- 1Key Laboratory of Mineral Resources, Institute of Geology and Geophysics, Chinese Academy of Sciences, Beijing, China
- 2Innovation Academy for Earth Science, Chinese Academy of Sciences, Beijing, China
- 3College of Earth and Planetary Sciences, University of Chinese Academy of Sciences, Beijing, China
- 4Xi’an Geophysical and Geochemical Exploration Co., Northwest Bureau of Geological Non-Ferrous Metals, Xi’an, China
An ideal metallogenic and prospecting model has important guiding significance for aluminum ore development and geophysical exploration. Previous research in this field only focused on ore body evaluations and metallogenic belts. Selection of reasonable geophysical methods for exploration of bauxite deposits is still challenging. To solve these problems, a new metallogenic model based on the new geological information is established firstly. The ore-bearing rock and ore bodies of sedimentary bauxite deposits are strictly controlled by the paleo-topographic conditions. The undulating shapes of the paleo-topography control the scales and shapes of ore-bearing rock series and bauxite ore bodies. Then, different geophysical methods applied for exploration of bauxite deposit are analyzed based on the petrophysical measurement. For the areas with thick overburden and large buried depths, it was found that fixed-loop transient electromagnetic (TEM), high-precision gravity, and seismic exploration methods were most effective. In regard to the areas with thin overburden and small buried depths, direct current (DC) sounding, high-precision gravity, seismic exploration, and TEM methods were considered to be effective. However, for the areas with very shallow buried depths, DC sounding and seismic exploration methods were deemed to be more suitable. This study selected the actual exploration processes of the Wanggudong bauxite mining area in western Henan as an example, and the application of a DC sounding method for sedimentary-type bauxite was successfully introduced.
1 Introduction
Although China’s bauxite mineral output is large, it cannot meet the needs of industrial development. The proven reserves of bauxite in Shanxi ranked first in China, accounting for 45% of the total reserves of bauxite in China. Henan’s bauxite reserves were ranked second in China. When the bauxite resources in Shandong and Shaanxi are considered together, the bauxite deposits in northern China account for more than 65% of the total bauxite resources (Sun et al., 2018). However, the minable time is only 14 years, which is far lower than the world’s average level of 102 years. Therefore, tapping into the potential of its domestic bauxite resources has become a difficult challenge for China in recent years.
The bauxite in northern China mainly occurs in the Benxi Formation of the Middle Carboniferous. The ore-bearing rock and ore bodies are strictly controlled by the paleo-topography conditions (Liu, 2011). It has been observed that the shapes of the bauxite change greatly, and it has been difficult to accurately delineate the favorable prospecting areas (limestone buckets). In recent years, geophysical explorations, remote sensing, and other prospecting methods have played important roles. Various methods have been successively applied to the investigation of metal mineral deposits, such as resistivity methods; high-precision magnetic methods; shallow seismic methods; transient electromagnetic (TEM) methods; controlled-source audio frequency magnetotelluric (CSAMT) methods, and so on (Xue et al., 2012; Manzi et al., 2013; Di et al., 2018; Di et al., 2020; Wu et al., 2020).
Li et al. (2002) used an electrical sounding method to detect concealed bauxite in loess-covered areas of Henan Province, and successfully inferred the shapes and spatial positions of the bauxite deposits. Li et al. (2013) and Xu et al. (2014) utilized CSAMT to delineate the occurrence sites of bauxite in the bauxite metallogenic areas of western Henan and Qinyuan in Shanxi Province. Ren, 2015 conducted gravity explorations in sedimentary bauxite. Zhang (2007) and Guo et al. (2015) explored bauxite deposits using a loop-source TEM method. The results showed that TEM method was able to accurately reflect the limestone buckets. Niu et al. (2017) adopted an induced polarization (IP) method to obtain the spatial locations of bauxite deposits. IP methods have been used as auxiliary methods for exploring potential bauxite resources. In addition, high-density resistivity methods have been successfully applied to distinguish bauxite with different occurrences and shapes, but the exploration depths tended to be limited. Liu et al., 2020 pointed out that shallow seismic reflection methods can be utilized to accurately obtain the buried depths and layer thicknesses of horizontal and gently inclined bauxite deposits.
In summary, it is still difficult to establish geological geophysical prospecting indicators for sedimentary bauxite in the northern China. Selection of the best observation methods is key technical problems and still challenging in exploration of bauxite deposit. Thus, we introduce the metallogenic model of the sedimentary type and establish the exploration methods for the bauxite deposits based on the petrophysical measurements. At last, one field survey is performed to verify the effectiveness of selected geophysical methods.
2 Metallogenic Models of the Sedimentary Bauxite Deposits in China
China’s bauxite deposits can be divided into two categories: Paleo-weathering crust bauxite deposits (Type I) and lateritic bauxite deposits (Type II). Among those types, Paleo-weathering crust bauxite deposits (Type I) accounts for more than 90% of China’s bauxite reserves, and those deposits are mainly distributed in northern and southwest China. Paleo-weathering crust bauxite deposits mainly include weathered crust allochthonous accumulation bauxite and paleo-weathering crust in-situ accumulation bauxite. Paleo-weathering crust bauxite deposits can be divided into sedimentary-type and accumulation-type bauxite deposits. Accumulation-type bauxite deposits are mainly distributed in Southwest China (Figure 1). They are Quaternary eluvial and slope deposits, and yellow, brown yellow and reddish brown block stones and soils as shown in Figure 2.
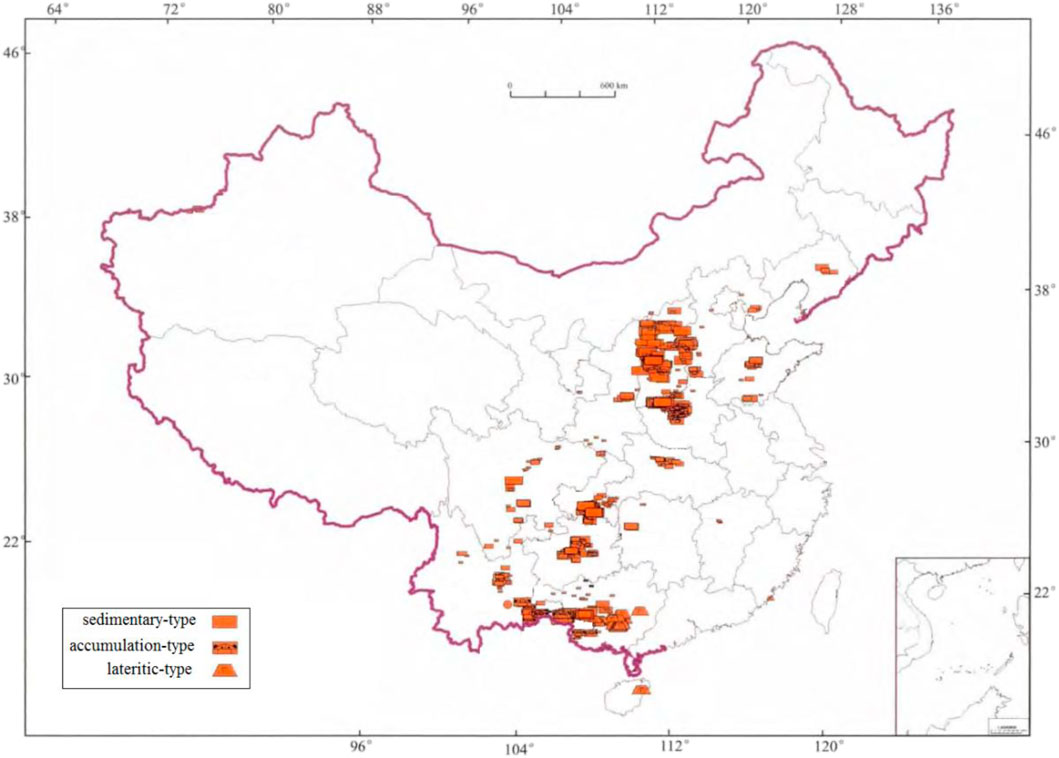
FIGURE 1. Distribution of bauxite deposits in China (modified after Gao et al., 2014).
For the weathering crust allochthonous accumulation bauxite, the bauxite has not accumulated in-situ but has migrated and accumulated via the lateritic weathering crust. Carboniferous bauxite and its ore-bearing rock series tend to overlap on the karst erosion surfaces of Cambrian and Ordovician carbonate rock formations. They are considered to be allochthonous accumulated bauxite deposits related to the lateritic paleo-weathering crust (Figure 3A). In addition, they are all composed of hematite and siderite lentils deposited in lake facies. Due to the fact that the underlying bedrock is carbonate rock, the residual Deluvial layers of the aluminum-rich calcium laterite is known to have been formed by weathering actions. The longer the erosion interruption and weathering timeframes are, the larger the amount and thicker the residual Deluvial aluminum rich calcium laterite layers formed by weathering will be. Also, the higher content levels of bauxite minerals and less content of clay minerals will be generated, thereby ensuring richer ore grades and larger thicknesses of ore body.
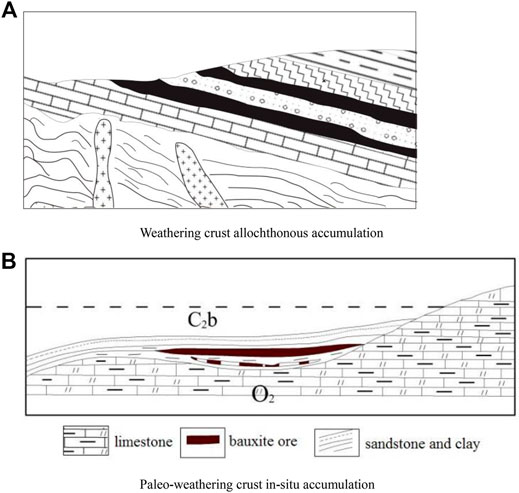
FIGURE 3. Metallogenic model of the weathered crust sedimentary bauxite. (A) Weathering crust allochthonous accumulation. (B) Paleo-weathering crust in-situ accumulation.
For the paleo-weathering crust in-situ accumulation type bauxite, it has been observed that the bauxite of this type of deposit will tend to directly cover the karst erosion surfaces of carbonate rock (Figure 3B). Therefore, it can be considered as a type of bauxite related to the in-situ accumulations of the lateritic paleo-weathering crust of carbonate rock. It is generally characterized by Shanxi-type iron ore without lacustrine deposition under the bauxite, which has accumulated in-situ, mainly in karst caves and buckets. The ore bodies are generally not longer than hundreds of meters, but their thicknesses have been found to be consistently large (40–60 m). In addition, if the erosion interruption timeframes were short, only the calcareous laterite eluvium will have formed, with slight migration and transportation characteristics. The majority of the previous studies have considered that the ore-forming materials originated from the underlying carbonate rock and other metamorphic rock (Mongelli et al., 2014; Economou-Eliopoulos et al., 2016).
The ore bodies were observed within the middle and upper sections of the upper Carboniferous iron aluminum rock series. Therefore, the occurrences of kaolinite, hydromica, chlorite, and other minerals were considered to be favorable for potential bauxite resources. It has been determined that iron, aluminum, clay, and coal are the products of the same climate conditions and often coexist together. Therefore, when coal is found in the upper parts and iron in the lower parts, such conditions may indirectly indicate favorable conditions for bauxite prospecting.
3 Petrophysical Characteristics and Selection of Geophysical Methods
Petrophysical characteristics are bridges connecting geology and geophysics. The physical properties of rocks and ores must be measured before geophysical exploration. The samples for petrophysical measurement are collected from the drilled cores. We use a Solartron-1260A impedance/gain-phase analyzer to measure complex resistivity measurements, and the frequencies 0.005 and 1,000 Hz are adopted for impedance spectroscopy. Table 1 shows the statistics of physical parameters of the rocks and ores.
In regard to resistivity properties, there has been found to be little difference between loess and the majority of the surrounding rock. The resistivity of loess generally ranges between 20 and 200 Ωm. Meanwhile, the resistivity of limestone is known to range from 2,500 to 6,000 Ωm while that of bauxite ore is 320 Ωm. Therefore, limestone has obviously higher resistivity characteristics compared with other types of rock. The resistivity values of bauxite fall between those of limestone and loess, and are generally very close to that of other surrounding rock. Therefore, it is difficult to directly explore ore bodies using electrical methods based on resistivity differences. Such methods can only indirectly explore ore bodies through investigating Ordovician limestone interface concave buckets. Transient electromagnetic methods are suitable for areas with less industrial interference. The responses of small and shallow concave buckets will not be obvious, and it may be difficult to distinguish bauxite or aluminous rock series. For monoclinic bauxite mining areas with layered distributions, which have only minimal relationships with limestone buckets, it tends to difficult to delineate the ore-bearing positions. However, direct current sounding with high resolution for shallow geo-electric structures can be adopted to locate hidden ore bodies.
In regard to velocity properties, it was determined that the velocity values of the surrounding rock ranged between 2,000 and 3,000 m/s, and that of the limestone was larger than 5,000 m/s. The upper section was a thin coal seam, and the lower section was limestone stratum with different weathering degrees. The wave impedance of the bauxite layers and the underlying limestone stratum were observed to be quite different, which was reflected in the form of strong interface reflections in the wave fields. The differences in the wave impedance between the bauxite layers and the overlying coal measures were minimal, and the energy of the interfaces reflected that the wave fields were weak. In addition, since the thickness of the Quaternary loess overburden was larger than 100 m, the absorption and attenuation of the wave fields were severe. It was considered that those conditions would directly affect the resolution of the reflected wave explorations. It has been found that strong wave impedance interfaces (positive phases) may be formed on the top surfaces of bauxite layers. Meanwhile, the top reflections of the overlying limestone may become weak reflection interfaces (both positive and negative phases). Therefore, it was believed that depression areas on the same phase axis should be considered as favorable areas for bauxite mineralization. Seismic wave fields contain large amounts of geological information, such as stratum fluctuations, lithology, rock thicknesses, and other changes. Therefore, the attribute parameters of the seismic wave fields can be extracted and the comprehensive prediction methods adopted. The thicknesses of the ore layers can also be determined. Shallow seismic methods for the exploration of bauxite ore layers in sedimentary rock area are considered to be highly reliable and accurate geophysical exploration methods. When such methods are adopted, the fluctuation characteristics of the limestone top surfaces can be reflected and the ore bearing favorable areas (buckets) delineated. In addition, the burial depths, structural fluctuations, and fault development of the bauxite deposits can be successfully revealed, which can be used to reflect the distribution characteristics of the bauxite deposits and predict their thicknesses.
In the current investigation, the density properties of the loess, carbonaceous mud stone, and coal were determined to be below 2.0 g/cm3. That of the sandstone and mudstone were 2.0–2.4 g/cm3; bauxite was 2.5 g/cm3; and the density of the limestone ranged between 2.6 and 2.7 g/cm3. The density properties of the majority of the surrounding rock were quite different from that of the bauxite and limestone. However, the differences between the bauxite and limestone were relatively small. It was found that the scale of the bauxite was also small and gravity explorations could only select the limestone depressions as the targets for indirect prospecting. The macroscopic height variations of gravity Bouguer anomalies reflected the depth variation characteristics of the limestone. Local low-gravity conditions were observed to often occur in the concave buckets, and the anomaly amplitude was determined to be 0.5 × 10–5 m/s2, which was considered to be a favorable sign for ore prospecting potential. Gravity data are used to understand the fluctuation characteristics of limestone top surfaces. When stratigraphic structures are complex, it has proven difficult to reach clear conclusions regarding the most favorable parts of ore-bearing regions. Only regional research investigations can effectively delineate the areas related to mineralization, such as basement depressions and structures, in order to determine the favorable sections and ranges of mineralization.
Based on the petrophysical results, gravity, electrical, and seismic exploration methods can be applied to determine the locations of bauxite ore bodies through measurements of the density differences, resistivity differences, velocity differences, and other properties between the bauxite ore bodies and the surrounding rock. After data processing is completed and apparent resistivity is calculated, the spatial positions, sizes, shapes, geological structure characteristics, and mineral distribution patterns of those geological bodies can be inferred.
4 Case Study
The Wanggudong mining area is located at the southern section of the western ore belt of the Shaan-Mianchi-Xin bauxite belt (Figure 4). The ore belts have good metallogenic geological conditions and great prospecting potential. The bauxite deposits in the Wanggudong mining area tend to be well exposed and shallowly buried.
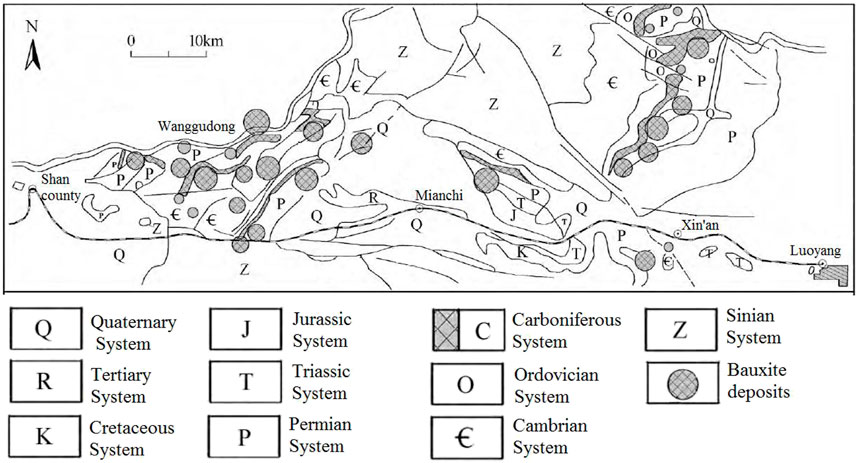
FIGURE 4. Geological map and distribution of the bauxite deposits in western Henan Province (modified after Li and Li, 2019).
The ore bodies in the study area were mainly quasi-layered, lenticular, or funnel shaped. There were also transition types among the three shapes, which could potentially appear within the same mining area and connect with each other. The shapes of the ore bodies were determined to be closely related to the ancient karst erosion surfaces. In the karst basins and depressions with flat and open ancient terrain, quasi-layered ore with stable thickness had formed. In the sections with large fluctuations of Ordovician erosion surfaces, funnel-shaped ore bodies with thin periphery were observed. The occurrences of the layered and lenticular ore bodies were basically consistent with those of the surrounding rock. This was particularly evident for the occurrences of the layered ore bodies, which were almost the same as those of upper and lower strata.
The thicknesses of the deposits generally range between 3.30 and 11.00 m, with an average thickness of 5.92 m. The ore grade tends to be high, and the Al2O3 content is generally 61.27 to 75.28 × 10−2, with an average of 68.81 × 10−2. The A/S is generally between 3.2 and 28.0, with an average of 7.6.
The geotectonic positions of the survey area were located in the northwestern section of Mianchi-Queshan depression fold of the Huaxiong platform margin depression of the Sino-Korean para-platform. The bauxite deposits in the study area were found to occur in the aluminous clay rock series of the Benxi Formation in the Middle Carboniferous system. The ore body was layered and bucket shaped. The thickness values of the ore body changed along the vertical and horizontal directions. The occurrence of the ore body was 140° and the dip angle was approximately 14°. The examined ore body was monoclinic, and the north side of the ore body was controlled by paleo uplift. It is generally considered that the greater the thickness of the ore was, the better the ore quality would be. The study area was found to contain developed sedimentary strata and rich coal and aluminum resources. It is considered to be one of the most important bauxite producing areas in China.
For the intermediate gradient device of the DC method, the measuring line was in the range of 1/3 to 2/3 of the transmitting line AB. The offset was less than 1/5 of the transmitting line; MN indicated the receiving electrode distance; and the sounding device was only required to be fixed in the receiving position and the length of the transmitting line AB changed, as shown in Figure 5. In this research investigation, the electrode distances of AB/2 varied as follows: 5, 10, 15, 20, 40, 60, 80, 100, 150, 200, 250, 300, 350, 400, 450, and 500 m, respectively. The measuring electrode distance MN/2 = AB/10 m. The distances between receiving electrodes were 10 and 20 m, respectively. The transmitting current is 1 A and the transmitting voltage reaches 800 V. The instrument used to observe the apparent resistivity parameters was a Canadian GDD-made high-power instrument. The power supply cycle is 8 s.
The observed chargeability at point D10 is shown in Figure 6. The chargeability curve is smooth and the values of error bars are smaller than 1.55%, which means that the quality of observed data is high.
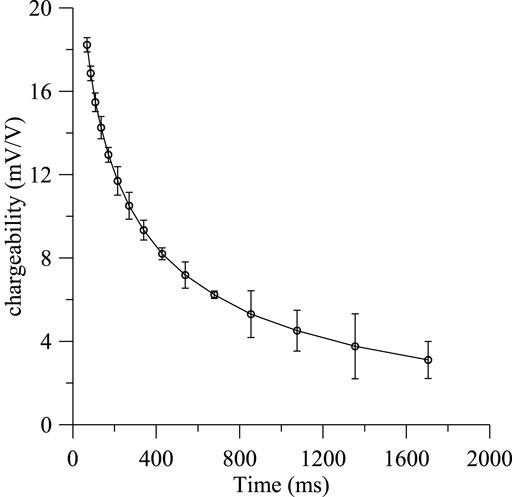
FIGURE 6. Observed chargeability curve and error bars at point D10. The maximum value of error bar is 1.55%.
The apparent resistivity can be calculated using following formula
where K is configuration parameters,
In order to understand the abnormal Shape 3 of the longitudinal section, a total of ten sounding points were arranged. It was found that the apparent resistivity values gradually increased from shallow to deep, and the local changes were caused by the non-uniformity of the electrical properties. It was found that depressions appeared at the deep parts of the D8, D10, and D14 sounding points, which were inferred to be caused by karst depressions (Figure 7). There was still considered to be the possibility of a bauxite deposit in the longitudinal direction. Drilling verifications ZK3181, ZK1576, and ZK0074 were carried out at the D6, D10, and D14 sounding points. The largest drilling depth was 180=m, and the buried depth of the bauxite was determined to be between 149 and 155 m, as shown in Figure 8.
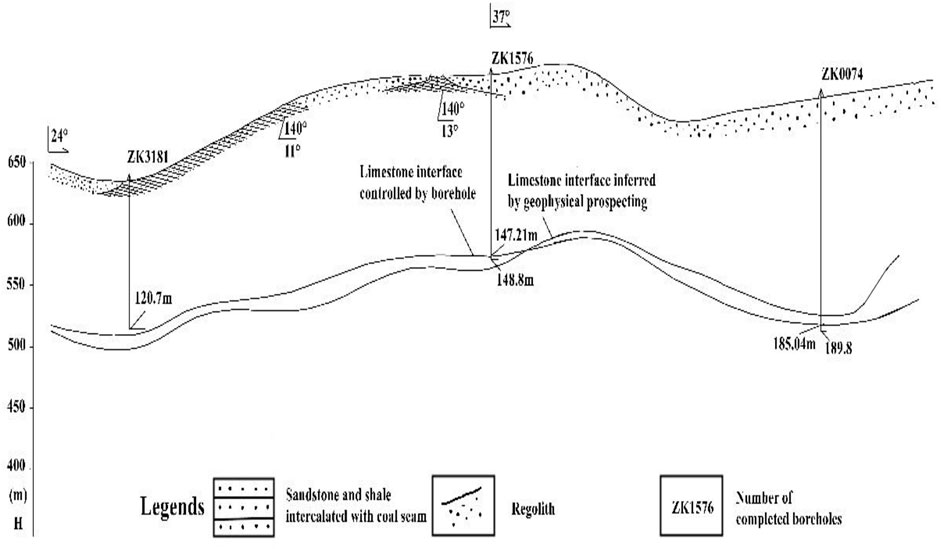
FIGURE 8. Geological interpretations in accordance with the drilling results. ZK denotes the drilling core.
5 Conclusion
The bauxite deposits in China are mainly weathering crust sedimentary types. The depressions located on the top of Ordovician limestone are considered to be favorable positions for bauxite mineralization. In regard to the bauxite with different geological characteristics, the selection of the most effective exploration method is the key to determining bauxite deposit locations. In the current study, geophysical prospecting was carried out in the Wanggudong mining area of western Henan Province. According to the characteristics of the occurrence locations, buried depths, scales, shapes, and buried depths of the karst buckets in the mining area, the distribution range of the low-resistivity region could be determined using the resistivity measurements. Based on the distribution of low-resistivity region, the buried depths and horizontal location of ore bodies were successfully determined.
Data Availability Statement
The original contributions presented in the study are included in the article/supplementary material, further inquiries can be directed to the corresponding author.
Author Contributions
All authors listed have made a substantial, direct, and intellectual contribution to the work and approved it for publication.
Funding
This research study was supported by the Natural Science Foundation of China (NSFC) (42030106, 41874162), and the Scientific Equipment Instrument and Development Project of the Chinese Academy of Sciences (YJKYYQ20190004).
Conflict of Interest
Author GW was employed by the company Xi’an Geophysical and Geochemical Exploration Co.
The remaining authors declare that the research was conducted in the absence of any commercial or financial relationships that could be construed as a potential conflict of interest.
Publisher’s Note
All claims expressed in this article are solely those of the authors and do not necessarily represent those of their affiliated organizations, or those of the publisher, the editors and the reviewers. Any product that may be evaluated in this article, or claim that may be made by its manufacturer, is not guaranteed or endorsed by the publisher.
References
Di, Q.-y., Xue, G.-q., Lei, D., Wang, Z.-x., Zhang, Y.-m., Wang, S., et al. (2018). Geophysical Survey over Molybdenum Mines Using the Newly Developed M-TEM System. J. Appl. Geophys. 158, 65–70. doi:10.1016/j.jappgeo.2018.07.008
Di, Q., Xue, G., Lei, D., Wang, Z., Li, H., and Olalekan, F. (2020). Demonstration of the Newly Developed MTEM Systems for Gold Detection in China. Geol. J. 55, 1763–1770. doi:10.1002/gj.3549
Economou-Eliopoulos, M., Frei, R., and Megremi, I. (2016). Potential Leaching of Cr(VI) from Laterite Mines and Residues of Metallurgical Products (Red Mud and Slag): An Integrated Approach. J. Geochemical Exploration 162, 40–49. doi:10.1016/j.gexplo.2015.12.007
Gao, L., Wang, D. H., Xiong, X. Y., and Yi, C. W. (2014). Summary on Aluminum Ore Deposits Minerogenetic Regulation in China. Acta Geologica Sinica 88 (12), 2284–2295. doi:10.1111/1755-6724.12374_12
Guo, W. B., Fan, J. S., Zhang, Y. F., Zhang, S. M., and Zhong, H. S. (2015). Rapid Evaluation Technology for bauxite Prospecting in north China. Annu. Meet. Chin. Geosci. Union 1728.
Li, B., Wang, X. Q., Ding, Y. H., Zhang, L., Wei, M. J., Li, S. X., et al. (2013). Application Study of CSAMT Method to Investigation and Evaluation Project of bauxite. J. Eng. Geology. 21 (06), 987–994.
Li, H. C., Zhou, K., and Dong, H. J. (2002). Application of Electric Sounding Method to the Prediction of Concealed bauxite in Loess Covered Area. Light Met. 11, 5–8.
Li, X., and Li, L. (2019). Geological Characteristics and Prospecting Indicators of Wumenggou Underclay (Coal) bauxite deposit in Shanxian County, Henan Province. Mineral. Resource Geology. 33 (3), 442–446.
Liu, X. F. (2011). “Material Composition and Ore-Forming Process of Bauxite (Clay) Deposits in Western Henan, China,” (Beijing: China University of Geosciences), 1–176. PhD Dissertation.
Liu, X., Wang, Q., Zhao, L., Peng, Y., Ma, Y., and Zhou, Z. (2020). Metallogeny of the Large-Scale Carboniferous Karstic bauxite in the Sanmenxia Area, Southern Part of the North China Craton, China. Chem. Geology. 556, 119851. doi:10.1016/j.chemgeo.2020.119851
Manzi, M. S. D., Hein, K. A. A., Durrheim, R., and King, N. (2013). Seismic Attribute Analysis to Enhance Detection of Thin Gold-Bearing Reefs: South Deep Gold Mine, Witwatersrand basin, South Africa. J. Appl. Geophys. 98, 212–228. doi:10.1016/j.jappgeo.2013.08.017
Mongelli, G., Boni, M., Buccione, R., and Sinisi, R. (2014). Geochemistry of the Apulian Karst Bauxites (Southern Italy): Chemical Fractionation and Parental Affinities. Ore Geology. Rev. 63, 9–21. doi:10.1016/j.oregeorev.2014.04.012
Niu, J., Feng, Y., Pan, X. S., and Zhang, Z. P. (2017). The Application and Research of Using Comprehensive Geophysical Methods to Prospecting Sedimentary bauxite in Wenshan District, Yunnan. J. Yunnan University( Nat. Sci. Edition) 39 (S2), 116–123.
Ren, F. L. (2015). “The Exploration and Research of the Gravity Method to Sedimentary bauxite Prospecting,” (Chang’ An University). Master's Thesis.
Sun, L., Xiao, K. Y., and Lou, D. B. (2018). Mineral Prospectivity of bauxite Resources in China[J]. Earth Sci. Front. 25 (3), 82–94.
Wu, G., Chen, G., Wang, D., Cheng, Q., Zhang, Z., Yang, J., et al. (2020). Identifying mineral Prospectivity Using Seismic and Potential Field Data in the Hongniangyu District, Inner Mongolia, China. Ore Geology. Rev. 119, 103317. doi:10.1016/j.oregeorev.2020.103317
Xu, X. X., Li, S. B., Yuan, H., Cao, P. J., Zheng, G. L., and Zheng, J. (2014). The Application of Integrated Geophysical Exploration to Concealed bauxite Deposits in Qingyuan of Shanxi Province. Geophys. Geochemical Exploration 38 (01), 41–45.
Xue, G.-Q., Qin, K.-Z., Li, X., Li, G.-M., Qi, Z.-P., and Zhou, N.-N. (2012). Discovery of a Large-Scale Porphyry Molybdenum Deposit in Tibet through a Modified TEM Exploration Method. Jeeg 17 (1), 19–25. doi:10.2113/jeeg17.1.19
Keywords: bauxite deposits, sedimentary type, geophysical prospecting, direct current sounding, north China
Citation: Guoqiang X, Nannan Z, Wenbo G and Shun Z (2022) A Metallogenic Model for Bauxite Deposits and Geophysical Prospecting Methods: Using the Sedimentary Type in Northern China as an Example. Front. Earth Sci. 10:791250. doi: 10.3389/feart.2022.791250
Received: 08 October 2021; Accepted: 14 February 2022;
Published: 17 March 2022.
Edited by:
Giovanni Martinelli, National Institute of Geophysics and Volcanology, ItalyReviewed by:
Yu Fan, Hefei University of Technology, ChinaYayun Liang, University of Science and Technology Beijing, China
Luca Piroddi, University of Cagliari, Italy
Copyright © 2022 Guoqiang, Nannan, Wenbo and Shun. This is an open-access article distributed under the terms of the Creative Commons Attribution License (CC BY). The use, distribution or reproduction in other forums is permitted, provided the original author(s) and the copyright owner(s) are credited and that the original publication in this journal is cited, in accordance with accepted academic practice. No use, distribution or reproduction is permitted which does not comply with these terms.
*Correspondence: Xue Guoqiang, cHB4dWVndW9xaWFuZ0AxNjMuY29t