- 1State Key Laboratory of Plateau Ecology and Agriculture, Qinghai University, Xining, China
- 2School of Soil and Water Conservation, Beijing Forestry University, Beijing, China
- 3State Key Laboratory of Earth Surface Processes and Resource Ecology, Beijing Normal University, Beijing, China
- 4Key Laboratory of Watershed Geographic Sciences, Nanjing Institute of Geography and Limnology, Chinese Academy of Sciences, Nanjing, China
Different strategies of water uptake by roots in a semi-arid desert are one way that plants adapt to the water-limited environment. In this study, stable isotopes of δ18O were used to analyze the variability in water sources of the native species Artemisia ordosica during the growing season in an alpine semi-arid desert on the Qinghai–Tibet Plateau, China. A. ordosica depended primarily on water from upper soil layers in the early growing season, except for those windward, which obtained water from three soil layers and groundwater from 0.19 to 0.28. In the summer, A. ordosica switched sources, and those individuals at the top of the dune used 0.85 of water from middle soil layers, whereas those on windward (0.27–0.33) and leeward slopes (0.31–0.37) absorbed water from three soil layers. Shallow soil water was the main water source at all sites at the end of the growing season. The water uptake pattern of A. ordosica was consistent with the root distribution in positions with different dune geomorphology. The results suggest that the micro-landforms of sand dunes affect vegetation growth not only through aeolian activities and soil properties but also through plant water use.
Introduction
The ability of plants to obtain water is a key factor that determines whether plants can adapt to the environment. Water availability varies according to geomorphic position, and therefore, the water utilization strategy of plants is adaptively adjusted, resulting in separate niches of plants based on geomorphology (Li et al., 2004). In arid and semi-arid areas, plants may change their source of water as the season changes, and some plants may use deep soil water or groundwater during drought. Plant roots are highly plastic and adaptable in order to maintain water absorption. To access the necessary soil water volume, plants can modify their root zones by lateral or vertical growth (Fan et al., 2017). In most ecosystems, water availability limits rooting depth rather than other resources (Yu et al., 2016). Plant roots were revealed as key drivers for water flow processes and different components of the water balance (Yu et al., 2016). In addition, root morphology and distribution also determine the areas from which plants absorb water (Xu and Li, 2009; Xu et al., 2011). When water is available in shallow soils, plants mainly rely on shallow roots to obtain near-surface water and rely less on deep soil water (Dai et al., 2014). However, soil moisture availability is very heterogeneous in arid and semi-arid areas. In these areas, plants no longer rely only on shallow soil water but use deep roots to respond to changes in soil moisture (Ehleringer et al., 1991). Topography is a good indicator of vegetation cover, rooting depth, root zone evaporation and transpiration deficits, soil properties, and even geology (Gao et al., 2018). Thus, the sources of water used by plants are clearly affected by topography (Song et al., 2014).
Stable isotopes are a powerful tool to identify water sources and determine water use patterns of woody species in arid and semi-arid regions (Zhang et al., 2017; Wu et al., 2019a), especially in soils with a high portion of sand (Beyer et al., 2020). Plant water sources can be determined by comparing the stable hydrogen and oxygen isotopes of all potential water sources with those extracted from xylem water (Ehleringer and Dawson, 1992; Song et al., 2014). Plants in semi-arid and arid ecosystems not only absorb most of their water from shallow soil layers under wet conditions but also obtain additional water from deep water pools (such as groundwater) under dry conditions (Dawson and Pate, 1996; McCole and Stern, 2007; Song et al., 2016; Wang-Erlandsson et al., 2016; Zhou et al., 2017). Shifts in the water sources used among different soil layers, depending on water availability, are an important strategy by which deciduous plants cope with drought in water-limited ecosystems (Hasselquist et al., 2010). The ability to extract water from different soil layers is closely related to root morphology and architecture (Xu and Li, 2006). Different species within the same habitat also exhibit considerable differentiation in the water sources used in order to minimize competition for water. Such differentiation is likely a potential mechanism that allows species to coexist, explaining the high diversity and high resilience of plant communities to drought in arid and semi-arid regions (Wu et al., 2014; Yang et al., 2015). In the Mu Us Desert, Artemisia ordosica (Ao) uses shallow groundwater with roots that reach a depth of 200 cm, but fine roots are mainly distributed in the upper 40 cm (Zhang et al., 2012). Deciduous species in seasonally arid ecosystems use different strategies to respond to drought stress. Species that can shift water sources can maximize their soil water use and avoid interspecific competition with other plants in the community, which may be an important survival mechanism in water-limited ecosystems (Ehleringer and Dawson, 1992).
Oxygen isotopes of water molecules are useful tools to reconstruct hydro-climatic variations, analyze hydrological processes and pathways, and better understand current metabolic pathways in plants (Szymczak et al., 2020). Stem water reflects the isotope value of the source water, because no oxygen isotopic fractionation of source water occurs during water uptake by roots (Dawson and Ehleringer, 1991), except in certain plants in extreme environments. Hence, the isotope value in stem water corresponds to that in the source water. However, the isotope signature of source water itself may change depending on soil properties, precipitation amount, season, and influence of groundwater or evaporative enrichment of soil water (Ehleringer and Dawson, 1992). Therefore, stem water δ18O can directly reflect source water δ18O in alpine semi-arid and arid zones (Jia et al., 2012; Wu et al., 2016a; Wu et al., 2016b; Wu et al., 2018).
Desertification is an extreme manifestation of degradation issue in the northern Tibet–Qinghai Plateau (Sun et al., 2019; Zong and Fu, 2021). Qinghai Lake is in the northeast of the Qinghai‒Tibet Plateau in a semi-arid ecologically fragile alpine district and a global climate change-sensitive area, which also be threatened by desertification around the lakeside. Previous studies in this area focused primarily on wind protection mechanisms and ecological functions of sand-fixing plants (Tian et al., 2019; Wu et al., 2019c; Tian et al., 2020). The water sources of revegetation species have received less attention (Wu et al., 2016b), especially those of native species under different conditions of water availability in different micro-landforms.
The aim of this study was to evaluate the water use of a native species on an alpine semi-arid dune, and three questions were addressed: 1) what are the seasonal water sources used by a native plant? 2) What are the key controls on differences in water use of plants at different topographical positions on a sand dune? 3) What are the effects of root distribution and environmental conditions on the water use patterns of a native species? The hypotheses were the following: 1) plants at different topographical positions on a dune would use different water use strategies at different times in the growing season, and 2) plants would tend to use deep soil water or groundwater in water-limited conditions. The results of this study will help guide plantings in alpine deserts to ensure ecological adaptation and structural optimization.
Materials and Methods
Study Site
The study was conducted in the Ketu Wind Prevention and Sand Fixation Experimental Range (WPSER; 36°40′N, 100°45′E; 3,224 m a.s.l.) in the southeast corner of Haiyan Bay of Qinghai Lake, China (for details, see Tian et al., 2019). The Ketu Sandy Land covers approximately 753 km2 and is the largest area on the eastern shore of Qinghai Lake. The field is primarily composed of barchans and transversal sand ridges that average 6–10 m in height and stretch from north to south. The WPSER is in an alpine climatic zone with relatively wet summers and cold winters. Daily meteorological data were provided by a weather station approximately 20 km from the study site from 1981 to 2019. The mean annual temperature was 0.93°C, and the annual average precipitation was 438.6 mm, approximately 80% of which occurred between May and September. Precipitation and temperature in the WPSER have increased since 2004, especially in 2010 (Cui and Li, 2015).
Ao is a typical species in fixed sand dunes. It was introduced as an afforestation species in the 1980s, but the current plants are naturally regenerated. Three plots of 10 × 10 m were selected at three different topographical positions on a dune (windward, top, and leeward).
Root Distribution
An individual Ao of similar height and crown width was selected at each site, and the soil was dug away until roots throughout the soil profile were exposed. The aboveground and underground morphology were photographed. Then, the individual growth morphology and root distribution characteristics were plotted in Photoshop software. Simultaneously, the numbers of coarse roots (root diameter Φ ≥ 5 mm), medium roots (2 mm ≤ Φ < 5 mm), and fine roots (Φ < 2 mm) were counted at 10 cm intervals to the bottom of the soil profile. Then, the root density was calculated for each interval in soil the profile as below “samples collection” section.
Sample Collection
Soil, plant, and rainwater samples were collected once a month from May to September, which was the growing season in 2019. Soil samples were collected beneath marked Aos in fixed plots using a 10-cm-diameter auger at 10 cm intervals from 0 to 20 cm and at 20-cm intervals from 20 to 120 cm. One part of a soil sample was oven-dried to determine soil water content (SWC). Another portion was quickly placed in 20-ml screw-cap glass vials sealed with parafilm. Simultaneously, soil samples were collected in the same soil layers, placed in ziplock bags and returned to the laboratory to analyze soil particle size composition by using a Matersizer 3,000 (Malvern Ltd., WR14 1XZ, United Kingdom). Three replicates of all soil water and isotope samples were collected. Three individuals from the three plots were randomly chosen for plant sampling on each sampling date (12 May, 11 June, 12 July, 12 August, and 15 September). Three- to 5-cm-long branch sections were cut with clippers. The phloem tissue was removed to avoid isotopic fractionation of xylem water and contamination by isotopic enrichment (Dawson, 1996). Then, the sections were immediately placed in airtight vials and sealed with a screw-lid and parafilm to avoid evaporation. Soil and plant xylem isotopic samples were stored in a car refrigerator for transport to the laboratory where they were stored at −18°C until extraction. Water in xylem and soil samples was extracted using a fully automatic vacuum condensation extraction system (LI-2100 Pro, LICA United Technology Limited, Beijing, China). The extraction rate of water from samples was >98%.
The rainwater collector was constructed with a 10-cm-diameter funnel connected to a glass bottle. A plastic ball was placed on the funnel to prevent evaporation (Yang et al., 2015). All precipitation samples were transferred to clean polyethylene bottles (30 ml) and then sealed with parafilm.
The depth of the interdune water table in the study area ranged from 1.5 to 5.0 m. Therefore, a soil auger was used to collect groundwater in the shallow water table at 1.5 m on the sampling dates. Then, immediately, a self-made long spoon was used to collect the groundwater and place it in airtight vials, which were sealed with parafilm to avoid evaporation. Precipitation and groundwater samples were filtered using a 0.22-μm filter and then refrigerated at 4°C until stable isotope analysis.
Data Analyses
The δ18O and δ2H in xylem water, soil water, and precipitation (0.5–1.5 ml) were measured by an Isotopic Ratio Infrared Spectroscopy (IRIS) system (Model DLT-100; Los Gatos Research, Mountain View, CA, United States). Previous studies report that hydrogen offsets occur and persist in semi-arid (Dawson and Ehleringer, 1991), saline (Ellsworth and Williams, 2007), and mild oceanic climate (Barbeta et al., 2019) environments. However, the reasons for the hydrogen fractionation remain unclear, and two explanations have been proposed. It proposes that hydrogen fractionation is related to the mode of water absorption by roots (Ellsworth and Williams, 2007), whereas Barbeta et al. (2019) suggested that it is mainly related to the heterogeneity of soil water signals and plant tissues in temperate deciduous trees. Both agree that fractionation in 2H is much stronger than that in 18O and that 18O therefore provides more satisfactory results. Thus, in this study, only oxygen isotopes (δ18O) were used to determine the sources of plant water. The stable isotopic composition was expressed as follows:
where Rsample and Rstandard represent the molar abundance ratios (18O/16O, 2H/1H) of the sample and the standard (Vienna Standard Mean Ocean Water for O and H), respectively. The Iso-Source model (http://www.epa.gov/wed/pages/models/stable-Isotopes/isosource/isosource.htm) was used to quantify the proportion of water uptake from each water source based on the mass balance of the isotope that was introduced, as detailed by Phillips and Gregg (2003).
For Ao growing in sand, the soil profile was subdivided into three potential water sources, the source increment was set at 1%, and the mass balance tolerance was set at 0.1%. The calculated results are reported as the distribution (e.g., maximum and minimum) of feasible solutions and the mean on each sampling date. The isotopic composition of each soil water layer was determined by the SWC-weighted mean approach (Snyder and Williams, 2003; Liu et al., 2015). According toWu et al. (2016b), the shallow soil layer was from 0 to 20 cm, the middle soil layer from 20 to 60 cm, and the deep soil layer from 60 to 150 cm.
One-way ANOVA combined with Tukey’s least significant difference test was applied to detect differences in plant water source from shallow, middle, and deep soil layers in different seasons. The significance level of the statistical analyses was set at 0.05. The statistical analyses were performed using the SPSS 20.0 software (SPSS Inc., Chicago, IL, United States).
Results
Environmental Factors
The study area had a semi-arid climate with low annual average temperature and low rainfall that was affected by the unique topography, altitude, and atmospheric circulation. Seasonally, precipitation in summer and autumn was relatively abundant, whereas it was relatively rare in winter and spring (Figure 1). In 2019, precipitation was 434.90 mm, with 77% occurring during the growing season from May to September, and the mean temperature was 1.65°C.
The deposits on the natural fixed dune were primarily composed of fine (45.08 ± 4.40%) and medium (34.56 ± 7.30%) sand. Clay and very coarse sand contents in the dune were negligible. In the windward position, fine sand includes very fine sand increased and coarse sand decreased as soil depth increased, whereas in the top and leeward dune positions, no changes occurred with the increase in depth (Table 1). Overall, windward deposits were finer than those at top and leeward positions, with significantly more very fine sand (p = 0.000). There was more medium sand at the leeward position than at the windward and top of dune positions (p = 0.000), whereas the contribution of coarse sand was greater in the shallow soil layer (20 cm depth) in the windward position than in the other two positions (p = 0.000).
With changes in the season, the SWC of different geomorphological positions changed significantly (Figure 2). The highest average SWC (3.68 ± 0.67%) was at the top of the dune, but it was not significantly different from that on windward (3.36 ± 0.56%) and leeward (3.29 ± 0.76%) slopes during the growing season (p > 0.05). The highest SWC at all sites was in July, whereas the lowest was either in June or August, with similar values.
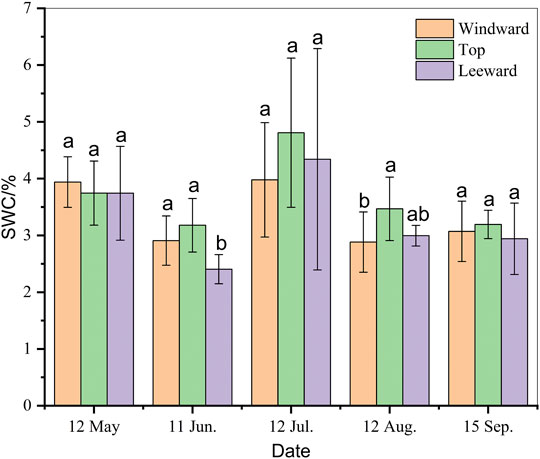
FIGURE 2. Seasonal variation in soil water content (SWC, %) beneath Artemisia ordosica (Ao) in positions with different dune geomorphology. The same letter means that there is no significant difference between different positions of dune (p > 0.05), whereas different letters refer to significant differences between micro-landforms in dune (p < 0.05).
Individual Features at Positions With Different Dune Geomorphology
Plant height, crown width, and coverage at the top of dune were greater than those on windward and leeward slopes (Figure 3). The height of Ao at the top of the dune (32.90 ± 12.94 cm) was significantly greater than that leeward (p = 0.048) but was not different from that windward (p = 0.482). The same trend was observed for N–S length of crown. The largest coverage of Ao was at the top of the dune (38.86 ± 1.03%), followed closely by that of plants windward (38.78 ± 0.68%) without significant difference, whereas the coverage of leeward plants was only 15.52 ± 0.46%. Nevertheless, the highest density was windward, followed by that at the top and leeward.
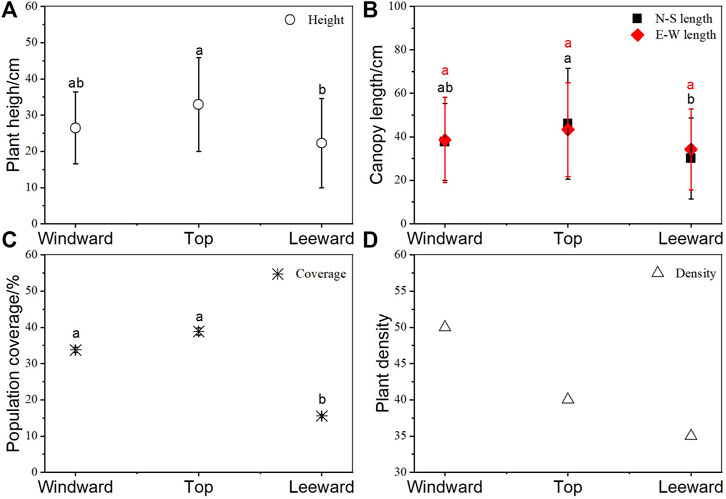
FIGURE 3. Artemisia ordosica (Ao) growth features in positions with different dune geomorphology. (A) Height, (B) crown width of different orientation, (C) coverage, and (D) density. The same letter means that there is no significant difference between different positions of dune (p > 0.05), whereas different letters refer to significant differences between micro-landforms in dune (p < 0.05).
The root system of windward Ao was distributed to the 220 cm soil depth, whereas that of Ao at the top of the dune was distributed to 20 cm and that of leeward Ao was distributed to 50 cm (Figure 4). The roots were concentrated in the upper 20 cm at all sites. Although the tap root of windward Ao extended to 220 cm, most roots were distributed in the top 90 cm. In the top 20 cm, windward Ao had 41.67% fine roots, 35.83% medium roots, and 22.50% coarse roots. Coarse roots decreased in middle soil layers (20–60 cm), whereas the highest density of fine roots was in deep soil layers (60–120 cm). The roots of Ao at the top of the dune were primarily in the shallow soil layer and were composed primarily of fine roots (82.88%). Fine roots of leeward Ao were distributed in the upper 40 cm.
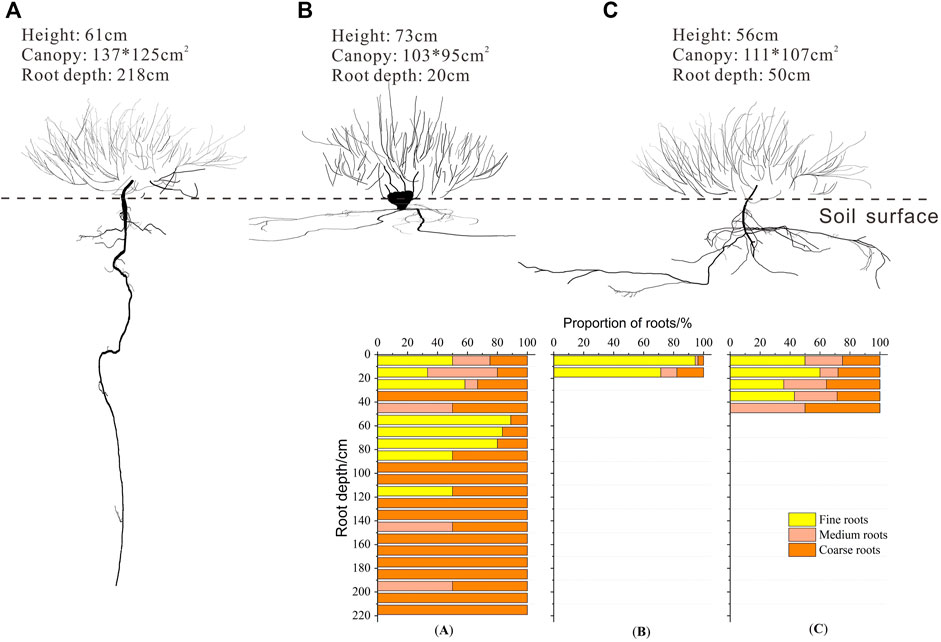
FIGURE 4. Root distribution of Artemisia ordosica (Ao) in positions with different dune geomorphology. (A) Windward, (B) top of the dune, and (C) leeward.
Vertical Variation in Soil Water Content and Isotope Composition
As shown in Figure 5, SWC fluctuated as soil depth increased, with the surface 20 cm particularly affected by precipitation and evaporation. The highest SWC windward (3.59 ± 1.14%) and at the top of dune (3.97 ± 0.97%) was in the top 20 cm, whereas the highest SWC leeward (3.74 ± 1.66%) was in the middle soil layer (40–60 cm). The causes for this phenomenon were the large fluctuations in SWC because of the highest rainfall in July, which resulted in different levels of soil moisture at different topographic positions and under different cover conditions. However, there was no change in SWC in deep soil layers during the growing season. Compared with the upper 20 cm, the SWC was lower below 60 cm in all study sites.
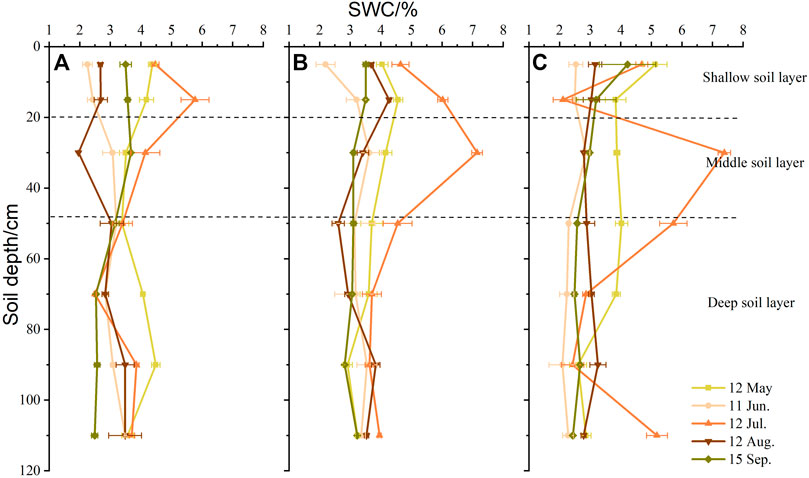
FIGURE 5. Vertical variation in soil water content (SWC, %) in positions with different dune geomorphology. (A) Windward, (B) top of the dune, and (C) leeward.
Nevertheless, the vertical changes in oxygen isotope values in soil water were different from those of SWC (Figure 6). Except for increases in δ18O values with increasing soil depth at all sites in July, the variation in soil water oxygen isotope values was the same in the other seasons and decreased as soil depth increased. There were the strong fluctuations in values that were concentrated in shallow soil layers. Windward, the soil water δ18O value ranged from −8.56 ± 0.42‰ to 2.40 ± 1.42‰ in the upper 20 cm. In the same soil layer, values ranged from −7.01 ± 0.22‰ to 3.74 ± 0.40‰ at the top of dune, whereas the largest range was leeward, from −10.39 ± 0.34‰ to 3.58 ± 0.80‰. In the middle soil layers, soil water oxygen isotope values decreased in all sites.
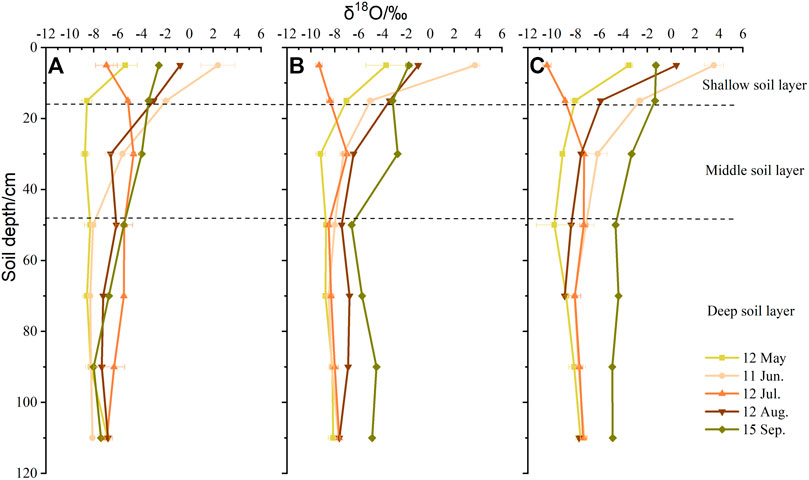
FIGURE 6. Vertical variation in soil water δ18O values in positions with different dune geomorphology. (A) Windward, (B) top of the dune, and (C) leeward.
Seasonal Variation in Isotope Composition of Xylem Water
The global meteoric water line (GMWL, δD = 8δ18O+ 10) indicates the connection between different water pools in the water cycle (Craig, 1961). From Figure 7, both slope and intercept of the local meteoric water line (LMWL, δD = 8.79δ18O+ 23.86) are greater than that of GMWL. Simultaneously, the soil evaporation line (SWL, δD = 5.39δ18O− 20.19) was to the lower right of the LMWL (Figure 7). The lower slope indicated that evaporation fractionation effects in the soil surface affected δD and δ18O enrichment in soil water. In addition, the δD and δ18O values in xylem water of individuals were distributed around those of soil water and groundwater, which indicated that the plants primarily take up soil water from different depths and the groundwater.
The δ18O of Ao twig water at positions with different dune geomorphology showed seasonal variation (p < 0.05), with the highest value (enriched) in June and the lowest (depleted) in July (Figure 8). At the beginning of the growing season (May), the δ18O values of Ao at the top of the dune and on the leeward slope were greater than those on the windward slope (p < 0.05). However, in the middle of growing season (July), there was greater enrichment on the windward slope (p < 0.05). At the end of the growing season (September), there was no significant difference among the different topographical positions (p > 0.05). Overall, the δ18O value of Ao twig water on the top of the dune (−4.05 ± 0.70‰) was higher than that on leeward (−4.42 ± 0.71‰) and windward (−4.56 ± 0.52‰) slopes.
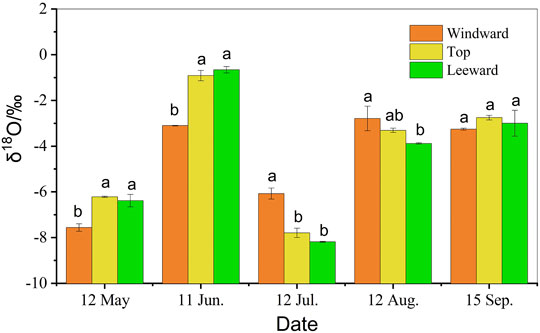
FIGURE 8. Seasonal variation in δ18O xylem water of Artemisia ordosica (Ao) in positions with different dune geomorphology. The same letter means that there is no significant difference between different positions of dune (p > 0.05), whereas different letters refer to significant differences between micro-landforms in dune (p < 0.05).
Seasonal Variation in Contributions to Water Uptake
At the same topographical position, Ao obtained water from different sources in different seasons (Figure 9). At windward, Ao used four potential water pools in May, and the relative water uptake was in the range from 0.19 to 0.28, which represent the portion of water obtained from each of the four pools. However, plants were not likely to use groundwater in future growing seasons, because they shifted use to large amounts of the three soil water pools in the middle of the growing season and to shallow soil water (0.90) at the end of the growing season. Plants used only three layers of soil water at the top of the dune and leeward. At the top of the dune, Ao absorbed shallow soil water at the beginning and the end of the growing season but absorbed soil water from middle layers in July. Nevertheless, Ao leeward first used shallow soil water in the growing season and then used shallow soil water as well as middle soil water in the following months. Overall, Ao used shallow soil water the most, and its water uptake pattern was consistent with the root distribution at positions with different dune geomorphology.
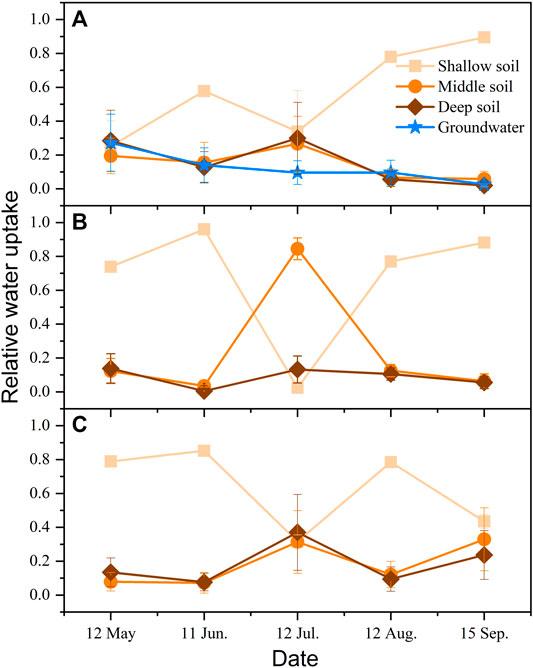
FIGURE 9. Relative water uptake of Artemisia ordosica (Ao) at positions with different dune geomorphology. (A) Windward, (B) top of the dune, and (C) leeward.
In the same growing season, Ao exploited different water pools in different topographical positions. Early in the growing season, Ao at the top of the dune and leeward relied on shallow soil water as the main water source, whereas windward Ao used three layers of soil water as well as groundwater for uptake. In the summer, Ao on the top of the dune switched and used 0.85 of middle soil water, whereas those windward and leeward absorbed soil water from three layers of soil. However, shallow soil water was the main water source at all sites at the end of the growing season. Overall, Ao at the top of the dune and leeward tended to use the upper 60 cm of soil water during the entire growing season, whereas windward Ao used all potential water sources at the beginning of the growing season.
Discussion
Potential Causes for Different Soil Water Contents in Positions With Different Dune Geomorphology
The SWC at the top and on windward and leeward slopes of the sand dune was not significantly different during the growing season in 2019 (p > 0.05). The SWC is easily affected by topography, soil texture, and vegetation (Hawley et al., 1983; Famiglietti et al., 1998), with slope often the most important factor affecting spatial changes in SWC. For example, the SWC in areas with steeper slopes is generally lower than that in areas with more gentle slopes (Famiglietti et al., 1998). Therefore, theoretically, the SWC on the top of the dune should be higher than that on windward and leeward slopes, which is consistent with the results in this study. On the natural fixed dune, soil particle sizes at different topographic positions were not significantly different (p > 0.05, Table 1). Nevertheless, the proportions of coarse sand and medium sand on the windward slope were greater than those on the leeward slope and at the top of the dune. In the relation between SWC and soil texture, SWC is positively correlated with the content of clay particles and negatively correlated with the content of coarse sand (Pan and Wang, 2009). In addition, vegetation height and coverage affect the distribution of soil moisture (Famiglietti et al., 2008). As shown in Figure 3, the height and crown width of individuals at the top of the dune were greater than those on windward and leeward slopes, which was consistent with the distribution of SWC. Pan et al. (2015) observed similar results in arid desert in northern China.
Soil moisture is one factor that limits growth of vegetation in the study area (Lu et al., 2012). The interdune groundwater level was 1.0–1.5 m on the windward side of the study dune; hence, the individuals on the windward slope could possibly absorb groundwater. However, the dune height was above 7 m, and as a result, plants at the top and on the leeward slope of dune likely had difficulty accessing groundwater. Precipitation is the first water source available for desert plants and determines the survival of plants in arid and water-limited environments (Schwinning et al., 2004). In deserts, fluctuations in precipitation during the growing season can be reflected in seasonal changes in soil moisture, as shown in Figure 2 for this study (Porporato et al., 2002). In addition, initial soil moisture content, vegetation transpiration, and soil evaporation also affect the seasonal distribution of SWC (Hawley et al., 1983; Berndtsson et al., 1996; Pan and Wang, 2009; Shen et al., 2015). In this study, the ground surface quickly thawed to replenish soil moisture as temperatures gradually increased in May. At that stage, plants began to germinate, and because seedling soil water consumption and transpiration were relatively weak and soil surface evaporation was low, the SWC remained relatively high. Then, as plants began to grow in June, consumption exceeded replenishment by rainfall, and the SWC decreased immediately. In July, which had the most precipitation of the year, SWC was also the highest of the entire growing season. Although precipitation remained relatively high in August, SWC reached the second lowest value because plant transpiration consumed much of the soil water and the highest evaporation occurred at the soil surface. In September, SWC increased as precipitation and evapotranspiration and soil water use decreased. Overall, the seasonal variation in SWC beneath Ao was similar at the positions with different dune geomorphology.
The vertical distribution of SWC is affected by soil type, precipitation, topographic factors, land cover, and root distribution (Hawley et al., 1983; Pan et al., 2015; Yu et al., 2016). The distribution of SWC in study site was influenced by the distribution of roots, soil particle size, and vegetation cover at the different topographic positions. In uplands in an arid climate in a previous study, shallow infiltration led to shallow rooting (Fan et al., 2017). The infiltration depth of precipitation in the study area is within the top 40 cm of soil (Lu et al., 2013), which is consistent with the results in Figures 5A–C. The SWC at the concentrated layer of a root system is relatively low (Yu et al., 2013; Yu and Jia, 2014). As shown in Figure 4, relatively few fine roots were distributed in the top 20 cm on the windward slope; whereas fine roots were primarily concentrated in the surface 20 cm at the top and on the leeward slope of the dune. In addition, the root–shoot ratio of Ao on the windward slope was much larger (1.00) than that of Ao at the top (0.40) and on the leeward slope (0.36). The aboveground portion of plants was also larger. Therefore, SWC on the windward slope was significantly lower than that in other positions in July. Moreover, the canopy, height, coverage, and density of Ao on the leeward slope were lower than those on the windward slope and at the top (Figure 3), which might cause evaporation to be greater than that at the other two sites. In addition, coarse soil particles on the leeward slope likely led to poor water holding capacity at the soil surface, resulting in greater infiltration to the middle and deep soil layers. Under the influence of multiple factors, the surface SWC was relatively low and the middle layer SWC was relatively high on the leeward slope.
Water Use Patterns at Positions with Different Dune Geomorphology
Seasonal variation occurred in the water sources of plants, which indicated that Ao absorbed water from different pools at different stages in the growing season (Figure 9). In general, the seasonal variation in water sources used by plants is determined by two factors: the distribution of plant root systems and seasonal variations in hydrological conditions (Zencich et al., 2002; Asbjornsen et al., 2008; Pan et al., 2020). In spring (May), Ao at the top and on the leeward slope absorbed more than 0.70 of shallow soil water, whereas Ao windward used water from three soil layers and groundwater because the contribution of shallow soil water was from 0.19 to 0.28. This result might be because the leeward slope soil contained more coarse sand, which led to a shallow distribution of roots that used a large amount of shallow soil water. As shown in Figures 2 and 5, the shallow soil water at the top of the dune and on the leeward slope was sufficient to support plant growth in the spring, whereas windward individuals had the largest amount and hence needed more water to germinate at the beginning of the growing season. In July, Ao on the top of the dune switched and used 0.85 of middle soil water, whereas water was absorbed from three soil layers soil on windward (0.26–0.33) and leeward (0.31–0.37) slopes. This water uptake pattern seemed inconsistent with the root distribution shown in Figure 4 of individuals dug outside of the sampled plot. However, where soil moisture is available, root activity rather than root presence can be a reliable indicator of actual water uptake dynamics in arid and semi-arid environments (Ehleringer and Dawson, 1992; Tiemuerbieke et al., 2018). It is most likely because of a trade-off between the activity of shallow and deep roots (Williams and Ehleringer, 2000; Martin-Gomez et al., 2015; Wang et al., 2019; Zhou et al., 2019). In addition, as shown in Figure 5, the highest SWC value was at 20–40 cm in July, which was consistent with the water uptake layer. The water uptake pattern of Ao in the summer was driven by SWC, consistent with other studies in the deserts of northern China (Pan et al., 2020). At the end of the growing season, all individuals used shallow soil water as the main water source, because SWC decreased as soil depth increased in September. Thus, the Ao at different topographic positions used different pools as the water source in different parts of the growing season, which was related to SWC, precipitation, and the activity of root systems under the influence of micro-topography. Overall, Ao likely used the upper 20 cm of soil water at all dune sites during the growing season.
The Impact of Climate and Topography on Root
The root zone water storage is the core of hydrological models as it controls the partitioning of available water for plant use (Gao et al., 2014). In this study, the root zone was different in three sites for same species (Figure 4). At the windward slope, the root zone was extending to more than 200 cm depth, whereas only 20 cm depth on the top of and 50 cm depth at the leeward slope. The ability of root zone water storage is controlled by root zone storage capacity SR (Gentine et al., 2012), which accounts for the volume of water accessible to roots (Gao et al., 2014), and determines the maximum amount of soil moisture potentially available for vegetation transpiration (Wang-Erlandsson et al., 2016). Gao et al. (2014) showed that vegetation in arid catchments requires a higher SR to store more water, which are mainly controlled by atmospheric moisture supply, canopy demand dynamics, and seasonality of precipitation. In semi-arid alpine desert, precipitation was concentrated in the summer so that vegetation could use sufficient water during the vigorous growth period to obtain the necessary soil water volume by lateral or vertical growth to adapt their root zones (Schenk, 2005). In addition, SR estimates implicitly capture the root density that is active in water uptake more than root zone depth. Thus, in next step, we should take more attention on the factors affected on root zone water storage capacity because it emphasizes the role of co-evolution of vegetation and hydrology and understands the hydrological response of revegetation individuals.
Besides, topography is often the dominant driver of water movement caused by prevailing hydraulic gradients (Gao et al., 2018) and rooting depth (Fan et al., 2017). Plant rooting depth affects ecosystem resilience to environmental stress such as drought (Fan et al., 2017). Roots are highly adaptive to local soil environments, which was approved by soil particle size distribution. In study sites, windward has the coarse sand in the surface 20 cm depth, whereas more very fine sand in the other two sites (Table 1), which was prone to precipitation infiltration and downward growth of roots approved by Fan et al. (2017). In water-limited environment, roots connect soil water or groundwater to the atmosphere, thus influencing the local hydrologic cycle and climate.
It was warmer and increased precipitation in northern Tibetan Plateau in recent years. However, different types of vegetation exhibited different sensitivities to climatic change, which were attributed to the different initial conditions and changes in climatic factors among the types of vegetation (Shen et al., 2014; Wang et al., 2015). Previous studies show that water availability had stronger effects on aboveground net primary productivity (Wang et al., 2022), whereas increased precipitation could increase soil moisture in alpine meadow ecosystem (Shen et al., 2015; Yu et al., 2019). In alpine deserts, shrubs on dunes mainly use soil water at different depths (Jia et al., 2012; Wu et al., 2016b). Warm temperatures are beneficial because they increase the water use efficiency of alpine plants, and humidity favors higher surface SWC and promotes the maximum use of shallow soil water by plants growing in sand. The effects of stress on sand-fixing shrubs promote the benign development of sandy ecosystems. Therefore, in future research, under the background of warming and humidification, the other focus will be on the water use patterns of different types of artificial sand-fixing plants under different planting modes.
Conclusion
In this study, the stable isotopes of δ18O and δD were used to analyze the variability in water sources of the native species Ao during the growing season in an alpine semi-arid desert. Although the species has high cold and drought tolerance in alpine environments and also an ability to adjust to water sources at different depths, soil water primarily recharged by precipitation is the critical factor controlling its survival and growth. Ao primarily depended on water from upper soil layers in the early growing season, except for those windward. Whereas in July, with the highest rainfall and SWC, plants likely used water in middle soil layers at all topographic positions on the dune. At the end of the growing season, the use shallow soil water became predominant again. Overall, Ao used shallow soil water the most, and its water uptake pattern was consistent with the root distribution at positions with different dune geomorphology. The results of this study indicate that the micro-landforms of sand dunes affect vegetation growth not only through aeolian activities and soil properties but also through plant water use.
Data Availability Statement
The raw data supporting the conclusion of this article will be made available by the authors, without undue reservation.
Author Contributions
HaW finished the data curation and formal analysis and wrote the original draft. LT played a guiding role in conceptualizing the field experiment, review, and editing the manuscript. HZ and HuW assisted in data analysis and sample collection. YY helped editing and reviewing the manuscript.
Funding
This research was funded by Natural Science Foundation of China (41961017), Qinghai Three Rivers Ecological Protection and Construction Phase II (2018-S-1), Science and Technology Project of Qinghai Province (2018-NK-A3), and State Key Laboratory of Earth Surface Processes and Resource Ecology (2020-KF-06).
Conflict of Interest
The authors declare that the research was conducted in the absence of any commercial or financial relationships that could be construed as a potential conflict of interest.
The handling editor is currently co-organizing a Research Topic with one of the authors YY and confirms the absence of any other collaboration.
Publisher’s Note
All claims expressed in this article are solely those of the authors and do not necessarily represent those of their affiliated organizations or those of the publisher, the editors, and the reviewers. Any product that may be evaluated in this article, or claim that may be made by its manufacturer, is not guaranteed or endorsed by the publisher.
Acknowledgments
We thank Huade Guan for his help in data analysis and Qiaoyu Wang for her kind assistance in field experiments for this study.
References
Asbjornsen, H., Shepherd, G., Helmers, M., and Mora, G. (2008). Seasonal Patterns in Depth of Water Uptake under Contrasting Annual and Perennial Systems in the Corn Belt Region of the Midwestern U.S. Plant Soil 308, 69–92. doi:10.1007/s11104-008-9607-3
Barbeta, A., Jones, S. P., Clavé, L., Wingate, L., Gimeno, T. E., Fréjaville, B., et al. (2019). Unexplained Hydrogen Isotope Offsets Complicate the Identification and Quantification of Tree Water Sources in a Riparian forest. Hydrol. Earth Syst. Sci. 23, 2129–2146. doi:10.5194/hess-23-2129-2019
Berndtsson, R., Nodomi, K., Yasuda, H., Persson, T., Chen, H., and Jinno, K. (1996). Soil Water and Temperature Patterns in an Arid Desert Dune Sand. J. Hydrol. 185, 20. doi:10.1016/0022-1694(95)02987-7
Beyer, M., Kühnhammer, K., and Dubbert, M. (2020). In Situ measurements of Soil and Plant Water Isotopes: a Review of Approaches, Practical Considerations and a Vision for the Future. Hydrol. Earth Syst. Sci. 24, 4413–4440. doi:10.5194/hess-24-4413-2020
Craig, H. (1961). Isotopic Variations in Meteoric Waters. Science 133, 1702–1703. doi:10.1126/science.133.3465.1702
Cui, B.-L., and Li, X.-Y. (2015). Stable Isotopes Reveal Sources of Precipitation in the Qinghai Lake Basin of the Northeastern Tibetan Plateau. Sci. Total Environ. 527-528, 26–37. doi:10.1016/j.scitotenv.2015.04.105
Dai, Y., Zheng, X.-J., Tang, L.-S., and Li, Y. (2014). Stable Oxygen Isotopes Reveal Distinct Water Use Patterns of Two Haloxylon Species in the Gurbantonggut Desert. Plant Soil 389, 73–87. doi:10.1007/s11104-014-2342-z
Dawson, T. E. (1996). Determining Water Use by Trees and Forests from Isotopic, Energy Balance and Transpiration Analyses: the Roles of Tree Size and Hydraulic Lift. Tree Physiol. 16, 263–272. doi:10.1093/treephys/16.1-2.263
Dawson, T. E., and Ehleringer, J. R. (1991). Streamside Trees that Do Not Use Stream Water. Nature 350, 3. doi:10.1038/350335a0
Dawson, T. E., and Pate, J. S. (1996). Seasonal Water Uptake and Movement in Root Systems of Australian Phraeatophytic Plants of Dimorphic Root Morphology: a Stable Isotope Investigation. Oecologia 107, 13–20. doi:10.1007/BF00582230
Ehleringer, J. R., and Dawson, T. E. (1992). Water Uptake by Plants: Perspectives from Stable Isotope Composition. Ptant, Cel Environ. 15, 10. doi:10.1111/j.1365-3040.1992.tb01657.x
Ehleringer, J. R., Phillips, S. L., Schuster, W. S., and Sandquist, D. R. (1991). Differential Utilization of Summer rains by Desert Plants. Oecologia 88, 430–434. doi:10.1007/BF00317589
Ellsworth, P. Z., and Williams, D. G. (2007). Hydrogen Isotope Fractionation during Water Uptake by Woody Xerophytes. Plant Soil 291, 93–107. doi:10.1007/s11104-006-9177-1
Famiglietti, J. S., Ryu, D., Berg, A. A., Rodell, M., and Jackson, T. J. (2008). Field Observations of Soil Moisture Variability across Scales. Water Resour. Res. 44. doi:10.1029/2006wr005804
Famiglietti, J. S., Rudnicki, J. W., and Rodell, M. (1998). Variability in Surface Moisture Content along a Hillslope Transect: Rattlesnake Hill, Texas. J. Hydrol. 210, 259–281. doi:10.1016/s0022-1694(98)00187-5
Fan, Y., Miguez-Macho, G., Jobbágy, E. G., Jackson, R. B., and Otero-Casal, C. (2017). Hydrologic Regulation of Plant Rooting Depth. Proc. Natl. Acad. Sci. USA 114, 10572–10577. doi:10.1073/pnas.1712381114
Gao, H., Hrachowitz, M., Schymanski, S. J., Fenicia, F., Sriwongsitanon, N., and Savenije, H. H. G. (2014). Climate Controls How Ecosystems Size the Root Zone Storage Capacity at Catchment Scale. Geophys. Res. Lett. 41, 7916–7923. doi:10.1002/2014gl061668
Gao, H. K., Birkel, C., Hrachowitz, M., Tetzlaff, D., Soulsby, C., and Savenije, H. H. G. (2018). A Simple Topography-Driven and Calibration-free Runoff Generation Module. Hydrol. Earth Syst. Sci. 23, 787–809. doi:10.5194/hess-23-787-2019
Gentine, P., D'odorico, P., Lintner, B. R., Sivandran, G., and Salvucci, G. (2012). Interdependence of Climate, Soil, and Vegetation as Constrained by the Budyko Curve. Geophys. Res. Lett. 39. doi:10.1029/2012gl053492
Hasselquist, N. J., Allen, M. F., and Santiago, L. S. (2010). Water Relations of evergreen and Drought-Deciduous Trees along a Seasonally Dry Tropical forest Chronosequence. Oecologia 164, 881–890. doi:10.1007/s00442-010-1725-y
Hawley, M. E., Jackson, T. J., and Mccuen, R. H. (1983). Surface Soil Moisture Variation on Small Agricultural Watersheds. J. Hydrol. 62, 22. doi:10.1016/0022-1694(83)90102-6
Jia, Z., Zhu, Y., and Liu, L. (2012). Different Water Use Strategies of Juvenile and Adult Caragana Intermedia Plantations in the Gonghe Basin, Tibet Plateau. PLoS One 7, e45902. doi:10.1371/journal.pone.0045902
Li, W., Shi, W., Wang, G., Han, X., and Li, Z. (2004). Analysis on Moisture Niche Fitness and Interspecific Coexistence of Artificial forest Plants in Shapotou Area. Acta Botanic Boreal -Occidentalia Sinica 24 (3), 384–391.
Liu, B., Zhao, W., Liu, Z., Yang, Y., Luo, W., Zhou, H., et al. (2015). Changes in Species Diversity, Aboveground Biomass, and Vegetation Cover along an Afforestation Successional Gradient in a Semiarid Desert Steppe of China. Ecol. Eng. 81, 301–311. doi:10.1016/j.ecoleng.2015.04.014
Lu, R., Tang, Q., and Sang, Y. (2012). Dynamic Changes of Soil Moisture in Different Dunes of Ketu Sand Land in Qinghai Lake Basin. Res. Soil Water Conservation 19 (2), 111–115.
Lu, R., Tang, Q., Wei, D., and Zhang, D. (2013). Rainwater Infiltration at Dunes under Various Rainfall Events in sandy Land to the East of Qinghai Lake. J. Desert Res. 33 (3), 797–803. doi:10.7522/j.issn.1000-694X.2013.00114
Martin-Gomez, P., Barbeta, A., Voltas, J., Peñuelas, J., Dennis, K., Palacio, S., et al. (2015). Isotope-ratio Infrared Spectroscopy: a Reliable Tool for the Investigation of Plant-Water Sources? New Phytol. 207, 914–927. doi:10.1111/nph.13376
McCole, A. A., and Stern, L. A. (2007). Seasonal Water Use Patterns of Juniperus Ashei on the Edwards Plateau, Texas, Based on Stable Isotopes in Water. J. Hydrol. 342, 238–248. doi:10.1016/j.jhydrol.2007.05.024
Pan, Y.-X., and Wang, X.-P. (2009). Factors Controlling the Spatial Variability of Surface Soil Moisture within Revegetated-Stabilized Desert Ecosystems of the Tengger Desert, Northern China. Hydrol. Process. 23, 1591–1601. doi:10.1002/hyp.7287
Pan, Y.-X., Wang, X.-P., Ma, X.-Z., Zhang, Y.-F., and Hu, R. (2020). The Stable Isotopic Composition Variation Characteristics of Desert Plants and Water Sources in an Artificial Revegetation Ecosystem in Northwest China. Catena 189, 104499. doi:10.1016/j.catena.2020.104499
Pan, Y.-X., Wang, X.-P., Zhang, Y.-F., and Hu, R. (2015). Spatio-temporal Variability of Root Zone Soil Moisture in Artificially Revegetated and Natural Ecosystems at an Arid Desert Area, NW China. Ecol. Eng. 79, 100–112. doi:10.1016/j.ecoleng.2015.04.019
Phillips, D. L., and Gregg, J. W. (2003). Source Partitioning Using Stable Isotopes: Coping with Too many Sources. Oecologia 136, 261–269. doi:10.1007/s00442-003-1218-3
Porporato, A., D'odorico, P., Laio, F., Ridolfi, L., and Rodriguez-Iturbe, I. (2002). Ecohydrology of Water-Controlled Ecosystems. Adv. Water Resour. 25, 14. doi:10.1016/s0309-1708(02)00058-1
Schenk, J. H. (2005). Vertical Vegetation Structure below Ground: Scaling from Root to Globe. Prog. Bot. 66, 341–373. doi:10.1007/3-540-27043-4_14
Schwinning, S., Sala, O. E., Loik, M. E., and Ehleringer, J. R. (2004). Thresholds, Memory, and Seasonality: Understanding Pulse Dynamics in Arid/semi-Arid Ecosystems. Oecologia 141, 191–193. doi:10.1007/s00442-004-1683-3
Shen, Z.-X., Li, Y.-L., and Fu, G. (2015). Response of Soil Respiration to Short-Term Experimental Warming and Precipitation Pulses over the Growing Season in an alpine Meadow on the Northern Tibet. Appl. Soil Ecol. 90, 35–40. doi:10.1016/j.apsoil.2015.01.015
Shen, Z., Fu, G., Yu, C., Sun, W., and Zhang, X. (2014). Relationship between the Growing Season Maximum Enhanced Vegetation Index and Climatic Factors on the Tibetan Plateau. Remote Sensing 6, 6765–6789. doi:10.3390/rs6086765
Snyder, K. A., and Williams, D. G. (2003). Defoliation Alters Water Uptake by Deep and Shallow Roots of Prosopis Velutina (Velvet Mesquite). Funct. Ecol. 17, 12. doi:10.1046/j.1365-2435.2003.00739.x
Song, L., Zhu, J., Li, M., and Yu, Z. (2014). Water Utilization of Pinus Sylvestris Var. Mongolica in a Sparse wood Grassland in the Semiarid sandy Region of Northeast China. Trees 28, 971–982. doi:10.1007/s00468-014-1010-5
Song, L., Zhu, J., Li, M., and Zhang, J. (2016). Water Use Patterns of Pinus Sylvestris Var. Mongolica Trees of Different Ages in a Semiarid sandy Lands of Northeast China. Environ. Exp. Bot. 129, 94–107. doi:10.1016/j.envexpbot.2016.02.006
Sun, J., Hou, G., Liu, M., Fu, G., Zhan, T., Zhou, H., et al. (2019). Effects of Climatic and Grazing Changes on Desertification of alpine Grasslands, Northern Tibet. Ecol. Indicators 107, 105647. doi:10.1016/j.ecolind.2019.105647
Szymczak, S., Barth, J., Bendix, J., Huneau, F., Garel, E., Häusser, M., et al. (2020). First Indications of Seasonal and Spatial Variations of Water Sources in pine Trees along an Elevation Gradient in a Mediterranean Ecosystem Derived from δ18O. Chem. Geology. 549, 119695. doi:10.1016/j.chemgeo.2020.119695
Tian, L., Wu, W., Zhang, D., and Yu, Y. (2020). Airflow Field Around Hippophae Rhamnoides in Alpine Semi-arid Desert. Land 9, 140. doi:10.3390/land9050140
Tian, L., Wu, W., Zhou, X., Zhang, D., Yu, Y., Wang, H., et al. (2019). The Ecosystem Effects of Sand-Binding Shrub Hippophae Rhamnoides in Alpine Semi-arid Desert in the Northeastern Qinghai-Tibet Plateau. Land 8, 183. doi:10.3390/land8120183
Tiemuerbieke, B., Min, X.-J., Zang, Y.-X., Xing, P., Ma, J.-Y., and Sun, W. (2018). Water Use Patterns of Co-occurring C3 and C4 Shrubs in the Gurbantonggut Desert in Northwestern China. Sci. Total Environ. 634, 341–354. doi:10.1016/j.scitotenv.2018.03.307
Wang, J., Fu, B., Lu, N., Wang, S., and Zhang, L. (2019). Water Use Characteristics of Native and Exotic Shrub Species in the Semi-arid Loess Plateau Using an Isotope Technique. Agric. Ecosyst. Environ. 276, 55–63. doi:10.1016/j.agee.2019.02.015
Wang, J. W., Li, M., Yu, C. Q., and Fu, G. (2022). The Change in Environmental Variables Linked to Climate Change Has a Stronger Effect on Aboveground Net Primary Productivity Than Does Phenological Change in Alpine Grasslands. Front. Plant Sci. 12. doi:10.3389/fpls.2021.798633
Wang, S.-H., Sun, W., Li, S.-W., Shen, Z.-X., and Fu, G. (2015). Interannual Variation of the Growing Season Maximum Normalized Difference Vegetation Index, MNDVI, and its Relationship with Climatic Factors on the Tibetan Plateau. Polish J. Ecol. 63, 424–439. doi:10.3161/15052249pje2015.63.3.012
Wang-Erlandsson, L., Bastiaanssen, W. G. M., Gao, H. K., Jägermeyr, J., Senay, G. B., Van Dijk, A. I. J. M., et al. (2016). Global Root Zone Storage Capacity from Satellite-Based Evaporation. Hydrol. Earth Syst. Sci. 20, 23. doi:10.5194/hess-20-1459-2016
Williams, D. G., and Ehleringer, J. R. (2000). Intra- and Interspecific Variation for Summer Precipitation Use in Pinyon–Juniper Woodlands. Ecol. Monogr. 70, 21. doi:10.2307/2657185
Wu, H., Li, J., Li, X.-Y., He, B., Liu, J., Jiang, Z., et al. (2018). Contrasting Response of Coexisting Plant's Water-Use Patterns to Experimental Precipitation Manipulation in an alpine Grassland Community of Qinghai Lake Watershed, China. PLoS One 13, e0194242. doi:10.1371/journal.pone.0194242
Wu, H., Li, X.-Y., Jiang, Z., Chen, H., Zhang, C., and Xiao, X. (2016b). Contrasting Water Use Pattern of Introduced and Native Plants in an alpine Desert Ecosystem, Northeast Qinghai-Tibet Plateau, China. Sci. Total Environ. 542, 182–191. doi:10.1016/j.scitotenv.2015.10.121
Wu, H., Li, X.-Y., Li, J., Zhang, C., He, B., Zhang, S., et al. (2019a). Age-related Water Uptake Patterns of alpine Plantation Shrubs in Reforestation Region of Qinghai-Tibetan Plateau Based on Stable Isotopes. Ecohydrology 12, e2049. doi:10.1002/eco.2049
Wu, H., Li, X. Y., Li, J., Jiang, Z., Chen, H., Ma, Y., et al. (2016a). Differential Soil Moisture Pulse Uptake by Coexisting Plants in an alpine Achnatherum Splendens Grassland Community. Environ. Earth Sci. 75, 914. doi:10.1007/s12665-016-5694-2
Wu, W., Zhang, D., Tian, L., Zhang, M., and Zhou, X. (2019c). Features of Artificial Plant Communications from the East Sand Region of the Qinghai Lake over the Last 10 Years. Acta Ecologica Sinica 39, 13. doi:10.5846/stxb201712292353
Wu, Y., Zhou, H., Zheng, X.-J., Li, Y., and Tang, L.-S. (2014). Seasonal Changes in the Water Use Strategies of Three Co-occurring Desert Shrubs. Hydrol. Process. 28, 6265–6275. doi:10.1002/hyp.10114
Xu, G.-Q., Li, Y., and Xu, H. (2011). Seasonal Variation in Plant Hydraulic Traits of Two Co-occurring Desert Shrubs, Tamarix Ramosissima and Haloxylon Ammodendron, with Different Rooting Patterns. Ecol. Res. 26, 1071–1080. doi:10.1007/s11284-011-0858-8
Xu, G., and Li, Y. (2009). Roots Distribution of Three Desert Shrubs and Their Response to Precipitation under Co-occurring Conditions. Acta Ecologica Sinica 29, 8. doi:10.1016/j.chnaes.2009.07.004
Xu, H., and Li, Y. (2006). Water-use Strategy of Three central Asian Desert Shrubs and Their Responses to Rain Pulse Events. Plant Soil 285, 5–17. doi:10.1007/s11104-005-5108-9
Yang, B., Wen, X., and Sun, X. (2015). Seasonal Variations in Depth of Water Uptake for a Subtropical Coniferous Plantation Subjected to Drought in an East Asian Monsoon Region. Agric. For. Meteorology 201, 218–228. doi:10.1016/j.agrformet.2014.11.020
Yu, C.-Q., Wang, J.-W., Shen, Z.-X., and Fu, G. (2019). Effects of Experimental Warming and Increased Precipitation on Soil Respiration in an alpine Meadow in the Northern Tibetan Plateau. Sci. Total Environ. 647, 1490–1497. doi:10.1016/j.scitotenv.2018.08.111
Yu, T., Feng, Q., Si, J., Xi, H., Li, Z., and Chen, A. (2013). Hydraulic Redistribution of Soil Water by Roots of Two Desert Riparian Phreatophytes in Northwest China's Extremely Arid Region. Plant Soil 372, 297–308. doi:10.1007/s11104-013-1727-8
Yu, Y., and Jia, Z. Q. (2014). Changes in Soil Organic Carbon and Nitrogen Capacities of Salix Cheilophila Schneid along a Revegetation Chronosequence in Semi-arid Degraded sandy Land of the Gonghe Basin, Tibet Plateau. Solid Earth 5, 1045–1054. doi:10.5194/se-5-1045-2014
Yu, Y., Loiskandl, W., Kaul, H.-P., Himmelbauer, M., Wei, W., Chen, L., et al. (2016). Estimation of Runoff Mitigation by Morphologically Different Cover Crop Root Systems. J. Hydrol. 538, 667–676. doi:10.1016/j.jhydrol.2016.04.060
Zencich, S. J., Froend, R. H., Turner, J. V., and Gailitis, V. (2002). Influence of Groundwater Depth on the Seasonal Sources of Water Accessed by Banksia Tree Species on a Shallow, sandy Coastal Aquifer. Oecologia 131, 8–19. doi:10.1007/s00442-001-0855-7
Zhang, C., Li, X., Wu, H., Wang, P., Wang, Y., Wu, X., et al. (2017). Differences in Water-Use Strategies along an Aridity Gradient between Two Coexisting Desert Shrubs (Reaumuria Soongorica and Nitraria Sphaerocarpa): Isotopic Approaches with Physiological Evidence. Plant Soil 419, 169–187. doi:10.1007/s11104-017-3332-8
Zhang, J., Han, H., Lei, Y., Yang, W., Li, H., Yang, D., et al. (2012). Correlations Between Distribution Characteristics of Artemisia ordosica Root System and Soil Moisture Under Different Fixation Stage of Sand Dunes. Journal of Southwest Forestry University 32(6), 1–5. doi:10.3969/j.issn.2095-1914.2012.06.001
Zhou, H., Zhao, W., He, Z., Yan, J., and Zhang, G. (2019). Variation in Depth of Water Uptake for Pinus Sylvestris Var. Mongolica along a Precipitation Gradient in sandy Regions. J. Hydrol. 577, 123921. doi:10.1016/j.jhydrol.2019.123921
Zhou, H., Zhao, W., and Zhang, G. (2017). Varying Water Utilization of Haloxylon Ammodendron Plantations in a Desert-Oasis Ecotone. Hydrol. Process. 31, 825–835. doi:10.1002/hyp.11060
Keywords: water uptake, root distribution, native species, different topography of dune, alpine semi-arid desert, stable oxygen isotopes
Citation: Wang H, Tian L, Zhang H, Yu Y and Wu H (2022) Water Uptake by Artemisia ordosica Roots at Different Topographic Positions in an Alpine Desert Dune on the Northeastern Qinghai–Tibet Plateau. Front. Earth Sci. 10:686441. doi: 10.3389/feart.2022.686441
Received: 26 March 2021; Accepted: 07 February 2022;
Published: 28 March 2022.
Edited by:
María Fernandez, Universidad de León, SpainReviewed by:
Hongkai Gao, East China Normal University, ChinaGang Fu, Institute of Geographic Sciences and Natural Resources Research (CAS), China
Copyright © 2022 Wang, Tian, Zhang, Yu and Wu. This is an open-access article distributed under the terms of the Creative Commons Attribution License (CC BY). The use, distribution or reproduction in other forums is permitted, provided the original author(s) and the copyright owner(s) are credited and that the original publication in this journal is cited, in accordance with accepted academic practice. No use, distribution or reproduction is permitted which does not comply with these terms.
*Correspondence: Lihui Tian, bGh0aWFuQHFodS5lZHUuY24=