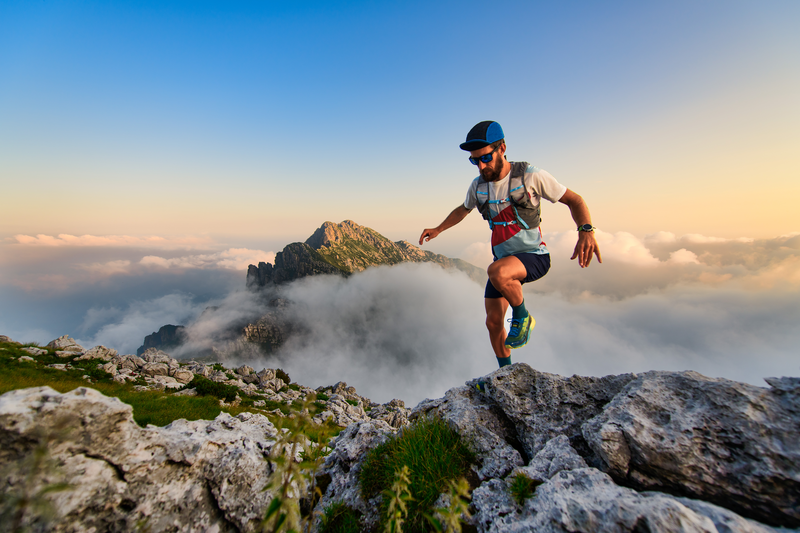
94% of researchers rate our articles as excellent or good
Learn more about the work of our research integrity team to safeguard the quality of each article we publish.
Find out more
ORIGINAL RESEARCH article
Front. Earth Sci. , 06 January 2023
Sec. Geochemistry
Volume 10 - 2022 | https://doi.org/10.3389/feart.2022.1068763
This article is part of the Research Topic Advances in Geochemistry and Macromolecular Structure of Coal View all 8 articles
Understanding the characteristic heavy metals and their migration-transformation behavior in mining areas is essential for the prevention and control of mining pollution. This study selected a gold mine in the Anqing-Guichi ore-cluster region in the Middle-Lower Yangtze metallogenic belt as the research area, the concentrations, and migration-transformation mechanisms of metalloid As and typical heavy metals (Cd, Zn, Pb, Cu, Cr, and Ni) in gold mining wastes (mine tailings and sewage sludge) and the surrounding soil (farmland soil and soil a mining area) were investigated. The results showed that the concentration of As was high in both mining wastes and soils, and the geo-accumulation index values of As in soils ranging from 1.44–6.70, indicated that As pollution was severe in the soil. Besides, a close correlation between the concentration of As and the content of iron was observed by XRF analysis, in conjunction with SEM observations, most As-bearing phases are embedded in Fe, O, and Si compounds. According to EDS and XPS results, the Fe-O-As particle was suggested to be Fe-(oxy)hydroxides with absorbed or co-precipitated As. Furthermore, the arsenic phase observed in the soils were consistent with the weathering oxidation products in the tailings, demonstrating that the mineral particles in the tailings could migrate into soils via atmospheric transport, rainwater leaching, surface runoff, etc., and consequently result in heavy metal accumulation. The sequential chemical extraction result showed that the residual state of As in the soil exceeded 60%, and As posed no risk to low risk according to the Risk assessment code result. However, due to the high concentration and high mobility of arsenic, its environmental impact cannot be ignored even if its bio-accessibility in mined area soil is low.
Different anthropogenic activities can bring distinctive contaminants (Liu et al., 2021). Mining activities lead to air and water pollution, soil acidification, erosion and heavy metals (HMs) pollution (Dudka and Adriano, 1997; Zhuang et al., 2009; Acosta et al., 2011; Li et al., 2014; Quadros et al., 2016). Ore mining and processing generates a large number of mine wastes such as tailings and mineral dust rich in hazardous elements (e.g., As, Hg, and HMs) (Duruibe et al., 2007; Chubb, 2016). These mine wastes are usually comprised of fine particles, easy to disperse to the surrounding environment and accumulate in nearby soil due to their characteristics of fine size and high mobility (Xu et al., 2007; Navarro et al., 2008). However, mine tailings are often left untreated and stored in open tailings ponds without plant coverage, dust suppression and remediation in the past years (Santibañez et al., 2012). Due to improper disposal and stockpiling of tailings, environmental pollution incidents have increased (Lee et al., 2001; Ferreira da Silva et al., 2009). As excessive heavy metals enter the soil, its quality and productivity decline, negatively affecting food security (Alloway, 2013). The crops produced in heavy metal contaminated farmland lead to bioaccumulation in the food chain, posing a threat to humans and ecosystems (Zhang et al., 2015; Lei et al., 2016). Alarmingly, many such farmlands are still being employed for agricultural production because farmers as well as authorities do not know that the soil has been polluted. Senoro et al. (2021) investigated the heavy metal pollution of agricultural production samples from six municipalities that exposed to acid mine drainage for 22–25 years in Marinduque, Philippines, and found that copper and zinc concentration in all the fruits fruit samples exceeded the WHO/FAO limit. Similarly, researches have showed that As, Cd, Mn, etc. are bio-accumulated in crops such as rice, corn and vegetables grown near active and abandoned mining areas (Tabelin and Igarashi, 2009; QingBin et al., 2013; Al-Othman et al., 2016).
Many researchers investigated the contamination level, spatial distribution pattern, and transformation machinimas of arsenic and HMs in the surrounding soil of mining areas (Sherriff et al., 2006; Loredo-Portales et al., 2020; Hao et al., 2022; Saha et al., 2022). Punia and Siddaiah (2019) assessed the mobility and behaviour of Cu, Zn, Ni, Cr, and Pb in mine tailings and neighbouring soils in the Khetri copper mine region, Rajasthan, India, finding the mobility order is Ni > Zn > Cu. The study by Wu et al. (2017) showed that the surface water of mining area was the main carrier of arsenic migration into soil, while wind and microorganisms played an important role in arsenic entering the atmosphere. Loredo-Portales et al. (2020) assessed the mobility and accessibility of As associated with an abandoned tailings impoundment generated by historical mining in central Sonora, Mexico, concluding that As was mainly associated with residual fraction in tailings with low mobility. The spatial distribution of heavy metals in the tailings of a typical lead-zinc mine in Guangdong Province, southern China was clarified to depend on the geochemical characteristics of the tailings. The surface runoff of the tailings reservoir was the main way for heavy metal migration (Chen et al., 2018).
Moreover, the distribution of heavy metals was reported to be affected by many factors such as the physicochemical properties of soil, climate, and occurrences of elements (Hou et al., 2019). Liu et al. (Liu et al., 2022) collected HM data from 263 published papers in China‘s mining areas, showing that the spatial distribution pattern of HM was highest correlated with mine type, clay content, soil organic carbon and precipitation, while HM accumulation was inversely proportional to soil pH and proportional to clay content, precipitation and temperature. Zhong et al. (2020) reported that climate in different regions can influence soil pH, which may further influence the spatial distribution patterns of heavy metals in soils. A study of Mine Stream Sediments from the Baccatoio Basin (Tuscany, Italy) indicated that Mn oxides likely acted as a sorbent for Zn, Ni, Sb, and Pb, iron oxyhydroxide precipitation played a major role in controlling As sequestration (Ghezzi et al., 2021). Additionally, a comprehensive assessment of heavy metals in non-ferrous metal tailings in Xijiao, Baotou, Inner Mongolia Autonomous Region, showed that the release of heavy metal elements from tailings was not only related to the total concentration, occurrence, and mobility of heavy metals, but also influenced by the pH (Jiang et al., 2021). Understanding the characteristic elements and their migration and transformation behavior of heavy metal pollution in mining area is crucial for the prevention and control of HMs pollution in the surrounding areas affected by mining (Akoto and Anning, 2021; Qin et al., 2021).
Numerous studies on heavy metal pollution caused by mining activities have been conducted in China (Liu et al., 2013; Xiao et al., 2017). Guichi District, Anhui Province in China is rich in various metallic such as gold, copper, and iron ore resources, with 139 mining plants. However, researches on soil pollution associated with metal ore mining activities, and the migration-transformation behavior of heavy metal from mine waste to soil in the region are limited. Therefore, the study selected a gold mine in the area as the research area to investigate: 1) the concentrations and occurrence of arsenic and typical HMs (Cd, Pb, Cr, Cu, Zn, and Ni) in gold mine wastes (tailings and sewage sludge) and the soil (farmland soil and other soil inside mining area); 2) the primary pollution element of ore mining on the surrounding environment; 3) the migration-transformation behavior of HMs elements in gold mining wastes and soil; 4) the pollution levels and potential ecological risks.
The Paodaoling gold mine is located in the Anqing-Guichi ore-cluster region in the middle-lower Yangtze River metallogenic belt, southwest Anhui Province, China (Duan et al., 2012) (Figure 1) (for detailed information, see supplementary materials). The sampling points are shown in Figure 1. Eleven samples were collected, including 1) two types of mine tailing mixed samples (Z1–Z6); 2) one sewage sedimentation pond sludge mixed sample (Z7), 3) and two soil samples from the gold mining area (S1, S2) and two soil mixed samples from farmland near the mining area (S3, S4). The sample IDs with details are listed in Supplementary Table S1.
FIGURE 1. Mining boundary and surrounding land use type. Location of study area and the distribution of Sampling.
All the samples were dried naturally in the laboratory, mixed thoroughly, ground, passed through a 200-mesh nylon sieve and stored in sealed bags for different experiments. According to the Chinese National Environmental Protection Standard (Chinese National Standard HJ 962–2018), the pH of each sample was analyzed in a 1:2.5 solid/water suspension using the glass electrode method (pH meter, METTLER TOLEDO, SevenCompact™ S210). After the samples of about 0.1 g were digested with mineral acids (HCl-HNO3-HF-HClO4) by graphite digestion method, the concentration of As, Cu, Cr, Ni, Cd, Pb, and Zn was measured by inductively coupled plasma optical emission spectrometry (ICP-OES, PerkinElmer, AvioTM 200, American), the concentration of Cd was repeatedly measured by inductively coupled plasma mass spectrometry (ICP-MS, ThermoFisher iCAP SQ, American). The limit of detection (LOD) for ICP-OES and ICP-MS are listed in the supplementary materials (Supplementary Table S13). The accuracies of the digestion procedure were controlled by a parallel analysis of the geochemical standard soil (GSS-5), as provided by the National Research Center for Geoanalysis of China. The recovery of the measured elements in the standard substance is between 82.6% and 115%. The reagents and chemicals were all the guaranteed grades.
The Tessier five-step sequential extraction method is an extensively used technique (Tessier et al., 1979) for determining the solid-phase distribution of heavy metals in soil (Tack and Verloo, 1995; Tabelin et al., 2014), mine tailings (Anju and Banerjee, 2010), and sewage sludge (Tack and Verloo, 1995; Tabelin et al., 2014; Huyen et al., 2019); it fractionates the heavy metal elements as: water-soluble and ion-exchangeable (F1), carbonate-bound (F2), Fe/Mn oxide-bound (F3), organic-bound (F4), and residual (F5). Then, 0.5 g of each sample was used for the initial step. The suspension produced from each step was centrifuged, vacuum filtered using a membrane filter (0.45 μm, Millipore, American) and washed using deionized water before the next leaching step. The recovery of the SCE was calculated by dividing the sum of heavy metals masses into five binding fractions by the total heavy metals mass in soil, which is in an acceptable range between 77.5% and 122%.
X-ray Fluorescence Spectroscopy (XRF, Shimadzu, Japan) was used to determine chemical composition. The mineralogy of samples was identified by X-ray Diffraction (XRD, Malvern Panalytical, England) with 2θ intervals of 10–70°. Scanning Electron Microscopy with Energy Dispersive X-ray spectroscopy (SEM-EDS, ZEISS GeminiSEM500, German) was used to investigate arsenic-containing, lead-containing, cadmium-containing and copper-containing phases in samples and the bulk surface chemistry and micro-morphology. Arsenic and Cadmium valence states were analyzed by X-ray Photoelectron Spectroscopy (XPS, ESCALAB 250 Xi, Thermos VG Scientific, American). XPS spectra of As 3d and Cd 3d were obtained with energy step size 0.05 eV, which were recorded using a spectrometer operating in a high vacuum of 5.0 × 10–7 Pa. The standard C (1s) at a value of 284.6 eV was adopted to calibrate binding energy.
The Igeo is a quantitative indicator introduced by Müller in 1969 (Muller, 1969), it has been widely used to assess the extent of sediment and soil pollution. The calculation equation of Igeo is as follows (Eq. 1):
Cn is the measured concentration of each assessed heavy metal element, Bn is the geochemical background value of this element found in the soil (Supplementary Table S4), and factor 1.5 is the natural fluctuation of heavy metal content during diagenesis. Igeo was divided into seven classes (Class0-Class6) (Supplementary Table S2; the highest (class 6) reflects a richness over 100 times higher than the background values (Förstner et al., 1990).
RAC assesses the availability of heavy metals in soil and sediments by calculating the ratio of exchangeable and carbonate factions to the total metals (Xiao et al., 2014). The calculation equation of RAC is as follows (Eq. 2):
Where F1 and F2 represent the concentration of Fraction 1(water soluble and ion exchangeable metals) and Fraction 2 (bound to carbonates metals) of each metal, respectively, TM is the total concentration obtained from the metal (Liu et al., 2008). The grading standard of RAC is shown in Supplementary Table S3.
The concentrations of HMs in mining waste were shown in Figure 2 and listed in Supplementary Table S5. The pH of flotation tailing (Z1) was 3.61, the concentrations of As, Cd, Zn, Pb, Cu, Cr and Ni in Z1 were 943.39, 0.99, 58.25, 39.80, 11.07, 38.25, and 3.52 mg kg−1. The floatation tailing was acidic because flotation was carried out under acidic conditions. The pH value of heap leaching tailings (Z2-Z6) was 2.73–8.58, and the mean concentrations of As, Cd, Zn, Pb, Cu, Cr, and Ni were 1171.03, 2.12, 310.32, 299.38, 306.56, 85.22, and 12.8 mg kg−1, respectively. During oxidation heap leaching of low-grade raw ore, lime was used as a heap leaching agent to adjust the pH during heap building, which was the reason for the alkaline pH of Z3, Z4, and Z5. The concentrations of As, Cd, Zn, Pb, Cu, Cr, and Ni in sewage sludge were 7527.74, 5.43, 290.31, 159.41, 162.01, 43.80, and 9.18 mg kg−1, respectively, and the pH was 2.86, which is strongly acidic.
FIGURE 2. Heavy metal concentrations (mg/kg) in soils (S1–S4), tailings (Z1–Z6), and sludge (Z7) (BV: Background values for soils in Guichi; SV1: Screening value of GB15618-2018; CV1:Controlling value of GB15618-2018; SV2: Screening value of GB15618-2018; CV2: Conltroling value of GB36600-2018)
The maximum concentrations of Cd, Zn, Pb, Cu, Cr, and Ni in tailings and sewage sludge appear in heap leaching tailing (Z2) and the maximum concentration of As appeared was sewage sludge (Z7), which was significantly higher than those in all soil samples. The characteristics of the high range of heavy metals in tailings sand and the easy migration of fine particles make it the leading cause of heavy metal pollution in the surrounding environment of the mining area (Cui et al., 2014).
The concentrations of HMs in soil were shown in Figure 2 and listed in Supplementary Table S6. The highest range of all heavy metals was found in sample S2 inside the gold mine, likely because it was located near the sewage sedimentation basin. The concentrations of As was significantly high in S2 (1907.75 mg kg−1), 13.6 times higher than the risk-controlling values of the Risk Control Standard for Soil Contamination of Development Land (GB36600-2018) (Ministry of Ecology and Environment and State Administration for Market Regulation, 2018b). The concentration of As in sample S1 (268.47 mg kg−1), located 50 m east of the sewage sedimentation basin, far exceeded the risk-controlling values of GB36600-2018 (Ministry of Ecology and Environment and State Administration for Market Regulation, 2018a) as well. This indicates that mining activities had significantly increased arsenic concentrations in the mining area (Liu et al., 2013).
According to the multi-purpose regional geochemical survey in Anhui Province (Jia, 2007), the farmland soils in the study area did not belong to mineralized areas (Table 1). The concentrations of As, Pb, Cd, Cu, and Zn in sample S3 exceeded their corresponding background values (Chun-Hui et al., 2018), indicating that the accumulation of heavy metals in soil has reached a severe level (Wei et al., 2006; Cheng et al., 2009; Xiao et al., 2017). The concentration of As in sample S3 was extremely high (322.56 mg kg−1), which exceeded the risk-controlling values of the Risk Control Standard for Soil Contamination of Agricultural Land (GB 15618-2018), and Cd exceeded the risk-screening values of GB 15618-2018. Gold usually occurs in fine-grained natural gold and lattice gold in the significant As-bearing minerals pyrite and arsenopyrite (Gieré et al., 2003), so the ore-bearing particles from the gold mine is a major As Source. This may be why the arsenic content in farmland adjacent to gold mines is exceptionally high. The pH values of S3 and S4 in farmland soil were 4.18 and 4.60 (acidic), respectively, which may also be caused by weathering and oxidation of pyrite (FeS2). As a result, in gold mining areas rich in As-bearing minerals, significant accumulation of arsenic in the soil (and consequently the food chain) may occur as mining activities proceed (Zhu et al., 2008; Cheng et al., 2009; Islam et al., 2013).
TABLE 1. The geochemical background values of heavy metal elements in soils (Jia, 2007).
The concentrations of As, Zn, Pb, and Cu at point S3 near the gold mine are higher than those at point S4, indicating that the heavy metal content in soil may be related to the location. Generally, the closer farmland soil is to the tailings storage, the more severe the contamination is. (Liu et al., 2013). Through the above analysis, the arsenic pollution in the soil near the gold mine has been severe enough to be unsuitable for agricultural production, and the cadmium pollution is also worthy of attention.
Generally, elements that exhibit a high degree of correlation may have the same origin and, under certain physiochemical circumstances, exhibit similar behaviors during transformation and migration (Zhang et al., 2019). The Spearman’s correlation coefficients for the heavy metal contents of tailings, sludge and soil are shown in Figure 3; Supplementary Table S7. A significantly positive correlation (p < 0.01) was found between Cd-As (0.927) and Cr-Pb (0.764). Meanwhile, five elemental pairs, Cd-Cu (0.709), Cd-Zn (0.655), Pb-Cu (0.691), As-Cu (0.673), Zn-Ni (0.682), had a low positive correlation (p < 0.05). Moreover, the content of heavy metals in soil is closely related to the content of tailing and sludge. As the gold ore grade is generally not high and there are many co- and associated elements, the amount of tailings produced by mining activities is quite large, and the polluting heavy metals are released into the environment through the tailings (Liao, 2005).
FIGURE 3. Correlation analysis of pH value and content of arsenic and HMs in soil, tailing and sludge.
The research showed that the content of As and Cd in tailings and sewage sludge is extremely high, and the enrichment of As and Cd in the soil is profound. Tailings contain solid particles, especially the tailings produced in the beneficiation process; the particle size is mostly 0.17–0.07 mm. Due to their small particle size and low gravity, tailings can easily migrate under the action of hydraulic and wind power, causing heavy metal contamination to the surrounding farmland soil (Santibañez et al., 2012). Zhou et al. obtained a conclusion consistent with the research results of this paper through theoretical simulation calculation (Zhou, 2009).
The toxicity and biological activity of heavy metals in soil, as well as their migration and transformation processes in organisms and the ecological environment, are associated with their different chemical forms in soil, which are in dynamic equilibrium with the changes in soil’s physical and chemical properties and environmental conditions (Liu et al., 2000; Matong et al., 2016). In Figure 4 The occurrences and distribution of arsenic and HMs in tailings, sludge, and soil, are given. Heavy metals in soil (except Cd) mainly exist in the form of residual, organic-bound and Fe/Mn oxide-bound states (average >95%). Cr and Ni are primarily present as residual conditions, at 90% and 84% of the total, respectively. As, Cd, Zn, and Pb residual states are also more than 60%. This indicates that the heavy metal elements in the soil of the study area are mainly contained in the mineral lattice in a residual state, which may be caused by the entry of mining wastewater and mineral dust into the soil (Chubb, 2016; Lian-Ke et al., 2016). Due to its high concentration and high mobility, the environmental impact of arsenic cannot be ignored even if its bioaccessibility in mine soil is low (Kim et al., 2014).
FIGURE 4. Occurrences and distribution of arsenic and HMs in soil, tailings, and sludge. (A) is the occurrences and distribution of arsenic and HMs in soil (S1–S4); (B) the occurrences and distribution of arsenic and HMs in tailing (Z1–Z6), and sludge samples (Z7).
Notably, the water-soluble and ion-exchangeable state of Cd in soil samples S3 and S4 accounted for 8% and 30% of the total, respectively. Cadmium is highly toxic and can be directly used by organisms, causing great harm to organisms (Chen et al., 2012). The Fe/Mn oxide-bound and the organic-bound state of As in S3 and S4 account for 46% and 49% of the total, and the Fe-Mn oxidation and the organic-bound state are considered as potential bio-effective components that may be activated with changes in the external environment and can be used by plants if they are in a strong acid medium (Ma et al., 2020). Therefore, the accumulation of As and Cd in soil pose a potential threat to surrounding farmland and residents, which should be taken seriously.
Although the distribution proportion of arsenic and HMs in the flotation tailings, cyanide-treated tailings and sewage sludge is different, the overall distribution trend is the same, which is mainly in residual, organic-bound and Fe/Mn oxide-bound, and the proportion of water-soluble and ion-exchangeable and carbonate-bound is deficient. Even though the current chemical activity of tailings is relatively stable and its migration ability is weak, which is not directly harmful to the ecological environment, as the sulfur-containing metal minerals are oxidized during the piling process, acidic wastewater is generated, so that some heavy metals with low biological effectiveness are activated and released, and migrate with the wastewater on a large scale, polluting the soil around the mining (Cui et al., 2014).
The XRF semi-quantitative results of each element in the samples showed that the samples contained similar elements, mainly Si, Al, Fe, and K (Supplementary Table S8). The contents of S, Na, Mg, and Ca are not high, and As, Cr, Ni, Cu, Pb, and Zn are extremely low. The highest concentrations of As are present in Z2, Z7, and S2, which is consistent with the highest iron and sulfur content in all samples (Supplementary Tables S5, S6). The high content of Fe and S in the samples may be related to Fe-O, sulfide minerals, and their weathering products. Thus, the observed correlation between As and Fe/S concentrations indicates that the Fe-O and sulfide minerals may play an essential role in regulating arsenic retention in soil (Kim et al., 2014). The highest cadmium content in soil samples appeared in S2, coinciding with the highest pH level and CaO, MnO, and Al2O3 analyzed by XRF in the soil samples. Soil pH directly affects the current form and adsorption amount of Cd in soil. The amount of H+ is inversely proportional to the pH value and the negative charge attached to the soil surface. The increase of negative charge on the soil’s surface benefits the adsorption of Cd2+on the earth’s surface, resulting in increased Cd content in the soil. Meanwhile, the CaO content has a synergistic effect with soil pH; the pH value increases with the increase of CaO content in the soil, increasing the Cd adsorption capacity (Tahervand and Jalali, 2016). Ran et al. confirmed that soils with rich clay content and high pH are usually conducive to the stability of heavy metals (Xiao et al., 2017). There is a positive correlation between the range of Al2O3 and Cd in soil because the higher the content of Al in soil, the more clay minerals, indicating that the clay minerals in the soil also affect the enrichment of Cd (Yang, 2021). Soil Mn, mainly in oxides and hydroxides, can adsorb the element Cd and affect its morphology, rendering it inactive (Baize et al., 2009). Therefore, soil Al2O3 and MnO are positively correlated with Cd, too.
The mineralogical composition of tailings, sewage sludge, and soils were characterized using XRD. According to the XRD analysis patterns (Figure 5), the dominant minerals found in the soil samples are quartz (SiO2) and muscovite (Kal2(AlSi3010) (OH)2). In addition, epidote (Ca2Fe3+Al2 [SiO4][Si2O7]O(OH)) was observed in the S1, epidote, microcline (K [AlSi3O8]), and kaolinite (Al2Si2O5(OH)4) were observed in the S3, microcline was observed in the S4. The tailings and sewage sludge samples have a similar mineral composition to the soil samples. In addition, calcite and kaolinite were observed in the Z2 and epidote in the Z5. Unlike the soil samples, microcline was not observed in tailings and sewage sludge samples.
FIGURE 5. The X-ray diffraction patterns of soils, tailings, and sludge. (A) is the XRD patterns of soil samples (S1–S4); (B) is the XRD patterns of tailing (Z1–Z6) and sludge samples (Z7).
The inorganic mineral component quartz is the most abundant in the samples, namely silica (SiO2), the most stable silicate, belongs to the oxide mineral of the trigonal system, and its adsorption capacity for metal ions is weak (Jia and Sun, 2001). Muscovite and kaolinite belong to clay minerals with layered aluminosilicate structures, with a negative charge on the surface, big specific surface area, and strong adsorption characteristics to the metal cations (Cheng and Reinhard, 2006; Sun et al., 2014). Microcline is one of the feldspar minerals, the most widely distributed minerals in the crust, belonging to the tectosilicate. Its prominent pore structure mineral characteristics are conducive to the adsorption of heavy metals (Li, 2007). Calcite is the most common type of natural carbonate mineral in the earth’s crust; it is often used as an adsorbent for environmental pollution remediation because of its large specific surface area and good adsorption capacity (Zhu, 2016). Epidote is an island silicate mineral, and the surface is mainly exposed to iron, aluminium, and other metal ions. In an aqueous solution, they combine with hydroxide and have a good adsorption capacity for metal ions (Jia and Sun, 2001).
We observed the micro-morphology of the samples by scanning electron microscope (SEM), Supplementary Figure S1 shows that the microscopic morphology of the tailings and sewage sludge surfaces were corroded, and many irregular and, uneven intermixtures of big and small particles we found attached to the surface, while the soil samples were irregular, with heterogeneous soil particles, significant agglomeration and a coarse surface with considerable weathering and erosion. After grinding, flotation, heap leaching and other processes, the surface of flotation tailings and heap leaching tailings are destroyed, and small particles are accumulated and adsorbed on large particles in the form of layered and spherical inclusions. At high multiples, the surface of particles is observed to be uneven and eroded. The irregular surface morphology indicates that the morphology and structure of these contaminated tailings and soils are complex (Lenthe et al., 2018).
The SEM-EDX element map of particles (Figure 6), shows that the areas with high arsenic content high overlap with Si, O, and Fe in samples. Inclusions of As-bearing minerals are irregularly shaped, probably a secondary As-bearing minerals—scorodite (FeAsO4 2H2O), a common weathering product of arsenic-bearing ore deposits which were often observed in mine waste sites and arsenic-contaminated soil (Dove and Rimstidt, 1985; Smedley and Kinniburgh, 2002). There are more than 300 types of arsenic-containing minerals are known, such as sulfides, oxides, arsenates, and arsenides, and arsenic-containing minerals in nature usually exist in the form of sulfides, including arsenopyrite (FeAsS), realgar (As4S4), and orpiment (As2S2) (Adriano, 2001; Jia and Demopoulos, 2005). Au is usually associated with arsenopyrites (FeAsS) and sulfides and a large amount of As-rich mine wastes were produced with the mining and beneficiation of gold ore. The mobilization and migration of As from these wastes into the environment can result from oxidation of arsenopyrites and sulfides. (Leblanc et al., 1996; Dudka and Adriano, 1997; Langmuir et al., 1999; Majzlan et al., 2014). Arsenopyrite (FeAsS) is transformed by weathering into As-rich pyrite (FeS2), which subsequently forms realgar (As4S4) and orpiment (As2S2), eventually creating an oxidized phase like scorodite (Nordstrom and Parks, 1987; Smedley and Kinniburgh, 2002; Corkhill and Vaughan, 2009a). Kim and Batchelor also observed that As and pyrite (FeS2) interact in the anoxic environment to form realgar (As4S4) and orpiment (As2S2) (Kim and Batchelor, 2009b). The oxidation of FeAsS occurs through several oxidation state changes of As and S by biological/non-biological reactions (Corkhill and Vaughan, 2009b). The chemical reactions are simplified as follows:
FIGURE 6. SEM-EDX elemental maps of the soil and slags (S3, Z2). The degree of brightness represents the relative element content, and the higher the relative element content when the brighter the area is.
We have found As-bearing minerals in the mine waste and soil samples based on the SEM-EDS analysis result. More importantly, the arsenic-mineral phases observed in the soil are matched with the weathering and oxidation products of tailings, which seemed to be Fe-(oxy)hydroxides absorbed by co-precipitated As, indicating that arsenic-containing particles in soils may partly from the mining wastes through atmospheric transport, rainwater leaching, overland runoff, and other means (Lee et al., 2020).
We analyzed all of the samples by X-ray photoelectron spectroscopy (XPS), which provides details on the oxidation states of As and Cd and their chemical forms on the surfaces of minerals (Kim and Batchelor, 2009a). The XPS narrow-scan spectra of As (3d) and Cd (3d) are illustrated in Figure 7; Supplementary Figure S2, and their corresponding peak parameters are summarized in Supplementary Tables S9, S10. Except for Z3, Z4, S3, and S4, the deconvolution of the As (3d3/2, 3d5/2) spectrum suggests two types of As-containing phases in the samples: 1) As (III)-O (43.8–45.17 eV), which was arsenite, and 2) As(V)-O (45.49–46.62 eV), which was arsenate. Meanwhile, the major peak of the As 3d spectrum of the farmland soil (S3, S4) and heap leaching tailings (Z3, Z4), which were located at 45.49–46.36 eV, was attributed to As(V)-O and did not present any reduced forms of As, for instance As(III)-O or As sulfide.
In conjunction with SEM observations, most As-bearing phases are embedded in Fe, O, and Si compounds, and we speculated that it might be secondary minerals oxidized from As-bearing minerals—scorodite. The deconvoluted peaks of the As spectrum, which revealed scorodite’s Fe-As(V)-O binding energies (45.49–46.62 eV), further confirmed the presence of scorodite (Tabelin et al., 2020).
Arsenic is a variable element that typically has four oxidations, including As−III (arsine), As0 (metallic arsenic), AsIII (As2O3, arsenite), and AsV (As2O5, arsenate). The different oxidation states of arsenic determine its toxicity and crystal-chemical reactivity (Sharma and Sohn, 2009; Zhao et al., 2010; Gupta, 2011). Among them, AsV is the most stable form because it can be firmly adsorbed on the surfaces of Fe, Al, and Mn oxides, so it is less mobile and toxic than AsIII in the environment (Li et al., 2017). The analysis in 3.4.2 shows that the arsenic in the ore is mainly in the form of metallic arsenide, while the arsenic in the tailings is mostly in the pentavalent oxidation state, indicating that during the mining, flotation and heap leaching process, most of the arsenic in the ore is released and oxidized, arsenic and Fe, O, Si compounds were connected and entered the tailings. At the same time, due to the open-pit accumulation of the tailings pond, no vegetation cover, and no wind and shower protection measures, the mineral particles in the tailings enter the soil near the mine through atmospheric transport, rainwater leaching and overland runoff, resulting in arsenic enrichment (Liao, 2005).
In most soil and mine wastes, As is absorbed to or co-precipitated with Fe (hydr) oxides, clay minerals, and organic matter under standard oxidizing conditions (Welch and Stollenwerk, 2003; Wang and Mulligan, 2006), and may be directly substituted into the crystal structure of a limited number of secondary minerals (Savage et al., 2005) and precipitated as secondary As minerals (Majzlan et al., 2014). Arsenic in soil usually coexists in oxidized and reduced states, and arsenite in acidic and oxidized soil has been found (Masscheleyn et al., 1991), which explains the presence of trivalent arsenic in some samples.
As shown in Supplementary Figure S2, two strong absorption peaks appear around 405.6 ev and 412.5 ev, which are attributed to Cd 3d5/2 and Cd 3d3/2 respectively. Both peaks are asymmetric and after fitting, the binding energies of the Cd 3d5/2 and Cd 3d3/2 peaks are located at 405.37–405.77 ev and 411.85–412.66 ev, respectively. The XPS standard spectrum of Cd combined with the relevant literature shows that Cd is present in the form of CdCO3 (Zhang et al., 2015).
As, Cd, Zn, and Pb residual states are also more than 60%. This indicates that the heavy metal elements in the soil of the study area are mainly contained in the mineral lattice in a residual state, which is very stable, and may be caused by the entry of mining wastewater and mineral dust into the soil.
Results in Supplementary Table S11; Figure 8A indicate that soil was practically uncontaminated by Cr and Ni (Igeo ≤0). For Zn, the Igeo value was <0 (which means practically uncontaminated) except for S2 (0<Igeo<1, which means uncontaminated to moderately contaminated). The Igeo values ranged from −0.09 to 2.03 for Cu. The most heavily polluted sites appear in S2 (2.03, which means moderately to heavily contaminated, the lightest polluted sites appear in S4 (−0.09, which means practically uncontaminated), and S1 and S3 fell in the range of 0–1 (uncontaminated to moderately contaminated). The Igeo levels of Pb at each site were essentially the same as of Cu; the most heavily polluted sites appear in S2 (1.48, which means Moderately contaminated), too.
The Igeo for As was the highest, followed by Cd. The As Igeo values ranging from 1.44–6.70 (moderately contaminated to extremely contaminated), the highest grade of the geo-accumulation index in site S2, reflected a 100-fold enrichment above background values, indicating that As pollution was severe in soil (Ho et al., 2010). For Cd, farmland soil (S3 and S4) was found to have similar levels of contamination (less than 1, which means practically uncontaminated). In contrast, the soil in the mine areas fell in moderately contaminated (S1) and heavily contaminated (S2), respectively.
In farmland soil, the As pollution was severe, but the pollution degree of As decreased significantly with the increased distance from the mining area. Therefore, it can be speculated that introducing As-containing mineral particles into farmland soil by mining wastewater discharge, atmospheric dust fall, and tailings leaching may cause arsenic accumulation in farmland soil near the mining area, which confirms the speculation of 3.1.1.
We calculated the RACs of tailings and sludge to assess the environmental risk of hazardous metals, which is critical for safety control and reutilization of tailings (Supplementary Table S12; Figure 8). According to RACs, Cr poses no risk (RAC <1%) to the environment, while As, Pb and Ni pose no risk (RAC <1%) and low risk (1% ≤ RAC < 10%). Cd and Zn pose a medium risk in Z2 (10%≤RAC <30%), while there is no risk (RAC <1%) to low risk in other tailings (1% ≤ RAC <10%). Cu poses a medium risk in Z1 and Z5 (10% ≤ RAC <30%) and low risk in other tailings (1% ≤RAC <10%). Arsenic and Cr, Pb, and Ni pose low or no risk in tailings, while Cd, Zn, and Cu pose a medium risk, which may limit the utilization of tailings. So, before the transportation, disposal, and utilization of tailings, a safety assessment should be carried out carefully, and corresponding measures should be taken to reduce their harmfulness.
Cr in the soil poses no risk (RAC <1%), while As, Zn, Pb, Cu, and Ni pose no risk (RAC <1%) and low risk (1% ≤RAC <10%) to the environment. Cd poses a relatively high risk, with a medium risk (10% ≤RAC <30%) at S2 and high risk (30% ≤ RAC < 50%) at S4, whereas a low risk at S1 and S3 (1% ≤ RAC < 10%). The As content in the soil was extremely high, but the RACs were low. Nevertheless, its environmental impact could be significant due to its high arsenic concentration and mobility (Kim et al., 2014).
A gold mine located in the Anqing-Guichi ore-cluster region in the Middle-Lower Yangtze metallogenic belt, southwest Anhui Province, China, produced by open pit mining from 2009 to 2013, with 200,000 tons of untreated waste residue remaining in the mine. Significant accumulation of heavy metals, especially arsenic in the soil has been found. The arsenic pollution in farmland soil near the gold mine was severe enough (322.56 mg kg−1) to be unsuitable for farmland production. The significant correlation between As and Fe/S concentrations was observed by XRF analysis, indicating that Fe-O and sulfide minerals may play an important role in regulating the retention of arsenic in the soil. According to the XRD analysis, tailings, sewage sludge, and soil samples showed similar mineral compositions. And the EDS and XPS results implied that As-containing phases and As speciation observed in soils were consistent with the weathering and oxidation products of mine waste, suggesting that the mineral particles in the mine waste migrated into the surrounding soil possibly through atmospheric transport, rainwater leaching, or surface runoff. Based on risk assessment results, the Igeo values of As ranged from 1.44–6.70 (moderately contaminated to extremely contaminated), further indicating that gold mining activities can lead to serious arsenic pollution in the surrounding areas. Thus, it is of great importance to manage the mining wastes properly both during and after the mining, and further studies regarding the migration-transformation behaviours of heavy metals in various mining sites should be conducted to make relevant treatment measures.
The original contributions presented in the study are included in the article/Supplementary Material, further inquiries can be directed to the corresponding author.
YC: Conceptualization, methodology, validation, investigation, sample collecting, experiment, software, formal analysis, data curation, visualization, writing—original draft. GL: Supervision, funding acquisition, project administration, resources. CZ: Investigation, sample collecting, review and editing. HZ: Conceptualization, sample collecting experiment, validation, visualization, review and editing. YW: Methodology, experiment, software. YL: Review and editing. All authors provided critical feedback and helped shape the research, analysis and manuscript.
The work was supported by the support from the National Key Research and Development Project of China (2020YFC1908601 and 2020YFC1908602).
We thank the editors and reviewers for giving us many constructive comments that significantly improved the paper.
The authors declare that the research was conducted in the absence of any commercial or financial relationships that could be construed as a potential conflict of interest.
The reviewer LM declared a shared affiliation with the author CZ to the handling editor at time of review.
All claims expressed in this article are solely those of the authors and do not necessarily represent those of their affiliated organizations, or those of the publisher, the editors and the reviewers. Any product that may be evaluated in this article, or claim that may be made by its manufacturer, is not guaranteed or endorsed by the publisher.
The Supplementary Material for this article can be found online at: https://www.frontiersin.org/articles/10.3389/feart.2022.1068763/full#supplementary-material.
Acosta, J. A., Faz, A., Martínez-Martínez, S., Zornoza, R., Carmona, D. M., and Kabas, S. (2011). Multivariate statistical and GIS-based approach to evaluate heavy metals behavior in mine sites for future reclamation. J. Geochem. Explor. 109, 8–17. doi:10.1016/j.gexplo.2011.01.004
Adriano, D. C. (2001). Trace elements in terrestrial environments : Biogeochemistry, bioavailability, and risks of metals. New York: Springer.
Akoto, R., and Anning, A. K. (2021). Heavy metal enrichment and potential ecological risks from different solid mine wastes at a mine site in Ghana. Environ. Adv. 3, 100028. doi:10.1016/j.envadv.2020.100028
Al-Othman, Z. A., Ali, R., Al-Othman, A. M., Ali, J., and Habila, A. M. (2016). Assessment of toxic metals in wheat crops grown on selected soils, irrigated by different water sources. Arabian J. Chem. 9, S1555–S1562. doi:10.1016/j.arabjc.2012.04.006
Alloway, B. J. (2013). Sources of heavy metals and metalloids in soils, heavy metals in soils. Dordrecht, Netherlands: Springer, 11–50.
Anju, M., and Banerjee, D. K. (2010). Comparison of two sequential extraction procedures for heavy metal partitioning in mine tailings. Chemosphere 78, 1393–1402. doi:10.1016/j.chemosphere.2009.12.064
Baize, D., Bellanger, L., and Tomassone, R. (2009). Relationships between concentrations of trace metals in wheat grains and soil. Agron. Sustain. Dev. 29, 297–312. doi:10.1051/agro:2008057
Chen, T., Lei, C., Yan, B., Li, L., Xu, D., and Ying, G. (2018). Spatial distribution and environmental implications of heavy metals in typical lead (Pb)-zinc (Zn) mine tailings impoundments in Guangdong Province, South China. Environ. Sci. Pollut. Res. 25, 36702–36711. doi:10.1007/s11356-018-3493-x
Chen, Y., Hong-Bing, J. I., Zhu, X. F., Huang, X. X., and Qiao, M. M. (2012). Fraction distribution and risk assessment of heavy metals in soils around the gold mine of detiangou-qifengcha, beijing city, China. J. Agro-Environ. Sci. 31 (11), 2142–2151.
Cheng, H., Hu, Y., Luo, J., Xu, B., and Zhao, J. (2009). Geochemical processes controlling fate and transport of arsenic in acid mine drainage (AMD) and natural systems. J. Hazard. Mater. 165, 13–26. doi:10.1016/j.jhazmat.2008.10.070
Cheng, H., and Reinhard, M. (2006). Sorption of trichloroethylene in hydrophobic micropores of dealuminated Y zeolites and natural minerals. Environ. Sci. Technol. 40, 7694–7701. doi:10.1021/es060886s
Chubb, L. (2016) Characterization of silica content in gold mine dust with respect to particle size.
Chun-Hui, L. I., Kong, X. K., and Han, Z. T. (2018). Pollution and potential ecological risk assessment of soil heavy metals in the South Mountain area of anhui——taking Chizhou city as an example. J. Anhui Agric. Sci. 46 (34), 105–110. doi:10.13989/j.cnki.0517-6611.2018.34.035
Corkhill, C. L., and Vaughan, D. J. (2009a). Arsenopyrite oxidation – a review. Appl. Geochem. 24, 2342–2361. doi:10.1016/j.apgeochem.2009.09.008
Corkhill, C. L., and Vaughan, D. J. (2009b). Arsenopyrite oxidation – a review. Appl. Geochem. 24, 2342–2361. doi:10.1016/j.apgeochem.2009.09.008
Cui, B., Zhu, M., Jiang, Y., Jiang, Y., Cao, H., and Xiaobing, L. I. (2014). Review on release and migration and bioavailability of heavy metals in tailings. Environ. Sci. Technol. 37, 12–19.
Dove, P. M., and Rimstidt, J. D. (1985). The solubility and stability of scorodite, FeAsO 4 .2H 2 O. Am. Mineral. 70, 838–844.
Duan, L., Yang, X., Wang, F., Deng, J., and Sun, W. (2012). Geochemistry and zircon U-Pb age of ore-bearing porphyry in the Paodaling gold deposit in Guichi, Middle-Lower Yangtze metallogenic belt. Acta Petrol. Sin. 28, 3241–3254.
Dudka, S., and Adriano, D. C. (1997). Environmental impacts of metal ore mining and processing: A review. J. Environ. Qual. 26, 590–602. doi:10.2134/jeq1997.00472425002600030003x
Duruibe, J. O., Ogwuegbu, M., and Egwurugwu, J. (2007). Heavy metal pollution and human biotoxic effects. Int. J. Phys. Sci. 2, 112–118.
Ferreira da Silva, E., Almeida, S. F., Nunes, M. L., Luis, A. T., Borg, F., Hedlund, M., et al. (2009). Heavy metal pollution downstream the abandoned Coval da Mo mine (Portugal) and associated effects on epilithic diatom communities. Sci. Total Environ. 407, 5620–5636. doi:10.1016/j.scitotenv.2009.06.047
Förstner, U., Ahlf, W., Calmano, W., Kersten, M., and Schoer, J. (1990). Assessment of metal mobility in sludges and solid wastes. Berlin, Heidelberg, German: Springer.
Ghezzi, L., Buccianti, A., Giannecchini, R., Guidi, M., and Petrini, R. (2021). Geochemistry of mine Stream sediments and the control on potentially toxic element migration: A case study from the Baccatoio Basin (tuscany, Italy). Mine Water Environ. 40, 722–735. doi:10.1007/s10230-021-00789-9
Gieré, R., Sidenko, N. V., and Lazareva, E. V. (2003). The role of secondary minerals in controlling the migration of arsenic and metals from high-sulfide wastes (Berikul gold mine, Siberia). Appl. Geochem. 18, 1347–1359. doi:10.1016/s0883-2927(03)00055-6
Gupta, D. K. (2011). Arsenic tolerance and detoxification mechanisms in plants. Berlin, Heidelberg, German: Springer, 169–180.
Hao, X., Yi, X., Dang, Z., and Liang, Y. (2022) Heavy metal sources, contamination and risk assessment in legacy Pb/Zn mining tailings area: Field soil and simulated rainfall. Bull. Environ. Contam. Toxicol. 109, 636–642. doi:10.1007/s00128-022-03555-x
Ho, H. H., Swennen, R., and Van Damme, A. (2010). Distribution and contamination status of heavy metals in estuarine sediments near Cua Ong Harbor, Ha Long Bay, Vietnam. Geol. Belg. 13, 37–47.
Hou, S., Zheng, N., Tang, L., Ji, X., and Li, Y. (2019). Effect of soil pH and organic matter content on heavy metals availability in maize (Zea mays L.) rhizospheric soil of non-ferrous metals smelting area. Environ. Monit. Assess. 191, 634. doi:10.1007/s10661-019-7793-5
Huyen, D. T., Tabelin, C. B., Thuan, H. M., Dang, D. H., Truong, P. T., Vongphuthone, B., et al. (2019). Geological and geochemical characterizations of sediments in six borehole cores from the arsenic-contaminated aquifer of the Mekong Delta, Vietnam. Data Brief. 25, 104230. doi:10.1016/j.dib.2019.104230
Islam, A. B. M. R., Maity, J. P., Bundschuh, J., Chen, C.-Y., Bhowmik, B. K., and Tazaki, K. (2013). Arsenic mineral dissolution and possible mobilization in mineral–microbe–groundwater environment. J. Hazard. Mater. 262, 989–996. doi:10.1016/j.jhazmat.2012.07.022
Jia, M. x., and Sun, C. y. (2001). Study on adsorption behavior of metal ions on some silicate minerals. Min. Metall. 10, 25–30.
Jia, S. (2007). Multi-purpose regional geochemical survey covering Yangtze River-huaihe river basin of Anhui Province. Anhui Province: Geological Survey Institute.
Jia, Y., and Demopoulos, G. (2005). Adsorption of arsenate onto ferrihydrite from aqueous solution: influence of media (sulfate vs nitrate), added gypsum, and pH alteration. Environ. Sci. Technol. 39, 9523–9527. doi:10.1021/es051432i
Jiang, L., Sun, H., Peng, T., Ding, W., Liu, B., and Liu, Q. (2021). Comprehensive evaluation of environmental availability, pollution level and leaching heavy metals behavior in non-ferrous metal tailings. J. Environ. Manage. 290, 112639. doi:10.1016/j.jenvman.2021.112639
Kim, E. J., and Batchelor, B. (2009a). Macroscopic and X-ray photoelectron spectroscopic investigation of interactions of arsenic with synthesized pyrite. Environ. Sci. Technol. 43, 2899–2904. doi:10.1021/es803114g
Kim, E. J., and Batchelor, B. (2009b). Macroscopic and X-ray photoelectron spectroscopic investigation of interactions of arsenic with synthesized pyrite. Environ. Sci. Technol. 43, 2899–2904. doi:10.1021/es803114g
Kim, E. J., Yoo, J. C., and Baek, K. (2014). Arsenic speciation and bioaccessibility in arsenic-contaminated soils: Sequential extraction and mineralogical investigation. Environ. Pollut. 186, 29–35. doi:10.1016/j.envpol.2013.11.032
Langmuir, D., Mahoney, J., Macdonald, A., and Rowson, J. (1999). Predicting arsenic concentrations in the porewaters of buried uranium mill tailings. Geochim. Cosmochim. Acta 63, 3379–3394. doi:10.1016/s0016-7037(99)00259-8
Leblanc, M., Achard, B., Othman, D. B., Luck, J. M., Bertrand-Sarfati, J., and Personné, J. C. (1996). Accumulation of arsenic from acidic mine waters by ferruginous bacterial accretions (stromatolites). Appl. Geochem. 11, 541–554. doi:10.1016/0883-2927(96)00010-8
Lee, C. G., Chon, H.-T., and Jung, M. C. (2001). Heavy metal contamination in the vicinity of the Daduk Au–Ag–Pb–Zn mine in Korea. Appl. Geochem. 16, 1377–1386. doi:10.1016/s0883-2927(01)00038-5
Lee, P.-K., Kang, M.-J., Jeong, Y.-J., Kwon, Y. K., and Yu, S. (2020). Lead isotopes combined with geochemical and mineralogical analyses for source identification of arsenic in agricultural soils surrounding a zinc smelter. J. Hazard. Mater. 382, 121044. doi:10.1016/j.jhazmat.2019.121044
Lei, K., Giubilato, E., Critto, A., Pan, H., and Lin, C. (2016). Contamination and human health risk of lead in soils around lead/zinc smelting areas in China. Environ. Sci. Pollut. Res. 23, 13128–13136. doi:10.1007/s11356-016-6473-z
Lenthe, W. C., Stinville, J. C., Echlin, M. P., Chen, Z., Daly, S., and Pollock, T. M. (2018). Advanced detector signal acquisition and electron beam scanning for high resolution SEM imaging. Ultramicroscopy 195, 93–100. doi:10.1016/j.ultramic.2018.08.025
Li, H. (2007). The Progression and Significance of the study on environmental mineral material renovating the heavy metal ion pollution in the water body. J. Graduates Sun Yat-Sen Univ. Nat. Sci. Med. 28, 35–42.
Li, J. S., Beiyuan, J., Tsang, D. C. W., Wang, L., Poon, C. S., Li, X. D., et al. (2017). Arsenic-containing soil from geogenic source in Hong Kong: Leaching characteristics and stabilization/solidification. Chemosphere 182, 31–39. doi:10.1016/j.chemosphere.2017.05.019
Li, Z., Ma, Z., van der Kuijp, T. J., Yuan, Z., and Huang, L. (2014). A review of soil heavy metal pollution from mines in China: pollution and health risk assessment. Sci. Total Environ. 468-469, 843–853. doi:10.1016/j.scitotenv.2013.08.090
Lian-Ke, Z., Hai-Peng, L. I., Xue-Min, H., Yu-Mei, L. I., Kun-Ling, J., Peng, S., et al. (2016). Soil heavy metal spatial distribution and source analysis around an aluminum plant in Baotou. Environ. Sci. 37, 1139–1146.
Liao, G. (2005). Research on the pollution assessment and the transport characteristics of heavy metals in a typical nonferrous metal mine. Changsha, Hunan Province, China: Central South University.
Liu, G., Tao, L., Liu, X., Hou, J., Wang, A., and Li, R. (2013). Heavy metal speciation and pollution of agricultural soils along Jishui River in non-ferrous metal mine area in Jiangxi Province, China. J. Geochem. Explor. 132, 156–163. doi:10.1016/j.gexplo.2013.06.017
Liu, H., Liqing, L. I., Yin, C., and Shan, B. (2008). Fraction distribution and risk assessment of heavy metals in sediments of Moshui Lake. J. Environ. Sci. 20, 390–397. doi:10.1016/s1001-0742(08)62069-0
Liu, Y., Liu, G., Yousaf, B., Zhou, C., and Shen, X. (2021). Identification of the featured-element in fine road dust of cities with coal contamination by geochemical investigation and isotopic monitoring. Environ. Int. 152, 106499. doi:10.1016/j.envint.2021.106499
Liu, H., Qu, M., Chen, J., Guang, X., Zhang, J., Liu, M., et al. (2022). Heavy metal accumulation in the surrounding areas affected by mining in China: Spatial distribution patterns, risk assessment, and influencing factors. Sci. Total Environ. 825, 154004. doi:10.1016/j.scitotenv.2022.154004
Liu, J. H., Wang, W. H., and Peng, A. (2000). Bioavailability of mercury in soil. J. Agro-Environ. Sci. 5, 216–220. doi:10.3321/j.issn:1672-2043.2000.04.007
Loredo-Portales, R., Bustamante-Arce, J., González-Villa, H. N., Moreno-Rodríguez, V., Del Rio-Salas, R., Molina-Freaner, F., et al. (2020). Mobility and accessibility of Zn, Pb, and as in abandoned mine tailings of northwestern Mexico. Environ. Sci. Pollut. Res. 27, 26605–26620. doi:10.1007/s11356-020-09051-1
Ma, H. H., Peng, M., Liu, F., Guo, F., Tang, S. Q., Liu, X. J., et al. (2020). Bioavailability, translocation, and accumulation characteristic of heavy metals in a soil-crop system from a typical carbonate rock area in guangxi, China. Huan Jing Ke Xue 41, 449–459. doi:10.13227/j.hjkx.201905040
Majzlan, J., Drahota, P., and Filippi, M. (2014). Parageneses and crystal chemistry of arsenic minerals. Rev. Mineral. Geochem. 79, 17–184. doi:10.2138/rmg.2014.79.2
Masscheleyn, P. H., Delaune, R. D., and Patrick, W. H. (1991). Effect of redox potential and pH on arsenic speciation and solubility in a contaminated soil. Environ. Sci. Technol. 25, 1414–1419. doi:10.1021/es00020a008
Matong, J. M., Nyaba, L., and Nomngongo, P. N. (2016). Fractionation of trace elements in agricultural soils using ultrasound assisted sequential extraction prior to inductively coupled plasma mass spectrometric determination. Chemosphere 154, 249–257. doi:10.1016/j.chemosphere.2016.03.123
Ministry of Ecology and Environment,State Administration for Market Regulation (2018a). Soil environmental quality Risk control standard for soil contamination of agricultural land (GB15618-2018). Beijing, China: Ministry of Ecology and Environment, State Administration for Market Regulation.
Ministry of Ecology and EnvironmentState Administration for Market Regulation (2018b). Soil environmental quality risk control standard for soil contamination of development land (GB15618-2018). Beijing, China: Ministry of Ecology and Environment, State Administration for Market Regulation.
Navarro, M. C., Pérez-Sirvent, C., Martínez-Sánchez, M., Vidal, J., Tovar, P. J., and Bech, J. (2008). Abandoned mine sites as a source of contamination by heavy metals: A case study in a semi-arid zone. J. Geochem. Explor. 96, 183–193. doi:10.1016/j.gexplo.2007.04.011
Nordstrom, D. K., and Parks, G. A. (1987). Solubility and stability of scorodite, FEASO4.2H2O - discussion. Am. Mineral. 72, 849–851.
Punia, A., and Siddaiah, N. S. (2019). Mobility and behaviour of metals in copper mine tailings and soil at Khetri, India. Mine Water Environ. 38, 385–390. doi:10.1007/s10230-018-00582-1
Qin, W., Han, D., Song, X., and Liu, S. (2021). Sources and migration of heavy metals in a karst water system under the threats of an abandoned Pb–Zn mine, Southwest China. Environ. Pollut. 277, 116774. doi:10.1016/j.envpol.2021.116774
QingBin, S., ChunQin, Y., JinFeng, D., and Li, Z. (2013). Investigation on heavy metal contamination of farmland soil and wheat(Triticum aestivum) nearby mining areas. J. Henan Agric. Sci. 4, 80–84. doi:10.15933/j.cnki.1004-3268.2013.04.004
Quadros, P., Zhalnina, K., Davis-Richardson, A. G., Drew, J. C., Menezes, F. B., Camargo, F., et al. (2016) Coal mining practices reduce the microbial biomass, richness and diversity of soil. Appl. Soil Ecol. 98, 195–203. doi:10.1016/j.apsoil.2015.10.016
Saha, A., Gupta, B. S., Patidar, S., and Villegas, N. M. (2022). Spatial distribution based on optimal interpolation techniques and assessment of contamination risk for toxic metals in the surface soil. J. S. Am. Earth Sci. 115, 103763. doi:10.1016/j.jsames.2022.103763
Santibañez, C., de la Fuente, L. M., Bustamante, E., Silva, S., León-Lobos, P., and Ginocchio, R. (2012). Potential use of organic- and hard-rock mine wastes on aided phytostabilization of Large-scale mine tailings under semiarid mediterranean climatic conditions: Short-term field study. Appl. Environ. Soil Sci. 2012, 1–15. doi:10.1155/2012/895817
Savage, K. S., Bird, D. K., and O'Day, P. A. (2005). Arsenic speciation in synthetic jarosite. Chem. Geol. 215, 473–498. doi:10.1016/j.chemgeo.2004.06.046
Senoro, D. B., Bonifacio, P. B., Mascareñas, D. R., Tabelin, C. B., Ney, F. P., Lamac, M. R. L., et al. (2021). Spatial distribution of agricultural yields with elevated metal concentration of the island exposed to acid mine drainage. J. Degrade. Min. Land Manage. 8, 2551–2558. doi:10.15243/jdmlm.2021.082.2551
Sharma, V. K., and Sohn, M. (2009). Aquatic arsenic: toxicity, speciation, transformations, and remediation. Environ. Int. 35, 743–759. doi:10.1016/j.envint.2009.01.005
Sherriff, B. L., Salzsauler, K. A., Simpson, S., Sidenko, N. V., and VanGulck, J. (2006). Arsenic mobility from arsenopyrite-rich gold mine waste in Snow Lake, Manitoba, Canada. Chin. J. Geochem. 25, 29–30. doi:10.1007/bf02839790
Smedley, P. L., and Kinniburgh, D. G. (2002). A review of the source, behaviour and distribution of arsenic in natural waters. Appl. Geochem. 17, 517–568. doi:10.1016/s0883-2927(02)00018-5
Sun, Y., Wu, Q. T., Lee, C. C., Li, B., and Long, X. (2014). Cadmium sorption characteristics of soil amendments and its relationship with the cadmium uptake by hyperaccumulator and normal plants in amended soils. Int. J. Phytoremed. 16, 496–508. doi:10.1080/15226514.2013.798617
Tabelin, C. B., Corpuz, R. D., Igarashi, T., Villacorte-Tabelin, M., Hiroyoshi, N., Yoo, K., et al. (2020). Acid mine drainage formation and arsenic mobility under strongly acidic conditions: Importance of soluble phases, iron oxyhydroxides/oxides and nature of oxidation layer on pyrite. J. Hazard. Mater. 399, 122844. doi:10.1016/j.jhazmat.2020.122844
Tabelin, C. B., Hashimoto, A., Igarashi, T., and Yoneda, T. (2014). Leaching of boron, arsenic and selenium from sedimentary rocks: II. pH dependence, speciation and mechanisms of release. Sci. Total Environ. 473-474, 244–253. doi:10.1016/j.scitotenv.2013.12.029
Tabelin, C. B., and Igarashi, T. (2009). Mechanisms of arsenic and lead release from hydrothermally altered rock. J. Hazard. Mater. 169, 980–990. doi:10.1016/j.jhazmat.2009.04.049
Tack, F. M. G., and Verloo, M. G. (1995). Chemical speciation and fractionation in soil and sediment heavy metal analysis: A review. Int. J. Environ. Anal. Chem. 59, 225–238. doi:10.1080/03067319508041330
Tahervand, S., and Jalali, M. (2016). Sorption, desorption, and speciation of Cd, Ni, and Fe by four calcareous soils as affected by pH. Environ. Monit. Assess. 188, 322–412. doi:10.1007/s10661-016-5313-4
Tessier, A. P., Campbell, P., and Bisson, M. X. (1979). Sequential extraction procedure for the speciation of particulate trace metals. Anal. Chem. 51, 844–851. doi:10.1021/ac50043a017
Wang, S., and Mulligan, C. N. (2006). Natural attenuation processes for remediation of arsenic contaminated soils and groundwater. J. Hazard. Mater. 138, 459–470. doi:10.1016/j.jhazmat.2006.09.048
Wei, C. Y., Sun, X., Wang, C., and Wang, W. Y. (2006). Factors influencing arsenic accumulation by pteris vittata: a comparative field study at two sites. Environ. Pollut. 141, 488–493. doi:10.1016/j.envpol.2005.08.060
Welch, A. H., and Stollenwerk, K. G. (2003). Geochemical processes controlling transport of arsenic in groundwater: A review of adsorption. US 10.1007/b101867. Boston, MA, United States: Springer, 67–100.
Wu, Y., Zhou, X.-y., Lei, M., Yang, J., Ma, J., Qiao, P.-w., et al. (2017). Migration and transformation of arsenic: Contamination control and remediation in realgar mining areas. Appl. Geochem. 77, 44–51. doi:10.1016/j.apgeochem.2016.05.012
Xiao, H., Zang, S., Guan, Y., Liu, S., Gao, Y., Sun, Q., et al. (2014). Assessment of potential risks associated with heavy metal contamination in sediment in Aobaopao Lake, China, determined from sediment cores. Ecotoxicology 23, 527–537. doi:10.1007/s10646-014-1220-z
Xiao, R., Wang, S., Li, R., Wang, J. J., and Zhang, Z. (2017). Soil heavy metal contamination and health risks associated with artisanal gold mining in Tongguan, Shaanxi, China. Ecotoxicol. Environ. Saf. 141, 17–24. doi:10.1016/j.ecoenv.2017.03.002
Xu, Y. N., Ke, H. L., and Zhao, A. I. (2007). Assessment of heavy metals contamination of farmland soils in some gold mining area of Xiao Qinling. Chin. J. Soil Sci.
Yang, Y. (2021). Geochemical characteristics of soil Cd and zoning of land safety use in Hezhou tin mining area of Guangxi. Beijing, China: China University of Geosciences.
Zhang, M., He, P., Qiao, G., Huang, J., Yuan, X., and Li, Q. (2019). Heavy metal contamination assessment of surface sediments of the Subei Shoal, China: Spatial distribution, source apportionment and ecological risk. Chemosphere 223, 211–222. doi:10.1016/j.chemosphere.2019.02.058
Zhang, X., Zhong, T., Liu, L., and Ouyang, X. (2015). Impact of soil heavy metal pollution on food safety in China. Plos One 10, e0135182. doi:10.1371/journal.pone.0135182
Zhao, F. J., Mcgrath, S. P., and Meharg, A. A. (2010). Arsenic as a food chain contaminant: Mechanisms of plant uptake and metabolism and mitigation strategies. Annu. Rev. Plant Biol. 61, 535–559. doi:10.1146/annurev-arplant-042809-112152
Zhong, X., Chen, Z., Li, Y., Ding, K., Liu, W., Liu, Y., et al. (2020). Factors influencing heavy metal availability and risk assessment of soils at typical metal mines in Eastern China. J. Hazard. Mater. 400, 123289. doi:10.1016/j.jhazmat.2020.123289
Zhou, y. (2009). Research on geochemical characters and environmental geochemical effects of representative tailings impoundments in the region of Tongling. Anhui Province,China: Hefei University of Technology.
Zhu, D. (2016). Containing calcite matters repair cadmium pollution soiland the mechanism of first exploration. Shenyang, Liaoning Province, China: Shenyang Agricultural University.
Zhu, Y. G., Sun, G. X., Lei, M., Teng, M., Liu, Y. X., Chen, N. C., et al. (2008). High percentage inorganic arsenic content of mining impacted and nonimpacted Chinese rice. Environ. Sci. Technol. 42, 5008–5013. doi:10.1021/es8001103
Keywords: mine tailing soil heavy metals arsenic risk assessment speciation transformation, mine tailing, soil, heavy metals, arsenic, risk assessment, speciation transformation
Citation: Chen Y, Liu G, Zhou C, Zhou H, Wei Y and Liu Y (2023) The influence of gold mining wastes on the migration-transformation behavior and health risks of arsenic in the surrounding soil of mined-area. Front. Earth Sci. 10:1068763. doi: 10.3389/feart.2022.1068763
Received: 13 October 2022; Accepted: 11 November 2022;
Published: 06 January 2023.
Edited by:
Dun Wu, Anhui Jianzhu University, ChinaReviewed by:
Wang Xingming, Anhui University of Science and Technology, ChinaCopyright © 2023 Chen, Liu, Zhou, Zhou, Wei and Liu. This is an open-access article distributed under the terms of the Creative Commons Attribution License (CC BY). The use, distribution or reproduction in other forums is permitted, provided the original author(s) and the copyright owner(s) are credited and that the original publication in this journal is cited, in accordance with accepted academic practice. No use, distribution or reproduction is permitted which does not comply with these terms.
*Correspondence: Yu Chen, Y3kwNDE4QG1haWwudXN0Yy5lZHUuY24=; Guijian Liu, bGdqQHVzdGMuZWR1LmNu
Disclaimer: All claims expressed in this article are solely those of the authors and do not necessarily represent those of their affiliated organizations, or those of the publisher, the editors and the reviewers. Any product that may be evaluated in this article or claim that may be made by its manufacturer is not guaranteed or endorsed by the publisher.
Research integrity at Frontiers
Learn more about the work of our research integrity team to safeguard the quality of each article we publish.