- 1Research Institute of Petroleum Exploration and Development (RIPED), Beijing, China
- 2Xi'an Center, China Geological Survey, Xi'an, China
- 3China University of Mining and Technology, Beijing, China
- 4Lancaster Environment Centre, Lancaster University, Lancaster, United Kingdom
Helium is a strategic resource with many scientific, technical and industrial applications. However, despite its importance and scarcity, there is a lack of understanding on its origin, migration and accumulation processes. In view of this, the distribution patterns and enrichment mechanisms of helium are studied based on systematic analysis of the genetic types of helium in petroliferous basins in China. The helium in the petroliferous basins in China is mainly radiogenic, which involves the decay of U and Th. As expected, we found that, in one gas field, the helium content in natural gas increases with the age of reservoir rock, and in the same layer of the same structure within one reservoir, the helium content in natural gas in high altitude part of the structure is higher than that in the lower part. Enrichment of helium is closely related to old deep groundwater. The helium produced by radioactive decay in old strata is dissolved in water and transported and enriched through water migration. Due to large uplift of the structure during the Himalayan period, the helium dissolved in water was exsolved and accumulated with natural gas in the trap above the water. And thus, the helium-rich natural gas reservoirs formed. So, the helium contents in natural gas fields increase gradually along the flow direction of groundwater. The area with large range of uplift often shows higher helium content. This study not only provides a basis for the study of helium enrichment research, but also provides a new idea for industrial helium resource exploration.
Introduction
Helium is an inert gas with the lowest melting and boiling point which has been found in nature so far. It demonstrates extremely strong permeability and thermal conductivity. Because of these characteristics of helium, it is widely used in aerospace, medical application (cooling of NMR magnets) and nuclear industry, as well as scientific research, high-tech and other fields. The global helium resources mainly come from natural gas, and the supply is scarce. According to the report by the United States Geological Survey (U.S. Geological Survey, 2021), the total global helium resources are about 519 × 108 m3. China’s helium resources account for only 2% of the total inventory. In 2020, the global annual production of helium is 1.44 × 108 m3, and China’s production of helium is almost zero. Although researchers in China have carried out helium studies since the 1980s, the studies mainly focused on using helium isotopes as an indicator for the sources of natural gas (Xu et al., 1979; Wang, 1989; Xu et al., 1990a; Wang et al., 1992; Dai et al., 1995; Xu, 1996) and the tectonic environment of basins (Xu et al., 1990a; Xu et al., 1990b; Sun, 1994; Xu et al., 1994; Xu, 1997). There is a lack of helium resource evaluation and potential estimation in China. Helium in some helium-rich natural gas reservoirs has been released into the atmosphere with the production and utilization of natural gas. Although potential helium resources have been found in central and western superimposed petroliferous basins and eastern rift basins in China, research on the enrichment mechanisms and distribution patterns of helium has not been sufficiently carried out. Our unknown about the enrichment mechanism of helium seriously affects the evaluation and exploration of helium resources in China, and at the same time, hinders the development of helium industry.
This article will summarize the content, origin and distribution of helium in natural gas in 11 major petroliferous basins on land in China. Based on this research, a preliminary discussion will be made on the distribution and enrichment mechanism of helium for future helium exploration.
Analytical Methods
Samples from Hetianhe gas field were analyzed in the Key laboratory of the Research Institute of Exploration and Development of PetroChina. Natural gas compositions were determined using an Agilent 6890N gas chromatograph (GC) with He and N2 as the carrier gases. Double thermal conductivity detectors (TCD) and a 30 m × 0.25 mm × 0.25 μm quartz capillary column were used. The inlet temperature was 150°C, and the TCD temperature was 200°C. The initial oven temperature was maintained at 40°C for 7.5 min isothermally, then rose from 40 to 90°C at 15°C/min, and finally rose from 90 to 180°C at 6°C/min.
The on-line analysis was conducted for the measurement of carbon isotopic compositions with a MAT 253 gas isotopic mass spectrometer. Natural gas samples were separated to methane, ethane, propane, butane and CO2 through the chromatography column of a SRI 8610C gas chromatograph. They were then transferred into combustion furnace by carrier gas (He) and oxidized into CO2 by CuO at 850°C. All of the converted species were transferred by carrier gas (He) into MS to measure the isotopic compositions. Dual inlet analysis was performed with international measurement standard of NBS-19 CO2 (δ13C, VPDB = 1.95 ± 0.04‰, International Atomic Energy Agency, 1995) and the stable carbon isotopic values were reported in the δ notation in per mil (‰) relative to the Peedee belemnite standard (VPDB). Reproducibility and accuracy were estimated to be ± 0.2‰ with respect to VPDB standard.
Data on helium content, helium and argon isotopes are collected from published articles. The early published data was mainly measured using the VG5400 mass spectrometer, and the data from papers published in recent years using the Noblesse SFT noble gas mass spectrometry.
Helium Contents and Genetic Types
Helium Content
According to the proportion of mantle-derived helium to the helium in natural gas, China’s petroliferous basins can be divided into three types: western basins which the helium is crust-derived with trace amount of mantle derived; central basins which the helium is only crust-derived; eastern basins which the helium is mixed of crust and mantle (Figure 1). The helium contents (by volume) in natural gases from these basins vary greatly, ranging from 0.0002 to 2.085% (Figure 2). Some natural gas samples with high helium content can be found sporadically in almost every basin (Table 1). At present, helium-rich gas fields include Weiyuan gas field in Sichuan Basin, Hetianhe gas field in Tarim Basin, and Dongping gas field in Qaidam Basin (Figure 1). The helium contents in samples from these gas fields are usually over 0.1%.
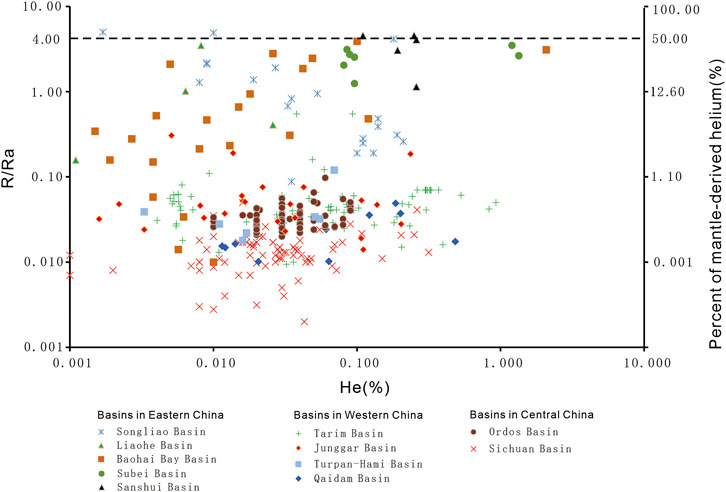
FIGURE 2. Relationship between helium contents and R/Ra rations in major petroliferous basins in China.
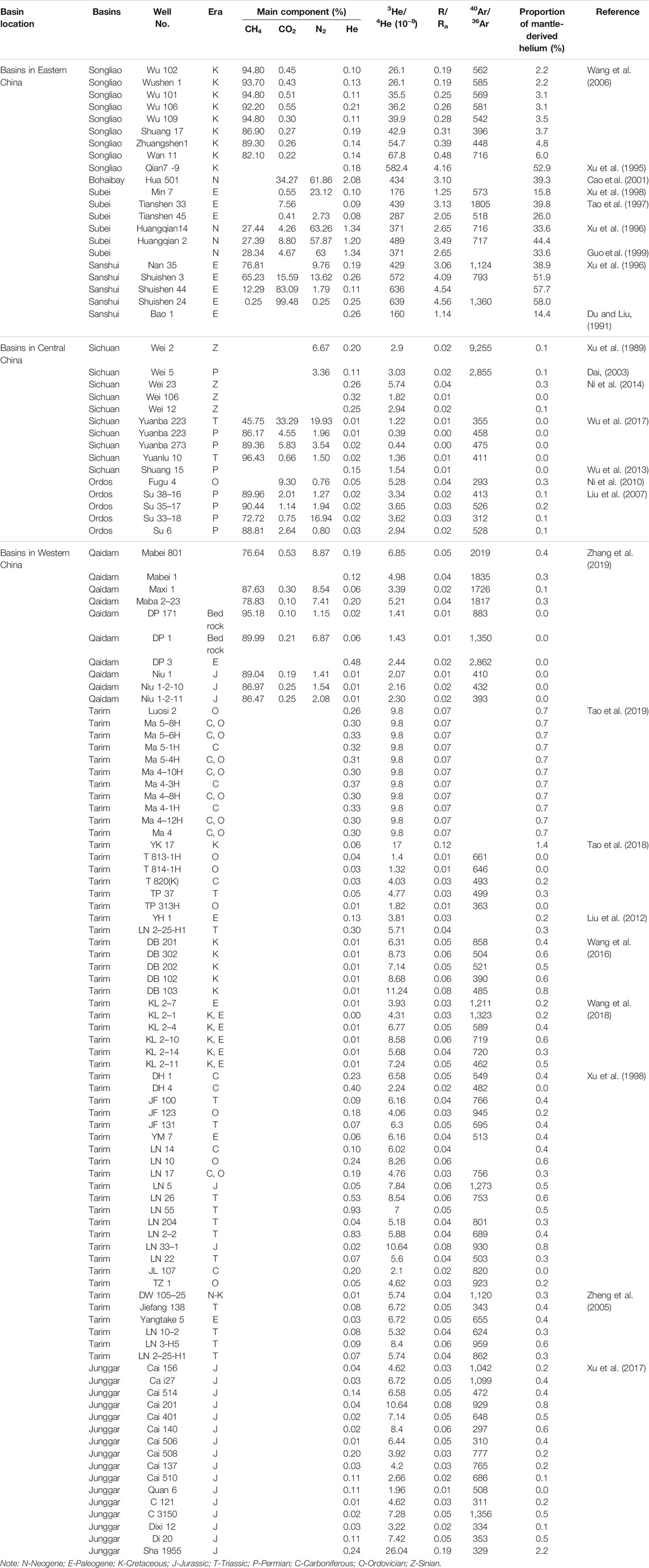
TABLE 1. Table of samples with helium content in some natural gas from petroliferous basins in China.
Helium Genetic Types
The genetic type of helium can be determined using the 3He/4He ratio. Helium in natural gas samples is mainly from 3 sources: crust source, mantle source and atmospheric source (Lupton, 1983; Ballentine and Burnard, 2002; Ballentine et al., 2002). Helium in the atmosphere can be brought into the underground environment and released into gas reservoir through dissolution into surface meteoric water. Because the content of helium in the atmosphere is very low, helium brought into the gas reservoir through surface water can be ignored. Therefore, the sources of helium in a gas reservoir should be mainly derived from the crust and mantle. Crust-derived helium comes from the decay of radioactive elements of U and Th in crustal rocks, while mantle-derived helium comes from the mantle, which is brought into the crust through magmatic activity. Helium has two isotopes, which are 3He and 4He. 3He is predominantly primordial, while both mantle-sourced and crust-sourced 4He are mainly radiogenic. Helium derived from different sources have different ratios of 3He/4He. The 3He/4He ratio has been used for years to determine the origin and source of helium. According to previous studies, the values of 3He/4He ratios in the atmosphere and crust are 1.4 × 10−6 and 2 × 10−8 respectively (Mamyrin and Tolstikhin, 1984). The 3He/4He ratio of 1.1 × 10−5 is usually used as the endmember of mantle-sourced helium (Kaneoka and Takaoka, 1985). The 3He/4He ratio of sample (R) normalized to the atmospheric 3He/4He value (Ra) is often used to show the helium isotope characteristics of the sample, that is R/Ra = (3He/4He)sample/(3He/4He)atmosphere. On average, the 3He/4He ratio in the sub-lithospheric mantle can be taken as 6Ra (Dunai and Baur, 1995; Ballentine et al., 2002). In this study, we consider samples with >0.1% (vol) helium concentrations as helium rich samples, because they exceed the atmospheric helium abundance by a factor of 200. As no natural processes are known to concentrate atmospheric helium in such concentrations, we assume that atmospheric helium does not play a significant role during helium enrichment, and hence, no atmospheric correction (e.g. by using 20Ne, 36Ar or N2) was applied to the helium isotopic ratios. Binary method can be used to calculate the proportion of mantle-derived helium in natural gas samples, following the equation below (Xu, 1997):
According to the equation, when R/Ra > 3.94, mantle-derived helium in natural gas is more than 50% of the total helium in the gas; when R/Ra > 1, the share of mantle-derived helium is more than 12%; when R/Ra > 0.1, the share of mantle-derived helium is more than 1.1%. Therefore, when R/Ra < 0.1, it is considered that the helium in natural gas mainly comes from the crust.
Helium isotopic ratios vary in a large range in petroliferous basins in China. R/Ra range from 0.002 to 4.99. However, distribution of helium isotopes shows a strong regular pattern. Relatively high 3He content is found in the eastern basins and lower 3He content is found in the central and western basins in China. According to analytical data from references of more than 350 samples, the R/Ra values in samples from petroliferous basins in Eastern China, such as Songliao Basin, Bohai Bay Basin, Sanshui Basin and Subei Basin, are between 0.01 and 4.99, with an average of 1.64 (Xu et al., 1995; Xu et al., 1996; Guo et al., 1999; Cao et al., 2001; Wang et al., 2006; Dai et al., 2017). Most samples show clear mantle-derived helium, and the genetic type of helium in these basins is a mixed crust-mantle type. The R/Ra values in samples from Sichuan and Ordos basins in Central China are between 0.002 and 0.097, with an average of 0.024 (Xu et al., 1989; Dai, 2003; Wu et al., 2013; Ni et al., 2014). It is clear that there is no addition of mantle-derived helium in these basins and all helium is of crustal origin. The R/Ra values in natural gases from Tarim Basin, Junggar Basin, Qaidam Basin and Turpan-Hami Basin in Western China are between 0.01 and 0.55, with an average of 0.06 (Xu et al., 1998; Liu et al., 2009; Liu et al., 2012; Xu et al., 2017; Tao et al., 2019; Zhang et al., 2019). Some samples show trace amount of helium from mantle source, and helium is also of crustal origin in general (Figure 2). It can be seen that the genetic types of helium in China have obvious characteristics of east-west zoning, which is related to the tectonic pattern in China. The petroliferous basins in Eastern China are extensional basins, and their development is related to the uplift of the upper mantle (Li, 1982). Therefore, mantle-derived helium is present. The petroliferous basins in Central China are intra-plate polycyclic depression basins, which are the most stable craton basins. The petroliferous basins in Western China are compressional basins, and the tectonic activity in this area is slightly stronger than that in Central China and much weaker than that in Eastern China (Li, 1982).
Although there is more mantle-derived helium mixed into the natural gases in the eastern basins, according to our calculation, for the samples with helium contents higher than 0.1% in the natural gases of the eastern basin, the majority of helium in the samples still comes from the radioactive decay of U and Th in the crust. A few samples with more than 50% of helium derived from mantle are mainly from non-hydrocarbon gas reservoirs with high N2 or CO2, which may be related to local volcanic activity. Volcanic activity brought a large amount of mantle fluid, and the fluid contains not only helium but also a large amount of non-hydrocarbon gases. The proportion of mantle-derived helium in gas reservoirs dominated by hydrocarbon gases is generally less than 50% (Table 1).
Helium Distribution Patterns
Previous studies have shown that helium contents in natural gases are related to geological ages of the storage reservoirs. In general, it follows a pattern that the older the geological age, the higher the helium content (Kong, 1997) (Figure 3). Some researchers suggested that the older the reservoir was, the earlier the helium would migrate to the reservoir, therefor, the more helium would be accumulated and the higher content of helium (Brown, 2010). However, by analyzing the relationship between helium content and reservoir age in China’s petroliferous basins, the above-mentioned helium distribution pattern is not obvious (Figure 4). The oldest Sinian gas reservoir is about 700–800 million years old. The samples with helium content greater than 0.1% are mainly from the Weiyuan gas field in the Sichuan Basin. In Paleozoic reservoirs, samples with a helium content of more than 0.1% only come from the Hetianhe gas field in the Tarim Basin. In the Paleozoic, Mesozoic, and Tertiary reservoirs, helium content distribution patterns are relatively consistent, and do not show a trend that the helium contents increase with the increasing ages of the reservoirs. In addition, according to the cumulative effect of argon isotope 40Ar, which is generated by radioactive decay of K, the older the geological formation is, the higher the 40Ar/36Ar ratio in the samples from the formation should be. However, the helium contents in samples from various petroliferous basins in China do not increase with the increase of 40Ar/36Ar ratios in samples (Figure 5). This demonstrates the complexity of helium-rich gas accumulation in China’s petroliferous basins. The degree of helium enrichment cannot be determined solely from the age of the geological strata where gas reservoirs are situated.
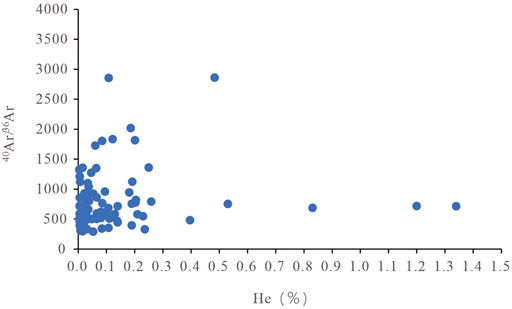
FIGURE 5. Relationship between helium contents and argon isotopic ratios in China’s petroliferous basins.
In this paper, the Weiyuan gas field, Anyue gas field and Hetianhe gas field, which have sufficient amount of analytical data for helium contents in gas samples (Dai et al., 1999; Dai, 2003; Zhu et al., 2007; Wei et al., 2014; Tao et al., 2019), are selected as case studies for the horizontal and vertical distribution of helium to reveal certain patterns of helium distribution and enrichment mechanisms.
The Weiyuan and Anyue gas fields are located on the Leshan-Longnüsi paleo-uplift in the Sichuan Basin, which is a large nose-like paleo-uplift that dips to the east (Figure 6A). In 1964, industrial gas flow was obtained from the Sinian Dengying Formation in the Weiyuan structure, and the Weiyuan gas field, the largest and oldest gas field in China at that time, was discovered. Subsequently, 7 exploratory wells were made in the Ziyang area to the north of the Weiyuan gas field, 3 industrial gas wells were obtained, and a small gas field in Ziyang was then discovered (Figure 6B). In 2011, the Anyue gas field with reservoirs of the Sinian Dengying Formation and Cambrian Longwangmiao Formation was discovered on the Gaoshi-Moxi structure to the northeast of the Weiyuan gas field. This discovery achieved a major breakthrough in natural gas exploration in the Sinian-Cambrian strata in the Leshan-Longnüsi paleo-uplift in Sichuan Basin. The scale of its reserves has exceeded one trillion cubic meters (Wei et al., 2015).
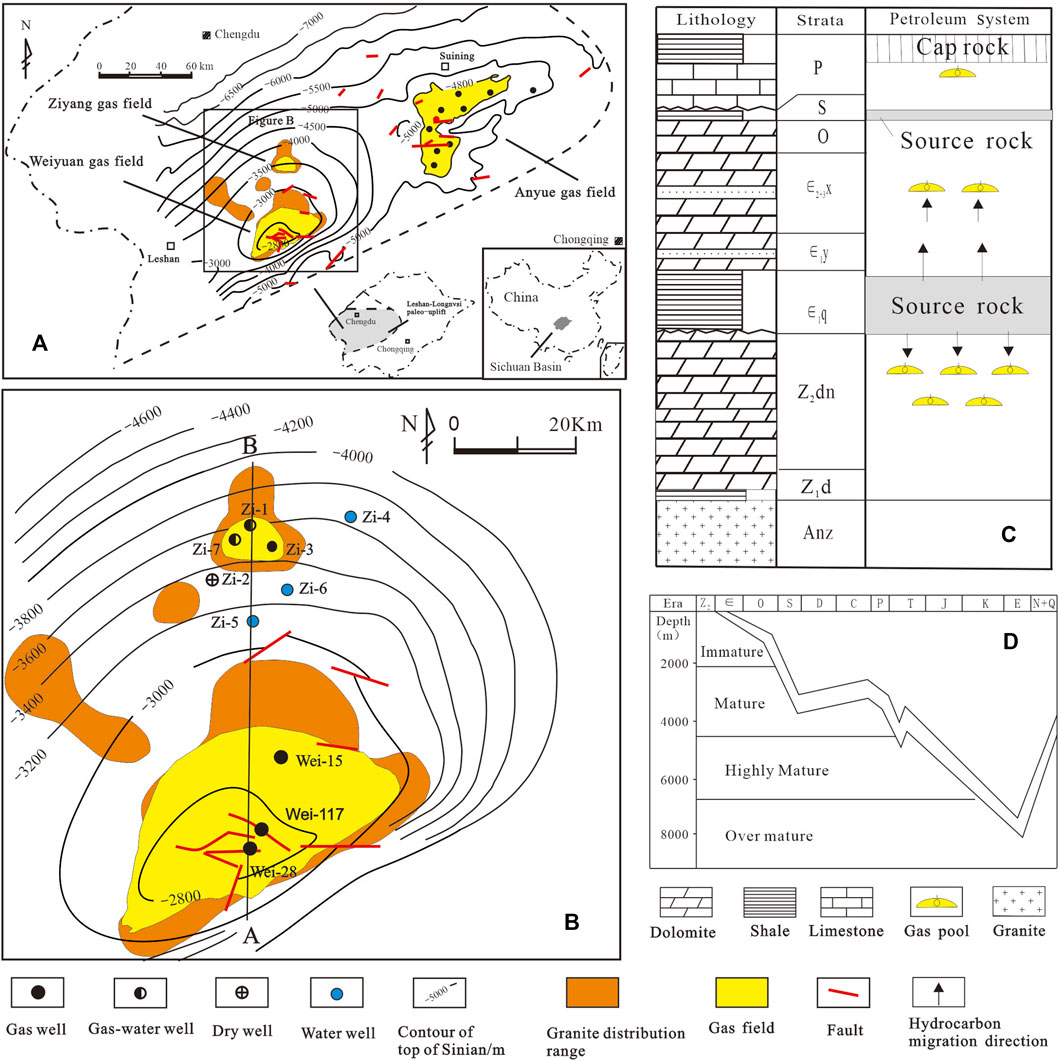
FIGURE 6. Gas field and granite distribution map of Leshan-Longnüsi paleo-uplift in Sichuan Basin, China (The location of Weiyuan gas field is shown in Figure 1). (A) Gas fields and granite distribution on Leshan-Longnüsi paleo-uplift. (B) More details of Weiyuan and Ziyan gas fields. (C) Petroleum system of Leshan-Longnüsi paleo-uplift. (D) Tectonic evolution of Leshan-Longnüsi paleo-uplift.
The main gas reservoirs of the Weiyuan gas field are located in the Sinian Dengying Formation (Z2d) and the Cambrian Xixiangchi Formation (∈2x). Small gas reservoirs are also developed in the Lower Permian (P1). The main gas reservoirs of the Anyue gas field are developed in the Dengying Formation and the Cambrian Longwangmiao Formation (∈1l). Natural gas is dominated by hydrocarbon gases, and non-hydrocarbon gases are mainly CO2, N2 and H2S, as well as a small amount of noble gases. Natural gas mainly comes from the high-over-mature argillaceous source rocks of the Lower Cambrian Qiongzhusi Formation (∈1q), as indicated by δ13C values of methane between -37 and -32 per mil (Figure 6C, Figure 9) (Dai et al., 1999; Zheng et al., 2014).
Increase of Helium Contents With Reservoir Ages
Both in the Weiyuan gas field and Anyue gas field, the helium content is related to the age of the reservoir. It is observed that the older the reservoir age, the higher the helium content (Figure 7). The average helium content in the samples from the Sinian Dengying Formation in the Weiyuan gas field is 0.28%. The average content of helium in the samples from the Cambrian Xixiangchi Formation is 0.18%. The average content of helium in the samples from the Lower Permian is only 0.056%. The average helium content of the samples from the lower gas reservoir (Z2d2) of the Dengying Formation in Anyue gas field is 0.05%. The average helium content in samples from the upper gas reservoir (Z2d4) of the Dengying Formation is 0.017%. The average helium content in the samples from the gas reservoir of the Cambrian Longwangmiao Formation is 0.0078%.
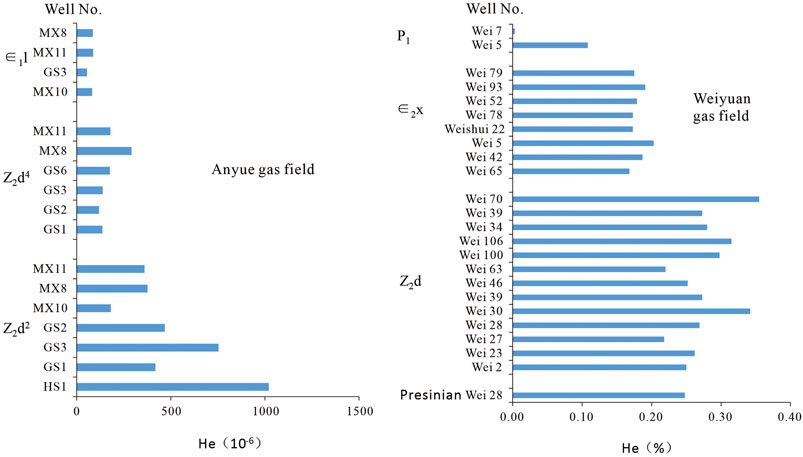
FIGURE 7. Helium contents in natural gases in different layers in the Anyue and Weiyuan gas fields (Data sited from Zhu et al., 2007; Wei et al., 2014; Zheng et al., 2014; Zhang et al., 2015).
The gas reservoirs of the Hetianhe gas field in the Tarim Basin are mainly Ordovician and Carboniferous. The gas layers are thin and adjacent to each other. There is no obvious change in helium content in the vertical successive geological formations (Figure 8).
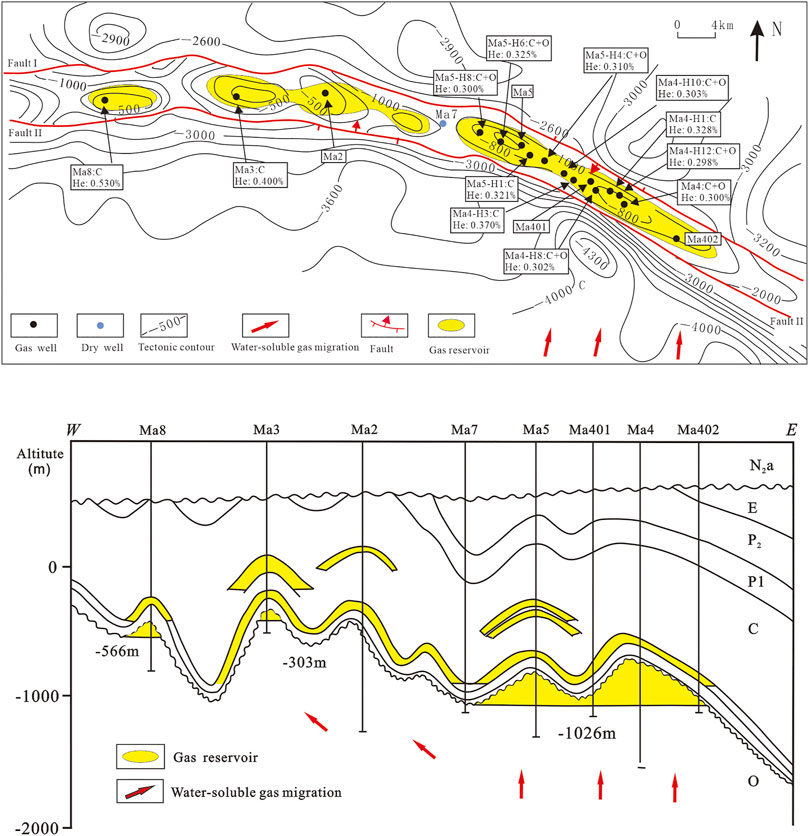
FIGURE 8. Schematic diagram of water-soluble gas accumulation in Hetianhe Gas Field (The location of Hetianhe gas field is shown in Figure 1; The helium data are cited from Tao et al., 2019).
Concentration of Helium in the Structural High Parts of the Same Horizon
The Anyue gas field has a relatively gentle structural slope, and the helium content does not vary significantly within the structure. However, in the Weiyuan structure, the helium content is closely related to the structural altitude. Both Weiyuan and Ziyang gas fields have been discovered on the Weiyuan structural belt, and tectonic movement that occurred after the Late Cretaceous caused the Weiyuan gas field rising much more than the Ziyang gas field (Huang and Chen, 1993; Dai, 2003), which made Weiyuan gas field a new high point on the Weiyuan structural belt (Figure 6D). A huge ascending slope formed from the Ziyang gas field to the Weiyuan gas field. The helium contents in samples from the Dengying Formation gas reservoirs gradually increased from the Ziyang gas field to the Weiyuan gas field. The average helium content in samples from the Ziyang gas field is 0.027%, while the helium content in the samples from the Weiyuan gas field is 0.277%, and the average content of helium in the transition zone between the two reservoirs is 0.205% (Dai et al., 1999; Dai, 2003; Zhu et al., 2007) (Figure 9).
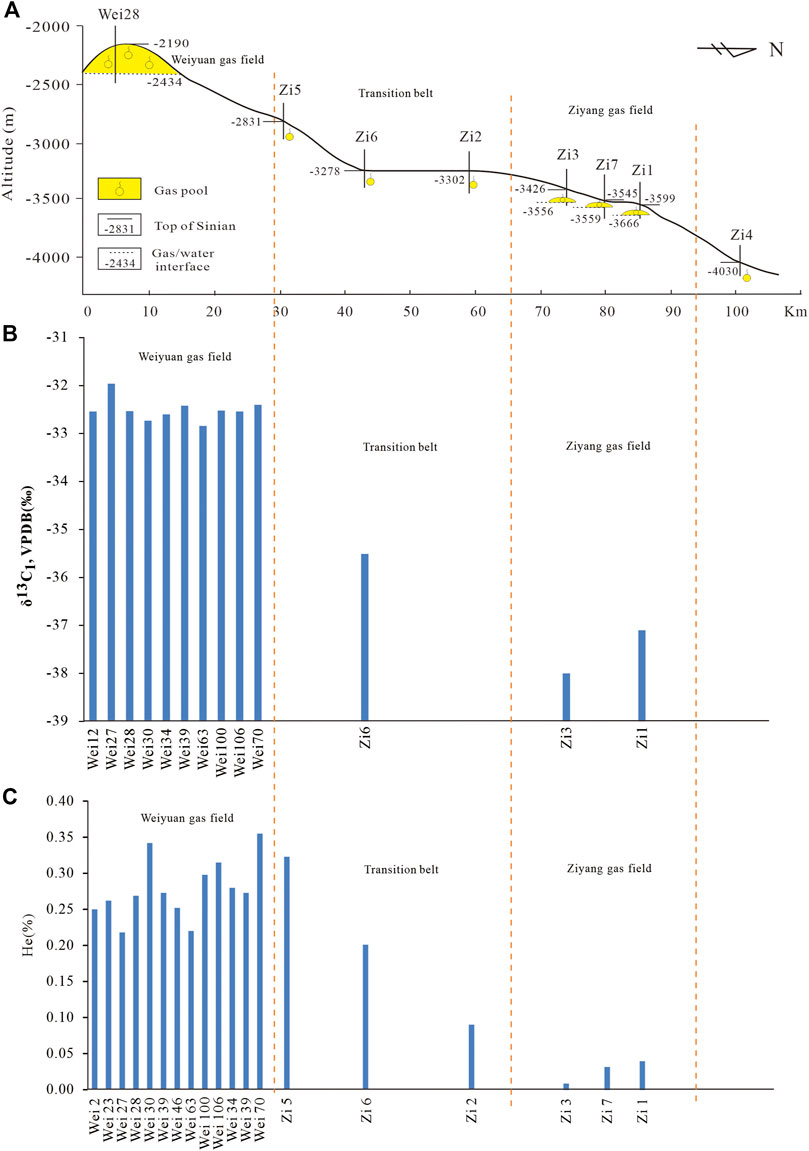
FIGURE 9. Helium content of natural gases in the Sinian Dengying Formation in the Weiyuan gas field and its adjacent areas. (The location of profile AB is shown in Figure 7. The helium data cited from Dai et al., 1999; Dai, 2003; Zhu et al., 2007). (A) Section of Weiyuan gas field and adjacent area. (B) The δ13C1 of Weiyuan gas field and adjacent area. (C) The helium content of Weiyuan gas field and adjacent area.
The phenomenon that helium in samples from the same geological formation is often enriched in high structural parts also occurs in the Hetianhe gas field in the Tarim Basin. The Hetianhe gas field is located on the Mazatag fault structural belt in the Bachu bulge in the eastern part of the central uplift of the Tarim Basin. The gas field is generally a long strip extending east-west (Figure 8). The gas field is composed of a series of structural high points. Among them, the wells on the high points of Ma 8 and Ma 3 in the west constitute of western well area, and the wells on high points of Ma 5 and Ma 4 constitute of eastern well area. The high point of Ma 2 is between the eastern and western wells. In general, the western structure of the gas field is higher than the eastern one, and the gas-water interface in the western well area is higher than those in the eastern well area. The contents of helium in the western wells are significantly higher than those in the wells in the eastern area (Figure 8). The helium content of wells Ma 8 and Ma 3 are 0.53 and 0.4%, respectively. The Ma 4 and Ma 5 well areas is between 0.298–0.328%, which is lower than the western well area.
Helium in helium-rich reservoirs mainly comes from deep basement rocks. The helium produced by the long-term decay of U and Th in the rock is dissolved in water and preserved. With the tectonic movement of Himalayan, the formation was uplifted, and some deep and large faults connecting the deep formation water and the overlying gas reservoir were generated. Driven by tectonic movement, the deep formation water with dissolved helium migrated up along the fault, and the released helium was mixed into the gas reservoir to form a helium-rich gas reservoir. Since the deep fluids tend to migrate to the high point of the structure, more helium was released from the formation water at the position of the high point of the structure.
Helium Enrichment Mechanism—Degassing of Dissolved Gas
Although U and Th concentrations are enriched in the crust relative to the mantle, they are still very low in the crust and their half-lives are extremely long. The helium produced by radioactive decay of U and Th is extremely dispersed in the crust. Due to these facts, helium gas reservoirs cannot be formed independently. It needs to be transported by other fluids in order to enrich and reach an industrial production level. The carrier phases may be other types of gases, or formation water. The study of the Weiyuan and Hetianhe gas fields has shown that the carrier phase is likely to be formation water other than other natural gases.
Weiyuan Gas Field
Previous studies have suggested that the helium in the Weiyuan gas field is of crustal origin via radioactive decay of U and Th in the Earth’s crust (Dai, 2003). However, the specific source of helium is difficult to determine. The helium may come from the source rocks in the Qiongzhusi Formation, which have high U and Th concentrations, or from the basement granite below the gas field. The U and Th concentrations of the Qiongzhusi Formation increased with the increase of TOC. The average concentrations of U and Th are 29 ppm (Zhao et al., 2019) and 11 ppm (Wang et al., 2021), respectively. The U and Th concentrations of the underlying granites are 6.7 and 32.5 ppm, respectively, (Gu et al., 2013). In view of the natural gas accumulation conditions, such as the source rocks, reservoirs, cap rocks, etc., the natural gas reservoirs in the Dengying and Longwangmiao formations in the Anyue gas field are similar to those in the Weiyuan gas field. The natural gases in both reservoirs dominantly come from Qiongzhusi Formation, which are the same source rocks in the Lower Cambrian (Dai, 2003; Wei et al., 2015). However, the helium content in the Weiyuan gas field is much higher than that in the Anyue gas field. This suggests that helium is unlikely originated from the hydrocarbon source rocks or the reservoirs themselves.
There is large granite body under the Sinian in the Weiyuan structural belt (Zhang et al., 2015), but there is no granite body beneath the Anyue gas field (Figure 6). Granite is considered by some researchers to be the main contributor to the helium in helium-rich gas reservoirs (Brown, 2010). This may be the main reason why natural gas in the Weiyuan gas field is rich in helium. Helium is likely coming from the pre-Sinian granite basement, where radioactive uranium and thorium are present. Radioactive decay of uranium and thorium can produce helium which is accumulated in the Weiyuan gas field afterwards. However, although both the Weiyuan gas field and Ziyang gas field are located in the area of the granite basement, they demonstrate different helium contents in the samples, where helium content in the natural gas in the Ziyang field is lower than that in the Weiyuan gas field. This can be explained by the special gas forming process of the Weiyuan gas field, which is the degassing of water-soluble gas during the gas pool formation. We ruled out the possibility that in the over-mature stage of the source rock, due to the small amount of gas generated, the degree of dilution of helium by natural gas was low, resulting in a higher content of helium in natural gas, because the natural gas generated before the source rock reaching the over-mature stage would migrate away with the helium generated by the decay of U and Th in the source rock during a long geological period. In the over-mature stage of the source rock, the time of U and Th decay is very short, and the amount of helium generated is fairly small, therefore, it was unlikely to be enriched in the over-mature natural gas.
The gas generation period of the source rocks in the Weiyuan gas field is before the Himalayan tectonic movement, then the trap is formed and shaped afterwards. Since the trap is formed later than the hydrocarbon generation period of the source rock, the natural gas generated by the source rock cannot be captured effectively. Therefore, gas accumulated in the reservoir is not directly from the source rock in the area. It is proposed that the natural gas in the Weiyuan gas field is migrated laterally from the Ziyang paleo-uplift. Before the Himalayan period, Ziyang was a structural high of the paleo-uplift, and oil and gas were migrated to and accumulated in the Ziyang area. After the Himalayan period, the new structural trap in the Weiyuan formed by tectonic uplift was higher than the trap in the Ziyang area, which led to the reverse migration of natural gas originally accumulated in the Ziyang Sinian paleo-gas reservoir. The gas was then re-accumulated in the present Weiyuan anticline trap (Yin et al., 2000; Wang et al., 2001).
The geochemical characteristics of the natural gas in the Weiyuan and Ziyang gas fields should be relatively similar, if the natural gas in the Weiyuan gas field had migrated laterally from the Ziyang gas field in the form of free gas. In addition, due to fractionation during migration, the carbon isotope signature of methane in the Weiyuan gas field should be a little more negative than that in the Ziyang gas field. However, the actual situation is opposite. The carbon isotope composition of methane in gas samples from the Weiyuan gas field is significantly less negative than that of the gas from the Ziyang gas field (Figure 9). The average δ13C1 in Weiyuan is −32.5‰, and Ziyang is −36.9‰. This does not explain the lateral movement of natural gas.
In fact, geological conditions at the Weiyuan gas field are favorable for the gases (including helium) to be stored in groundwater, then degas and accumulate in the current reservoir. In the area where the reservoir is located, there is abundant groundwater, which can dissolve large amount of natural gas, forming a considerable reserve of water-soluble gas. The formation water in the Weiyuan gas field is ancient connate water, which provides favorable condition for the preservation of water-soluble gas. The Himalayan structural movement uplifted the formation and caused degassing of the water-soluble gas and migration of the gas into the current traps (Qin, et al., 2016). Previous studies have shown that the carbon isotopic values of methane degassed from water is significantly less negative than that of the samples collected at the wellhead at the same time (Qin, 2012). Therefore, the less negative carbon isotopic values in samples from the Weiyuan gas field support the argument that natural gas in this field mainly comes from gas previously dissolved in formation water. At the same time, the helium produced by the radioactive decay of U and Th from the granite in the basement of the Weiyuan gas field is migrated out of the mineral lattice and dissolved into the pore water. Due to the uplift of geological formations during Himalayan structural movement in the Sichuan basin, the helium dissolved in the formation water was exsolved due to the decrease of pressure and temperature. In addition, near the gas-water interface, the partial pressure of helium in the gas reservoir is extremely low, while the partial pressure of helium in water is relatively high. Helium can easily enter the gas reservoir from water and accumulate with natural gas in the trap to form helium-rich natural gas reservoir. This can explain the formation of a helium-rich gas reservoir in the Weiyuan gas field. The tectonic movement in the Himalayan period lifted the Weiyuan gas field by 4,000 m, and the structural high point was transferred from Ziyang to Weiyuan. As a consequence, groundwater migrated to the Weiyuan gas field, causing dissolved-methane in groundwater to exsolve in the Weiyuan gas reservoir. The groundwater from deep granite also migrated to the Weiyuan gas field, where the dissolved helium gas is released to form a helium-rich gas field. In the Ziyang gas field, which is at the north of the Weiyuan gas field, due to the small uplift during the Himalayan period, the groundwater in the deep granite was migrated to the Weiyuan gas field, but did not move upwards, resulting in a lower helium content in the Ziyang gas field. (Figure 10).
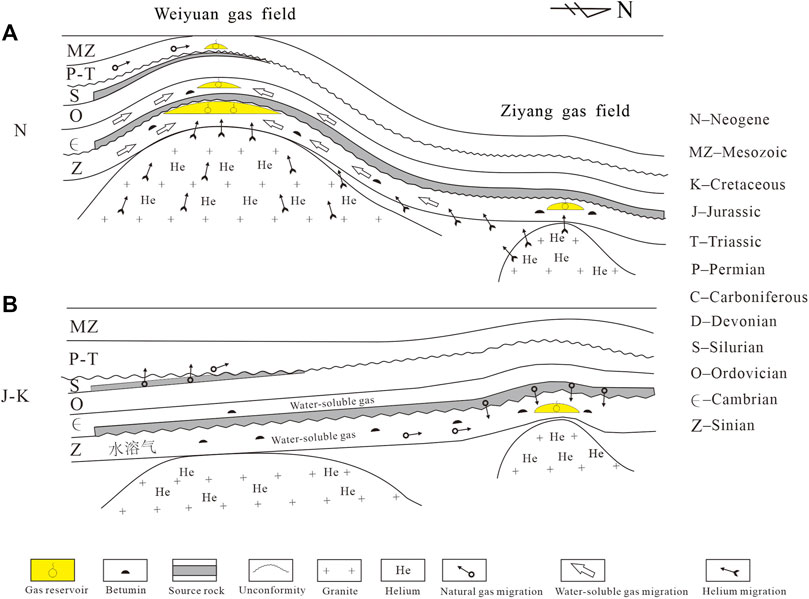
FIGURE 10. The helium Enrichment mechanism of Weiyuan gas field. (A) Structure map after the Himalayan period. (B) Structure map before the Himalayan period.
Hetianhe Gas Field
The helium accumulation mechanism at the Hetianhe gas field is similar to that at the Weiyuan gas field. It is also related to the water-soluble gas. The natural gas in the Hetianhe gas field mainly comes from the high-over-mature Cambrian marine source rocks in the Southwest Depression to the field (Zhao, 2000; Cai et al., 2002; Qin et al., 2002). Carbon isotopic values of methane in the western part of the field are significantly less negative than those in the gas in the eastern part of the field. (Table 2, Figure 8). The helium content in the western area is also significantly higher than those in the eastern area (Figure 8). Obviously, the less negative of δ13C1, the higher the helium content. It shows that the formation water is more fully degassed in the western region than in the eastern region. This can also be seen from the trends in CO2 content. Gas reservoirs in the west of the field have higher CO2 content than in the east. CO2 is more soluble in water, and it will be released later from the formation water. The formation water of the gas field migrates from the east to the west of the gas field.
Gas traps in the Hetianhe field were formed mainly during the late Himalayan movement. Large amount of natural gas was generated in the over-mature stage of Cambrian source rocks in the Southwest Depression. They were strip-shaped traps sandwiched by two reverse faults in the south and north, which were formed under the compression stress of the Himalayan movement (Figure 8). These two large faults are 90 km in length, and they cut the geological stratum from Cambrian to Neogene. At the same time, the Bachu bulge continued to uplift, causing FaultⅠand FaultⅡto move and open again. It is during this time, the natural gas produced by the deep Cambrian high and overmature source rocks was dissolved into formation water under the abnormally high pressure. Then gas and water migrated upward along the effective migration channel in the eastern part of the gas field to the trap in the lower eastern part of the Hetianhe gas field. Then part of the released free gas accumulated in the eastern trap of the gas field. The water below the gas-water interface in the eastern well area continued to migrate laterally along the unconformity for a long distance from the eastern high-pressure area to the western low-pressure area. The continuous decrease in pressure during the migration of the water body caused the continuous release of water-soluble gas to form free natural gas phase, which accumulated along the migration path, forming the Hetianhe gas field in a strip-like reservoir (Qin et al., 2006). The long distance gas migration has caused the difference in the geochemical characteristics of natural gas in the east and west areas of the gas field (Qin et al., 2002). Although research is ongoing on whether there is an ancient granite basement in the Southwest Depression of Tarim Basin, U and Th in the ancient basement strata and Cambrian source rocks have produced considerable amount of helium gas through radioactive decay over a long period of time. The helium has been dissolved in the formation water and released into the upper trap along with the uplift during the Himalayan movement. Helium is accumulated together with natural gas in the Hetianhe gas field.
Helium-Rich Gas Fields in Eastern Basins
Although the features of helium-rich gas fields in eastern basins of China are remarkably different from those in Sichuan and Tarim basins, however, the helium is mainly from deep water in most of the helium-rich gas fields in eastern basins. Despite that the helium is crust-mantle mixed origin, the proportion of mantle-derived helium is much less than 50% in most of the fields, except a few non-hydrocarbon gas fields. The helium is still manly from crust. The natural gas produced by source rock diluted the helium produced by itself. Therefore, an additional main source of helium is required to form a helium-rich gas field. This additional source came from deep crust. Helium produced in the deep crust dissolved in water and migrated into the gas fields along fractures created by subsequent magmatic activity, releasing helium.
The helium in many large helium-rich gas fields in the world also comes from water-soluble gas. For instance, the Panhandle-Hugoton gas field. Correlated research of Hugoton-Panhandle giant gas field has showed the association collection, transport, and focusing mechanism for the 4He. The N2*/20Ne ratio study in this area also indicated that the migration mechanism of the gas field must be carried out in geofluid (Ballentine, et al., 2002; Ballentine and Lollar, 2002).
Conclusion
This study suggests that helium in China’s petroliferous basins is mainly produced by radioactive decay of U and Th in the crust and mantle. Due to the existence of deep and large faults in the basins in eastern China, although some mantle-derived helium is mixed in natural gas reservoirs, helium in the basins in eastern China is still mainly composed of radiogenic helium. The distribution of helium-rich natural gas is closely related to ancient granite or ancient basement. Helium gas produced by U and Th decay in the ancient rock formations during a long geological period was dissolved and preserved in the formation water. Tectonic uplift of the geological formations can release the helium gas dissolved in the water together with the dissolved natural gas. They accumulate in the traps to form helium-rich natural gas reservoirs. Along the direction of groundwater flow, the helium contents in natural gas gradually increase, while the carbon isotopic values of methane become heavier. We found that reservoirs with large uplift in geological history tend to have gases with high helium concentrations.
Data Availability Statement
The original contributions presented in the study are included in the article/Supplementary Material, further inquiries can be directed to the corresponding author.
Author Contributions
SQ: work concept or design, drafting papers. DX: data collection. JL: Drawing. ZZ: Make important revisions to the paper.
Funding
This study was jointly sponsored by National Natural Science Foundation of China (Grant No. 41872162, 42141022) and PetroChina Science and Technology Project (Grant No. 2019D-5010-27).
Conflict of Interest
The authors declare that the research was conducted in the absence of any commercial or financial relationships that could be construed as a potential conflict of interest.
Publisher’s Note
All claims expressed in this article are solely those of the authors and do not necessarily represent those of their affiliated organizations, or those of the publisher, the editors and the reviewers. Any product that may be evaluated in this article, or claim that may be made by its manufacturer, is not guaranteed or endorsed by the publisher.
Acknowledgments
We thank the editor for handling this manuscript and reviewers for helpful comments and suggestions that have improved the manuscript.
Supplementary Material
The Supplementary Material for this article can be found online at: https://www.frontiersin.org/articles/10.3389/feart.2022.675109/full#supplementary-material
References
Ballentine, C. J., Burgess, R., and Marty, B. (2002). Tracing Fluid Origin, Transport and Interaction in the Crust. Rev. Mineralogy Geochem. 47 (1), 539–614. doi:10.2138/rmg.2002.47.13
Ballentine, C. J., and Burnard, P. G. (2002). Production, Release and Transport of noble Gases in the continental Crust. Rev. Mineralogy Geochem. 47 (1), 481–538. doi:10.2138/rmg.2002.47.12
Ballentine, C. J., and Lollar, S. B. (2002). Regional Groundwater Focusing of Nitrogen and noble Gases into the Hugoton-Panhandle Giant Gas Field, USA. Geochimica et Cosmochimica Acta 66 (14), 2483–2497. doi:10.1016/S0016-7037(02)00850-5
Brown, A. (2010). Formation of High Helium Gases: A Guide for Explorationists 2010. AAPG Conference. New Oleans, Louisiana, USA: AAPG Convention, 11–14.
Cai, C., Worden, R. H., Wang, Q., Xiang, T., Zhu, J., and Chu, X. (2002). Chemical and Isotopic Evidence for Secondary Alteration of Natural Gases in the Hetianhe Field, Bachu Uplift of the Tarim Basin. Org. Geochem. 33, 1415–1427. doi:10.1016/S0146-6380(02)00111-0
Cao, Z., Che, Y., Li, J., and Li, H. (2001). Accumulation Analysis on a Helium-Enriched Gas Reservoir in Huagou Area, the Jiyang Depression. Pet. Geology. Exp. 23 (4), 395–399.
Dai, H., Wang, S., Wang, H., and Huang, Q. (1999). Formation Characteristics of Natural Gas Reservoirs and Favorable Exploration Areas in Sinian -Cambrian Petroleum System of Sichuan basin. Pet. Exploration Development 26 (5), 16–20.
Dai, J., Ni, Y., Qin, S., Huang, S., Gong, D., Liu, D., et al. (2017). Geochemical Characteristics of He and CO 2 from the Ordos (Cratonic) and Bohaibay (Rift) Basins in China. Chem. Geology. 469, 192–213. doi:10.1016/j.chemgeo.2017.02.011
Dai, J. (2003). Pool-forming Periods and Gas Sources of Weiyuan Gas Field. Pet. Geology. Exp. 25 (5), 473–480. doi:10.1007/BF02974893
Dai, J., Song, Y., Dai, C., Chen, A., Sun, M., and Liao, Y. (1995). Inorganic Gas and its Gas Reservoir Forming Conditions in Eastern China. Beijing: Science Press.
Du, J., and Liu, W. (1991). Helium and Argon Isotopes in Natural Gas in Sanshui Basin. Nat. Gas Geosci. 2 (6), 283–285.
Dunai, T. J., and Baur, H. (1995). Helium, Neon, and Argon Systematics of the European Subcontinental Mantle: Implications for its Geochemical Evolution. Geochimica et Cosmochimica Acta 59 (13), 2767–2783. doi:10.1016/0016-7037(95)00172-V
Gu, Z., Zhai, X., Jiang, X., Huang, P., Qian, H., and Qiao, L. (2013). Geoichemical Characteristics and Tectonic Environment of Basal Granite in Weiyuan Structure, Sichuan Basin. Earth Science-Journal China Univ. Geosci. 38 (Suppl. 1), 31–42. doi:10.3799/dqkx.2013.S1.004
Guo, N., You, X., and Xu, J. (1999). Geological Character of Xiqiao Helium-Bearing Gas Field and Prospecting of Helium-Bearing Natural Gas in North Jiangsu Basin. Pet. Exploration Development 26 (5), 24–26.
Huang, J., and Chen, S. (1993). Hydrocarbon Source Analysis of Sinian Gas Reservoir Forming Conditions of Sichuan Basin:a Case History of Weiyuan Gas Field. Nat. Gas Geosci. 4 (4), 16–20.
International Atomic Energy Agency (1995). Reference and Intercomparison Materials for Stable Isotopes of Light Elements.
Kaneoka, I., and Takaoka, N. (1985). Noble-gas State in the Earth's interior - Some Constraints on the Present State. Chem. Geology. Isotope Geosci. section 52, 75–95. doi:10.1016/0168-9622(85)90008-9
Li, D. (1982). Tectonic Types of Oil and Gas Basins in China. Acta Petrolei Sinica 3 (3), 1–12. doi:10.7623/syxb198203001
Liu, Q., Dai, J., Jin, Z., and Li, J. (2009). Geochemistry and Genesis of Natural Gas in the Foreland and Platform of the Tarim Basin. Acta Geologic Sinica 83 (1), 107–114. doi:10.1016/S1874-8651(10)60080-4
Liu, Q., Liu, W., Xu, Y., Li, J., and Chen, M. (2007). Analysis of Natural Gas Migration and Gas Source in Sulige Gas Field. Nat. Gas Geosci. 18 (5), 697–702. doi:10.3969/j.issn.1672-1926.2007.05.014
Liu, Q., Zhijun, J., Jianfa, C., Krooss, B. M., and Qin, S. (2012). Origin of Nitrogen Molecules in Natural Gas and Implications for the High Risk of N2 Exploration in Tarim Basin, NW China. J. Pet. Sci. Eng. 81, 112–121. doi:10.1016/j.petrol.2011.12.013
Lupton, J. E. (1983). Terrestrial Inert Gases: Isotope Tracer Studies And Clues to Primordial Components in the Mantle. Annu. Rev. Earth Planet. Sci. 11, 371–414. doi:10.1146/annurev.ea.11.050183.002103
Ni, C., Zhou, X., Wang, G., and Wang, Y. (2010). Geochemistry Characteristics and Genesis of Natural Gas in Fuxian Area, Ordos Basin. J. Xi’an Shiyou Univ. (Natural Sci. Edition) 25 (5), 20–24. doi:10.3969/j.issn.1673-064X.2010.05.005
Ni, Y., Dai, J., Tao, S., Wu, X., Liao, F., Wu, W., et al. (2014). Helium Signatures of Gases from the Sichuan Basin, China. Org. Geochem. 74, 33–43. doi:10.1016/j.orggeochem.2014.03.007
Qin, S. (2012). Carbon Isotopic Composition of Water-Soluble Gases and Their Geological Significance in the Sichuan Basin. Pet. Exploration Development 39 (3), 335–342. doi:10.1016/S1876-3804(12)60049-4
Qin, S., Jia, C., and Li, M. (2002). The Difference on Geochemical Characteristics of Natural Gases between East and West Part in Hetianhe Gas Field of Tarim Basin and its Origin. Pet. Exploration Development 28 (5), 16–18. doi:10.3321/j.issn:1000-0747.2002.05.005
Qin, S., Zhou, G., Li, W., Hou, Y., and Lü, F. (2016). Geochemical Evidence of Water-Soluble Gas Accumulation in the Weiyuan Gas Field, Sichuan Basin. Nat. Gas Industry B 3, 37–44. doi:10.1016/j.ngib.2016.02.004
Qin, S., Zou, C., Dai, J., Li, M., Hu, J., Zhang, Q., et al. (2006). Water-soluble Gas Accumulation Process of Hetianhe Gas Field in Tarim Basin, NW China. Pet. Exploration Development 33 (3), 280–288. doi:10.3321/j.issn:1000-0747.2006.03.005
Sun, M. (1994). The Role of Helium Isotopes in Natural Gas Geology. Nat. Gas Geosci. 5 (5), 1–5. doi:10.11764/j.issn.1672-1926.1994.05.1
Tao, C., Liu, W., Yang, H., Luo, H., Wang, J., and Wang, P. (2018). Analysis of Concentration and Isotope Ratio of noble Gases in Natural Gas. J. Chin. Mass Spectrom. Soc., 201–208. doi:10.7538/zpxb.2017.0039
Tao, M., Shen, P., Xu, Y., Fei, F., and Luo, J. (1997). Characteristics and Formation Conditions of Mantle-Derived Helium Gas Reservoirs in Subei Basin. Nat. Gas Geosci. 8 (3), 1–8.
Tao, X., Li, J., Zhao, L., Li, L., Zhu, W., Xing, L., et al. (2019). Helium Resources and Discovery of First Supergiant Helium reserve in China: Hetianhe Gas Field. Earth Sci. 44 (3), 1024–1041. doi:10.3799/dqkx.2018.381
U.S. Geological Survey (2021). Mineral Commodity Summaries. Available at: https://www.usgs.gov/centers/nmic/helium-statistics-and-information.
Wang, J., Chen, J., Wang, T., Feng, Z., Lin, T., and Wang, X. (2006). Gas Source Rocks and Gas Genetic Type in Shuangcheng-Taipingchuan Area of Songliao Basin. Acta Petrolei Sinica 27 (3), 16–21.
Wang, P., Zou, C., Li, X., Ma, L., Jiang, L., Jia, D., et al. (2021). Geochemical Characteristics of Element Qiongzhusi Group in Dianqianbei Area and Paleoenvironmental Signification. Joural China Univ. Pet. (Edition Nat. Science) 45 (2), 51–62. doi:10.3969/j.issn.1673-5005.2021.02.006
Wang, X., Chen, J., Li, Z., Li, J., Wang, D., Wang, Y., et al. (2016). Rare Gases Geochemical Characteristics and Gas Source Correlation for Dabei Gas Field in Kuche Depression, Tarim Basin. Energy Exploration & Exploitation 34 (1), 113–128. doi:10.1177/0144598715623673
Wang, X., Chen, J., Xu, S., Yang, H., Xue, X., and Wang, W. (1992). Geochemistry Characteristics of Hot spring Gases in Earthquake Zone. Sci. China (Series B) 22 (8), 849–854. doi:10.1360/zb1992-22-8-849
Wang, X., Wei, G., Li, J., Chen, J., Gong, S., Li, Z., et al. (2018). Geochemical Characteristics and Origins of noble Gases of the Kela 2 Gas Field in the Tarim Basin, China. Mar. Pet. Geology. 89, 155–163. doi:10.1016/j.marpetgeo.2017.02.013
Wang, Y., Chen, S., and Xu, S. (2001). Natural Gas Accumulation Conditions and Exploration Technology of Upper Proterozoic-Paleozoic in Sichuan Basin. Beijing: Petroleum Industry Press, 166–168.
Wei, G., Du, J., Xu, C., Zou, C., Yang, W., Shen, P., et al. (2015). Characteristics and Accumulation Modes of Large Gas Reservoirs in Sinian-Cambrian of Gaoshiti-Moxi Region, Sichuan Basin. Acta Petrolei Sinica 36 (1), 1–12. doi:10.7623/syxb201501001
Wei, G., Wang, D., Wang, X., Li, J., Li, Z., Xie, Z., et al. (2014). Characteristics of noble Gases in the Large Gaoshiti-Moxi Gas Field in Sichuan Basin. Pet. Exploration Development 41 (5), 533–538. doi:10.1016/S1876-3804(14)60069-0
Wu, X., Dai, J., Liao, F., and Huang, S. (2013). Origin and Source of CO2 in Natural Gas from the Eastern Sichuan Basin. Sci. China Earth Sci. 56, 1308–1317. doi:10.1007/s11430-013-4601-x
Wu, Z., Liu, X., Li, X., Wang, X., and Zheng, J. (2017). The Application of noble Gas Isotope in Gas-Source Correlation of Yuanba Reservoir, Sichuan Basin. Nat. Gas Geosci. 28 (7), 1072–1077. doi:10.11764/j.issn.1672-1926.2017.06.005
Xu, S., Nakai, S., Wakita, H., Xu, Y., and Wang, X. (1995). Helium Isotope Compositions in Sedimentary Basins in China. Appl. Geochem. 10 (18), 643–656. doi:10.1016/0883-2927(95)00033-X
Xu, S., Zheng, G., Zheng, J., Zhou, S., and Shi, P. (2017). Mantle-derived Helium in Foreland Basins in Xinjiang, Northwest China. Tectonophysics 694, 319–331. doi:10.1016/j.tecto.2016.11.015
Xu, Y. (1997). Helium Isotope Distribution of Natural Gasses and its Structural Setting. Earth Sci. Front., 4. 185–190.
Xu, Y., Shen, P., and Li, Y. (1989). The Oldest Gas Pool of China:Weiyuan Sinian Gas Pool, Sichuan Province. Acta Sedimentologica Sinica 7 (4), 3–13. doi:10.1007/s00376-010-1000-5
Xu, Y., Shen, P., Liu, W., Tao, M., Sun, M., and Du, J. (1998). Noble Gas Geochemistry in Natural Gases. Beijing: Science Press.
Xu, Y., Shen, P., Tao, M., and Liu, W. (1997). Geochemistry on Mantle-Derived Volatiles in Natural Gases from Eastern China Oil/gas Provinces (II). Sci. China Ser. D-earth Sci. 40 (2), 315–321. doi:10.1007/BF02877541
Xu, Y., Shen, P., Tao, M., and Sun, M. (1994). Distribution of Helium Isotopes in Natural Gas of Petroliferous Basins in China. Chin. Sci. Bull. 9 (16), 1505–1508.
Xu, Y., Sun, M., Shen, P., and Wang, X. (1990a). Isotopic Measurements of Helium and Their Application in Natural Gas. Exp. Pet. Geology. 12 (3), 316–325.
Xu, Y., Shen, P., Tao, M., and Sun, M. (1990b). Industrial Accumulation of Mantle Source Helium and the Tancheng-Lujiang Fracture Zone. Chin. Sci. Bull. 12, 932–935.
Xu, Y., Wang, X., Wu, R., Shen, P., Wang, Y., and He, Y. (1979). Rare Gas Isotopic Composition of Natural Gases. Geochemica 8 (4), 271–282. doi:10.19700/j.0379-1726.1979.04.001
Yin, C., Wang, T., Wang, S., and Lin, F. (2000). Natural Gas and Petroleum Generation, Migration and Accumulation in Sinian Reservoirs of Weiyuan Area, Sichuan. Geology-Geochemistry 28 (1), 78–82. doi:10.3969/j.issn.1672-9250.2000.01.014
Zhang, J., Yang, W., Yi, H., Xie, W., Xie, Z., Zeng, F., et al. (2015). Feasibility of High-Helium Natural Gas Exploration in the Presinian Strata, Sichuan Basin. Nat. Gas Industry B 2, 88–94. doi:10.1016/j.ngib.2015.02.007
Zhang, W., Li, Y., Zhao, F., Han, W., Zhou, J., Holland, G., et al. (2019). Quantifying the Helium and Hydrocarbon Accumulation Processes Using noble Gases in the North Qaidam Basin, China. Chem. Geology. 525, 368–379. doi:10.1016/j.chemgeo.2019.07.020
Zhao, J., Jin, Z., Lin, C., Liu, G., Liu, K., Liu, Z., et al. (2019). Sedimenrary Environment of the Lower Cambrian Qiongzhusi Formation Shale in the Upper Yangtze Region. Oil Gas Geology. 40 (4), 701–715. doi:10.11743/ogg20190402
Zhao, M. (2000). Special Source of the Natural Gases of the Hotan River Gas Field and the Origin of its Non-hydrocarbon Gases. Geol. Rev. 48 (5), 480–486. doi:10.1080/12265080208422884
Zheng, J., Liu, W., Sun, G., et al. (2005). Characteristics of helium isotopes in natural gas and their tectonic significances in stable and sub-stable basins. Progress in Natural Science, 15(8), 951–957.
Zheng, P., Shi, Y., Zou, C., Kong, L., Wang, L., and Liu, J. (2014). Natural Gas Sources in the Dengying and Longwangmiao Fms in the Gaoshiti Maoxi Area, Sichuan Basin. Nat. Gas Industry 34 (3), 50–54. doi:10.3787/j.issn.1000-0976.2014.03.008
Keywords: petroliferous basin, helium, genetic type, distribution pattern, enrichment mechanism, water-soluble gas
Citation: Qin S, Xu D, Li J and Zhou Z (2022) Genetic Types, Distribution Patterns and Enrichment Mechanisms of Helium in China’s Petroliferous Basins. Front. Earth Sci. 10:675109. doi: 10.3389/feart.2022.675109
Received: 02 March 2021; Accepted: 03 March 2022;
Published: 24 March 2022.
Edited by:
Giovanni Martinelli, National Institute of Geophysics and Volcanology, Section of Palermo, ItalyReviewed by:
Guodong Zheng, Northwest Institute of Eco-Environment and Resources (CAS), ChinaDongya Zhu, SINOPEC Petroleum Exploration and Production Research Institute, China
Copyright © 2022 Qin, Xu, Li and Zhou. This is an open-access article distributed under the terms of the Creative Commons Attribution License (CC BY). The use, distribution or reproduction in other forums is permitted, provided the original author(s) and the copyright owner(s) are credited and that the original publication in this journal is cited, in accordance with accepted academic practice. No use, distribution or reproduction is permitted which does not comply with these terms.
*Correspondence: Shengfei Qin, cXNmQHBldHJvY2hpbmEuY29tLmNu