- 1School of Geosciences, University of South Florida, Tampa, FL, United States
- 2Geological Sciences, University of North Carolina Chapel Hill, Chapel Hill, NC, United States
Understanding relationships between different components involved in subduction-related recycling of sediment at arc volcanic systems has long been a focus of study. It is generally understood that volcanic arc lavas incorporate materials from the down-going slab including fluids, fluid mobile elements (FME), and fluid modified large ion lithophile elements (LILE), largely derived from subducted sediments and serpentinized ultramafic rocks. Past studies have presumed bulk sediment contributions rather than addressing localized variations in sediment composition. We examine trace element variability along the Lesser Antilles Island Arc (LAIA) and show that sediment inputs via fluid transfer to the mantle wedge are necessary to explain high B (up to 50 ppm), high B/La ratios, and higher As, Cs, Rb in the central LAIA. In contrast, the northern portion of LAIA is enriched in Ba, Sr and U/Th, with low FME. Grenada has low FME abundances, low Ba/La, but higher Sm/La. Our results suggest that modification of LAIA mantle source by fluid addition is important for the central islands but find that sediment enrichment is more likely in the northern section of the LAIA. Li/Yb ratios suggest the Grenada signature is chemically more comparable to sediment from DSDP Site 144 (Demerara Rise), while Martinique magmas in the central LAIA are affiliated to sediments from Site 543 (offshore Dominica). Unexpectedly, the northern portion of LAIA is not directly relatable to Site 543 sediments but instead trends back toward a sediment source similar in composition to Site 144 but with lower B, lower La/Sm and higher Ba. Binary mixing models suggest the northern islands are likely influenced by small amounts of sediment melt similar to average Site 144 sediments, but with Ba concentrations ranging from 1,250 to 1,500 ppm. Trace element ratios then likely result from different levels of contribution from sediment packages both spatially and temporally along the arc, with some variation evident among samples from the same island. We suggest that the process of recycling subducted sediment along the arc is variable along the length of the LAIA, wherein the trace element relationships are likely strongly influenced by the types of sediment being subducted along strike.
Introduction
Over the past three decades, work on the volcanic products of subduction zones has shown that elemental enrichments characteristic of arc magmas arise largely from subducted altered oceanic crust and sediments, and are sampled via fluid-mediated slab material released into the convecting mantle wedge, over a range of slab depths (Tera et al., 1986; Plank and Langmuir 1993; Ryan et al., 1995; Tatsumi and Eggins 1995; Elliott et al., 1997; Bebout et al., 1999; Johnson and Plank 1999; Class et al., 2000; Straub and Layne 2002; Savov et al., 2005, Savov et al., 2007; Grove et al., 2006; Tonarini et al., 2007; Walker et al., 2009; Labanieh et al., 2012). Melts are generated primarily due to hydrous phase equilibria of fluid fluxed mantle material in the wedge above the subducting lithosphere (Kushiro 1972; Grove and Kinzler 1986; Kushiro 1990; Peacock 1990; Gaetani et al., 1993; Hawkesworth et al., 1993; Tatsumi & Eggins 1995; Thirlwall et al., 1996; Gaetani and Grove. 2003). Given the importance of hydrous fluids in arc melting, the trace elements that are readily mobile in metasomatic fluids are those that are distinctively enriched in arc magmas. The specifics of the geochemical signatures of arc lavas can be markedly different from arc to arc, from volcano to volcano within arcs, and can sometimes vary by eruptive event in a single volcano, though in nearly all cases the “ingredients” in the lavas can largely (if not always directly) be connected back to the makeup of the materials input to adjacent deep-sea trenches (Plank and Langmuir 1993; Plank and Langmuir 1998; Plank 2014).
Typically, ratios of large ion lithophile elements (LILE), such Ba, K, Sr, U and Pb, versus high field strength elements (HFSE: Nb, Ta, Th, Y), or rare earth elements (REE), along with key radiogenic isotope ratios (207Pb/204Pb; 87Sr/86Sr; 143Nd/144Nd) have been used to identify the effects of crustal or sediment melt/fluid additions (Miller et al., 1994; Class et al., 2000). Fluid mobile elements, or FME (B, As, Sb, ±Cs, Rb, ±Pb; Leeman and Sisson, 1996) have shared chemical affinities that make them highly sensitive to material transfers mediated by metasomatic fluids in subduction zones, in particular those fluids generated by prograde metamorphic reactions on the down-going plate at shallow depths (Bebout et al., 1993, Bebout et al., 1999; Ryan et al., 1995, Ryan et al., 1996; Leeman and Sisson 1996; Noll et al., 1996; Smith et al., 1997; Benton et al., 2001; Savov et al., 2007; Ryan and Chauvel, 2014). Data for the FME suite in the Lesser Antilles have thus far been limited to an early whole rock study in Martinique (Smith et al., 1997) whereas B and δ11B have been examined in melt inclusion suites along-arc (Cooper et al., 2020) and from Grenada and St. Vincent (Bouvier et al., 2008, Bouvier et al., 2010). These studies have focused on recent (<5 Ma) products, and FME have not been investigated for older islands or across-arc to assess evolution from old (>20 Ma) to recent (5 Ma-present) volcanics. While single-center studies can provide insights, the sensitivity of B and other fluid-mobile species to arc-scale parameters, such as slab surface temperatures and/or the morphology of the down-going plate (Singer et al., 2007; Ryan and Chauvel, 2014) means that any single-volcano dataset for these species needs to be considered in the context of its arc-scale systematics. This work presents an arc-scale dataset for FME abundances in whole rocks from the Lesser Antilles subduction system, taking advantage primarily of a large and well-documented suite of Cenozoic-to-recent volcanic rocks from the active volcanic centers along the arc. These data are compared to other works including melt inclusions (Bouvier et al., 2008; Bouvier et al., 2010; Cooper et al., 2020) with the understanding that while useful, melt inclusion compositions may represent specific moments in magma evolution and are not always representative of the melt that erupt on the surface. Associated whole rock comparisons to other similar lavas from the same center, and lavas from other centers provide key information about slab enrichment process(es). Such studies provide information about regional differences derived from variable slab input, related to physical dynamics of subduction or variability in trench inputs. A comprehensive whole rock elemental suite is necessary to constrain the averaged signatures of the volcanic output (thereby constraining likely slab inputs) and to identify anomalous petrogenetic melt components.
Background
Fluid mobile elements as tracers of subduction enrichment
Fluid-mobile element (FME) abundance systematics (after Leeman and Sisson, 1996) have been examined in many subduction systems either directly or as part of larger studies (Sunda, Izu-Bonin-Mariana, Kurile-Kamchatka, Aleutians, Cascades, Mexico, Central America, Andes, Sandwich; see Ryan and Chauvel (2014) for a comprehensive list of projects). In a few arcs and arc segments, B and other FMEs show strong correlations with other large-ion-lithophile elements (LILE: Central America, see Leeman and Sisson 1996; Walker et al., 2003), though in most other arcs FME-LILE correlations, if they are evident at all, indicate complexities. Boron is often considered the quintessential FME that exhibits the most dramatic abundance variability. It is currently the only FME with a well-characterized stable isotopic system and has been used to examine the influence of fluid-mediated slab inputs on the makeup of the sub-arc and deep oceanic mantle (Leeman and Sisson 1996; Ryan et al., 1996; Ryan et al., 1996; Turner et al., 2007). Boron systematics track the fluid flux that triggers arc melting (e.g., B/Nb and B/Be ratios increase with increasing melt extent: Ryan and Langmuir 1993; Ryan et al., 1996). Other FMEs have been less comprehensively utilized, in part because less is known about their behaviors in solid/melt and solid/fluid systems, and because their geochemical profiles are, in some cases, complex (i.e., As and Sb are strong chalcophiles which concentrate in sulfide phases in MORBs, while they show lithophile-like systematics in subduction settings). Of the FME, B and Cs are probably the best understood geochemically, and B is particularly valuable as one can track both its abundance and stable isotopic variations to study metasomatic and/or magmatic processes in terrestrial and planetary systems (e.g., Ishikawa and Nakamura 1994; Chaussidon 1998; Lentz et al., 2001). Ryan and Langmuir (1993) and Ryan et al. (1995) documented regular across-arc depletion systematics for B in the Aleutians and Kuriles that were subsequently observed in other settings (Edwards et al., 1993; Walker et al., 2001; Walker et al., 2003) and in other FME (Cs, As, Pb, Sb: Noll et al., 1996; Leeman and Sisson, 1996), while Singer et al. (2007) documented along-arc variations in B that correlated strongly to increased sediment inputs related to morphologic variations in the down-going Pacific plate. These across-arc depletions mimicked the depletions in B and Cs observed during prograde subduction metamorphism (Bebout et al., 1993; Bebout et al., 1999; Moran et al., 1994; Leeman and Sisson 1996; London et al., 1996) with a strong correlation to the loss of C-O-H fluids associated with these changes (Schmidt and Poli 1998; 2003).
A handful of studies have examined B in lavas as well as xenoliths from the Lesser Antilles (Smith et al., 1997; Bouvier et al., 2008; Bouvier et al., 2010; Ruscitto et al., 2012; Cooper et al., 2020). Thus far none have looked in detail at other FME (As, Cs, Rb or Sb). Recent work on boron and B isotopes in melt inclusions in the Lesser Antilles have sought to examine indicators of subducted components via comparison of isotopic abundances to fluid (either as H2O, or Li, Cl, F). To explain the large range of boron and lithium, elemental and isotopic values observed (in Grenada, 2–20 ppm B; 3–20 ppm Li; δ11B: −20 to +10‰; δ7Li: −6 to +7‰) the authors call on a complex suite of fractionation and mixing processes involving fluid phases derived from sediment, altered crust, and serpentinite (Bouvier et al., 2008; Bouvier at al., 2010; Cooper et al., 2020), varying off a modeling approach used by Rose et al. (2001) for the Cascades. Comparisons to associated whole rock results, to similar lavas from the same center, and to similar lavas from other centers along the arc can provide key information as to the dominant slab enrichment process(es) acting beneath a particular volcano or along a volcanic trend. It can provide information about regional, arc-length changes in the makeup of the slab input, related either to changes in trench inputs or to the physical and dynamic parameters of subduction. This information is necessary both to constrain the averaged signatures of the volcanic output (thereby constraining likely slab inputs) at specific locations along the arc, and to identify those melt compositions that may point to petrogenetic anomalies. An important part of the problem may relate to the inferences made regarding endmember compositions, and in particular for the sediments, which are largely based on limited data which in turn limits an accurate determination of likely endmember compositions that could be used to constrain the along-arc input variability (as per Plank and Langmuir 1998). We will return to this point in subsequent sections, but it is important to note here that the source data for B, Li and other FME is still sparse.
B versus large ion lithophile elements and importance for source comparisons
The “enriched” LILE (Ba, K, Sr, U, Pb, Th) and the REE and HFSE (Nb, Ta, Ti, Zr, Y) are commonly ratioed to produce diagnostic geochemical “fingerprints” of different kinds of slab-involvement in subduction zone magmatism. LILE/REE and LILE/HFSE have been used to quantify contributions from subducted sediments and/or mafic crust to the source regions of arc magmas (see Plank and Langmuir 1993; Elliott et al., 1997; Class et al., 2000). B enrichments in arc lavas, measured as B/Be, B/La, or B/Nb ratios (Morris et al., 1990; Ryan and Langmuir 1993; Ishikawa and Nakamura 1994), have been used to assay the magnitude of fluid contributions to arc source regions (i.e., Leeman et al., 1994; Ryan et al., 1995; Hochstaedter et al., 1996; Noll et al., 1996; Cameron et al., 2003; Walker et al., 2003), though in detail the relationship between FME and H2O measured in lavas is complicated (see Ryan and Chauvel 2014, for a full discussion). A more accurate statement is that the FME document the involvement of an H2O -rich subduction component most likely derived from the forearc, though the B and FME in this reservoir are ultimately sourced from the down-going sediments and crust. The fact that B (and FME) enrichments decline regularly as a function of distance from the trench (i.e., Edwards et al., 1993; Ryan and Langmuir 1993; Ryan et al., 1995; Ishikawa and Nakamura 1994; Hochstaedter et al., 1996; Walker et al., 2003), and that B/LILE or B/HFSE ratios typically correlate inversely with La/Sm and other lithophile element ratios sensitive to changes in extent of melting in primitive basalt suites (i.e., Ryan et al., 1996) suggests that B (and by extension, other FME) are semi-quantitative measures of the “flux” which triggers melting in arcs.
As H2O -rich fluids from subducted sediments and altered oceanic crust are thought to be the main contributors of fluids to arc sources (Leeman et al., 1994; Noll et al., 1996; Ryan et al., 1996; Class et al., 2000), FME/HFSE ratios may be better indicators for fluid versus melt contributions to magma. To date, LILE/HFSE ratios are routinely used as proxies for subducted sediment contributions (Carr. 1984; Leeman et al., 1994; Reagan et al., 1994; Elliott et al., 1997; Patino et al., 2000; Walker et al., 2001; Rüpke et al., 2002; Abers et al., 2003; Elliott 2003; Jicha et al., 2004; Kimura and Yoshita 2006), while more recent work has focused on the relationship between B and a particularly useful LILE, barium (Ba) in determination of the influence of subducted sediments.
Caribbean geology and geochronology
The Lesser Antilles subduction system has been volcanically active for the past 40–50 Ma, as old Atlantic Ocean crust subducts beneath the Caribbean plate (Westercamp 1988; Figure 1A). The geometry and location of the subduction zone has changed substantially over time, as the Lesser Antilles have been the focus of volcanism over much of the past 40 Ma (Nagle et al., 1976; Brown et al., 1977; Briden et al., 1979; Hawkesworth and Powell 1980; Wadge and Shepard 1984; Legendre et al., 2018; Noury et al., 2021). Volcanism was restricted to the present Limestone Caribbees and the islands south of 15°N (Nagle et al., 1976) until ∼20 Ma, when volcanism stopped in the northern part of the arc. After volcanic activity in the northeastern islands ended, extensive calcareous sedimentation began during the Miocene (e.g., more than 500 m at Antigua, Mascle and Westercamp 1983; Hawkesworth and Powell 1980, Westercamp and Andreieff 1983) before the platform was uplifted. Volcanism returned to the northern arc about 7 Ma ago, shifted west of the original arc axis (Briden et al., 1979; Germa et al., 2011). From Dominica northward, a chain of young islands has been built up to 50 km west of the former arc. Most of this activity is younger than 3 Ma. By contrast, active volcanism has been continuous from Martinique southward over the past 25 Ma (Briden et al., 1979). In Martinique volcanism shifted westward, so the Oligocene-Miocene volcanic centers occur on the east and southeast, Miocene centers built the central axis of the island, and recent (<5 Ma) centers to the west (Germa et al., 2011). For the islands to the south, the older and younger volcanic centers overlap. This spatial migration of volcanic loci has been attributed to tectonic reorganization north of 15°N, after a series of aseismic ridges present on the Atlantic floor intersected the trench, momentarily halting subduction (Bouysse et al., 1990). This phenomenon is thus very important to understand as regards temporal changes in chemistry as the arc migrated into its present position. Depleted and enriched endmembers define mixing trends between older (>7 Ma) and younger (<7 Ma) lavas in Martinique Island, which is seen as representative of the Lesser Antilles geochemical evolution (Labanieh et al., 2012).
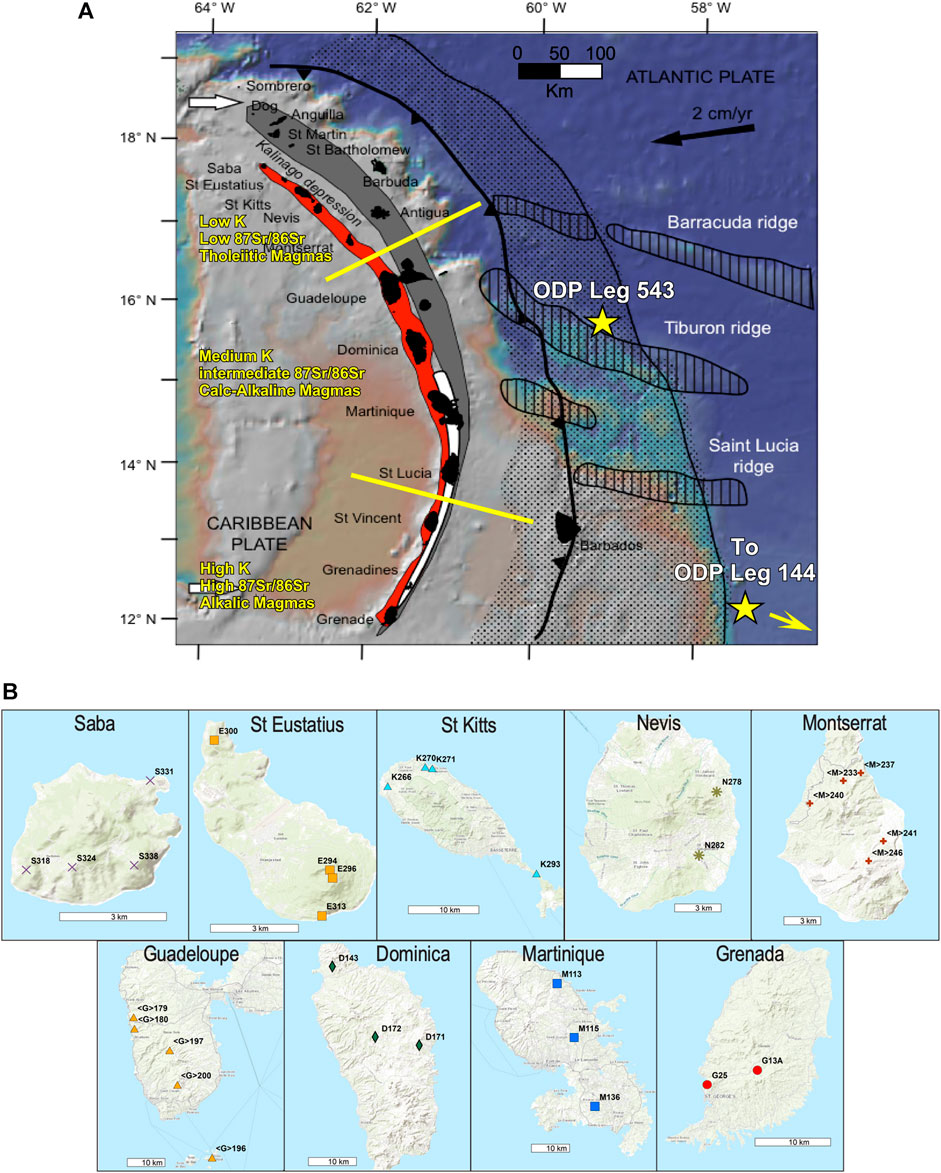
FIGURE 1. (A) Map of the Lesser Antilles Volcanic arc showing the recent arc (0–7 Ma; red), intermediate arc (7–15 Ma; white) and the older arc (15–25 Ma; gray) inferred extent. In the north a thin layer of oceanic sediments is expected to subduct whereas in the south we can see a thick layer of continental sediments (Barbados accretionary prism, dotted area) due to Influx from Amazon ± Orinoco River. Also shown are the aseismic St. Lucia, Tiburon and Barracuda Ridges as well as the location of DSDP Leg 78A Site 543 (yellow star). DSDP Leg 14 Site 144 is on the Demarara Rise located on the northern edge of South America between the Amazon and Orinoco deltas. Also noted are the chemical classifications of magmas for north, central and southern LAIA as denoted by White and Dupree (1986). Figure modified from Germa et al., 2011, DuFrane et al., 2009 and Davidson 1987. (B) Detailed map of sampling locations for rocks collected by F. Nagle and J. Stipp during their 1969 field campaign. Precise locations are based on a highly detailed hand drawn map and associated field notes created by Nagle. Some sampling locations are listed in Nagle et al. (1976).
A significant body of early work in the Lesser Antilles describes and documents its major element systematics, and early studies using trace elements in conjunction with radiogenic isotopes discussed the possible role of sediments (Cawthorne et al., 1973; Sigurdsson et al., 1973; Arculus 1976; Brown et al., 1977; Hawkesworth and Powell 1980; White and Dupree 1986; White and Durpe. 1986; White and Durpe. 1986; Davidson 1987; Hu et al., 2021). More recent trace element and isotopic studies have focused on specific islands or individual volcanic centers, seeking to resolve the nature of the various source contributions to Lesser Antilles lavas. Radiogenic isotope signatures of Antilles lavas tend to be enriched in Sr and Pb in the southern and central arc and decrease to the north (Hawkesworth and Powell 1980; Davidson 1987). This strongly suggests the incorporation of a continentally derived crustal component into Lesser Antilles parental magmas, likely derived from the extensive sedimentary output of the Orinoco basin with diminishing continental sediment input in the northern arc. A challenge in evaluating this hypothesis is the relatively limited sampling of the sedimentary columns outboard of the Antilles arc: only DSDP Leg 78A, Hole 543 (hereafter referred to as Site 543) samples the sedimentary column directly outboard of the central Lesser Antilles (see Figure 1A). Comprehensive data for Orinoco-derived sediments now exists, which should be comparable to the sediment compositions outboard of Grenada (DSDP Leg 14, Hole 144; Carpentier et al., 2008; Caerpentier et al., 2009, hereafter referred to as Site 144). In the north, a much thinner sequence of subducted pelagic sediment (see Westbrook et al., 1984) should yield chemical signatures distinguishable from those to the south. Plank and Langmuir (1998) produced a compositional profile of Lesser Antilles incoming sediments based only on samples from Site 543. A comparable profile for Site 144 is necessary to define compositional endmembers for the incoming sedimentary column along the arc, and thereby to better characterize mixing between the pelagic and terrigenous endmembers.
The continental signatures in Lesser Antilles lavas have been ascribed to relatively low degree inputs of sediment melts, but these early models failed to reproduce the observed trace element signatures without the addition of other components. Subsequent radiogenic isotope and trace element studies seem to confirm early results that suggest small amounts (∼2%) of sediment melt are likely involved in the generation of some Lesser Antilles magmas (White and Dupree 1986; Davidson 1987). Potassium isotopes from Martinique support this view suggesting that no more than 5% sediment melt is involved in the generation of LAIA magmas (Hu et al., 2021). In other locations, fluid additions are the dominant slab inputs proposed (Carpentier et al., 2008; Carpentier et al., 2009; DuFrane et al., 2009; Labanieh et al., 2010, Labanieh et al., 2012). These studies evoke differing sources of sediment to explain the chemical variation seen between volcanics in the northern and southern Lesser Antilles. Most recently, Cooper et al. (2020) suggest that serpentine is needed to produce higher fluid inputs and higher B isotopic values (up to 10‰ seen in the central LAIA including St. Vincent), however data from ODP sites 671 and 672 show δ11B in excess of ∼40‰ as part of the Barbados ridge (You et al., 1993). Based on the wide ranges of several stable isotopic systems (δ11B, δ18O, d δ34S and δ7Li), Bouvier et al. (2008), Bouvier et al. (2010) suggested that some combination of serpentine organic sediment and sediment derived fluid component is necessary to explain the diversity of melt inclusion compositions found in St Vincent magmas. The mobilization of fluids versus the generation of sediment melts is still a topic of debate but it appears likely that sediment melt does play at least some role in element variation during the generation of magmas at several subduction zones (Turner and Langmuir 2022) whereas fluids derived from sediment and the dehydration of specific mineral phases are also well known to contribute a bevy of elements to magmas.
We possess a comprehensive suite of volcanic rocks collected along the length of the Lesser Antilles arc. This whole rock suite permits us to examine along-arc trace element systematics. We also present a comprehensive FME dataset to build on past work and elucidate on the sources and petrogenesis of these lavas.
Materials and methods
This work examines volcanic rocks from the Lesser Antilles collected by the late Dr. F. Nagle and Dr. J. J. Stipp during a summer field campaign in 1969. This suite of rocks samples nearly every accessible volcanic center in the Lesser Antilles that has been active since the late Cenozoic and exposed as of the date of sampling. Some of these rocks are either now buried under newer flows or have become inaccessible due to construction and urbanization on some of the islands. Therefore, some of the rocks in this suite are no longer available for collection. This work examines a subset of this suite (Figure 1B). Samples were chosen to be representative of the geographic and chronologic variation along the modern, active LAIA. While there is significant chronologic variation in the Lesser Antilles, especially in the north, this study addresses only the recent (<7 Ma) part of the LAIA with samples from Grenada, Martinique, Dominica, Guadeloupe Montserrat, St. Kitts, Nevis, St Eustatius, and Saba. Samples were inspected for freshness and cut to remove any exterior alteration before being crushed in a 99.5% pure alumina ball mill (SPEX Sample Prep) and sieved prior to chemical analysis. All rocks were then characterized for major and trace elements, and most were analyzed secondarily for FME including Boron.
Major element analyses
Concentrations of major elements were measured on an ARL direct-current plasma emission spectrometry (DCP-OES) system or a Perkin Elmer Avio 200 ICP-OES in USF’s Center for Geochemical Analysis. All powdered samples were digested following LiBO2 fluxed-fusion methodologies (modified from Peterson and Ryan. 2009). For DCP analysis Germanium was added to each sample as an internal standard while ICP analysis added Ge in line via an ESI FAST sample introduction system. All samples were run along with natural standards used as both calibration and run check accuracy and drift monitors. Accuracy and precision are reported in Supplementary Table SB (DCP-OES) and Supplementary Table SC (ICP-OES). Errors on both instruments are typically less than 5% for elements above 1 wt% and less than 10% for minor elements.
Trace constituents
Trace element concentrations were measured on a Perkin-Elmer Elan DRC quadrupole ICP-MS system of USF’s Center for Geochemical Analysis, which includes a Dynamic Reaction Cell system for broad-spectrum, low-level trace metal analyses. We employed standard HF-HNO3 digestion techniques (see Kelley et al., 2003 for a full description). The reaction cell technology permits direct measurement of As and Sb in the low ppb to ppt range irrespective of reagent usage on the instrument.
Boron and fluid mobile elements
Boron measurements were performed on separate digestions using the USF ICP-MS system. Often Cs ± As and Sb are measured as part of standard ICP-MS elemental analysis packages. If B is analyzed, it is generally examined separately, with Be and Li abundances, or along with B ± Li isotopes, in part because of the peculiar dissolution behavior of B (e.g., it can form volatile complexes in acidic aqueous solutions) but also due to early affiliations among these elements established by Ryan and Langmuir (1987), Ryan and Langmuir (1988), Ryan and Langmuir (1993), and Morris and coworkers (Morris et al., 1990), or because techniques for the measurement of B, Li, and Be isotopes require accurate abundance determinations. To measure B in LAIA samples we used Na2CO3 flux fusion methods following Ryan and Langmuir (1993), which are acceptable for samples containing >5 ppm B and avoided the use of the HF-HCl digestion of Ishikawa and Nakamura (1993); Ishikawa and Nakamura, (1994) due to possible chloride interferences. A dedicated B-free PFA front end assembly with a sapphire injector is used for B measurement and yields reproducible, low-blank analyses. All reagents were cleaned for B using sub-boiling distillation systems for H2O and acids. Acids and an aliquot of water were cleaned with Mannitol while a second aliquot of ammoniated water was cleaned via amberlite resin. Both waters and the nitric acid reagents were tested as blanks for B and all yielded sub ppb concentrations.
Na2CO3 digestions yielded excellent results for B on standards while other FME could be sample specific. Some FME are measurable in HF-HNO3 digestions (As, Cs, Rb and Sb) and were included in these analyses to compare to FME specific digestions (Supplementary Table SD). Therefore, we consistently measure As, Cs, Rb and Sb using both techniques. Regardless, repeated measurement on standard reference materials and samples from the Caribbean yield precision for B, Cs, and As, < 4% while Sb is lower in abundance and thus precision on measurement is ±7%. Errors versus CRM JB-3 and USGS BHVO-1 are 10% for As, ∼2% for B and Cs and 16% for Sb (Supplementary Table SE).
Results
In general, all the rocks chosen for analysis from the examined islands included plagioclase and clinopyroxene phenocrysts in varying proportions. Olivine was observed in few samples, and when it was identified it was commonly resorbed or rimmed. In Caribbean samples, including some from our suite which were described in Nagle et al. (1976), hornblende is reported as an intermittent phenocryst phase.
Variation of major elements
Along the arc SiO2 ranges from close to 45 wt% to nearly 70 wt% (Figure 2; Table 1). Interestingly the island of Martinique shows a greater range in composition than any other island in SiO2, ranging from 48.5 to 67.4 wt%. In Martinique this corresponds with the variation of other major elements, and likely reflect a combination of differentiation and magma mixing (Germa et al., 2011). Some of the other islands also display what appear to be differentiation trends (Guadeloupe, Montserrat, Nevis and St. Kitts), while other islands (St. Eustatius and Saba) show no obvious correlations with SiO2. Atypical major element compositions have been well documented in Grenada lavas (Sigurdsson et al., 1973; Brown et al., 1977; Hawkesworth and Powell 1980) and unsurprisingly our Grenada samples have the lowest SiO2 of any of the islands studied here.
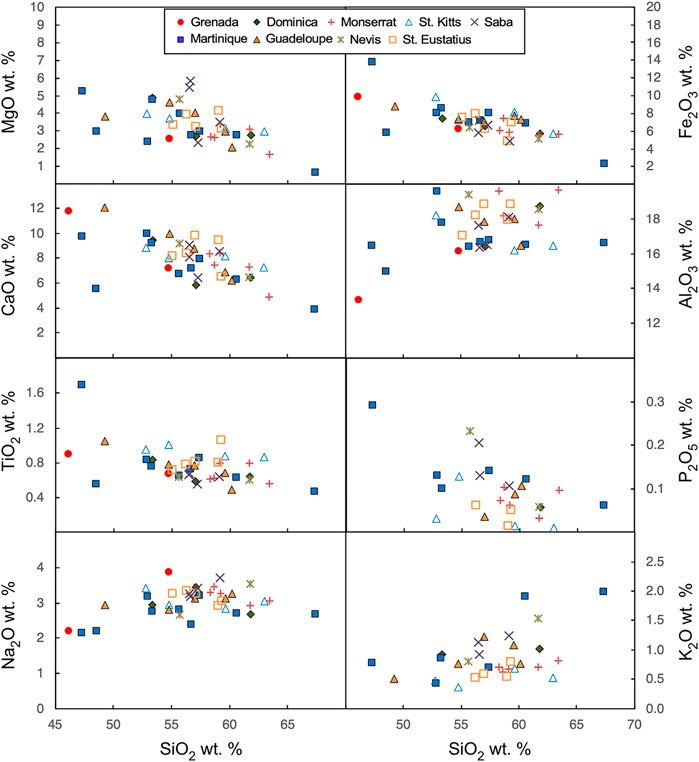
FIGURE 2. Major element variation for the Lesser Antilles. Data from this study is combined with that of Germa et al., 2010, Germa et al., 2011, and Labanieh et al., 2010, Labanieh et al., 2012 for Martinique. LAIA samples form a quasi-continuous suite, whereas specific islands on to themselves do not necessarily display differentiation trends.
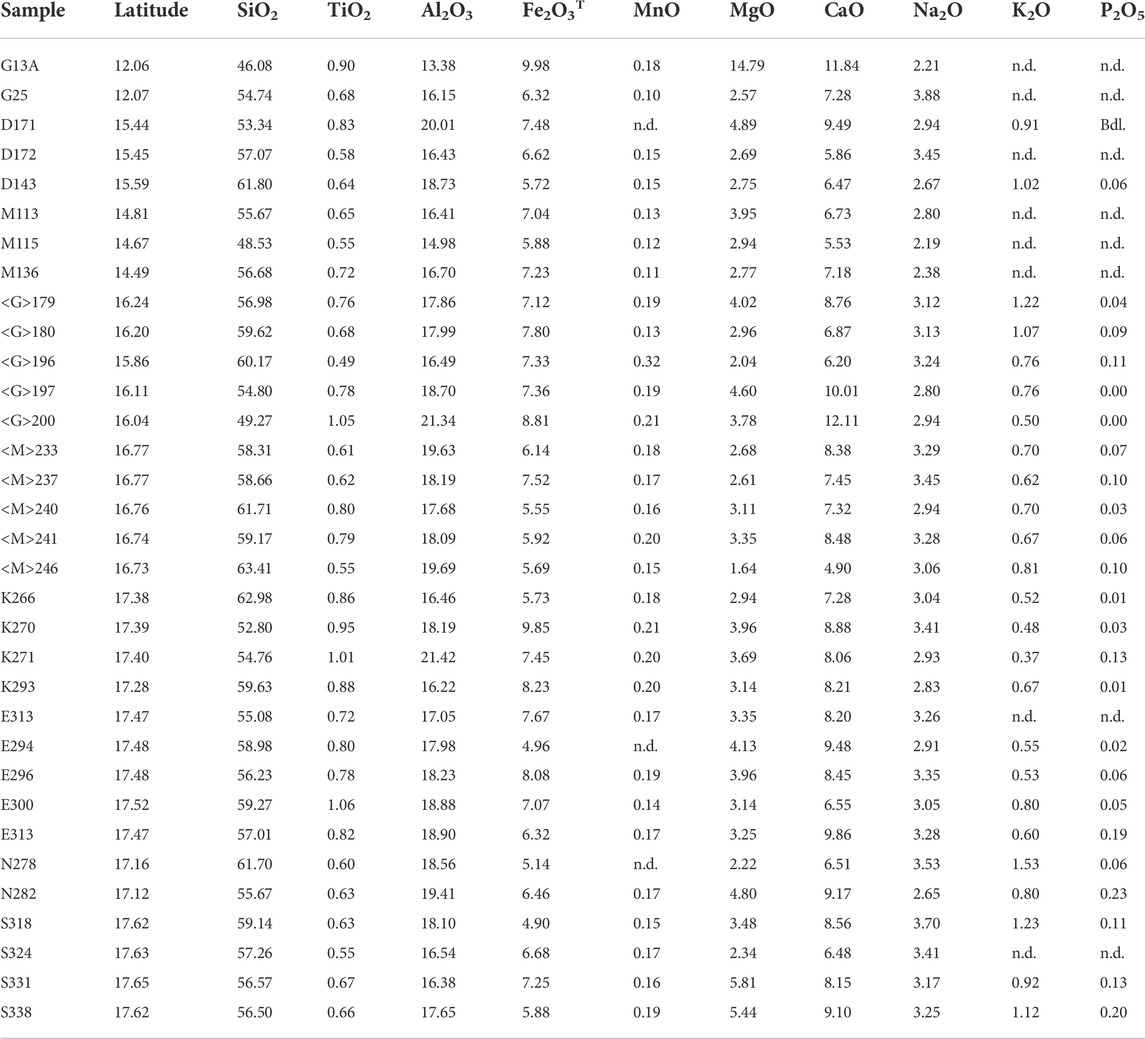
TABLE 1. Major element abundances of selected samples from the LAIA. Major elements were measured either on an ARL DCP-OES or a Perkin Elmer Avio 200 ICP-OES after digestion by LiBO2 flux fusion. n.d.-no data obtained, bdl.—data was below detection limit for this methodology (G, Grenada, D, Dominica, M, Martinique, <G>, Guadeloupe, <M>, Montserrat, K, St. Kitts, E, St. Eustatius, N, Nevis, S, Saba).
If we consider our along-the-arc sampling as a single suite, several relationships become apparent. First, MgO correlates fairly well with SiO2, becoming more variable at lower SiO2. The overall trend extends to an MgO content of roughly 7 wt% at 45 wt% SiO2. We suggest that 7 wt% MgO generally reflects primitive melts in the arc as a whole, in accord with prior work (Brown et al., 1977; Westercamp 1988; Bezard et al., 2014). However, some significantly higher MgO lavas have been reported here (Sigurdsson et al., 1973; Defant et al., 2001; DuFrane et al., 2009; Bouvier et al., 2010; Melekhova et al., 2015). Higher MgO whole rock occurrences tend to be rare and often associated with xenolith bearing magmas or atypical alkalic magmas such as those found in Grenada.
CaO forms a tight array with SiO2 in the modern arc, and trends back to approximately 12 wt% CaO at 45 wt% SiO2. Some specific islands (St. Eustatius, Saba and to some extent St. Kitts) do not follow a clear differentiation trend with respect to CaO. This may be in part due to our sample selection of the visually most primitive rocks from each island but given the measured composition of any given island we would not expect to see large variations in CaO, MgO & TiO2 over a 5% range in silica due to crystallization alone. Interestingly, both St. Eustatius and Saba show positive correlations between K2O and SiO2, but the variation is quite small. Iron also appears to be negatively correlated to SiO2 both along the full arc and in each island studied. These trends are largely consistent with magmatic differentiation, although in some cases inconsistent with crystallization of the mineral phases found in most of these lavas.
Trace elements
We find systematic along-arc variations in most trace elements. Systematic variation of K2O and 87Sr/86Sr have been well documented in the Lesser Antilles (Brown et al., 1977; Hawkesworth and Powell 1980; Davidson, 1987; Bouysse et al., 1990; DuFrane et al., 2009). Despite considerable scatter in previously collected data (GEOROC Database), Sr concentrations vary little in our samples from the recent arc, except for Grenada and Nevis (Figure 3; Supplementary Table SA). However, Sr/Th ratios in our samples are generally higher in the northern islands, while from Montserrat southward Sr/Th and Sr are both reasonably constant. These patterns suggest that Sr concentrations are not necessarily correlated with 87Sr/86Sr as in previous work. Grenada does show higher Sr concentrations and slightly higher Sr/Ce values, but still lower than is seen in the northern islands. Higher Sr/Ce has been found to be correlated with high 87Sr/86Sr (Hawkesworth and Powell 1980). However, we find Sr/Ce as well as Sr/Th to be higher overall in the northern part of the arc, which has low 87Sr/86Sr (Hawkesworth and Powell 1980; Davidson 1987; DuFrane et al., 2009; Labanieh et al., 2010).
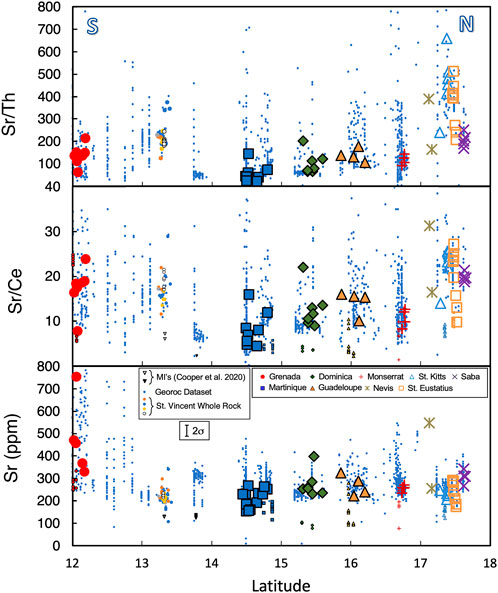
FIGURE 3. Along arc variation of Sr, Sr/Ce and Sr/Th for LAVA samples studied. Sr concentrations are elevated in Grenada while both Sr/Ce and Sr/Th are significantly higher in samples north of Montserrat. Higher Sr/Ce is expected to be correlated with high 87Sr/86Sr (Hawkesworth and Powell 1980), which if true, would make our data contradictory to previous findings (Hawkesworth and Powell 1980; Davidson 1987; DuFrane 2009). Error for Sr variation is shown, and the reader is referred to Supplementary Table SB through D for all analytical uncertainties. Data for St. Vincent (various colored closed and open circles at ∼13.3oN Latitude) are from Lewis 1973, Bardintzeff 1992 and Heath et al., 1998; Melt inclusion data for each island from Cooper et al. (2020) are shown as small versions of the same symbol shapes and colors except as otherwise noted. Small blue dots represent data previously published, extracted from a precompiled expert dataset downloaded from https://georoc.mpch-mainz.gwdg.de//georoc/with references therein.
Variations in La/Sm ratios have been used to document sediment melting, while elevated Ba/La and U/Th are said to indicate fluid additions from the slab via sediment dehydration (Patiño et al., 2000; Plank et al., 2002; Carr et al., 2003; Elliot 2003). Figure 4 plots La/Sm, Ba/La, and U/Th along the length of the arc. The highest La/Sm ratios are in Grenada, and La/Sm decreases systematically northward in the modern arc. Grenada also shows the highest Ni and Cr values along the arc, consistent with higher degrees of mantle melting (Supplementary Table SA). Ba/La as well as U/Th are enriched in samples from north of Montserrat whereas Ba/La is only slightly higher than Grenada and Montserrat in the central islands of Martinique and Dominica. U/Th ratios are comparatively low in these same central islands. When we plot Sm vs. La in Figure 5, LAIA samples are positively correlated both with each other and with the various reported components of local trench sediments (Plank and Langmuir 1998; Carpentier 2007). While compositionally the Grenada whole rocks are largely similar to their associated melt inclusions, the melting curve of Bouvier et al. (2010) does not seem to explain most of these data, so it is likely that differentiation and/or mixing of mantle with varying sediment sources are primarily responsible for the LAIA data array.
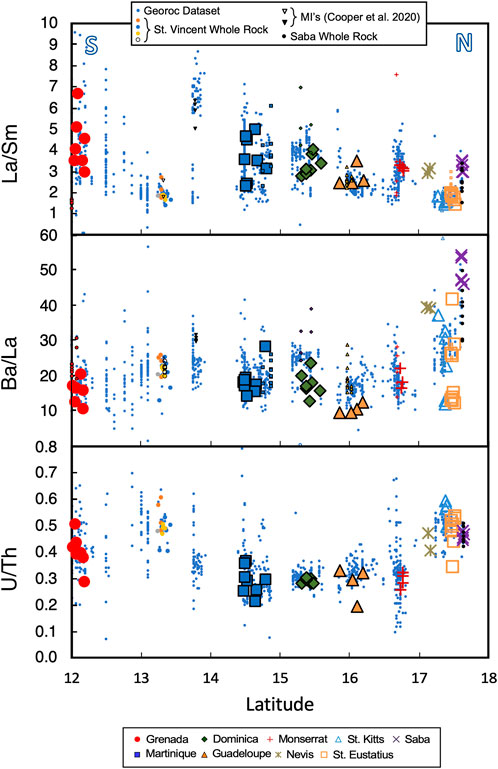
FIGURE 4. Variation of element ratios typically used as measures of subducted sediment enrichment applied to the Lesser Antilles Volcanic Arc. Ratios of Ba/La and U/Th are typically low in the central arc and quite high in the recent northern islands, suggesting higher sediment recycling for the northern arc despite much thicker accretionary wedge in the south. La/Sm tends to decrease from the south to the north. Data points for Saba from Defant et al., 2001, otherwise symbols are as in Figure 3 or as noted.
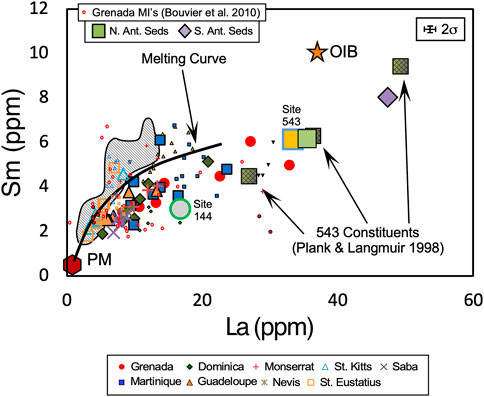
FIGURE 5. Lanthanum versus Samarium for whole rock LAIA samples (this work) along with melt inclusions of Cooper et al. (2020) (symbols are as in Figure 3) and end member compositions of Primitive Mantle (PM) (red hexagon), OIB (orange star) from both Sun and McDonough, (1995). N. Antilles sediment (light green square), S. Antilles sediment (purple diamond) of Plank and Langmuir 1998. Average composition of sediments from ODP Leg 543 (large orange and blue square) and ODP Leg 144 (gray and green circle) from Carpentier (2007). Also plotted are the composition of red clay, zeolite clay and Fe rich carbonate clay from ODP leg 543 (Plank and Langmuir 1998) as well as the sediment melting curve of Bouvier et al. (2010) along with Grenada MI’s (small red circles) and the field of St. Vincent MI’s.
Fluid mobile elements
B/Nb and B/La are all highly elevated in the central islands of Martinique and Dominica (Figure 6). Boron contents are very low in islands north of Guadeloupe, and Guadeloupe itself has low B contents, but intermediate B/La and B/Nb. Grenada is extremely low in B and has the lowest B/La and B/Nb in the arc. B, As, Rb and Cs are all reasonably well correlated to each other plotted against Ba showing essentially the same patterning on every island studied (Figure 7). FME from two main groups: a low FME group corresponding to Grenada and the northern islands extending to high Ba and a high FME group corresponding to the central islands of Martinique and Dominica. Interestingly, As is somewhat elevated in Grenada where two samples look more like the central group and three like the northern group (Figure 7). We also note that there are a few samples from both Martinique and Dominica that have low FME, and all samples analyzed thus far from Guadeloupe contain low FME. For Rb and Cs data from Sites 543 and 144 exists for the range of sediment thought to influence Caribbean Lavas and were studied in detail by Carpentier (2007) and Carpentier et al. (2008), Carpentier et al. (2009). Interestingly, Site 543 sediments as a whole plot with the higher FME group while Site 144 has both the low FME-Ba trend and high FME-Ba trend we see in our samples (Figure 7). Lithium also correlates with B suggesting that it too behaves as a FME in the LAIA system (Figure 8).

FIGURE 6. Variation of Boron, B/Nb and B/La for the Lesser Antilles Volcanic Arc. Boron is low in Grenada whole rock, enriched in the central islands, reaching peak concentration in St. Lucia and decreasing northward. Ba/Nb and Ba/La are very low for Grenada lava whereas these same ratios are slightly higher in the northern islands suggesting that both Nb and La are more enriched in the south. Symbols as in Figure 3 with the addition of Grenada and St. Vincent MI’s (small red and blue circles) from Bouvier et al. (2008), Bouvier et al. (2010).
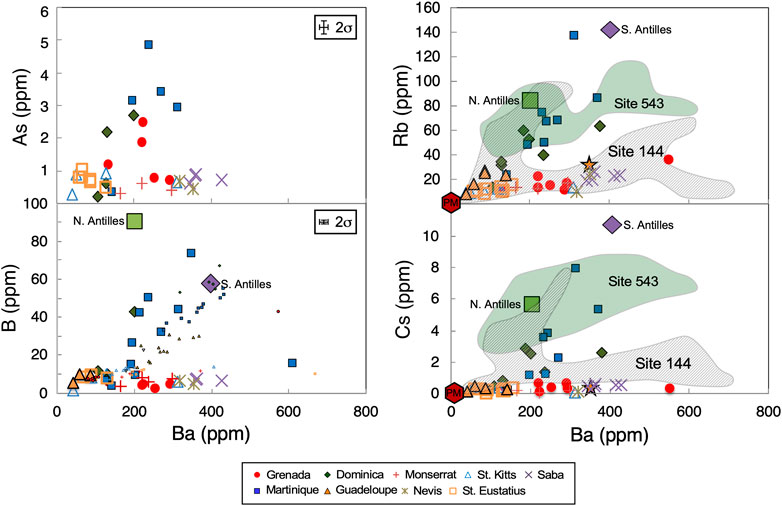
FIGURE 7. Fluid mobile elements (B, As, Cs and Rb) plotted against Barium. Enrichments in FME are obvious for Martinique and Dominica and are consistent among all FME. Grenada shows low B, Cs and Rb but anomalously higher As, whereas lavas from the northern LAIA are all depleted in FME but enriched in Barium. Fields shown for Cs and Rb plots are for individual Site 543 (green shading) and Site144 analysis (hatched) compiled by Carpentier (2007). Interestingly Site 144 sediments also have a diverging split between high Ba low Cs and Rb and higher Cs and Rb compositions. Error bars for the FME (2σ) are shown where variation is large enough to display, but Ba errors are consistent throughout plots.
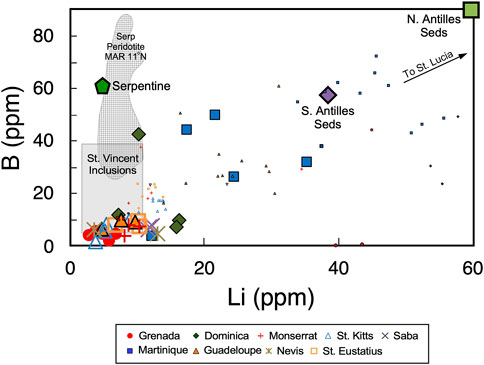
FIGURE 8. Variation of lithium and boron. Caribbean lava forms a roughly linear array for most samples whereas samples from Martinique and Dominica show both higher B and higher Li. Melt inclusions (Cooper et al., 2020) show similar trends but more variation with greater B and Li enrichment in the central islands. Average serpentine (green pentagram) is from Dessimoulie et al., 2020, and the field for serpentinized peridotite from the mid-Atlantic Ridge (MAR) is from Boschi et al. (2013). 2σ error are encompassed by the large symbols for whole rock (see Supplementary Tables SD, SE).
Discussion
The range of chemical variations in island arc lavas is usually due to a combination of contributions from the subducted material, the mantle wedge, and upper crust. Partial melting at arc settings has generally been attributed to the lowering of the peridotite solidus by volatiles supplied by these sources. Early trace element and isotopic studies on Lesser Antilles volcanics emphasized the examination of contributions from subducted sediment to the formation of the parental arc magmas (Arculus 1976; Hawkesworth and Powell 1980; White and Dupre 1986; Davidson 1987; Othman et al., 1989; see also Plank and Langmuir 1998). These works were motivated by the obvious variation in the magnitude of sediment inputs to the trench related to the substantial continental outflux from the nearby Orinoco River system. As well, radiogenic Sr and Pb decrease from south to north and suggesting decreasing continental sedimentary inputs along the arc. A challenge faced in these early studies was that information on the makeup of the sediments reaching the trench was limited to a single drill site (DSDP Leg 78, site 543), so possible variations in sediment sources and input fluxes in different segments of the arc were inadequately captured. More recent studies (Carpentier et al., 2008, Carpentier et al., 2009; DuFrane et al., 2009; Bouvier et al., 2010; Labanieh et al., 2012; Freymuth et al., 2016; Cooper et al., 2020) have benefited from newer drill site data for incoming sediments and have improved our understanding of the chemical role(s) of a compositionally variable incoming sedimentary pile. Revisiting the recovered core materials have shown that local sediment sourcing may be an important contributing factor to the diversity of Lesser Antilles magmatic products. Also, recent studies have suggested that a large portion of the aqueous fluids playing a role in melt generation might come from the subducted basalts (Labanieh et al., 2012), altered oceanic crust (Bouvier et al., 2008; Bouvier et al., 2010), and even serpentinites heterogeneously distributed in the descending slab (Bouvier et al., 2019; Cooper et al., 2020).
While many past studies have made use of lithophile trace element ratios and/or radiogenic isotopes, few have thus far taken full advantage of the fluid mobile elements (FME). As the FMEs are particularly sensitive to fluid-mediated slab contributions (as opposed to sediment or basaltic melt inputs: Elliott et al., 1997; Class et al., 2000; Kelemen et al., 2003) the respective roles of fluid addition versus sediment/crustal melting should be apparent both from FME concentrations as well as in FME/immobile element ratios (i.e., B/Be, B/Nb Cs/Th, As/Ce, Pb/Ce etc.). As part of our FME studies, in the sections that follow we examine relationships between LILE and immobile elements in the context of fluid vs. sediment additions to Lesser Antilles lavas. Correlations between FME and fluid sensitive LILE may indicate enrichments via metasomatic fluids derived from subducted sediment sources. Alternatively, if elements typically enriched in sediments are not evident, then fluids may have instead been sourced in altered and/or serpentinized oceanic crust (AOC).
Variation of fluid mobile elements and potential fluid enrichment
FME systematics in arc magmas are intertwined with competing models for the origins of these enrichments in sub-arc source regions. While the common presumption is that the elemental enrichments of arc magmas arise from the slab-derived fluid/melt fluxes that trigger melting in the sub-arc mantle, studies of FME systematics during forearc metamorphism indicate substantial early mobility for many of these species, pointing to the involvement of serpentinites, either as part of the down-going slab assemblage, or along the subduction interface, beginning in the forearc (e.g., Ulmer and Trommsdorf 1995; Bebout et al., 1999; Benton et al., 2001, Benton et al., 2004; Straub and Layne 2002; Savov et al., 2005; Savov et al., 2007; Hattori and Guillot 2007; Tonarini et al., 2007; Deschamps et al., 2010, Deschamps et al., 2011; Saumur et al., 2010; Ryan and Chauvel, 2014; Scambelluri et al., 2019; Cooper et al., 2020). Serpentinite-derived fluid inputs arise from its decomposition at T > 600°C, and the early redistribution of FMEs from the slab into wedge-derived serpentines in the subduction channel is largely unaccounted for (e.g., large fractions of slab B, and to a lesser extent As and Cs, appear to be entrained in forming wedge serpentines during shallow fluid releases; Benton et al., 2001; Savov et al., 2005; Savov et al., 2007; Hattori and Guillot 2007; Deschamps et al., 2011). Lithium, which is compatible in serpentine minerals, also shows greater mobility at higher temperatures (Scambelluri et al., 2004; Benton et al., 2004; Marschall et al., 2007) and therefore correlation with FME are expected if it is released into the subduction system by fluids at high temperature (Walker et al., 2009).
We have examined comparative B and FME systematics in our LAIA whole rocks along the arc. Boron is highest in the central part of the LAIA and generally quite low in both the north and south. Boron concentrations seems to peak in whole rock samples from Martinique, where concentrations often exceed 15 ppm (Figure 6). B/Nb ratios are also highest in the central part of the arc, in accord with prior findings (e.g., Cooper et al., 2020). Enrichments in As, Cs and Rb as well as Li can also be seen in the lavas from Martinique and Dominica (Figures 7, 8). Combined, the higher B, Li, As and other FME abundances are suggestive of a serpentinite-originating fluid source in the central arc. Interestingly, the central Lesser Antilles islands (Dominica, Guadeloupe, Martinique) have long been linked to higher magma production rates and higher fluid fluxes from the down-going plate (Wadge and Shepard, 1984; Lahitte et al., 2012). These higher fluxes might explain the higher FME abundances in the central islands with respect to the north and south parts of the arc.
B and FME are generally low in lavas from Grenada. However, rocks from Grenada contain significant As. We would expect B and As to correlate if serpentinites were involved (Savov et al., 2005; Kodolanyi et al., 2012; Scambelluri, et al., 2019). Low Li, Cs and Rb in the Grenada lavas may indicate that As may be inherited from some other source, or that early fluid loss from the down-going slab preferentially removed B and other species or the As enrichment is not due to fluid enrichment. Previous studies of melt inclusions in Grenada generally do not have higher B than our whole rocks (as compared to the central arc, Figure 7) so while it is plausible that serpentine is involved here with preferential loss of B (and other FME species), there is no clear evidence one way or the other. We should be careful to point out that any higher B magmas in Grenada are from highly silicic melt inclusions (Cooper et al., 2020) or very low silica melt inclusions (Bouvier et al., 2010) whereas most Grenada inclusions have <20 ppm B. We also note that our whole rocks are in good agreement with the andesitic inclusions studied by Cooper et al. (2020). Grenada may therefore represent a special case, but we surmise that our rocks record additions from an As-enriched component, possibly fluids heavily influenced by Orinoco sediments (e.g., Hattori and Guillot, 2007).
B and FME are low the northern group lavas. As discussed, in the south and central islands, high B, As and other FME may be indicative of serpentine (Ulmer and Trommsdorf 1995; Scambelluri, et al., 2004). However, in the northern part of the arc B and all other FME concentrations are low for whole rock and melt inclusions. This suggests that fluids are also low, inconsistent with enrichment from serpentine dehydration. Moreover, in the north there is limited evidence for forearc serpentinization (Bebout et al., 1999; Savov et al., 2005; Savov et al., 2007; Cooper et al., 2020). Barium concentrations are high and rival that of the central LAIA rocks but exhibit a very different trend where FME remain low in relation to Ba (Figure 7). This implies that Ba is either decoupled from FME somewhere in the magmatic system or not modified by fluids in the northern segment of LAIA but is instead enriched by another process.
Fluid mobile elements vs. large ion lithophile elements
Comparisons of FME to the enriched LILE can help resolve sediment- or ocean-crust derived inputs from fluid-mediated inputs, and identify linkages between likely fluxes (e.g., a fluid derived from sediments vs. a sediment melt: see Class et al., 2000). LILE and FME systematics are different in the northern part of the arc than in the south, and both are also distinct from the central parts of the arc. FME concentrations are low north of Montserrat, despite high Ba contents, a feature often assumed to be related to fluid inputs (Carr. 1984; Reagan et al., 1994; Elliott et al., 1997; Patiño et al., 2000; Walker et al., 2001; Plank et al., 2002, Carr et al., 2003, Elliott 2003; Jicha et al., 2004; and others). If certain LILE such as Ba are elevated by fluid enrichment, then one might expect to see correlations between Ba and FME along arc, but this is not observed.
Data for B from endmember sediment compositions exists for northern and southern Lesser Antilles sediments (Plank 2014). When plotted against our LAIA samples, these suggest that B/Be ratios for the central islands could be influenced significantly by sediments (Figure 9). However, in the central LAIA Ba/La ratios are too high for most samples to be controlled by sediment enrichment alone. Instead, we suggest some combination of serpentine-driven fluid flux plus sediment melt inputs as responsible for Martinique and Dominica. Such a model reconciles the FME, B/Be and Ba/La systematics of the central islands and is consistent with the findings of Cooper et al. (2020). In the northern islands Ba/La is very high while B/Be and FME enrichments are low (Figure 9). The LAIA samples appear to splay in two directions, with central LAIA samples displaced towards higher B/Be and northern arc samples towards high Ba/La. Again, if we only invoke fluids to control the behavior of both B as an FME and Ba as a fluid sensitive LILE, then we should see a correlation between FME, Ba and Ba ratios in all samples studied. Instead, we see such correlations in the central arc, but not in the northern segment of LAIA. Therefore, we suggest that Ba is decoupled from the FME, and its behavior is not controlled entirely by slab fluid additions here.
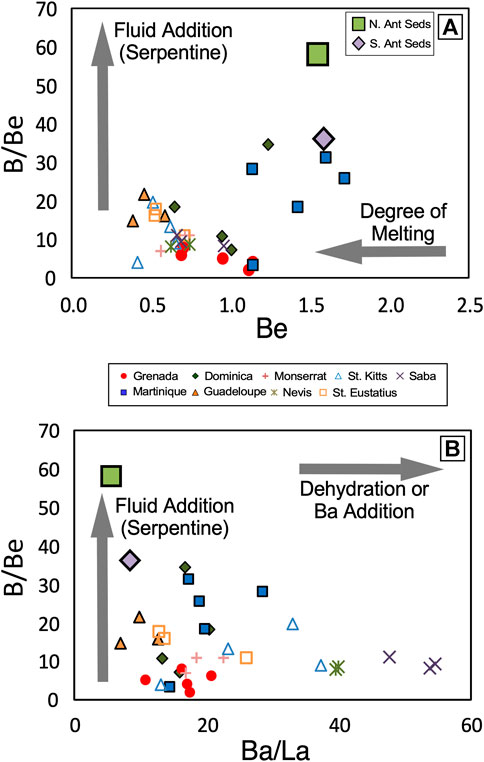
FIGURE 9. Plot of B/Be versus Be (A) and versus Ba/La ratios (B). Higher B/Be is expected from increased slab fluid addition (Hochstaedter et al., 1996), whereas serpentines have B/Be = 100–7,000 (Savov et al., 2005; Dessimoulie et al., 2020). Degree of melting will effectively dilute Be concentration and are synonymous to La/Sm systematics (Ryan et al., 1996). Conversely increased Ba/La is thought to be due to higher mantle metasomatism or more simply the dehydration of subducted sediments but is at odds with fluid addition (Hochstaedter et al., 1996; Elliott 2003; Bouvier et al., 2010). Our data show an increase in both B/Be and Ba/La as well as variable Be occurring concurrently.
Enrichment of barium
Analysis of mafic lavas along arcs along the Pacific margin and in the South Sandwich Islands show systematic behavior of B with respect to Ba (Figure 10; Ryan and Chauvel 2014). Ba/La and B/La ratios for such hot subduction systems lie between the expected ratio for a B-depleted sediment and that of the upper mantle. Other more “typical” arcs in terms of thermal structure (Kuriles, South Sandwich, Aleutians) span from low-B sediment endmember toward higher B ratios with little or no change in Ba/La. B/La covaries with Ba/La in the Central American arc, reaching peak values in the central portion of the arc, which various workers have suggested is due to enrichments via sediment-derived fluids (Patiño et al., 2000; Plank et al., 2002; Carr et al., 2003). By comparison, some LAIA samples show low Ba/La and low B/La ratios, akin to what is seen in the Trans-Mexican Volcanic Belt (Figure 10). Ryan and Chauvel (2014) attribute B and Ba systematics in Mexico to hot slab subduction. However, the subducting plate at the LAIA is much older (80–105 Ma, Carpentier et al., 2008), and presumably colder, and therefore not consistent with this hypothesis. Saba and Nevis samples tend to trend towards higher Ba/La and are more like magmas from Central America. Some samples from Martinique St. Kitts, St. Eustatius and Dominica have somewhat higher B/La ratios and thus plot more like south Sandwich or Aleutian lavas. These samples follow a trend resembling that of the bulk of St. Vincent and Grenada melt inclusions (Bouvier et al., 2010) although at least one St Vincent MI has high Ba/La and plots similarly to the Central American trend. We attribute these two trends to incorporation of a high B component, likely derived from subducted fluids, and incorporation of a high Ba component we believe to be derived from sediments delivered locally to the trench along the down-going slab. Other workers have used Ba/Th ratios to determine the influence of slab dehydration, which irrevocably adds fluids to the mantle wedge (Elliott 2003). Here we once again examine La/Sm and Ba/Th ratios to determine the effects of melting (Figure 11). We find that northern LAIA samples trend towards high Ba/Th, while southern and central samples form a tight array trending toward higher La/Sm. While we do not rule out the influence of fluid additions, for which we see evidence for throughout the LAIA, we also consider the effects of the melting of differing sediment compositions for magmas found along the arc.
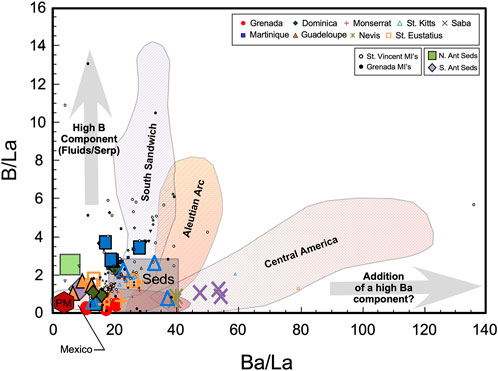
FIGURE 10. Ba/La versus B/La for LAIA samples investigated in this work compared to the compositional fields for Central America, the Aleutian Arc, the South Sandwich Arc and Mexico (Ryan and Chauvel 2014). Mantle (PM) as well as North and South Antilles Sediment (light green square and purple diamond) reported by Plank and Langmuir (1998) are shown. Serpentines average 12–37 for Ba/La and 105–2000 for average B/La (Savov et al., 2005; Dessimoulie et al., 2020) and may represent the high B component. Lesser Antilles compositions from this study and melt inclusion from St Vincent and Grenada (Bouvier et al., 2008 and Bouvier et al., 2010 respectively) show that some samples seem to be a mixture of mantle and a higher boron component while northern island seem to trend towards high Ba/La along a Central American “trajectory.” Figure modeled after Ryan and Chauvel 2014.
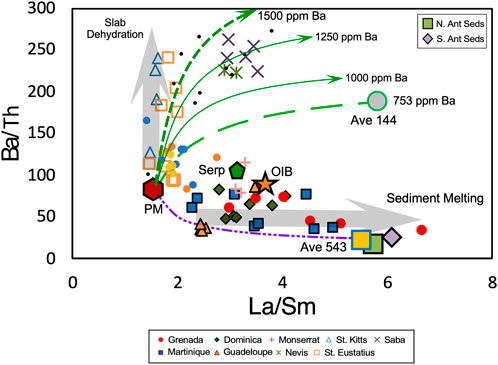
FIGURE 11. Plot showing trends caused by dehydration of the subducting slab (vertical trends) versus sediment melting (horizontal trend) in relation to LAIA samples. The Central Islands of Martinique, Dominica, Guadeloupe as well as the southern island of Grenada all plot along a proposed sediment melting trend while the northern islands of St Eustatius, St. Kitts Saba, and Nevis all plot at increasing Ba/Th. Both trends seem to originate from primitive mantle values (PM) and are compared to binary mixing curves (after Langmuir, 1978) trending to Site 543 (purple dot-dashed line) and Site 144 (green dashes). Compositions for the northern islands can be reproduced without requiring slab dehydration via binary mixing between mantle and a Site 144 “like” sediment but with elevated barium.
Carpentier (2007) analyzed sediments from Site 144 in detail, finding that several horizons in this section have >800 ppm Ba (average Ba = 753, 1σ =1,503). Two samples had more than 5,000 ppm Ba, while La and Th are roughly consistent throughout the core, averaging 15.9 ppm (1σ = 7.17) and 4.53 ppm (1σ = 2.52) respectively. Ba/La and Ba/Th in these samples are exceedingly high and stand out in the core stratigraphy (sample 144–36 has Ba/La = 650 and Ba/Th = 2,645; Carpentier, 2007). A low degree partial melt derived from this high-Ba sediment (or some addition of a sediment with comparably high Ba) could deliver substantial Ba to mantle melts without significant perturbation of other trace species. We tested this hypothesis by looking at binary mixing (equations from Langmuir, 1978) of a primitive mantle source with averaged sediments from Site 144, with and without added Ba, and assimilation of average Site 144 sediments during fractional crystallization (using AFC model of DePaolo, 1981). We find that for Ba/Th we can reproduce trends like those we see in our data by mixing with sediment that must be similar to the average of Site 144 in respect to Ba/Th and La/Sm, but with Ba concentrations between 1,000 and 1,500 (Figure 11). This binary mixing model fits our Caribbean data better than slab dehydration for both Saba and Nevis which extend toward higher La/Sm. For St. Eustatius and St. Kitts, the slab dehydration model (Elliott, 2003 and references therein) fits well, but these islands also fall close to the binary mixing curves and could also be satisfied by mixing with high Ba sediments. While we are not suggesting that sediments from Site 144 are subducting in the north, we do recognize that the northern part of LAIA is influenced by some similar composition (and henceforth Site 144 sediments and site 144-like sediments can be used synonymously). We modeled binary mixing between primitive mantle and Site 543 and find that Guadeloupe and Martinique generally follow this trend. Interestingly Montserrat lies between the “southern” grouping, extending toward Site 543 sediments along the sediment melting trend and the “northern” trend that is well described by our Site 144 + Ba mixing models. We find similar results when plotting Ba/La vs. Th/Yb (Figure 12). Here, binary mixing between primitive mantle and average Site 144 sediment does not completely satisfy our data. Mixing between primitive mantle and a Site144-like source containing variably high Ba, >1,000 ppm, can explain all our data. AFC models using the same primitive mantle and average Site 144 compositions fail to explain the high Ba/La lavas from the northern islands. Mixing between mantle and any Site 543 sediment composition cannot explain the bulk of our data and are inconsistent with high Ba/Th (>150; Figure 11). As seen in Figure 6, the northern islands show very high Ba while all the FME are low. Therefore, we suggest that Ba enrichments are not due exclusively to fluids but instead are derived primarily from low degree partial melt of specific sediment (Turner and Langmuir 2022). We suggest that the incorporation of a few percent of a high Ba sediment-derived melt controls much of the variation we see in the northern LAIA, while the central LAIA have higher FME, high Li and moderate to high Ba, more consistent with slab dehydration and fluid enrichment during mantle melting. Thus, low FME and low Li combined with high Ba suggest that these elements are ostensibly decoupled within specific parts of the arc. This inference is much more in line with past estimates of the percent contributions of sediments to the LAIA source regions (<2% melt and 5% fluid, (Davidson 1987; Westercamp 1988; Othman et al., 1989; Cousens et al., 1994; Singer et al., 2007; DuFrane et al., 2009; Bouvier et al., 2010; Labanieh et al., 2010).
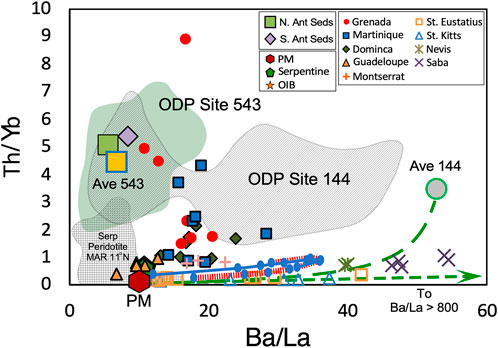
FIGURE 12. Abundance of Ba/La (fluid component) vs. Th/Yb (sediment component) for LAIA whole rock samples. Grenada samples follow some Martinique and Dominica whole rock samples. Samples from Nevis, St. Kitts, St. Eustatius and Saba follow a distinct high Ba - low Th trend towards an ultra-high Ba/La endmember. Samples from Guadeloupe, Montserrat and a few samples form Martinique and Guadeloupe also trend towards high Ba/La but with more elevated Th/Yb. Blue and red lines are AFC models (DePaolo, 1981) at F = 5% melting with varying r (red dashed line) and r = 0.8 with varying F (blue solid line) using a bulk D close to that described by Hu et al. (2021) after Labanieh et al. (2010) but extrapolated for the general assemblage of the arc with a starting composition equivalent to the average of all Site 144 sediments from Carpentier (2007). Green dashed line is binary mixing (Langmuir, 1978) of primitive mantle (PM; Sun and McDonough, 1989) with average Site 144 (Carpentier, 2007) and a Site 144 average component with 1,250 ppm Ba and Ba/La >800.
Deep sea drill cores from Central America (Site 495) and from the Izu Bonin arc (Site U1438) show that specific sediment layers can contain up to 4,000 ppm barium and 3,000 ppm strontium (Plank and Langmuir 1993; Plank and Langmuir 1998; Hickey Vargas et al., 2018). Plank and Langmuir (1998) identify these layers as diatomaceous mud and silicic clays. Patiño et al. (2000) reports mean carbonaceous sediments in Central America with more than 2000 ppm Ba and 1,500 ppm Sr (Ba/La = 244 and Sr/Th = 9,400). Potentially comparable calcareous and silicic clays have been recognized in the Barbados accretionary prism (Capet et al., 1986). The highest Ba sediment recorded in the Caribbean region is at the bottom of the Site 144 core (Carpentier, 2007). Moreover, Defant et al., 2001 suggested a geochemical role for biogenic sediment subducted under Saba however no outboard core has been collected north of Site 543. Therefore, while we have no direct evidence that specifically fingerprints biologic sediment influence throughout LAIA, we do note that it is in a tropical region with high amounts of biologic sedimentation so we should not rule it out. Recent molybdenum isotope data shows higher δ98/95Mo in the southern part of the arc associated with black shales from the middle of the Site 144 core (Freymuth et al., 2016). Based on its geographic location, it is not unreasonable to expect contributions of both calcareous and silicic sediments anywhere along the Lesser Antilles arc (Wilson and Costelloe 2011). Other potential high Ba components can be envisioned such as gypsum or assimilation of crust, but many can be ruled out based on other factors such as Sr isotopes which are low in the northern arc (Hawkesworth and Powell 1980; Davidson 1987).
While it is not the direct focus of this work to investigate the specific sediment type, we surmise that the sediment variability atop the down-going slab effects the element abundances and subsequently the element ratio variations along strike. We hypothesize that influences are more local and vary in both location and time. Central American magmas that show enrichments in specific trace element ratios beyond what one might expect based on fluid addition alone (Patiño et al., 2000; Plank et al., 2002; Atlas 2008; Ryan and Chauvel 2014) and we suggest this also is the case for the LAIA. Clearly more work needs to be done, but we stress that our northern arc samples record elevated Ba/La and Ba/Th signatures consistent with sediment containing higher barium not necessarily correlated with FME and fluid enrichments.
Variable sediment end member compositions
There is a significant variation in the composition of sediments along the LAIA that influence the compositions of erupted arc lavas. Few studies have examined the compositional variability of drilled sediments from the Caribbean region in detail (Plank and Langmuir 1998; Carpentier 2007). However, more recent studies have begun to examine the influence of individual components in or on top of the down-going plate (Carpentier et al., 2008; Tang et al., 2014; Freymuth et al., 2016). Components identified as contributing to the compositional signal include compositionally distinct sediment packages from the down-going slab, serpentinite, hydrated altered oceanic crust (Hawkesworth et al., 1993, Plank and Langmuir 1998, Class et al., 2000, Elliott 2003, Carpentier 2007, Carpentier et al., 2008, Bouvier et al., 2010, Tang et al., 2014, Freymuth et al., 2016, Scambelluri et al., 2019, Hu et al., 2021, and many others) and potentially additions from the overlying crust (Davidson. 1987; van Soest et al., 1998, van Soest et al., 2002). While it is not the intention of this work to specifically identify every component nor determine the precise percentage addition of specific components, we stress that these additions are not contributed equally along the length of LAIA. Instead, we suggest that the central arc and northern arc must have some significant differences in both sediment and fluid influence. Moreover, the southern arc while similar to the central arc in some respects, also shares some compositional similarities with the north.
Prior work has documented significant variation in sediment compositions within a single drill site outboard the LAIA. Plank and Langmuir (1998) examined the elemental makeup of zeolite clays, iron rich calcareous clays and red clays in Site 543. Their results suggest that the trace element compositions of these sediments at Site 543 generally varies by a factor of two or less. They list a single composition for “South Antilles” sediment that is generally consistent with the Site 543 sediment record. The averages they report also fall within the compositional field of Site 543 compiled by Carpentier (2007) and Carpentier et al. (2008). This suggests that the average Site 543 sediment values may be a good representation of one specific sediment endmember for the LAIA. Sediments from Site 144 on the Demerara Rise on the South American continental shelf have been proposed to contribute to the trench sediment inputs along the southern Antilles. Site 144 data from Carpentier (2007) shows 4-fold variations in Rb, Li and Th, a 10-fold for Zr, Cs, and Sm and even greater variations in Ba (100–9000ppm). By contrast, data for site 543 from Carpentier (2007) document Ba concentrations of 243 ± 135 ppm (1σ). So, depending on how one chooses to consider the composition of sediments reaching the trench outboard of the LAIA, the ratios commonly used to determine sediment influx may be markedly different. In Figure 5 the LAIA lavas overall plot between primitive mantle and a sediment composition like average Site 543 sediments but would require considerable sedimentary inputs to reconcile some LAIA compositions after the effects of crystallization are removed.
Further complications are indicated given that Grenada lavas plot beyond average Site 144 compositions in respect to both La and Sm, though we know that small degrees of partial melting can create significantly enriched compositions. The melting curve for La vs. Sm of Bouvier et al. (2010, Figure 5) therefore would require addition of a different component to explain the variation we see along the length of the arc. We have already shown that Grenada samples are enriched with respect to arsenic as are samples from Dominica and Martinique. Boron is low in Grenada but high in the central islands. Serpentine is a known sink for both B, As and Li (Scambelluri et al., 2004; Savov et al., 2005; Deschamps et al., 2010; Kodolanyi et al., 2012; Ryan and Chauvel 2014; Scambelluri et al., 2019; Dessimoulie et al., 2020) and upon dehydration we would expect these ostensibly fluid mobile elements to be released and contribute to the geochemical signature of the magmas produced. If slab temperatures are high (>400 °C) we would also expect release of Li and Cs (Deschamps et al., 2010). While complete trace element data sets are scarce for marine serpentine and serpentine muds, ratios of Ba/La are generally low (∼12–37) and barium concentrations rarely exceed 2 ppm (Savov et al., 2005; Dessimoulie et al., 2020). Conversely B/Be tends to be very high averaging 1,200–2000 due to the high B concentrations in serpentine. When we examine serpentine as an endmember composition as compared to our data (Figures 8–13), we find that any addition from serpentine fails to reconcile the high Ba and Ba ratios seen in the north but is not prohibited in the central Antilles. Moreover, Bouvier et al. (2010) pointed out that serpentine is involved in the generation of magmas at St. Vincent, which is in general agreement with its Li and B concentrations (Figure 8) but inconsistent with the northern Antilles, and only marginally consistent with the samples for the central islands. Therefore, upon close examination, trace element ratios suggests that the influence of serpentine is at best variable along the arc and that invoking an average sediment or bulk sediment falls short of reconciling many compositions in the LAIA.
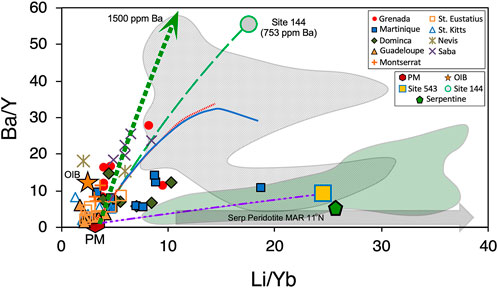
FIGURE 13. Variation of LAIA whole rock samples versus the fields of compositions for Site 543 and 144. Site 543 has high Li with respect to Ba while 144 extends to higher Ba. Note that average serpentines from Savov et al. (2005) plot close to site 543 on this diagram. Mixing endmember compositions of average Site 543 and 144 can reconcile many LAIA compositions but require both northern magmas and Grenada magmas to be mixed with a sediment component similar to each other and Site 144. Red and blue lines are AFC models and green mixing curves are as described in Figure 13 and require a high Ba component. Purple dot-dashed line is binary mixing between PM and Site 543.
Site 543 and 144 sediments show wide compositional ranges, so averaged values for either or both may be insufficient to fully describe their possible inputs (Figure 12). Calculated melting curves between the Average Site 144 sediments and primitive mantle fails to reconcile much of our whole rock data from the arc. However, one can envision additional melting curves between the mantle and a series of specific Site 144 compositions that could satisfy these constraints. Labanieh et al. (2010) also shows considerable variation in isotopic ratios where their mixing arrays point more towards Site144 sediments. Our results demonstrate that many central LAIA trace element ratios cannot be a mixture of primitive mantle and any Site 543 composition (in accord with Labanieh et al., 2010), but instead must include a component that is intermediate between 144 and 543 compositions, with low to moderate Ba/La and higher Th/Yb. We also note that in the northern and southern samples there is no evidence for significant fluid enrichment as there is in the central arc. Grenada samples exhibit high arsenic but are otherwise low in FME and Li similar to the northern islands. Variations in Li/Yb (Figure 13) generally support this finding with the exception of two rocks from Grenada which could have been influenced by serpentine additions and some fluids despite other FME depletions. However, magmas enriched by fluids from serpentine dehydration at higher temperature should also show lithium enrichments and also have elevated B and Cs (Deschamps et al., 2010; Figure 7), as do many samples from Martinique and Dominica. Whereas rocks from St. Eustatius northward have low FME, low Li and Li/Yb but high Ba and Ba ratios. Simply, if high Ba is inherited from fluids in the north it should be correlated with higher B and FME and likely high Li which it is not. Therefore, in our view based on whole rock, along arc trends in lava composition reflect varying subducted sediment and slab derived inputs that may have been influenced by preferential incorporation of specific components within the down going package. The geochemical signatures in the south have variable fluid enrichment and potentially influence from serpentine whereas the central islands exhibit high FME, high Ba ratios and high Li likely derived from fluid enrichment involving both sediments and serpentine. The northern islands of LAIA do not have high FME or lithium but do have high Ba and Ba ratios most likely inherited from a high Ba component within the down-going sediment pile.
Conclusion
The compositions of subduction zone magmas have been shown to be strongly influenced by a combination of sources, including but not limited to subducted sediment and subducted fluid inputs, both of which can vary along strike in a given arc. These components are delivered to mantle sources either as hydrous melts or as supercritical fluids in low percentages. These fluids can arise from hydrous subducting materials, including muds and clays in the down-going sediments, and serpentine in the subducted crust. Compositions of whole rocks along the Lesser Antilles displays variable fluid signatures that are consistent with higher fluid inputs in lavas from the central islands, lower fluid inputs to the north, and variable inputs in the southern islands. In Grenada, low B and Li and high As and Sr are consistent with serpentine dehydration and early, low temperature loss of B. The central islands show high B, high FME, high Li and high B/Be. When compared to Ba/La and Ba/Th these FME enrichments are consistent with sediment inputs ± serpentine dehydration from a source that can be tied back to the sediments of ODP site 543. To the north low B, low FME and low Li/Yb suggest that the observed high Ba/La, Ba/Th and Ba/Y ratios in these lavas were created without significant fluid addition, but instead via additions of small amounts of a highly enriched sediment component similar to a high Ba, “Site 144 like” sediment (Ba ≥ 1,250 ppm). We suggest that for LAIA, variable enrichments of highly specific components in the down-going slab are preferentially added to mantle melts by fluid fluxing and sediment melting. Highly enriched components are recognizable in specific layers or horizons in sediment sections outboard of LAIA, and can contain up to 9,000 ppm Ba, with Ba/La ratios above 400. Our modeling suggests that mixing between the mantle and enriched sediment components is needed to reconcile the compositions we see in the northern LAIA. In the central islands, and to some extent southern islands, fluid addition from dehydration of sediments and serpentine, plus some addition from sediment melting is more consistent with the element variations we record. While we do not suggest that fluid-mediated slab additions are completely absent in the northern part of LAIA, we conclude that these influences are comparatively minor in contrast to the strong fluid signatures in the volcanic products from the larger islands of the central LAIA. Our findings indicate that we must look beyond global or even arc-length average compositions for trench sediments or crust, and instead consider individual components in subducting sediment sequences as possible endmembers.
Data availability statement
The original contributions presented in the study are included in the article/Supplementary Material, further inquiries can be directed to the corresponding author.
Author contributions
ZA: Main author. Contributed to all aspects of this work including intellectual design, implementation writing, etc. AG: contributed to intellectual design, data interpretation and revising critically important content. BB: substantial contributions to the acquisition and analysis of data for the work. OM: substantial contributions to the acquisition, analysis and interpretation of data. AW: substantial contributions to the acquisition, analysis or interpretation of data for the work. JR: contributed to intellectual design, data interpretation and revised content.
Funding
This work was partially funded by the School of Geosciences at the University of South Florida with additional support from the USF Center for Geochemical Analysis.
Acknowledgments
This work is dedicated to the late Fred Nagle, without whom these samples would not have been collected and preserved for study. His notes and his humor within these have been invaluable to this work. Special thanks to Harold Wanless for access to Fred’s collection and to Grenville Draper for his input on the early part of this work. We also want to acknowledge the Center for Geochemical Analysis at the University of South Florida for use of the ICP’s and give thanks to several undergraduates who volunteered their time to work with these samples. We appreciate the constructive comments from H. Freymuth and A. S. Bouvier who helped review and improve this manuscript.
Conflict of interest
The authors declare that the research was conducted in the absence of any commercial or financial relationships that could be construed as a potential conflict of interest.
Publisher’s note
All claims expressed in this article are solely those of the authors and do not necessarily represent those of their affiliated organizations, or those of the publisher, the editors and the reviewers. Any product that may be evaluated in this article, or claim that may be made by its manufacturer, is not guaranteed or endorsed by the publisher.
Supplementary material
The Supplementary Material for this article can be found online at: https://www.frontiersin.org/articles/10.3389/feart.2022.782179/full#supplementary-material
References
Abers, G. A., Plank, T., and Hacker, B. R. (2003). The wet Nicaraguan slab. Geophys. Res. Lett. 30 (2), 70. doi:10.1029/2002gl015649
Arculus, R. J. (1976). Geology and geochemistry of the alkali basalt-andesite association of Grenada, Lesser Antilles Island arc. Geol. Soc. Am. Bull. 87, 612–624. doi:10.1130/0016-7606(1976)87<612:gagota>2.0.co;2
Atlas, Z. D. (2008). Volatiles in melt inclusions form Mexican and Nicaraguan volcanoes: Implications for complex degassing processes (Coral Gables, Florida: University of Miami), 131pp. Ph.D. Thesis.
Bardintzeff, J-M. (1992). Magma mixing processes in volcanic contexts, a thermodynamic approach with the examples of St Vincent Soufrière volcano, West lndies and Cerro Chiquito, Guatemala. Terra nova. 4, 553–556. doi:10.1111/j.1365-3121.1992.tb00597.x
Bebout, G. E., Ryan, J. G., and Leeman, W. P. (1993). B-Be systematics in subduction-related metamorphic rocks: Characterization of the subducted component. Geochimica Cosmochimica Acta 57, 2227–2237. doi:10.1016/0016-7037(93)90565-e
Bebout, G. E., Ryan, J. G., Leeman, W. P., and Bebout, A. E. (1999). Fractionation of trace elements by subduction-zone metamorphism: Effect of convergent-margin thermal evolution. Earth Planet. Sci. Lett. 171, 63–81. doi:10.1016/s0012-821x(99)00135-1
Benton, L. D., Ryan, J. G., and Savov, I. P. (2004). Lithium abundance and isotope systematics of forearc serpentinites, Conical Seamount, Mariana forearc: Insights into the mechanics of slab-mantle exchange during subduction. Geochem. Geophys. Geosyst. 5. doi:10.1029/2004GC000708
Benton, L. D., Ryan, J. G., and Tera, F. (2001). Boron isotope systematics of slab fluids as inferred from a serpentine seamount, Mariana forearc. Earth Planet. Sci. Lett. 187, 273. doi:10.1016/S0012-821X(01)00286-2
Bezard, R., Davidson, J. P., Turner, S., Macpherson, C. G., Lindsay, J. M., and Boyce, A. J. (2014). Assimilation of sediments embedded in the oceanic arc crust: Myth or reality? Earth Planet. Sci. Lett. 395, 51–60. doi:10.1016/j.epsl.2014.03.038
Boschi, C., Bonatti, E., Ligi, M., Brunelli, D., Cipriani, A., Dallai, L., et al. (2013). Serpentinization of mantle peridotites along an uplifted lithospheric section, Mid Atlantic Ridge at 11 N. Lithos 178, 3–23. doi:10.1016/j.lithos.2013.06.003
Bouvier, A. S., Deloule, E., and Métrich, N. (2010). Fluid inputs to magma sources of St. Vincent and Grenada (lesser Antilles): New insights from trace elements in olivine-hosted melt inclusions. J. Petrology 51 (8), 1597–1615. doi:10.1093/petrology/egq031
Bouvier, A.-S., Manzini, M., Rose-Koga, E. F., Nichols, A. R. L., and Baumgartner, L. P. (2019). Tracing of Cl input into the sub-arc mantle through the combined analysis of B, O and Cl isotopes in melt inclusions. Earth Planet. Sci. Lett. 507, 30–39. doi:10.1016/j.epsl.2018.11.036
Bouvier, A. S., Métrich, N., and Deloule, E. (2008). Slab-derived fluids in the magma sources of St. Vincent (lesser Antilles arc): Volatile and light element imprints. J. Petrology 49 (8), 1427–1448. doi:10.1093/petrology/egn031
Bouysse, P., Westercamp, D., and Andreieff, P. (1990). The Lesser Antilles island arc. Proc. Ocean Drill. Program, Sci. Results 110, 29–44.
Briden, J. C., Rex, D. C., Faller, A. M., and Tomblin, J. F. (1979). K-Ar geochronology and paleomagnetism of volcanic rocks in the lesser Antilles island arc. Philosophical Trans. R. Soc. Lond. 291 (1383), 485–528.
Brown, G. M., Holland, J. G., Sigurdsson, H., Tomblin, J. F., and Arculus, R. J. (1977). Geochemistry of the Lesser Antilles volcanic island arc. Geochim. Cosmochim. Acta 41, 785–801. doi:10.1016/0016-7037(77)90049-7
Cameron, B. I., Walker, J. A., Carr, M. J., Patino, L. C., Matias, O., and Feigenson, M. D. (2003). Flux versus decompression melting at stratovolcanoes in southeastern Guatemala. J. Volcanol. Geotherm. Res. 119, 21–50. doi:10.1016/s0377-0273(02)00304-9
Capet, X., Chamley, H., Beck, C., and Holtzapffel, T. (1986). “6. Clay mineralogy of sites 671 and 672, Barbados ridge accretionary complex and Atlantic abyssal plain: Paleoenvironmental and diagenetic implications 1,” in Proceedings of the Ocean Drilling Program: Scientific results. Editors J. C. Moore, and A. Mascle (College Station, TX), 110, 85.
Carpentier, M., Chauvel, C., and Mattielli, N. (2008). Pb-Nd isotopic constraints on sedimentary input into the Lesser Antilles arc system. Earth Planet. Sci. Lett. 272, 199–211. doi:10.1016/j.epsl.2008.04.036
Carpentier, M., Chauvel, C., Maury, R., and Mattielli, N. (2009). The “zircon effect” as recorded by the chemical and Hf isotopic compositions of Lesser Antilles forearc sediments. Earth Planet. Sci. Lett. 287, 86–99. doi:10.1016/j.epsl.2009.07.043
Carpentier, M. (2007). “Composition chimique des sédiments entrant dans la zone de subduction des Petites Antilles,” (Belgium: Université Joseph-Fourier-Grenoble I; Université Libre de Bruxelles). Doctoral dissertation.
Carr, M. J. (1984). Symmetrical and segmented variation of physical and geochemical characteristics of the Central American volcanic front. J. Volcanol. Geotherm. Res. 20, 231–252. doi:10.1016/0377-0273(84)90041-6
Carr, M. J., Feigenson, M. D., Patino, L. C., and Walker, J. A. (2003). Volcanism and geochemistry in central America: Progress and problems. Geophys. Monograph-American Geophys. Union 138, 153–174. doi:10.1029/138GM09
Cawthorne, G., Curran, E. B., and Arculus, R. J. (1973). A petrogenetic model for the origin of the calc-alkaline suite of Grenada, lesser Antilles. J. Petrology 14 (2), 327–337. doi:10.1093/petrology/14.2.327
Chaussidon, M., and Robert, F. (1998). 7Li/6Li and 11B/10B variations in chondrules from the Semarkona unequilibrated chondrite. Earth Planet. Sci. Lett. 164, 577–589. doi:10.1016/s0012-821x(98)00250-7
Class, C., Miller, D. M., Goldstein, S. L., and Langmuir, C. H. (2000). Distinguishing melt and fluid subduction components in umnak volcanics, Aleutian Arc. Geochem. Geophys. Geosyst. 1. paper no. 1999GC000010. doi:10.1029/1999gc000010
Cooper, G. F., Macpherson, C. G., Blundy, J. D., Maunder, B., Allen, R. W., Goes, S., et al. (2020). Variable water input controls evolution of the Lesser Antilles volcanic arc. Nature 582 (7813), 525–529. doi:10.1038/s41586-020-2407-5
Cousens, B. L., Allan, J. F., and Gorton, M. P. (1994). Subduction-modified pelagic sediments as the enriched component in back-arc basalts from the Japan Sea: Ocean Drilling Program Sites 797 and 794. Contr. Mineral. Pet. 117 (4), 421–434. doi:10.1007/bf00307275
Davidson, J. P. (1987). Crustal contamination versus subduction zone enrichment: Examples from the Lesser Antilles and implications for mantle source compositions of island arc volcanic rocks. Geochimica Cosmochimica Acta 51, 2185–2198. doi:10.1016/0016-7037(87)90268-7
Defant, M. J., Sherman, S., Maury, R. C., Bellon, H., De Boer, J., Davidson, J., et al. (2001). The geology, petrology, and petrogenesis of Saba island, lesser Antilles. J. Volcanol. Geotherm. Res. 107 (1-3), 87–111. doi:10.1016/s0377-0273(00)00268-7
DePaolo, D. J. (1981). Trace element and isotopic effects of combined wallrock assimilation and fractional crystallization. Earth Planet. Sci. Lett. 53 (2), 189–202. doi:10.1016/0012-821X(81)90153-9
Deschamps, F., Guillot, S., Godard, M., Andreani, M., and Hattori, K. (2011). Serpentinites act as sponges for fluid-mobile elements in abyssal and subduction zone environments. Terra nova. 23, 171–178. doi:10.1111/j.1365-3121.2011.00995.x
Deschamps, F., Guillot, S., Godard, M., Chauvel, C., Andreani, M., and Hattori, K. (2010). In situ characterization of serpentinites from forearc mantle wedges: Timing of serpentinization and behavior of fluid-mobile elements in subduction zones. Chem. Geol. 269, 262–277. doi:10.1016/j.chemgeo.2009.10.002
Dessimoulie, L., Delacour, A., Guillaume, D., Chevet, J., and Cottin, J. Y. (2020). Major and trace elements exchanges during fluid-rock interaction at ultraslow-spreading oceanic lithosphere: Example of the South West Indian Ridge (SWIR). Lithos 352, 105233. doi:10.1016/j.lithos.2019.105233
DuFrane, S. A., Turner, S., Dosseto, A., and van Soest, M. (2009). Reappraisal of fluid and sediment contributions to Lesser Antilles magmas. Chem. Geol. 265, 272–278. doi:10.1016/j.chemgeo.2009.03.030
Edwards, C. M. H., Morris, J. D., and Thirlwall, M. F. (1993). Separating mantle from slab signatures in arc lavas using B/Be and radiogenic isotope systematics. Nature 362, 530–533. doi:10.1038/362530a0
Elliott, T., Plank, T., Zindler, A., White, W., and Bourdon, B. (1997). Element transport from slab to volcanic front at the Mariana arc. J. Geophys. Res. 102, 14991–15019. doi:10.1029/97jb00788
Elliott, T. (2003). “Tracers of the slab,” in Inside the subduction factory. Editor J. Elier (United Kingdom: Geophysical Monograph Series), 138, 23–45.
Freymuth, H., Elliott, T., Van Soest, M., and Skora, S. (2016). Tracing subducted black shales in the Lesser Antilles arc using molybdenum isotope ratios. Geology 44 (12), 987–990. doi:10.1130/g38344.1
Gaetani, G. A., Grove, T. L., and Bryan, W. B. (1993). The influence of water on the petrogenesis of subductionrelated igneous rocks. Nature 365, 332–334. doi:10.1038/365332a0
Gaetani, G. A., and Grove, T. L. (2003). Experimental constraints on melt generation in the mantle wedge. Inside subduction Fact. 2003(138), 107–134. doi:10.1029/138gm07
Germa, A. (2008). “Evolution volcano-tectonique de l'île de la Martinique (arc insulaire des petites Antilles): Nouvelles contraintes géochronologiques et géomorphologiques,” (France: Université Paris Sud-Paris XI). Doctoral dissertation.
Germa, A., Quidelleur, X., Labanieh, S., Chauvel, C., and Lahitte, P. (2011). The volcanic evolution of Martinique Island: Insights from K–Ar dating into the Lesser Antilles arc migration since the Oligocene. J. Volcanol. Geotherm. Res. 208, 122–135. doi:10.1016/j.jvolgeores.2011.09.007
Germa, A., Quidelleur, X., Labanieh, S., Lahitte, P., and Chauvel, C. (2010). The eruptive history of Morne Jacob volcano (Martinique Island, French West Indies): Geochronology, geomorphology and geochemistry of the earliest volcanism in the recent Lesser Antilles arc. J. Volcanol. Geotherm. Res. 198 (3-4), 297–310. doi:10.1016/j.jvolgeores.2010.09.013
Grove, T. L., Chatterjee, N., Parman, S. W., and Médard, E. (2006). The influence of H2O on mantle wedge melting. Earth Planet. Sci. Lett. 249 (1-2), 74–89. doi:10.1016/j.epsl.2006.06.043
Grove, T. L., and Kinzler, R. J. (1986). Petrogenesis of andesites. Annu. Rev. Earth Planet. Sci. 14, 417–454. doi:10.1146/annurev.ea.14.050186.002221
Hattori, K. H., and Guillot, S. (2007). Geochemical character of serpentinites associated with high-to ultrahigh-pressure metamorphic rocks in the Alps, Cuba, and the Himalayas: Recycling of elements in subduction zones. Geochem. Geophys. Geosyst. 8 (9). doi:10.1029/2007gc001594
Hawkesworth, C. J., Gallagher, K., Hergt, J. M., and McDermott, F. (1993). Mantle and slab contributions in arc magmas. Annu. Rev. Earth Planet. Sci. 21, 175–204. doi:10.1146/annurev.ea.21.050193.001135
Hawkesworth, C. J., and Powell, M. (1980). Magma Genesis in the lesser Antilles Island Arc. Earth Planet. Sci. Lett. 51, 297–308. doi:10.1016/0012-821x(80)90212-5
Heath, E., Macdonald, R., Belkin, H., Hawkesworth, C., and Sigurdsson, H. (1998). Magmagenesis at soufriere volcano, St vincent, lesser Antilles arc. J. Petrology 39 (10), 1721–1764. doi:10.1093/petroj/39.10.1721
Hickey-Vargas, R., Yogodzinski, G. M., Ishizuka, O., McCarthy, A., Bizimis, M., Kusano, Y., et al. (2018). Origin of depleted basalts during subduction initiation and early development of the Izu-Bonin-Mariana island arc: Evidence from IODP expedition 351 site U1438, Amami-Sankaku basin. Geochimica Cosmochimica Acta 229, 85–111. doi:10.1016/j.gca.2018.03.007
Hochstaedter, A. F., Ryan, J. G., Luhr, J. F., and Hasenake, T. (1996). On B/Be ratios in the Mexican volcanic Belt. Geochimica Cosmochimica Acta 60, 613–628. doi:10.1016/0016-7037(95)00415-7
Hu, Y., Teng, F. Z., and Chauvel, C. (2021). Potassium isotopic evidence for sedimentary input to the mantle source of Lesser Antilles lavas. Geochimica Cosmochimica Acta 295, 98–111. doi:10.1016/j.gca.2020.12.013
Ishikawa, T., and Nakamua, E. (1994). Origin of the slab component in arc lavas from across-arc variation of B and Pb isotopes. Nature 370, 205–208. doi:10.1038/370205a0
Ishikawa, T., and Nakamura, E. (1993). Boron isotope systematics of marine sediments. Earth Planet. Sci. Lett. 117 (3-4), 567–580. doi:10.1016/0012-821x(93)90103-g
Jicha, B. R., Singer, B. S., Brophy, J. G., Fournelle, J. H., Johnson, C. M., Beard, B. L., et al. (2004). Variable impact of the subducted slab on aleutian island Arc magma sources: Evidence from Sr, Nd, Pb, and Hf isotopes and trace element abundances. J. Petrology 45 (9), 1845–1875. doi:10.1093/petrology/egh036
Johnson, M. C., and Plank, T. (1999). Dehydration and melting experiments constrain the fate of subducted sediments. Geochem. Geophys. Geosyst. 1 (1), 1007. doi:10.1029/1999GC000014
Kelemen, P. B., Yogodzinski, G. M., and Scholl, D. W. (2003). Along-strike variation in lavas of the Aleutian island arc: Implications for the genesis of high Mg# andesite and the continental crust. Inside subduction Fact. Geophys. Monogr. 138, 223–276. doi:10.1029/138GM11
Kelley, K. A., Plank, T., Ludden, J., and Staudigel, H. (2003). Composition of altered oceanic crust at ODP Sites 801 and 1149. Geochem. Geophys. Geosyst. 4 (6). doi:10.1029/2002GC000435
Kimura, J-I., and Yoshida, T. (2006). Contributions of slab fluid, mantle wedge and crust to the origin of quaternary lavas in the NE Japan arc. J. Petrology 47 (11), 2185–2232. doi:10.1093/petrology/egl041
Kodolányi, J., Pettke, T., Spandler, C., Kamber, B. S., and Gméling, K. (2012). Geochemistry of ocean floor and fore-arc serpentinites: Constraints on the ultramafic input to subduction zones. J. Petrology 53 (2), 235–270. doi:10.1093/petrology/egr058
Kushiro, I. (1972). Effect of water on the composition of magmas formed at high pressures. J. Petrology 13, 311–334. doi:10.1093/petrology/13.2.311
Kushiro, I. (1990). Partial melting of mantle wedge and evolution of island arc crust. J. Geophys. Res. 95, 15929–16015. doi:10.1029/jb095ib10p15929
Labanieh, S., Chauvel, C., Germa, A., Quidelleur, X., and Lewin, E. (2010). Isotopic hyperbolas constrain sources and processes under the Lesser Antilles arc. Earth Planet. Sci. Lett. 298, 35–46. doi:10.1016/j.epsl.2010.07.018
Labanieh, S., Chauvel, C., Germa, A., and Quidelleur, X. (2012). Martinique: A clear case for sediment melting and slab dehydration as a function of distance to the trench. J. Petrology 53 (12), 2441–2464. doi:10.1093/petrology/egs055
Lahitte, P., Samper, A., and Quidelleur, X. (2012). DEM-based reconstruction of southern Basse-Terre volcanoes (Guadeloupe archipelago, FWI): Contribution to the Lesser Antilles Arc construction rates and magma production. Geomorphology 136 (1), 148–164. doi:10.1016/j.geomorph.2011.04.008
Langmuir, C. H., Vocke, R. D., Hanson, G. N., and Hart, S. R. (1978). A general mixing equation with applications to Icelandic basalts. Earth Planet. Sci. Lett. 37 (3), 380–392. doi:10.1016/0012-821X(78)90053-5
Leeman, W. P., Carr, M. J., and Morris, J. D. (1994). Boron geochemistry of the central American Volcanic arc: Constraints on the Genesis of subduction-related magmas. Geochimica Cosmochimica Acta 68, 149–168. doi:10.1016/0016-7037(94)90453-7
Leeman, W. P., and Sisson, V. B. (1996). Geochemistry of boron and its implications for crustal and mantle processes. Boron, Mineralogy, Petrology Geochem. Rev. Mineralogy 33, 645–707.
Legendre, L., Philippon, M., Münch, P., Leticée, J. L., Noury, M., Maincent, G., et al. (2018). Trench bending initiation: Upper plate strain pattern and volcanism. Insights from the Lesser Antilles arc, St. Barthelemy Island, French West Indies. Tectonics 37, 2777–2797. doi:10.1029/2017TC004921
Lentz, R. C. F., McSween, H. Y., Ryan, J. G., and Riciputi, R. L. (2001). Water in Martian magmas: Clues from light lithophile elements in shergottite and nakhlite pyroxenes. Geochimica Cosmochimica Acta 65, 4551–4565. doi:10.1016/s0016-7037(01)00756-6
Lewis, J. F. (1973). Petrology of the ejected plutonic blocks of the soufriere volcano, St. Vincent, west indies. J. Petrology 14 (1), 81–112. doi:10.1093/petrology/14.1.81
London, D., Morgan, G. B., and Wolf, M. B. (1996). Boron in granitic rocks and their contact aureoles. Boron, Mineralogy, Petrology Geochem. Rev. Mineralogy 33, 299–330.
Marschall, H. R., Altherr, R., and Rüpke, L. (2007). Squeezing out the slab—modelling the release of Li, Be and B during progressive high-pressure metamorphism. Chem. Geol. 239 (3–4), 323–335. doi:10.1016/j.chemgeo.2006.08.008
Mascle, A., and Westercamp, D. (1983). Géologie d'Antigua, petites Antilles. Bull. Société Géologique Fr. 7 (XXV6), 855–866. doi:10.2113/gssgfbull.s7-xxv.6.855
Melekhova, E., Blundy, J., Robertson, R., and Humphreys, M. C. (2015). Experimental evidence for polybaric differentiation of primitive arc basalt beneath St. Vincent, Lesser Antilles. J. Petrology 56 (1), 161–192. doi:10.1093/petrology/egu074
Miller, D. M., Goldstein, S. L., and Langmuir, C. H. (1994). Cerium/lead and lead isotope ratios in arc magmas and the enrichment of lead in the continents. Nature 368 (6471), 514–520. doi:10.1038/368514a0
Moran, A. E., Sisson, V. B., and Leeman, W. P. (1994). Boron depletion during progressive metamorphism: Implications for subduction processes. Earth Planet. Sci. Lett. 111, 331–349. doi:10.1016/0012-821x(92)90188-2
Morris, J. D., Leeman, W. P., and Tera, F. (1990). The subducted component in Island Arc lavas: Constraints from Be isotopes and B–Be systematics. Nature 344, 31–36. doi:10.1038/344031a0
Nagle, F., Stipp, J. J., and Fisher, D. E. (1976). K-Ar geochronology of the Limestone Caribbees and Martinique, lesser Antilles, west indies. Earth Planet. Sci. Lett. 29, 401–412. doi:10.1016/0012-821x(76)90145-x
Noll, P. D., Newsom, H. E., Leeman, W. P., and Ryan, J. G. (1996). The role of hydrothermal fluids in the production of subduction zone magmas: Evidence from siderophile and chalcophile trace elements and boron. Geochimica Cosmochimica Acta 60, 587–611. doi:10.1016/0016-7037(95)00405-x
Noury, M., Philippon, M., Cornée, J.-J., Bernet, M., Bruguier, O., Montheil, L., et al. (2021). Evolution of a shallow volcanic arc pluton during arc migration: A tectono-thermal integrated study of the St. Martin granodiorites (northern Lesser Antilles). Geochem. Geophys. Geosystems 22, 27. doi:10.1029/2020GC009627
Othman, D. B., White, W. M., and Patchett, J. 1 (1989). HfNdSr isotopes and incompatible element abundances in island arcs: Implications for magma origins and crust-mantle evolution. Earth Planet. Sci. Lett. 94, 167–185. doi:10.1016/0012-821x(84)90112-2
Patiño, L. C., Carr, M. J., and Feigenson, M. D. (2000). Local and regional variations in Central American arc lavas controlled by variations in subducted sediment input. Contrib. Mineral. Pet. 138, 265–283. doi:10.1007/s004100050562
Peacock, S. A. (1990). Fluid processes in subduction zones. Science 248 (4953), 329–337. doi:10.1126/science.248.4953.329
Peterson, V., and Ryan, J. G. (2009). Petrogenesis and structure of the Buck Creek mafic-ultramafic suite, southern Appalachians: Constraints on ophiolite evolution and emplacement in collisional orogens. Geol. Soc. Am. Bull. 121 (3-4), 615–629. doi:10.1130/b26302.1
Plank, T., Balzer, V., and Carr, M. (2002). Nicaraguan volcanoes record paleoceanographic changes accompanying closure of the Panama gateway. Geol. 30 (12), 1087–1090. doi:10.1130/0091-7613(2002)030<1087:nvrpca>2.0.co;2
Plank, T., and Langmuir, C. H. (1998). The chemical composition of subducting sediment and its consequences for the crust and mantle. Chem. Geol. 145, 325–394. doi:10.1016/s0009-2541(97)00150-2
Plank, T., and Langmuir, C. H. (1993). Tracing trace elements from sediment input to volcanic output at subduction zones. Nature 362 (6422), 739–743. doi:10.1038/362739a0
Plank, T. (2014). The chemical composition of subducting sediments. Amsterdam, Netherlands: Elsevier.
Reagan, M. C., Morris, J. D., Herrstrom, E. A., and Murrell, M. T. (1994). Uranium series and beryllium isotope evidence for an extended history of subduction modification of the mantle below Nicaragua. Geochim. Cosmochim. Acta 58 (19), 4199–4212. doi:10.1016/0016-7037(94)90273-9
Rose, E. F., Shimizu, N., Layne, G. D., and Grove, T. L. (2001). Melt production beneath Mt. Shasta from boron data in primitive melt inclusions. Science 293, 281–283. doi:10.1126/science.1059663
Rüpke, L. H., Morgan, J. P., Hort, M., and Connolly, J. A. D. (2002). Are the regional variations in Central American arc lavas due to differing basaltic versus peridotitic slab sources of fluids? Geol. 30 (11), 1035–1038. doi:10.1130/0091-7613(2002)030<1035:atrvic>2.0.co;2
Ruscitto, D. M., Wallace, P. J., Cooper, L. B., and Plank, T. (2012). Global variations in H2O/Ce: 2. Relationships to arc magma geochemistry and volatile fluxes. Geochem. Geophys. Geosyst. 13 (3). doi:10.1029/2011gc003887
Ryan, J. G., and Langmuir, C. H. (1988). Beryllium systematics in young volcanic rocks: Implications for 10Be. Geochimica Cosmochimica Acta 52, 237–244. doi:10.1016/0016-7037(88)90073-7
Ryan, J. G., and Langmuir, C. H. (1987). The systematics of lithium abundances in young volcanic rocks. Geochimica Cosmochimica Acta 51, 1727–1741. doi:10.1016/0016-7037(87)90351-6
Ryan, J. G., and Chauvel, C. (2014). The subduction-zone filter and the impact of recycled materials on the evolution of the mantle. Oxford: Elsevier.
Ryan, J. G., and Langmuir, C. H. (1993). The systematics of boron abundances in young volcanic rocks. Geochimica Cosmochimica Acta 57, 1489–1498. doi:10.1016/0016-7037(93)90008-k
Ryan, J. G., Leeman, W. P., Morris, J. D., and Langmuir, C. H. (1996). The boron systematics of intraplate lavas: Implications for crust and mantle evolution. Geochimica Cosmochimica Acta 60 (3), 415–422. doi:10.1016/0016-7037(95)00402-5
Ryan, J. G., Morris, J., Bebout, G., and Leeman, W. P. (1996). “Describing chemical fluxes in subduction zones: Insights from "depth profiling" studies of arc and forearc rocks,” in Subduction: Top to bottom. Editors G. E. Bebout, D. W. Scholl, S. H. Kirby, and J. P. Platt (New Jersey, United States: Wiley), 96, 263–268. AGU Monograph.
Ryan, J. G., Morris, J., Tera, F., Leeman, W. P., and Tsvetkov, A. (1995). Cross-arc geochemical variations in the Kurile Arc as a function of slab depth. Science 270 (5236), 625–627. doi:10.1126/science.270.5236.625
Saumur, B. M., Hattori, K. H., and Guillot, S. (2010). Contrasting origins of serpentinites in a subduction complex, northern Dominican Republic. Geol. Soc. Am. Bull. 122 (1-2), 292–304. doi:10.1130/b26530.1
Savov, I. P., Ryan, J. G., D’Antonio, M., and Fryer, P. (2007). Shallow slab fluid release across and along the Mariana arc-basinsystem: Insights from geochemistry of serpentinized peridotites from the Mariana fore arc. J. Geophys. Res. 112, B09205. doi:10.1029/2006jb004749
Savov, I. P., Ryan, J. G., Kelley, K., and Mattie, P. D. (2005). Geochemistry of serpentinized peridotites from the mariana forearc conical seamount, ODP leg 125: Implications for the elemental recycling at subduction zones. Geochem. Geophys. Geosyst. 6. doi:10.1029/2004GC000777
Scambelluri, M., Cannaò, E., and Gilio, M. (2019). The water and fluid-mobile element cycles during serpentinite subduction. A review. Eur. J. Mineral. 31 (3), 405–428. doi:10.1127/ejm/2019/0031-2842
Scambelluri, M., Fiebig, J., Malaspina, N., Müntener, O., and Pettke, T. (2004). Serpentinite subduction: Implications for fluid processes and trace-element recycling. Int. Geol. Rev. 46 (7), 595–613. doi:10.2747/0020-6814.46.7.595
Schmidt, M. W., and Poli, S. (1998). Experimentally based water budgets for dehydrating slabs and consequences for arc magma generation. Earth Planet. Sci. Lett. 163, 361–379. doi:10.1016/s0012-821x(98)00142-3
Sigurdsson, H., Tomblin, J. F., Brown, G. M., Holland, J. G., and Arculus, R. J. (1973). Strongly undersaturated magmas in the Lesser Antilles island arc. Earth Planet. Sci. Lett. 18, 285–295. doi:10.1016/0012-821x(73)90067-8
Singer, B. S., Jicha, B. R., Leeman, W. P., Rogers, N. W., Thirlwall, M. F., Ryan, J., et al. (2007). Along-strike trace element and isotopic variation in Aleutian Island arc basalt: Subduction melts sediments and dehydrates serpentine. J. Geophys. Res. 112 (B6), B06206. doi:10.1029/2006jb004897
Smith, H. J., Leeman, W. P., Davidson, J., and Spivack, A. J. (1997). The B isotopic composition of arc lavas from Martinique, Lesser Antilles. Earth Planet. Sci. Lett. 146 (1-2), 303–314. doi:10.1016/s0012-821x(96)00209-9
Straub, S., and Layne, G. (2002). The systematics of boron isotopes in Izu arc front volcanic rocks. Earth Planet. Sci. Lett. 198, 25–39. doi:10.1016/s0012-821x(02)00517-4
Sun, S. S., and McDonough, W. F. (1989). Chemical and isotopic systematics of oceanic basalts: Implications for mantle composition and processes. Geol. Soc. 42 (1), 313–345. doi:10.1144/GSL.SP.1989.042.01.19
Tang, M., Rudnick, R. L., and Chauvel, C. (2014). Sedimentary input to the source of lesser Antilles lavas: A Li perspective. Geochimica Cosmochimica Acta 144, 43–58. doi:10.1016/j.gca.2014.09.003
Tatsumi, Y., and Eggins, S. M. (1995). Subduction zone magmatism. Cambridge, Mass: Blackwell Science, 211pp.
Tera, F., Brown, L., Morris, J., Sacks, I. S., Klein, J., and Middleton, R. (1986). Sediment incorporation in island-arc magmas: Inferences from 10Be. Geochimica Cosmochimica Acta 50 (4), 535–550. doi:10.1016/0016-7037(86)90103-1
Thirlwall, M. F., Graham, A. M., Arculus, R. J., Harmon, R. S., and Macpherson, C. G. (1996). Resolution of the effects of crustal assimilation, sediment subduction, and fluid transport in Island Arc magmas: PbSrNdO isotope geochemistry of Grenada, lesser Antilles. Geochimica Cosmochimica Acta 60 (23), 4785–4810. doi:10.1016/s0016-7037(96)00272-4
Tonarini, S., Agostini, S., Doglioni, C., Innocenti, F., and Manetti, P. (2007). Evidence for serpentinite fluid in convergent margin systems: The example of El Salvador (Central America) arc lavas. Geochem. Geophys. Geosyst. 8, 29. doi:10.1029/2006gc001508
Turner, S. J., and Langmuir, C. H. (2022). Sediment and ocean crust both melt at subduction zones. Earth Planet. Sci. Lett. 584, 117424. doi:10.1016/j.epsl.2022.117424
Turner, S., Tonarini, S., Bindeman, I., Leeman, W. P., and Schaefer, B. F. (2007). Boron and oxygen isotope evidence for recycling of subducted components over the past 2.5 Gyr. Nature 447 (7145), 702–705. doi:10.1038/nature05898
Ulmer, P., and Trommsdorf, V. (1995). Serpentine stability to mantle depths and subduction-related magmatism. Science 268, 858–861. doi:10.1126/science.268.5212.858
Van Soest, M. C., Hilton, D. R., and Kreulen, R. (1998). Tracing crustal and slab contributions to arc magmatism in the Lesser Antilles island arc using helium and carbon relationships in geothermal fluids. Geochimica Cosmochimica Acta 62 (19-20), 3323–3335. doi:10.1016/s0016-7037(98)00241-5
Van Soest, M. C., Hilton, D. R., MacPherson, C. G., and Mattey, D. P. (2002). Resolving sediment subduction and crustal contamination in the lesser Antilles island arc: A combined He–O–Sr isotope approach. J. Petrology 43 (1), 143–170. doi:10.1093/petrology/43.1.143
Wadge, G., and Shepard, J. B. (1984). Segmentation of the lesser Antilles subduction zone. Earth Planet. Sci. Lett. 71, 297–304. doi:10.1016/0012-821x(84)90094-3
Walker, J. A., Feigenson, M. D., Patino, L. C., and Carr, M. J. (2001). Slab control over HFSE depletions in Central Nicaragua. Earth Planet. Sci. Lett. 192 (4), 533–543. doi:10.1016/s0012-821x(01)00476-9
Walker, J. A., Roggensack, K., Patino, L. C., Cameron, B. I., and Matias, O. (2003). The water and trace element contents of melt inclusions across an active subduction zone. Contributions Mineralogy Petrology 146, 62–77. doi:10.1007/s00410-003-0482-x
Walker, J. A., Teipel, A. P., Ryan, J. G., and Syracuse, E. (2009). Light elements and Li isotopes across the northern portion of the Central American subduction zone. G3 Geochem. Geophys. Geosyst. 10 (6), 23. doi:10.1029/2009GC002414
Westbrook, G. K., Mascle, A., and Biju-Duval, B. (1984). Geophysics and the structure of the lesser Antilles forearc. Initial Rep. Deep Sea Drill. Proj. 78, 23–38.
Westercamp, D., and Andreieff, P. (1983). Saint barthelemy et ses ilets, Antilles françaises: Stratigraphie et evolution mag-mato-structurale. Bull. Soc. Geol. Fr. 1983, 873–883. doi:10.2113/gssgfbull.s7-xxv.6.873
Westercamp, D. (1988). Magma generation in the lesser Antilles: Geological constraints. Tectonophysics 149, 145–163. doi:10.1016/0040-1951(88)90123-0
White, W. M., and Dupré, B. (1986). Sediment subduction and magma Genesis in the lesser Antilles: Isotopic and trace element constraints. J. Geophys. Res. 91 (B6), 5927–5941. doi:10.1029/jb091ib06p05927
Wilson, B., and Costelloe, A. (2011). Benthonic foraminiferal paleoecology of the pleistocene in DSDP Hole 148, aves ridge, eastern caribbean sea. J. Foraminifer. Res. 41 (4), 363–370. doi:10.2113/gsjfr.41.4.363
Keywords: subduction recycling, fluid mobile elements, trace elements, Lesser Antilles arc, barium and boron enrichment
Citation: Atlas ZD, Germa A, Boss B, Meireles O, Ward A and Ryan JG (2022) Variable element enrichment sources and contributions to volcanic rocks along the Lesser Antilles Island Arc. Front. Earth Sci. 10:782179. doi: 10.3389/feart.2022.782179
Received: 23 September 2021; Accepted: 13 October 2022;
Published: 21 November 2022.
Edited by:
Mattia Pistone, University of Georgia, United StatesReviewed by:
Heye Freymuth, University of Cambridge, United KingdomAnne-Sophie Bouvier, Université de Lausanne, Switzerland
Copyright © 2022 Atlas, Germa, Boss, Meireles, Ward and Ryan. This is an open-access article distributed under the terms of the Creative Commons Attribution License (CC BY). The use, distribution or reproduction in other forums is permitted, provided the original author(s) and the copyright owner(s) are credited and that the original publication in this journal is cited, in accordance with accepted academic practice. No use, distribution or reproduction is permitted which does not comply with these terms.
*Correspondence: Zachary D. Atlas, zatlas@usf.edu