- 1Dipartimento Scienze della Terra, Università di Pisa, Pisa, Italy
- 2Dipartimento Scienze, Università Roma Tre, Roma, Italy
- 3Dipartimento Scienze della Terra, Università di Firenze, Firenze, Italy
- 4Istituto Nazionale di Geofisica e Vulcanologia, Sezione di Napoli–Osservatorio Vesuviano, Napoli, Italy
- 5Istituto Nazionale di Geofisica e Vulcanologia, Sezione di Bologna, Bologna, Italy
- 6Istituto Nazionale di Geofisica e Vulcanologia, Sezione di Palermo, Palermo, Italy
A crucial feature to manage a volcanic crisis is the ability of volcanologists to promptly detect an impending eruption. This is often affected by significant uncertainty, mainly for the difficulty in interpreting the monitoring signals in terms of the exact timing of a possible eruption. Here we contribute to this problem, focusing on the states of active volcanoes with closed conduit, as deduced from monitoring data. Four main states can be identified. In the quiescence state 1) the monitoring data lie on a baseline, suggesting the lack of shallow magma/fluid movement. The unrest state is highlighted by minor 2) to major 3) variations in the intensity and rate of monitoring data; in both cases, radial ground deformation pattern and non-migrating seismicity imply shallow magma and/or fluid accumulation. The state of impending eruption 4) is characterised by non-radial, asymmetric ground deformation pattern and migrating seismicity, which suggest that magma approaches the surface through a propagating dyke. As early recognition of this distinctive state is crucial for timely eruption forecast, monitoring activity should be aimed at its prompt detection. The application of this rationale to two types of active volcanoes in densely inhabited areas, a restless caldera (Campi Flegrei) and a quiescent stratovolcano (Vesuvio), highlights its feasibility and importance in eruption forecasting. This rationale may foster a general reference framework to be adopted in case of unrest, supporting in interpreting the monitoring data, as well as more effective: 1) operationally-oriented, monitoring system; 2) probabilistic forecast; 3) use of volcanic alert levels.
Introduction
With the increase of global population, human settlements near active volcanoes have increased dramatically, and nowadays about 800 million people live within 100 km from a volcano that has the potential to erupt (Papale and Marzocchi, 2019). Also, due to its increasing complexity, modern society is becoming more and more exposed and vulnerable to volcanic phenomena, as demonstrated by the moderate 2010 Eyjafjallajokull (Iceland) eruption, which affected 25 countries, cancelling or delaying more than 100,000 flights with a direct cost to airlines estimated in billions of euros (Reichardt et al., 2017, and references therein). Yet, the last century has been relatively quiet in terms of volcanic activity, as only eruptions with VEI ≤ 6 have occurred (Simkin and Siebert, 2000). Indeed, larger eruptions (VEI > 6), which have a lower recurrence rate, have not been witnessed, although representing the most extreme and destructive natural events which may be generated by our planet, whose impact is commonly underestimated (Mason et al., 2004; Self, 2006; Rougier et al., 2018; Papale and Marzocchi, 2019).
A factor that contributes to underrating of volcanic risks is the common belief in the society that a volcanic eruption may be anticipated with modern monitoring techniques, conversely to other types of hazard, most notably earthquakes. In the last decades volcanology has made enormous progresses in widening the conceptual understanding of how volcanoes work, at the same time monitoring volcanoes with a wealth of sophisticated instruments and developing probabilistic methods for volcanic hazards assessment. However, despite these advances, our ability to forecast hazardous volcanic events is still limited. In addition to cases where forecasting has been timely and accurate and the operational management of the crisis effective, there are in fact many examples of total or partial failures, with an alert issued too late or not issued at all in about 80% of the unrest episodes from the last decades, depending on the volcano type, eruption size and monitoring network (Winson et al., 2014). This limited forecasting capability is confirmed by the recent tragedies that occurred at Bromo volcano (Indonesia) in 2004, Ontake volcano (Japan) in 2014, Fuego (Guatemala) in 2018, Anak Krakatau (Indonesia) in 2018 and White Island (New Zealand) in 2019 (Global Volcanism Program, Smithsonian Institution, 2013; OCHA Reliefweb, 2022). Moreover, cases like the Calbuco (Chile) in 2015 remind us that even for large explosive eruptions the pre-eruptive signals may be weak and detected only very shortly (a few hours) before, further complicating forecast efforts (Castruccio et al., 2016; Delgado et al., 2017). On the other hand, experiences of volcano emergencies occurred worldwide over the past decades have contributed to increase awareness of volcanologists and civil protection authorities about some critical issues: 1) in densely inhabited settlements exposed to hazards from long-dormant volcanoes people usually have low perception of risk, and decision-makers often demonstrate little familiarity to deal with volcanic risks; 2) forecast is accompanied by large uncertainties on when, where and how an eruption can occur; 3) crisis management requires preparing complex pre-crisis contingency plans for a range of likely event scenarios.
In this complicated framework, high-hazard volcanoes threatening metropolitan areas are particularly challenging for developing and testing the best scientific and operational strategies to mitigate risk (IAVCEI Task-group on Crises Protocols, 2016). In these areas, one of the major concerns is how to accomplish timely measures of risk mitigation (including evacuation), also minimizing the chances of both false and missed alarms. A false alarm implies enormous economic costs for measures like evacuation and maintenance of refugees, for the interruption of economic activities, as well as societal costs related to temporary or long-term reorganization of population at risk and the loss of credibility of scientists and civil authorities. On the other hand, a missed alarm may have catastrophic consequences in terms of human life loss. Therefore, during the timeline of a volcanic crisis, the “critical window” (that is the time when an eruption appears to be imminent and important land-use and response decisions must be made) should be defined (Newhall et al., 2021). Indeed, increasing the capability of timely and reliable forecasting volcanic phenomena is necessary for the scientific community to guide appropriate response during volcanic crisis. However, codified methodologies for reliable and reproducible forecast are still ahead of us, especially for closed-conduit volcanoes (Poland and Anderson, 2020). In fact, these volcanoes are commonly characterized by the lack of monitoring data related to past eruptive crisis, hindering to understand their behaviour before the eruption, a limitation which may not hold for well-known and frequently erupting open-conduit volcanoes (e.g., Ripepe et al., 2018). The best way to account for the uncertainties about if and when a volcano will erupt, and define the associated hazards, is to present probabilistic short-term forecast based on scientific evidence and involving the opinion of scientists (e.g., Sparks, 2003; Sparks and Aspinall, 2004; Bebbington et al., 2018; Poland and Anderson, 2020). The most commonly used methods are based on the definition of expected probability (and related uncertainty) along the nodes of event or logic trees (e.g., Newhall and Hoblitt, 2002; Marzocchi et al., 2004; Marzocchi et al., 2008).
Here we offer a theoretical frame based on the experience of researchers from both the academia and volcano observatories. The problem is first approached considering a general framework for the state of a closed conduit volcano, which has experienced several decades or centuries without erupting, and may be characterized by quiescence or unrest. The volcano state is defined considering the general processes of accumulation and transfer of magma (and related fluids), as suggested by the physical and chemical variations in the monitoring data. This allows proposing a framework whose feasibility and importance (discussed in Defining the State of a Volcano and Volcano States and Monitoring Activity) are tested at two different types of high-risk volcanoes: Campi Flegrei and Vesuvio (Worked Examples: Campi Flegrei and Vesuvio).
Defining the State of a Volcano
We first define terms and processes. The shallow plumbing system of a volcano is defined as the upper portion of a magma reservoir (shallower than approximately 5 km depth, although this depth may vary) and any intrusion it feeds (e.g., Burchardt, 2018), whose dynamics can be detected through monitoring. The term magma reservoir is here used in a broad sense, to identify a zone of magma storage, without implications of geometric configuration or melt distribution (e.g., Cashman and Giordano, 2014; Sparks and Cashman, 2017; Edmonds et al., 2019; Sparks et al., 2019). The term volcanic system here refers to the volcanic edifice, the plumbing system and magma reservoir(s), including any hydrothermal system. The dynamics of a volcanic system in closed conduit conditions (which is the focus of our work) may be associated with three main processes. 1) deep magma and fluids transfer, occurring from a deep source region to any part of the volcanic system, which may result also largely or completely unnoticed to monitoring systems. 2) shallow magma and fluids accumulation, characterized by either the repeated emplacement or continuous supply of magma or volatiles within the shallow plumbing system, usually detectable by a monitoring system. 3) shallow magma transfer, in which the magma rises from the zones of shallow accumulation towards the surface, also detectable through monitoring.
Volcanoes commonly spend most of their life in a state of quiescence, defined as a baseline during which none of the three processes described above occurs and is characterized by a nearly stationary state. During quiescence, ground deformation, seismicity, and degassing typically show limited variations around a baseline (Figure 1). Such a baseline represents a long-term (decades or more) state, which may be accompanied by different features at different volcanoes. For example, quiescence at stratovolcanoes may be characterized by no or limited seismicity, deformation and degassing. At restless calderas, the baseline is more difficult to define (Newhall and Dzurisin, 1988; Acocella et al., 2015). In fact, while restless calderas do not really experience a truly quiescent state, minor subsidence, sporadic seismicity and minor degassing activity (as currently observed at Askja, or at Aso in the decades before 2016, or at Campi Flegrei between 1984 and 2005) may still be considered as a baseline for quiescence. Despite any difficulty in defining a-priori a baseline for a given volcano, the point here is that the quiescence state should be interpreted as characterized by the lack of evidence for shallow magma and fluids accumulation or transfer.
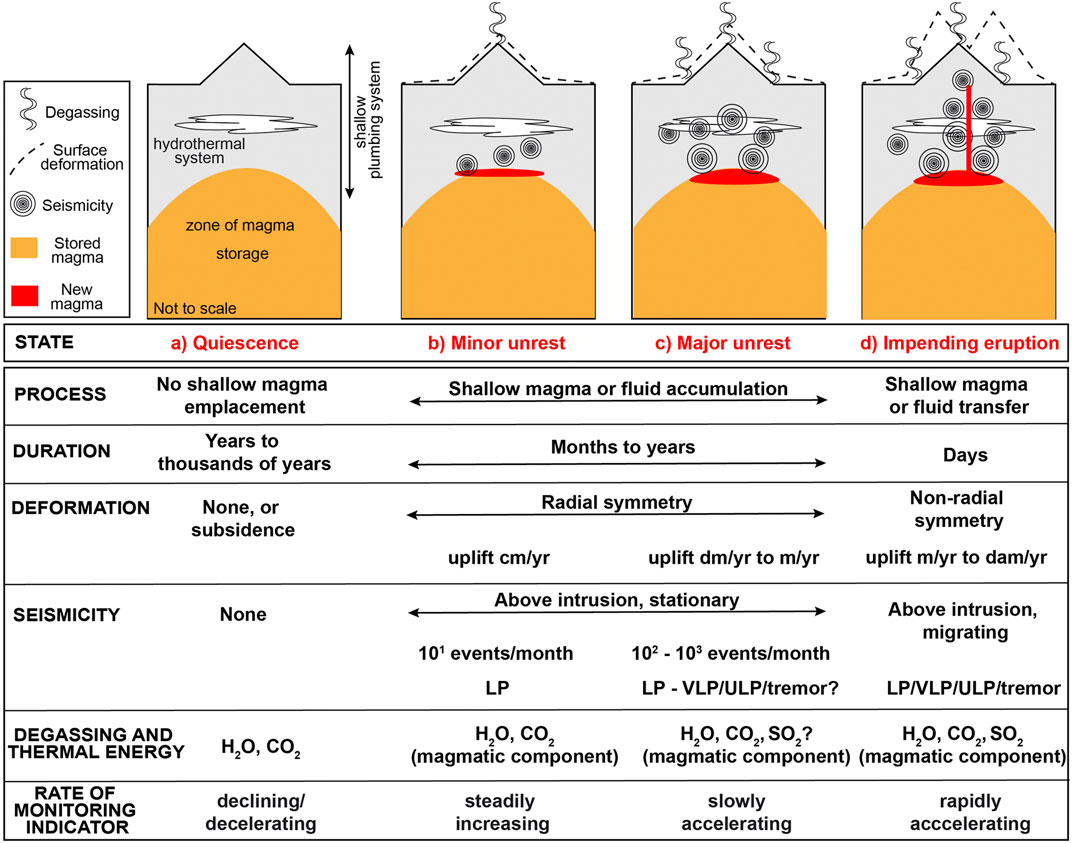
FIGURE 1. Summary of the four main states of a volcano, and associated features. New magma = newly emplaced magma responsible for unrest; stored magma = residual magma in the magmatic system, associated with the quiescent state of the volcano.
Defining the state of a volcano becomes particularly important during unrest, characterized by processes that cause a departure from the baseline of the monitored geophysical and geochemical parameters, resulting from magmatic, hydrologic, and/or tectonic processes (Newhall and Dzurisin, 1988). Magmatic processes involve migration of magma, volatiles and thermal energy within the shallow plumbing system. Hydrologic processes involve the dynamics of subsurface aquifers, or changing the physical or chemical characteristics of aquifer-hosted ground water by interaction with magmatic fluids. Tectonic processes occur in country rocks and dominantly involve changes in mechanical energy, possibly contributing to activate magmatic processes or to enhance hydrothermal activity, when and where far field stresses act as external forcing. The latter may result from short-term dynamic (transient passage of seismic waves) and/or long-term static (stress accumulation) processes. Probably, the anomalies signalling unrest are never purely magmatic, tectonic or hydrologic, as magmatic and tectonic changes invariably influence the ground water system and vice versa, and magma (if present) interacts with the local (tectonic) stress field or ground water system. Nevertheless, available studies highlight that, when determined, the cause of unrest is mainly attributed to the shallow emplacement and/or transfer of magma (Newhall and Dzurisin, 1988; Phillipson et al., 2013; Acocella et al., 2015; Edmonds and Wallace, 2017; Sparks and Cashman, 2017).
Whatever the triggering factors, unrest commonly reflects the emplacement and accumulation of magma and fluids within the shallow plumbing system. When magma is directly involved, this step usually precedes a possible, although not ubiquitous, unrest state characterized by magma migration towards the surface, which may feed eruption. Therefore, unrest does not necessarily culminate in eruption. Based on this, a state of unrest induced by shallow magma accumulation should be distinguished from a state that leads to the final magma rise to the surface. The exact recognition of an unrest state and nature becomes thus fundamental for forecasting an impending eruption. These different states of unrest may be in principle detected and distinguished through an appropriate monitoring system, as proposed below.
As for unrest due to magma and fluid accumulation, we distinguish two different states. A state of minor unrest can result from the slow (at low rates) accumulation of magma and magmatic fluids within a shallow reservoir. This condition occurred and has been monitored in many unrest episodes, especially at felsic calderas, as at Santorini in 2011 and Campi Flegrei in 2005–2021, when shallow magma emplaced (Parks et al., 2012; D’Auria et al., 2015; Chiodini et al., 2016; Chiodini et al., 2021). In some cases, unrest may mainly derive from direct transfer of magma-derived fluids within the hydrothermal system, as at Ontake in 2014 (Kato et al., 2015). Recognition of the nature of the unrest (magmatic and/or hydrothermal) is sometimes not straightforward and we suggest that, in case of ambiguous information, magma involvement should be assumed, especially at densely populated, high risk volcanoes. A state of minor unrest can persist for months to years. In this state, shallow magma intrusion and accumulation in sill-like bodies is expected to produce low-level seismicity, marked by minor swarms, minor degassing and minor amplitude and rate of ground deformation, which shows an overall radial symmetry from the zone of maximum deformation, with the surface deformation pattern approximated as axisymmetric; here the term “minor” can be quantified considering the order of magnitude of the parameters and the processes reported in Figure 1, with the monitoring parameter usually showing a steady increase.
We define a state of major unrest as due to faster and/or larger magma and fluid accumulation rate within the shallow plumbing system. The transition from minor to major unrest is marked by a slow acceleration (quantified considering the order of magnitude of the parameters and the processes reported in Figure 1) in intensity of all the different geophysical and geochemical parameters already recognized during minor unrest. The appearance of SO2 is possible but is also dependent on the scrubbing from shallow groundwater.
At closed conduit volcanoes the state of final magma rise is coincident with magma propagation towards the surface. This commonly occurs through the propagation of a dyke: a dyke may propagate vertically, feeding eruptions within the volcanic edifice, or horizontally, opening vents at distal locations, as observed along rift zones or within mafic volcanoes (e.g., Acocella and Neri, 2009; Sigmundsson et al., 2015). While there is widespread evidence that eruptions at closed conduit volcanoes are fed by dykes, in some cases, the original dyke configuration may be complicated by stress or thermal variations, reaching different final shapes (Acocella, 2021, and references therein). Shallow stresses due to the unbuttressing within a steep volcanic edifice may promote the growth of massive intrusions (cryptodomes) fed by the dyke, as postulated for example for Mount St. Helens in 1980. Thermal variations along a dyke may promote magma channelling in some portions and freezing in others, passing from a linear to punctiform distribution (i.e., cylindrical conduit; Costa et al., 2007) of magma towards the surface. Despite these possible complications, which typically take place at very shallow levels, it can be safely postulated that the rise of magma from a magma chamber in a volcano with closed conduit initiates through dykes that may progressively change their initial configuration at different depths or times. During its rise magma can undergo mechanisms other than through dyking, especially in volcanoes where the presence of a closed conduit may not be easily identified, In a few cases, magma may also rise to the surface through the propagation of inclined magmatic sheets (magma-filled fractures with medium to high dip) or of saucer-shaped sills (sills with inclined to subvertical lateral terminations).
This magma transfer at shallow levels defines the state of impending (or likely) eruption (Figure 1). Available observations indicate that dykes usually reach the surface within days, depending on magma overpressure and rheology, volatile content, and physical characteristics of the country rocks. However, the nucleation and propagation of a dyke do not necessarily guarantee that magma will reach the surface and erupt, as dykes may arrest at depth (Gudmundsson, 2002; Gardine et al., 2011; Moran et al., 2011; Taisne et al., 2011); therefore, the impending eruption state more appropriately represents a state of probable eruption marked by evidence of dyke nucleation and propagation. Propagation of upwards directed dykes results in a rapid acceleration of the surface deformation (Nishimura, 2006) and, most importantly, a change of the surface deformation pattern, passing from radial symmetry (with "bell-shaped" uplifted area) to non-radial symmetry (“directional” deformation), as the maximum vertical and horizontal deformation occurs off to the side of the dyke, and the minimum vertical and horizontal deformation occurs along the strike of the dyke (Figure 2; e.g., Lisowski, 2007). Although this deformation pattern is diagnostic to distinguish a propagating dyke towards the surface, in case of magma accumulation and contemporaneous magma transfer, both the radial and non-radial patterns may coexist. Magma movement towards the surface should also induce a rapid, progressive acceleration of seismicity and degassing and, expectedly, a migration and progressive shallowing of the hypocentres (Segall, 2013), with a strong increase in the number of LP signals associated with the onset of seismic tremor (Figure 1). Nevertheless, many eruptions have been preceded by a lack of shallowing seismicity (e.g., McNutt and Roman, 2015) and any degassing of more soluble magmatic species (SO2) could be scrubbed by aquifers. The real-time tracking of a nucleated dyke through monitoring data (mainly deformation and seismicity) may allow to define how the dyke propagation is progressing and where the dyke will reach the surface and feed an eruption or not. The state of impending eruption thus marks a fundamental change in a volcano, which passes from shallow magma and fluid accumulation to the transfer of magma towards the surface. In fact, while magma accumulation is often observed at volcanoes, this does not imply that the stored magma will rise to the surface and erupt in a short time. Conversely, if the accumulated magma starts migrating towards the surface, the chances of an eruption increase significantly and, due to the short times involved in the propagation of dykes, appropriate risk mitigation measures should be promptly taken. As from the risk mitigation perspective, the states of minor and major unrest differ from the state of impending eruption, shallow dyke propagation is the most important process to be timely detected, to anticipate a probable eruption. This condition represents a main challenge for volcano monitoring aimed at risk mitigation, as the appearance of the state of impending eruption is critical to the activation of the operational procedures needed to safeguard human lives and economic activities. However, due to the wide range of parameters controlling magma transfer (e.g., depth of the magma accumulation, host rock properties, magma composition, volatile content, rheology, buoyancy and eruptible volume), the duration of the state of impending eruption can vary from few hours to days/weeks. For this reason, monitoring should also be prepared to track, in almost real time, the progression of the process as well as to anticipate the site where a vent is expected to open. Even in the unlikely case of magma rising not through dykes we expect a change in the associated deformation and seismic patterns (with stronger lateral component, more localized uplift, variations on the hypocenters location) respect to those accompanying the deeper-originated unrest.
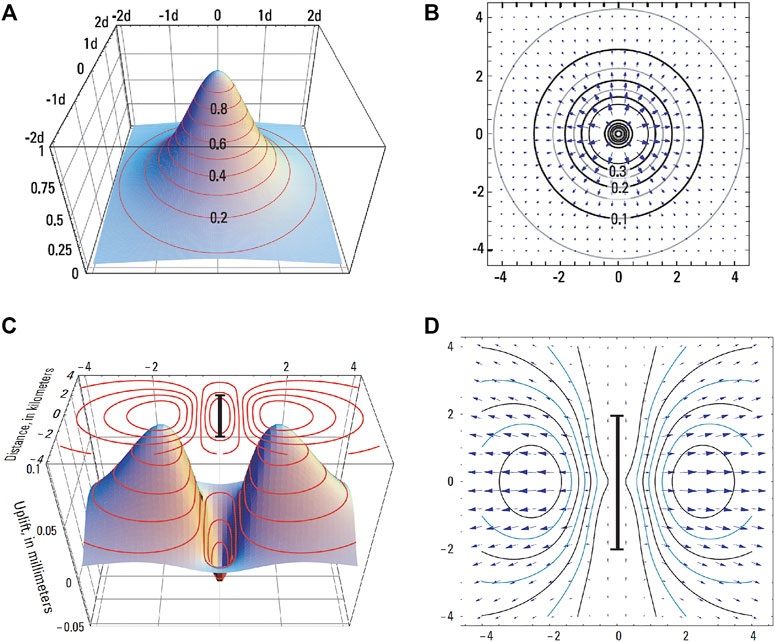
FIGURE 2. (A) and (B) Vertical (oblique view; left) and horizontal (map view; right) ground deformation pattern induced by a source with radial symmetry, responsible for magma accumulation; the source of deformation lies below the most uplifted area. (C) and (D) Vertical (oblique view; left) and horizontal (map view; right) ground deformation pattern induced by a source with non-radial symmetry (dyke), responsible for magma propagation; the source of deformation lies below the black bar (modified after Lisowski, 2007).
Provided that no major change occurs in the configuration of a monitoring system (a feature that may generate interpretative bias), the four states here identified (quiescence, minor unrest, major unrest, and impending eruption) may adequately describe the pre-eruptive conditions at a closed conduit volcano, and are specifically addressed to trace a scenario of magma accumulation or transfer in the volcano.
These states may occur in sequence, with transition from one to the other in days to months, up to years, or be non-sequential, with transitions that can take place even in very short time (days). Indeed, while a gradual and detectable in-sequence transition from one state to another is usually expected, the possibility of a rapid transition from quiescence to major unrest, or even from minor unrest to impending eruption (with any rapid transition through major unrest too short to be identified), cannot be discarded, as observed at a few active volcanoes (Castro and Dingwell, 2009; Johnson et al., 2010). This possibility also depends on the occurrence of previous prolonged/repeated unrest episodes at the same volcano. In fact, repeated unrest may lead to potential degradation of the mechanical properties of the host rocks, for example following prolonged increase in temperature or fluid circulation (e.g., Di Luccio et al., 2015; Vanorio and Kanitpanyacharoen, 2015; Chiodini et al., 2016), or to stress memory effects (Sigmundsson et al., 2018). In this context, a minor unrest at a mechanically and thermally weakened volcano may more easily progress toward a state of impending eruption.
An out-of-sequence transition of states may be also related to the possibility of a temporal release of magmatic fluids following an increase in the permeability in the host rock after a magma-induced pressure build up; this release would just temporarily relax the magmatic system, masking any further accumulation of magma (e.g., Moretti et al., 2020a). The sequential or non-sequential transition of the states observed at a volcano during unrest may also depend upon external triggering factors, as the occurrence of distal seismicity perturbing a magmatic system (e.g., Wang and Manga, 2010; White and McCausland, 2016).
Volcano States and Monitoring Activity
The ability of volcano observatories to detect variations in the states of the volcano depends on their capacity to efficiently collect, rapidly process and evaluate monitoring data and also to link monitor data to volcanic processes. One implication of our approach consists of providing a different perspective of how multi-parametric volcano monitoring may be carried out, especially with regard to impending eruption, based on the identification of a few important processes which necessarily accompany unrest at a volcano.
Although it is desirable to reach a holistic interpretation of all the different monitoring data to understand and forecast volcano behaviour (Poland and Anderson, 2020), not all the monitoring parameters should have the same weight at the same time to define the state of a closed-conduit volcano. To detect the rise and shallow accumulation of magma or volatiles fluxing from deeper levels which characterize the early stage of unrest, measures of extensive parameters of gas and fluids, surface deformation and seismicity data may play a relevant role. During and following magma/fluids recharge volatiles eventually migrate upward, mixing with meteoric fluids in hydrothermal systems. For this reason, especially in the early stage on unrest, geochemistry may play a primary role in the recognition of the nature of the unrest (i.e., magma vs fluids recharge) (Inguaggiato et al., 2018). Pressure increase due to shallow magma and fluids accumulation will result in surface inflation associated with radial symmetry. This process also causes dominant VT seismicity. During the impending eruption state, surface deformation and seismicity represent the most appropriate indicators to track dyke propagation and identify the location of the opening of a possible vent. In particular, a shift from a radial to a non-radial symmetry of the deformation pattern and any migration and acceleration of seismicity (if detected) should be considered evidence of the rise of the magma and fluids accumulated during unrest.
Because the propagation of dykes can be quick, in the impending eruption stage, it is essential that the monitoring system is capable to promptly detect, in real time, the signal of a non-radial deformation pattern and any migrating seismicity. To this aim, continuous, high-density, GPS and tiltmeter data appear, at present, the most suitable instruments set to provide a real-time tracking of vertical and horizontal surface deformation (Cannavò et al., 2015). These may be accompanied by continuous strainmeters, whose accuracy may allow an even earlier detection of propagating dykes and imminent vent opening, as for example witnessed immediately before the 2018 Etna eruption (Bonaccorso et al., 2020). Conversely, InSAR data, for the revisit time (a few days) of the satellites and the inability to fully capture the horizontal deformation (also lacking the N-S component) appear less effective to promptly detect and track dyke propagation, at least in the time window necessary for issuing an alert and taking the related emergency measures. These concepts are further discussed in Implications of the Approach, where the possibility to rely on a more operational monitoring to promptly detect the impending eruption phase is considered.
Worked Examples: Campi Flegrei and Vesuvio
As different closed conduit volcanoes may behave in different ways, the proposed rationale is now applied to two different types of high-risk volcanoes, Campi Flegrei and Vesuvio. In particular, Campi Flegrei provides the opportunity to apply our approach to a restless caldera with areal volcanism, characterized by the availability of monitoring data from several unrest episodes, whereas Vesuvio allows us to extend the approach also to a dormant, central stratovolcano for which no monitoring data on past phases of unrest are available (this depends also on the fact that no real unrest occurred after the last 1944 eruption, and activity preceding that eruption was practically continuous throughout about three centuries) and most of the information is obtained from long-term geological data. These two examples offer a wide perspective on the applicability of the proposed approach.
Here, we first focus on a general and simplified conceptual model for each volcano, which allows tailoring the above-mentioned states to the specific case (e.g., Newhall et al., 2021, and references therein). This conceptual model is based on appropriate (scientifically sound), simple (conservative), shared (accountable), encoded (clear), and useful (with an operational perspective) information: reaching this goal can be itself a main challenge, as scientists usually have different opinions on how a volcano works. The conceptual model takes into account for a few evidence-based, key elements widely accepted by most of the scientific community. These include volcanological, structural (geological-geophysical) and petrological-geochemical data, as well as information on the current state of the volcano derived from the monitoring system. To build these simplified conceptual models, we have evaluated all available literature related to Campi Flegrei and Vesuvio and possible analogues (i.e., volcanoes with similar behaviour), and openly discussed to find the combination of the key-elements that delineate a working reference model for each volcano, in terms of magma supply, shallow magmatic storage and mode of final magma rise to surface. In case of ambiguity, the most hazardous scenario (i.e., a magmatic one with regard to a hydrothermal one) should be taken into account in the model; this is also consistent with the fact that available evidence suggests that unrest at calderas usually has a magmatic origin, and this may eventually trigger increased hydrothermal activity.
The importance of the conceptual model is threefold: 1) it must result from a process of assimilation of data and knowledge from the widest scientific community, representing the best shared view on how the volcano is expected to work; 2) it represents an explicit framework to help deciphering the wealth of monitoring data during unrest and focusing scientists opinions, favouring also the adoption of probabilistic approaches (e.g., Elicitations, Bayesian Event Trees, Bayesian Belief Networks); 3) it can be used to establish a more effective operational approach and may be also of help in guiding a more effective monitoring strategy. During this step, we have interviewed several scientists, experts of each volcano and/or in unrest and crises management, whose list appears in the Acknowledgements Section. The interviews were aimed at openly sharing the views of those scientists about the creation of a conceptual model and asking advice on specific points, although the models here presented are only in charge of this Authors’ group. This approach allowed us to propose a conceptual model and to apply to Campi Flegrei and Vesuvio the previously-mentioned volcanic states approach. In order to more appropriately represent the many views of the scientific community, a workshop, advertised and open to the Italian volcanological community and sponsored by the Italian Civil Protection, was held to present the results and receive further feedback.
While we acknowledge that the adopted methodology is top-down and that not all scientists may identify their views with the proposed conceptual models, we believe that this approach minimizes any discrepancy considering the diversity of possible interpretations. We are aware that procedures to share scientific views may vary substantially across different cultures (IAVCEI Task Group on Crises Protocols, 2016), so while we are not assuming that our methodology can be adopted as it is elsewhere, we stress that the adoption of a shared and simplified conceptual model for a volcano would ease the communication among scientists and stakeholders during crises. We would also like to stress that, despite the adoption of a preferred conceptual model, any dogmatic use should be avoided and, wherever possible, during a volcanic crisis alternative conceptual models should be also considered (e.g., Newhall et al., 2021).
A Reference Model for Campi Flegrei Caldera
CFc is a volcanic system characterised by high heat flow, resurgence and fed by alkaline magmas (Figure 3). Starting from ∼15 ka (age of the most recent caldera-forming eruption) the caldera showed periods of quiescence, lasting millennia, alternated with three periods, lasting at least several centuries each, characterized by clusters of eruptions, named eruptive epochs. The development of a resurgence with circular symmetry accompanied the last 15 ka of activity of the caldera (Di Vito et al., 2016, and references therein). Within the caldera, there is a significant ascent of fluids of magmatic and thermo-metamorphic origin, currently focused in the Solfatara-Pisciarelli area (Figure 3; Chiodini et al., 2012, and references therein). Below the caldera, an upper layer focuses seismicity down to 3–4 km; at this depth active intrusions have been repeatedly emplaced in the last ∼5 ka at least (De Siena et al., 2010; Di Vito et al., 2016; De Siena et al., 2017). These intrusions can eventually represent the shallower portion of the magmatic system that, between ∼9 and ∼3.5 km of depth, traps and accumulates deeper magma (Amoruso et al., 2017). Magmatic pressure at ∼3.5 km of depth is considered the main process responsible of the recent uplift episodes within the central part of the caldera. In fact, since 1950 CFc has experienced four episodes of uplift (Figure 3), with two major episodes accompanied by seismicity in 1969–72 and 1982–84 (Figure 3), with a cumulated uplift of ∼3.6 m (Del Gaudio et al., 2010). From 2005, a new inflation has started, which, with increasing rates, is still ongoing (Figure 3; De Martino et al., 2021), with a total uplift of 0.89 m as of December 2021 (INGV/Osservatorio Vesuviano, 2021). This uplift has been accompanied by minor seismicity, increased fumarolic activity and compositional variations in the fumarolic emissions (Figure 3) (Chiodini et al., 2015; Tamburello et al., 2019; Tramelli et al., 2021).
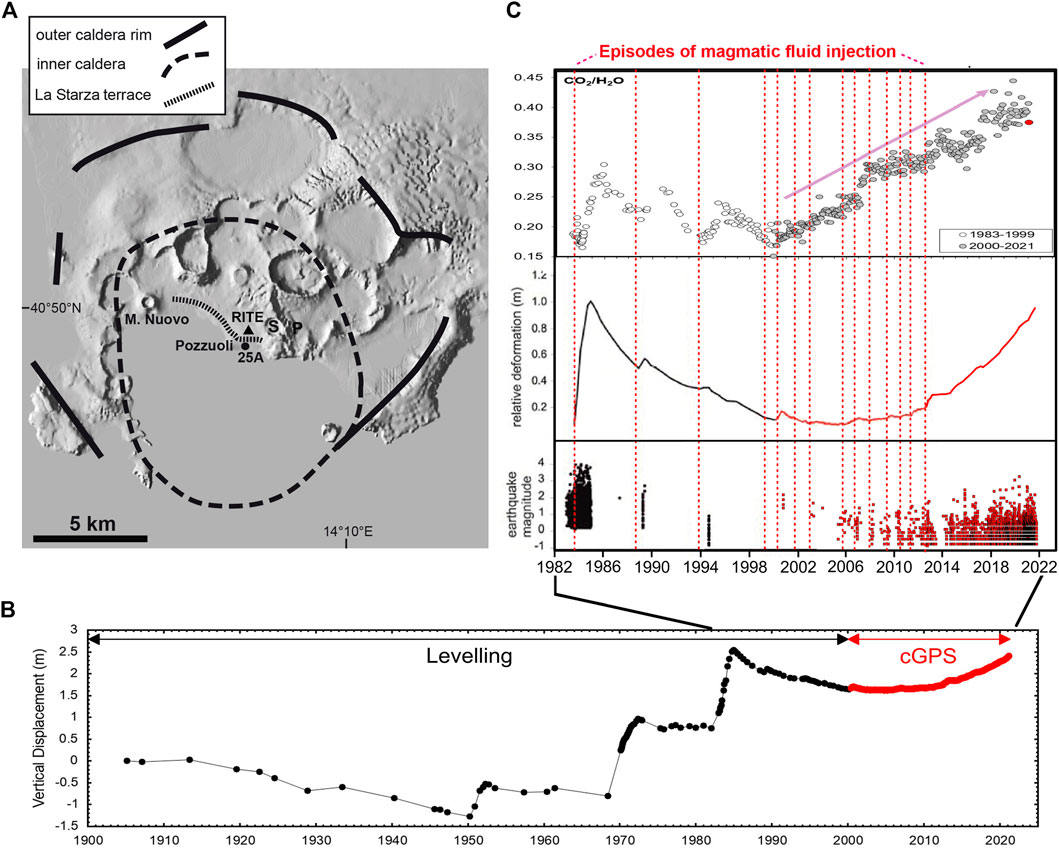
FIGURE 3. (A) Simplified structural map of Campi Flegrei caldera, Italy, including the location of the resurgent dome (highlighted by the La Starza marine terrace between Pozzuoli and Monte Nuovo). S indicates La Solfatara crater and P the Pisciarelli fumaroles, where the maximum fluid flux is currently detected; (B) Elevation changes of benchmark 25a (location in a) from 1905 to 1999 (after Del Gaudio et al., 2010) merged with more recent (2000–2020) vertical displacement at cGPS station RITE (location in a); (C) Measured fumarolic CO2/H2O ratio (top); ground deformation (centre) and earthquake magnitudes (bottom); dashed lines refer to times of injection of magmatic fluids into hydrothermal aquifer (after Chiodini et al., 2012).
A remarkable feature of the current and past (due to the longer-term resurgence) uplift episodes is the persistence of a deformation pattern with vertical and horizontal radial symmetry (bell-shaped in section view), with the same shape and areal extent, culminating in the caldera centre (town of Pozzuoli), implying a persistent central magmatic source active in the last few ka at depth between 3 and 4 km (Amoruso et al., 2014a; Amoruso et al., 2014b, Amoruso et al., 2017, and references therein; Di Vito et al., 2016). The recent history of CFc therefore demonstrates a restless behaviour resulting from magma accumulation, without necessarily producing eruptions. Volcanic centres from the last 15 ka show an inward migrating pattern inside the caldera and the probability of opening of a new vent is the highest along the on-land periphery of the resurgent area (Selva et al., 2012; Rivalta et al., 2019; Bevilacqua et al., 2020).
This conceptual model for Campi Flegrei stems from all the considerations on most hazardous scenario made in the previous section. We are aware that this model may not completely explain all the available evidence, and alternative models that have been proposed may be considered (Troise et al., 2019, and references therein; Moretti et al., 2020b). The latter models mostly focus on the predominant role played by the hydrothermal system, which would be the main responsible for the ongoing unrest phase.
The States for Campi Flegrei Caldera
The states of activity for CFc can be defined through geophysical (mainly ground deformation and seismicity) and geochemical (variations of composition and flux of degassing) monitoring data (Figure 4); the reported values of the monitoring parameters in Figure 4 suggest the most likely order(s) of magnitude for a parameter in each state, although a specific state may be accompanied by a different order of magnitude.
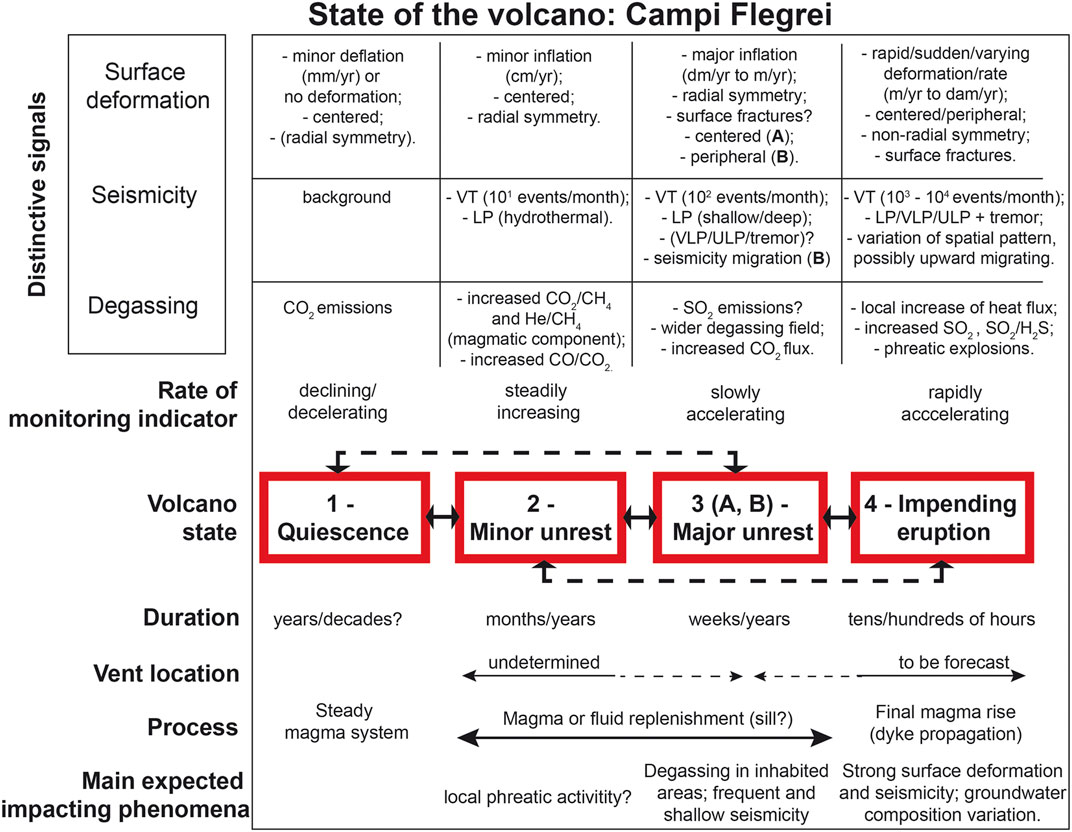
FIGURE 4. States proposed for Campi Flegrei caldera and related parameters. The reported values of the monitoring parameters suggest the most likely order(s) of magnitude for a parameter in each state, although a specific state may be accompanied by a different order of magnitude. The most likely rate associated with the monitoring parameters in each state (in terms of deceleration, steady increase, slow or rapid acceleration) is also suggested.
The definition of the quiescence state at the restless CFc is not straightforward. Here the continuous activity implies a physiological background unrest, making the definition of a state of quiescence not trivial. Probably in the past (pre-1950s), the caldera persisted in a state of quiescence, associated with an overall, steady subsidence (average 1–2 cm/yr). In the last decades, conditions most similar to a state of quiescence possibly occurred between the end of the 1982–1984 unrest and the onset of the current unrest in 2005. The 1985–2004 period was generally characterized by ground subsidence (on the order of several cm/yr, with radial symmetry centred on Pozzuoli), absence of seismicity, limited CO2 outgassing (with average emission <1,000 t/d in the Solfatara area) and a released energy of around 100 MW (Solfatara area; Chiodini et al., 2010). The combination of these parameters can be considered representative of a state of quiescence for CFc.
The current state (January 2022) of CFc can be considered as of minor unrest. The detected geochemical parameters proved valuable in defining the onset of unrest and in revealing the role of deep magma in the release of fluids, although the variations at depth of the monitored volcanic gases at the surface, such as CO2, are characterized by a delay of several months with respect to ground deformation and seismicity. This delay has been discussed in terms of the transit time of fluids from the magmatic reservoir to the surface (Chiodini et al., 2012). These features are associated with a slow, not constant, uplift of the caldera, with rates from cm/yr to approximately 15 cm/yr and radial symmetry, centred at Pozzuoli. A weak volcano-tectonic seismic activity (with frequency lower than tens of earthquakes/month with M > 0.8) accompanies this state of minor unrest. The pressurization of the hydrothermal fluids and caprock deformation may explain the ongoing minor unrest at CFc (Akande et al., 2021; Chiodini et al., 2021). As it stands, such a state is not expected to pose significant danger, except for the possibility of triggering local phreatic explosions due to the pressurization of the aquifer and/or hydrothermal system (especially in areas where the degassing is more intense, such as at Solfatara-Pisciarelli).
A state of major unrest at CFc would be characterized by a significant increase in the rates of ground deformation and seismicity. Expected ground uplift, still with radial symmetry centred at Pozzuoli (similar to Figure 2A), may exceed rates of the order of a few dm/yr. Because of such strong ground deformation, elasticity threshold could be locally overcome near the surface, producing surface fractures. Nearly continuous seismicity of volcano-tectonic origin (with frequency of occurrence of tens to hundreds of earthquakes/month with M > 0.8 and peaks of maximum magnitude with M > 4) is expected to accompany the uplift. Different seismic signals, such as LP (Long Period), VLP (Very Long Period), ULP (Ultra Long Period) and, possibly, volcanic tremor are also expected, together with a generalized increase of the fluxes and areas of gas emission. Very shallow earthquakes (<3 km), with high frequency of occurrence (tens of events/day) remain however likely to occur due to the increasing fracturing of the upper crust. In specific areas, these earthquakes could be accompanied by a generalized increase of gas flux, locally potentially harmful (e.g., Granieri et al., 2013). The probability of phreatic explosions, also favoured or triggered by seismic activity, should increase in areas affected by intense degassing. A state of major unrest was reached during the 1982–1984 crisis, when an uplift of ∼1.8 m was accompanied by high seismicity, increase in degassing (Del Gaudio et al., 2010) and surface fracturing (Orsi et al., 1999) at Solfatara.
The states of minor and major unrest described above reflect a process of shallow magma accumulation, with constant geometry of the ground deformation pattern and the spatial distribution of seismicity, concentrated in the caldera centre. On the basis of what may have occurred before the 1538 Monte Nuovo eruption (Di Vito et al., 2016), a state of major unrest could also show spatial variations of the ground deformation and seismicity patterns, produced by the lateral migration of the magma source (similar to Figure 2B). A lateral shift of the bell-shaped deformation pattern, which became non-centred on Pozzuoli, was observed in the 2 years preceding the Monte Nuovo eruption (Di Vito et al., 2016). Similarly, any future lateral migration of the area of maximum uplift could lead to an increased probability of vent opening.
As for the state of impending eruption, the propagation of a dyke at CFc should be accompanied by large and sudden variations in the geometry of the ground deformation pattern (from radial to non-radial), with a possible increase in the local (close to the vertical projection on the upper dyke tip) uplift rates up to values of m/yr. Surface deformation is expected above the propagating dyke, with an extent depending on the dyke depth, orientation, thickness, length and aspect ratio. This deformation should not necessarily focus in the central part of the caldera. The expected seismicity should be characterized by VT earthquakes (with frequency of occurrence higher than a thousand of events per month, with maximum expected magnitude M < 5), LP, VLP, and ULP, related to the fracturing of host rock due to dyke propagation, magma ascent and fluid movement, which should also be associated with volcanic tremor. Dyke propagation could also result in a progressive upward migration of the seismic source and the onset of hybrid signals, possibly coupled with fracture-induced permeability promoting transfer of hydrothermal fluids. The integration of seismic and ground deformation parameters would play a primary role in the early identification of magma transfer towards the surface and enabling the location of the most likely area of opening of vent(s). The duration of the state of impending eruption is expected to last from tens of hours to days/weeks. The shallowing of magma could induce local ground uplift (metres), opening of new fractures with local emission of large volumes of fluids, variation of water level in wells, increasing both the local average heat flux and the total energy release, and intense, frequent, and localized, destructive, shallow seismicity (<2 km), as well as phreatic eruptions.
The possible relationships between the described pre-eruptive states are shown through the continuous and discontinuous arrows of Figure 4. In general, we expect a progressive transition from one state to another, with the possibility of inversion of this progression in each state of unrest (double arrows with continuous lines). However, a discontinuous progression of events may occur, with a rapid transition from quiescence to major unrest, or even from minor unrest to impending eruption, as observed at Rabaul (Papua New Guinea) in the 80’s and ’90’s (Acocella et al., 2015, and references therein). In the last case, the intensification of fluid circulation, due to the increase of fractures and permeability and the heating of the rock matrix, could inhibit local seismicity or change the character of the waveforms (Chiodini et al., 2016; Zarin et al., 2016), thus shortening any state of major unrest to a duration that may not allow its operational recognition. Such a possibility imposes caution in the correct interpretation of events and their eventual concatenation.
Implications for Operational Monitoring of Campi Flegrei Caldera
The great concern posed by the Campi Flegrei emergency plan is the timely evacuation of ∼500,000 people currently possibly affected by pyroclastic density currents. This highlights the relevance of an effective operational monitoring system. The persistence of the bell-shaped, radially spreading ground deformation pattern since at least the last epoch of activity at CFc(Amoruso et al., 2017), is a crucial feature to consider in eruption forecasting. This provides explicit indication of shallow (<4 km depth) magma and gas accumulation. Conversely, the onset of deviation from such a consistent radial pattern (both vertical and horizontal), especially if associated with an increased deformation rate and significant change in the pattern of the vertical and horizontal component, would represent an indicator for the propagation of a dyke and thus the rise of magma, heralding an impending eruption. Tracking in real-time both the shape and rate of the surface deformation provides a fundamental indication of the dyke propagation and where a vent may open.
In Figure 5 the present continuous geodetic network (GPS and Tiltmeters) at CFc is superposed to the bell-shaped uplift centred on the caldera (a) and to the long-term probability map of vent opening (b; Selva et al., 2012; Bevilacqua et al., 2015; Bevilacqua et al., 2020). The present spatial distribution of GPS stations is satisfactory to test the invariance of the bell-shaped deformation pattern. However, there is a consistent margin of improvement in the capability of the geodetic network to better constrain the deformation in the sectors with highest probability of vent opening (Figure 5B). In particular, in the NE proximal sector, where there is the highest probability of vent opening (Selva et al., 2012; Bevilacqua et al., 2015; Rivalta et al., 2019), a larger number of GPS stations may allow tracking more accurately any final magma propagation to the surface, including the location of a future vent. Seismicity rate changes coupled with real-time tracking of hypocentres associated with dyke propagation can further improve the capability to track the rise of magma towards the surface (e.g., Segall, 2013).
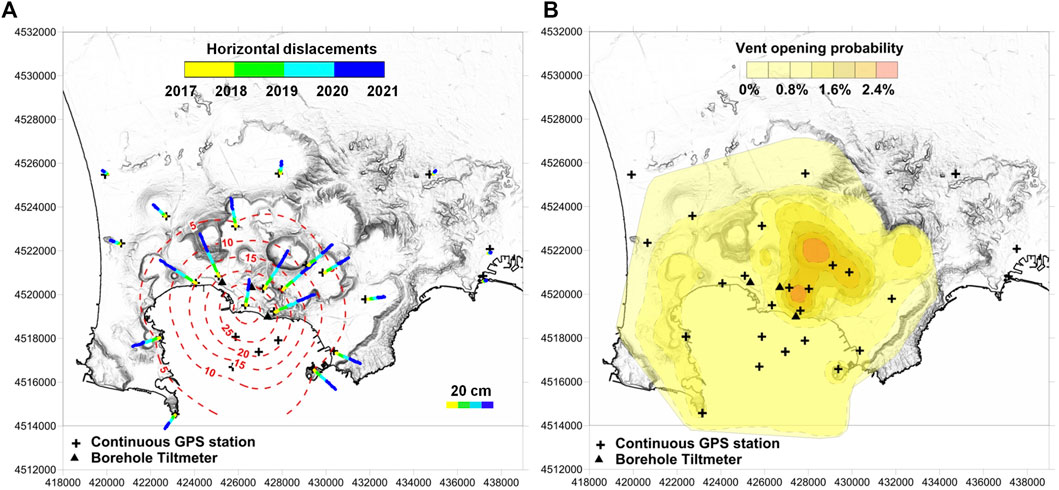
FIGURE 5. Comparison of the present continuous geodetic network (GPS and Tiltmeters) with the horizontal (coloured vectors) and vertical (red isolines) deformation patterns (A) and with the long-term probability of new vent opening at CFc (B). A higher density of stations in the area of highest vent-opening could better assist early detection of the possible location of future vent opening.
A Reference Model for States of Vesuvio
Vesuvio is an active stratovolcano which has been quiescent from ∼80 years, for which we lack unrest monitoring data. As this situation is common to many quiescent volcanoes, the proposed scheme may find a wider applicability.
Vesuvio is a central volcano with poly-phased summit caldera and minor parasitic cinder cones along the volcano flanks. Its dominant eruption style, after the first caldera collapse at 22 ka, has been explosive, with eruptions of variable intensity and magnitude (Cioni et al., 2008). The summit caldera results from at least four main episodes of collapse related to Plinian events (Cioni et al., 1999). After the famous last Plinian 79 AD eruption, the highest intensity events were subPlinian, dated at 472AD and 1631AD. The 1631AD eruption was followed by a semi-persistent activity that lasted until 1944, characterized by prolonged effusive periods accompanied by minor explosivity and occasionally interrupted by short-lived larger, violent-Strombolian eruptions. Since 1944 the volcano is quiescent. Based on petrological and geophysical evidence, its past plumbing system can be schematized as follows (De Natale et al., 2006; Santacroce et al., 2008; Scaillet et al., 2008; Pappalardo and Mastrolorenzo, 2010). 1) A sill-like reservoir, at 8–10 km depth, fed by periodic recharges of basic magma (Auger et al., 2001); there is no evidence for the present thermal state and composition of the magma within; 2) A smaller reservoir at 4–5 km depth, recharged from the deep reservoir, and feeding the main eruptions in the last 2000 years (Scaillet et al., 2008; Balcone Boissard et al., 2016; Cannatelli, 2020). There is no evidence of melt presently stored in this shallow region, as suggested by the seismicity focusing within a tomographically detected rigid body at 4 km depth (Scarpa et al., 2002, and references therein). Shallow seismicity occurs along the axis of the volcano and down to the limestone basement (around 1,500 m b.s.l.), where a high temperature hydrothermal system characterized by chloride rich brines is inferred (Caliro and Chiodini, 2011; Del Pezzo et al., 2013). This hydrothermal system interacts with the upwelling of deep-seated fluids, modifying their composition and flow rates to the surface.
Magma recharge could occur for one or both reservoirs, which may become then the source(s) of a next future eruption. The geologic record indicates that evolved magmas associated with the most intense eruptions originated directly from the deep reservoir (8–10 km, Scaillet et al., 2008). Moreover, variously evolved magmas, including the subPlinian eruptions of 472 and 1,631, and the more recent violent Strombolian activity, issued from the shallow reservoir, recharged by the arrival of variably evolved magma from the deeper reservoir (Lima et al., 2003; Bardeglinu et al., 2020).
Since the last 1944 eruption there has been no evidence of unrest, and the volcano has been in a quiescent state. Volcanological data highlight a positive correlation between the duration of the quiescence and the intensity and magnitude of the eruptions that start an eruptive period (Cioni et al., 2008), especially for the largest eruptions that were preceded by pluri-secular quiescence. During the last 4,000 years, quiescence periods with length similar to the present (several tens of years) were followed by eruptions of variable size, intensity and style, making it difficult to specify just one scenario for the next eruption on this basis (Marzocchi et al., 2004; Cioni et al., 2008).
The States at Vesuvio
At Vesuvio no unrest episodes have occurred in the last decades. For this reason, the states of the volcano can be defined only based on the above-mentioned reference model of the volcano and the historical information on the duration and type of precursory phenomena, as for the 1631 eruption (Bertagnini et al., 2006). The transition from one state to another implies a large uncertainty in the expected monitoring values.
The present state of the volcano is quiescence, characterized by the substantial lack of phenomena directly correlated to magma dynamics, as it has been occurring since the last 1944 eruption. Seismicity is on average low, with from ∼10 to ∼300 shallow events/month and strain release from ∼500 to ∼9000 GJ, with several episodes (few months long) characterized by higher values (Figure 6). Seismicity focuses in the upper crust (∼4 km) of the crater area with mean Magnitude from −1 to 2.8 in the last 5 years. In some cases, seismic activity correlates with an increase in the CO2 flux linked to the migration of deep fluids in the hydrothermal system of the central conduit (Del Pezzo et al., 2013). The entire edifice and particularly the summit caldera are characterized by continuous subsidence of ∼1 cm/yr (Figure 6; De Natale et al., 2006; Folco Pingue et al., 2013; Tammaro et al., 2013). The fumarolic activity is weak and confined at the summit of the cone, with diffuse degassing of ∼150 t/day of CO2 both magmatic and deriving from thermal decarbonation (Iacono Marziano et al., 2009; Granieri et al., 2013). Fumarole temperatures reach 100°C and their activity is declining since the last eruption (Figure 6). In the geological record of Vesuvio, the quiescence state could last from years to centuries.
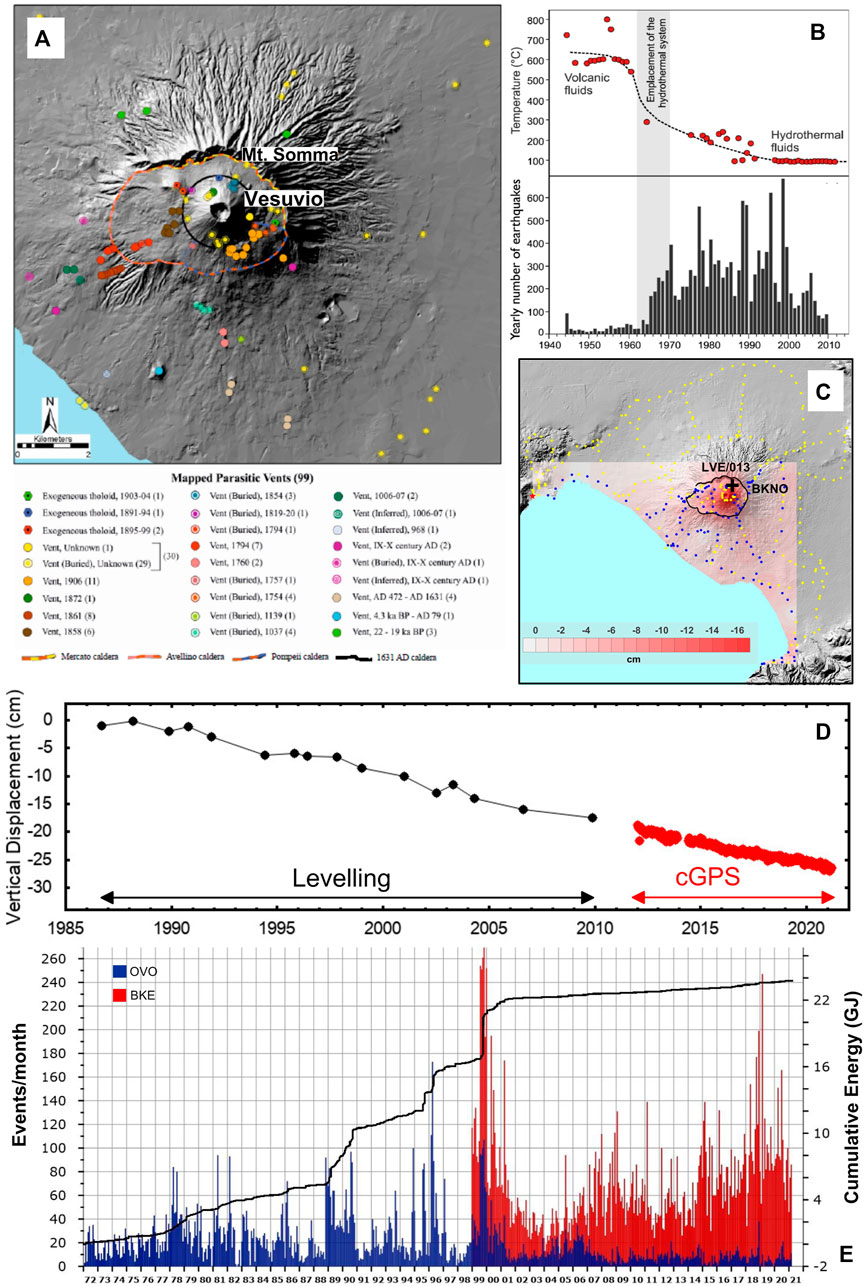
FIGURE 6. (A) Map of Somma-Vesuvio volcano, including the location of the parasitic vents (modified from Tadini et al., 2017). (B) Time behaviour of the fumaroles temperature and number of earthquakes per year since 1944 (modified from Del Pezzo et al., 2013). (C) Map of the vertical displacement field for the period 2000–2009. The levelling benchmarks common to the two surveys are shown in blue (modified from Folco Pingue et al., 2013). (D) Elevation changes of benchmark LVE/013 (location in c) from 1986 to 2010 (after Folco Pingue et al., 2013) merged with more recent (2012–2020) vertical displacement at the continuous GPS station BKNO (location in c). (E) Number of earthquakes per month since 1972 recorded at stations OVO (Vesuvian Observatory; about 2.5 km WNW from the crater) and BKE (located about 1 km ESE from the crater); note that: i) after 2006 the seismicity, being concentrated in the very shallow part of the crater area, has been characterized by low magnitude and cumulative energy released (continuous black line; referred to OVO station).
The state of minor unrest may correspond to the onset of magma accumulation in the deep reservoir, and/or magma accumulation at shallower levels, anticipating the development of an upper reservoir. In alternative a state of minor unrest could also be activated by transfer of deep fluids to the volcanic system. During minor unrest the volcano is expected to show limited variations in the monitoring parameters, such as small amplitude changes in seismicity, gas emissions and ground deformation (as an inversion or a decrease of the present subsidence trend). Given the possible magma dynamics in the two reservoirs, the deformation source during this state may be deep, superficial or both. A seismicity increase (from 5 to 8 km or below, and around 4 km) is expected (mainly VT events, with possible LP events occurrence), due to the pressurization and fracturing associated with magma transfer. Progressive increase of the hydrothermal activity triggered by magma-released fluids could be associated with the increase of CO2 emission, and variations of the composition, temperature and fluxes of the fumaroles, summit vents and soils, although these signals could be largely buffered by the hydrothermal system. A weak increase of pCO2 in cold and thermal waters located on the volcano flanks may also occur. The minor unrest state could last from months to years.
A state of major unrest is expected to be characterized by the continuous magma supply to the volcano plumbing system and the progressive transfer and accumulation of magma from deeper to shallower levels. Major unrest would imply strong variations in the pattern and/or rate of surface deformation depending on the depth at which magma accumulation is occurring. Continued magma accumulation can be associated with a significant increase in seismic activity due to the pressurization of the system. An increase in seismicity (VT and, possibly, LP, VLP, ULP events) in the seismogenetic volume located inside or immediately below the edifice could be also related to fluid migration and pressurization in the shallow aquifers. Increased variations in temperature, composition (e.g., increase in magmatic fluid components like the SO2, He, CO2), fluxes (from point or diffuse sources) and an enlargement of the degassing area are expected. Similar to past Vesuvio eruptions, strong variations of physical and compositional parameters (increase in temperature, variation of pCO2, etc.) could also occur in the groundwater, due to the continuous supply of magmatic fluids. The general pressurization of the system results in an intensification of the ground deformation rate, still preserving a general radial pattern. However, the maximum deformation area could migrate toward more peripheral portions of the edifice, as a precursor to the opening of distal vents. The expected increase of the degassing activity could also coincide with an increase in the probability of phreatic explosions at the summit. The transition to a state of major unrest could be fast (impulsive recharge of one or more reservoirs with sharp change in thermobaric conditions) or slow (volatiles in the previously identified reservoirs approaching over-saturation conditions). This state is expected to last from weeks to months. Defining the transition to the state of major unrest could be difficult if magma from a deep reservoir is directly transferred to the surface from a state of minor unrest (dashed arrow in Figure 7). A magma supply limited to the deep system, without feeding the shallow reservoir, during a state of minor unrest does not exclude a rapid evolution towards eruptive conditions, resulting in a very short (possibly not detectable) passage to a state of impending eruption (dashed arrow in Figure 7), characterized by rapid magma ascent from the deep reservoir feeding eruptive vents, possibly at the periphery of the edifice.
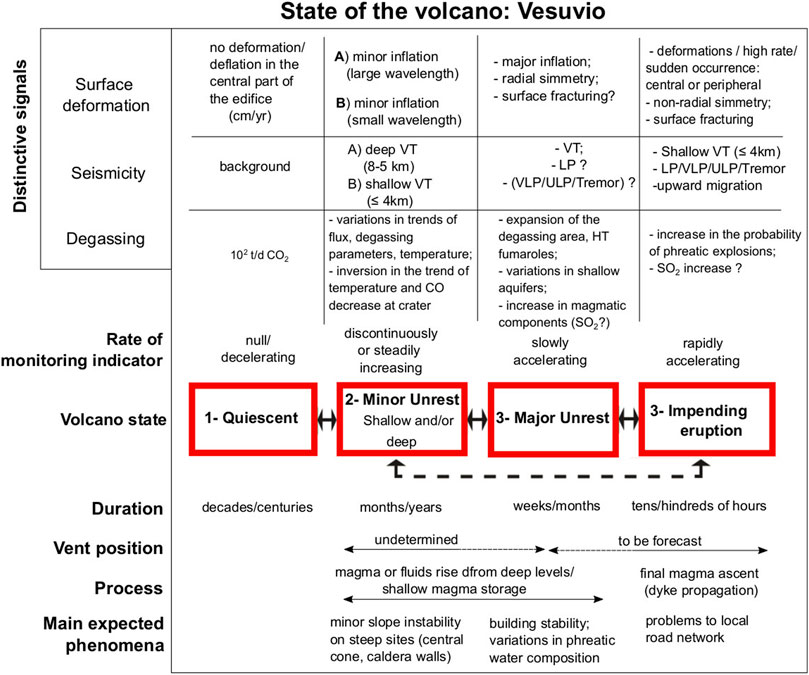
FIGURE 7. States proposed for Vesuvio and related parameters. The reported values of the monitoring parameters suggest the most likely order(s) of magnitude for a parameter in each state, although a specific state may be accompanied by a different order of magnitude. The most likely rate associated with the monitoring parameters in each state (in terms of deceleration, steady increase, slow or rapid acceleration) is also suggested.
The state of impending eruption should be marked by the onset of rapid magma ascent to the surface through dyke propagation from one of the magmatic reservoirs. This state involves sudden changes in the monitoring parameters. Significant variations in the deformation rates and patterns (from radial to non-radial symmetry) are expected, with maximum values within the volcanic edifice (probable magma ascent from the shallow reservoir) or in the periphery of the volcano (in the case of magma ascent from the deeper and wider reservoir). The strong ground deformation may lead to local surface fracturing, with an increase in landslide hazard along the caldera rims or the crater walls. In this state, the seismicity is expected to be characterized by shallow (<4 km depth) VT earthquakes, with progressive and rapid (tens of hours) upward migration of hypocentres. The frequency and intensity of the earthquakes might increase over time with the acceleration of the magma ascent. Long Period earthquakes (associated with fluid migration) and tremor are also expected. The degassing activity should increase both in areal distribution and mass output of volatiles and energy, with compositional parameters strongly marked by magmatic contribution (probable increase in SO2). Interaction of released fluids with the shallow aquifer system can lead to its rapid pressurization, triggering phreatic explosions. According to what was observed in past activity (Bertagnini et al., 2006), duration of this state can range from a few tens of hours to days/weeks before the eruption. The typical properties of Vesuvio magmas, with high volatile content and low viscosity, suggest that magma ascent towards the surface could be fast (few tens of hours?). In this case, it is possible that some of the phenomena described above (e.g., the geochemical parameters variations or the phreatic explosions) would not be detected.
Implications for Operational Monitoring of Vesuvio
Similar to CFc, the main concern posed by the Vesuvio emergency plan is the timely evacuation of ∼700,000 people currently living in the zone possibly affected by pyroclastic density currents. Considering the reference model and in particular that all the past Plinian and subPlinian events have been fed by vents within the Somma caldera, i.e. the area presently occupied by the Vesuvio cone, we expect that the next eruption will also occur within this area (Figure 8). Similarly to CFc and taking into account the specific characteristics of Vesuvio, we thus consider that the present multiparametric volcano monitoring be ideally complemented by a more operationally-oriented monitoring focused on the enhanced capacity of the system to promptly detect early evidence of magma rise to the surface (i.e., onset of a dyke from the one of the reservoirs and its vertical propagation) and forecast the site where the future vent may open. The early assessment of vent location is in fact of paramount importance for the PDC distribution (Neri et al., 2008), in relation to the present topography of the caldera (Vesuvio cone and Somma caldera wall Figure 6A). In order to efficiently record rapid changes in the ground deformation pattern, the configuration of the present continuous GPS and tiltmetric networks appears to be well designed, at least for the possibility of a vent opening within the caldera (Figure 8). A further implementation of the GPS network could be planned in response to unrest onset.
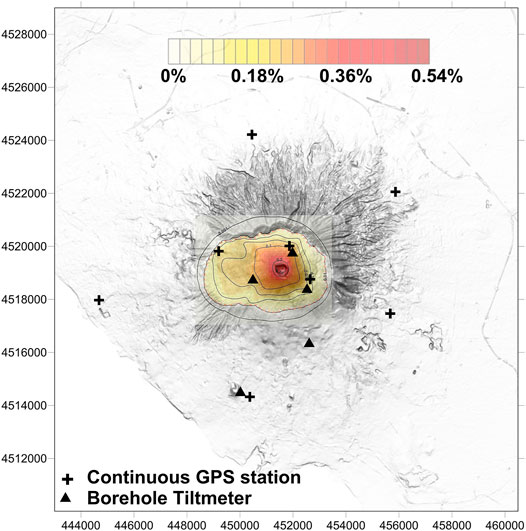
FIGURE 8. Comparison of the present continuous geodetic network (GPS and tiltmeters) with the long-term probability of new vent opening inside the caldera for Plinian and sub-Plinian eruptions for Vesuvio (redrawn from Tadini et al., 2017).
An interesting strategy may be to adopt an extensive deployment of sensors in borehole(s) at depth taking advantage of the fact that the expected volume focusing magma ascent is limited. Available data derived from a previous geothermal exploratory well down to ∼2000 m moreover indicate that the Somma caldera is characterized at depth by mild physico-chemical conditions, so that an instrument array including seismometers, tiltmeters, and volumetric strain meters could be easily deployed. Scientific deep boreholes have been so far successfully accomplished at Long Valley (United States), Unzen (Japan), Hawaii (United States) and Krafla (Iceland). Such an approach at Vesuvio could offer an important opportunity to have a higher sensitivity for signals related to any future magma rise, better supporting the operational management of the crisis.
Discussion
Strengths and Limits of Adopting a Reference Model
The transition between the different states of the volcano, grounded on variations of observables and/or monitoring parameters, cannot rely on the simple identification of pre-defined values or thresholds for the different parameters, as there is no default and unique value which defines a specific state of a volcano. In fact, also in the favourable case of available monitoring information related to previous crises, it may be dangerous to establish threshold values based on the limited past observations, as the physical state of the volcano could have undergone important changes. Therefore, the signals related to the different phenomena, or their temporal sequences and spatial variations, can be better characterized with regard to an expected range of values, which can also vary over an order of magnitude (more consistent with a fuzzy rather than Boolean logic). The development of a conceptual model for the volcano would facilitate a rational use of observational data derived from the identification of analogue volcanoes (Newhall et al., 2017; 2021; Tierz et al., 2019) for the interpretation of the expected monitoring signals and could be a very useful starting point also for any attempt to use a probabilistic approach (expert elicitation, BET or whatever) to eruption forecasting. Our approach, defining a conceptual model and the states of a volcano, applied to CFc (restless caldera) and Vesuvio (quiescent stratovolcano), may be extended to any other active monitored volcano, whose long-term (geological) and/or short-term (monitored) activity is known. In this case, the specific behaviour of the volcano on the long- and/or the short-term should be also taken into account, incorporating the related variations in the conceptual model. The aim of the conceptual model is to define a frame to better identify the states of a volcano, the modalities of passage from a state to another and in particular the crucial detection of the passage to the state of impending eruption.
Our approach includes a forecasting component, which aims at identifying and recognizing those signals that indicate the propagation of a dyke towards the surface, in order to identify the state of impending eruption. We propose that this top-down approach is particularly helpful when, during unrest, a wealth of different monitoring signals become available. This procedure may be more efficient than the bottom-up interpretation of a multitude of monitoring data, which could delay the fast detection of the critical information on dyke propagation. Indeed, the proposed conceptual model is meant to provide a sort of guideline to help promptly detecting and interpreting the most appropriate monitoring signals to forecast the passage to a state of impending eruption. One outcome of this procedure is that the classical multi-parametric monitoring networks should be complemented with monitoring specifically aimed at detecting the impending eruption state. The prompt evaluation of this state may finally respond to the necessity to identify the onset of the “critical window” during unrest (e.g., Newhall et al., 2021).
Implications of the Approach
The approach proposed in this study has three main implications, as discussed below.
A first, important implication is that any attempt to interpret changes in geophysical or geochemical parameters of a volcano should be done with an in-depth knowledge of that specific volcanic system, which should be finalised to the definition of a model of the possible expected behaviour of the volcano in its different states. The continuous (and fundamental) increase of technical capabilities to monitor volcanic activity has in some cases shifted into the background the efforts to improve our knowledge of the structure and behaviour of each volcanic system.
A second implication emphasizes the role of operational monitoring in relationships to the characteristics of the volcanic system and the processes we intend to identify. For high-risk volcanoes the timely recognition, during unrest, of the state of impending eruption is the crucial step of the proposed approach. For this reason, also following what mentioned in Section 3, it is suggested that, in addition to the ordinary type of monitoring that could be defined as “scientific-multiparametric”, a further type of monitoring, here defined as “operational”, should be considered. While the scientific-multiparametric monitoring has a clear intrinsic value for defining a volcanic system and providing indications for the definition of the different states of unrest, the operational monitoring should be focused on its ability to promptly identify the condition of impending eruption. Not all anomalies (geodetic, geophysical, and geochemical) may offer an equal contribution to the identification of the final magma migration towards the surface. Indeed, operational monitoring should emphasize the role of the surface deformation and seismicity data on the real-time detection of an impending eruption, as these indicators usually provide faster, stronger, clearer monitoring signals with a wider areal distribution. In particular, deformation data should mainly rely on significant number of continuous GPS complemented with tiltmeters and strainmeters, which allow real time detection of the non-radial vertical and/or horizontal deformation patterns related to dyke propagation. Seismicity data should be oriented at detecting, in real-time, any migration of seismicity and change in the frequency and type (especially the low-frequency associated with shallow magma and fluids) of the seismic events. This operational monitoring should be characterized by high density of survey, high quality of instruments, high robustness of the instrumental network, and reliability of real-time acquisition, transmission, elaboration and plotting of data. This monitoring network should also be shaped on the knowledge of the volcanic system (as included in the conceptual model and related information on the long-term probability of vent opening), to be ready to capture any significant change. To be effective, the collection of monitoring data should also be accompanied by an efficient, expert and quick elaboration and dissemination of the collected data.
So far, no standard signal analysis protocols exist worldwide for data interpretation and evaluation, and the accomplishment of shared evaluations and reliable forecasting transferable to the users through collegial inter-disciplinary data analysis can be slow. The difficulty of providing short-time rigorous and synthetic evaluations of monitoring data has been addressed through algorithm-based tools in which different parameters have been weighted via procedures of expert elicitation. Procedures based on Bayesian Event Trees (BET; Newhall and Hoblitt, 2002; Marzocchi et al., 2008; Sandri et al., 2009) represent an acknowledged, rapid and useful probabilistic forecast. We suggest that the herein proposed approach, in which the states of a volcano are defined in terms of expected changes in the magma dynamics on the basis of the conceptual model, could similarly represent an important contribution for those approaches aimed at establishing procedures of eruption forecasting, as BET_EF (Marzocchi et al., 2008). In particular, in the case of BET_EF, the above-mentioned forecasting component of our procedure may provide a more effective evaluation of the probabilities at the “eruption node”.
A third implication of our study concerns the use of Volcano Alert Levels (VAL). VAL were originally associated with the description of the state of the volcano, so that they could be used by authorities to decide on mitigation actions (Newhall et al., 1984; Winson et al., 2014, and references therein). However, the currently used VAL have not yet received a general international standardization or codification, and there are different applications and modulations of VAL in the scientific community (e.g., Fearnley et al., 2012; Winson et al., 2014). Indeed, more recently VAL have achieved a broader meaning, with implications also for the operational procedures to be adopted in response to the detected state of the volcano (e.g., Fearnley et al., 2012; Winson et al., 2014). In fact, in some cases the description of volcano status is mixed with operational indications/recommendations.1, which makes the use of VAL particularly delicate and potentially binding for volcanologists, so that it has been proposed that the scientific community should refrain from using VAL (Papale, 2017).
While we confirm the ambiguities and inconsistencies associated with the current VAL systems and share the perplexities in their use, we stress here that the definition of different states of unrest for a volcano (as for example discussed by Newhall et al., 1984), supported by a conceptual model of how that volcano works, may help in a more effective use of the VAL. In our approach, the states of a volcano are in fact related to different degrees of propensity to approach eruption (as suggested by Winson et al., 2014), as derived from the conceptual model for that volcano. We believe that such a state-supported VAL scheme, accompanied with a probabilistic approach (see Strengths and Limits of Adopting a Reference Model), should meet the requirements of “scientific credibility, acceptability, and relevance” so that those who receive such communication are effectively induced to adopt measures proportionate to the real needs. This approach could provide decision makers with a shared (accountable), encoded (clear), appropriate (scientifically sound), and useful scientific outcome, to be used as basis for operational decisions.
This study has focused on closed conduit volcanoes. Minor modifications may allow extending our approach also to open-conduit volcanoes, with the main difference that here a major unrest state is expected to be less common. Conversely, we do not foresee an easy application of our approach to phreatic eruptions. In fact, 1) the signals associated with the rise of the pressurized gases feeding the phreatic eruptions cannot be compared in quality and intensity to those associated with dykes (e.g., Kato et al., 2015); 2) the pressurized gases feeding the phreatic eruptions do not necessarily rise through dykes, making it more difficult to identify any impending eruption stage; 3) fluid pressurization in hydrothermal systems can reach critical conditions at times that can be completely out-of-sequence respect to the proposed progression of magmatic unrest states, with a non-negligible probability of occurrence of phreatic explosions also during the states of minor or major unrest.
Conclusion
Considering that residents on volcanoes seek predictive, practical, and reliable alerts including place, time, and magnitude directly linked to disaster mitigation activities it is of paramount importance to match such expectation in the most effective and transparent way. This study underlines the pivotal importance of evaluating the states of a volcano for risk mitigation. The quiescence state consists of the lack of anomalous shallow transfer of magma and fluids; the unrest state testifies a minor to major shallow accumulation of magma and fluids; the impending eruption state consists of magma transfer towards the surface. These states may be identified through a properly structured monitoring system. In particular, the impending eruption state may be distinguished by the unrest states on the appearance of distinctive signals, which may indeed acquire an operational status. These include non-radial surface deformation (best and earlier detected through continuous, high-density GPS and tiltmeter data) and migrating seismicity.
As magma during the impending eruption can rapidly move from a pressurized reservoir towards the surface, it is of pivotal importance that in densely inhabited areas the monitored data are processed in real time using shared protocols (merged with probabilistic forecasting approaches) to detect the appearance of any distinctive signal of this state and to track in real time magma migration, forecasting where a vent may open.
Our approach may also allow implementing existing early warning protocols, including a stronger state-supported use of the Volcano Alert Levels.
Data Availability Statement
The original contributions presented in the study are included in the article/Supplementary Material, further inquiries can be directed to the corresponding author.
Author Contributions
All authors listed have made a substantial, direct, and intellectual contribution to the work and approved it for publication.
Conflict of Interest
The authors declare that the research was conducted in the absence of any commercial or financial relationships that could be construed as a potential conflict of interest.
Publisher’s Note
All claims expressed in this article are solely those of the authors and do not necessarily represent those of their affiliated organizations, or those of the publisher, the editors, and the reviewers. Any product that may be evaluated in this article, or claim that may be made by its manufacturer, is not guaranteed or endorsed by the publisher.
Acknowledgments
This work would have not been possible without the initiative of the National Department of Civil Protection of Italy which requested INGV to appoint the national panel to better constrain the Volcanic Alert Levels of Vesuvio and Campi Flegrei; the panel was composed by all the Authors of this study, and was coordinated by BF and RM. AC also acknowledge the EU (DG ECHO) Project EVE (g.n. 82692). The Authors wish to primarily acknowledge all the experts who have generously end openly offered their contribution of analysis and evaluations during meetings, in particular Giovanni Chiodini, Luca Crescentini, Chris Kilburn, Matteo Lupi, Roberto Isaia, and Eleonora Rivalta. Franco Barberi and Chris Newhall are particularly acknowledged for putting at disposal of the panel their vast experience in the management of volcanic crisis, critically reviewing the document originally delivered to the Civil Protection. R.S.J. Sparks is warmly thanked for his frank, constructive criticism which helped us to increase the focus of our suggestions. The proposed ideas remain however of unique responsibility of the Authors. We greatly acknowledge the two referees, Chris Newhall and Roberto Moretti, and editor Luis Lara, who provided constructive and helpful comments, which improved the article.
Footnotes
1See for example the following institutional websites: https://www.vmgd.gov.vu/vmgd/index.php/geohazards/volcano/volcano-info/volcanic-alert-level, https://www.phivolcs.dost.gov.ph/index.php/volcano-hazard/volcano-alert-level, https://www.data.jma.go.jp/svd/vois/data/tokyo/STOCK/kaisetsu/English/level.html, https://www2.sgc.gov.co/Paginas/niveles-actividad-volcanes.aspx, https://twitter.com/Sernageomin/status/1079747342943903744.
References
Acocella, V., Di Lorenzo, R., Newhall, C., and Scandone, R. (2015). An Overview of Recent (1988 to 2014) Caldera Unrest: Knowledge and Perspectives. Rev. Geophys. 53, 896–955. doi:10.1002/2015RG000492
Acocella, V., and Neri, M. (2009). Dike Propagation in Volcanic Edifices: Overview and Possible Developments. Tectonophysics. 471, 67–77. doi:10.1016/j.tecto.2008.10.002
Akande, W. G., Gan, Q., Cornwell, D. G., and De Siena, L. (2021). Thermo‐Hydro‐Mechanical Model and Caprock Deformation Explain the Onset of an Ongoing Seismo‐Volcanic Unrest. J. Geophys. Res. Solid Earth. 126, e2020JB020449. doi:10.1029/2020JB020449
Amoruso, A., Crescentini, L., D’Antonio, M., and Acocella, V. (2017). Thermally-Assisted Magma Emplacement Explains Restless Calderas. Sci. Rep. 7, 7948. doi:10.1038/s41598-017-08638-y
Amoruso, A., Crescentini, L., and Sabbetta, I. (2014a). Paired Deformation Sources of the Campi Flegrei Caldera (Italy) Required by Recent (1980-2010) Deformation History. J. Geophys. Res. Solid Earth. 119, 858–879. doi:10.1002/2013JB010392
Amoruso, A., Crescentini, L., Sabbetta, I., De Martino, P., Obrizzo, F., and Tammaro, U. (2014b). Clues to the Cause of the 2011-2013 Campi Flegrei Caldera Unrest, Italy, from Continuous GPS Data. Geophys. Res. Lett. 41, 3081–3088. doi:10.1002/2014GL059539
Auger, E., Gasparini, P., Virieux, J., and Zollo, A. (2001). Seismic Evidence of an Extended Magmatic Sill under Mt. Vesuvius. Science. 294, 1510–1512. doi:10.1126/science.1064893
Balcone-Boissard, H., Boudon, G., Cioni, R., Webster, J. D., Zdanowicz, G., Orsi, G., et al. (2016). Chlorine as a Geobarometer for Alkaline Magmas: Evidence from a Systematic Study of the Eruptions of Mount Somma-Vesuvius. Sci. Rep. 6, 1–11. doi:10.1038/srep21726
Bardeglinu, I., Cioni, R., and Scaillet, B. (2020). Experimental Constraints on Pre-eruption Conditions of the 1631 Vesuvius Eruption. J. Volcanology Geothermal Res. 406, 107076. doi:10.1016/j.jvolgeores.2020.107076
Bebbington, M. S., Stirling, M. W., Cronin, S., Wang, T., and Jolly, G. (2018). National-level Long-Term Eruption Forecasts by Expert Elicitation. Bull. Volcanol. 80 (6), 56. doi:10.1007/s00445-018-1230-4
Bertagnini, A., Cioni, R., Guidoboni, E., Rosi, M., Neri, A., and Boschi, E. (2006). Eruption Early Warning at Vesuvius: The AD 1631 Lesson Geophys. Res. Lett. 33, L18317. doi:10.1029/2006gl027297
Bevilacqua, A., Isaia, R., Neri, A., Vitale, S., Aspinall, W. P., Bisson, M., et al. (2015). Quantifying Volcanic hazard at Campi Flegrei Caldera (Italy) with Uncertainty Assessment:1. Vent Opening Maps. J. Geophys. Res.120, 1–21. doi:10.1002/(ISSN)2169-9356
Bevilacqua, A., Neri, a., De Martino, P., Isaia, R., Novellino, A., D’Assisi Tramparulo, F., et al. (2020). Radial Interpolation of GPS and Leveling Data of Ground Deformation in a Resurgent Caldera: Application to Campi Flegrei (Italy). J. Geodesy. 94, 24.
Bonaccorso, A., Currenti, G., Linde, A., Sacks, S., and Sicali, A. (2020). Advances in Understanding Intrusive, Explosive and Effusive Processes as Revealed by the Borehole Dilatometer Network on Mt. Etna Volcano. Front. Earth Sci. 7, 357. doi:10.3389/feart.2019.00357
Burchardt, S. (2018). Volcanic and Igneous Plumbing Systems; Understanding Magma Transport, Storage, and Evolution in the Earth’s Crust. Elsevier, 356. doi:10.1016/b978-0-12-809749-6.00014-5
Caliro, S., and Chiodini, G. (2011). Long Time-Series of Chemical and Isotopic Compositionsof Vesuvius Fumaroles: Evidence for Deep and Shallow Processes. Ann. Geophys. 54, 137–149. doi:10.4401/ag-5034
Cannatelli, C. (2020). Tracing Magma Evolution at Vesuvius Volcano Using Melt Inclusions: A Review. Vesuvius, Campi Flegrei, and Campanian Volcanism. Editors B. De Vivo, H. E. Belkin, and G. Rolandi (Elsevier), 121–139. doi:10.1016/b978-0-12-816454-9.00006-7
Cannavò, F., Camacho, A. G., González, P. J., Mattia, M., Puglisi, G., and Fernández, J. (2015). Real Time Tracking of Magmatic Intrusions by Means of Ground Deformation Modeling during Volcanic Crises. Sci. Rep. 5, 10970. doi:10.1038/srep10970
Cashman, K. V., and Giordano, G. (2014). Calderas and Magma Reservoirs. J. Volcanology Geothermal Res. 288, 28–45. doi:10.1016/j.jvolgeores.2014.09.007
Castro, J. M., and Dingwell, D. B. (2009). Rapid Ascent of Rhyolitic Magma at Chaitén Volcano, Chile. Nature. 461, 780–783. doi:10.1038/nature08458
Castruccio, A., Clavero, J., Segura, A., Samaniego, P., Roche, O., Le Pennec, J.-L., et al. (2016). Eruptive Parameters and Dynamics of the April 2015 Sub-plinian Eruptions of Calbuco Volcano (Southern Chile). Bull. Volcanol. 78 (9), 62. doi:10.1007/s00445-016-1058-8
Chiodini, G., Caliro, S., Avino, R., Bini, G., Giudicepietro, F., De Cesare, W., et al. (2021). Hydrothermal Pressure-Temperature Control on CO2 Emissions and Seismicity at Campi Flegrei (Italy). J. Volcanology Geothermal Res. 414. doi:10.1016/j.jvolgeores.2021.107245
Chiodini, G., Caliro, S., Cardellini, C., Granieri, D., Avino, R., Baldini, A., et al. (2010). Long-term Variations of the Campi Flegrei, Italy, Volcanic System as Revealed by the Monitoring of Hydrothermal Activity. J. Geophys. Res. 115, B03205. doi:10.1029/2008JB006258
Chiodini, G., Caliro, S., De Martino, P., Avino, R., and Gherardi, F. (2012). Early Signals of New Volcanic Unrest at Campi Flegrei Caldera? Insights from Geochemical Data and Physical Simulations. Geology. 40, 943–946. doi:10.1130/g33251.1
Chiodini, G., JeanVandemeulebrouck, J., Vandemeulebrouck, J., Caliro, S., D'Auria, L., De Martino, P., et al. (2015). Evidence of thermal-driven Processes Triggering the 2005-2014 Unrest at Campi Flegrei Caldera. Earth Planet. Sci. Lett. 414, 58–67. doi:10.1016/j.epsl.2015.01.012
Chiodini, G., Paonita, A., Aiuppa, A., Costa, A., Caliro, S., De Martino, P., et al. (2016). Magmas Near the Critical Degassing Pressure Drive Volcanic Unrest Towards a Critical State. Nat. Commun. 7, 13712. doi:10.1038/ncomms13712
Cioni, R., Bertagnini, A., Santacroce, R., and Andronico, D. (2008). Explosive Activity and Eruption Scenarios at Somma-Vesuvius (Italy): towards a New Classification Scheme. J. Volcanology Geothermal Res. 178 (3), 331–346. doi:10.1016/j.jvolgeores.2008.04.024
Cioni, R., Santacroce, R., and Sbrana, A. (1999). Pyroclastic Deposits as a Guide for Reconstructing the Multi-Stage Evolution of the Somma-Vesuvius Caldera. Bull. Volcanology. 61 (4), 207–222. doi:10.1007/s004450050272
Costa, A., Melnik, O., and Sparks, R. S. J. (2007). Controls of Conduit Geometry and Wallrock Elasticity on Lava Dome Eruptions. Earth Planet. Sci. Lett. 260, 137–151. doi:10.1016/j.epsl.2007.05.024
D’Auria, L., Antonietta M. Esposito, A. M., and Domenico Lo Bascio, D. L. (2013). The Recent Seismicity of Mt. Vesuvius: Inference on Seismogenic Processes. Ann. Geophys. 56, 1–12. doi:10.4401/ag-6448
D’Auria, L., Pepe, S., Castaldo, R., Giudicepietro, F., Macedonio, G., Ricciolino, P., et al. (2015). Magma Injection beneath the Urban Area of Naples: a New Mechanism for the 2012-2013 Volcanic Unrest at Campi Flegrei Caldera. Sci. Rep. 5, 13100. doi:10.1038/srep13100
De Martino, P., Dolce, M., Brandi, G., Scarpato, G., and Tammaro, U. (2021). The Ground Deformation History of the Neapolitan Volcanic Area (Campi Flegrei Caldera, Somma-Vesuvius Volcano, and Ischia Island) from 20 Years of Continuous GPS Observations (2000-2019). Remote Sensing. 13 (14), 2725. doi:10.3390/rs13142725
De Siena, L., Chiodini, G., Vilardo, G., Del pezzo, E., Castellano, M., Colombelli, S., et al. (2017). Source and Dynamics of a Volcanic Caldera Unrest: Campi Flegrei, 1983-84. Sci. Rep. 7, 8099. doi:10.1038/s41598-017-08192-7
De Siena, L., Del Pezzo, E., and Bianco, F. (2010). Seismic Attenuation Imaging of Campi Flegrei: Evidence of Gas Reservoirs, Hydrothermal Basins, and Feeding Systems. J. Geophys. Res. 115, B09312. doi:10.1029/2009JB006938
Del Gaudio, C., Aquino, I., Ricciardi, G. P., Ricco, C., and Scandone, R. (2010). Unrest Episodes at Campi Flegrei: A Reconstruction of Vertical Ground Movements during 1905-2009. J. Volcanology Geothermal Res. 195, 48–56. doi:10.1016/j.jvolgeores.2010.05.014
Del Pezzo, E., Chiodini, G., and Caliro, S. (2013). New Insights into Mt. Vesuvius Hydrothermal System and its Dynamic Based on a Critical Review of Seismic Tomography and Geochemical Features. Ann. Geophys. 56, 137–149. doi:10.4401/ag-6450
Delgado, F., Pritchard, M. E., Ebmeier, S., González, P., and Lara, L. (2017). Recent Unrest (2002-2015) Imaged by Space Geodesy at the Highest Risk Chilean Volcanoes: Villarrica, Llaima, and Calbuco (Southern Andes). J. Volcanology Geothermal Res. 344, 270–288. doi:10.1016/j.jvolgeores.2017.05.020
Denatale, G., Troise, C., Pingue, F., Mastrolorenzo, G., and Pappalardo, L. (2006). The Somma-Vesuvius Volcano (Southern Italy): Structure, Dynamics and hazard Evaluation. Earth-Science Rev. 74, 73–111. doi:10.1016/j.earscirev.2005.08.001
Di Luccio, F., Pino, N. A., Piscini, A., and Ventura, G. (2015). Significance of the 1982-2014 Campi Flegrei Seismicity: Preexisting Structures, Hydrothermal Processes, and hazard Assessment. Geophys. Res. Lett. 42 (18), 7498–7506. doi:10.1002/2015gl064962
Di Vito, M. A., Acocella, V., Aiello, G., Barra, D., Battaglia, M., Carandente, A., et al. (2016). Magma Transfer at Campi Flegrei Caldera (Italy) before the 1538 AD Eruption. Sci. Rep. 6, 32245. doi:10.1038/srep32245
Edmonds, M., Cashman, K. V., Holness, M., and Jackson, M. (2019). Architecture and Dynamics of Magma Reservoirs. Phil. Trans. R. Soc. A. 377, 20180298. doi:10.1098/rsta.2018.0298
Edmonds, M., and Wallace, P. J. (2017). Volatiles and Exsolved Vapor in Volcanic Systems. Elements. 13, 29–34. doi:10.2113/gselements.13.1.29
Fearnley, C. J., McGuire, W. J., Davies, G., and Twigg, J. (2012). Standardisation of the USGS Volcano Alert Level System (VALS): Analysis and Ramifications. Bull. Volcanol. 74, 2023–2036. doi:10.1007/s00445-012-0645-6
Folco Pingue, F., Milena Bottiglieri, M., Cataldo Godano, C., Francesco Obrizzo, F., Umberto Tammaro, U., Teodoro Esposito, T., et al. (2013). Spatial and Temporal Distribution of Vertical Ground Movements at Mt. Vesuvius in the Period 1973-2009. Ann. Geophys. 56, S0451. doi:10.4401/ag-6457
Gardine, M., West, M., Werner, C., and Doukas, M. (2011). Evidence of Magma Intrusion at Fourpeaked Volcano, Alaska in 2006-2007 from a Rapid-Response Seismic Network and Volcanic Gases. J. Volcanology Geothermal Res. 200, 192–200. doi:10.1016/j.jvolgeores.2010.11.018
Global Volcanism Program, Smithsonian Institution (2013). Global Volcanism Program, Smithsonian Institution. Available at: https://volcano.si.edu/.
Granieri, D., Carapezza, M. L., Avino, R., Caliro, S., Cardellini, C., Chiodini, G., et al. (2013). Level of Carbon Dioxide Diffuse Degassing from the Ground of Vesuvio: Comparison between Extensive Surveys and Inferences on the Gas Source. Ann. Geophys. 56 (4), 0449. doi:10.4401/ag-6455
Gudmundsson, A. (2002). Emplacement and Arrest of Sheets and Dykes in central Volcanoes. J. Volcanology Geothermal Res. 116, 279–298. doi:10.1016/s0377-0273(02)00226-3
Iacono-Marziano, G., Gaillard, F., Scaillet, B., Pichavant, M., and Chiodini, G. (2009). Role of Non-mantle CO2 in the Dynamics of Volcano Degassing: The Mount Vesuvius Example. Geol 37, 319–322. doi:10.1130/G25446A.1
IAVCEI Task Group on Crisis Protocols (2016). Toward IAVCEI Guidelines on the Roles and Responsibilities of Scientists Involved in Volcanic hazard Evaluation, Risk Mitigation, and Crisis Response. Bull. Volcanology. 78, 1–3.
Inguaggiato, S., Diliberto, I. S., Federico, C., Paonita, A., and Vita, F. (2018). Review of the Evolution of Geochemical Monitoring, Networks and Methodologies Applied to the Volcanoes of the Aeolian Arc (Italy). Earth-Science Rev. 176, 241–276. doi:10.1016/j.earscirev.2017.09.006
INGV/Osservatorio Vesuviano (2021). Bollettino di Sorveglianza Osservatorio Vesuviano. Campi Flegrei. Napoli, Dicembre 2021. Available at: https://www.ov.ingv.it/index.php/bollettini/bollettini-di-monitoraggio/bollettini/mensili-dei-vulcani-della-campania/campi-flegrei/anno-2021-2/1033-bollettino-mensile-campi-flegrei-2021-12/file.
Johnson, J. H., Prejean, S., Savage, M. K., and Townend, J. (2010). Anisotropy, Repeating Earthquakes, and Seismicity Associated with the 2008 Eruption of Okmok Volcano, Alaska. J. Geophys. Res. 115, B00B04. doi:10.1029/2009JB006991
Kato, A., Terakawa, T., Yamanaka, Y., Maeda, Y., Horikawa, S., Matsuhiro, K., et al. (2015). Preparatory and Precursory Processes Leading up to the 2014 Phreatic Eruption of Mount Ontake, Japan. Earth Planet. Sp. 67, 111. doi:10.1186/s40623-015-0288-x
Lanari, R., De Natale, G., Berardino, P., Sansosti, E., Ricciardi, G. P., Borgstrom, S., et al. (2002). Evidence for a peculiar Style of Ground Deformation Inferred at Vesuvius Volcano. Geophys. Res. Lett. 29 (9), 6–1. doi:10.1029/2001gl014571
Lima, A., Danyushevsky, L. V., De Vivo, B., and Fedele, L. (2003). A Model for the Evolution of the Mt. Somma-Vesuvius Magmatic System Based on Fluid and Melt Inclusion Investigations. Dev. Volcanology. 5, 227–249. doi:10.1016/s1871-644x(03)80032-3
Lisowski, M. (2007). “Analytical Volcano Deformation Source Models,” in Volcano Deformation Geodetic Monitoring Techniques. Editor D. D. Dzurisin (Springer–Praxis Books in Geophysical Sciences), 279–304. doi:10.1007/978-3-540-49302-0_8
Lupi, M., Frehner, M., Weis, P., Skelton, A., Saenger, E. H., Tisato, N., et al. (2017). Regional Earthquakes Followed by Delayed Ground Uplifts at Campi Flegrei Caldera, Italy: Arguments for a Causal Link. Earth Planet. Sci. Lett. 474, 436–446. doi:10.1016/j.epsl.2017.07.006
Marzocchi, W., Sandri, L., Gasparini, P., Newhall, C., and Boschi, E. (2004). Quantifying Probabilities of Volcanic Events: the Example of Volcanic hazard at Mount Vesuvius. J. Geophys. Res. Solid Earth. 109 (B11), B11201. doi:10.1029/2004jb003155
Marzocchi, W., Sandri, L., and Selva, J. (2008). BET_EF: a Probabilistic Tool for Long- and Short-Term Eruption Forecasting. Bull. Volcanol. 70 (5), 623–632. doi:10.1007/s00445-007-0157-y
Marzocchi, W., and Woo, G. (2009). Principles of Volcanic Risk Metrics: Theory and the Case Study of Mount Vesuvius and Campi Flegrei, Italy. J. Geophys. Res. 114, B03213. doi:10.1029/2008JB005908
Mason, B. G., Pyle, D. M., and Oppenheimer, C. (2004). The Size and Frequency of the Largest Explosive Eruptions on Earth. Bull. Volcanol. 66, 735–748. doi:10.1093/petrology/26.3.726
McNutt, S. R., and Roman, D. C. (2015). “Volcanic Seismicity,” in The Encyclopedia of Volcanoes. Editors H. Sigurdsson, B Houghton, S. McNutt, H. Rymer, and J. Stix. 2nd edition (Elsevier Academic Press), 1011–1034. doi:10.1016/b978-0-12-385938-9.00059-6
Moran, S. C., Newhall, C., and Roman, D. C. (2011). Failed Magmatic Eruptions: Late-Stage Cessation of Magma Ascent. Bull. Volcanol. 73, 115–122. doi:10.1007/s00445-010-0444-x
Moretti, R., Komorowski, J.-C., Ucciani, G., Moune, S., Jessop, D., de Chabalier, J.-B., et al. (2020a). The 2018 Unrest Phase at La Soufrière of Guadeloupe (French West Indies) Andesitic Volcano: Scrutiny of a Failed but Prodromal Phreatic Eruption. J. Volcanology Geothermal Res. 393, 106769. doi:10.1016/j.jvolgeores.2020.106769
Moretti, R., Natale, G. D., and Troise, C. (2020b). Hydrothermal Versus Magmatic: Geochemical Views and Clues into the Unrest Dilemma at Campi Flegrei. Vesuvius, Campi Flegrei, and Campanian Volcanism.Editors B. De Vivo, H. E. Belkin, and G. Rolandi (Elsevier), 371–406. doi:10.1016/b978-0-12-816454-9.00014-6
Neri, A., Aspinall, W. P., Cioni, R., Bertagnini, A., Baxter, P. J., Zuccaro, G., et al. (2008). Developing an Event Tree for Probabilistic hazard and Risk Assessment at Vesuvius. J. volcanology geothermal Res. 178 (3), 397–415. doi:10.1016/j.jvolgeores.2008.05.014
Newhall, C. G., Costa, F., Ratdomopurbo, A., Venezky, D. Y., Widiwijayanti, C., Win, N. T. Z., et al. (2017). WOVOdat - an Online, Growing Library of Worldwide Volcanic Unrest. J. Volcanology Geothermal Res. 345, 184–199. doi:10.1016/j.jvolgeores.2017.08.003
Newhall, C. G., and Dzurisin, D. D. (1988). Historical Unrest at Large Calderas of the World. Washington: US Geological Survey Professional Paper, 1109.
Newhall, C. G., Pallister, J. S., and Miller, C. D. (2021). “A Checklist for Crisis Operations within Volcano Observatories,” in Forecasting and Planning for Volcanic Hazards, Risks, and Disasters. Editor P. Papale, 493–544. doi:10.1016/B978-0-12-818082-2.00013-5
Newhall, C. G. (1984). Semi-quantitative Assessment of Changing Volcanic Risk at Mount St. Helens. Washington (No. 84-272): US Geological Survey.
Newhall, C., and Hoblitt, R. (2002). Constructing Event Trees for Volcanic Crises. Bull. Volcanol. 64, 3–20. doi:10.1007/s004450100173
Nishimura, T. (2006). Ground Deformation Due to Magma Ascent with and without Degassing. Geophys. Res. Lett. 33 (23), L23309. doi:10.1029/2006gl028101
Nostro, C., Stein, R. S., Cocco, M., Belardinelli, M. E., and Marzocchi, W. (1998). Two-way Coupling between Vesuvius Eruptions and Southern Apennine Earthquakes, Italy, by Elastic Stress Transfer. J. Geophys. Res. 103, 24487–24504. doi:10.1029/98jb00902
OCHA Reliefweb (2022). Available at: htttps://reliefweb.int
Orsi, G., Civetta, L., Del Gaudio, C., de Vita, S., Di Vito, M. A., Isaia, R., et al. (1999). Short-term Ground Deformations and Seismicity in the Resurgent Campi Flegrei Caldera (Italy): an Example of Active Block-Resurgence in a Densely Populated Area. J. Volcanology Geothermal Res. 91, 415–451. doi:10.1016/s0377-0273(99)00050-5
Papale, P., and Marzocchi, W. (2019). Volcanic Threats to Global Society. Science. 363, 1275–1276. doi:10.1126/science.aaw7201
Papale, P. (2017). Rational Volcanic hazard Forecasts and the Use of Volcanic Alert Levels. J. Appl. Volcanol. 6, 13. doi:10.1186/s13617-017-0064-7
Pappalardo, L., and Mastrolorenzo, G. (2010). Short Residence Times for Alkaline Vesuvius Magmas in a Multi-Depth Supply System: Evidence from Geochemical and Textural Studies. Earth Planet. Sci. Lett. 296 (1-2), 133–143. doi:10.1016/j.epsl.2010.05.010
Parks, M. M., Biggs, J., England, P., Mather, T. A., Nomikou, P., Palamartchouk, K., et al. (2012). Evolution of Santorini Volcano Dominated by Episodic and Rapid Fluxes of Melt from Depth. Nat. Geosci. 5, 749–754. doi:10.1038/ngeo1562
Phillipson, G., Sobradelo, R., and Gottsmann, J. (2013). Global Volcanic Unrest in the 21st century: An Analysis of the First Decade. J. Volcanology Geothermal Res. 264, 183–196. doi:10.1016/j.jvolgeores.2013.08.004
Poland, M. P., and Anderson, K. R. (2020). Partly Cloudy with a Chance of Lava Flows: Forecasting Volcanic Eruptions in the Twenty‐first century. J. Geophys. Res. Solid Earth. 125 (1), e2018JB016974. doi:10.1029/2018jb016974
Reichardt, U., Ulfarsson, G. F., and Petursdottir, G. (2017). Cooperation Between Science and Aviation-Sector Service Providers in Europe for Risk Management of Volcanic Ash. Transportation Res. Rec. 2626, 99–105. doi:10.3141/2626-12
Ripepe, M., Marchetti, E., Delle Donne, D., Genco, R., Innocenti, L., Lacanna, G., et al. (2018). Infrasonic Early Warning System for Explosive Eruptions. J. Geophys. Res. 123, 11 9570–9585. doi:10.1029/2018jb015561
Rivalta, E., Corbi, F., Passarelli, L., Acocella, V., Davis, T., and Di Vito., M. A. (2019). Stress Inversions to Forecast Magma Pathways and Eruptive Vent Location. Sci. Adv. 5, eaau9784. doi:10.1126/sciadv.aau9784
Romero, J. E., Morgavi, D., Arzilli, F., Daga, R., Caselli, A., Reckziegel, F., et al. (2016). Eruption Dynamics of the 22-23 April 2015 Calbuco Volcano (Southern Chile): Analyses of Tephra Fall Deposits. J. Volcanology Geothermal Res. 317, 15–29. doi:10.1016/j.jvolgeores.2016.02.027
Rougier, J., Sparks, R. S. J., Cashman, K. V., and Brown, S. K. (2018). The Global Magnitude-Frequency Relationship for Large Explosive Volcanic Eruptions. Earth Planet. Sci. Lett. 482, 621–629. doi:10.1016/j.epsl.2017.11.015
Sandri, L., Guidoboni, E., Marzocchi, W., and Selva, J. (2009). Bayesian Event Tree for Eruption Forecasting (BET_EF) at Vesuvius, Italy: a Retrospective Forward Application to the 1631 Eruption. Bull. Volcanol. 71, 729–745. doi:10.1007/s00445-008-0261-7
Santacroce, R., Cioni, R., Marianelli, P., Sbrana, A., Sulpizio, R., Zanchetta, G., et al. (2008). Age and Whole Rock-Glass Compositions of Proximal Pyroclastics from the Major Explosive Eruptions of Somma-Vesuvius: A Review as a Tool for Distal Tephrostratigraphy. J. Volcanology Geothermal Res. 177, 1–18. doi:10.1016/j.jvolgeores.2008.06.009
Scaillet, B., Pichavant, M., and Cioni, R. (2008). Upward Migration of Vesuvius Magma Chamber over the Past 20,000 Years. Nature. 455, 216–219. doi:10.1038/nature07232
Scarpa, R., Tronca, F., Bianco, F., and Del Pezzo, E. (2002). High Resolution Velocity Structure beneath Mount Vesuvius from Seismic Array Data. Geophys. Res. Lett. 29, 415. doi:10.1785/012000028710.1029/2002gl015576
Segall, P. (2013). Volcano Deformation and Eruption Forecasting. Geol. Soc. Lond. Spec. Publications. 380 (1), 85–106. doi:10.1144/sp380.4
Self, S. (2006). The Effects and Consequences of Very Large Explosive Volcanic Eruptions. Phil. Trans. R. Soc. A. 364, 2073–2097. doi:10.1098/rsta.2006.1814
Selva, J., Orsi, G., Di Vito, M. A., Marzocchi, W., and Sandri, L. (2012). Probability hazard Map for Future Vent Opening at the Campi Flegrei Caldera, Italy. Bull. Volcanol. 74, 497–510. doi:10.1007/s00445-011-0528-2
Sigmundsson, F., Hooper, A., Hreinsdóttir, S., Vogfjörd, K. S., Ófeigsson, B. G., Heimisson, E. R., et al. (2015). Segmented Lateral Dyke Growth in a Rifting Event at Bárðarbunga Volcanic System, Iceland. Nature. 517, 191–195. doi:10.1038/nature14111
Sigmundsson, F., Parks, M., Pedersen, R., Jónsdóttir, K., Ófeigsson, B. G., Grapenthin, R., et al. (2018). Magma Movements in Volcanic Plumbing Systems and Their Associated Ground Deformation and Seismic Patterns. Volcanic Igneous Plumbing SystemsEditors S. Burchardt (Elsevier), 285–322. doi:10.1016/b978-0-12-809749-6.00011-x
Simkin, T., and Siebert, L. (2000). Earth’s Volcanoes and Eruptions: An Overview. Encyclopedia of Volcanoes. Editors H. Sigurdsson, B. Houghton, H. Rymer, J. Stix, and S. McNutt (San Diego: Academic Press), 249–262.
Sparks, R. S. J., Annen, C., Blundy, J. D., Cashman, K. V., Rust, A. C., and Jackson, M. D. (2019). Formation and Dynamics of Magma Reservoirs. Philos. Trans. A. Math. Phys. Eng. Sci. 377, 20180019. doi:10.1098/rsta.2018.0019
Sparks, R. S. J., and Aspinall, W. P. (2004). Volcanic Activity: Frontiers and Challenges in Forecasting, Prediction and Risk Assessment. The State of the Planet: Frontiers and Challenges in Geophysics. 150, 359–371.
Sparks, R. S. J., and Cashman, K. V. (2017). Dynamic Magma Systems: Implications for Forecasting Volcanic Activity. Elements. 13, 35–40. doi:10.2113/gselements.13.1.35
Sparks, R. S. J. (2003). Forecasting Volcanic Eruptions. Earth Planet. Sci. Lett. 210, 1–15. doi:10.1016/s0012-821x(03)00124-9
Tadini, A., Bisson, M., Neri, A., Cioni, R., Bevilacqua, A., and Aspinall, W. P. (2017). Assessing Future Vent Opening Locations at the Somma-Vesuvio Volcanic Complex: 1. A New Information Geodatabase with Uncertainty Characterizations. J. Geophys. Res. Solid Earth. 122, 4336–4356. doi:10.1002/2016jb013858
Taisne, B., Tait, S., and Jaupart, C. (2011). Conditions for the Arrest of a Vertical Propagating Dyke. Bull. Volcanol. 73 (2), 191–204. doi:10.1007/s00445-010-0440-1
Takahashi, H. (2008). Real-Time Eruption Magnitude Estimation from Far-Field Geodetic Data: A Proposal for Volcanic Early Warning. J. Disaster Res. 3 (4), 253. doi:10.20965/jdr.2008.p0252
Tamburello, G., Caliro, S., Chiodini, G., De Martino, P., Avino, R., Minopoli, C., et al. (2019). Escalating CO2 Degassing at the Pisciarelli Fumarolic System, and Implications for the Ongoing Campi Flegrei Unrest. J. Volcanology Geothermal Res. 384, 151–157. doi:10.1016/j.jvolgeores.2019.07.005
Tierz, P., Loughlin, S. C., and Calder, E. S. (2019). VOLCANS: an Objective, Structured and Reproducible Method for Identifying Sets of Analogue Volcanoes. Bull. Volcanol. 81 (12), 76. doi:10.1007/s00445-019-1336-3
Tramelli, A., Godano, C., Ricciolino, P., Giudicepietro, F., Caliro, S., Orazi, M., et al. (2021). Statistics of Seismicity to Investigate the Campi Flegrei Caldera Unrest. Sci. Rep. 11, 7211doi. doi:10.1038/s41598-021-86506-6
Troise, C., De Natale, G., Schiavone, R., Somma, R., and Moretti, R. (2019). The Campi Flegrei Caldera Unrest: Discriminating Magma Intrusions from Hydrothermal Effects and Implications for Possible Evolution. Earth-Science Rev. 188, 108–122. doi:10.1016/j.earscirev.2018.11.007
Umberto Tammaro, F., Prospero De Martino, P., Francesco Obrizzo, F., Giuseppe Brandi, G., Andrea D’Alessandro, A., Mario Dolce, M., et al. (2013). Somma Vesuvius Volcano: Ground Deformations from CGPS Observations (2001-2012). Ann. Geophys. 56, 1–8. doi:10.4401/ag-6462
Vanorio, T., and Kanitpanyacharoen, W. (2015). Rock Physics of Fibrous Rocks Akin to Roman concrete Explains Uplifts at Campi Flegrei Caldera. Science. 349 (6248) 617–621. doi:10.1126/science.aab1292
Wang, C. Y., and Manga, M. (2010). Hydrologic Responses to Earthquakes and a General Metric. Geofluids. 10, 206–216. doi:10.1111/j.1468-8123.2009.00270.x
White, R., and McCausland, W. (2016). Volcano-tectonic Earthquakes: A New Tool for Estimating Intrusive Volumes and Forecasting Eruptions. J. Volcanology Geothermal Res. 309, 139–155. doi:10.1016/j.jvolgeores.2015.10.020
Winson, A. E. G., Costa, F., Newhall, C. G., and Woo, G. (2014). An Analysis of the Issuance of Volcanic Alert Levels during Volcanic Crises. J. Appl. Volcanol. 3, 14. doi:10.1186/s13617-014-0014-6
Keywords: volcano unrest, magma accumulation, dyke propagation, eruption, volcano monitoring
Citation: Rosi M, Acocella V, Cioni R, Bianco F, Costa A, De Martino P, Giordano G and Inguaggiato S (2022) Defining the Pre-Eruptive States of Active Volcanoes for Improving Eruption Forecasting. Front. Earth Sci. 10:795700. doi: 10.3389/feart.2022.795700
Received: 15 October 2021; Accepted: 26 January 2022;
Published: 17 February 2022.
Edited by:
Luis E. Lara, Servicio Nacional de Geología y Minería de Chile (SERNAGEOMIN), ChileReviewed by:
Chris Newhall, Mirisbiris Garden and Nature Center, PhilippinesRoberto Moretti, UMR7154 Institut de Physique du Globe de Paris (IPGP), France
Copyright © 2022 Rosi, Acocella, Cioni, Bianco, Costa, De Martino, Giordano and Inguaggiato. This is an open-access article distributed under the terms of the Creative Commons Attribution License (CC BY). The use, distribution or reproduction in other forums is permitted, provided the original author(s) and the copyright owner(s) are credited and that the original publication in this journal is cited, in accordance with accepted academic practice. No use, distribution or reproduction is permitted which does not comply with these terms.
*Correspondence: Mauro Rosi, maurogiovarosi@gmail.com