounding areas, illustrating the pattern of foliation on the basement. (C) Typical angular pattern of boundary faults of the Chow Bahir basin (see text for details); (D) structural sketch. (E) Distribution of inherited basement fabrics and boundary faults, illustrated as plots of weighted fault azimuths. Note the similarity between the trend of basement fabrics and that of boundary faults, supporting that the development of the latter is strongly influenced by basement inherited weaknesses. Panels (A), (C), (D), (E) modified from Corti (2009); panel (B) modified from Philippon et al. (2014).
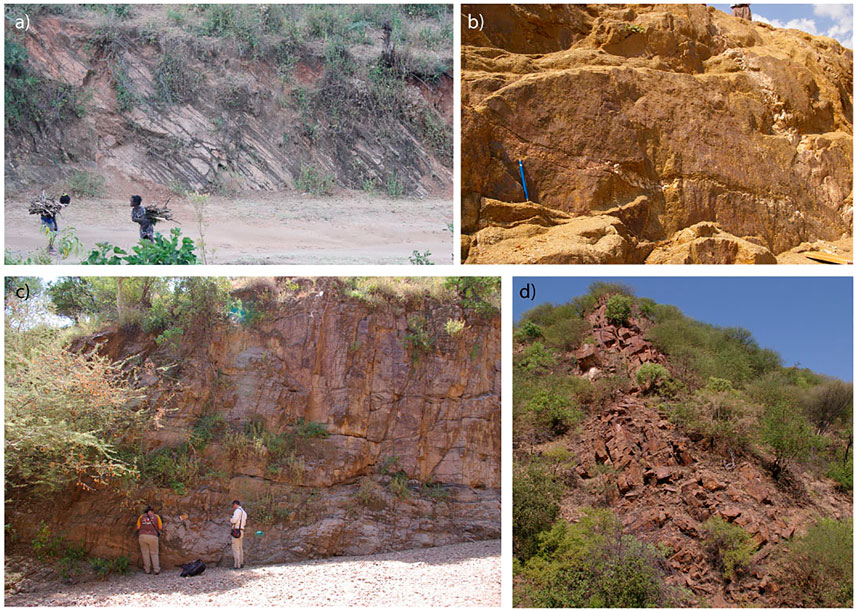
FIGURE 8. Field examples of structures affecting the Precambrian basement in the southern MER: (A) magmatic foliation and (B) Cenozoic fault plane affecting basement and cutting quartz vein and aplitic dike in the Gofa province; (C) System of sub parallel fractures reactivated and associated to a major normal fault (not visible of the left) in the Chew Bahir basin; (D) basement foliation sub parallel to the major fault plane delimiting the Chew Bahir basin.
A similar control has been suggested by recent works in the Ririba rift, at the southern termination of the Ethiopian Rift (Corti et al., 2019). In this rift, besides the angular fault pattern and the parallelism between faults and inherited fabrics, the main boundary faults are characterised by an anomalously low displacement/length ratio (i.e. long faults have very low displacement) and by displacement/length (D-L) curves (with flat top profiles, low-end gradients and low D-L ratio values) typical of a ‘constant length model’ of fault growth (see Supplementary Fig. 4 in Corti et al., 2019). This growth model is representative of reactivated fault systems in which fault lengths are inherited from underlying structures and established almost instantaneously on geological timescales, as also suggested for the nearby Kino Sogo fault belt in Kenya (Vétel et al., 2005; Vétel and Le Gall, 2006).
Another typical example is the Langano (or Haroresa) Rhomboidal Fault System (Le Turdu et al., 1999), located East of Lake Langano (Figure 9). In this area, the NE-SW-trending Asela-Langano escarpment curve to acquire a NW-SE trend and the interaction between NE-SW and NW-SE-trending structures give rise to a complex pattern of normal faults, with typical S- or Z-shaped plan-view geometries (Figure 9). This pattern and the curvature of the escarpment have been suggested to be controlled by a major NW-SE pre-existing crustal weakness zone, roughly parallel to the trend of the Red Sea (e.g., Korme et al., 2004). The existence of such pre-existing transverse structure close the Lake Langano is also supported by gravity data (Korme et al., 2004) which evidence the presence of NW-SE graben below the rift depression. A similar example of control on the local-scale fault pattern of inherited structures includes NW-SE faults East of Addis Ababa (Wolenchiti area) defining a NW-SE-trending graben filled by Pleistocene diatomite deposits (Korme et al., 2004).
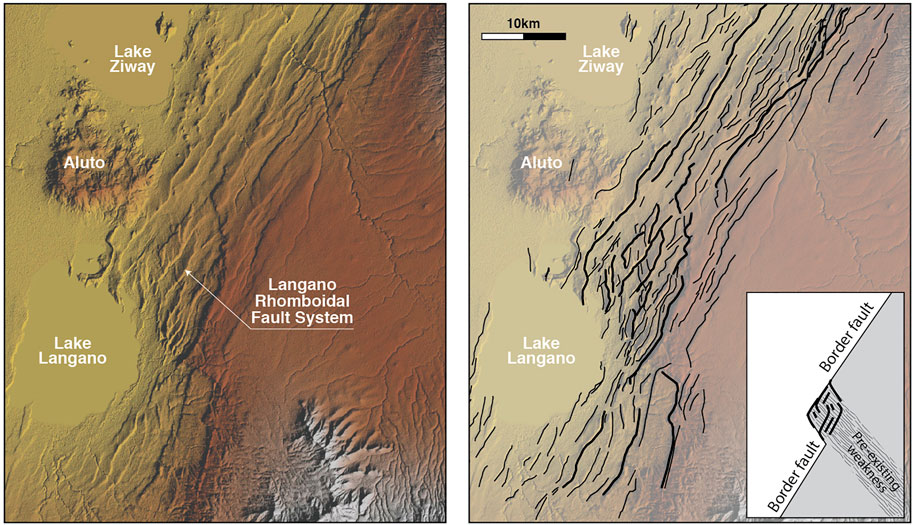
FIGURE 9. Digital elevation model (NASA-Shuttle Radar Topography Mission, SRTM 30 m resolution; left panel), and faults superimposed onto a SRTM digital elevation model (right panel) of the Langano Rhomboidal Fault System. Inset in the bottom right shows a schematic representation of the possible influence of a NW-SE-trending inherited weakness on the fault pattern. See text for details.
Control of Pre-existing Structures on the Distribution Volcanism
Many examples in the MER document a strong control exerted by pre-existing structures on the distribution of volcanic vents and edifice geometries. In the Ririba rift, at the southern termination of the MER, the Quaternary volcanic fields are aligned in a NE-SW direction and show no apparent relationship with the N-S-trending Pliocene boundary faults of the rift (Figure 10) therefore indicating that these structures do not exert a control on the pathways of magma ascent. This volcanism aligns parallel with regional, NE-SW/NNE-SSW-trending pre-existing lineaments (such as the Buluk Fault Zone in Figure 10) suggesting that the distribution of volcanic centres may have been controlled by these major deep inherited structures (e.g., Vétel and Le Gall, 2006; Corti et al., 2019; Franceschini et al., 2020). Magma ascent along these pre-existing structures may have been caused by abandonment of the Ririba rift and consequent deactivation of the main rift faults, and a stress re-organization due to gradients in crustal thickness (Franceschini et al., 2020). This resulted in buoyancy forces causing a local stress field with maximum horizontal stress orthogonal to the Turkana depression, enabling the uprising and emplacement of magma along NE-SW pre-existing structures. At a more local scale, the distribution of vents and their preferential directions of elongation (within individual volcanic fields) suggest a second-order control by inherited basement fabrics (Franceschini et al., 2020), as documented by the above-mentioned angular networks of minor normal faults observed in the area. Overall, the Ririba example supports that major, lithospheric-scale inherited structures may represent zones of crustal weakness that magma can exploit during its ascent, controlling the volcanic spatial and temporal evolution, volcanic morphology, magma volume, and eruptive dynamics (e.g., Le Corvec et al., 2013; Wadge et al., 2016).
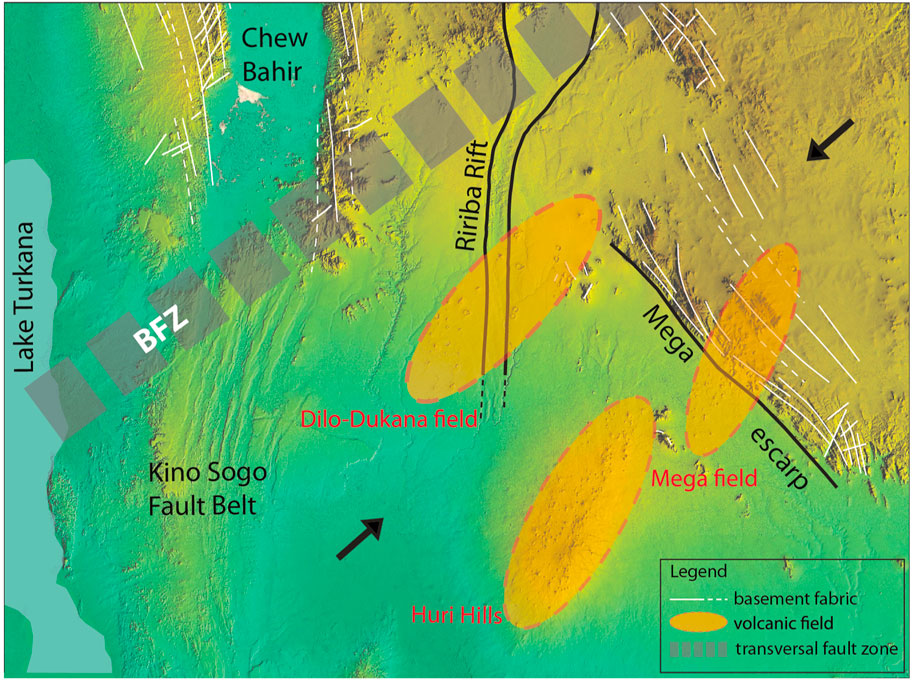
FIGURE 10. Schematic relations between the distribution of the Quaternary volcanic fields of Dilo, Mega and Huri Hills (orange ellipses) and major inherited structures (dashed lines in the figure) such as the BFZ (Buluk Fault Zone), modified from Franceschini et al. (2020). Black arrows schematically indicate the main compressive stress resulting from buoyancy forces in the area related to variations in crustal thickness and topography. See text for details.
In a recent compilation, Maestrelli et al. (2021) suggested that at least some calderas in the MER (e.g., Fantale, Kone, Gedemsa and Corbetti) may have experienced a tectonic control exerted by pre-existing faults reactivated during the collapse (i.e., fault-controlled caldera rim; Figure 11). Furthermore, Acocella et al. (2002) hypothesized a control exerted by inherited structures, reactivated during rift extension, on the localization for Fantale, Kone and Gedemsa calderas. In this regard, Lloyd et al. (2018) suggested the presence of a E-W deep rooted inherited structures controlling the localization and the structural setting of the Corbetti Caldera. As supported by Corti et al. (2018b), this structure may be related to the regional-scale Goba Bonga lineament.
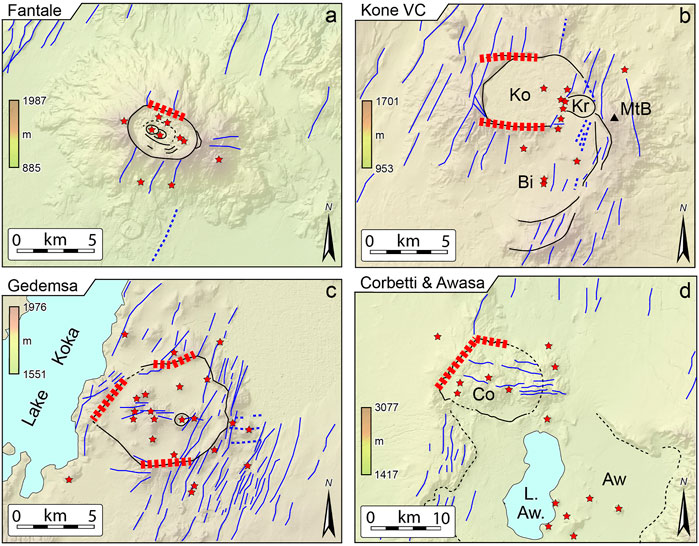
FIGURE 11. Structural control on collapsed caldera margin at (A) Fantale, (B) Kone Volcanic complex (KVC), (C) Gedemsa and (D) Corbetti. Red dashed lines indicate caldera ring faults controlled by inherited structures reactivated during the collapse. Red stars mark volcanic emission points (modified from Maestrelli et al., 2021). Ko: Kone caldera; Kr: Korke caldera; Bi: Birenti caldera; MtB: Mount Birenti; Co.: Corbetti; L. Aw, Lake Awasa; Aw: Awasa caldera remnants.
Previous works have also suggested the influence of inherited structures on the development of off-axis (or flank) volcanoes (Figure 12). Such volcanic edifices are located in the plateaus surrounding the rift, in an off-axis position with respect to the tectonic depression; a classic example of this volcanism is the Galama range, located in the Somalian plateau (Figure 12). Different models of magma generation and/or migration have been applied to explain the development of such volcanism (e.g., Bonini et al., 2001; Maccaferri et al., 2014; Chiasera et al., 2018). However, in most of the different models, the final uprising of magma at shallow crustal levels, resulting in the alignment of dikes and scoria cones in an area of thicker crust, has been related to ascending mafic dikes exploiting pre-existing basement faults and fractures slightly oblique to the rift margins (Mohr and Potter, 1976; Chiasera et al., 2018). Off-axis volcanism is also associated with pre-existing E-W lithospheric structures corresponding the Yerer-Tullu Wellel and Goba Bonga transversal lineaments (Abebe Adhana, 2014; Corti et al., 2018b).
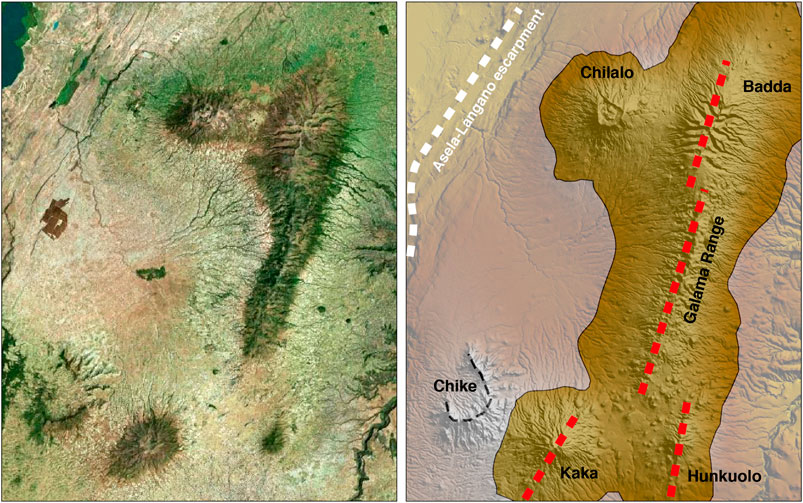
FIGURE 12. Satellite image (left) and main off-axis volcanoes (right) in the Somalian Plateau. Red dashed lines indicate the trend of inherited fabrics that possibly feed the volcanoes.
Discussions and Implications for Reactivation of Pre-existing Structures During Continental Rifting
Understanding how the pre-existing structure of the continental lithosphere influences rifting is of primary importance, as it may have an impact on several aspects of the rifting process and its outcomes. These may include the potential architecture (e.g., symmetry/asymmetry) of resulting passive margins, the possible segmentation of oceanic domains and transform faults separating them, the characteristics (length, depth extent, segmentation) of seismogenic faults (with implications for maximum magnitude of earthquakes), the distribution, volumes, and dynamics of associated volcanism (influencing volcanic risks and geo-resources such as geothermal energy). Because of this, the relationship between the Proterozoic crustal/lithospheric framework and the pattern of rift-related structures in East Africa has attracted considerable debate over the last decades (see Purcell, 2017).
Examples from the MER provide useful insights into this debate. Specifically, these examples document a significant control exerted by inherited heterogeneities at all different scales, which is illustrated in Figure 13.
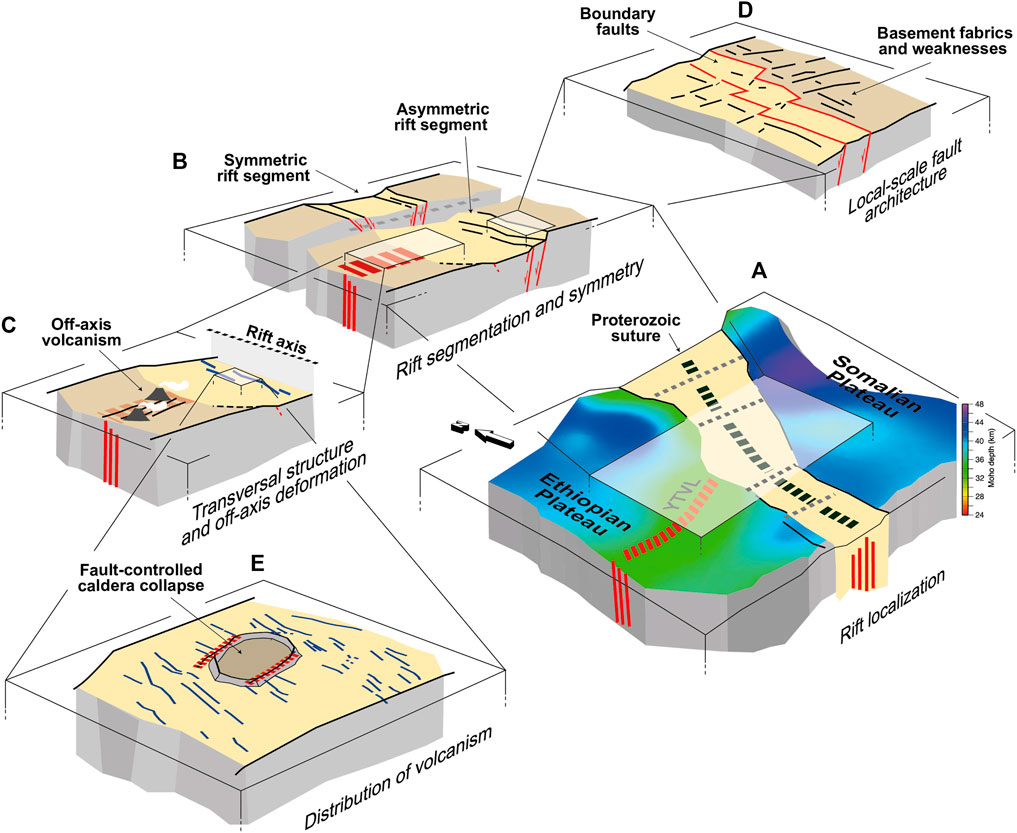
FIGURE 13. Schematic illustration of the control exerted by inherited heterogeneities at different scales in the MER. From regional-scale to local-scale, we observe: (A) Rift localization controlled by Proterozoic suture. YTVL: Yerer-Tullu Wellel transversal structure; (B) Rift segmentation and asymmetry induced by large-scale transversal structures; (C) Off-Axis volcanism controlled by large-scale transversal structures; (D) Local-scale architecture controlled by inherited basement fabrics; (E) Distribution and setting of volcanism controlled by local scale-inherited structures.
At a large scale (up to ∼800–1,000 km), the localisation of extensional deformation and the plan-view geometry of the rift valley is largely controlled by a NE-SW- to N-S-trending, lithospheric-scale Precambrian suture zone (Figure 13A). Suture zones may be indeed weaker than the normal lithosphere, because of processes including increased heat production in the thickened crust and the presence of inherited faults that can weaken the crust (Buiter and Torsvik, 2014). This explains why suture zones are typically reactivated during extension and localize extension-related deformation, as documented in many examples worldwide (see review in Buiter and Torsvik, 2014). In Ethiopia, the along-axis variations in the trend of the suture and the resulting rift valley, determine along-axis differences in the kinematics of rifting, from orthogonal in the southern MER to moderately oblique in the northern MER, which have important implications for rift architecture and evolution (see Keir et al., 2015).
Within the rift, the inherited rheological heterogeneities and different strength of the lithospheric domains surrounding the rift, and the presence of transversal structures, control the interaction between the Ethiopian and Kenyan rift, as well as the along-axis segmentation of the rift valley (Figure 13B). Specifically, the strength difference (or similarity) of the lithosphere beneath the plateaus controlled the development of 80–100 km-long rift portions characterised by asymmetry (or symmetry) of the rift valley. Recent analysis from other continental rifts (e.g., Malawi Rift, Upper Rhine Graben; Laó-Dávila et al., 2015; Grimmer et al., 2017) support that along-axis variations in inherited structures (e.g., basement fabrics) have a significant influence on basin architecture and segmentation, and on the characteristics of the rift margins.
Roughly E-W, lithospheric-scale inherited weaknesses control the development of off-axis volcano-tectonic activity in the plateaus surrounding the rift, an activity which may extend hundreds of kilometres away from the rift (Figure 13C). Transversal pre-existing structures may have controlled other characteristics of the MER, such as its plan-view geometry and its deflection southeast of Addis Ababa. Similar controls have been described in other regions of East Africa: for instance, the inherited Aswa Shear Zone has been suggested to control rift deflection in northern Kenya and the transfer of strain from the Western Branch to the Eastern Branch of the EARS (e.g., Purcell, 2017 and references therein).
At a local scale (<80 km), pre-existing structures may control the geometry of extension-related normal faults, causing anomalous fault patterns (Figure 13D): when controlled by inherited structures, normal faults may deviate from linear to zig-zag or sigmoidal plan-view geometries, with atypical displacement/length (D/L) curves and fault lengths established almost instantaneously on geological timescales (constant length model of fault growth). Examples from other sectors of the EARS (e.g., Malawi) confirm that pre-existing basement fabrics may have an important influence on the architecture of later rift-related faults (e.g., Williams et al., 2019; Wedmore et al., 2020; Kolawole et al., 2021). However, this may not always be the case: other studies have shown that pre-existing weaknesses only locally control border fault geometry at subsurface (e.g., Hodge et al., 2018) or that high-angle normal faults may cut through low-angle basement fabrics (e.g., Ebinger et al., 1989). In the Kenya rift, recent studies (Muirhead and Kattenhorn, 2018) points to a complex time-evolution of inheritance during rifting, with reactivation of pre-existing structures documented to postdate rift initiation and occur in an advanced rifting stage. In this case, later activation of inherited fabrics may reflect a complex contribution by magma-assisted deformation (Muirhead and Kattenhorn, 2018). A time-dependent reactivation of inherited structures has been also documented in other regions of the EARS, such as the Turkana depression of southern Ethiopia and northern Kenya. There, contrarily to what suggested for the Kenya rift, field analyses and seismic reflection data indicate that some NE-SW-trending basement structures have been reactivated during initial rifting but then abandoned during progressive extension, given their non-optimal orientation with respect to the roughly E-W extension direction (Nutz et al., 2021). Similarly, NW-SE-trending faults related to a previous Cretaceous-Early Paleogene extension phase are non-optimally oriented with respect to the roughly E-W extension of Cenozoic rifting. In this case, geodetic observations, and analysis of present-day deformation (Knappe et al., 2020) indicate no reactivation of these pre-existing faults during later extension, as also supported by analogue modelling of extension in the region (Wang et al., 2021). These analogue models indicate that the absence of fault reactivation may be related to a limited development of structures during the early rift phase, with a small volume of crust affected by pre-existing weak zones and a low reduction in strength in the brittle crust, and to their obliquity with respect to the later extension direction. In general, the strength contrast between the undeformed crust and that affected by pre-existing structures and the orientation, size and depth of inherited faults with respect to the extension direction are controlling factors in the reactivation of pre-existing structures as documented in many modelling works (e.g., Bellahsen and Daniel, 2005; Maestrelli et al., 2020; Molnar et al., 2017, 2019, 2020; Osagiede et al., 2021; Samsu et al., 2021; Schori et al., 2021; Wang et al., 2021, Zwaan and Schreurs, 2017; Zwaan et al., 2021a,b).
Inherited structures also control the patterns of migration and emplacement of rift-related magmas (Figure 13E), which may at some places show no direct relations to rift related faults and rather cut them. The MER examples support that pre-existing structures may control the spatial and temporal evolution of volcanic activity, its volume and eruptive dynamics, as observed in other parts of the EARS (such as the Chyulu Hills in the Kenya Rift; e.g., Mazzarini and Isola, 2021) and other regions undergoing extension (e.g., Gómez-Vasconcelos et al., 2020).
Concluding Remarks
We have shown how inherited structures have controlled the development of the MER from regional to local-scale. In general, as typically observed in other rift settings, the influence of inheritance on rift-related deformation is rather obvious at a regional scale, as rift valleys localise within lithospheric-scale weak zones avoiding stronger regions. Similarly, large, lithospheric-scale transversal structures influenced the MER segmentation, symmetry and off-axis volcanic activity. Examples in the MER document a local-scale influence of inherited structures on normal fault geometries and rift-related volcanism. However, comparison with other examples from the EARS suggests that the relations between pre-rift structures and individual rift basins or faults are more complex and several aspects of fault reactivation at a local scale remain enigmatic. These include, among others, the time-space variations of reactivation during rift progression and its dependence on parameters such as: the volume of crust affected by pre-existing weak zones and/or their dimensions, the strength contrast required for their re-use, and their dip and orientation with respect to the extension direction. Additional detailed studies in locations where we can clearly analyse, in 3D, crustal faults and ancient structures are therefore needed to improve our knowledge of these complex relations.
Author Contributions
GC conceptualized and wrote the work; FS and DM contributed to the writing.
Conflict of Interest
The authors declare that the research was conducted in the absence of any commercial or financial relationships that could be construed as a potential conflict of interest.
Publisher’s Note
All claims expressed in this article are solely those of the authors and do not necessarily represent those of their affiliated organizations, or those of the publisher, the editors and the reviewers. Any product that may be evaluated in this article, or claim that may be made by its manufacturer, is not guaranteed or endorsed by the publisher.
Acknowledgments
We thank the reviewers for the comments that helped to improve previous versions of the manuscript. This work has been funded by the Italian Ministero dell’Università e della Ricerca (MiUR) through PRIN Grant 2017P9AT72.
References
Abbate, E., Bruni, P., and Sagri, M. (2015). “Geology of Ethiopia: A Review and Geomorphological Perspectives,” in Landscapes and Landforms of Ethiopia, World Geomorphological Landscapes. Editor P. Billi (Dordrecht: Springer Science+Business Media), 33–64. doi:10.1007/978-94-017-8026-1_2
Abbate, E., and Sagri, M. (1980). Volcanites of Ethiopian and Somali Plateaus and Major Tectonic Lines. Atti Convegni Lincei 47, 219–227.
Abdelsalam, M. G., and Stern, R. J. (1996). Sutures and Shear Zones in the Arabian-Nubian Shield. J. Afr. Earth Sci. 23, 289–310. doi:10.1016/s0899-5362(97)00003-1
Abebe Adhana, T. (2014). The Occurrence of a Complete continental Rift Type of Volcanic Rocks Suite along the Yerer-Tullu Wellel Volcano Tectonic Lineament, Central Ethiopia. J. Afr. Earth Sci. 99, 374–385. doi:10.1016/j.jafrearsci.2014.02.008
Abebe, T., Mazzarini, F., Innocenti, F., and Manetti, P. (1998). The Yerer-Tullu Wellel Volcanotectonic Lineament: a Transtensional Structure in central Ethiopia and the Associated Magmatic Activity. J. Afr. Earth Sci. 26, 135–150. doi:10.1016/s0899-5362(97)00141-3
Acocella, V., Korme, T., Salvini, F., and Funiciello, R. (2002). Elliptic Calderas in the Ethiopian Rift: Control of Pre-existing Structures. J. Volcanol. Geotherm. Res. 119 (1–4), 189–203. doi:10.1016/S0377-0273(02)00342-6
Agostini, A., Bonini, M., Corti, G., Sani, F., and Mazzarini, F. (2011). Fault Architecture in the Main Ethiopian Rift and Comparison with Experimental Models: Implications for Rift Evolution and Nubia-Somalia Kinematics. Earth Planet. Sci. Lett. 301, 479–492. doi:10.1016/j.epsl.2010.11.024
Autin, J., Bellahsen, N., Leroy, S., Husson, L., Beslier, M.-O., and d'Acremont, E. (2013). The Role of Structural Inheritance in Oblique Rifting: Insights from Analogue Models and Application to the Gulf of Aden. Tectonophysics 607, 51–64. doi:10.1016/j.tecto.2013.05.041
Bastow, I. D., Nyblade, A. A., Stuart, G. W., Rooney, T. O., and Benoit, M. H. (2008). Upper Mantle Seismic Structure beneath the Ethiopian Hot Spot: Rifting at the Edge of the African Low-Velocity Anomaly. Geochem. Geophys. Geosyst. 9 (12), na. doi:10.1029/2008GC002107
Bastow, I. D., Pilidou, S., Kendall, J.-M., and Stuart, G. W. (2010). Melt-induced Seismic Anisotropy and Magma Assisted Rifting in Ethiopia: Evidence from Surface Waves. Geochem. Geophys. Geosyst. 11 (6), na. doi:10.1029/2010GC003036
Bastow, I. D., Stuart, G. W., Kendall, J.-M., and Ebinger, C. J. (2005). Upper-mantle Seismic Structure in a Region of Incipient continental Breakup: Northern Ethiopian Rift. Geophys. J. Int. 162 (2), 479–493. doi:10.1111/j.1365-246x.2005.02666.x
Bell, R. E., Jackson, C. A. L., Whipp, P. S., and Clements, B. (2014). Strain Migration during Multiphase Extension: Observations from the Northern North Sea. Tectonics 33 (10), 1936–1963. doi:10.1002/2014tc003551
Bellahsen, N., and Daniel, J. M. (2005). Fault Reactivation Control on normal Fault Growth: an Experimental Study. J. Struct. Geology. 27, 769–780. doi:10.1016/j.jsg.2004.12.003
Bendick, R., McClusky, S., Bilham, R., Asfaw, L., and Klemperer, S. (2006). Distributed Nubia-Somalia Relative Motion and dike Intrusion in the Main Ethiopian Rift. Geophys. J. Int. 165 (1), 303–310. doi:10.1111/j.1365-246x.2006.02904.x
Benoit, M. H., Nyblade, A. A., and Pasyanos, M. E. (2006). Crustal Thinning between the Ethiopian and East African Plateaus from Modeling Rayleigh Wave Dispersion. Geophys. Res. Lett. 33 (13), L13301. doi:10.1029/2006GL025687
Berhe, S. M. (1990). Ophiolites in Northeast and East Africa: Implications for Proterozoic Crustal Growth. J. Geol. Soc. 147, 41–57. doi:10.1144/gsjgs.147.1.0041
Beutel, E., van Wijk, J., Ebinger, C., Keir, D., and Agostini, A. (2010). Formation and Stability of Magmatic Segments in the Main Ethiopian and Afar Rifts. Earth Planet. Sci. Lett. 293 (3-4), 225–235. doi:10.1016/j.epsl.2010.02.006
Birhanu, Y., Bendick, R., Fisseha, S., Lewi, E., Floyd, M., King, R., et al. (2016). GPS Constraints on Broad Scale Extension in the Ethiopian Highlands and Main Ethiopian Rift. Geophys. Res. Lett. 43 (13), 6844–6851. doi:10.1002/2016gl069890
Boccaletti, M., Bonini, M., Mazzuoli, R., Abebe, B., Piccardi, L., and Tortorici, L. (1998). Quaternary Oblique Extensional Tectonics in the Ethiopian Rift (Horn of Africa). Tectonophysics 287, 97–116. doi:10.1016/s0040-1951(98)80063-2
Bonini, M., Corti, G., Innocenti, F., Manetti, P., Mazzarini, F., Abebe, T., et al. (2005). Evolution of the Main Ethiopian Rift in the Frame of Afar and Kenya Rifts Propagation. Tectonics 24, na. doi:10.1029/2004TC001680
Bonini, M., Sokoutis, D., Mulugeta, G., Boccaletti, M., Corti, G., Innocenti, F., et al. (2001). Dynamics of Magma Emplacement in Centrifuge Models of continental Extension with Implications for Flank Volcanism. Tectonics 20, 1053–1065. doi:10.1029/2001tc900017
Brune, S., Corti, G., and Ranalli, G. (2017). Controls of Inherited Lithospheric Heterogeneity on Rift Linkage: Numerical and Analog Models of Interaction between the Kenyan and Ethiopian Rifts across the Turkana Depression. Tectonics 36, 1767–1786. doi:10.1002/2017TC004739
Buck, W. R. (2004). “1. Consequences of Asthenospheric Variability on Continental Rifting,” in Rheology and Deformation of the Lithosphere at Continental Margins. Editors G. D. Karner, B. Taylor, N. W. Droscoll, and D. L. Kohlstedt (New York: Columbia Univ. Press), 1–30. doi:10.7312/karn12738-002
Buiter, S. J. H., and Torsvik, T. H. (2014). A Review of Wilson Cycle Plate Margins: a Role for Mantle Plumes in continental Break-Up along Sutures. Gondwana Res. 26, 627–653. doi:10.1016/j.gr.2014.02.007
Casey, M., Ebinger, C., Keir, D., Gloaguen, R., and Mohamed, F. (2006). “Strain Accommodation in Transitional Rifts: Extension by Magma Intrusion and Faulting in Ethiopian Rift Magmatic Segments,” in The Afar Volcanic Province within the East African Rift System. Editors G. Yirgu, C. J. Ebinger, and P. K. H. Maguire (London: Geological Society Special Publication), 259, 143–163. doi:10.1144/gsl.sp.2006.259.01.13Geol. Soc. Lond. Spec. Publications
Chang, S.-J., Kendall, E., Davaille, A., and Ferreira, A. M. G. (2020). The Evolution of Mantle Plumes in East Africa. J. Geophys. Res. 125, e2020JB019929. doi:10.1029/2020JB019929
Chiasera, B., Rooney, T. O., Girard, G., Yirgu, G., Grosfils, E., Ayalew, D., et al. (2018). Magmatically Assisted Off-Rift Extension-The Case for Broadly Distributed Strain Accommodation. Geosphere 14 (4), 1544–1563. doi:10.1130/GES01615.1
Chorowicz, J., Collet, B., Bonavia, F. F., and Korme, T. (1994). Northwest to North-Northwest Extension Direction in the Ethiopian Rift Deduced from the Orientation of Extension Structures and Fault-Slip Analysis. Geol. Soc. America Bull. 106, 1560–1570. doi:10.1130/0016-7606(1994)105<1560:ntnned>2.3.co;2
Chorowicz, J. (2005). The East African Rift System. J. Afr. Earth Sci. 43, 379–410. doi:10.1016/j.jafrearsci.2005.07.019
Claesson, S., Pallister, J. S., and Tatsumoto, M. (1984). Samarium-neodymium Data on Two Late Proterozoic Ophiolites of Saudi Arabia and Implications for Crustal and Mantle Evolution. Contr. Mineral. Petrol. 85, 244–252. doi:10.1007/bf00378103
Cornwell, D. G., Maguire, P. K. H., England, R. W., and Stuart, G. W. (2010). Imaging Detailed Crustal Structure and Magmatic Intrusion across the Ethiopian Rift Using a Dense Linear Broadband Array. Geochem. Geophys. Geosyst. 11, na. doi:10.1029/2009GC002637
Corti, G., Cioni, R., Franceschini, Z., Sani, F., Scaillet, S., Molin, P., et al. (2019). Aborted Propagation of the Ethiopian Rift Caused by Linkage with the Kenyan Rift. Nat. Commun. 10, 1309. doi:10.1038/s41467-019-09335-2
Corti, G. (2009). Continental Rift Evolution: from Rift Initiation to Incipient Break-Up in the Main Ethiopian Rift, East Africa. Earth-Science Rev. 96, 1–53. doi:10.1016/j.earscirev.2009.06.005
Corti, G. (2008). Control of Rift Obliquity on the Evolution and Segmentation of the Main Ethiopian Rift. Nat. Geosci 1, 258–262. doi:10.1038/ngeo160
Corti, G. (2012). Evolution and Characteristics of continental Rifting: Analog Modeling-Inspired View and Comparison with Examples from the East African Rift System. Tectonophysics 522-523, 1–33. doi:10.1016/j.tecto.2011.06.010
Corti, G., Molin, P., Sembroni, A., Bastow, I. D., and Keir, D. (2018a). Control of Pre-rift Lithospheric Structure on the Architecture and Evolution of continental Rifts: Insights from the Main Ethiopian Rift, East Africa. Tectonics 37, 477–496. doi:10.1002/2017TC004799
Corti, G., Philippon, M., Sani, F., and Keir, D. (2013). Re-orientation of the Extension Direction and Pure Extensional Faulting at Oblique Rift Margins: Comparison between the Main Ethiopian Rift and Laboratory Experiments. Terra Nova 25, 396–404. doi:10.1111/ter.12049
Corti, G., Sani, F., Agostini, S., Philippon, M., Sokoutis, D., and Willingshofer, E. (2018b). Off-axis Volcano-Tectonic Activity during continental Rifting: Insights from the Transversal Goba-Bonga Lineament, Main Ethiopian Rift (East Africa). Tectonophysics 728-729, 75–91. doi:10.1016/j.tecto.2018.02.011
Corti, G., Sani, F., Florio, A. A., Greenfield, T., Keir, D., Erbello, A., et al. (2020). Tectonics of the Asela-Langano Margin, Main Ethiopian Rift (East Africa). Tectonics 39, e2020TC006075. doi:10.1029/2020TC006075
Corti, G., van Wijk, J., Cloetingh, S., and Morley, C. K. (2007). Tectonic Inheritance and continental Rift Architecture: Numerical and Analogue Models of the East African Rift System. Tectonics 26 (6), na. doi:10.1029/2006tc002086
Courtillot, V., Jaupart, C., Manighetti, I., Tapponnier, P., and Besse, J. (1999). On Causal Links between Flood Basalts and continental Breakup. Earth Planet. Sci. Lett. 166, 177–195. doi:10.1016/s0012-821x(98)00282-9
Daly, E., Keir, D., Ebinger, C. J., Stuart, G. W., Bastow, I. D., and Ayele, A. (2008). Crustal Tomographic Imaging of a Transitional continental Rift: the Ethiopian Rift. Geophys. J. Int. 172, 1033–1048. doi:10.1111/j.1365-246x.2007.03682.x
Daniels, K. A., Bastow, I. D., Keir, D., Sparks, R. S. J., and Menand, T. (2014). Thermal Models of Dyke Intrusion during Development of Continent-Ocean Transition. Earth Planet. Sci. Lett. 385, 145–153. doi:10.1016/j.epsl.2013.09.018
Deng, C., Fossen, H., Gawthorpe, R. L., Rotevatn, A., Jackson, C. A. L., and FazliKhani, H. (2017). Influence of Fault Reactivation during Multiphase Rifting: the Oseberg Area, Northern North Sea Rift. Mar. Pet. Geol. 86, 1252–1272. doi:10.1016/j.marpetgeo.2017.07.025
Deng, C., Gawthorpe, R. L., Fossen, H., and Finch, E. (2018). How Does the Orientation of a Preexisting Basement Weakness Influence Fault Development during Renewed Rifting? Insights from Three-Dimensional Discrete Element Modeling. Tectonics 37 (7), 2221–2242. doi:10.1029/2017tc004776
Díaz-Alvarado, J., Pedrera, A., Azor, A., García-Senz, J., Alberto Díaz de Neira, J., and Rodríguez-Fernández, L. R. (2021). Submarine Basaltic Magmatism in the Subbetic Basin (Southern Spain): Insights into Melt-Weakening Processes during Mesozoic Continental Rifting. Lithosphere 2021 (1), 5505884. doi:10.2113/2021/5505884
Duffy, O. B., Bell, R. E., Jackson, C. A. L., Gawthorpe, R. L., and Whipp, P. S. (2015). Fault Growth and Interactions in a Multiphase Rift Fault Network: Horda Platform, Norwegian North Sea. J. Struct. Geol. 80, 99–119. doi:10.1016/j.jsg.2015.08.015
Dugda, M. T., Nyblade, A. A., Jordi, J., Langston, C. A., Ammon, C. J., and Simiyu, S. (2005). Crustal Structure in Ethiopia and Kenya from Receiver Function Analysis: Implications for Rift Development in Eastern Africa. J. Geophys. Res. 110 (B1), B01303. doi:10.1029/2004JB003065
Dunbar, J. A., and Sawyer, D. S. (1989). Continental Rifting at Pre-existing Lithospheric Weaknesses. Nature 242, 565–571.
Ebinger, C. (2005). Continental Breakup: The East African Perspective. Astron. Geophys. 46, 216–221. doi:10.1111/j.1468-4004.2005.46216.x
Ebinger, C. J., and Casey, M. (2001). Continental Breakup in Magmatic Provinces: An Ethiopian Example. Geology 29, 527–530. doi:10.1130/0091-7613(2001)029<0527:cbimpa>2.0.co;2
Ebinger, C. J., Yemane, T., Harding, D. J., Tesfaye, S., Kelley, S., and Rex, D. C. (2000). Rift Deflection, Migration, and Propagation: Linkage of the Ethiopian and Eastern Rifts, Africa. Geol. Soc. America Bull. 112 (2), 163–176. doi:10.1130/0016-7606(2000)112<163:rdmapl>2.0.co;2
Ebinger, C. J., Deino, A. L., Drake, R. E., and Tesha, A. L. (1989). Chronology of Volcanism and Rift Basin Propagation: Rungwe Volcanic Province, East Africa. J. Geophys. Res. 94, 15785–15803.
Edel, J. B., Schulmann, K., and Rotstein, Y. (2007). The Variscan Tectonic Inheritance of the Upper Rhine Graben: Evidence of Reactivations in the Lias, Late Eocene–Oligocene up to the Recent. Int. J. Earth Sci. 96 (2), 305. doi:10.1007/s00531-006-0092-8
Emishaw, L., and Abdelsalam, M. G. (2019). Development of Late Jurassic‐Early Paleogene and Neogene‐Quaternary Rifts within the Turkana Depression, East Africa from Satellite Gravity Data. Tectonics 38 (7), 2358–2377. doi:10.1029/2018tc005389
Erbello, A., Corti, G., Agostini, A., Sani, F., Kidane, T., and Buccianti, A. (2016). Modeling along-axis Variations in Fault Architecture in the Main Ethiopian Rift: Implications for Nubia-Somalia Kinematics. J. Geodynamics 102, 24–38. doi:10.1016/j.jog.2016.07.002
Franceschini, Z., Cioni, R., Scaillet, S., Corti, G., Sani, F., Isola, I., et al. (2020). Recent Volcano-Tectonic Activity of the Ririba Rift and the Evolution of Rifting in South Ethiopia. J. Volcanology Geothermal Res. 402, 106989. doi:10.1016/j.jvolgeores.2020.106989
Gallacher, R. J., Keir, Derek., Harmon, Nicholas., Stuart, G., Leroy, Sylvie., Hammond, James., et al. (2016). The Initiation of Segmented Buoyancy-Driven Melting during continental Breakup. Nat. Commun. 7, 13110. doi:10.1038/ncomms13110
Gani, N. D. S., Abdelsalam MGGera, S., and Gani, M. R. (2009). Stratigraphic and Structural Evolution of the Blue Nile Basin, Northwestern Ethiopian Plateau. Geol. J. 44, 30–56. doi:10.1002/gj.1127
Gashawbeza, E. M., Klemperer, S. L., Nyblade, A. A., Walker, K. T., and Keranen, K. M. (2004). Shear-wave Splitting in Ethiopia: Precambrian Mantle Anisotropy Locally Modified by Neogene Rifting. Geophys. Res. Lett. 31, L18602. doi:10.1029/2004GL020471
Gómez-Vasconcelos, M. G., Macías, J., Avellán, Denis., Sosa-Ceballos, Giovanni., Garduño-Monroy, Víctor., Cisneros, Guillermo., et al. (2020). The Control of Preexisting Faults on the Distribution, Morphology, and Volume of Monogenetic Volcanism in the Michoacán-Guanajuato Volcanic Field. GSA Bull. 132, 2455–2474. doi:10.1130/B35397.1
Grimmer, J. C., Ritter, J. R. R., Eisbacher ·, G. H., and Fielitz, V. (2017). The Late Variscan Control on the Location and Asymmetry of the Upper Rhine Graben. Int. J. Earth Sci. (Geol Rundsch) 106, 827–853. doi:10.1007/s00531-016-1336-x
Hayward, N. J., and Ebinger, C. J. (1996). Variations in the along-axis Segmentation of the Afar Rift System. Tectonics 15, 244–257. doi:10.1029/95tc02292
Henstra, G. A., Berg Kristensen, T., Rotevatn, A., and Gawthorpe, R. L. (2019). How Do Pre-existing normal Faults Influence Rift Geometry? A Comparison of Adjacent Basins with Contrasting Underlying Structure on the Lofoten Margin, Norway. Basin Res. 31 (6), 1083–1097. doi:10.1111/bre.12358
Henstra, G. A., Rotevatn, A., Gawthorpe, R. L., and Ravnås, R. (2015). Evolution of a Major Segmented normal Fault during Multiphase Rifting: the Origin of Plan-View Zigzag Geometry. J. Struct. Geol. 74, 45–63. doi:10.1016/j.jsg.2015.02.005
Hodge, M., Fagereng, A., Biggs, J., and Mdala, H. (2018). Controls on Early‐rift Geometry: New Perspectives from the Bilila‐Mtakataka Fault, Malawi. Geophys. Res. Lett. 45 (9), 3896–3905. doi:10.1029/2018gl077343
Kazmin, V., Seife, M. B., Nicoletti, M., and Petrucciani, C. (1980). “Evolution of the Northern Part of the Ethiopian Rift,” in Geodynamic Evolution of the Afro-Arabian Rift System (Roma: Accademia Nazionale Dei Lincei, Atti dei Convegni Lincei), 47, 275–292.
Kazmin, V., Shifferaw, A., and Balcha, T. (1978). The Ethiopian Basement: Stratigraphy and Possible Manner of Evolution. Geologische Rundschau 67 (2), 531–546. doi:10.1007/bf01802803
Keir, D., Bastow, I. D., Corti, G., Mazzarini, F., and Rooney, T. O. (2015). The Origin of Along-Rift Variations in Faulting and Magmatism in the Ethiopian Rift. Tectonics 34, 464–477. doi:10.1002/2014TC003698
Keir, D., Ebinger, C. J., Stuart, G. W., Daly, E., and Ayele, A. (2006). Strain Accommodation by Magmatism and Faulting as Rifting Proceeds to Breakup: Seismicity of the Northern Ethiopian Rift. J. Geophys. Res. 111 (B5), B05314. doi:10.1029/2005JB003748
Kendall, J. M., Stuart, G. W., Ebinger, C. J., Bastow, I. D., and Keir, D. (2005). Magma Assisted Rifting in Ethiopia. Nature 433, 146–148. doi:10.1038/nature03161
Keranen, K., and Klemperer, S. L. (2008). Discontinuous and Diachronous Evolution of the Main Ethiopian Rift: Implications for the Development of continental Rifts. Earth Planet. Sci. Lettters 265, 96–111. doi:10.1016/j.epsl.2007.09.038
Keranen, K., Klemperer, S. L., and Gloaguen, R.Eagle working group (2004). Three-dimensional Seismic Imaging of a Protoridge axis in the Main Ethiopian Rift. Geology 32, 949–952. doi:10.1130/g20737.1
Keranen, K., Klemperer, S. L., Julia, J., Lawrence, J. L., and Nyblade, A. (2009). Low Lower-Crustal Velocity across Ethiopia: Is the Main Ethiopian Rift a Narrow Rift in a Hot Craton. Geochem. Geophys. Geosyst. 10, Q0AB01. doi:10.1029/2008GC002293
Knappe, E., Bendick, R., Ebinger, C., Birhanu, Y., Lewi, E., Floyd, M., et al. (2020). Accommodation of East African Rifting across the Turkana Depression. J. Geophys. Res. Solid Earth 125 (2), e2019JB018469. doi:10.1029/2019jb018469
Kogan, L., Fisseha, S., Bendick, R., Reilinger, R., McClusky, S., King, R., et al. (2012). Lithospheric Strength and Strain Localization in continental Extension from Observations of the East African Rift. J. Geophys. Res. 117, B03402. doi:10.1029/2011JB008516
Kolawole, F., Phillips, T. B., Atekwana, E. A., and Jackson, C. A. L. (2021). Structural Inheritance Controls Strain Distribution during Early Continental Rifting, Rukwa Rift. Front. Earth Sci. 9, 707869 doi:10.3389/feart.2021.707869
Korme, T., Acocella, V., and Abebe, B. (2004). The Role of Pre-existing Structures in the Origin, Propagation and Architecture of Faults in the Main Ethiopian Rift. Gondwana Res. 7, 467–479. doi:10.1016/s1342-937x(05)70798-x
Kroner, A., Todt, W., Hussein, I. M., Mansour, M., and Rashwan, A. A. (1992). Dating of Late Proterozoic Ophiolites in Egypt and the Sudan Using the Single Grain Zircon Evaporation Technique. Precambrian Res. 59, 15–32. doi:10.1016/0301-9268(92)90049-t
Laó-Dávila, D. A., Al-Salmi, H. S., Abdelsalam, M. G., and Atekwana, E. A. (2015). Hierarchical Segmentation of the Malawi Rift: The Influence of Inherited Lithospheric Heterogeneity and Kinematics in the Evolution of continental Rifts. Tectonics 34, 2399–2417. doi:10.1002/2015TC003953
Le Corvec, N., Menand, T., and Lindsay, J. (2013). Interaction of Ascending Magma with Pre-existing Crustal Fractures in Monogenetic Basaltic Volcanism: an Experimental Approach. J. Geophys. Res. Solid Earth 118, 968–984. doi:10.1002/jgrb.50142
Le Turdu, C., Tiercelin, J. J., Gibert, E., Travi, Y., Lezzar, K. E., Richert, J. P., et al. (1999). The Ziway–Shala lake basin System, Main Ethiopian Rift: Influence of Volcanism, Tectonics, and Climatic Forcing on basin Formation and Sedimentation. Palaeogeogr. Palaeoclimatol. Palaeoecol. 150, 135–177. doi:10.1016/S0031-0182(98)00220-X
Lloy, R., Biggs, J., Wilks, M., Nowacki, A., Kendall, J. M., Ayele, A., et al. (2018). Evidence for Cross Rift Structural Controls on Deformation and Seismicity at a Continental Rift Caldera. Earth Planet. Sci. Lett. 487, 190–200. doi:10.1016/j.epsl.2018.01.037
Maccaferri, F., Rivalta, E., Keir, D., and Acocella, V. (2014). Off-rift Volcanism in Rift Zones Determined by Crustal Unloading. Nat. Geosci. 7, 297–300. doi:10.1038/ngeo2110
Mackenzie, G. H., Thybo, G. H., and Maguire, P. (2005). Crustal Velocity Structure across the Main Ethiopian Rift: Results from 2-dimenional Wide-Angle Seismic Modeling. Geophys. J. Int. 162, 994–1006. doi:10.1111/j.1365-246x.2005.02710.x
Maestrelli, D., Corti, G., Bonini, M., Montanari, D., and Sani, F. (2021). Caldera Collapse and Tectonics along the Main Ethiopian Rift: Reviewing Possible Relationships. Comptes Rendus Geosci. 353 (S2), 1–19. doi:10.5802/crgeos.63
Maestrelli, D., Montanari, D., Corti, G., Del Ventisette, C., Moratti, G., and Bonini, M. (2020). Exploring the Interactions between Rift Propagation and Inherited Crustal Fabrics through Experimental Modeling. Tectonics 39 (12), e2020TC006211. doi:10.1029/2020TC006211
Mazzarini, F., and Isola, I. (2021). Vent Distribution and Structural Inheritance in an Embryonic Rift: The Example of the Chyulu Hills Off-Rift Magmatic Range (South Kenya). J. Volcanology Geothermal Res. 416, 107268. doi:10.1016/j.jvolgeores.2021.107268
Michon, L., and Sokoutis, D. (2005). Interaction between Structural Inheritance and Extension Direction during Graben and Depocentre Formation: an Experimental Approach. Tectonophysics 409 (1–4), 125–146. doi:10.1016/j.tecto.2005.08.020
Mohr, P. A., and Potter, E. C. (1976). The Sagatu Ridge dike Swarms, Ethiopian Rift Margin. J. Volcanol. Geotherm. Res. 1, 55–71. doi:10.1016/0377-0273(76)90018-4
Mohr, P. (1983). Volcanotectonic Aspects of the Ethiopian Rift Evolution. Bull. Centre Recherches Elf Aquitaine Exploration Prod. 7, 175–189.
Molin, P., and Corti, G. (2015). Topography, River Network and Recent Fault Activity at the Margins of the Central Main Ethiopian Rift (East Africa). Tectonophysics 664, 67–82. doi:10.1016/j.tecto.2015.08.045
Molnar, N., Cruden, A., and Betts, P. (2020). The Role of Inherited Crustal and Lithospheric Architecture during the Evolution of the Red Sea: Insights from Three Dimensional Analogue Experiments. Earth Planet. Sci. Lett. 544, 116377. doi:10.1016/j.epsl.2020.116377
Molnar, N. E., Cruden, A. R., and Betts, P. G. (2019). Interactions between Propagating Rifts and Linear Weaknesses in the Lower Crust. Geosphere 15 (5), 1617–1640. doi:10.1130/GES02119.1
Molnar, N. E., Cruden, A. R., and Betts, P. G. (2017). Interactions between Propagating Rotational Rifts and Linear Rheological Heterogeneities: Insights from Three‐dimensional Laboratory Experiments. Tectonics 36 (3), 420–443. doi:10.1002/2016TC004447
Moore, J. M., and Davidson, A. (1978). Rift Structure in Southern Ethiopia. Tectonophysics 46, 159–173. doi:10.1016/0040-1951(78)90111-7
Morley, C. K., Haranya, C., Phoosongsee, W., Pongwapee, S., Kornsawan, A., and Wonganan, N. (2004). Activation of Rift Oblique and Rift Parallel Pre-existing Fabrics during Extension and Their Effect on Deformation Style: Examples from the Rifts of Thailand. J. Struct. Geol. 26 (10), 1803–1829. doi:10.1016/j.jsg.2004.02.014
Morley, C. K. (1999). “Influence of Pre-existing Fabrics on Rift Structure,” in Geoscience of Rift Systems, Evolution of East Africa. Editor C. K. Morley (Tulsa, Oklahoma: AAPG Studies in Geology), 44, 151–160.
Morley, C. K. (2010). Stress Re-orientation along Zones of Weak Fabrics in Rifts: An Explanation for Pure Extension in ‘oblique’ Rift Segments. Earth Planet. Sci. Lett. 297, 667–673. doi:10.1016/j.epsl.2010.07.022
Muirhead, J. D., and Kattenhorn, S. A. (2018). Activation of Preexisting Transverse Structures in an Evolving Magmatic Rift in East Africa. J. Struct. Geology. 106, 1–18. doi:10.1016/j.jsg.2017.11.004
Nutz, A., Ragon, T., and Schuster, M. (2021). Cenozoic Tectono-Sedimentary Evolution of the Northern Turkana Depression (East African Rift System) and its Significance for continental Rifts. Earth Planet. Sci. Lett., 117285. doi:10.1016/j.epsl.2021.117285
Osagiede, E. E., Rosenau, M., Rotevatn, A., Gawthorpe, R., Jackson, C. A. L., and Rudolf, M. (2021). Influence of Zones of Pre‐Existing Crustal Weakness on Strain Localization and Partitioning during Rifting: Insights from Analog Modeling Using High‐Resolution 3D Digital Image Correlation. Tectonics 40 (10), e2021TC006970. doi:10.1029/2021TC006970
Pallister, J. S., Stacey, J. S., Fischer, L. B., and Premo, W. R. (1988). Precambrian Ophiolites of Arabia: Geologic Settings, U-Pn Geochronology, Pb-Isotope Characteristics, and Implications for continental Accretion. Precambrian Res. 38, 1–54. doi:10.1016/0301-9268(88)90092-7
Pérez-Gussinyé, M., Metois, M., Fernández, M., Vergés, J., Fullea, J., and Lowry, A. R. (2009). Effective Elastic Thickness of Africa and its Relationship to Other Proxies for Lithospheric Structure and Surface Tectonics. Earth Planet. Sci. Lett. 287, 152–167. doi:10.1016/j.epsl.2009.08.004
Philippon, M., Corti, G., Sani, F., Bonini, M., Balestrieri, M. L., Molin, P., et al. (2014). Evolution, Distribution and Characteristics of Rifting in Southern Ethiopia. Tectonics 33, 485–508. doi:10.1002/2013TC003430
Philippon, M., Willingshofer, E., Sokoutis, D., Corti, G., Sani, F., Bonini, M., et al. (2015). Slip Re-orientation in Oblique Rifts. Geology 43, 147–150. doi:10.1130/g36208.1
Phillips, T. B., Fazlikhani, H., Gawthorpe, R. L., Fossen, H., Jackson, C. A. L., Bell, R. E., et al. (2019). The Influence of Structural Inheritance and Multiphase Extension on Rift Development, the Northern North Sea. Tectonics 38 (12), 4099–4126. doi:10.1029/2019tc005756
Pizzi, A., Coltorti, M., Abebe, B., Disperati, L., Sacchi, G., and Salvini, R. (2006). “The Wonji Fault belt (Main Ethiopian Rift): Structural and Geomorphological Constraints and GPS Monitoring,” in The Afar Volcanic Province within the East African Rift System. Editors G. Yirgu, C. J. Ebinger, and P. K. H. Maguire (Geol. Soc. Spec. Publ.), 259, 191–207. doi:10.1144/gsl.sp.2006.259.01.16
Pongwapee, S., Morley, C. K., and Won-in, K. (2019). Impact of Pre-existing Fabrics and Multi-phase Oblique Extension on Cenozoic Fault Patterns, Wichianburi Sub-basin of the Phetchabun Rift, Thailand. J. Struct. Geology. 118, 340–361. doi:10.1016/j.jsg.2018.11.012
Purcell, P. G. (2017). Re-imagining and Re-imaging the Development of the East African Rift. Pet. Geosci. 24 (1), 21–40. doi:10.1144/petgeo2017-036
Ritsema, J., van Heijst, H. J., and Woodhouse, J. H. (1999). Complex Shear Wave Velocity Structure Imaged beneath Africa and Iceland. Science 286, 1925–1928. doi:10.1126/science.286.5446.1925
Rosendahl, B. L. (1987). Architecture of continental Rifts with Special Reference to East Africa. Annu. Rev. Earth Planet. Sci. 15, 445–503. doi:10.1146/annurev.ea.15.050187.002305
Samsu, A., Cruden, A. R., Molnar, N. E., and Weinberg, R. F. (2021). Inheritance of Penetrative Basement Anisotropies by Extension‐Oblique Faults: Insights from Analogue Experiments. Tectonics 40 (5), e2020TC006596. doi:10.1029/2020TC006596
Saria, E., Calais, E., Stamps, D. S., Delvaux, D., and Hartnady, C. J. H. (2014). Present-day Kinematics of the East African Rift. J. Geophys. Res. Solid Earth 119, 3584–3600. doi:10.1002/2013JB010901
Schori, M., Zwaan, F., Schreurs, G., and Mosar, J. (2021). Pre-existing Basement Faults Controlling Deformation in the Jura Mountains Fold-And-Thrust belt: Insights from Analogue Models. Tectonophysics 814, 228980. doi:10.1016/j.tecto.2021.228980
Schumacher, M. E. (2002). Upper Rhine Graben: Role of Preexisting Structures during Rift Evolution. Tectonics 21 (1), 61–617. doi:10.1029/2001TC900022
Smith, M., and Mosley, P. (1993). Crustal Heterogeneity and Basement Influence on the Development of the Kenya Rift, East Africa. Tectonics 12, 591–606. doi:10.1029/92TC01710
Sokoutis, D., Corti, G., Bonini, M., Brun, J. P., Cloetingh, S., Mauduit, T., et al. (2007). Modelling the Extension of Heterogeneous Hot Lithosphere. Tectonophysics 444 (1-4), 63–79. doi:10.1016/j.tecto.2007.08.012
Stern, R. J. (1994). Arc Assembly and continental Collision in the Neoproterozoic East African Orogen. Annu. Rev. Earth Planet. Sci. 22, 319–351. doi:10.1146/annurev.ea.22.050194.001535
Stern, R. J. (2002). Crustal Evolution in the East African Orogen: a Neodymium Isotopic Perspective. J. Afr. Earth Sci. 34, 109–117. doi:10.1016/s0899-5362(02)00012-x
Stern, R. J., Nielsen, K. C., Best, E., Sultan, M., Arvidson, R. E., and Kroner, A. (1990). Orientation of the Late Precambrian Sutures in the Arabian-Nubian Shield. Geology 18, 1103–1106. doi:10.1130/0091-7613(1990)018<1103:oolpsi>2.3.co;2
Tommasi, A., and Vauchez, A. (2001). Continental Rifting Parallel to Ancient Collisional Belts: an Effect of the Mechanical Anisotropy of the Lithospheric Mantle. Earth Planet. Sci. Lett. 185, 199–210. doi:10.1016/s0012-821x(00)00350-2
Vail, J. R. (1983). Pan-African Crustal Accretion in the North-East Africa. J. African Earth Sci. 1, 285–294.
Vauchez, A., Tommasi, A., and Barrruol, G. (1998). Rheological Heterogeneity, Mechanical Anisotropy and Deformation of the continental Lithosphere. Tectonophysics 296, 61–86. doi:10.1016/s0040-1951(98)00137-1
Vétel, W., and Le Gall, B. (2006). Dynamics of Prolonged continental Extension in Magmatic Rifts: the Turkana Rift Case Study (North Kenya). Geol. Soc. Lond. Spec. Publications 259, 209–233. doi:10.1144/gsl.sp.2006.259.01.17
Vétel, W., Le Gall, B., and Walsh, J. J. (2005). Geometry and Growth of an Inner Rift Fault Pattern: the Kino Sogo Fault Belt, Turkana Rift (North Kenya). J. Struct. Geol. 27, 2204–2222. doi:10.1016/j.jsg.2005.07.003
Wadge, G., Biggs, J., Lloyd, R., and Kendall, J. M. (2016). Historical Volcanism and the State of Stress in the East African Rift System. Front. Earth Sci. 4, 86. doi:10.3389/feart.2016.00086
Wang, L., Maestrelli, D., Corti, G., Zou, Y., and Shen, C. (2021). Normal Fault Reactivation during Multiphase Extension: Analogue Models and Application to the Turkana Depression, East Africa. Tectonophysics 811, 228870. doi:10.1016/j.tecto.2021.228870
Wedmore, L. N. J., Williams, J. N., Biggs, J., Fagereng, A., Mphepo, F., Dulanya, Z., et al. (2020). Structural Inheritance and Border Fault Reactivation during Active Early-Stage Rifting along the Thyolo Fault, Malawi. J. Struct. Geology. 139, 104097. doi:10.1016/j.jsg.2020.104097
Whipp, P. S., Jackson, C. A. L., Gawthorpe, R. L., Dreyer, T., and Quinn, D. (2014). Normal Fault Array Evolution above a Reactivated Rift Fabric; a Subsurface Example from the Northern Horda Platform, Norwegian North Sea. Basin Res. 26 (4), 523–549. doi:10.1111/bre.12050
Will, T. M., and Frimmel, H. E. (2017). Where Does a Continent Prefer to Break up? Some Lessons from the South Atlantic Margins. Gondwana Res. 53, 9–19. doi:10.1016/j.gr.2017.04.014
Williams, J. N., Fagereng, A., Wedmore, L. N., Biggs, J., Mphepo, F., Dulanya, Z., et al. (2019). How Do Variably Striking Faults Reactivate during Rifting? Insights from Southern Malawi. Geochem. Geophys. Geosystems 20 (7), 3588–3607. doi:10.1029/2019gc008219
WoldeGabriel, G., Aronson, J. L., and Walter, R. C. (1990). Geology, Geochronology, and Rift basin Development in the central Sector of the Main Ethiopia Rift. Geol. Soc. America Bull. 102, 439–458. doi:10.1130/0016-7606(1990)102<0439:ggarbd>2.3.co;2
Wolfenden, E., Ebinger, C., Yirgu, G., Deino, A., and Ayale, D. (2004). Evolution of the Northern Main Ethiopian Rift: Birth of a Triple junction. Earth Planet. Sci. Lett. 224, 213–228. doi:10.1016/j.epsl.2004.04.022
Wu, L., Mei, L., Paton, D. A., Liu, Y., Shen, C., Liu, Z., et al. (2020). Basement Structures Have Crucial Influence on Rift Development: Insights from the Jianghan Basin, Central China. Tectonics 39 (2). doi:10.1029/2019tc005671
Zwaan, F., Chenin, P., Erratt, D., Manatschal, G., and Scheurs, G. (2021a). Competition between 3D Structural Inheritance and Kinematics during Rifting: Insights from Analogue Models. Basin Res. 00, 1–31 doi:10.1111/bre.12642
Zwaan, F., Chenin, P., Erratt, D., Manatschal, G., and Schreurs, G. (2021b). Complex Rift Patterns, a Result of Interacting Crustal and Mantle Weaknesses, or Multiphase Rifting? Insights from Analogue Models. Solid Earth Discuss. 12 (7), 1473–1495. doi:10.5194/se-12-1473-2021
Keywords: continental rifting, extensional tectonics, East African Rift System (EARS), Main Ethiopian Rift (MER), pre-existing structures, tectonic inheritance
Citation: Corti G, Maestrelli D and Sani F (2022) Large-to Local-Scale Control of Pre-Existing Structures on Continental Rifting: Examples From the Main Ethiopian Rift, East Africa. Front. Earth Sci. 10:808503. doi: 10.3389/feart.2022.808503
Received: 03 November 2021; Accepted: 10 January 2022;
Published: 26 January 2022.
Edited by:
D. Sarah Stamps, Virginia Tech, United StatesReviewed by:
Ken McCaffrey, Durham University, United KingdomAntonio Pedrera, Instituto Geológico y Minero de España (IGME), Spain
Copyright © 2022 Corti, Maestrelli and Sani. This is an open-access article distributed under the terms of the Creative Commons Attribution License (CC BY). The use, distribution or reproduction in other forums is permitted, provided the original author(s) and the copyright owner(s) are credited and that the original publication in this journal is cited, in accordance with accepted academic practice. No use, distribution or reproduction is permitted which does not comply with these terms.
*Correspondence: Giacomo Corti, giacomo.corti@igg.cnr.it