- 1Department of Earth and Planetary Sciences, Mechanical Engineering and Materials Science, Yale Institute for Biospheric Studies, Yale University, New Haven, CT, United States
- 2Department of Geology and Geophysics, University of Utah, Salt Lake City, UT, United States
- 3Sensing and Perception Lab, General Robotics, Automation, Electrical and Systems Engineering, University of Pennsylvania, Philadelphia, PA, United States
The fossil record represents the world’s largest historical dataset of biodiversity. However, the biomechanical and ecological potential of this dataset has been restricted by various unique barriers obstructing experimental study. Fossils are often partial, modified by taphonomy, or lacking modern analogs. In the past, these barriers confined many studies to descriptive and observational techniques. Fortunately, advances in computer modeling, virtual simulations, model fabrication, and physical experimentation now allow ancient organisms and their biomechanics to be studied like never before using “Defossilized Organismal Proxies” (DOPs). Although DOPs are forging new approaches integrating ecology, evolutionary biology, and bioinspired engineering, their application has yet to be identified as a unique, independent methodological approach. We believe that techniques involving DOPs will continue revolutionizing paleontology and how other related fields interact with and draw insights from life’s evolutionary history. As the field of paleontology moves forward, identifying the framework for this novel methodological development is essential to establishing best practices that maximize the scientific impact of DOP-based experiments. In this perspective, we reflect on current literature innovating the field using DOPs and establish a workflow explaining the processes of model formulation, construction, and validation. Furthermore, we present the application of DOP-based techniques for non-specialists and specialists alike. Accelerating technological advances and experimental approaches present a host of new opportunities to study extinct organisms. This expanding frontier of paleontological research will provide a more holistic view of ecology, evolution, and natural selection by breathing new life into the fossil record.
Introduction
Organisms have adapted to constantly changing environments throughout the earth’s history. These changes are documented in the fossil record, providing a critical deep-time context for the evolution and structure of modern ecosystems and organisms. Beyond paleontology, fossils give macroevolutionary context to interrelated fields like biology, ecology, and bioinspired engineering (Takita et al., 2003; Roberts et al., 2011; Fukuoka and Akama, 2014; Park et al., 2014). Yet distinct challenges are associated with studying the functional morphology of organisms whose behavior cannot be directly observed. Paleontologists have continuously developed new techniques to explore past life at a level that more closely matches our understanding of modern organisms. Early studies prioritized describing novel, extinct organisms and constructing their evolutionary framework (e.g., Cope, 1878; Sherwood Romer, 1968) using environmental information and modern analogs to generate ecological hypotheses. Later, functional morphologists analyzed the potential function of fossil morphologies. As the number of described fossil taxa and individuals increased, investigators created quantitative approaches to study evolution, extinction, and ecological trends through morphological change (Parrington and Parrington, 1977; Holmes, 1989; Vermeij, 1993; Hutchinson and Garcia, 2002). Today, the study of form and function is transforming again, as computational defossilization creates new means to study organisms previously rendered static by fossilization experimentally. We define computational defossilization as the process of resurrecting fossils as virtual and/or physical proxies of once-living organisms’ morphologies. We refer to these virtual and physical models as Defossilized Organismal Proxies (DOPs). Rapidly developing 3D printing and computational modeling technologies have helped DOPs flourish, bringing investigators closer than ever to subjecting the morphologies of extinct organisms to biomechanical tests akin to those conducted on extant organisms (Johnson and Carter, 2019; Pandolfi et al., 2020). However, as the experimental design using DOPs is rooted in techniques from fields in which, classically, many paleontologists do not have foundational training, paleontologists must apply these technologies meaningfully by ensuring an understanding of experimental inputs, assumptions, and limitations.
We, the authors, have broad interests in using the process of computational defossilization for biomechanical experiments, primarily applying these techniques to physical experiments regarding functional morphology and its implications for macroevolution and paleoecology. While individually, we focus on disparate topics and taxa, we have identified fundamental commonalities in our experimental goals and approaches. Going forward, we anticipate that paleontology will emphasize a broadened understanding of how form and function are related to interactions between organisms (e.g., predator-prey interactions) and across complex anatomical systems of single organisms (e.g., interactions between the spine and limbs during locomotion). Analyzing such biomechanical interactions will require physical models that can accommodate complex morphologies and the chaotic circumstances of real-world environments. In this perspective, we synthesize a generalized experimental workflow, highlight methodological limitations, and suggest best practices to make biomechanical DOP-based experiments repeatable and meaningful in a broader evolutionary context. Lastly, we discuss future directions for the field to develop the skills needed for increasingly interdisciplinary experimentation for macroevolutionary research using DOPs.
Experimental design
The first step in designing a DOP-based experiment is identifying an ecological or evolutionary hypothesis that would benefit from empirical testing. Often, these are long-standing hypotheses from the literature that propose drivers of macroevolution or the function of morphological adaptations. However, investigators must first strategically narrow the vast scale of macroevolutionary hypotheses to questions with scopes that can be addressed empirically (Table 1). The resulting empirical scope often targets aspects of morphology that can be isolated. While the technology to create DOPs has existed for many years (Cunningham et al., 2014; Pandolfi et al., 2020), many researchers within the field have not yet adopted computational defossilization in combination with physical experiments to establish fundamental, empirical relationships between form and function (i.e., first-order approximations of functional morphology). Investigators can subsequently incorporate new levels of complexity to systematically probe models increasingly similar to taxa of interest (Figure 1).
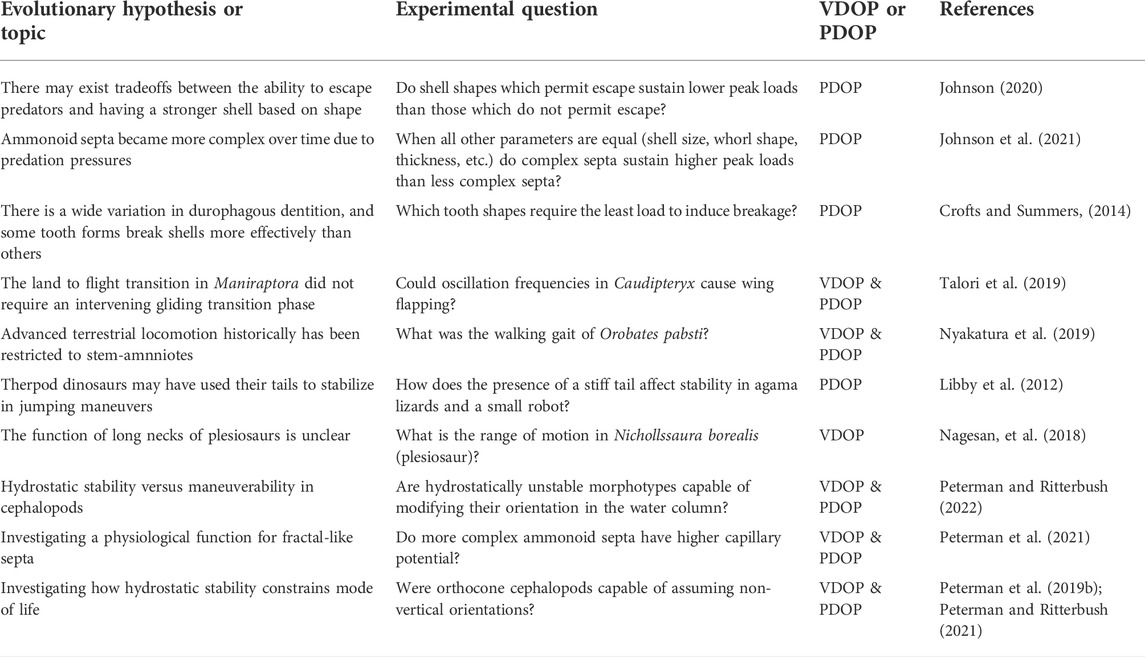
TABLE 1. Examples of macroevolutionary hypotheses and topics that have been addressed using DOPs. Each topic must be narrowed to an appropriate scope for an experimental question before a VDOP or PDOP can be used.
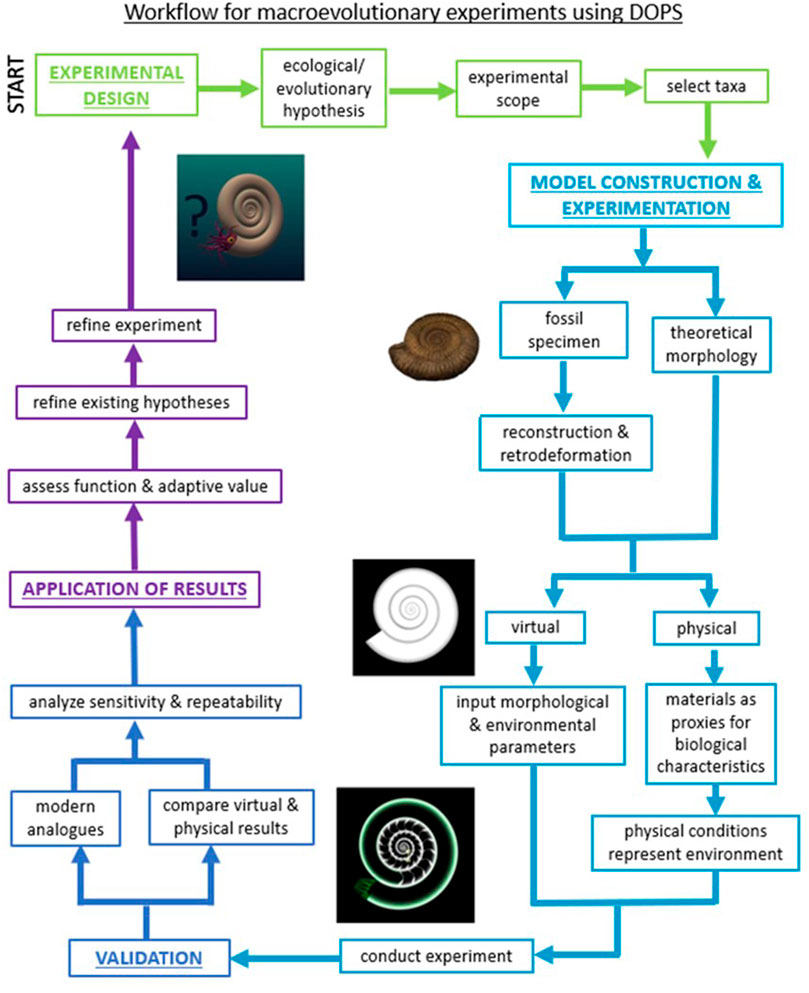
FIGURE 1. Generalized workflow for using DOPs to study macroevolutionary hypotheses showing steps to experimental design, model construction, experimentation, validation, and application of results. The workflow is iterative by design, allowing for models and experiments to be refined as new results are generated.
The limitations of taphonomy and taxonomy for a chosen fossil group dictate the morphological assumptions needed to create a DOP, thus, informing whether virtual models are sufficient or if physical models are required. Investigators must consider taphonomic variation across taxa, morphologies, fossil completeness, levels of taxonomic identification, and sampling frequency (Tarver et al., 2007; Starrfelt and Liow, 2016). These limitations influence the level of abstraction needed for a DOP, affecting whether a DOP is intended to demonstrate strict mimicry or some level of inspiration from fossils. For example, in cases of exceptional preservation of morphology, direct mimicry from a specimen may be more achievable (Perricone et al., 2022). In contrast, fossils that exhibit greater taphonomic distortion require a larger level of abstraction to fill morphological gaps. Investigators, therefore, may instead aim to create a DOP inspired to probe unique morphological features that unite a taxon in its biomechanical applications. We will expand upon these concepts in the following sections.
Model construction and experimentation
The goal of a DOP is to represent the living animal and/or the critical parts of its morphology for experimentation. Sometimes this requires reconstruction and/or retrodeformation while a DOP is virtual (VDOP) (Srivastava and Shah, 2006; Molnar et al., 2012; Tallman et al., 2014; Johnson et al., 2017; Pohle and Klug, 2018; Schlager et al., 2018). Investigators can mitigate taphonomic distortion by assuming symmetry to complete a partial specimen (Schlager et al., 2018), using modern analogs as guides (Molnar et al., 2012; Tallman et al., 2014), forming composites from multiple individuals (Ibrahim et al., 2014; Peterman et al., 2020b), or reconstructing morphologies mathematically (Raup, 1967; Peterman et al., 2020a; Peterman et al., 2020c; Moron Alfonso et al., 2020; Chirat et al., 2021).
Mathematical reconstruction is uniquely valuable for creating theoretical morphologies that “simplify” characters and isolate morphological variables of interest (Johnson, 2020). These models can serve as a foundation for increasingly complex models, modifying a few morphological characters at a time. In addition to avoiding taphonomic distortion altogether, theoretical morphologies help compare the form and function of non-existent morphologies to realized (extant and extinct) morphologies (Hebdon et al., 2020b; Johnson, 2020). Testing non-existent morphologies can reveal evolutionary, functional, and sometimes developmental constraints on ancient and extant forms (Raup, 1966). In any case, one must recognize that all abstractions (e.g., morphology, biological material properties, size) made to create a DOP for biomechanical experiments will likely influence results. Therefore, choices made in retrodeformation and reconstruction must be clearly outlined in publications so that future investigators may make meaningful comparisons to their work and understand precisely how virtual components were constructed (mesh size, density, smoothing, etc.) so that they may replicate methods.
There are many determining factors for choosing to use a VDOP, a PDOP, or both. Experiments on VDOPs can often be cost-effective as many iterations of an experiment may be run while changing single parameters (e.g., kinematic studies, multi-body dynamic analysis, computational fluid dynamics) (Sellers et al., 2009; Hebdon et al., 2020b; Jones et al., 2021). Virtual experiments are also valuable tests of expected outcomes for further physical experiments or settings that are not feasible for a physical laboratory setting (e.g., those which require extremely large spaces or amounts of materials) (Díez Díaz et al., 2020). For example, investigators have performed hydrostatic simulations to determine the buoyancy and mass distributions of organisms submerged in water (Peterman et al., 2019a). Others have performed computational fluid dynamics (CFD) simulations on similar models to determine aero- and hydrodynamic drag, lift, and movement capabilities (Rahman, 2017; Hebdon et al., 2020a; Hebdon et al., 2020b). VDOPs have also been used to study structural resistance to mechanical stress (Lemanis et al., 2016; Lemanis and Zlotnikov, 2018; Lemanis, 2020), bite force (Rayfield, 2007; Walmsley et al., 2013; Cox et al., 2015), and other properties with various finite element analysis (FEA) utilities (Bright, 2014). Additionally, the mechanics of movement have been derived from VDOPs (Snively et al., 2013; Nyakatura et al., 2015; Sellers et al., 2017; Bishop et al., 2018; Bishop et al., 2021).
However, virtual analyses remain computationally limited in several important areas of biomechanical study, including modeling fracture, material properties of soft tissues, ground reaction forces, and the mechanics of locomotion on soft substrates. Additionally, the properties of many biological materials are still unknown, requiring investigators to assume similarity to taxa whose material properties are known (which may significantly change results). Since virtual studies are highly dependent on chosen inputs and assumptions, it is crucial that investigators clearly state these choices in their methods. While methodological transparency may seem like an obvious scientific caveat, it is of particular importance for paleontological studies, which often draw conclusions from relative comparisons rather than the absolute magnitudes of simulated results to account for necessary estimations (e.g., bulk material properties) (Tseng et al., 2011).
Finally, it is imperative to thoroughly explain virtual methodologies because not knowing what lies behind prefabricated programs can lead to mistakes in choosing inputs or interpreting results. It is also essential to understand how researchers in other fields use tools that paleontologists have adopted (e.g., FEA, MBDA from engineering). For example, finite element methods have become common practice as complete paleontological studies examining the morphologies of ancient organisms. However, in our experience in engineering labs, virtual or mathematical models are often a first step used to approximate the outcome of a physical model, which researchers will build subsequently. The virtual model does not stand alone; instead, it serves to help the investigator detect areas of weakness in their design, anticipate possible outcomes, and troubleshoot and validate ideas that researchers will put into practice physically. Thus, we suggest that using physical DOPs (PDOPs) creates a valuable complement and point of validation for existing VDOPs.
Experiments with PDOPs incorporate real-world variability unaccounted for in virtual settings. Recent advances in the fabrication of physical models, most notably 3D printing, have created direct conversions of virtual models to physical ones (Long et al., 2006; Mcinroe et al., 2016; Talori et al., 2018; Johnson and Carter, 2019; Nyakatura et al., 2019; Talori et al., 2019; Ibrahim et al., 2020; Johnson et al., 2021). Now, in addition to performing experiments on casts or physical approximations of specimens (Chamberlain, 1976; Jacobs, 1992; Schulp, 2005; Whitenack and Herbert, 2015), more intricate morphologies can be created at high levels of detailed combinations for uses spanning robotic gait reconstruction (Nyakatura et al., 2019; Ibrahim et al., 2020), compression experiments (Johnson, 2020; Johnson et al., 2021), hydrostatic balancing (Peterman et al., 2020d) and dynamic fluid flow experiments (Peterman and Ritterbush, 2021). These experiments may not always reflect absolute physical properties or behaviors; however, they provide valuable insight from relative comparisons of results between different morphologies using materials with similar bulk properties (Johnson et al., 2021). Even the most fundamental syn vivo capabilities have not yet been determined for many extinct organisms, especially those lacking clear modern analogs. Again, this illustrates the importance of defining an appropriate scope for DOP-based experiments for meaningful interpretation and validation.
Validation
The inherent challenges of working with fossils necessitate methods to confirm that experimental design accurately captures modeled behavior and does not reflect experimental conditions alone. DOPs can be validated in multiple ways:
1) Researchers can conduct sensitivity analyses during the experimental design process. Sensitivity analysis entails systematically changing parameters to determine effects on results (Tseng et al., 2011; Bijlert et al., 2021; Bishop et al., 2021) and demonstrate methodological constraints. Such tests are often used to validate VDOP studies and to tune parameters over many iterations. In contrast, PDOP studies often require a priori decisions, which may affect sensitive parameters.
2) Once an experiment is complete, one can demonstrate that an experimental design produces results within a range generated using extant taxa with similar material properties, morphologies, or behaviors (Sellers et al., 2009; Pierce et al., 2012; Nagesan et al., 2018; Talori et al., 2019; Ibrahim et al., 2020). However, there are biases in this approach: similar structures may not be functionally or environmentally homologous when organisms are considered in their paleoecological context (Pierce et al., 2013). Some paleontologists can alleviate this by using recently-extinct taxa or taxa with living descendants (Dzemski and Christian, 2007). In cases without relevant modern analogs, validation can be conducted using different taxa from various phylogenetic backgrounds. The farther apart the modern taxa are phylogenetically, the less likely a method will capture data present due to homoplasy alone. Given these constraints, many investigators also benefit from cross-methodological validation.
3) Comparing VDOPs and PDOPs can ground a virtual model in real-world conditions (Nyakatura et al., 2019; Ijspeert, 2020). Using VDOPs and PDOPs in conjunction reduces the chance that experimental design choices dominate results. PDOPs help constrain and assess the real-world plausibility of a simulation using a VDOP. For example, cross-method validation has been used in reconstructing walking gaits in a stem-amniote and flapping motion in an early bird (Nyakatura et al., 2019; Talori et al., 2019). A VDOP model predicted accepted results, and physical experiments validated virtual results in both cases. VDOPs and PDOPs have also been used in concert to investigate liquid retention in extinct cephalopods (Peterman et al., 2021).
Experiments using DOPs are frequently interdisciplinary, generating results that investigators can apply across ecology, evolution, engineering, paleontology, and other fields in several ways: 1) Collecting empirical data allows investigators to assess the potential function of morphology and hypothesize about its adaptive value, if any, exists. For example, DOPs can be used to evaluate morphological tradeoffs between different roles, like defense and locomotion (Johnson, 2020) or stability and maneuverability (Peterman and Ritterbush, 2022). Functional experimental studies are also valuable to modern animal behaviorists interested in evolutionary processes governing behaviors (Hsieh and Plotnick, 2020). Understanding the behaviors of extinct organisms also expands the range of taxa that can be used for bioinspired design in engineering (Perricone et al., 2022; Tamborini, 2022). 2) DOP-based studies are useful for refining existing deep-time eco-evolutionary hypotheses previously understood primarily from description. Incorporating empirical data sharpens our understanding of existing macroevolutionary hypotheses. 3) Finally, the results of experiments using DOPs are highly informative for future experimental design. DOPs aid in establishing fundamental, first-order approximations of form and function. Thus, DOP-based experiments create a foundation for future work that can incorporate new levels of complexity once a framework has been developed. These more detailed models will further aid macroevolutionary studies that contextualize how past life was influenced by changing environmental and ecological conditions. Understanding long-term evolutionary patterns, in turn, informs the advancement of studies of modern life and ecosystems.
Future directions and synthesis
In this perspective, we have discussed a generalized workflow for the effective use of DOPs in paleontology studies. For the first time, we can understand extinct taxa with the same rigor as their extant counterparts. This new scientific horizon will revolutionize how the fossil record is utilized across many fields. As paleobiology embarks on this journey, it is essential to consider how to approach this venture meaningfully.
Recently, there has been increasing emphasis on the applications of the fossil record for paleomimetics and paleo-bioinspired design. Mimetic approaches are appropriate for species-specific questions (i.e., Nyakatura et al., 2019; Perricone et al., 2022). However, for questions encompassing generalized forms relevant to multiple taxa (e.g., macroevolutionary inquiry aligned), abstracted models using paleo-bioinspiration (McInroe et al., 2016) are more valuable. Abstracted models can incorporate parameters tuned to match a host of animals (i.e., a test bed). As paleontology is further integrated into studies in related fields like robotics and engineering, investigators should carefully consider the significant differences between these approaches and ensure that their choices match their questions. Furthermore, it is essential to correctly interpret the results from either type of model and ensure other fields understand the evolutionary context of the results from any DOP-based study.
While related fields are becoming increasingly aware of the value of understanding the history of past life, macroevolution often remains misused as a means to support the development of bioinspired work. For example, the previously common misconception that modern animals are the result of many iterations of failure and improvement in morphology over geologic time (i.e., evolution is an optimizing process) has mainly been mitigated (Flammang, B.E., 2022). However, it remains challenging to interpret a DOP-based result without a robust paleontological background to understand a taxon within its geologic framework (time, environment, ecological composition). The biotic and abiotic conditions that lead to the evolution of extant taxa have changed over time (e.g., environments change, predators are introduced, and gene duplication events occur). Extinct animals were sufficient for life during their given time periods. While scientists have the power of retrospection, evolution does not. Without understanding the broader evolutionary and geologic context for functional morphology, investigators in other fields may employ tests that do not capture their aims or misinterpret paleontological trends.
Lastly, as paleontologists continue to embrace rapidly developing technologies, we must ensure our scientists are trained to utilize these advancements to their full potential. Paleontologists are traditionally heavily trained in geology and biology; however, it is becoming increasingly apparent that having training in areas like engineering, manufacturing, and mathematics enables a host of new PDOP-based experiments. Not only are those skills practical within the field, but also being trained in these areas also has the potential to attract and retain more diverse paleontologists from a wide range of backgrounds and interests. By utilizing skills from other fields and drawing members from these fields, paleontology can recruit and retain more diverse scientists (which has been a historical challenge (Carter, et al., 2022)). Paleontology has always had one foot in the past and one foot in the present. However, with modern technologies and DOP-based approaches, we can now make good on the adage: “What’s past is prologue” and take a step into the future. It will require reassessing our model types and changing how we teach our students, but this is doable for a field that has taken on the challenge of investigating life from its beginning.
Data availability statement
The original contributions presented in the study are included in the article/supplementary material, further inquiries can be directed to the corresponding author.
Author contributions
EJ, DP, and AC wrote the manuscript and created the table and figure.
Funding
This project was supported by the Gaylord Donnelley Postdoctoral Environmental Fellowship (Yale Institute for Biospheric Studies (EJ), the National Science Foundation through award EAR-2052663 (EJ), the National Science Foundation through award EAR-1952756 (DP), and the University of Pennsylvania Vice Provost of Diversity Fellowship (AC).
Conflict of interest
The authors declare that the research was conducted in the absence of any commercial or financial relationships that could be construed as a potential conflict of interest.
Publisher’s note
All claims expressed in this article are solely those of the authors and do not necessarily represent those of their affiliated organizations, or those of the publisher, the editors and the reviewers. Any product that may be evaluated in this article, or claim that may be made by its manufacturer, is not guaranteed or endorsed by the publisher.
References
Bijlert, P. A. V., Soest, A. J. K. V., and Schulp, A. S. (2021). Natural frequency method : Estimating the preferred walking speed of tyrannosaurus rex based on tail natural frequency. R. Soc. open Sci. 8, 201441. doi:10.1098/rsos.201441
Bishop, P. J., Hocknull, S. A., Clemente, C. J., Hutchinson, J. R., Barrett, R. S., and Lloyd, D. G. (2018). Cancellous bone and theropod dinosaur locomotion. Part II—a new approach to inferring posture and locomotor biomechanics in extinct tetrapod vertebrates. PeerJ 6, e5779. doi:10.7717/peerj.5779
Bishop, P. J., Cuff, A. R., and Hutchinson, J. R. (2021). How to build a dinosaur: Musculoskeletal modeling and simulation of locomotor biomechanics in extinct animals. Paleobiology 47 (1), 1–38. doi:10.1017/pab.2020.46
Bright, J. A. (2014). A review of paleontological finite element models and their validity. J. Paleontol. 88 (4), 760–769. doi:10.1666/13-090
Carter, A. M., Johnson, E. H., and Schroeter, E. R. (2022). Long-term Retention of diverse paleontologists requires increasing accessibility. Front. Ecol. Evol. 595. doi:10.3389/fevo.2022.876906
Chamberlain, J. A. (1976). Flow patterns and drag coefficient of cephalopod shells. Palaeontology 19, 539–563.
Chirat, R., Goriely, A., and Moulton, D. E. (2021). The physical basis of mollusk shell chiral coiling. Proc. Natl. Acad. Sci. U. S. A. 118 (48), e2109210118. doi:10.1073/pnas.2109210118
Cope, E. D. (1878). Descriptions of extinct batrachia and reptilia from the permian formation of Texas. Proc. Am. philosophical Soc. 17 (101), 505–530. doi:10.5962/bhl.title.104569
Cox, P. G., Rinderknecht, A., and Blanco, R. E. (2015). Predicting bite force and cranial biomechanics in the largest fossil rodent using finite element analysis. J. Anat. 226 (3), 215–223. doi:10.1111/joa.12282
Crofts, S. B., and Summers, A. P. (2014). How to best smash a snail: The effect of tooth shape on crushing load. J. R. Soc. Interface 11 (92), 20131053. doi:10.1098/rsif.2013.1053
Cunningham, J. A., Rahman, I. A., Lautenschlager, S., Rayfield, E. J., and Donoghue, P. C. (2014). A virtual world of paleontology. Trends Ecol. Evol. 29 (6), 347–357. doi:10.1016/j.tree.2014.04.004
Díez Díaz, V., Demuth, O. E., Schwarz, D., and Mallison, H. (2020). The tail of the Late Jurassic sauropod Giraffatitan brancai: Digital reconstruction of its epaxial and hypaxial musculature, and implications for tail biomechanics. Front. Earth Sci. (Lausanne). 8, 160. doi:10.3389/feart.2020.00160
Dzemski, G., and Christian, A. (2007). Flexibility along the neck of the ostrich (Struthio camelus) and consequences for the reconstruction of dinosaurs with extreme neck length. J. Morphol. 268 (8), 701–714. doi:10.1002/jmor.10542
Flammang, B. E. (2022). Bioinspired design in research: Evolution as beta-testing. Integr. Comp. Biol., 1–10. doi:10.1093/icb/icac134
Fukuoka, Y., and Akama, J. (2014). Dynamic bipedal walking of a dinosaur-like robot with an extant vertebrate’s nervous system. Robotica 32 (6), 851–865. doi:10.1017/S0263574713001045
Hebdon, N., Ritterbush, K., and Choi, Y. (2020a). Assessing the morphological impacts of ammonoid shell shape through systematic shape variation. Integr. Comp. Biol. 60 (5), 1320–1329. doi:10.1093/icb/icaa067
Hebdon, N., Ritterbush, K., and Choi, Y. (2020b). Computational fluid dynamics modeling of fossil ammonoid shells. Palaeontol. Electron 23, a21. doi:10.26879/956
Holmes, R. (1989). Functional interpretations of the vertebral structure in Paleozoic labyrinthodont amphibians. Hist. Biol. 2 (2), 111–124. doi:10.1080/08912968909386495
Hsieh, S., and Plotnick, R. E. (2020). The representation of animal behaviour in the fossil record. Anim. Behav. 169, 65–80. doi:10.1016/j.anbehav.2020.09.010
Hutchinson, J. R., and Garcia, M. (2002). Tyrannosaurus was not a fast runner. Nature 415 (6875), 349–1021. doi:10.1038/416349b
Ibrahim, N., Sereno, P. C., Dal Sasso, C., Maganuco, S., Fabbri, M., Martill, D. M., et al. (2014). Semiaquatic adaptations in a giant predatory dinosaur. Science 345 (6204), 1613–1616. doi:10.1126/science.1258750
Ibrahim, N., Maganuco, S., Dal Sasso, C., Fabbri, M., Auditore, M., Bindellini, G., et al. (2020). Tail-propelled aquatic locomotion in a theropod dinosaur. Nature 581 (7806), 67–70. doi:10.1038/s41586-020-2190-3
Ijspeert, A. J. (2020). Amphibious and sprawling locomotion: From biology to robotics and back. Annu. Rev. Control Robot. Auton. Syst. 3 (1), 173–193. doi:10.1146/annurev-control-091919-095731
Jacobs, D. K. (1992). Shape, drag, and power in ammonoid swimming. Paleobiology 18 (2), 203–220. doi:10.1017/s009483730001397x
Johnson, E. H., Anderson, B. M., and Allmon, W. D. (2017). What can we learn from all those pieces? Obtaining data on drilling predation from fragmented high-spired gastropod shells. Palaios 32 (5), 271–277. doi:10.2110/palo.2016.088
Johnson, E. H., DiMarco, B. M., Peterman, D. J., Carter, A. M., and Allmon, W. D. (2021). Did shell-crushing predators drive the evolution of ammonoid septal shape? Paleobiology 47 (4), 666–679. doi:10.1017/pab.2021.13
Johnson, E. H. (2020). Experimental tests of bivalve shell shape reveal potential tradeoffs between mechanical and behavioral defenses. Sci. Rep. 10 (1), 1–12. doi:10.1038/s41598-020-76358-x
Johnson, E. H., and Carter, A. M. (2019). Defossilization: A review of 3D printing in experimental paleontology. Front. Ecol. Evol. 7. doi:10.3389/fevo.2019.00430
Jones, K. E., Brocklehurst, R. J., and Pierce, S. E. (2021). AutoBend: An automated approach for estimating intervertebral joint function from bone-only digital models. Integr. Org. Biol. 3 (1), obab026. doi:10.1093/iob/obab026
Lemanis, R. (2020). The ammonite septum is not an adaptation to deep water: Re-evaluating a centuries-old idea. Proc. R. Soc. B 287, 20201919. doi:10.1098/rspb.2020.1919
Lemanis, R., Zachow, S., and Hoffmann, R. (2016). Comparative cephalopod shell strength and the role of septum morphology on stress distribution. PeerJ 4, e2434. doi:10.7717/peerj.2434
Lemanis, R., and Zlotnikov, I. (2018). Finite element analysis as a method to study molluscan shell mechanics. Adv. Eng. Mat. 20 (3), 1700939. doi:10.1002/adem.201700939
Libby, T., Moore, T. Y., Chang-Siu, E., Li, D., Cohen, D. J., Jusufi, A., et al. (2012). Tail-assisted pitch control in lizards, robots and dinosaurs. Nature 481 (7380), 181–184. doi:10.1038/nature10710
Long, J. H., Schumacher, J., Livingston, N., and Kemp, M. (2006). Four flippers or two? Tetrapodal swimming with an aquatic robot. Bioinspir. Biomim. 1 (1), 20–29. doi:10.1088/1748-3182/1/1/003
McInroe, B., Astley, H. C., Gong, C., Kawano, S. M., Schiebel, P. E., Rieser, J. M., et al. (2016). Tail use improves performance on soft substrates in models of early vertebrate land locomotors. Science 353 (6295), 154–158. doi:10.1126/science.aaf0984
Molnar, J. L., Pierce, S. E., and Clack, J. A. (2012). Idealized landmark-based geometric reconstructions of poorly preserved fossil material: A case study of an early tetrapod vertebra.
Moron Alfonso, D. A., Peterman, D. J., Cichowolski, M., Hoffmann, R., and Lemanis, R. E. (2020). Virtual 3D modeling of the ammonoid conch to study its hydrostatic properties.
Nagesan, R. S., Henderson, D. M., and Anderson, J. S. (2018). A method for deducing neck mobility in plesiosaurs, using the exceptionally preserved Nichollssaura borealis. R. Soc. open Sci. 5 (8), 172307. doi:10.1098/rsos.172307
Nyakatura, J. A., Allen, V. R., Lauströer, J., Andikfar, A., Danczak, M., Ullrich, H. J., et al. (2015). A three-dimensional skeletal reconstruction of the stem amniote orobates pabsti (diadectidae): Analyses of body mass, centre of mass position, and joint mobility. PLoS ONE 10 (9). doi:10.1371/journal.pone.0137284
Nyakatura, J. A., Melo, K., Horvat, T., Karakasiliotis, K., Allen, V. R., Andikfar, A., et al. (2019). Reverse-engineering the locomotion of a stem amniote. Nature 565, 351–355. doi:10.1038/s41586-018-0851-2
Pandolfi, L., Raia, P., Fortuny, J., and Rook, L. (2020). Editorial: Evolving virtual and computational paleontology. Front. Earth Sci. (Lausanne). 8, 591813. doi:10.3389/feart.2020.591813
Park, J., Lee, J., Lee, J., Kim, K.-S., and Kim, S. (2014). “Raptor: Fast bipedal running and active tail stabilization,” in Proceeding of the 2014 11th International Conference on Ubiquitous Robots and Ambient Intelligence (URAI), Kuala Lumpur, Malaysia, November 2014 (IEEE), 215.
Parrington, F. R., and Parrington, F. R. P. (1977). Intercentra: A possible functional interpretation.
Perricone, V., Grun, T., Raia, P., and Langella, C. (2022). Paleomimetics: A conceptual framework for a biomimetic design inspired by fossils and evolutionary processes. Biomimetics 7 (3), 89. doi:10.3390/biomimetics7030089
Peterman, D. J., Barton, C. C., and Yacobucci, M. M. (2019a). The hydrostatics of Paleozoic ectocochleate cephalopods (Nautiloidea and Endoceratoidea) with implications for modes of life and early colonization of the pelagic zone. Palaeontol. Electron. 22, 1–29. doi:10.26879/884
Peterman, D. J., Ciampaglio, C. N., Shell, R. C., and Yacobucci, M. M. (2019b). Mode of life and hydrostatic stability of orthoconic ectocochleate cephalopods: Hydrodynamic analyses of restoring moments from 3D printed, neutrally buoyant models. Acta Palaeontol. Pol. 64 (3). doi:10.4202/app.00595.2019
Peterman, D. J., Shell, R., Ciampaglio, C. N., and Yacobucci, M. M. (2020a). Stable hooks: Biomechanics of heteromorph ammonoids with U-shaped body chambers. J. Molluscan Stud. 86 (4), 267–279. doi:10.1093/mollus/eyaa018
Peterman, D. J., Yacobucci, M. M., Larson, N. L., Ciampaglio, C., and Linn, T. (2020b). A method to the madness: Ontogenetic changes in the hydrostatic properties of Didymoceras (Nostoceratidae: Ammonoidea). Paleobiology 46 (2), 237–258. doi:10.1017/pab.2020.14
Peterman, D. J., Mikami, T., and Inoue, S. (2020c). The balancing act of Nipponites mirabilis (Nostoceratidae, Ammonoidea): Managing hydrostatics throughout a complex ontogeny. PLoS One 15 (8), e0235180. doi:10.1371/journal.pone.0235180
Peterman, D. J., Hebdon, N., Ciampaglio, C. N., Yacobucci, M. M., Landman, N. H., and Linn, T. (2020d). Syn vivo hydrostatic and hydrodynamic properties of scaphitid ammonoids from the US Western Interior. Geobios 60, 79–98. doi:10.1016/j.geobios.2020.04.004
Peterman, D. J., Ritterbush, K. A., Ciampaglio, C. N., Johnson, E. H., Inoue, S., Mikami, T., et al. (2021). Buoyancy control in ammonoid cephalopods refined by complex internal shell architecture. Sci. Rep. 11 (1), 1–12. doi:10.1038/s41598-021-87379-5
Peterman, D. J., and Ritterbush, K. A. (2022). Resurrecting extinct cephalopods with biomimetic robots to explore hydrodynamic stability, maneuverability, and physical constraints on life habits. Sci. Rep. 12 (1), 1–16. doi:10.1038/s41598-022-13006-6
Peterman, D. J., and Ritterbush, K. A. (2021). Vertical escape tactics and movement potential of orthoconic cephalopods. PeerJ 9, e11797. doi:10.7717/peerj.11797
Pierce, S. E., Clack, J. A., and Hutchinson, J. R. (2012). Three-dimensional limb joint mobility in the early tetrapod Ichthyostega. Nature 486 (7404), 523–526. doi:10.1038/nature11124
Pierce, S. E., Hutchinson, J. R., and Clack, J. A. (2013). Historical perspectives on the evolution of tetrapodomorph movement. Integr. Comp. Biol. 53 (2), 209–223. doi:10.1093/icb/ict022
Pohle, A., and Klug, C. (2018). Body size of orthoconic cephalopods from the late Silurian and Devonian of the Anti-Atlas (Morocco). Lethaia 51 (1), 126–148. doi:10.1111/let.12234
Rahman, I. A. (2017). Computational fluid dynamics as a tool for testing functional and ecological hypotheses in fossil taxa. Palaeontology 60 (4), 451–459. doi:10.1111/pala.12295
Raup, D. M. (1966). Geometric analysis of shell coiling: General problems. J. paleontology 40 (5), 1178–1190.
Raup, D. M. (1967). Geometric analysis of shell coiling: Coiling in ammonoids. J. Paleontology 41 (1), 43–65.
Rayfield, E. J. (2007). Finite element analysis and understanding the biomechanics and evolution of living and fossil organisms. Annu. Rev. Earth Planet. Sci. 35, 541–576. doi:10.1146/annurev.earth.35.031306.140104
Roberts, B., Lind, R., and Chatterjee, S. (2011). Flight dynamics of a pterosaur-inspired aircraft utilizing a variable-placement vertical tail. Bioinspir. Biomim. 6 (2), 026010. doi:10.1088/1748-3182/6/2/026010
Schlager, S., Profico, A., Vincenzo, F. D., and Manzi, G. (2018). Retrodeformation of fossil specimens based on 3D bilateral semi-landmarks: Implementation in the R package “Morpho”. PLoS ONE 13 (3), e0194073. doi:10.1371/journal.pone.0194073
Schulp, A. S. (2005). Feeding the mechanical mosasaur: What did carinodens eat? Geol. Mijnb. 84 (3), 345–357. doi:10.1017/s0016774600021132
Sellers, W. I., Manning, P. L., Lyson, T., Stevens, K., and Margetts, L. (2009). Virtual palaeontology: Gait reconstruction of extinct vertebrates using high performance computing. Palaeontol. Electron. 12. Retrieved from: http://palaeo-electronica.orghttp//palaeo-electronica.org/2009_3/180/index.html.
Sellers, W. I., Pond, S. B., Brassey, C. A., Manning, P. L., and Bates, K. T. (2017). Investigating the running abilities of Tyrannosaurus rex using stress-constrained multibody dynamic analysis. PeerJ 2017 (7), e3420. doi:10.7717/peerj.3420
Sherwood Romer, A. (1968). Evolution of the Amphibia: The Origin of Terrestrial Vertebrates . I. I. Schmalhausen. Translated from the Russian edition (Moscow, 1964) by Leon Kelso. Keith Stewart Thomson, Ed. Academic Press, New York, 1968. xii + 314 pp., illus. $15. Science 162 (3850), 250–251. doi:10.1126/science.162.3850.250
Snively, E., Cotton, J. R., Ridgely, R., and Witmer, L. M. (2013). Multibody dynamics model of head and neck function in Allosaurus (Dinosauria, Theropoda). Palaeontol. Electron. 16 (2), 21. doi:10.26879/338
Srivastava, D. C., and Shah, J. (2006). Digital method for strain estimation and retrodeformation of bilaterally symmetric fossils. Geol. 34 (7), 593–596. doi:10.1130/g22374.1
Starrfelt, J., and Liow, L. H. (2016). How many dinosaur species were there? Fossil bias and true richness estimated using a Poisson sampling model. Phil. Trans. R. Soc. B 371 (1691), 20150219. doi:10.1098/rstb.2015.0219
Takita, K., Katayama, T., and Hirose, S. (2003). Development of Dinosaur-like Robot TITRUS -Its Dynamics and the Motion Utilizing the Dynamic Effect of the Neck and Tail. IEEE Int. Conf. Intelligent Robots Syst. 1, 607–612. doi:10.1109/iros.2003.1250696
Tallman, M., Amenta, N., Delson, E., Frost, S. R., Ghosh, D., Klukkert, Z. S., et al. (2014). Evaluation of a new method of fossil retrodeformation by algorithmic symmetrization: crania of papionins (Primates, Cercopithecidae) as a test case. PloS One 9 (7), e100833. doi:10.1371/journal.pone.0100833
Talori, Y. S., Liu, Y. F., Zhao, J. S., Sullivan, C., O’Connor, J. K., and Li, Z. H. (2018). Winged forelimbs of the small theropod dinosaur Caudipteryx could have generated small aerodynamic forces during rapid terrestrial locomotion. Sci. Rep. 8 (1), 1–14. doi:10.1038/s41598-018-35966-4
Talori, Y. S., Zhao, J. S., Liu, Y. F., Lu, W. X., Li, Z. H., and O’connor, J. K. (2019). Identification of avian flapping motion from non-volant winged dinosaurs based on modal effective mass analysis. PLoS Comput. Biol. 15 (5). doi:10.1371/journal.pcbi.1006846
Tamborini, M. (2022). A Plea for a New Synthesis: From Twentieth-Century Paleobiology to Twenty-First-Century Paleontology and Back Again. Biology 11 (8), 1120. doi:10.3390/biology11081120
Tarver, J. E., Braddy, S. J., and Benton, M. J. (2007). The effects of sampling bias on Palaeozoic faunas and implications for macroevolutionary studies. Palaeontology 50 (1), 177–184. doi:10.1111/j.1475-4983.2006.00618.x
Tseng, Z. J., Mcnitt-Gray, J. L., Flashner, H., Wang, X., and Enciso, R. (2011). Model sensitivity and use of the comparative finite element method in mammalian jaw mechanics: Mandible performance in the Gray Wolf. PLoS ONE 6 (4), e19171. doi:10.1371/journal.pone.0019171
Vermeij, G. J. (1993). Evolution and escalation: An ecological history of life. Princeton University Press.
Walmsley, C. W., Smits, P. D., Quayle, M. R., McCurry, M. R., Richards, H. S., Oldfield, C. C., et al. (2013). Why the long face? The mechanics of mandibular symphysis proportions in crocodiles. PLoS One 8 (1), e53873. doi:10.1371/journal.pone.0053873
Keywords: functional morphology, experimental paleontology, biomechanics, 3D printing, paleoecology
Citation: Johnson E, Peterman D and Carter A (2022) Updating studies of past life and ancient ecologies using defossilized organismal proxies. Front. Earth Sci. 10:1048662. doi: 10.3389/feart.2022.1048662
Received: 19 September 2022; Accepted: 18 October 2022;
Published: 01 November 2022.
Edited by:
Rodrigo Temp Müller, Federal University of Santa Maria, BrazilReviewed by:
Brian Lee Beatty, New York Institute of Technology, United StatesCopyright © 2022 Johnson, Peterman and Carter. This is an open-access article distributed under the terms of the Creative Commons Attribution License (CC BY). The use, distribution or reproduction in other forums is permitted, provided the original author(s) and the copyright owner(s) are credited and that the original publication in this journal is cited, in accordance with accepted academic practice. No use, distribution or reproduction is permitted which does not comply with these terms.
*Correspondence: Erynn Johnson, ZXJ5bm4uam9obnNvbkB5YWxlLmVkdQ==