- 1ELKH-PE Air Chemistry Research Group, Veszprém, Hungary
- 2ELKH-PE Environmental Mineralogy Research Group, Veszprém, Hungary
- 3Research Institute of Biomolecular and Chemical Engineering, University of Pannonia, Veszprém, Hungary
- 4Institute of Mineralogy and Geology, University of Miskolc, Miskolc, Hungary
- 5Department of Mineralogy, Eötvös Loránd University, Budapest, Hungary
Since carbonate formation is an important process linking inorganic and biological components of freshwater ecosystems, we characterized the formation of modern carbonate sediments in a large, shallow, calcareous lake (Lake Balaton in Hungary). We measured the amount of allochtonous mineral particles delivered to the lake by tributaries and through the atmosphere over a 2-year period, and estimated the mass of carbonate minerals that precipitated from lakewater. Chemical and structural features of mineral particles from various sources were also studied. Both the mineralogical character and the amount of particles delivered by streams and through the atmosphere were similar, and formed a minor fraction of the annual sediment increment (∼5%–6% by mass). Since the watercourses feeding the lake had high concentrations of Ca2+, Mg2+, and HCO3− (with a Mg/Ca mol ratio ranging from 1 to 4), Mg-bearing calcite (with 2–17 mol% MgCO3) was found to continually precipitate in the lake. According to X-ray powder diffraction measurements, the Mg content of calcite increased from West to East, in parallel with changes in water chemistry. Dolomite was detected as a minor phase, and in the eastern part of the lake it typically produced a split 104 peak in X-ray diffractograms, suggesting two distinct sources: stoichiometric dolomite was allochtonous, whereas a Ca-rich protodolomite fraction formed in the lake. Mg-bearing calcite precipitating in the lake was found by far the largest contributor to sediment formation, with an estimated annual accumulation of about 0.75–0.9 mm consolidated sediment; thus, ∼89% of the currently forming sediment consists of autochtonous carbonate. In addition to providing new estimates for the rates of accumulation of distinct sediment fractions, our results also provide a baseline for further studies on the retention and release of nutrients by sediment minerals.
1 Introduction
Carbonate minerals in lake sediments play important roles in nutrient cycles (Talbot 1990) and can be used as archives of past climate changes (Mutti and Hallock 2003). The formation and preservation of carbonates in lakes are influenced by both biological and inorganic processes (Dittrich and Obst 2004). While carbonate formation is an indirect consequence of photosynthesis (Gierlowski-Kordesch 2010), it also depends on water chemistry, which is primarily controlled by the catchment geology and affected by seasonality, water level and lakewater circulation patterns, all of which are governed by meteorological conditions (Kelts and Hsü 1978; Tucker and Wright 1990; Fedo et al., 1995; Khan et al., 2022). Water chemistry is also responsible for either the long-term preservation or potential dissolution of carbonate minerals in lake sediments (Reimers et al., 1996). In this study, we evaluate the processes that lead to the accumulation of carbonate minerals in the sediments of Lake Balaton, Hungary. In addition to satisfying scientific interest, a study of the interplay of the factors that control carbonate deposition in shallow lakes and, in particular, Lake Balaton, has important practical motivations: both sediment accumulation and algal blooms (triggered by nutrients mobilized from sediments) have consequences for the commercial and recreational uses of the lake.
Some unique features of Lake Balaton make it a special case of a freshwater “carbonate factory” (Figure 1A). It is the largest lake in Central Europe by surface area (596 km2) but is extremely shallow (on average 3.3 m), typically resulting in the lack of stratification and the frequent stirring up of its sediments by winds. The Tihany Peninsula divides the lake into the Western (360 km2) and Eastern Basins (236 km2). Water delivered by precipitation and inflows is balanced by evaporation and outflow through a controlled outlet (Figure 1B); except for extremely dry years, such as from 2001 to 2003, the natural water balance of the lake is slightly positive (Honti and Istvánovics 2011). The most significant inflow, the Zala River delivers about half to two-third of the annual inflow and discharges into the lake at its western end. The only (artificial and temporary) outflow, the Sió Canal, drains the lake near its eastern end. As a result, the lake is characterized by W–E gradients in both chemistry and trophic state (from typically eutrophic at its western, and meso- or oligotrophic at its eastern end (Palmer et al., 2017)). The catchment area is dominated by Miocene to Quaternary sand, clay and loess deposits, and Mesozoic, predominantly Triassic limestone and dolostone north of the lake (Budai et al., 1999) (Figure 1A). As a result, the lakewater is characterized by high HCO3−, Ca2+ and Mg2+ concentrations, with Mg/Ca molar ratios ranging from about 1–4, and a pH of around 8.5 (Tompa et al., 2014). Evaporation concentrates dissolved ions from West to East, except for Ca2+ and HCO3−, which are reduced by the precipitation of calcite from water (Müller et al., 1978; Tullner and Cserny 2003; Nyirő-Kósa et al., 2018).
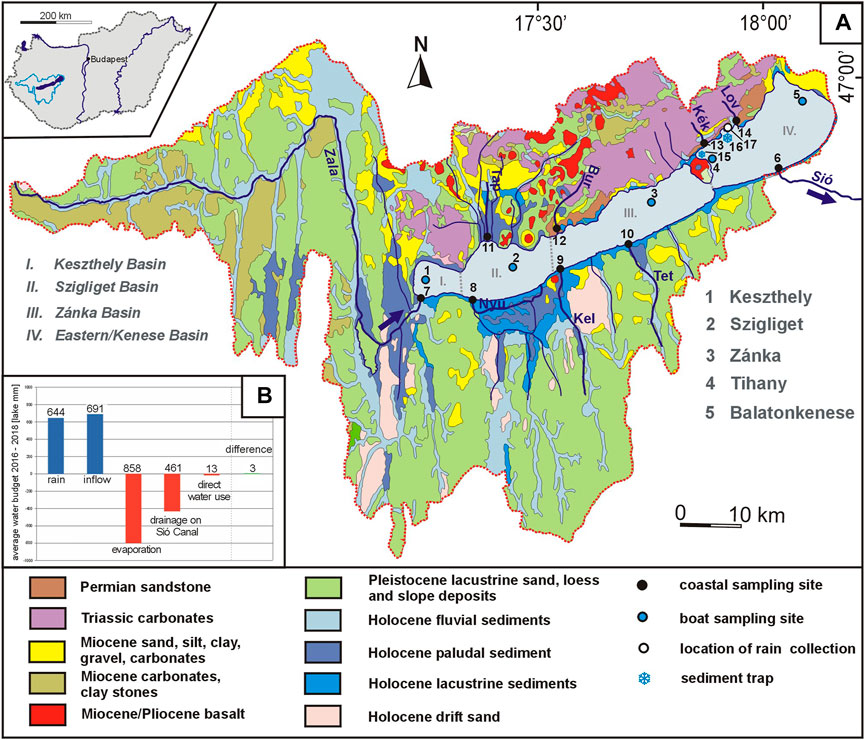
FIGURE 1. (A) Simplified geological map of the catchment area of Lake Balaton (after Budai et al., 1999) with sampling sites marked. The location of lake samples is shown by blue dots (1 Keszthely, 2 Szigliget, 3 Zánka, 4 Tihany, 5 Balatonkenese). Black dots indicate sampling sites at the only outflow (6 Sió Canal) and major inflows (7 Zala River, 8 Nyugati-övcsatorna/Nyu, 9 Keleti-bozót árok/Kel, 10 Tetves-patak/Tet, 11 Tapolca-patak/Tap, 12 Burnót-patak/Bur, 13 Kéki Séd/Kék, 14 Lovasi Séd/Los). The two snowflakes show sites (15. Diós Bay, 16. Csopak) where sediment traps were used under ice. White dot marks the location of rainfall collection (17 Csopak). The flow directions of the main tributary (Zala River) and the only artificial outflow (Sió Canal) are also indicated. (B) Average water budget of Lake Balaton (2016–2018; KDT, 2017; KDT, 2018; KDT, 2019).
Calcareous sediment of variable thickness (0–9 m) accumulated during the 12–15-ky history of the lake (Cserny and Corrada 1990; Visnovitz et al., 2015a, Visnovitz et al., 2015b). Strong winds and the resulting turbulence regularly stir up the upper few cm of the unconsolidated mud. Redeposition of the particles is controlled by the hydrodynamical characteristics of the sub-basins (Torma and Wu 2019; Lükő et al., 2020). As a result, the sediment reaches 6 m average thickness in the Keszthely and Szigliget Basins, whereas it is practically absent in the strait of Tihany. Due to the prevailing northerly wind direction, sand-sized particles are deposited along the south shore, whereas silt-sized particles (particle size 2–10 μm) dominate elsewhere in the sediment (Máté 1987; Cserny 2006). Coarse grains are mainly of allochtonous origin and therefore contain more quartz, feldspar and mica than fine mud, which is composed mainly of carbonate minerals and clays (Tompa et al., 2014). Since the variable but relatively high Mg concentration of the water results in the precipitation of Mg-bearing calcite, the Mg content of which reflects the actual water chemistry, autochtonous carbonates can be used as proxies for changes between dry and humid climatic conditions (Müller et al., 1978; Tullner and Cserny 2003; Nyirő-Kósa et al., 2018).
The potential silting of shallow Lake Balaton raised concern already about a century ago (Lóczy 1913; Cholnoky 1918), and still poses problems for recreational uses. Several attempts were made to determine the rate of sediment accumulation and to identify the various sources of minerals in the sediment (Supplementary Table S1). Assuming continuous sediment formation through the history of the lake and considering its age of 12–15 thousand years (depending on the particular sub-basin), an average sediment thickness of 6 m suggests a deposition rate of 0.4–0.5 mm y−1 of consolidated sediment (Cserny 2006). However, current rates may differ from the 15-ky average. Some recent estimates vary wildly (Supplementary Table S1; Zlinszky et al., 2010; Korponai et al., 2011; Buczkó et al., 2019); thus, a revision of current sediment deposition rates is warranted.
The strengths of individual sources (both allochtonous and autochtonous) contributing to the mineral budget of the sediment are even less well known. Through the larger part of the 20th century either wind-blown dust (Treitz 1911; Lóczy 1913) or biogenic organic matter (Szesztay 1961) were thought to be the main constituents of bottom sediments. The significance of carbonates precipitating from lakewater was only recognized in the 1960s, and their annual contribution to the sediment was estimated either by the direct analysis of the sediment (Entz et al., 1963) or by using thermodynamic models (Gelencsér et al., 1982). Nevertheless, the estimates for individual sources of sediment minerals vary significantly, particularly for the amount of precipitating carbonates (Supplementary Table S1). In addition, the significance of individual sources may change with time as a result of changing land use (Jordán et al., 2005) or the large amount of biogenic carbonates produced by invasive mussels (Báldi et al., 2019). A reevaluation of sediment source strengths is thus useful for understanding current geochemical processes in the lake.
Sediment minerals play key roles in the cycles of nutrients in lakes. In particular, carbonate and iron oxide minerals are known to be major reservoirs of phosphorus (P) in Lake Balaton (Gelencsér et al., 1982; Tátrai et al., 2000), binding P either by adsorption or coprecipitation. Changes in lakewater chemistry, pH or redox conditions can lead to the remobilization of P (Elser et al., 2007). Eutrophication of Lake Balaton was a major problem in the 1980s and 1990s. Following some major changes in lake management, summer algal blooms disappeared for more than 20 years (Istvánovics et al., 2004). However, in August 2019 an unexpected bloom of a cyanobacterium and a green alga species occurred (Istvánovics et al., 2022). For the observed amount of primary production an estimated 17.3 t of P was required within a 3-weeks period; in lack of any substantial external input, the sediment was identified as the likely source (Istvánovics and Honti 2021). However, it remains unknown which mineral fraction and through what particular process supplied the P for the bloom.
The aim of the present study was to characterize the formation of modern sediments in Lake Balaton. We measured the amount of allochtonous mineral particles delivered to the lake by tributaries and through the atmosphere, and estimated the mass of carbonate minerals that precipitated from lakewater. In addition to establishing a “mineral budget” for Lake Balaton sediments, we also studied chemical and structural features of mineral particles from various sources, in order to identify characteristics that could provide clues about the origins and potential transformations of minerals in the lake sediment. The results are useful for estimating the rate of sediment accumulation and for providing a baseline for further studies on the retention and release of nutrients by sediment minerals in shallow, calcareous lakes.
2 Materials and methods
2.1 Sampling of water and suspended solid matter
Water and suspended solid particles were analyzed from Lake Balaton, its tributaries, and atmospheric precipitation. We collected samples over a period of two and a half years, from May 2016 to October 2018. In total, 55 samples from Lake Balaton, 11 from Sió Canal, 107 from tributaries, and 46 rain samples were collected (Supplementary Table S2). Physical parameters (water temperature, pH, electrical conductivity and oxygen saturation) were measured at the time of sampling. All water samples were returned to the laboratory within a few hours of being taken. For water chemistry, subsamples were prepared by membrane filtering (using Millipore and Prat Dumas mixed cellulose ester membranes with 0.45 µm nominal pore size), then the water samples were stored frozen. The amount of total suspended matter (TSM) in lake and stream samples was determined by letting the solid content of 40-l water samples settle for 7 days, and after decantation the remaining sludge (with a volume of about 4–5 L) was then membrane filtered. Then the filters were dried at 40°C, weighed, and stored in a desiccator. In the case of rain samples, the mass of TSM was determined by filtering the total amount of collected water.
Four tributaries each on the north and south shores of the lake (Tapolca-patak, Burnót-patak, Kéki Séd, Lovasi Séd and Tetves-patak, Keleti-bozót árok, Nyugati-övcsatorna, Zala River, respectively) were sampled at least 12 times, with the main inflow, the Zala River, sampled more often (Figure 1A; Supplementary Table S2). Water samples were taken from the shores of streams (except for the Zala River, where we used a boat), close to their mouths. From Lake Balaton we collected total column samples from a boat at 15 dates, at five locations (Keszthely, Szigliget, Zánka, Tihany, Balatonkenese) along the long axis of the lake, and also at the locks of the Sió Canal. Meteorological data (air temperature, wind speed and precipitation) were obtained from the National Meteorological Service (NMS; Supplementary Table S3) for the day of sampling and the preceding 2 days, in order to identify calm periods when resuspension of lake sediments by wind-driven turbulence was assumed to be negligible. During our study period the lake froze over only once, over a 4-week period in January and February 2017, providing an opportunity to collect freshly precipitated material, presumably devoid of resuspended sediment. On this occasion, we placed home-designed sediment traps under the ice (with 40–100 cm water column above the collection vial) for 26 and 24 days in Csopak and Diós Bay, respectively, with both samples recovered on the 3rd of February (Figure 1A; Supplementary Table S2).
Rainwater samples were collected in Csopak, a village on the northern shore, about 800 m from the lake, over a year from April 2016 to March 2017 (Figure 2A). In this period, 74 precipitation events occurred at the sampling location and 46 samples were collected (Figure 2B). When less than 3 mm of precipitation fell, it was impossible to obtain an appreciable amount of solid material and the sample was discarded. Samples were collected in a clean polycarbonate plastic container on a raised platform. The collector was deployed just before the onset of rainfall and withdrawn immediately at the end of the event.
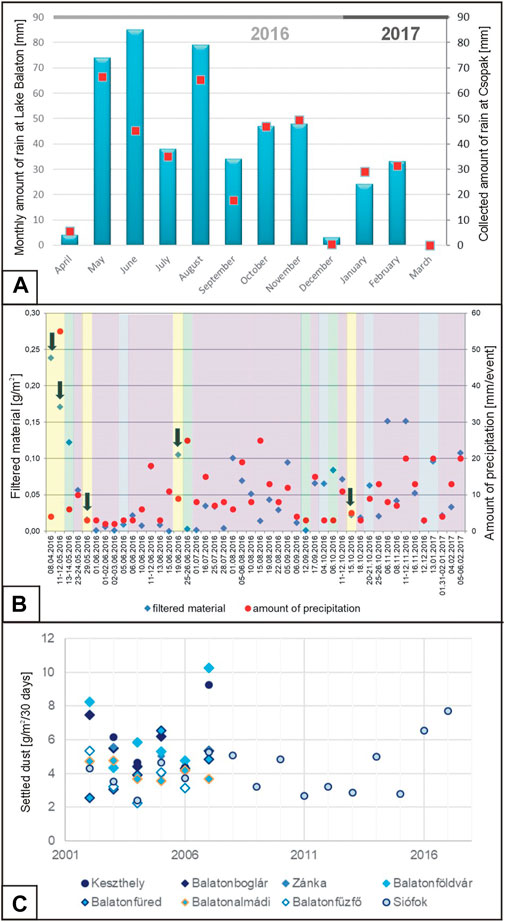
FIGURE 2. Atmospheric input of material into Lake Balaton. (A) Monthly amount of rain in the area of Lake Balaton from 08 April 2016 to 31 March 2017, based on daily meteorological reports by the Hungarian Meteorological Survey (HMS) (blue columns), and monthly amount of rainwater collected in Csopak over the same period (red squares). (B) Amount of solid material filtered from rainwater (g/m2), and daily amount of rain (mm/event), based on daily meteorological reports of HMS. The background colors indicate the origins of air masses: yellow for South, green for East, blue for North, and pink for West. Black arrows indicate Saharan dust events (08 April 2016, 11–12 May 2016, 29 May 2016, 19 June 2016, 15 October 2016). (C) Average amount of settled dust (g/m2/30 days) based on data published by the Hungarian Air Quality Network. (An outlier data point of settled dust in Keszthely (18.41 g/m2/30 days in 2002) has been deleted and was not considered in our calculations, since it was probably influenced by a local source.).
2.2 Analysis of water and solid matter
Quantitative concentrations of dissolved species were analyzed using inductively coupled optical emission spectrometry (ICP–OES) (for Na+, K+, Mg2+, and Ca2+), ion chromatography (IC) (for Cl−, NO3−, SO42-), and by titration (for HCO3−). In order to determine ion concentrations, samples at room temperature were re-filtered through a 0.2 μm syringe filter and diluted 10-fold with MilliQ water. A DIONEX ICS instrument with Dionex IonPac AS17-C type hydroxide selective anion exchange column was used for ion chromatography (Tcolumn=28°C). The eluent was a 0.8 mM KOH solution, prepared from analytic grade stock solution. For quantification, calibration series (10–4 M, 5 × 10−4 M, 10–3 M, 3 × 10−3 M and 5 × 10−3 M) were prepared from analytic grade stock solution and chromatograms for each standard were recorded. From the retention times of chromatographic peaks, qualitative information was obtained based on the area under the peak, using calibration lines.
The mineralogical composition of filtered solid material was determined from X-ray powder diffraction (XRD) patterns obtained using a Siemens D5000 instrument equipped with a graphite monochromator, using CuKα radiation (λ = 1.5406 Å) at 40 kV voltage and at 40 mA tube current. Diffractograms were recorded first in the 2–65° 2θ range with a step size of 0.05° and a counting time of 2 s, then in the 26.1°–31.4° 2ϑ range with 0.01° 2ϑ step size and 12 s/step counting time in order to achieve higher resolution. The morphologies, compositions and structures of mineral particles from various sources were studied using both scanning (SEM) and transmission electron microscopy (TEM). We used a field-emission gun ApreoLoVac SEM instrument (ThermoFisher), equipped with an Octane Elect Plus (AMETEK) spectrometer for energy-dispersive X-ray spectrometry (EDS). Accelerating voltages of 2 and 10 kV were used for obtaining secondary electron images and EDS spectra, respectively. For TEM analyses, particle suspensions were placed on carbon-covered lacey TEM copper mesh grids. We used a Talos F200X G2 instrument (ThermoFisher), operated at 200 kV accelerating voltage, and equipped with a field-emission gun and a four-detector Super-X energy-dispersive X-ray spectrometer. Selected-area electron diffraction (SAED) patterns, bright-field (BF) and high-resolution (HRTEM) images were obtained in conventional TEM mode, whereas high-angle annular dark-field (HAADF) images and EDS elemental maps were collected in scanning transmission (STEM) mode.
2.3 Modeling of air mass transport and carbonate stability
For atmospheric trajectory and dispersion calculations, the HYSPLIT (HYbrid Single-Particle Lagrangian Integrated Trajectory) software was used (Draxler and Rolph 2012). The model allows an air parcel to be tracked backwards in time from a given location, and to calculate changes in its physical properties and provide information about its transport routes (Stein et al., 2015). The trajectories were calculated for 48 or 72-h total run time at three altitude ranges: 500–1,500, 1,500–3,000, and 3,000–6,000 m. The model was run backwards in time for the days when precipitation occurred.
In order to assess the thermodynamic stability of various carbonate minerals under water chemical conditions typical for Lake Balaton, we calculated the saturation indices (SIs) of several CaCO3 and (Mg,Ca)CO3 phases. The SI was defined as SI = log (IAP/Ksp), where IAP was the ion activity product and Ksp the solubility product of the given phase. We calculated the SIs using the PHREEQC software (Parkhurst and Appelo 2013), and used the -logKsp values of 17.09 for ordered dolomite, 16.54 for disordered dolomite, 8.38 for Mg-bearing calcite, and 8.48 for calcite (Plummer and Busenberg, 1982; Mackenzie et al., 1983; Bénézeth et al., 2018).
3 Results
3.1 Atmospheric wet and dry deposition
Based on daily meteorological reports of the Hungarian Meteorological Survey (HMS), from April 2016 to March 2017 the distribution of precipitation was fairly uniform geographically over the surface of Lake Balaton (596 and 543 mm in the Eastern and Western Basins, respectively) but highly variable over time. From 46 rain events in Csopak (with precipitation >3 mm in each case) we measured a total of 393 mm, amounting to 66% of the annual rainfall registered by the HMS for the Eastern Basin of the lake (Figure 2A). With the exception of June, the monthly amount of collected rain followed the pattern registered by the HMS. The number of events was largest in June (11), followed by October (7) and August (6), with no rain observed in March. March and April were extremely dry, followed by a relatively wet summer (Figure 2A). Seasonal differences in rainfall are shown in Supplementary Table S4.
The atmospheric pathways of moisture transport leading to the observed precipitation events were evaluated using a Lagrangian trajectory model. On the basis of 48-h backward trajectories (Figure 3), four source sectors were classified as (1) Northern Europe (contributing 11% of rain events), (2) Eastern Europe (9%), (3) Mediterranean (11%) and (4) Atlantic (69%). The origins of air masses carrying precipitation showed seasonal changes (Figure 2B). Significant amount of rainfall arrived mainly from the Atlantic sector (West), particularly in summer and autumn, whereas the other three sectors contributed little to the annual precipitation. Rainfall events caused by air masses arriving from the Mediterranean deserve special attention, since Saharan dust (SAD) events are known to deliver significant amount of solid material to the region (Varga 2020). SAD transport was identified by modeling 72-h trajectories for air masses arriving from the South. As a result, five individual SAD events were detected in the sampling period, three in spring (with 4, 55 and 3 mm rain recorded), one in summer (9 mm), and one in autumn (5 mm).
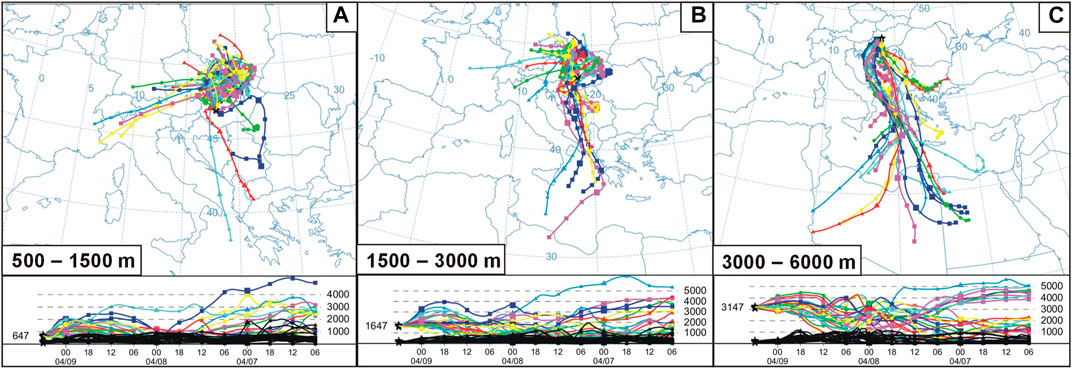
FIGURE 3. 72-h airmass back-trajectories for a Saharan dust event (08 April 2016), for height ranges of (A) 500–1,500, (B) 1,500–3,000 and (C) 3,000–6,000 m (using the HYSPLIT model).
The amount of filtered solid material at each rain event varied from below detection limit to 0.24 g·m−2 (Figure 2B). On an event by event basis, no relationship was apparent between the amount of precipitation and the mass of solid material. While transport from the Atlantic sector resulted in most of the collected precipitation (73% of the total), these events delivered only 58% of the measured TSM. In contrast, three of the five identified SAD events delivered a large amount solid material. One of these events (on May 11–12, 2016) also brought the heaviest rain of the year (55 mm), but the other two events (8 April 2016 and 19 June 2016) had relatively little precipitation associated with significant amount of solid material. Thus, compared to their contribution to the annual precipitation, SAD events delivered a disproportionately large amount of allochtonous minerals into the lake. We studied the mineralogy of solid material collected during the SAD event of 8 April 2016 by using SEM and XRD. The sample was dominated by common rock-forming minerals, including clays, quartz, feldspars and carbonates, with grain sizes reaching several tens of microns. In addition, pollen were also abundant. Given the high variability of the measured solid content of individual rain events, and the fact that we collected only 69% of the annual rainfall, an upper limit of 2,100 ty–l of solid matter delivered by rain to the lake can be assumed.
Dry deposition of atmospheric particles also contributes to the mineral budget of Lake Balaton. Annual concentrations of settling dust were measured by the National Air Pollution Measurement Network at several locations around the lake until 2007, and only in Siófok after 2007 (Figure 2C). No apparent relationship could be observed between the amounts of annual dust concentrations and precipitation; 2003 and 2009 were extremely dry years but the annual dust concentrations did not show outstanding values. In lack of seasonally resolved data it was impossible to identify and separate the effects of distant and local sources. As seen from the data reported for the years before 2007, there were significant differences in the measured concentrations among the individual locations, in some years reaching a factor of 4 between the lowest and highest values. Apparently, local sources from construction, agriculture and traffic might have strongly affected the amount of settling dust, limiting the accuracy of any estimation of total dust deposition. Based on the average of all available data for the period from 2003 to 2017 (Figure 2C), we estimated a total of 33,000 ty–l dry deposition directly onto the lake surface, a value about 16 times larger than the amount of solid material delivered by precipitation. In the present study we did not collect and analyze settling dust; however, several earlier studies found that dry atmospheric deposition in the region is dominated by common rock-forming minerals, including silicates and carbonates (Hlavay and Polyák 1998; Hlavay et al., 2001; Jancsek-Turóczi et al., 2013).
3.2 Tributaries: Suspended solids and water chemistry
Tributaries bring both water and solid particles into the lake. While the chemistry of the former determines mineral-forming processes within the lake, the latter is responsible for delivering a significant fraction of the allochtonous constituents of the lake sediment. The amount of incoming solid material per unit volume of water was estimated by measuring the mass of filtered particles (in g m−3) in all major and some smaller tributaries (Supplementary Figure S1A, panel marked ‘TSM’). Water discharge showed large variations, both annually (the total discharge by tributaries into Lake Balaton was 174, 120 and 193 m3·–s in 2016, 2017 and 2018, respectively) and seasonally, particularly for the smaller streams. During flooding higher loads of TSM per unit volume were carried than under normal conditions; values larger than about 15 g·m−3 TSM in Supplementary Figure S1 correspond to conditions characterized by rainfall or melting snow. Since we could not take samples continuously, the variations in TSM input between high- and low-water conditions could not be accurately accounted for. Nevertheless, each stream was sampled 12 times and the Zala river 23 times over a 2-year period (Supplementary Table S2), and both normal and high-water conditions were encountered, so we chose to take the average of the values of measured TSM per unit volume of water for each watercourse. The average amount of TSM over the sampled 2-year period varied between 3.9 and 11.9 g·m−3 for the 12 sampled inflows. Only three tributaries were responsible for more than 90% of the water discharge into the lake (Figure 4A), making these inflows the largest suppliers of allochtonous minerals to the lake. Zala river, Nyugati-övcsatorna and Keleti-Bozót-árok were characterized by 3.9, 4.8 and 7.4 g·m−3 average TSM values, respectively.
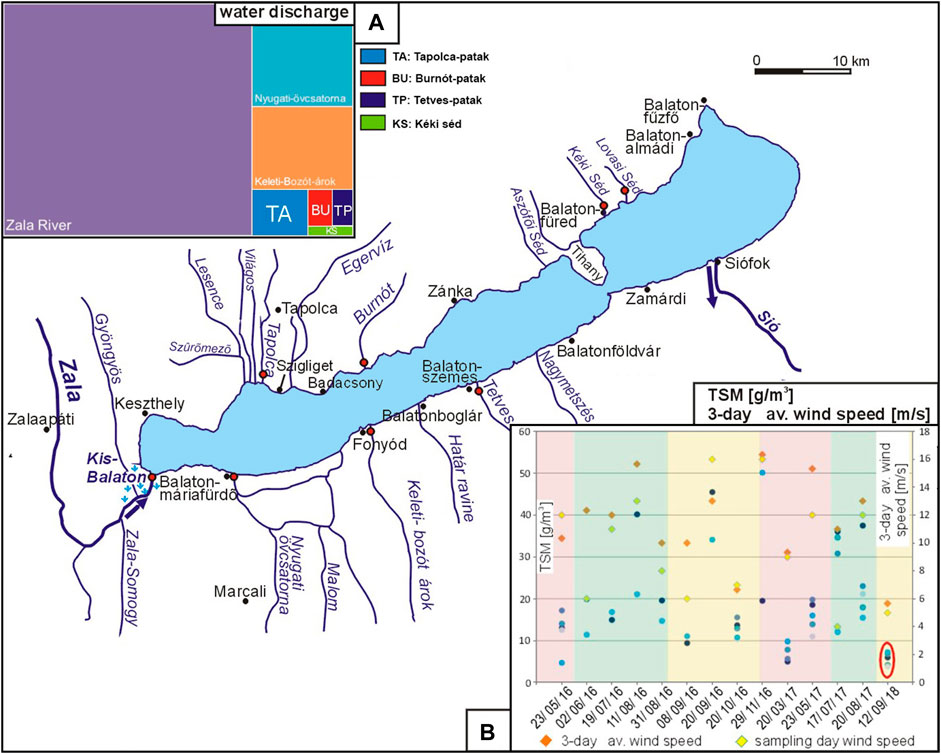
FIGURE 4. (A) Water discharge of the studied tributaries between 2016 and 2018, based on monthly average values (obtained from the official reports of the Central Transdanubia Water Directorate). (B) Total suspended matter (TSM; g m−3) measured in Lake Balaton water and wind speed (m s−1) averaged for 3-day periods preceding (and including) the date of sampling. The background colors of the charts mark seasons: pink for spring, green for summer, yellow for autumn, and blue for winter. Red ellipse indicates a sample taken during a calm period (12 September 2018).
The total amount of suspended matter delivered by tributaries into the lake was estimated by multiplying the measured average TSM per unit volume for each stream with the annual water discharge of the stream, as reported by the Central Transdanubian Water Directorate (CTWD), and then adding the mass of material carried by all streams. Since we did not sample some of the small tributaries (many of which are ephemeral), we applied correction factors for the total water discharge. The CTWD divides the lake into four parts from West to East (I. Keszthely Basin, II. Szigliget Basin, III. Zánka Basin and IV. Eastern/Kenese Basin; see Figure 1), and reports the total water discharge for each basin annually. The watercourses we sampled contribute close to 100% of the reported discharge in Basin I, 60% in Basin II, 30% in Basin III and only 14% of Basin IV. Therefore, we multiplied the measured annual TSM mass with 1, 1.7, 3.5, and 7.1 for Basins I, II, III, and IV, respectively. Since the vast majority of water input arrived in Basins I and II, and the amount of TSM delivered into Basins III and IV was almost negligible, this procedure was assumed to carry a relatively small error. In summary, annual mass of TSM delivered by tributaries was calculated as 24,000 t·y–l, with most of the material arriving into Basin I (11,000 t·y–l) and Basin II (10,000 t·y–l).
The measured elemental concentrations of major cations (Na+, K+, Mg2+, and Ca2+) and anions (HCO3−, Cl−, NO3−, and SO42-) showed some fluctuations, particularly in the smaller streams (Supplementary Figure S1, S2). In general, all watercourses were characterized by relatively high concentrations of HCO3− (averaging 5.5, 6.2 and 6.4 mmol·L−1in the Zala River and in northern and southern shore inflows, respectively), Ca2+ (averaging 1.6 mmol·l−1) and Mg2+ (with averages of 1.4 mmol·l−1 in the Zala River and 2.1 mmol·l−1 in the smaller streams) (Supplementary Figure S1A). The Mg/Ca molar ratio averaged 1.7. Outlier Mg/Ca values were measured in Burnót-patak (12), Tetves-patak (13) and Keleti-bozót árok (8.5) on two sampling dates (06.04.2017 and 23.05.2017) and probably resulted from measurement errors and were thus omitted from further analysis. The concentrations of Na+, K+, Cl−, NO3−, and SO42- are shown in Supplementary Figure S2; among these, the relatively higher concentrations of Na+ (1.2 mmol·l−1) and Cl− (0.8 mmol·l−1) in the Zala River deserve to be mentioned. A constrained cluster analysis was carried out on the basis of principal components of main ion concentrations (Figure 5). The distribution of tributaries in clusters appeared to correspond primarily to the geology of the provenance: the carbonate-rich catchment area of some north shore inflows separated them from the other smaller tributaries arriving from mixed geological terrain, whereas the Zala River was distinct from all other streams (Figure 5A).
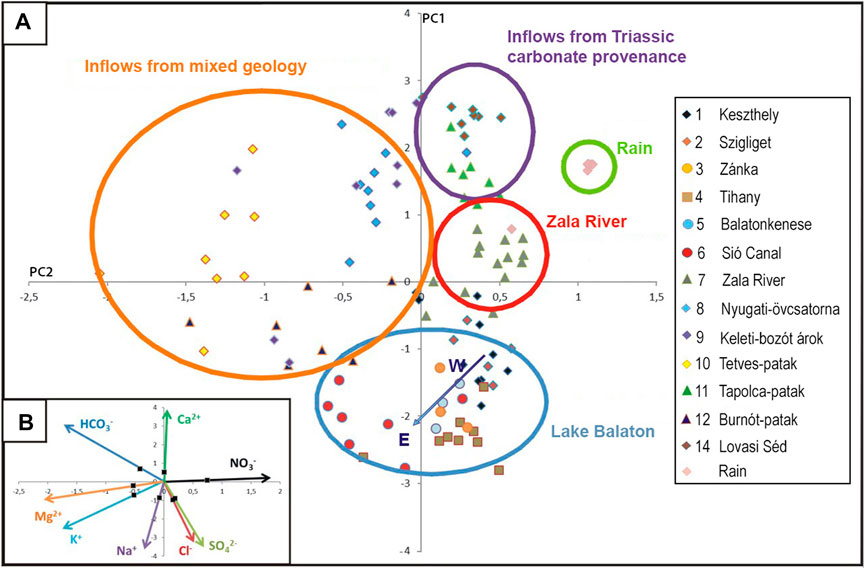
FIGURE 5. (A) Principal component and cluster analysis of water chemical parameters at the sampling sites in tributaries and Lake Balaton, based on concentrations of the ions HCO3−, NO3−, SO42-, Cl−, Ca2+, Mg2+, Na+ and K+. (B) Effects of increasing concentrations of analyzed ions, squares represent their weight.
3.3 Mineral formation in Lake Balaton
3.3.1 Lakewater chemistry
The chemistry of shallow Lake Balaton is sensitive to changes in the annual water budget (Szilágyi 2005). However, over the 2 years of our sampling campaign the lake had a relatively high water level, without any significant fluctuations. Therefore, our 66 individual lakewater samples from six locations (Keszthely, Szigliget, Zánka, Tihany, Balatonkenese and Sió Canal) show minor variability in chemical composition both in space and time (Supplementary Figure S1B). Concerning the ions that form carbonate minerals, the lakewater had significantly lower concentrations of Ca2+ and HCO3− than the tributaries (while the concentration of Mg2+ was essentially the same as in the streams). Along the longitudinal axis of the lake the average concentration of Mg2+ changed from 2.0 (Western Basin) to 2.8 mmol·l−1 (Siófok, Eastern Basin), while the average Ca2+ concentration decreased from West (Keszthely, 0.83 mmol·l−1) to East (Siófok, 0.67 mmol·l−1); as a result, average Mg/Ca mol ratios were lowest at Keszthely and highest in the Eastern Basin (at Tihany and Balatonkenese). The primary reason for the contrasting gradients of Ca2+ and Mg2+ is the precipitation of low-magnesian calcite from lakewater, a process that removes part of the dissolved Ca2+ while causes little change in the Mg2+ concentration (Müller et al., 1978; Tullner, 2002; Nyirő-Kósa et al., 2018). A secondary reason is the difference in the balance of the water budgets between the western and eastern sections of the lake, the latter receiving negligible direct input through tributaries, and therefore being more susceptible to the concentrating effect of evaporation. Because of this secondary effect, values of Na+, K+, SO42- and Cl− concentrations were also slightly higher in the Eastern than in the Western Basin (Supplementary Figure S3; Supplementary Table S5). The West–East chemistry gradients were also apparent in the positions of lakewater data points in the cluster analysis (Figure 5). The pH of the water did not show a systematic variation with geography, with typical values around 8.5, except in the spring and early summer of 2016 when values higher than 9 were measured (Supplementary Figure S1B).
Lakewater chemistry is responsible for some of the differences in the properties of allochtonous and autochtonous carbonate minerals of the sediment. Allochtonous carbonate particles from distinct sources (carried by tributaries and deposited dry or wet from the atmosphere) have similar mineralogies as the lake sediment, since both contain calcite and dolomite. However, detrital carbonate particles were found mostly in grains of several tens of μm, and typically with euhedral morphologies (Figures 6A–D). In contrast, particles that make up the lake sediments (Figure 6E) were found to be dominated by low-magnesian calcite with a distinct, elongated, aggregate-looking morphology and a rather constrained, smaller particle size (Figure 6F) between about 2 and 8 μm (Nyirő-Kósa et al., 2018). In addition, their compositions and, consequently, the cell parameters of autochtonous carbonates distinguish them from their detrital counterparts.
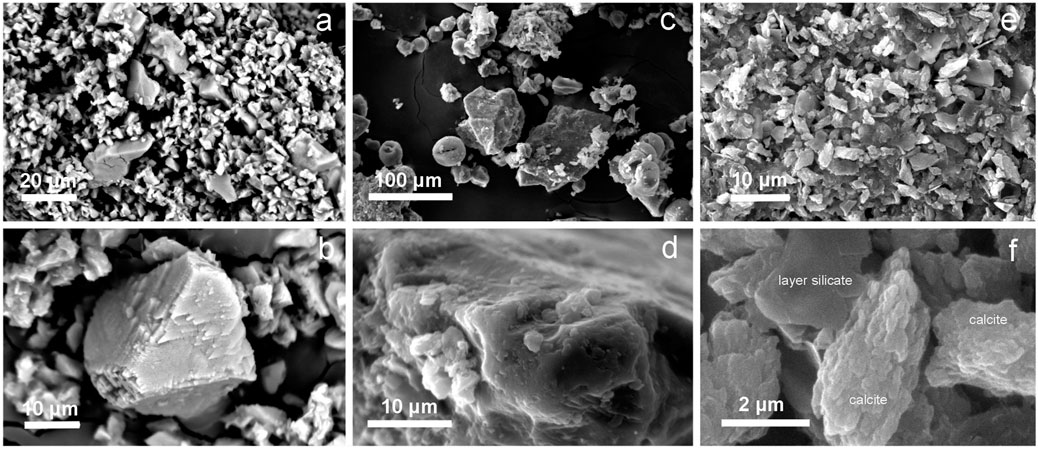
FIGURE 6. Secondary electron SEM images of typical mineral particles filtered from stream, rain and lakewater. (Note the different length scales of the images.) (A) Tributary-originated mineral grains with various sizes (small particles are calcite and clay minerals, large ones are quartz crystals). (B) A weathered calcite particle from Lovasi Séd stream. (C) Large quartz grains, pollen and unidentified mineral particles from a rainwater sample. (D) A characteristic quartz particle with an eroded surface from a Saharan dust sample; (E–F) Mg-bearing calcite particles that precipitated from lakewater and allochtonous layer silicates from a lakewater sample; the morphologies and sizes of aggregate-looking, elongated Mg-bearing calcite crystals are typical for particles precipitated from Lake Balaton.
3.3.2 Structures and compositions of precipitating carbonates
Shifts in the values of cell parameters of calcite and dolomite, as measured using XRD, have long been used for the analysis of their Mg content (Goldsmith and Graf, 1958; Reeder and Sheppard, 1984; Zhang et al., 2010; Tompa et al., 2014). Because of the smaller size of the Mg2+ ion relative to Ca2+, its presence in calcite causes a shrinkage of the unit cell; conversely, dolomite with an anomalous, Ca-rich composition has a larger unit cell than stoichiometric dolomite. We measured the d104 lattice spacings of both calcite and dolomite in XRD patterns obtained from the suspended material that was filtered from 40-l water samples (Figure 7). Whereas the measured d104 values of calcite particles carried by the tributaries suggested a MgCO3 content between 0 and 4 mol%, the same parameter was smaller in calcite from the lake, corresponding to an average Mg content of about 6–8 mol% [as calculated using the calibration curve by Zhang et al. (2010)]. The difference in the Mg content of calcite types reflected their distinct origins: we assume the streams carried calcite abraded from mostly Triassic rocks (Figure 1), whereas the suspended particles in the lake represented predominantly autochtonous material that precipitated from the Mg-rich lakewater. We note that the compositions of individual Mg-bearing calcite particles from the lake fall into the ranges commonly termed low-Mg calcite (LMC) and high-Mg calcite (HMC); however, since the observed range of compositions was continuous between these categories, we use simply “Mg-bearing calcite” in this paper.
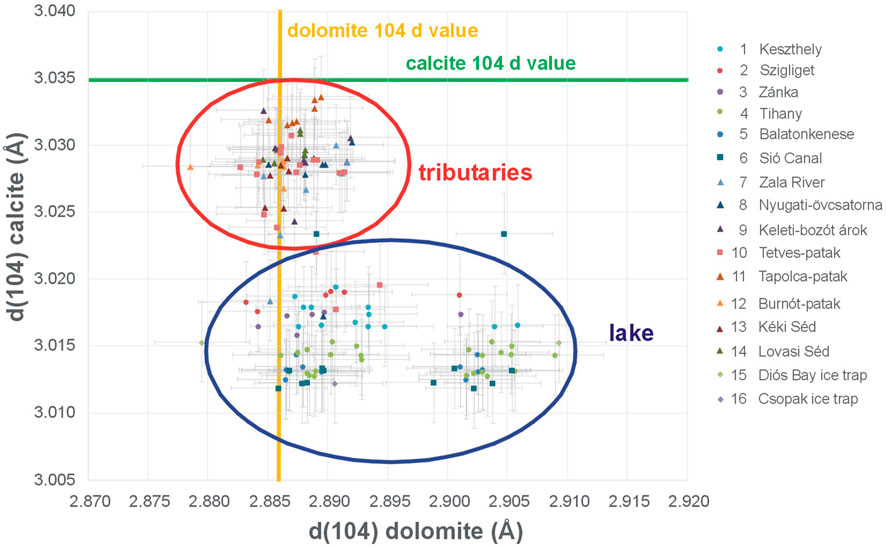
FIGURE 7. Calcite and dolomite d (−104)-values measured on XRD patterns obtained from suspended particles collected from tributaries, Lake Balaton, and the Sió Canal. Each datapoint represents a sample, except for some samples from the Eastern Basin of the lake, for which the dolomite −104 peak was split and thus two data points correspond to each sample (error bars: ± 0.003 Å). The d (−104) values of stoichiometric calcite and dolomite are represented by the horizontal green and the vertical yellow lines, respectively.
Since dolomite was a minor component in our samples, it produced a relatively low-intensity 104 peak in the XRD patterns. Nevertheless, a shift in the position of this peak between different samples was clearly observable (Figure 7). The measured d104 lattice spacings scattered around the value for stoichiometric dolomite (2.885 Å) in the samples collected from tributaries. On the other hand, the majority of suspended matter samples collected from the lake, particularly the ones from the Eastern Basin, contained dolomite that produced a split 104 peak in XRD patterns (Figure 7), suggesting the presence of at least two different varieties. One type of dolomite produced d104 values close to that of the stoichiometric mineral, presumably indicating its detrital origin. The other type appeared to be a Ca-rich variant (with a larger d104 value), and its presence in the Eastern Basin suggested a relationship between its formation and the higher dissolved Mg/Ca ratio of the water than in the western part of the lake.
Our TEM observations of individual particles confirmed the large variation of Mg content in Mg-bearing calcite particles and the close association of calcite with nm-scale flakes of the clay mineral smectite, as reported by Nyirő-Kósa et al. (2018). In addition, the anomalous, Ca-rich dolomite fraction identified by XRD could be characterized using combined information from TEM images, SAED patterns and EDS element maps (Figure 8). A group of carbonate particles and a larger diatom fragment are shown in Figure 8A, from a sample collected in a sediment trap in Csopak (Eastern Basin) in January 2017. Whereas four of the numbered carbonate particles were Mg-bearing calcite with a Mg/Ca mol ratio of 0.1 or less (Figure 8E), particles #2 and #6 had very high Mg contents (Mg/Ca = 0.6 in the case of #6 (Figure 8F). A SAED pattern obtained from the same particle #6 (Figure 8H) displayed rows of reflections (marked by arrows) that could either suggest a dolomite-like ordering of Ca and Mg in its structure or were produced by multiple diffraction from a calcite-type structure. Further evidence for a nanoparticle with dolomite-type structure is presented in Supplementary Figure S5. Therefore, we interpret the anomalous dolomite reflections observed in XRD patterns as produced by “protodolomite” particles (such as #2 and #6); we use this term to indicate that protodolomite has some ordering of Ca and Mg in its structure but can be enriched in Ca relative to proper dolomite (in which Mg/Ca = 1).
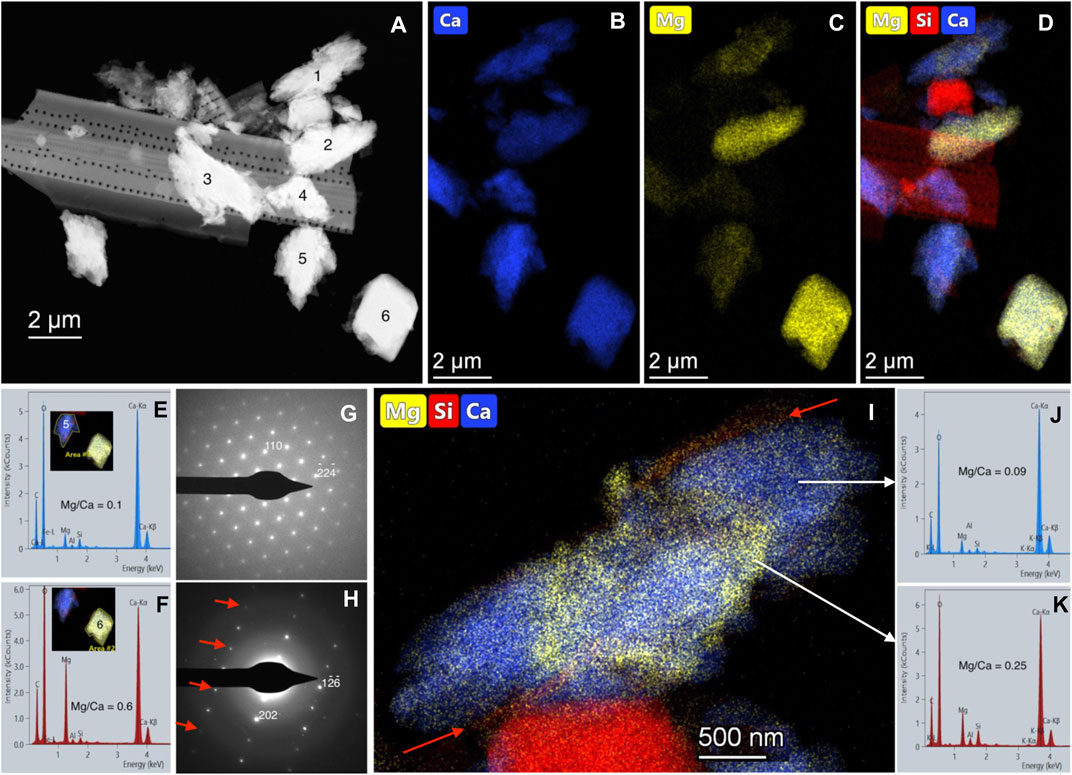
FIGURE 8. TEM analysis of typical autochtonous carbonate particles in Lake Balaton. (A) High-angle annular dark-field (HAADF) STEM image of carbonate particles (numbered) and a larger diatom fragment from suspended matter. (B–D) Ca, Mg, and combined Ca+Mg+Si energy-dispersive STEM X-ray spectrometry (EDS) element maps. (E) and (F) EDS spectra and Mg/Ca mol ratios of particle #2 (Mg-bearing calcite) and particle #6 (protodolomite), respectively. (G–H) Selected-area electron diffraction (SAED) patterns of particles #2 and #6; the red arrows mark rows of reflections that are forbidden by the calcite space group; they could arise either from multiple diffraction effects or from the ordering of Mg and Ca in particle #6. (I) Composite of Ca, Mg and Si maps of particle #1. The red arrows mark flakes of smectite. (J–K) EDS spectra and Mg/Ca ratios of selected Mg-poor and Mg-rich regions (as indicated by the white arrows in (I) in particle 1#.
STEM-EDS element maps revealed compositional variety even within some single particles. An example is shown by a composite of Ca, Mg, and Si maps of particle #1 (Figure 8I) that displayed a zone of high Mg content (Mg/Ca = 0.25; Figure 8K) within a typical low-magnesian calcite host (having Mg/Ca = 0.09; Figure 8J). The Mg-rich zone appeared to occur along the rim of an approximately rhombohedral phantom crystal. Although we found that low-magnesian calcite with homogeneous composition (such as particles #3, 4, and 5 in Figure 8) was the dominant carbonate particle type in suspended matter, both Ca-rich “protodolomite” (such as particles #2 and 6) and low-magnesian calcite with a non-uniform Mg distribution (such as particle #1) appeared to be ubiquitous minor constituents.
3.3.3 Estimated mass of precipitating carbonate
The mass of annually precipitating carbonate can be estimated in several ways, all of which produce rather inaccurate results. For example, annual carbonate precipitation can be estimated by considering the balance of dissolved Ca2+ and Mg2+ arriving through tributaries and rain and leaving through the Sió Canal, and assuming that the difference constitutes the precipitated material (with the composition of lakewater being approximately constant over the residence time of water; the balance of HCO3− is not considered, since its concentration is affected by the dissolution of atmospheric CO2). By using our measured water chemistry data for tributaries, rain and the Sió Canal (Supplementary Figures S1, S4; Supplementary Table S5), and taking an average water balance of the 5 years from 2014 to 2018 (the residence time of lakewater is 4.7 years; Kutics, 2019), we calculated about 8.3 × 109 mol Ca2+ and 7.8 × 109 mol Mg2+ “remaining” in the lake annually. These values convert to nearly 1.5 × 106 t Ca-rich dolomite with a composition of Ca0.52Mg0.48CO3, equivalent to 0.88 mm consolidated sediment thickness.
Measuring the amount of suspended material in the lake may appear the most direct way of estimating the mass of precipitating carbonate minerals. However, this approach is also ridden with uncertainties, since sediments in the shallow lake are prone to resuspension by turbulence resulting from even relatively gentle winds (Rákóczi 1987), thereby mixing freshly precipitated and older carbonate particles. Moreover, the intensity of carbonate precipitation is highly heterogeneous both spatially and temporally (for example, phytoplankton blooms can result in “whiting” events), making point measurements hardly representative. In our 66 samples the mass of total suspended matter (TSM) ranged between 3.81 and 50.20 g·m−3 (see the inset in Figure 4B). The average mass of TSM measured in the Western Basin (17.85 g·m−3) was significantly higher than in the Eastern Basin (8.41 g·m−3), probably due to the fact that the eastern part of the lake is deeper. Material in the Sió Canal is less affected by resuspension, and is characterized by an average TSM mass of 6.11 g·m−3 (1,300 t·y–l). The TSM graph in Figure 4B shows the average wind speed both on the day of sampling and over the 3 days preceding sampling. As expected, high wind speeds result in larger amounts of TSM, although there is significant variation in the measured TSM mass between different parts of the lake. We assumed that the sample taken on 12th September 2018, when a 3-day calm period preceded our sampling, did not contain much resuspended sediment and was thus the most representative for freshly precipitated carbonate. On this day we measured 5.68 g·m−3 TSM in the Western and 5.56 g·m−3 in the Eastern Basin. Based on Stokes’ law, calcite grains with a diameter of 5 μm need 3 days to settle 3 m, whereas grains of 2 μm size need a week. On this basis, it was considered that the amount of suspended material measured in the water sample of 12th September 2018 represented freshly precipitated calcite formed in about 3 days. Based on this figure and assuming continual calcite precipitation over a year, annually about 1.3 × 106 t carbonate forms in the lake, producing a layer of 0.76 mm thickness.
4 Discussion
4.1 Sediment budget
We estimated the annual contributions of distinct sources to the sediment budget of Lake Balaton, based on the amount of suspended material in inflows, rain and lakewater, as measured over a 2-year period, and using data for atmospheric dry deposition (Figure 9). This budget includes mineral constituents only. Organic matter, such as biological debris, has not been considered. Nevertheless, in Lake Balaton organic matter rapidly decomposes due to the lake’s shallowness, frequent mixing and good oxygen supply (Szilágyi 2005), resulting in typically <2% organic carbon content in the upper 10 cm of the sediment (Máté 1987). As far as external sources are considered (streams, rain and dry atmospheric deposition), our estimates for the amount of solid matter delivered to the lake (Figure 9) are basically consistent with the results of earlier studies (Supplementary Table S1). We found a smaller amount of solid material delivered by tributaries (24,000 t·y–l) than former estimates (30,000–50,000 t·y–l, Virág, 1998), probably reflecting the effect of the gradual restoration of the Kis-Balaton wetlands since the 1980s that resulted in less solid matter discharge into the lake by the Zala River (Hatvani et al., 2014; Honti et al., 2020). A noteworthy result of our measurements is the observation of significant contributions of Saharan dust events to the solid material in rainwater, and probably also to dry deposition (Rostási et al., 2022). Since climate change appears to have rearranged synoptic meteorological patterns (Varga 2020), the incursion of southern air masses became more frequent in recent years (Varga et al., 2013), and the amount of Saharan dust reaching Lake Balaton is expected to increase in the future.
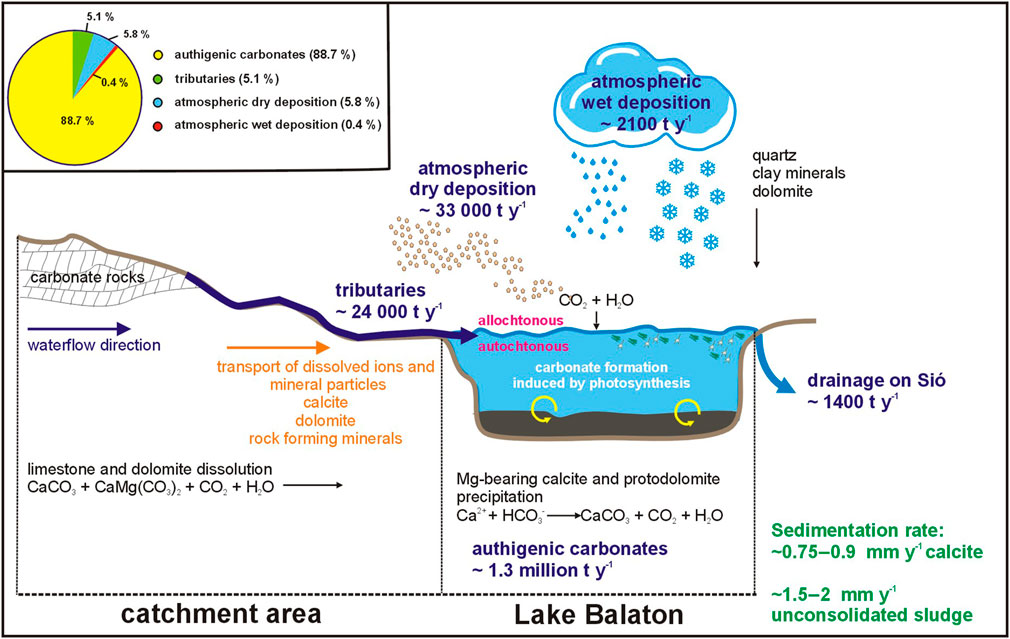
FIGURE 9. Sketch of estimated mineral budgets from distinct sources and sedimentation rates in Lake Balaton, with the main mineralogical features and controlling factors.
In contrast to external sources of sediment material, our results significantly differ from earlier data concerning authigenic mineral formation (Supplementary Table S1). According to our estimates, carbonates precipitating from lakewater overwhelm material from other sources, contributing almost 90% of the mass of current sediment formation (Figure 9). The average thickness of lake sediment is about 6 m, deposited over 15 k years (Cserny et al., 1995). If we consider 2/3 of this material to consist of autochtonous carbonate minerals, then an annual increment of 0.27 mm pure carbonate results. This figure does not take into account variations in the intensity of carbonate precipitation over time. In the cooler, rainier climates of the first few thousand years of the lake’s history a large amount of siliciclastic debris was delivered into the lake (Müller et al., 1978; Cserny and Nagy-Bodor 2000). Thus, current rates of carbonate accumulation may be larger than the value obtained from the average sediment thickness.
As mentioned above, any estimate of the rate of carbonate accumulation carries a large error. Nevertheless, similar values resulted from our two different approaches: 0.76 mm·y−1 from the mass of suspended matter, and 0.88 mm·y−1 from the balance of dissolved Mg+Ca ions. Since sediment resuspension in the shallow lake is a common phenomenon, we used the data obtained for the calmest period (Figure 4B; Supplementary Figure S1B and Supplementary Table S2) as the upper bound of the precipitating calcite mass. Our measured average TSM mass for these calm conditions (∼5.6 g·m−3) is in agreement with the lower ranges of TSM concentrations measured by others. For example, LIDAR observations resulted in a TSM range of 2.93–35 g·m−3 (Palmer et al., 2013) and 10–80 g·m−3 (Pelevin et al. (2017), with the latter data also validated by satellite imaging. Earlier studies, based on mass measurements of deposited material from water also reported background TSM concentrations of 2–5 (Gelencsér et al., 1982) and 2–7 g·m−3 Virág (1998). Temporal changes in calcite precipitation notwithstanding, we believe our estimates from the measured TSM mass can be regarded as the upper limits of carbonate accumulation.
The above carbonate sedimentation rates were calculated for the density of calcite (2.71 g·cm−3); thus, these values refer to solid rock. However, at least the upper half meter of the sediment is a loose mud with a large water content (assumed to be 70%; Gelencsér et al., 1982), resulting in an annual increment of the sediment about twice the thickness of pure calcite. Therefore, our measurements suggest a current annual rate of carbonate sludge accumulation of 1.5–1.7 mm·y−1, as averaged over the entire lake. These values are significantly larger than the 0.4–0.6 mm·y−1 rate that was calculated on the basis of radiocarbon and palynological dating (Cserny 2006). However, recent studies suggested an even larger rate for the last few decades. On the basis of zebra mussel (Dreissena polymorpha) remains, the sediment accumulation rate was estimated at ∼5.5 mm·y−1 year for the topmost (40 cm) sediment (Korponai et al., 2011), whereas seismic measurements in the Eastern part of the lake were interpreted to reflect a ∼8.8 mm·y−1 year accumulation since ∼1980 (Zlinszky et al., 2010). Dating of a sediment core obtained in the middle of the lake using a combination of 14C, 210Pb and 137Cs data suggested that the upper 32 cm of the sediment accumulated over just ∼150 years (Pálfi et al., 2021), yielding an accumulation rate of 2.1 mm·y−1, slightly larger than (but generally consistent with) our estimates. Since turbulence and currents move and redeposit sediment material, the sediment accumulation rate varies by location, as was shown by both sediment stable isotope (Cserny et al., 1995) and seismic measurements (Zlinszky et al., 2010; Visnovitz et al., 2021). In summary, we estimate an average rate of ∼1.5–2 mm·y−1 sludge accumulation, with current sediment formation dominated by the precipitation of carbonate minerals.
4.2 Formation and properties of authigenic carbonates
Our XRD and TEM results confirm that Mg-bearing calcite is the main precipitating carbonate phase, and that its Mg content increases along a chemical gradient in the lakewater from west to east. While these facts have been reported before (Müller et al., 1978; Tullner et al., Cserny et al., 2009; Tompa et al., 2014; Nyirő-Kósa et al., 2018), we showed for the first time that the occurrence of protodolomite also follows the same chemical gradient (Figure 7). Protodolomite is present in the Eastern Basin of the lake, and its appearance is clearly related to Mg-rich conditions in water chemistry.
In addition to its spatial pattern, temporal changes also occur in the dissolved Mg/Ca ratio, according to the hydrological balance. Dry periods result in a lowering of lake level, increased ion concentrations, and higher Mg/Ca ratios (Müller et al., 1978; Tullner 2002). The annual water budget of Lake Balaton is determined by the balance of inflow+precipitation (I+P) vs. evaporation+drainage (E+D) (van Straten et al., 1979), typically expressed in changes of water level (in mm y−1). The 2 years of our study were characterized by slightly contrasting water balances, with a 90 mm·y−1 excess water in 2016 (I=705, p=652, E=787 and D=480 mm·y−1), and a deficit of −80 mm·y−1 in 2017 (I=530, p=587, E=897, and D=300 mm·y−1, KDT, 2017; KDT, 2018). However, such small changes in the water budget did not result in noticeable differences in the average composition of Mg-bearing calcite or the relative amount of protodolomite in our suspended matter samples. Studies of sediment cores showed the presence of either one (Müller et al., 1978) or two distinct, protodolomite-rich layers ∼5,300 and 8,100 years before present (Pálfi et al., 2021), attesting to specific events in the history of the lake with higher Mg concentration in lakewater and presumably much drier conditions than today.
Our observations suggest that protodolomite forms by similar processes as the dominant carbonate, Mg-bearing calcite. In addition to their co-occurrence in the suspended matter in the Eastern Basin, particles of the two minerals have similar sizes, morphologies, and both are attached to the clay mineral smectite (Figure 8). However, the exact timescale of the formation of these carbonates is difficult to assess. When calculating the amount of precipitating calcite, we assumed that under calm conditions the suspended material consists of freshly precipitated particles. Indeed, whiting events in the lake appear to produce calcite instantaneously (Pósfai, 2020). In contrast, in laboratory experiments designed to simulate Lake Balaton conditions (including water chemistry, temperature, pH, and the presence of smectite), aragonite was the first carbonate phase to precipitate, and composite particles of protodolomite and Mg-bearing calcite appeared only after a few months of aging (Molnár et al., 2021). It is possible that factors absent from our laboratory setup accelerate the precipitation of calcite-type, Mg-rich carbonates in the lake. For example, organics (Fang et al., 2022) and dissolved silica (Fang and Xu, 2022a, Fang and Xu, 2022b) have been shown to catalyze dolomite formation; however, these species were neither included in our experiments, nor measured in the lake. On the other hand, we cannot exclude the possibility of some particles in the suspension representing aged material, recycled from the topmost layers of the sediment. Nevertheless, our results strongly suggest that instead of being a product of transformation from calcite within the sediment over extended periods of time, as have been implied before (Müller et al., 1978; Cserny et al., 1991), protodolomite forms in contact with lakewater.
The observed intraparticle heterogeneity of Mg content (such as in Figure 8I) may suggest that dissolution-reprecipitation processes are important in forming protodolomite with a partially ordered distribution of Mg and Ca atoms in its structure. According to thermodynamic modeling, the typical Mg-bearing calcite in Lake Balaton is metastable at any range of conditions (including T, pH and water chemistry) occurring at present in the lake (Figure 10). In contrast, calcite, dolomite and disordered dolomite are stable most of the time. The dissolution of a thermodynamically metastable phase may produce high local supersaturation at the surface of the mineral, triggering its replacement by a more stable phase, the direct precipitation of which is kinetically hindered (Putnis 2009). Such coupled interfacial dissolution-reprecipitation phenomena have been recognized as important in forming Mg-rich phases on carbonate surfaces (Ruiz-Agudo et al., 2014), and could also have resulted in the observed Mg-rich zones in particles from Lake Balaton. In our previous studies on carbonates from Lake Balaton (Nyirő-Kósa et al., 2018) and on their model systems (Fodor et al., 2020; Molnár et al., 2021) we identified the important role of smectite clay in the nucleation and growth of Mg-bearing calcite and protodolomite. The clay mineral was shown to serve as a suitable surface for the nucleation of Mg-bearing calcite. In addition, we assume that the nm-scale flakes of smectite could provide compartmentalized spaces in which high local supersaturations can be achieved when metastable Mg-bearing calcite locally dissolves, eventually leading to the reprecipitation of Mg-rich carbonate, including protodolomite. Whether these dissolution-reprecipitation phenomena happen in the water column or at the sediment/water interface (where aerobic oxidation of organic matter lowers the pH) should be the subject of future studies; nevertheless, the processes are rather complex and even advanced stable isotope studies may return ambiguous results (James et al., 2021).
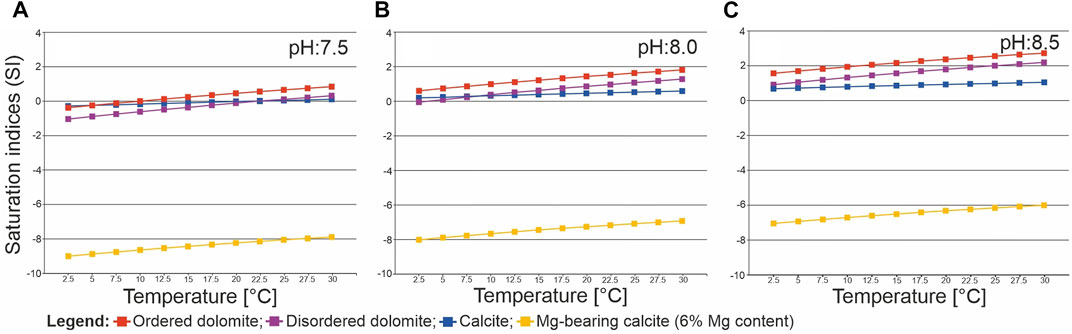
FIGURE 10. Calculated saturation indices for Mg-bearing calcite (with a composition Ca0.94Mg0.06CO3), calcite, disordered dolomite and ordered dolomite, as a function of temperature and considering a typical Lake Balaton water chemistry, at three different pH values that are roughly representative of (A) the sediment, (B) the sediment-water interface, and (C) the water column.
4.3 Implications
Arguably, the most important aspect of mineral accumulation (and potential dissolution) in lakes is the role these minerals play in nutrient cycles. The key nutrient, P originates from external sources; however, today the amount of total P delivered by the Zala river is about the fourth of the values that were typical in the 1970s and 1980s (Istvánovics et al., 2007; Honti et al., 2020). This is consistent with our data that suggest a relatively minor fraction of the currently depositing solid material being delivered to Lake Balaton by tributaries and through the atmosphere. This shift in the strength of P sources, primarily resulting from large-scale lake management measures, highlights the importance of intralake processes in controlling the bioavailability of nutrients, especially in the case of extreme events, including a record-setting algal bloom in 2019 (Istvánovics et al., 2022).
The majority of P stored in the sediment is likely bound to carbonate minerals, and to a lesser extent to iron oxides (van Straten et al., 1979; Gelencsér et al., 1982). The detection limit of our EDS technique is ∼0.1 w% P when it is associated with Ca, about an order of magnitude larger than the expected P concentration. Nevertheless, the results discussed above (see Figure 10) suggest that dissolution (and reprecipitation) of Mg-bearing calcite likely occurs, particularly within the surface layer of the sediment where oxidative dissolution of organic matter produces CO2 and lowers the pH. Whereas cycles of carbonate dissolution could release bioavailable P, under typical lake conditions carbonate precipitation and the associated P adsorption would balance this effect, as suggested by our estimate of a net increase of the amount of carbonate in the sediment. A comprehensive analysis of hydrometeorological data identified anaerobic P release as the only feasible driver of the algal bloom of the summer of 2019 in the western part of Lake Balaton (Istvánovics et al., 2022). However, the mineralogical basis of an anaerobic P release is not trivial. With a very low concentration of iron oxides in the sediment (not even detected in XRD patterns and TEM micrographs), carbonate minerals appear to be the most likely source of P, even though they are not supposed to be sensitive to redox changes (Bańkowska-Sobczak et al., 2020). Further studies should address the question whether in some indirect ways, perhaps involving biological intermediaries, anaerobic conditions lead to P release from carbonate minerals.
Climate change will inevitably affect, and is already affecting, sedimentation processes. While warming coupled with high lake levels may lead to bottom hypoxia, as discussed above, extended hot and dry periods in the summer have also become more common in the 21st century, with the largest drop in lake level recorded in 2003–2004 (Somlyódi and Honti 2005). Heterogeneity in the temporal distribution of precipitation is predicted to increase in the future, with drier springs and summers than at present (Kis et al., 2017). Such conditions will result in lower lake levels, higher ionic strength and increased Mg/Ca ratio of lakewater, and the formation of more Mg-rich carbonates, including protodolomite. Changing regional weather patterns (Varga et al., 2021) may also result in more Saharan dust reaching the lake. Although this process contributes a minor fraction to the sediment, the long-range transport of dust and possibly nutrients deserves further study. Although our results were obtained for Lake Balaton that has some specific features, most of the above implications hold for any shallow lake with carbonate-dominated sediments. Our estimate of almost 90% of the currently forming sediment being authigenic carbonate highlights the significance of intralake processes in cases when long-term water management measures reduced the amount of materials from external sources.
Data availability statement
The original contributions presented in the study are included in the article/Supplementary Material, further inquiries can be directed to the corresponding author.
Author contributions
ÁR initiated and carried out field measurements, collected and interpreted data. KR and MF performed bulk chemical measurements, BT and TW performed and interpreted XRD measurements, ZM calculated geochemical models, MP performed TEM measurements and took part in planning the study. ÁR and MP wrote the manuscript.
Funding
Research by ÁR was supported by the National Research, Development and Innovation Office (NKFIH) (PD 121088). Further financial support for this study was provided by NKFIH under grants K134559 and RRF-2.3.1-21-2022-00014.
Acknowledgments
The authors would like to thank Csaba Kis and Géza Dobos for their help with sample collection. We acknowledge the help of Judit Szauer, Valéria Andirkó and Miklós Jakab with sample preparation, analytical measurements and SEM analysis, respectively. We are grateful to Vera Istvánovics for her helpful comments. The published trajectories were provided by the HYSPLIT transport model website (https://ready.arl.noaa.gov/hypub-bin/trajsrc.pl) of NOAA Air Resources Laboratory (AAL).
Conflict of interest
The authors declare that the research was conducted in the absence of any commercial or financial relationships that could be construed as a potential conflict of interest.
Publisher’s note
All claims expressed in this article are solely those of the authors and do not necessarily represent those of their affiliated organizations, or those of the publisher, the editors and the reviewers. Any product that may be evaluated in this article, or claim that may be made by its manufacturer, is not guaranteed or endorsed by the publisher.
Supplementary material
The Supplementary Material for this article can be found online at: https://www.frontiersin.org/articles/10.3389/feart.2022.1067105/full#supplementary-material
References
Báldi, K., Balogh, C., Sztanó, O., Buczkó, K., B-Muskó, I., G-Tóth, L., et al. (2019). Sediment contributing invasive dreissenid species in a calcareous shallow lake – possible implications for shortening life span of lakes by filling. Elem. Sci. Anthropocene 7 (1). doi:10.1525/elementa.380
Bańkowska-Sobczak, A., Blazejczyk, A., Eiche, E., Fischer, U., and Popek, Z. (2020). Phosphorus inactivation in lake sediments using calcite materials and controlled resuspension—Mechanism and efficiency. Minerals 10 (3), 223. doi:10.3390/min10030223
Bénézeth, P., Berninger, U. N., Bovet, N., Schott, J., and Oelkers, E. H. (2018). Experimental determination of the solubility product of dolomite at 50–253 C°. Geochimica Cosmochimica Acta, 224, 262–275. doi:10.1016/j.gca.2018.01.016
Buczkó, K., Ács, É., Báldi, K., Pozderka, V., Braun, M., Kiss, K. T., et al. (2019). The first high resolution diatom record from Lake Balaton, Hungary in Central Europe. Limnetica 38 (1), 417–430. doi:10.23818/limn.38.24
Budai, T., Császár, G., Csillag, G., Dudko, A., Koloszár, L., and Majoros, Gy. (1999). A Balaton-felvidék földtana (magyarázó a Balaton-felvidék földtani térképéhez, 1:50 000). [Geology of the Balaton highland (explanation to the geological map of the Balaton highland, 1:50000)]. Occas. Pap.197, 171–257.
Cholnoky, J. (1918). “A Balaton hidrografiája,”, A Balaton tudományos tanulmányozásának eredményei I/. Editor L. Lóczy, 2, 1–318. (in Hungarian).
Cserny, T., and Corrada, R. (1990). Magyar Állami Földtani Intézet évi jelentése az 1989. évről (annual report of the Hungarian geological Institute of 1989, 169. Budapest: Hungarian Geological Institute–176.
Cserny, T., Hertelendi, E., and Tarján, S. (1995). Results of isotope-geochemical studies in the sedimentological and environmental geologic investigations of Lake Balaton. Acta Geol. Hung. 38, 355–376.
Cserny, T. (2006). “Lake Balaton, Hungary,”. Editors E. Gierlowski-Kordesch, and K. Kelts (Cambridge University Press), Vol. 1, 395–399.Glob. Geol. Rec. Lake Basins
Cserny, T., Nagy-Bodor, E., and Hajós, M. (1991). “Contributions to the sedimentology and evolution history of Lake Balaton,” in Quaternary environment in Hungary. Studies in geography in Hungary. Editors M. Pécsi, and F. Schweitzer (Budapest: Akadémiai Kiadó), 26, 75–84.
Cserny, T., and Nagy-Bodor, E. (2000). Limnogeological investigations on Lake Balaton. in In: E. Gierlowski-Kordesch, and K. Kelts (ed.) Lake basins though space and time, Tulsa, OK: American Association of Petroleum Geologists, 2000 605–618.
Dittrich, M., and Obst, M. (2004). Are picoplankton responsible for calcite precipitation in lakes? AMBIO a J. Hum. Environ. 33, 559–564. doi:10.1579/0044-7447-33.8.559
Draxler, R. R., and Rolph, G. D. (2012). HYSPLIT (HYbrid single-particle Lagrangian integrated trajectory) model access via NOAA ARL READY. MD: NOAA Air Resource s Laboratory, Silver Spring.
Elser, J. J., Bracken, M. E. S., Cleland, E. E., Gruner, D. S., Harpole, W. S., Hillebrand, H., et al. (2007). Global analysis of nitrogen and phosphorus limitation of primary producers in freshwater, marine and terrestrial ecosystems. Ecol. Lett. 10, 1135–1142. doi:10.1111/j.1461-0248.2007.01113.x
Entz, B., Ponyi, J., and Tamás, G. (1963). Sedimentuntersuchungen im südwestlichsten Teile des Balaton, in der Bucht von Keszthely in 1962. Ann. Biol. Tihany 30, 103–125.
Fang, Y., and Xu, H. (2022a). Coupled dolomite and silica precipitation from continental weathering during deglaciation of the Marinoan Snowball Earth. Precambrian Res. 380, 106824. doi:10.1016/j.precamres.2022.106824
Fang, Y., and Xu, H. (2022b). Dissolved silica-catalyzed disordered dolomite precipitation. Am. Mineralogist 107, 443–452. doi:10.2138/am-2021-7474
Fang, Y., Zhang, F., Farfan, G. A., and Xu, H. (2022). Low-temperature synthesis of disordered dolomite and high-magnesium calcite in ethanol–water solutions: The solvation effect and implications. ACS Omega 7, 281–292. doi:10.1021/acsomega.1c04624
Fedo, C. M., Nesbitt, H. W., and Young, G. M. (1995). Unraveling the effects of potassium metasomatism in sedimentary rocks and paleosols, with implications for paleoweathering conditions and provenance. Geology 1, 921–924. doi:10.1130/0091-7613(1995)023<0921:UTEOPM>2.3.CO;2
Fodor, M. A., Ható, Z., Kristóf, T., and Pósfai, M. (2020). The role of clay surfaces in the heterogeneous nucleation of calcite: Molecular dynamics simulations of cluster formation and attachment. Chem. Geol. 538, 119497. doi:10.1016/j.chemgeo.2020.119497
Gelencsér, P., Szilágyi, F., Somlyódi, L., and Lijklema, L. (1982). A study on the influence of sediment in the phosphorus cycle in Lake Balaton. Available at: http://pure.iiasa.at/2074.
Gierlowski-Kordesch, E. H. (2010). Chapter 1 Lacustrine Carbonates. Dev. Sedimentology 61, 1–101. doi:10.1016/S0070-4571(09)06101-9
Goldsmith, J. R., and Graf, D. L. (1958). Structural and compositional variations in some natural dolomites. J. Geol. 66 (6), 678–693. doi:10.1086/626547
Hatvani, I. G., Clement, A., Kovács, J., Kovács, I. S., and Korponai, J. (2014). Assessing water-quality data: The relationship between the water quality amelioration of Lake Balaton and the construction of its mitigation wetland. J. Gt. Lakes. Res. 40 (1), 115–125. doi:10.1016/j.jglr.2013.12.010
Hlavay, J., and Polyák, K. (1998). Chemical speciation of elements in sediment samples collected at Lake Balaton. Microchem. J. 58, 281–290. doi:10.1006/mchj.1997.1557
Hlavay, J., Polyák, K., and Weisz, M. (2001). Monitoring of the natural environment by chemical speciation of elements in aerosol and sediment samples. J. Environ. Monit. 3, 74–80. doi:10.1039/b006567f
Honti, M., Gao, C., Istvánovics, V., and Clement, A. (2020). Lessons learnt from the long-term management of a large (re)constructed wetland, the Kis-Balaton protection system (Hungary). Water 12 (3), 659. doi:10.3390/w12030659
Honti, M., and Istvánovics, V. (2011). Online monitoring of phytoplankton light response curves using a novel delayed fluorescence device. Lakes &. Reserv. 16 (2), 153–158. doi:10.1111/j.1440-1770.2011.00458.x
Istvánovics, V., Clement, A., Somlyódy, L., Specziár, A., G.-Tóth, L., and Padisák, J. (2007). Updating water quality targets for shallow Lake Balaton (Hungary), recovering from eutrophication. Hydrobiologia 581 (1), 305–318. doi:10.1007/s10750-006-0509-1
Istvánovics, V., and Honti, M. (2021). Stochastic simulation of phytoplankton biomass using eighteen years of daily data-predictability of phytoplankton growth in a large, shallow lake. Sci. Total Environ. 764, 143636. doi:10.1016/j.scitotenv.2020.143636
Istvánovics, V., Honti, M., Torma, P., and Kousal, J. (2022). Record-setting algal bloom in polymictic Lake Balaton (Hungary): A synergistic impact of climate change and (mis)management. Freshw. Biol. 00, 1091–1106. doi:10.1111/fwb.13903
Istvánovics, V., Osztoics, A., and Honti, M. (2004). Dynamics and ecological significance of daily internal load of phosphorus in shallow Lake Balaton, Hungary. Freshw. Biol. 49 (3), 232–252. doi:10.1111/j.1365-2427.2004.01180.x
James, D. H., Bradbury, H. J., Antler, G., Steiner, Z., Hutchings, A. M., Sun, X., et al. (2021). Assessing sedimentary boundary layer calcium carbonate precipitation and dissolution using the calcium isotopic composition of pore fluids. Front. Earth Sci. (Lausanne). 9, 601194. doi:10.3389/feart.2021.601194
Jancsek-Turóczi, B., Hoffer, A., Nyírő-Kósa, I., and Gelencsér, A. (2013). Sampling and characterization of resuspended and respirable road dust. J. Aerosol Sci. 65, 69–76. doi:10.1016/j.jaerosci.2013.07.006
Jordán, G., Rompaey, A. van, Szilassi, P., Csillag, G., Mannaerts, C., and Woldai, T. (2005). Historical land use changes and their impact on sediment fluxes in the Balaton basin (Hungary). Agric. Ecosyst. Environ. 108 (2), 119–133. doi:10.1016/j.agee.2005.01.013
K.D.T., V. I. Z. I. G. (2017). Nutrient balance of Lake Balaton in 2016 – monitoring and modelling. Székesfehérvár: Central Transdanubian Water Authority. [In Hungarian].
K.D.T., V. I. Z. I. G. (2018). Nutrient balance of Lake Balaton in 2017 – monitoring and modelling. Székesfehérvár: Central Transdanubian Water Authority. [In Hungarian].
K.D.T., V. I. Z. I. G. (2019). Nutrient balance of Lake Balaton in 2018 – monitoring and modelling. Székesfehérvár: Central Transdanubian Water Authority. [In Hungarian].
Kelts, K., and Hsü, K. J. (1978). “Freshwater carbonate sedimentation,” in Lakes – chemistry, geology, physics. Editor A. Lerman (Springer-Verlag New York), 295–323. doi:10.1007/978-1-4757-1152-3_9
Khan, H., Marcé, R., Laas, A., and Obrador Sala, B. (2022). The relevance of pelagic calcification in the global carbon budget of lakes and reservoirs. Limnetica 41, 17–25. doi:10.23818/limn.41.02
Kis, A., Pongrácz, R., and Bartholy, J. (2017). Multi-model analysis of regional dry and wet conditions for the Carpathian Region. Int. J. Climatol. 37 (13), 4543–4560. doi:10.1002/joc.5104
Korponai, J., Varga, K. A., Lengré, T., Papp, I., Tóth, A., and Braun, M. (2011). Paleolimnological reconstruction of the trophic state in Lake Balaton (Hungary) using Cladocera remains. Hydrobiologia 676 (1), 237–248. doi:10.1007/s10750-011-0898-7
Kutics, K. (2019). Evolution of water quality of Lake Balaton. Ecocycles 5 (2), 44–73. doi:10.19040/ecocycles.v5i2.149
Lóczy, L. (1913). A Balaton környékének geológiai képződményei és ezeknek vidékek szerinti telepedése. A Balaton tudományos tanulmányozásának eredményei I 1, 1–618. (in Hungarian).
Lükő, G., Torma, P., Krámer, T., Weidinger, T., Vecenaj, Z., and Grisogono, B. (2020). Observation of wave-driven air–water turbulent momentum exchange in a large but fetch-limited shallow lake. Adv. Sci. Res. 17, 175–182. doi:10.5194/asr-17-175-2020
Mackenzie, F. T., Bihoff, W. D., Bishop, F. C., Loijens, M., Schoonmaker, J., and Wollast, R. (1983). Magnesian calcites: Low temperature occurrence, solubility, and solid solution behavior. Carbonates Mineralogy Chem. I I, 97–144. doi:10.1016/0016-7037(87)90325-5
Máté, F. (1987). Magyar Állami Földtani Intézet évi jelentése az 1985. évről. (Annual report of the Hungarian Geological Institute of 1985. Budapest: Hungarian Geological Institute, 367–379.A Balaton-meder recens üledékeinek térképezése. [Mapping of modern Lake Balaton bottom sediments]
Molnár, Z., Pekker, P., Dódony, I., and Pósfai, M. (2021). Clay minerals affect calcium (magnesium) carbonate precipitation and aging. Earth Planet. Sci. Lett. 567, 116971. doi:10.1016/j.epsl.2021.116971
Müller, G., and Wagner, F. (1978). Holocene carbonate evolution in Lake Balaton (Hungary): A response to climate and impact of man. Modern and ancient lake sediments, 2. Blackwell Scientific Publications, 57–81.
Mutti, M., and Hallock, P. (2003). Carbonate systems along nutrient and temperature gradients: Some sedimentological and geochemical constraints. Int. J. Earth Sci. 92 (4), 465–475. doi:10.1007/s00531-003-0350-y
Nyirő-Kósa, I., Rostási, Á., Bereczk-Tompa, É., Cora, I., Koblar, M., Kovács, A., et al. (2018). Nucleation and growth of Mg-bearing calcite in a shallow, calcareous lake. Earth Planet. Sci. Lett. 496, 20–28. doi:10.1016/j.epsl.2018.05.029
Pálfi, I., Pósfai, M., Kristály, F., Veres, D., Arnaud, F., Szalai, Z., et al. (2021). Geochemical survey of Lake Balaton sediments: Holocene paleoenvironment and paleoclimate. EGU General Assem. 19, 15654.
Palmer, S. C. J., Zlinszky, A., Balzter, H., Nicolás-Perea, V., and Tóth, V. R. (2017). “Copernicus framework for monitoring Lake Balaton phytoplankton,” in Earth observation for land and emergency monitoring. Editor H. Balzter (John Wiley & Sons), 193.
Palmer, S. C., Pelevin, V. V., Goncharenko, I., Kovács, A. W., Zlinszky, A., Présing, M., et al. (2013). Ultraviolet fluorescence LiDAR (UFL) as a measurement tool for water quality parameters in turbid lake conditions. Remote Sens. 5 (9), 4405–4422. doi:10.3390/rs5094405
Parkhurst, D. L., and Appelo, C. A. J. (2013). Description of input and examples for PHREEQC version 3: A computer program for speciation, batch-reaction, one-dimensional transport, and inverse geochemical calculations (No. 6-A43). Reston, VA: US Geological Survey.
Pelevin, V., Zlinszky, A., Khimchenko, E., and Tóth, V. (2017). Ground truth data on chlorophyll-a, chromophoric dissolved organic matter and suspended sediment concentrations in the upper water layer as obtained by LIF lidar at high spatial resolution. Int. J. Remote Sens. 38 (7), 1967–1982. doi:10.1080/01431161.2016.1274446
Plummer, L. N., and Busenberg, E. (1982). The solubilities of calcite, aragonite and vaterite in CO2-H2O solutions between 0 and 90 C°, and an evaluation of the aqueous model for the system CaCO3-CO2-H2O. Geochimica Cosmochimica Acta 46 (6), 1011–1040. doi:10.1016/0016-7037(82)90056-4
Pósfai, M. (2020). A Balaton üledékének ásványai. Földt. Közl. 150 (4), 511. doi:10.23928/foldt.kozl.2020.150.4.511
Putnis, A. (2009). Mineral replacement reactions. Rev. Mineral. Geochem. 70, 87–124. doi:10.2138/rmg.2009.70.3
Rákóczi, L. (1987). A tavi üledék felkeveredése. Vízügyi Közlemények. 69 (1), 86–101. (in Hungarian).
Reeder, R. J., and Sheppard, C. E. (1984). Variation of lattice parameters in some sedimentary dolomites. Am. Mineralogist 69/5, 6 520–527.
Reimers, C. E., Ruttenberg, K. C., Canfield, D. E., Christiansen, M. B., and Martin, J. B. (1996). Porewater pH and authigenic phases formed in the uppermost sediments of the Santa Barbara Basin. Geochim. Cosmochim. Acta 60, 4037–4057. doi:10.1016/s0016-7037(96)00231-1
Rostási, Á., Topa, B. A., Weiszburg, G. T., Gresina, F., Gelencsér, A., and Varga, G. (2022). Saharan dust deposition in Central Europe in 2016 - A representative year of the increased North African dust removal over the last decade. Front. Earth Sci. (Lausanne). 10, 1. doi:10.3389/feart.2022.869902
Ruiz-Agudo, E., Putnis, C. V., and Putnis, A. (2014). Coupled dissolution and precipitation at mineral-fluid interfaces. Chem. Geol. 383, 132–146. doi:10.1016/j.chemgeo.2014.06.007
Somlyódy, L., and Honti, M. (2005). The case of Lake Balaton: How can we exercise precaution? Water Sci. Technol. 52 (6), 195–203. doi:10.2166/wst.2005.0168
Stein, A. F., Draxler, R. R., Rolph, G. D., Stunder, B. J. B., Cohen, M. D., and Ngan, F. (2015). NOAA’s HYSPLIT atmospheric transport and dispersion modeling system. Bull. Am. Meteorol. Soc. 96, 2059–2077. doi:10.1175/BAMS-D-14-00110.1
Szilágyi, F. (2005). A vízpótlás hatása a Balaton és a Zala vizének ionösszetételére. Vízügyi Közlemények 87, 145–166. (in Hungarian).
Talbot, M. R. (1990). A review of the palaeohydrological interpretation of carbon and oxygen isotopic ratios in primary lacustrine carbonates. Chem. Geol. 80 (4), 261–279. doi:10.1016/0168-9622(90)90009-2
Tátrai, I., Mátyás, K., Korponai, J., Paulovits, G., and Pomogyi, P. (2000). The role of the Kis-Balaton Water Protection System in the control of water quality of Lake Balaton. Ecol. Eng. 16 (1), 73–78. doi:10.1016/S0925-8574(00)00091-4
Tompa, É., Nyirő-Kósa, I., Rostási, Á., Cserny, T., and Pósfai, M. (2014). Distribution and composition of Mg-calcite and dolomite in the water and sediments of Lake Balaton. Cent. Eur. Geol. 57, 113–136. doi:10.1556/CEuGeol.57.2014.2.1
Torma, P., and Wu, C. (2019). Temperature and circulation dynamics in a small and shallow lake: Effects of weak stratification and littoral submerged macrophytes. Water 11 (1), 128. doi:10.3390/w11010128
Treitz, P. (1911). “A Balaton tó fenéki iszapjának és altalajának fizikai alakulása és ásványtani összetétele. A Balatonnak és környékének fizikai földrajza,”. A Balaton tudományos tanulmányozásának eredményei. Magyar Királyi Földrajzi Társaság Balaton-bizottsága. Editor L. Lóczy (Budapest, I. VI. 1–26 (in Hungarian).
Tucker, M. E., and Wright, V. P. (1990). Carbonate sedimentology. Blackwell Scientific Publication, 482. doi:10.1002/9781444314175
Tullner, T. (2002). Changes in the water level of Lake Balaton on the basis of GIS processing of its geoscientific database, PhD Thesis. Budapest: Eötvös Loránd University, Department of Geology, 142.
Tullner, T., and Cserny, T. (2003). New aspects of lake-level changes: Lake Balaton, Hungary. Acta Geol. Hung. 46, 215–238. doi:10.1556/ageol.46.2003.2.8
van Straten, G., Jolánkai, G., and Herodek, S. (1979). Review and evaluation of research on the eutrophication of Lake Balaton--A background report for modeling. Austria: International Institute for Applied Systems Analysis: Laxenburg, 110.
Varga, G. (2020). Changing nature of Saharan dust deposition in the Carpathian Basin (Central Europe): 40 years of identified North African dust events (1979–2018). Environ. Int. 139, 105712. doi:10.1016/j.envint.2020.105712
Varga, G., Dagsson-Walhauserová, P., Gresina, F., and Helgadottir, A. (2021). Saharan dust and giant quartz particle transport towards Iceland. Sci. Rep. 11 (1), 11891. doi:10.1038/s41598-021-91481-z
Varga, G., Kovács, J., and Újvári, G. (2013). Analysis of Saharan dust intrusions into the Carpathian Basin (Central Europe) over the period of 1979–2011. Glob. Planet. Change 100, 333–342. doi:10.1016/j.gloplacha.2012.11.007
Visnovitz, F., Bodnár, T., Tóth, Z., Spiess, V., Kudó, I., Timár, G., et al. (2015a). Seismic expressions of shallow gas in the lacustrine deposits of Lake Balaton, Hungary. Near Surf. Geophys. 13 (5), 433–447. doi:10.3997/1873-0604.2015026
Visnovitz, F., Horváth, F., Fekete, N., and Spiess, V. (2015b). Strike-slip tectonics in the Pannonian basin based on seismic surveys at Lake Balaton. Int. J. Earth Sci. 104 (8), 2273–2285. doi:10.1007/s00531-015-1179-x
Visnovitz, F., Jakab, B., Czecze, B., Hámori, Z., Székely, B., Fodor, L., et al. (2021). High resolution architecture of neotectonic fault zones and post-8-Ma deformations in Western Hungary: Observations and neotectonic characteristics of the fault zone at the Eastern Lake Balaton. Glob. Planet. Change 203, 103540. doi:10.1016/j.gloplacha.2021.103540
Zhang, F., Xu, H., Konishi, H., and Roden, E. E. (2010). A relationship between d 104 value and composition in the calcite-disordered dolomite solid-solution series. Am. Mineralogist 95, 1650–1656. doi:10.2138/am.2010.3414
Keywords: carbonate precipitation, lake sediment, Lake Balaton, mineral budget, sedimentation rate
Citation: Rostási Á, Rácz K, Fodor MA, Topa B, Molnár Z, Weiszburg TG and Pósfai M (2022) Pathways of carbonate sediment accumulation in a large, shallow lake. Front. Earth Sci. 10:1067105. doi: 10.3389/feart.2022.1067105
Received: 11 October 2022; Accepted: 30 November 2022;
Published: 20 December 2022.
Edited by:
Shailesh Kumar Singh, National Institute of Water and Atmospheric Research (NIWA), New ZealandReviewed by:
Huifang Xu, University of Wisconsin-Madison, United StatesJános Haas, Eötvös Loránd University, Hungary
Copyright © 2022 Rostási, Rácz, Fodor, Topa, Molnár, Weiszburg and Pósfai. This is an open-access article distributed under the terms of the Creative Commons Attribution License (CC BY). The use, distribution or reproduction in other forums is permitted, provided the original author(s) and the copyright owner(s) are credited and that the original publication in this journal is cited, in accordance with accepted academic practice. No use, distribution or reproduction is permitted which does not comply with these terms.
*Correspondence: Ágnes Rostási, rostasi.agnes@mk.uni-pannon.hu