- 1Research Institute of Petroleum Exploration and Development Northwest Branch, Lanzhou, China
- 2Northwest Sichuan Gas Mine of PetroChina Southwest Oil and Gas Field Company, Jiangyou, China
The enrichment law of tight sandstone gas in the Upper Triassic Xujiahe Formation in the Sichuan Basin has not been fully revealed. In this study, the controlling effect of source-reservoir assemblage on gas accumulation in the Xujiahe Formation was systematically investigated. The results show that pores and fractures are developed in the tight sandstone reservoirs of the Xujiahe Formation. The main pore types are intragranular dissolved and intergranular pores. The quality of sandstone reservoirs in the Xujiahe Formation is controlled by sedimentation, diagenesis and tectonic processes. Underwater distributary river channels and estuary bars are favorable microfacies for reservoir development, and chlorite cemented facies and dissolution-kaolinite cemented facies are the most favorable diagenetic facies. In addition, the natural gas composition and carbon isotope characteristics of the Xujiahe Formation are significantly different in different intervals of the same gas field and the same interval of adjacent gas fields. This shows that the natural gas has no obvious vertical mixing and lateral migration, and it has the distribution characteristics of “local enrichment”. Then, the natural gas will be preferentially charged in the feldspar lithic sandstone and feldspar quartz sandstone with large thickness and good physical properties by means of short-range migration. According to the research, the hydrocarbon supply capacity of the single layer in each interval is weak, and it leads to the low filling degree of the gas reservoir and the insufficient separation of gas and water. On the whole, four sets of extra-source “lower generation and upper storage” assemblages and two sets of “self-generation and self-storage” source-reservoir assemblages are developed in the Xujiahe Formation. The development scale of natural gas is mainly controlled by the type of source-reservoir combination, and the areas with close source-reservoir contact and high hydrocarbon generation intensity are high-quality reservoir development areas.
1 Introduction
The Upper Triassic Xujiahe Formation in the Sichuan Basin is rich in natural gas resources (Dai et al., 2005; Hu et al., 2014). So far, 32 gas fields and gas-bearing structures have been discovered in the Xujiahe Formation in the Zhongba, Bajiaochang, Chongxi, Guang’an and Hechuan areas. They are mainly distributed in the central and western Sichuan Basin. Therefore, the Xujiahe Formation has become one of the most important natural gas producing layers in the Sichuan Basin. The accumulation law of natural gas in the Xujiahe Formation in the Sichuan Basin has received extensive attention from many scholars (Dai et al., 2009; Dai et al., 2012; Dai, 2014; Wang et al., 2020; Li, 2021). The previous believed that the “sandwich” type reservoir-caprock assemblage of the Xujiahe Formation provided the basic geological conditions for the large-scale accumulation of natural gas. Moreover, it has been widely recognized that “continuous gas reservoirs” are widely developed in the Xujiahe Formation.
In recent years, with the deepening of exploration and development, discontinuous gas reservoirs have been discovered in the Xu 2, Xu 4, and Xu 6 Members, and the gas-water relationship is very complex. The source of the Xujiahe Formation is the Xu 1, Xu 3, and Xu 5 Members. Furthermore, the main controlling factors of hydrocarbon accumulation in continental tight reservoirs are not well understood (Du et al., 2007; Yu et al., 2014; Du et al., 2019). Therefore, the study of the enrichment law of natural gas in the Xujiahe Formation has become a hotspot. In this study, the characteristics of the source-reservoir assemblages of the Xujiahe Formation and their controlling effects on natural gas accumulation were systematically analyzed. This study can provide technical support for the efficient exploration and development of the Xujiahe Formation.
2 Geological background
The Sichuan Basin is a large hydrocarbon-bearing superimposed basin in western China, with an area of about 18 km2 × 104 km2. The structural units in the Sichuan Basin are divided into the Western Sichuan Foreland Depression Area, the Central Sichuan Gentle Fold Area, the Northern Sichuan Thrust Fold Area, the Eastern Sichuan High-steep Tectonic Area, the Southern Sichuan Low-steep Fold Area, and the Southwestern Sichuan Uplift Area (Figure 1) (Dai et al., 2012).
During the Indosinian Movement in the late Triassic, the continuous uplift of the periphery of the Sichuan Basin transformed the basin into an inland lake basin (Dai., 2014). The strata of the Upper Triassic Xujiahe Formation were deposited with huge thick sand and mudstone. The deposition of the Xujiahe Formation is controlled by factors such as multiple provenances, multiple periods of tectonic movements, paleo-erosion surfaces and foreland basins (Du et al., 2019). The sedimentary thickness of the Xujiahe Formation in the western part of the basin is relatively large, up to more than 3,000 m, while that in the northern, eastern and western parts is relatively small, generally about 200 m–300 m. From bottom to top, the Xujiahe Formation is divided into Xu 1–Xu 6 Members (Figure 2). Among them, the Xu 1, Xu 3, and Xu 5 Members are mainly gray-black mudstone, carbonaceous mudstone interbeded with siltstone and thin coal seams; while the Xu 2, Xu 4, and Xu 6 Members are mainly gray-white, gray fine-medium-grained sandstone interbeded with a small amount of black mudstone and thin coal seams (Liao et al., 2014).
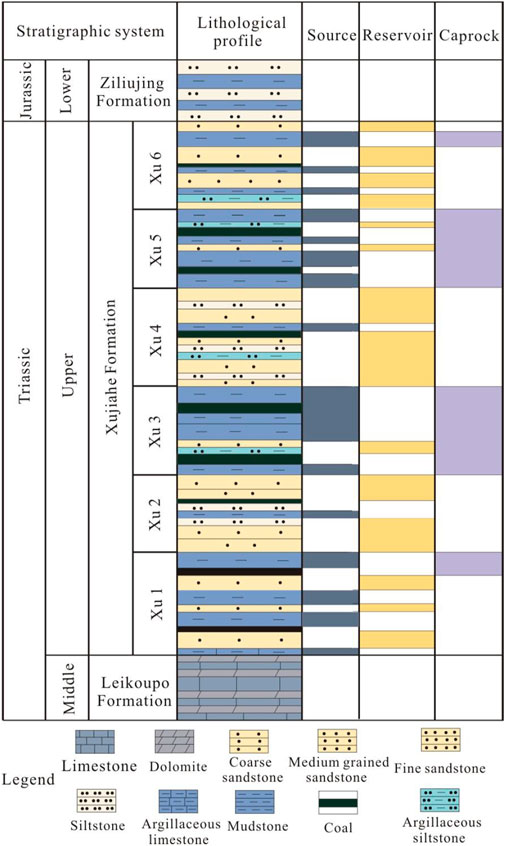
FIGURE 2. Source-reservoir assemblages of the Upper Triassic Xujiahe Formation in the Sichuan Basin.
3 Experiments and methods
In this study, all natural gas samples were taken from the Xujiahe Formation of the Bajiaochang, Chongxi, Nanchong and Guang’an Gas Fields. Also, these samples were taken from the double valve cylinder at the outlet of the wellhead pressure gauge. In order to exclude the influence of external factors on natural gas samples, the samples were taken from normal production wells with a long production time and no chemical reagents have been added recently. A HP6890 gas chromatograph was used for chemical composition analysis, and individual hydrocarbon gas components were separated by capillary column (Plot Al2O3 50*0.53 mm). The gas chromatograph oven temperature was first set at 30°C and held for 10 min, then increased to 180°C at a rate of 10°C/min. In addition, the carbon isotope analysis was performed using a Delta S GC/C/IRMS isotope mass spectrometer. The gas components were separated by a gas chromatograph and then converted into CO2 and injected into the mass spectrometer. The individual alkane gas components (C1-C5) and CO2 were separated by column chromatography (Plot Q 30 m). The column ramp was ramped from 35°C to 80°C (8°C/min ramp rate) to 260°C (5°C/min ramp rate), and the oven temperature was maintained for 10 min at the final temperature. Each sample was analyzed 3 times, and its analytical precision was ± 0.5‰.
The rock thin sections were polished on both sides to a thickness of 0.03 mm. Further, thin sections were identified using a Zeiss Axio Scope A1 polarizing microscope. The cores were cut into cubes of about 1 cm3 for scanning electron microscope observation. An FEI Quanta 450 FEG was used for environmental SEM observations. Its maximum magnification is 1 million times, the working voltage is 20 kV, and the electron beam incident beam current is 4 × 10−10 A.
4 Results
4.1 Source-reservoir combination of extra-source “lower generation and upper storageˮ
The source rocks of the Upper Triassic Xujiahe Formation in the Sichuan Basin are the black shales and thin coal seams in the Xu 1, Xu 3, and Xu 5 Members. Thin mudstone and thin coal beds are also developed in the middle and lower parts of the Xu 2 and Xu 4 Members and the middle and upper parts of the Xu 6 Member. The Xujiahe Formation source rocks in the western Sichuan Basin are thicker, up to 1,000 m, while those in the northeastern and southern Sichuan Basin are thinner, generally less than 50 m–100 m. The organic carbon content of the Xujiahe Formation source rocks generally ranges from 0.5% to 9.7%, with an average of 1.95%. Moreover, the types of organic matter are mainly Type II2 and Type III. The microscopic composition of kerogen is mainly vitrinite, while the content of inertite and chitinite is low, and saprolite is basically absent (Figure 3). The source rocks of the Xu 1, Xu 3, and Xu 5 Members are superimposed with the reservoirs of the Xu 1 (middle part), Xu 2, Xu 4, and Xu 6 Members, and four sets of good source-reservoir assemblages of extra-source “lower generation and upper storage” have been formed. The 4 sets of source-reservoir assemblages include: lower source rock (A1) and middle Xu 1 Member (A2) assemblage, upper Xu 1 Member source rock (A3) and Xu 2 Member reservoir (B1) assemblage, Xu 3 Member hydrocarbons source rock (B2) and Xu 4 Member reservoir (C1) assemblage, Xu 5 Member source rock (C2) and Xu 6 Member reservoir (C3) assemblage (Figure 2).
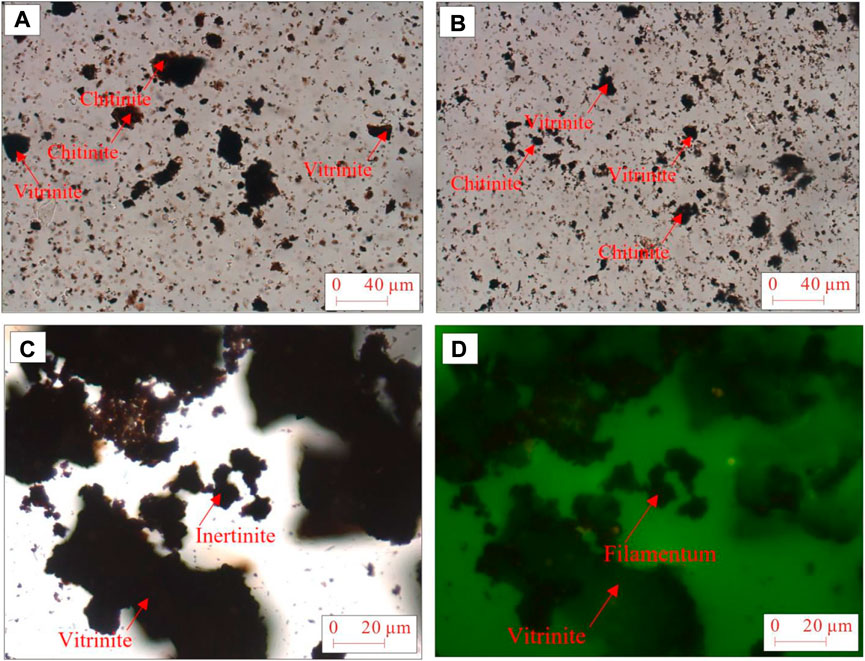
FIGURE 3. Microcomponents of kerogen in source rocks of Xujiahe Formation. (A) Jinhe Profile, Xu 1 Member; (B) Zhanggongling Profile, Xu 5 Member; (C) and (D) Well Jin 31, 3,017.92 m.
The reservoir rock types are mainly fine-medium-coarse-grained lithic feldspar sandstone, feldspar lithic sandstone, and lithic sandstone, followed by lithic quartz sandstone and feldspar quartz sandstone (Figure 4). Among them, the Xu 2 Member mainly develops feldspar quartz sandstone and lithic feldspar sandstone; the Xu 4 Member mainly develops lithic feldspar sandstone and feldspar lithic sandstone, followed by feldspar or lithic quartz sandstone; the Xu 6 Member mainly develops feldspar lithic sandstone, lithic sandstone, and lithic feldspar sandstone. The Xujiahe Formation reservoir rocks have the characteristics of low compositional maturity and high structural maturity, and the compositional maturity index (quartz/feldspar + debris) is generally between 1.5 and 4.0. The difference of maturity is mainly caused by the difference of surrounding provenance. In addition, the compositional maturity of the Xujiahe Formation reservoir rocks shows a downward trend from the Xu 2 to the Xu 6 Members. However, on the plane, the maturity of the reservoir rocks in the central Sichuan area is relatively high, while that in the peripheral areas is relatively low.
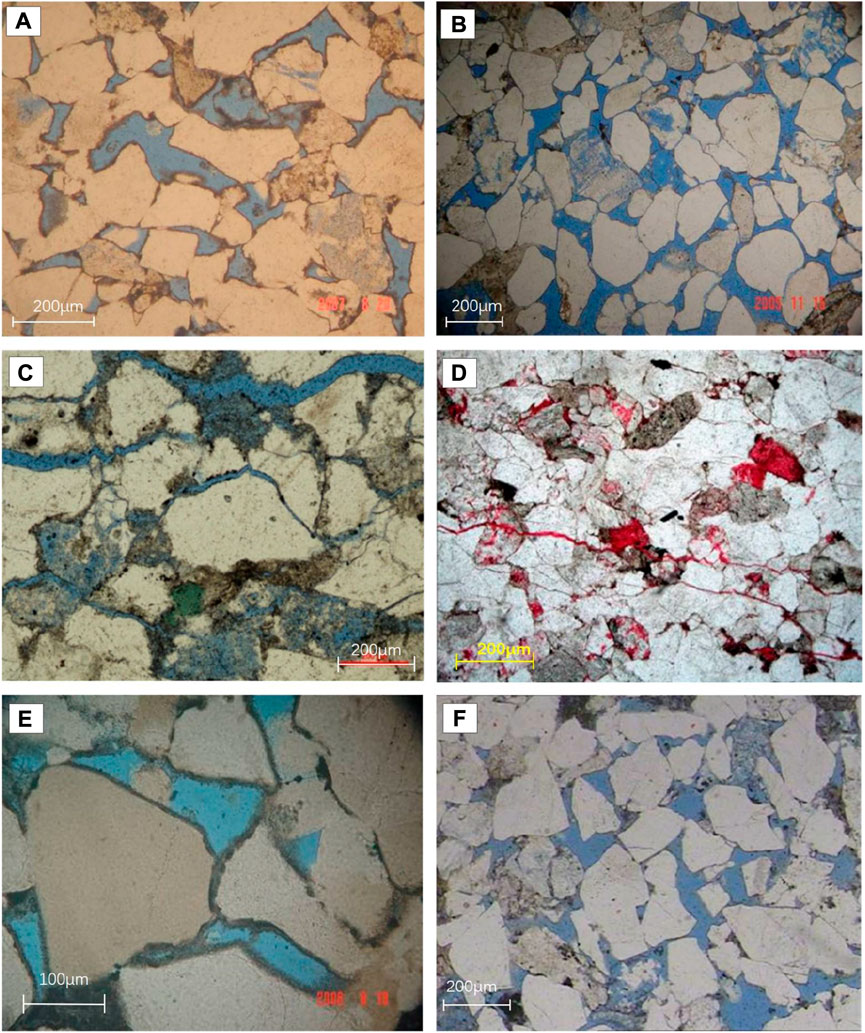
FIGURE 4. Microstructure characteristics of tight sandstones of Xujiahe Formation in the study area. (A) Well Hechuan 7, 2,191.18 m, detrital feldspar sandstone with developed intergranular pores; (B) Well Guang’an 101, 2080.15 m, detrital feldspar sandstone; (C) Well Penglai 11, 2,360.54 m, feldspar detrital sandstone; (D) Well Songhua 2, 3,595.19 m, detrital sandstone; (E) Well Guang’an 128, 2,337.45 m, detrital quartz sandstone; (F) Well Tong 6, 2,449.1 m, feldspar quartz sandstone.
4.2 In-source “self-generation and self-storage” source-reservoir combination
For a long time, the natural gas exploration of the Upper Triassic Xujiahe Formation in the Sichuan Basin has always regarded the Xu 1, Xu 3, and Xu 5 Members as source rocks, and the Xu 2, Xu 4, and Xu 6 Members as reservoirs. However, there are few summaries on the distribution law of natural gas in the self-generation and self-storage combination within the source. Through the review of old wells, it is found that there are frequent oil and gas shows in the Xu 3 and Xu 5 members during the drilling process. At present, high-yield gas flows have been obtained in the Xu 3 and Xu 5 Members of many wells in the Jiulongshan, Zhongtaishan and Qiulin areas. In the source rocks of the Xu 3 and Xu 5 Members, not only mudstone and coal seams are developed, but sandstones are also extremely developed, and the sand bodies are widely distributed. Especially in the source rock areas in the western Sichuan Basin, the average sandstoned ratio is more than 30%. It was found that the in-source sandstones in these areas have large thickness, continuous lateral distribution and good physical properties. Therefore, the sandstones distributed in the source rock series are more likely to form large-scale accumulation of natural gas than the Xu 2, Xu 4, and Xu 6 Members.
4.2.1 Source-reservoir combination within the source of the Xu 3 Member
The Xu 3 Member is distributed in the whole basin and belongs to shallow lake deposits. Lacustrine sand bars are locally developed in the central Sichuan area. Alluvial fans and delta deposits are mainly developed in the northwest part of the basin, and delta deposits are mainly developed in the northeast and southeast parts of the basin. Distributary channels and sheet sands are the main microfacies types. Statistics show that the thickness of a single sand layer ranges from 5 m to 10 m, the maximum can reach 40 m, and its cumulative thickness can reach more than 150 m. The reservoir spaces of the tight sandstones are mainly intergranular dissolved pores, intragranular dissolved pores and microfractures. The average porosity is 4.28%, and the average permeability is 0.13 μm2 × 10−3 μm2. These reservoirs are distributed in a thin-layered or lenticular form, and they constitute a good source-reservoir assemblage with the mudstones developed in the Xu 3 Member (Figure 5).
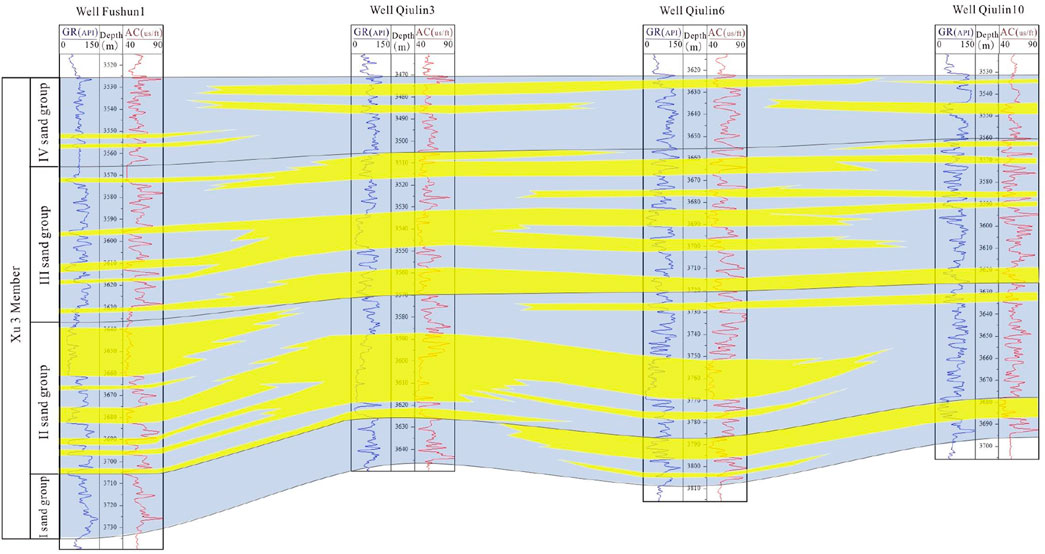
FIGURE 5. Well-connected profile of the source-reservoir assemblage within the source of the Xu 3 Member (the plane position is shown in Figure 8). Yellow represents sandstone and grey represents mudstone.
4.2.2 Source-reservoir combination within the source of the Xu 5 Member
The sedimentary environment of the Xu 5 Member is similar to the Xu 3 Member. However, due to the uplift and denudation in the northwest part of the basin, the direction of the main water system has changed, and the main water system is mainly injected into the basin along the northeast and south directions. Delta deposits are mainly developed in the northern, northeastern and southern areas of the basin. Statistics show that the thickness of a single sand layer ranges from 10 m to 15 m, the maximum can reach 30 m, and the cumulative thickness can reach more than 100 m. Intergranular dissolved pores, intragranular dissolved pores and microfractures are the main types of pores. The average porosity is 4.48%, and the average permeability is 0.35 μm2 × 10−3 μm2. Thin-layered or lenticular tight sandstones and widely developed mudstones in the Xu 5 Member constitute a good source-reservoir assemblage (Figure 6). The surface porosity of micro-fractures in the reservoir is usually only 0.5%–1%, but their existence plays an important role in improving the seepage capacity of the reservoir.
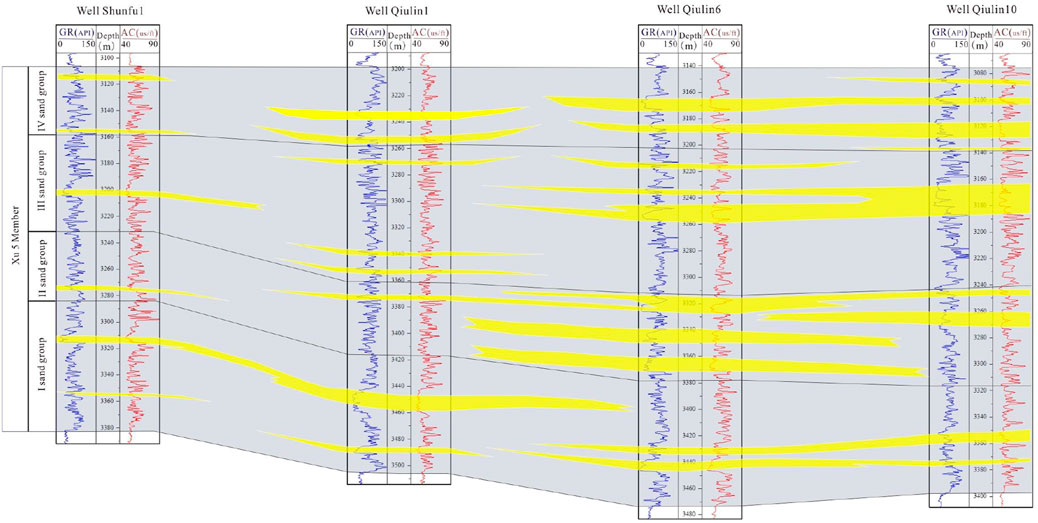
FIGURE 6. Well-connected profile of the source-reservoir assemblage within the source of the Xu 5 Member (the plane position is shown in Figure 8). Yellow represents sandstone and grey represents mudstone.
5 Discussion
5.1 Controlling effect of independent source-reservoir combination on natural gas distribution
5.1.1 Differences in natural gas characteristics in different layers of the same gas field
The natural gas in the Xujiahe Formation in the Sichuan Basin is dominated by hydrocarbon gas, and the total hydrocarbon volume coefficient is more than 95%. Among the hydrocarbon components, CH4 dominates, while the non-hydrocarbon gases have lower contents of CO2 and N2, and most areas do not contain H2S. It can be seen from Table 1 that the methane content and drying coefficient of the natural gas in some typical gas fields of the Xujiahe Formation in the central Sichuan area show a decreasing trend from the Xu 2 to Xu 4, and to Xu 6 Members. It shows that the natural gas sources of each interval are different.
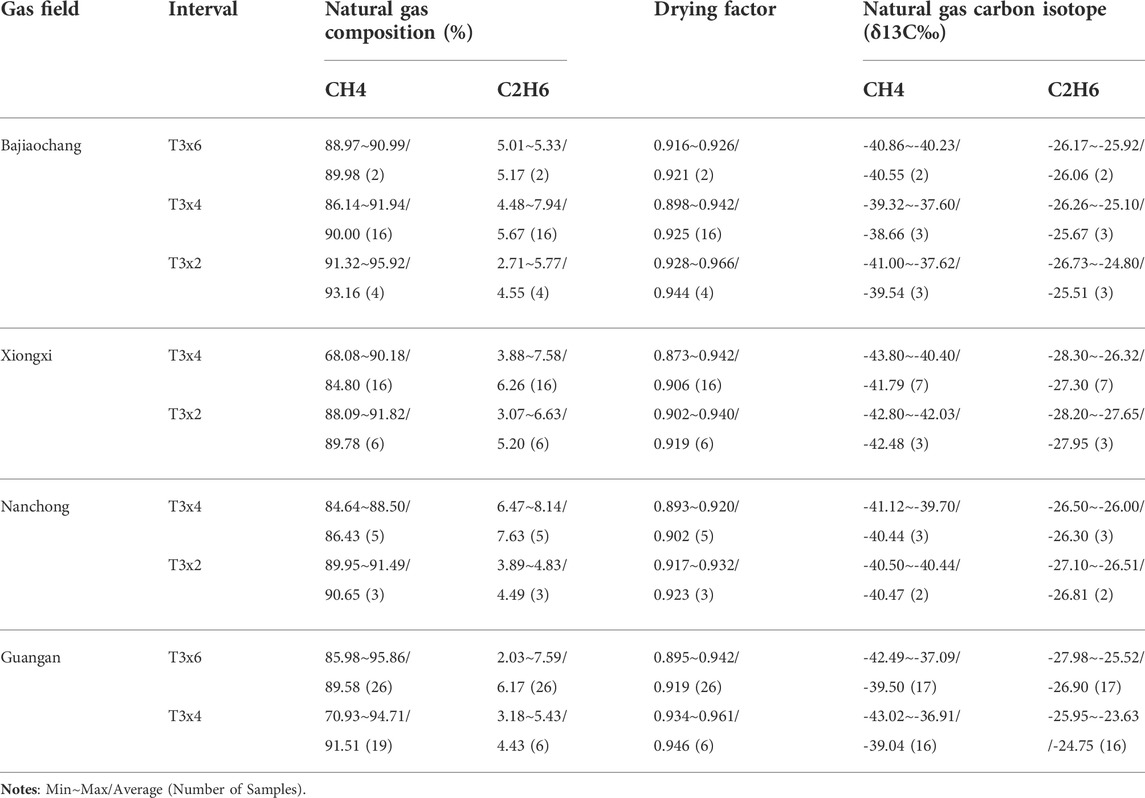
TABLE 1. Carbon isotopic characteristics of natural gas in typical gas fields of the Upper Triassic Xujiahe Formation in the Central Sichuan Basin.
Taking the Guang’an Gas Field as an example, the differences in the methane content and drying coefficient of the natural gas in the Xu 6 and Xu 4 Members of the Xujiahe Formation are obvious. The methane content of the natural gas in the Xu 6 Member of the Guang’an Gas Field is between 85.98% and 95.86%, with an average of 89.58%; and the drying coefficient is distributed between 0.895 and 0.942, with an average of 0.919. However, the content of methane in the natural gas of the Xu 4 Member ranges from 70.93% to 94.71%, with an average of 91.51; the drying coefficient is distributed between 0.934 and 0.961, with an average of 0.946. From bottom to top, the methane content and drying coefficient of the Xujiahe Formation gas gradually decreased, indicating that the natural gas in the Xu 4 and Xu 6 Members may have different sources.
The carbon isotope value of ethane in the natural gas of the Xu 6 Member of the Guang’an Gas Field ranges from −27.98‰ to −25.52‰, with an average of −26.90‰; while the ethane carbon isotope value of the natural gas in the Xu 4 Member ranges from −25.95‰ to −23.63‰, with an average of −24.75‰. The ethane carbon isotope value of natural gas in the Xu 4 Member is significantly higher than that in the Xu 6 Member (Figure 7). Therefore, the sources of natural gas in each interval of the Xujiahe Formation are obviously different. There is no mixing of source rock products in different intervals. In addition, the drying coefficient of the natural gas in the Xu 4 Member is significantly higher than that in the Xu 6 Member (Table 1). This shows that the maturity of the source rock supplying the natural gas of the Xu 4 Member is higher than that of the source rock supplying the natural gas of the Xu 6 Member. The natural gas in the Xu 4 Member did not migrate to the Xu 6 Member. Therefore, there is no obvious vertical mixing of the natural gas in the Xu 4 and Xu 6 gas reservoirs in the Guang’an Gas Field, and they are most likely to come from the Xu 3 and Xu 5 source rocks in the lower part of the gas reservoir, respectively.
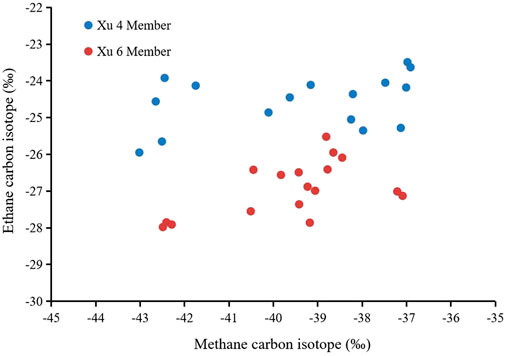
FIGURE 7. Relationship between methane and ethane carbon isotopes in the Xu 4 and Xu 6 Members of Guang’an Gas Field.
5.1.2 Differences in natural gas properties in the same interval of adjacent gas fields
For a long time, it has been controversial whether the natural gas has obvious lateral migration in the same interval of adjacent gas fields. In this study, we compared the geochemical characteristics of natural gas in the Xu 4 Member in the Guang’an and Nanchong Gas Fields of the central Sichuan Basin (Table 1). For the Xu 4 Member of the Guang’an Gas Field, the average methane content of natural gas is 91.51%, the average drying coefficient is 0.946, the average carbon isotope of methane is −24.75‰, and the average carbon isotope of ethane is −24.75‰. However, for the Xu 4 Member of the Nanchong Gas Field, the average methane content of natural gas is 86.43%, the average drying coefficient is 0.902, the average methane carbon isotope is −40.44‰, and the average ethane carbon isotope is −26.30‰. The differences in natural gas composition and carbon isotope values indicate that the gas sources of the Xu 4 Member are different in the Guang’an and Nanqiang Gas Fields. In addition, the drying coefficient of natural gas in the Xu 4 Member of the Guang’an Gas Field is also significantly higher than that in the Nanchong Gas Field. This is because the maturity of the source rocks supplied by the natural gas in the Xu 4 Member of the Guang’an Gas Field is higher than that of the Nanchong Gas Field, and this phenomenon is not caused by the component fractionation caused by the lateral migration of natural gas. If the high drying coefficient of natural gas in the Xu 4 Member of the Guang’an Gas Field is caused by migration and fractionation, then its methane carbon isotope should not be higher than that of the Xu 4 Member of the Nanchong Gas Field. Therefore, the difference in the geochemical characteristics of natural gas in the same interval of adjacent gas fields is caused by the different maturity of the source rocks supplying hydrocarbons in the gas reservoir, rather than by the lateral migration and fractionation of natural gas (Xiao et al., 2008; Luo et al., 2019; Wang et al., 2020).
5.2 Controlling effect of closely contacted source-reservoir assemblages on natural gas enrichment
5.2.1 Control of source rock on filling degree of hydrocarbons
Due to the dramatic changes in the plane depositional environment of the Xujiahe Formation, the distribution of source rocks is extremely uneven. The dark mudstone in the Xujiahe Formation has a large thickness. It gradually thins from the northwest to the southeast of the basin. In contrast, coal seams and carbonaceous mudstones are relatively thin in thickness and vary greatly on the plane. The thickness center of the Xujiahe Formation source rocks is distributed in the western Sichuan area, with an average thickness of 100 m–400 m, while that in the central Sichuan area is generally 20 m–60 m. Moreover, the source rocks in the Xujiahe Formation are basically in the early and middle stages of massive gas generation. The degree of thermal evolution decreased gradually from the Xu 1 Member (Ro is between 1.1% and 2.2%) to the Xu 3 Member (Ro is between 1.0% and 1.8%) and Xu 5 Member (Ro is between 0.8% and 1.4%). The thermal evolution degree of the Xujiahe Formation in the western Sichuan Basin is the highest (Ro is between 0.9% and 2.2%), followed by the northeastern Sichuan Basin (Ro is between 0.8% and 1.6%), and that in te central and southern Sichuan Basin are the lowest (Ro is less than 1.2%). The cumulative gas generation intensity of the Xujiahe Formation source rocks is between 10 m3/km2 and 100 m3/km2 × 108 m3/km2. Among them, the gas generation intensity in the western Sichuan region is relatively high (generally greater than 20 m3/km2 × 108 m3/km2), while that in the central and southern Sichuan regions is relatively small (10∼20 m3/km2 ×108 m3/km2).
It is difficult for the natural gas generated from the source rocks of the Xujiahe Formation to migrate laterally on a large scale after entering the highly heterogeneous reservoir. Short-range migration is the main migration mode of hydrocarbons (Jiu et al., 2013; Liao et al., 2014; Lan et al., 2021; Zhang et al., 2021). For the Xujiahe Formation in the central Sichuan area, the source rocks have a large cumulative thickness, a wide distribution range, and a high cumulative hydrocarbon generation intensity, but the gas generation intensity of each layer of source rocks is relatively small. For example, the average gas generation intensity of the Xu 1, Xu 3, and Xu 5 Members are 2.06 m3/km2 × 108 m3/km2, 3.75 m3/km2 × 108 m3/km2, and 6.29 m3/km2 × 108 m3/km2, respectively. Due to the near-source accumulation of hydrocarbons, the natural gas generated by the source rocks of the Xu 1, Xu 3, and Xu 5 Members will be preferentially charged from the sandstones with good physical properties, and then supplied to the Xu 2, Xu 4, and Xu 6 Members, respectively. The single-layer hydrocarbon supply capacity of each interval is weak. The amount of natural gas generated is far less than the storage space of sandstone. Therefore, the charging of natural gas is insufficient, the gas reservoirs are independent of each other and the separation of gas and water is insufficient (Ni et al., 2011; Zhou et al., 2019; Morozov et al., 2021). It can be seen from Figure 8 that the high-yield wells are mainly distributed in the regions with gas saturation greater than 57.5%. In addition, the gas saturation has a good positive correlation with the hydrocarbon generation intensity of the source rocks. Areas with hydrocarbon generation intensity greater than 4 m3/km2 × 108 m3/km2 have higher gas saturation and natural gas productivity.
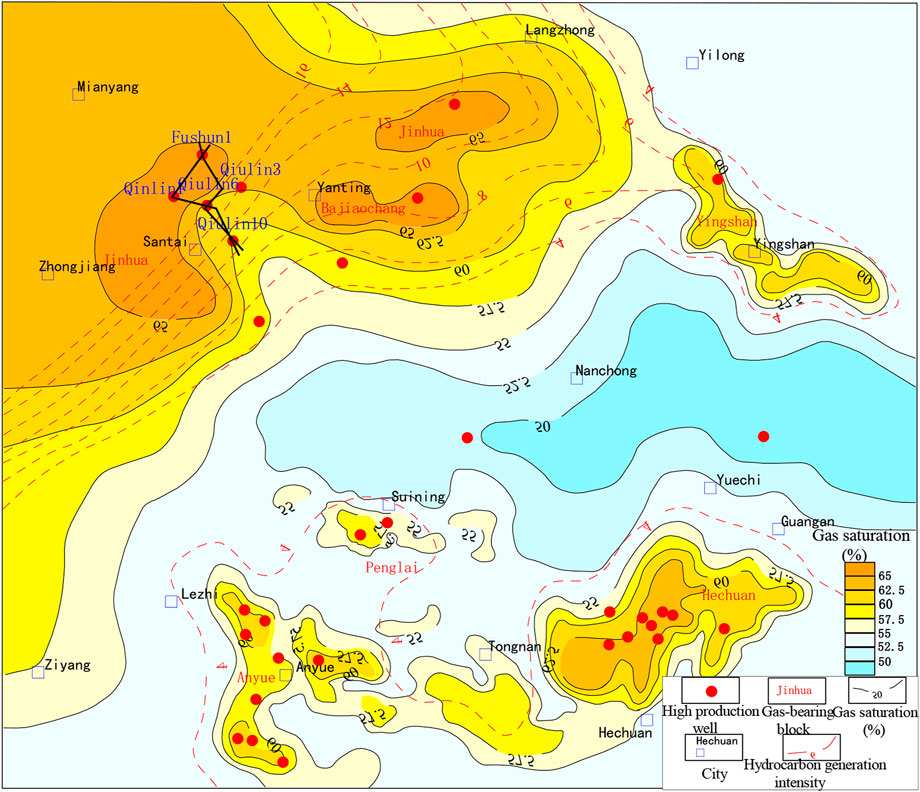
FIGURE 8. Coupling relationship between the gas saturation of the Xu 2 Member and the hydrocarbon generation intensity of the underlying Xu 1 Member in the central and western Sichuan areas.
5.2.2 Control of reservoir performance on production capacity of natural gas
The Xujiahe Formation is a set of coal-measure strata, and the developed tight sandstone reservoirs contain pores and fractures. Pores are the main reservoir space, and the types of pores include intragranular dissolved pores, intergranular pores, intragranular pores, intergranular dissolved pores, mold pores, matrix dissolved pores, and intercrystalline micropores of clay minerals (Figure 9). Among them, intragranular dissolved pores and intergranular pores are the main types of pores. The pore types have obvious regional distribution differences. Intergranular pores are mainly developed in the depression areas of the northwestern Sichuan Basin, while dissolution pores are not developed. Dissolution pores are mainly developed in the southwestern Sichuan Basin, especially the feldspar intragranular dissolved pores and intergranular dissolved pores, while the intergranular pores are rare. For the central, eastern and central-south transition zones of the Sichuan Basin, intergranular pores and intragranular dissolved pores are well developed. The pores in the southern Sichuan area are generally underdeveloped, and dissolved pores are the main pore type.
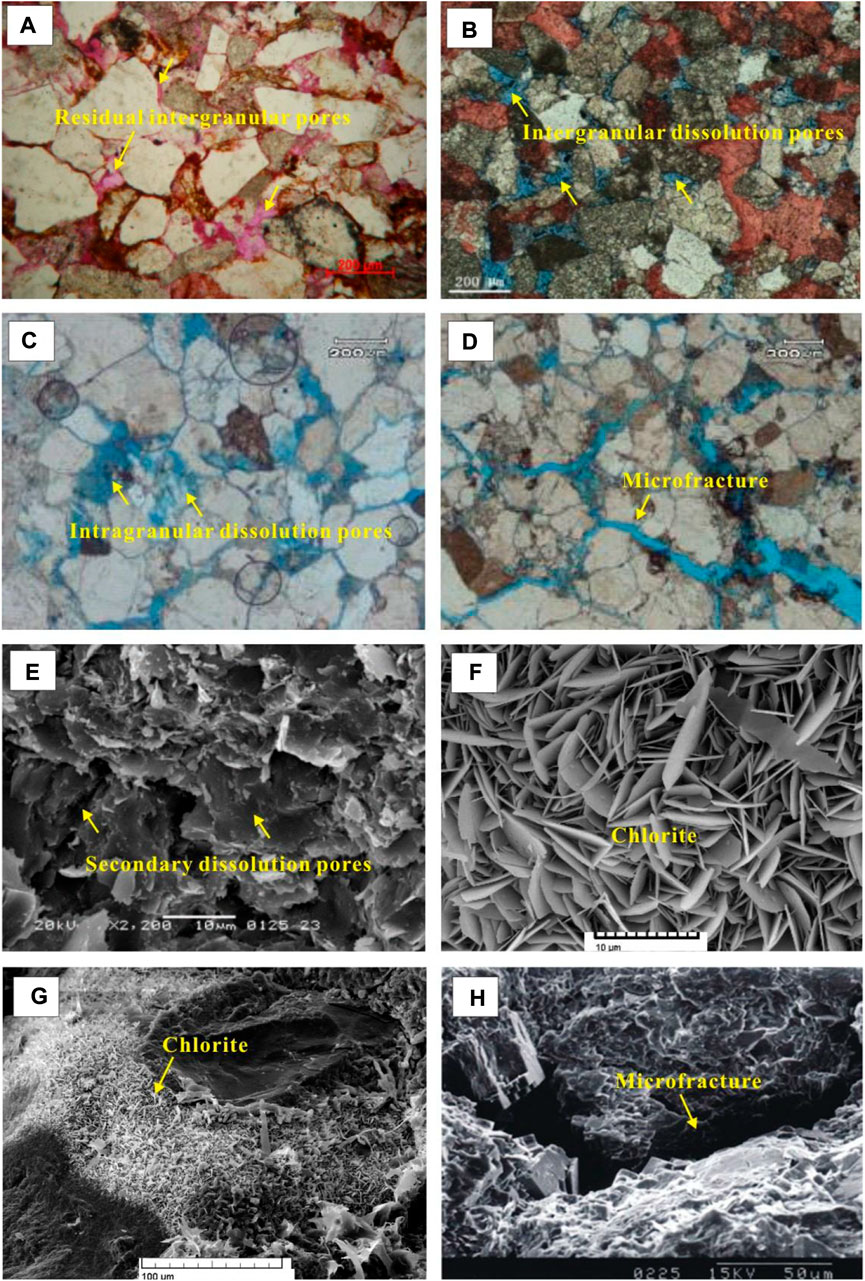
FIGURE 9. Microscopic characteristics of sandstone reservoirs in Xujiahe Formation. (A) Well Yu 1, 4,379.55 m, residual intergranular pores and intergranular dissolved pores; (B) Well Jinhua 3, 3,298.00 m, intergranular dissolved pores; (C) Well W6, 1,127.80 m, intergranular pores and intragranular dissolved pores; (D) Well Ma 14, 1,317.10 m, microfractures; (E) Well Qiulin 7, 3298 m, secondary dissolved pores; (F) Well Jiao 41, 3,076.0 m, needle-like and leaf-like chlorite; (G) Well Baoqian 001–16, 1,585.5 m, coniferous chlorite; (H) Well Guan 2, 3,665.85 m, open microfractures with dissolved surface.
The regional differences in pore types are closely related to the reservoir rock types and diagenesis (Fan et al., 2005; Hao et al., 2016; Nicula1 et al., 2021; Saein and Riahi., 2017). When the feldspar and chlorite contents are high, the pores are relatively developed (Hu and Xie., 1997; Hu et al., 2012; Wan et al., 2012). The development scale of tight reservoirs in the Xujiahe Formation is mainly controlled by sedimentation, diagenesis and tectonic processes. Sedimentary facies, especially microfacies, are the basis for reservoir development (Yin et al., 2019; Mondal et al., 2021; Li., 2022). Distributary channels and estuary bars are favorable microfacies for reservoir development, while reservoirs in fluvial and lacustrine facies have poor physical properties. Compaction, dissolution and annular chlorite cementation are the main diagenetic processes that affect the development and evolution of reservoirs (Zhao et al., 2013; Yin et al., 2018; Zhao et al., 2020). Chlorite cemented facies and dissolution-kaolinite cemented facies are the most favorable diagenetic facies, while siliceous cemented facies and strong compaction facies are unfavorable diagenetic facies. The fractures formed by the Himalayan Movement can significantly improve the permeability of tight reservoirs.
Reservoir performance has obvious control effect on the filling degree of natural gas and the productivity of a single well (Montgomery et al., 2005; Lai et al., 2018; Hower and Groppo, 2021). Generally, the cumulative productivity of a single well exhibits a positive correlation with both reservoir thickness and porosity (Wu et al., 2015; Wu et al., 2016; Yang et al., 2021). The energy storage coefficient of high-yield wells is usually greater than 0.8, and the reservoir thickness is greater than 10 m. Under the background of low hydrocarbon generation intensity, natural gas will preferentially charge into sand bodies with good reservoir physical properties and relatively large pore diameters (0.1 μm–10 μm). Therefore, sand bodies with relatively high porosity and high permeability are the preferred targets for the preferential charging of natural gas.
According to the experimental analysis results of porosity, permeability and saturation of multiple sample points in the Xu 6 Member of Well GA108, the physical properties of the reservoir are positively correlated with gas saturations. In the 1931.00 m–1951.00 m interval, the average porosity of the reservoir is 8.73%, the average permeability is 0.236 μm2 × 10−3 μm2, and the average gas saturation is 60.73%. Obviously, good reservoir physical properties correspond to high gas saturations. However, in the 1926.22 m–1931.00 m well interval, the average porosity of the reservoir is 5.18%, the average permeability is 0.057 μm2 ×10−3 μm2, and the average gas saturation is only 39.68%. In addition, rock type and fractures also significantly affect the gas-bearing properties of the reservoir. The lithology of the Xujiahe Formation is mainly composed of detrital feldspar sandstone, feldspar detrital sandstone, detrital quartz sandstone and feldspar quartz sandstone. According to the observation results of 11 wells in the Guang’an Gas Field, the reservoir lithology in the wells with output greater than 2 m3/d × 104 m3/d is mainly feldspar detrital sandstone and feldspar quartz sandstone. When the Xujiahe Formation reservoir is relatively tight, only the effective combination of pores and fractures can form a high-efficiency reservoir. For example, the productivity of gas layers drilling fractures of Well Y104 in the Anyue Gas Field is usually greater than 15 m3/d × 104 m3/d.
5.2.3 Control of close-contact source-reservoir combination on large-scale enrichment of natural gas
The closely contacted source-reservoir assemblage has a significant controlling effect on the enrichment of natural gas in the Xujiahe Formation. Judging from the source-reservoir combination of the Xu 3 Member (Figure 10), the commercial gas wells that have been discovered so far are mainly distributed in areas with a sandstone ratio of more than 25%, while no commercial gas wells have been found in areas with a sandstone ratio of less than 25%. This is because areas with a sandstone ratio lower than 25% are usually pre-delta or lacustrine deposits, and the thickness of a single layer of sandstone is thin (1 m∼3 m). Moreover, due to the large thickness of the mudstone around the sandstone, the compaction and cementation exerted on the sandstone are very strong, and the physical properties of the sandstone become very poor (Huang et al., 1996; Holt et al., 2015; Zhai et al., 2017). Therefore, the natural gas enrichment areas of the “self-generation and self-storage” source-reservoir combination in the Xu 3 Member are mainly distributed in the northwestern Sichuan area where the source and reservoir are in close contact and the sandstone ratio is greater than 25%.
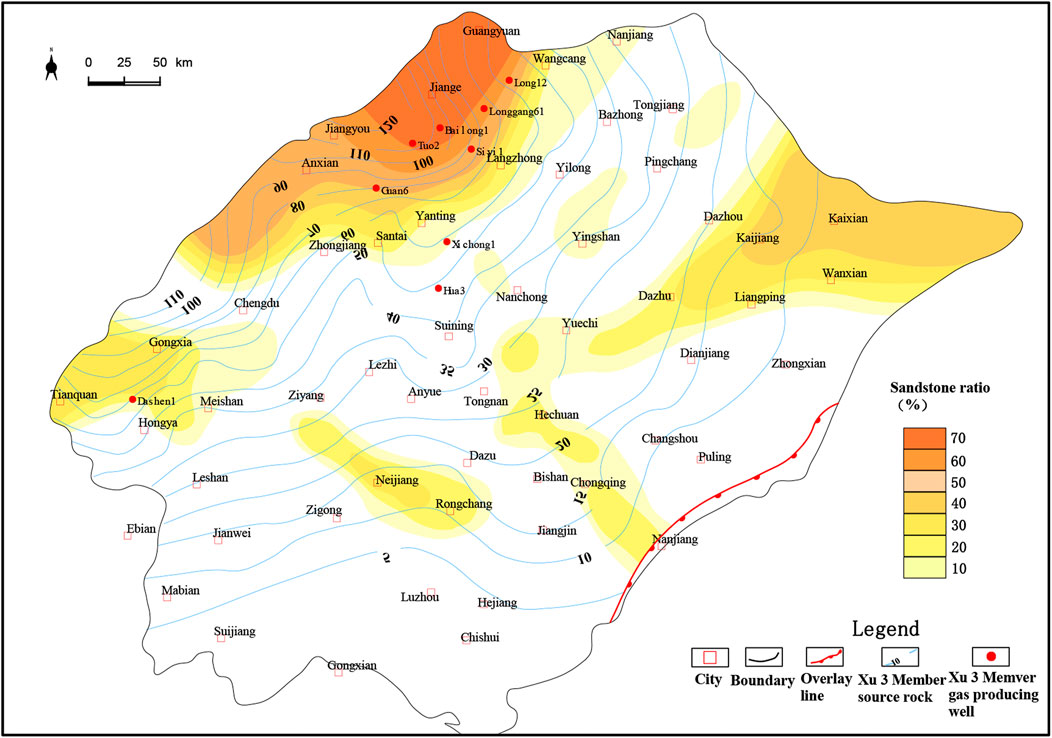
FIGURE 10. Superposition of source rock thickness and sandstone ratio of Xujiahe Formation in the Sichuan Basin.
The average organic carbon content of dark mudstone in the Xujiahe Formation is 1.95%, while that in the carbonaceous mudstone is generally more than 10%, and that in the coal seams is as high as 60%. The average hydrocarbon generation potentials of coal seams, carbonaceous mudstone, and dark mudstone in the Xujiahe Formation are: 97 mg/g, 15 mg/g, and 2.4 mg/g, respectively. Therefore, carbonaceous mudstone and coal seams with high organic carbon content and hydrocarbon generation potential are the main source rocks of the Xujiahe Formation. The reservoir thickness and physical properties of the Xu 4 Member gas reservoir in the Guang’an Gas Field are roughly the same as those of the Xu 6 Member. However, the movable water content of the Xu Member is generally high, and the gas production per well is very low. Then, the development of natural gas reserves in the Xu 4 Member is difficult. This is because the source rocks of the Xu 5 Member below the gas reservoir of the Xu 6 Member are a set of coal seams with an average thickness of more than 10 m. This set of coal seams is continuously distributed and the gas generation intensity is high (10 m3/km2 × 108 m3/km2). However, the source rocks of the Xu 3 Member below the Xu 4 Member are mainly mudstone, and the thickness of the coal seam is relatively thin, basically 3 m–5 m, and the gas generation intensity of the source rock is low. It can be seen from Figure 11 that the discovered gas reservoirs and gas-bearing structures in the Xu 4 Member in the central Sichuan Basin are mainly distributed in areas where carbonaceous mudstone and coal seams are relatively developed in the Xu 3 Member and the areas where the reservoir thickness of the Xu 4 Member is greater than 20 m. This fully shows that the natural gas enrichment areas of the source-reservoir combination of extra-source “lower generation and upper storage” are mainly distributed in the overlying reservoirs of the source rock development areas with close source-reservoir contact and strong hydrocarbon generation intensity (Wang., 1994; Qin et al., 2019; Zhao et al., 2021).
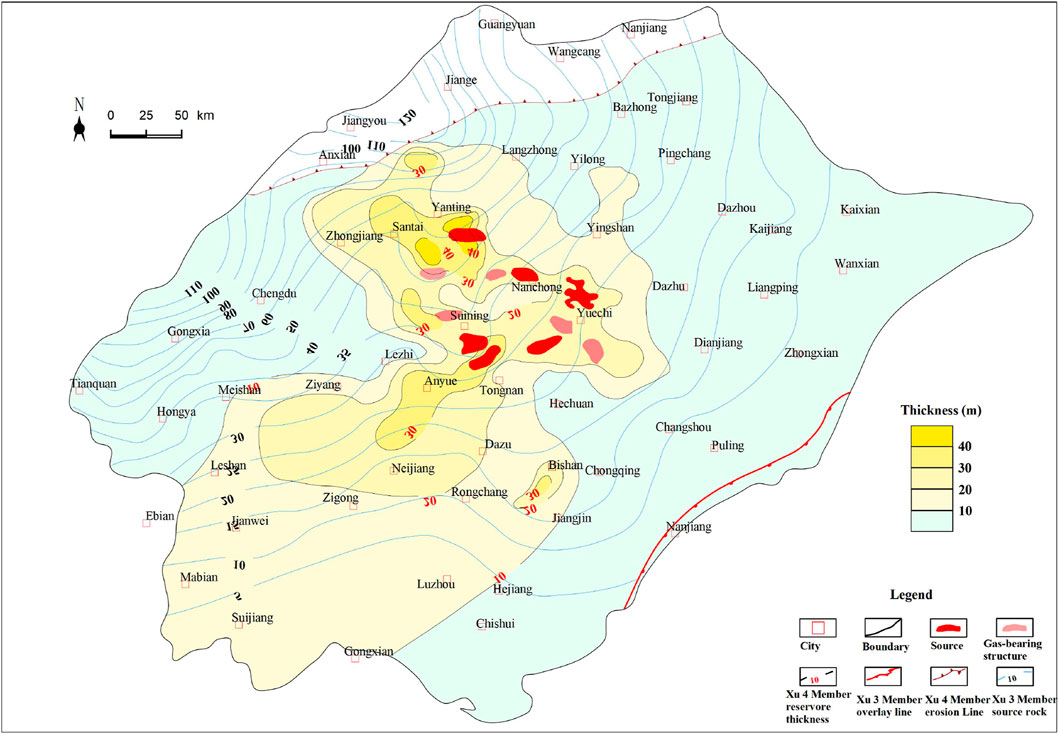
FIGURE 11. Relationship between the thickness of carbonaceous mudstone and coal seam in the Xu 3 Member and the reservoir thickness and natural gas distribution of the Xu 4 Member in the central and western Sichuan Basin.
6 Conclusion
1) The main pore types of tight sandstones in the Xujiahe Formation are intragranular dissolved pores and intergranular pores. The quality of sandstone reservoirs is controlled by sedimentation, diagenesis and tectonic processes. Underwater distributary channels and estuary bars are favorable microfacies for reservoir development. Moreover, chlorite cemented facies and dissolution-kaolinite cemented facies are the most favorable diagenetic facies.
2) The natural gas composition and carbon isotope characteristics of the Xujiahe Formation are significantly different in different intervals of the same gas field and the same interval of adjacent gas fields. This shows that the natural gas has no obvious vertical mixing and lateral migration, and it has the distribution characteristics of “local enrichment”. Natural gas is preferentially charged in the feldspar lithic sandstones and feldspar quartz sandstones with large thickness and good physical properties by means of short-range migration.
3) On the whole, four sets of extra-source “lower generation and upper storage” assemblages and two sets of “self-generation and self-storage” source-reservoir assemblages are developed in the Xujiahe Formation. The areas with close source-reservoir contact and high hydrocarbon generation intensity are high-quality reservoir development areas.
Data availability statement
The original contributions presented in the study are included in the article/supplementary material, further inquiries can be directed to the corresponding authors.
Author contributions
KT is responsible for the idea and writing of this paper and JY, JC, DT, YQ, and QW areresponsible for the drawing and experimental testing.
Conflict of interest
Authors KT, JY, JC, YQ, and QW were employed by the Research Institute of Petroleum Exploration and Development Northwest Branch and author DT was employed by the Northwest Sichuan Gas Mine of PetroChina Southwest Oil and Gas Field Company.
Publisher’s note
All claims expressed in this article are solely those of the authors and do not necessarily represent those of their affiliated organizations, or those of the publisher, the editors and the reviewers. Any product that may be evaluated in this article, or claim that may be made by its manufacturer, is not guaranteed or endorsed by the publisher.
References
Dai, J. (2014). Giant coal-derived gas fields and the source in China. Beijing: Science Press, 420–424. doi:10.1016/b978-0-12-805093-4.00001-x
Dai, J., Ni, Y., and Zou, C. (2012). Stable carbon and hydrogen isotopes of natural gases sourced from the Xujiahe Formation in the Sichuan Basin, China. Org. Geochem. 43 (2), 103–111. doi:10.1016/j.orggeochem.2011.10.006
Dai, J., Qin, S., and Tao, S. (2005). Developing trends of natural gas industry and the significant progress on natural gas geological theories in China. Nat. Gas. Geosci. 16 (2), 127–142. doi:10.3969/j.issn.1672-1926.2005.02.001
Dai, J. X., Ni, Y. Y., and Zong, N. (2009). Carbon isotope features of al-kane gases in the coal measures of the Xujiahe Formation inthe Sichuan Basin and their significance to gas-source correlation. Oil Gas Geol. 30 (5), 519–529. doi:10.11743/ogg20090501
Du, H., Wang, W., Shi, Z., Tan, J., Cao, H., and Yin, X. (2019). Geochemical characteristics and source of natural gas of the upper triassic Xujiahe Formation in malubei are a,northeastern Sichuan Basin. Oil Gas Geol. 40 (1), 34–38. doi:10.11743/ogg20190104
Du, M., Wang, S., Wan, M., Zhang, Q., and Wang, L. (2007). Geochemical characteristics and genetic types of natural gas in upper triassic Xujiahe Formation, Sichuan Basin. Nat. Gas Explor. Dev. 30 (2), 26–29.
Fan, R., Zhou, H., and Cai, K. (2005). Carbon isotopic geochemistry and origin of natural gases in the southern part of the Western Sichuan Depression. Acta Geosci. Sin. 26 (2), 157–162. doi:10.3321/j.issn:1006-3021.2005.02.009
Hao, B., Hu, S., and Huang, S. (2016). Geochemical characteristics and its significance of reservoir bitumen of longwangmiao Formation in moxi area, Sichuan Basin. Geoscience 30 (3), 614–626.
Holt, R. M., Fjær, E., Stenebråten, J. F., and Nes, O. M. (2015). Brittleness of shales: Relevance to borehole collapse and hydraulic fracturing. J. Petroleum Sci. Eng. 131, 200–209. doi:10.1016/j.petrol.2015.04.006
Hower, J. C., and Groppo, J. G. (2021). Rare Earth-bearing particles in fly ash carbons: Examples from the combustion of eastern Kentucky coals. Energy Geosci. 2 (2), 90–98. doi:10.1016/j.engeos.2020.09.003
Hu, G., Wang, W., and Liao, F. (2012). Geochemical characteristics and its influencing factors of light hydrocarbon in coal-derived gas: A case study of Sichuan Basin. Acta Petrol. Sin. 28 (3), 905–916. doi:10.3799/dqkx.2021.033
Hu, G., and Xie, Y. (1997). Carboniferous gas fields in high steep structure of eastern Sichuan. BeiJing: Petroleum industry press, 47–62.
Hu, W., Zhu, Y., and Li, Y. (2014). Geochemical charac-teristics and origin of natural gases from terrestrial strata inYuanba area of the northeastern Sichuan Basin. J. Zhejiang Univ. Sci. Ed. 41 (4), 468–476. doi:10.3785/j.issn.1008-9497.2014.04.020
Huang, J., Chen, S., Song, J., Wang, L., Gou, X., Wang, T., et al. (1997). Hydrocarbon source systems and formation of gas fields in Sichuan Basin. Sci. China Ser. D-Earth Sci. 40 (6), 32–42. doi:10.1007/BF02878579
Jiu, K., Ding, W. L., Huang, W. H., You, S. G., Zhang, Y. Q., and Zeng, W. T. (2013). Simulation of paleotectonic stress fields within Paleogene shale reservoirs and prediction of favorable zones for fracture development within the Zhanhua Depression, Bohai Bay Basin, east China. J. Petroleum Sci. Eng. 110, 119–131. doi:10.1016/j.petrol.2013.09.002
Lai, J., Wang, G. W., Wang, S., Cao, J. T., Li, M., Pang, X. J., et al. (2018). Review of diagenetic facies in tight sandstones: Diagenesis, diagenetic minerals, and prediction via well logs. Earth-Science Rev. 185, 234–258. doi:10.1016/j.earscirev.2018.06.009
Lan, S. R., Song, D. Z., Li, Z. L., and Liu, Y. (2021). Experimental study on acoustic emission characteristics of fault slip process based on damage factor. J. Min. Strata Control Eng. 3 (3), 033024. doi:10.13532/j.jmsce.cn10-1638/td.20210510.002
Li, H. (2022). Research progress on evaluation methods and factors influencing shale brittleness: A review. Energy Rep. 8, 4344–4358. doi:10.1016/j.egyr.2022.03.120
Li, Y. (2021). Mechanics and fracturing techniques of deep shale from the Sichuan Basin, SW China. Energy Geosci. 2 (1), 1–9. doi:10.1016/j.engeos.2020.06.002
Liang, Y., Li, Y., Fu, X., Yuan, X., Yang, J., and Zheng, J. (2006). Origin and wholehydrocarbon geochemical characteristics of oil and gas from Xujiahe group of Chuanzhong-Chuannan Transitional Belt. Nat. Gas. Geosci. 17 (4), 593–596. doi:10.3969/j.issn.1672-1926.2006.04.034
Liao, F., Yu, C., and Wu, W. (2014). Stable carbon and hydrogen isotopes of natural gas from the Zhongba Gasfield inthe Sichuan Basin and implication for gas-source correlation. Nat. Gas. Geosci. 25 (1), 79–86. doi:10.11764/j.issn.1672-1926.2014.01.0079
Liu, Q., Dai, J., Li, J., and Zhou, Q. (2008). Hydrogen isotope composition of natural gases from the Tarim Basin and its indication of depositional environments of the source rocks. Sci. China Ser. D-Earth Sci. 51 (12), 300–311. doi:10.1007/s11430-008-0006-7
Luo, Y., Huang, H., Jakobsen, M., Yang, Y., Zhang, J., and Cai, Y. (2019). Prediction of porosity and gas saturation for deep-buried sandstone reservoirs from seismic data using an improved rock-physics model. Acta Geophys. 67, 557–575. doi:10.11743/ogg1995031510.1007/s11600-019-00274-6
Mondal, S., Chatterjee, R., and Chakraborty, S. (2021). An integrated approach for reservoir characterisation in deep-water krishna-godavari basin, India: A case study. J. Geophys. Eng. 18 (1), 134–144. doi:10.1093/jge/gxab002
Montgomery, S. L., Jarvie, D. M., Bowker, K. A., and Pollastro, R. M. (2005). Mississippian Barnett Shale, Fort Worth basin, north-central Texas: Gas-shale play with multi-trillion cubic foot potential. Bulletin 89 (2), 155–175. doi:10.1306/0209060513210.1306/09170404042
Morozov, V. P., Jin, Z., Liang, X., Korolev, E. A., Liu, Q., Kolchugin, A. N., et al. (2021). Comparison of source rocks from the lower silurian longmaxi Formation in the yangzi platform and the upper devonian semiluksk Formation in east European platform. Energy Geosci. 2 (1), 63–72. doi:10.1016/j.engeos.2020.10.001
Ni, Y., Liao, F., and Yao, L. (2019). Stable hydrogen isotopic characteristicsof natural gas from the Xujiahe Formation in the central Sichuan Basin and its implications for water salinization. Nat. Gas. Geosci. 30 (6), 880–896. doi:10.11764/j.issn.1672-1926.2019.05.015
Ni, Y., Ma, Q., Ellis, G., Dai, J., Katz, B., Zhang, S., et al. (2011). Fundamental studies on kinetic isotope effect (KIE) of hydrogen isotope fractionation in natural gas systems. Geochimica Cosmochimica Acta 75 (10), 2696–2707. doi:10.1016/j.gca.2011.02.016
Nicula, A., Ionescu, A., Pop, I., Roba, C., Forray, F. L., Orăşeanu, I., et al. (2021). Geochemical features of the thermal and mineral waters from the apuseni mountains (Romania). Front. Earth Sci. 9, 1–8. doi:10.3389/feart.2021.64817910.1016/j.jvolgeores.2019.02.014
Qin, S., Huang, C., and Zhang, B. (2019). Relationships of the iCa/nC4 and iCs/nCs ratios with maturity of coal-derived gases of Triassic Xujiahe Formation in central Sichuan Basin, SW China. Petroleum Explor. Dev. 46 (3), 474–481. doi:10.1016/S1876-3804(19)60030-3
Saein, A., and Riahi, Z. (2017)., 156. Iran, 1092–1104. doi:10.1017/S001675681700034610.1017/S0016756817000346Controls on fracture distribution in Cretaceous sedimentary rocks from the Isfahan region, IranGeol. Mag.
Wan, M., Xie, B., Chen, S., Zou, C., Zhang, Q., and Ran, Y. (2012). Hydrocarbon source correlation of the upper Triassic reservoirs in the Sichuan Basin. Nat. Gas. Ind. 32 (3), 22–24.
Wang, H., Shi, Z., Zhao, Q., Liu, D., Sun, S., Guo, W., et al. (2020). Stratigraphic framework of the Wufeng-Longmaxi shale in and around the Sichuan Basin, China: Implications for targeting shale gas. Energy Geosci. 1 (3–4), 124–133. doi:10.1016/j.engeos.2020.05.006
Wang, S. (1994). Chemical characteristics of jurassic-sinian gasin Sichuan Basin. Nat. Gas. Ind. 14 (6), 1–5.
Wu, X., Liu, G., and Liu, Q. (2015). Geochemicalcharacteristics and origin of natural gas in the Xujiahe forma-tion in yuanba-tongnanba area of Sichuan Basin. Oil Gas Geol. 36 (6), 955–962. doi:10.11743/ogg20150610
Wu, X., Wang, P., and Liu, Q. (2016). The source of natural gas reservoir in the 5th member of the upper triassic Xujiahe Formation in xinchang gas field, the western sichuan depression and its implication. Nat. Gas. Geosci. 27 (8), 1409–1418.
Xiao, Z., Xie, Z., and Li, Z. (2008). Isotopic characteristics ofnatural gas of Xujiahe Formation in southern and middle of Si-chuan Basin. Geochimica 37 (3), 245–250. doi:10.3863/j.issn.1000-2634.2008.04.007
Yang, J. X., Luo, M. K., Zhang, X. W., Huang, N., and Hou, S. J. (2021). Mechanical properties and fatigue damage evolution of granite under cyclic loading and unloading conditions. J. Min. Strata Control Eng. 3 (3), 033016. doi:10.13532/j.jmsce.cn10-1638/td.20210510.001
Yin, S., Lv, D. W., and Ding, W. L. (2018). New method for assessing microfracture stress sensitivity in tight sandstone reservoirs based on acoustic experiments. Int. J. Geomechanics 18, 1–16. doi:10.1061/(ASCE)GM.1943-5622.0001100
Yin, S., Xie, R. C., Wu, Z. H., Liu, J., and Ding, W. L. (2019). In situ stress heterogeneity in a highly developed strike-slip fault zone and its effect on the distribution of tight gases: A 3D finite element simulation study. Mar. Petroleum Geol. 99 (1), 75–91. doi:10.1016/j.marpetgeo.2018.10.007
Yu, C., Gong, D., and Huang, S. (2014). Geochemical char-acteristics of carbon and hydrogen isotopes for the Xujiahe Formation natural gas in Sichuan Basin. Nat. Gas. Geosci. 25 (1), 87–97.
Zhai, L., Ni, Y., and Wu, C. (2017). Geochemical characteristics of the natural gas from the Xujiahe Formation in the central Sichuan Basin, China. Nat. Gas. Geosci. 28 (4), 539–549. doi:10.11764/j.issn.1672-1926.2017.02.016
Zhang, L., Liu, D., Gao, Y. J., and Zhang, M. (2021). Geochemical characteristics of gas and flowback water in lake facies shale: A case study from the junggar basin, China. Front. Earth Sci. 9, 1–14. doi:10.3389/feart.2021.63589310.3389/feart.2021.635893
Zhao, K. K., Jiang, P. F., Feng, Y. J., Sun, X. D., Cheng, L. X., and Zheng, J. W. (2021). Investigation of the characteristics of hydraulic fracture initiation by using maximum tangential stress criterion. J. Min. Strata Control Eng. 3 (2), 023520. doi:10.13532/j.jmsce.cn10-1638/td.20201217.001
Zhao, W., Bian, C., and Xu, Z. (2013). Similarities and differences between natural gas accumulations in Sulige gas field in Ordos Basin and Xujiahe gas field in central Sichuan Basin. Petroleum Explor. Dev. 40 (4), 400–406. doi:10.1016/S1876-3804(13)60054-3
Zhao, Z., Wu, K., Fan, Y., Guo, J., Zeng, B., and Yue, W. (2020). An optimization model for conductivity of hydraulic fracture networks in the Longmaxi shale, Sichuan Basin, Southwest China. Energy Geosci. 1 (1–2), 47–54. doi:10.1016/j.engeos.2020.05.001
Keywords: sichuan basin, xujiahe formation, pore structure, natural gas composition, carbon
Citation: Tan K, Yao J, Chen J, Tang D, Qin Y and Wu Q (2022) Controlling effect of source-reservoir assemblage on natural gas accumulation: A case study of the upper triassic xujiahe formation in the sichuan basin. Front. Earth Sci. 10:1028439. doi: 10.3389/feart.2022.1028439
Received: 26 August 2022; Accepted: 07 September 2022;
Published: 23 September 2022.
Edited by:
Wenlong Ding, China University of Geosciences, ChinaReviewed by:
Chao Han, Shandong University of Science and Technology, ChinaYing Tang, Xi’an Shiyou University, China
Copyright © 2022 Tan, Yao, Chen, Tang, Qin and Wu. This is an open-access article distributed under the terms of the Creative Commons Attribution License (CC BY). The use, distribution or reproduction in other forums is permitted, provided the original author(s) and the copyright owner(s) are credited and that the original publication in this journal is cited, in accordance with accepted academic practice. No use, distribution or reproduction is permitted which does not comply with these terms.
*Correspondence: Kaijun Tan, dGFua2FpanVuMjBAMTI2LmNvbQ==