- 1Shaanxi Yanchang Petroleum (Group) Corp. Ltd., Nanniwan Oil Production Plant, Yan’an, Shaanxi, China
- 2The Fifth Oil Production Plant of Petrochina Changqing Oil Field Company, Xi’an, Shaanxi, China
Evaluation of tight oil reservoir properties is of great significance to the exploration of oil and gas in tight reservoirs. The Chang 1 Member of the Yanchang Formation in the Wanhua Area, Ordos Basin is a new exploration stratum for tight sandstone oil. The lack of understanding of reservoir characteristics and crude oil enrichment rules has seriously restricted the efficient development of oil and gas resources in this stratum. In this study, the reservoir characteristics of the Chang 1 Member in the Wanhua area and the effects of superimposed sand bodies, structures and paleogeomorphology on accumulation of hydrocarbons were systematically studied. The Chang 1 sandstone is a typical ultra-low porosity-ultra-low permeability reservoir, and it has experienced destructive diagenesis of mechanical compaction, pressure solution and cementation, and constructive diagenesis of dissolution. Strong pressure solution caused the secondary enlargement of quartz and feldspar and the formation of patchy dense mosaic structures. The target layer has experienced argillaceous, siliceous and carbonate cementations. Moreover, the sandstone reservoir in the Chang 1 Member also experienced strong dissolution, and it is the main factor for the formation of secondary pores and the improvement of reservoir physical properties. The study also found that the main types of pores in the Chang 1 Member are intergranular dissolved pores and remaining intergranular pores. Superimposed sand bodies, nose-shaped uplifts, dominant facies and eroded paleo-highlands have significant effects on the hydrocarbon accumulation. Based on this study, it was found that the migration and accumulation mode of hydrocarbons in the Chang 1 reservoir belongs to the ladder-like climbing migration + structural ridge accumulation type. In addition, sand body thickness is an important controlling factor for the hydrocarbon accumulation. At present, the discovered crude oil in the Chang 1 Member is always distributed in the areas with thick sand bodies (>20 m), and most of the sand bodies have a thickness in the range of 25–40 m, and the effective thickness is in the range of 2–6 m. In addition, the eroded highlands are the highest topographic units, they are favorable areas for the large-scale accumulation of oil and gas.
1 Introduction
Continental tight sandstone oil is an important type of unconventional oil and gas resource (Li et al., 2012; Huang et al., 2019; Asante-Okyere et al., 2021). The core of the study of unconventional tight oil and gas reservoirs is the study of the microscopic characteristics of the reservoir and the law of hydrocarbon enrichment (Zhang et al., 2014; Liao et al., 2020; Cui and Radwan., 2022; Jiang et al., 2022; Zhang et al., 2022). With the rapid decline of China’s conventional oil and gas reserves, the importance of the exploration and development of unconventional oil and gas resources has become increasingly prominent. As we know, the efficient production of continental tight oil in the Ordos Basin relies on the application of horizontal well + volume fracturing technology. Improving the drilling encounter rate of oil layers is one of the core issues of drilling and evaluation of horizontal wells (Sun et al., 2013; Radwan., 2020; Yin et al., 2020; Zhang et al., 2020). At present, the prediction methods for the “sweet spots” of clastic tight oil reservoirs are all based on the controlling factors of reservoir properties. Therefore, it is of great significance to clarify the petrology, pore types, diagenesis, and physical properties of tight oil reservoirs for the successful exploration of “sweet spots” in tight sandstone reservoirs (Sun et al., 2014; Bukar et al., 2021; Katz et al., 2021).
In recent years, with the large-scale development of relatively high-quality reservoirs in tight oil reservoirs, the growth of oil and gas reserves has become difficult. Then, the efficient development of ultra-low permeability tight oil reservoirs with poor physical properties has become a new hot spot for the growth of oil and gas reserves. The current exploration level of the Chang 1 Member of the Yanchang Formation in Wanhua area is low. At present, only 25 development wells and 16 exploration wells were drilled in the study area. It has been found that fine sandstones developed in the middle part of the river channel has good oil and gas display. However, the influencing factors of hydrocarbon distribution in the Chang 1 Member have not been determined. Therefore, fine evaluation of the properties of the tight reservoirs in the Chang 1 Member is of great significance to the increase of oil and gas reserves and productivity in this area. In this study, the reservoir characteristics of the Chang 1 Member in the Wanhua area and the effects of superimposed sand bodies, structures and paleogeomorphology on the hydrocarbon accumulation were systematically studied. This research can provide scientific guidance for formulating an efficient development strategy for the Chang 1 Member in the future.
2 Geological background
The study area is located in the Wanhua area of Nanniwan Oilfield in the Ordos Basin. The Nanniwan Oilfield is located in the Baota District, Yan’an City, Shaanxi Province. It spans 9 townships including Guanyu, Linzhen, Madongchuan, Nanniwan, Pine Forest, Wanhua, Zaoyuan, Heyuping and Liqu. The Wanhua area is 16 km long from north to south, 25 km wide from east to west, and covers an area of about 400 km2 (Figure 1). Regionally, the study area is located in the southeastern part of the Yishan Slope in the Ordos Basin (Figure 1). This area is a gentle monocline sloping westward, and the formation dip is 0.5° to 1°. A series of low-amplitude nose-like uplifts dipping from east to west are developed on the slope. The superimposed areas of these nose-shaped uplifts and thick sand bodies indicate favorable areas for hydrocarbon enrichment.
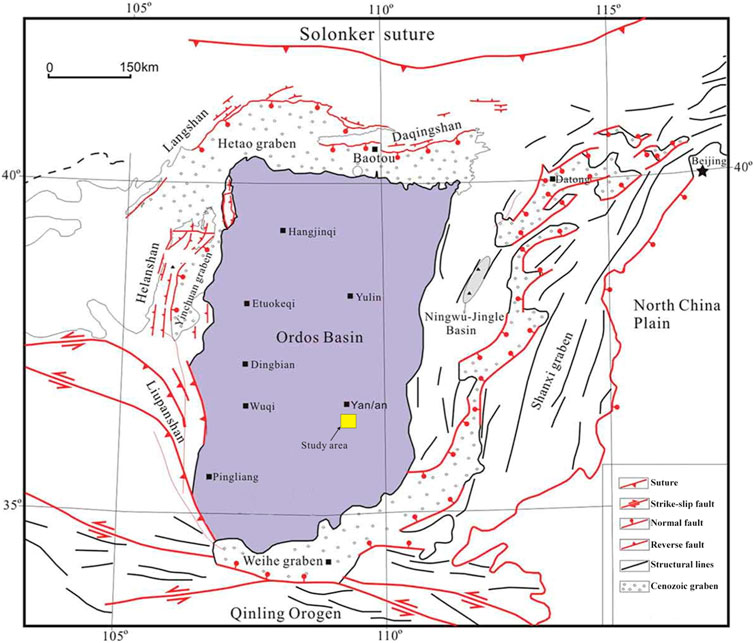
FIGURE 1. Location of the Wanhua area in the Ordos Basin (modified after Li et al., 2016).
The Chang 1 Member of the Upper Triassic Yanchang Formation in this area is the exploration target. Drilling revealed that continental freshwater lake delta deposits are developed in the Yanchang Formation. The stratigraphic sequences of the Yanchang Formation include a set of interbeds of gray-green, gray-black mudstone, argillaceous siltstone and gray-green, gray-white medium-fine feldspar sandstone. As the study area was eroded by the Ganshan Ancient River in the Jurassic period, the Chang 1 oil layer group was eroded to different degrees. The residual thickness of the Chang 1 oil layer group in the Yanchang Formation in this area varies greatly, and its thickness ranges from 25 to 90 m. (Figure 2)
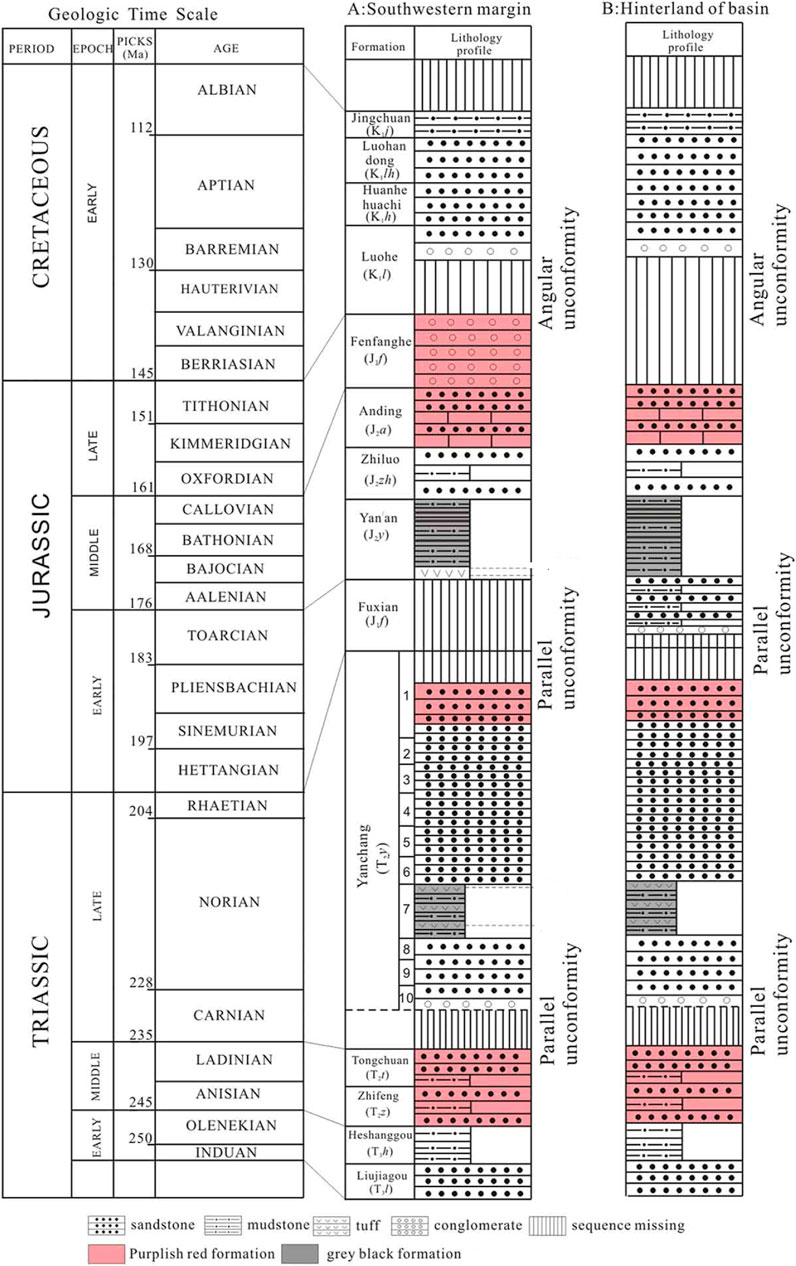
FIGURE 2. Division of stratigraphic units in the Ordos Basin (modified after Li et al., 2016). (A) The southwestern margin of the Ordos Basin; (B) Hinterland of the Ordos Basins.
3 Databases and methods
Core observations, physical property tests, thin section and scanning electron microscope observations were carried out on the wells of Nan 141, Nan 116, Nan 117, Nan 128, Xi 41, and Xi 63 in the study area. These wells are all exploratory wells, and the samples are all sandstone collected from the river channel.
The data used in this paper are from physical property, thin section, scanning electron microscope experiments and logging data. Among them, the physical property test adopts the overburden porosimeter CMS-300, and the test standard is SY/T 5336-2006. First, the samples were processed into small columns measuring 2.5 cm in diameter and 5 cm in diameter. Then, the samples were washed and dried for 2 h. High-pressure nitrogen gas is used to apply confining pressures. The test gas source is high purity helium. Then, the CMS300 rock autoanalyzer’s power and gas source were switched on to automatically measure the porosity and permeability of the samples. The measurements are based on the Boyle’s law (porosity) and the Darcy’s law for unsteady flows (permeability). The accuracy of CMS300 is higher than 2,412.9 Pa-cm3/min.
Thin section experiments are performed using a LEICA 4M research microscope and the test standard is SYT 5368-2016 (Li et al., 2012). The experimental temperature is 20°C and the relative humidity is 20%. Scanning electron microscope observations adopt a Tescan field emission electron microscope MAIA-3, and the test standard is GB/T 20307-2006. Based on the observations of thin section and scanning electron microscope of the Chang 1 Member in the study area, the petrology, pore types, and diagenesis of tight oil reservoirs have been systematically studied (Wang and Yang., 2017).
Single well logging data are used for stratigraphic divisions and sand body identifications (Li et al., 2012). On the basis of sand body identification, the effective thickness of sand bodies is also determined. There are argillaceous rocks or tight impermeable layers inside the oil layer, which have no effect on the acquisition of industrial oil flows and are deducted from the effective thickness. The argillaceous interlayers show low micro-electrode resistance value, no obvious amplitude difference, low concave resistivity, spontaneous potential and natural gamma values of abnormal amplitude return on the electrical measurement curves (Wang and Yang., 2017). The thickness of the physical property interlayers on the electrical measurement curves is lower than the effective thickness standard, and the micro-resistivity curves are close to overlap. The calcareous interlayers show high resistivity values, high peak bayonet-like microelectrodes, returning spontaneous potentials, and reduced acoustic wave time differences on logs. According to the resolution of the logging series, the effective thickness is higher than 0.4 m, and the critical thickness of the interlayers is higher than 0.2 m.
4 Results
4.1 Types of sedimentary facies
The sandstones in the Chang 1 Member in the study area are mainly light gray and gray-white, and a small proportion are light brown, gray-brown and yellow-green (Figures 3A,B). These sandstones are river channel deposits. However, mudstone, argillaceous siltstone and silty mudstone are mostly dark gray, black and gray-black, reflecting inter-channel or inter-distributary bay deposits.
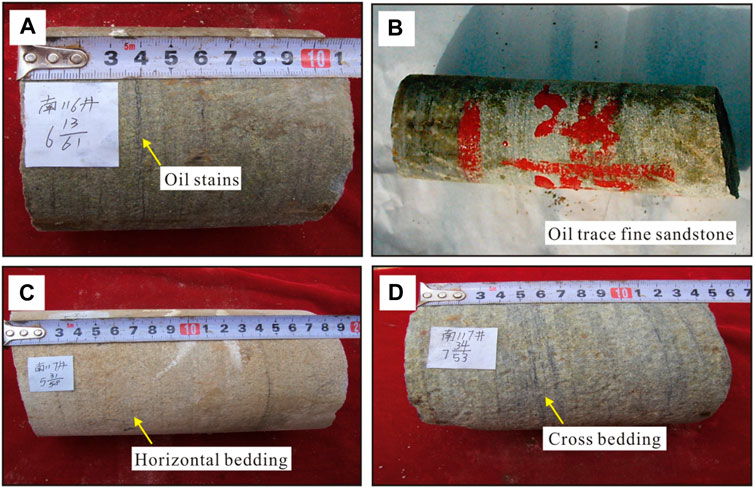
FIGURE 3. Core images of the Chang 1 Member sandstones in the study area. (A) Light gray oil traced fine sandstone, Well Nan116, 711.96–712.06 m; (B) Gray oil trace fine sandstone, Well Xi63, 726.31–726.57 m; (C) Parallel bedding, Well Nan117, light gray fine sandstone, 714.26–714.44 m; (D) Cross bedding, Well Nan117, light gray fine sandstone, 734.49–734.63 m.
The sedimentary structures in clastic rocks are the direct reflection of the sedimentary environment and hydrodynamic conditions in the rock. Parallel and cross beddings are widely developed in the Chang 1 Member sandstones in the study area (Figures 3C,D). Horizontal beddings are rectilinear and usually parallel to the bedding. It is a common type of bedding found in argillaceous rocks. Further, it reflects the deposition of suspended matter in a low-fluid form in a low-energy environments (e.g., inter-fluvial depressions, lacustrine and swamp environments). However, parallel beddings generally occur in rapids and high-energy environments, such as river channels, and often coexist with large cross beddings. In addition, cross-beddings can occur in sedimentary environments such as water distributary channels and delta front estuarine bars (Liu et al., 2009; Wang and Yang., 2017). The Chang 1 Member of the study area belongs to meandering river facies and is divided into distributary channel (channel retention, sand bar) and interdistributary channel, and floodplain microfacies. The skeleton sand bodies are sandy deposits developed in the middle part of the river channel (Figure 3).
4.2 Reservoir petrological characteristics
The lithology of the Chang 1 reservoir in this area is mainly sandy fine-grained feldspar sandstone. As shown in Figure 4, the different samples have similar petrological characteristics. The main mineral components of the Chang 1 sandstones are quartz, accounting for 42.0%–53.0%, with an average of 47.55%; the second is feldspar, accounting for 40.0%–50.0%, with an average of 43.95%; the content of debris is between 4.0% and 11.0%, with an average of 7%; in addition, the content of mica varies little, ranging from 1.0% to 3.0%, with an average of 1.3%. The debris components in the samples are mainly metamorphic rock debris, followed by igneous rock debris and a small amount of sedimentary rock debris.
The content of interstitials in the Chang 1 Member reservoirs in this area ranges from 2.0% to 18.0%, with an average of 8.2%; the main components of cements are ankerite, turbidite, dolomite and calcite, and their average contents are 4.05%, 1.4%, 1%, 0.625%, and 0.5%, respectively; and the matrix are mainly mud components, and the average content is 1.4%.
In addition, the sandstones of the Chang 1 Member in this area have relatively uniform clastic particles, and the main particle size between 0.15 and 0.35 mm accounts for more than 85% of all samples; the particles mainly have medium sorting, followed by good sorting; the particles have sub-edge-sub-circular roundness; the grains are in point or point-line contact; and pore type and film-pore type cementations are mainly developed.
For the relation between sedimentary facies and reservoir petrological characteristics, it is found that fine sandstones are mainly developed in the middle section of the river channel, and fine-siltstones are developed in the flanks of the river channel.
4.3 Diagenesis
The Chang 1 sandstone of the Yanchang Formation in the study area is a typical ultra-low porosity-ultra-low permeability reservoir, and its diagenetic process is very complicated. In the process of burial diagenesis, various diagenesis have certain effects on the primary pores or preservation or destruction of sandstone and the development of secondary pores. It has experienced destructive diagenesis such as mechanical compaction, pressure solution and cementation, and has experienced constructive diagenesis such as dissolution (Li et al., 2016;).
4.3.1 Compaction and pressure solution
Mechanical compaction runs through the entire process of burial diagenetic stage, and is one of the main reasons for the reduction of sandstone porosity (Nabawy et al., 2022). The compaction-pressure-dissolution effect experienced by the Chang 1 sandstone reservoir is very strong. Compaction rotates, orients, deforms the detrital particles, and mechanical fracture occurs in rigid particles. Moreover, line, surface, asperity and suture contacts appeared in the detritus particles under pressure. For example, the bending deformation of mudstone debris and mica is even squeezed into the intergranular pores to form a false matrix. In particular, mica deformed by strong compaction can block pores and throats (Figure 5). In the later stage of compaction, the main clay mineral in the feldspar sandstones, the chlorite film, is precipitated. Chlorite is attached to the surface of detrital particles in the form of films or combs and blocks pores and throats (Wang et al., 2019; Su et al., 2022). Thin-section analysis shows that sandstones with high content of plastic particles such as mica and mudstone debris are significantly affected by mechanical compaction, and their surface porosities are generally low (El-Gendy et al., 2022).
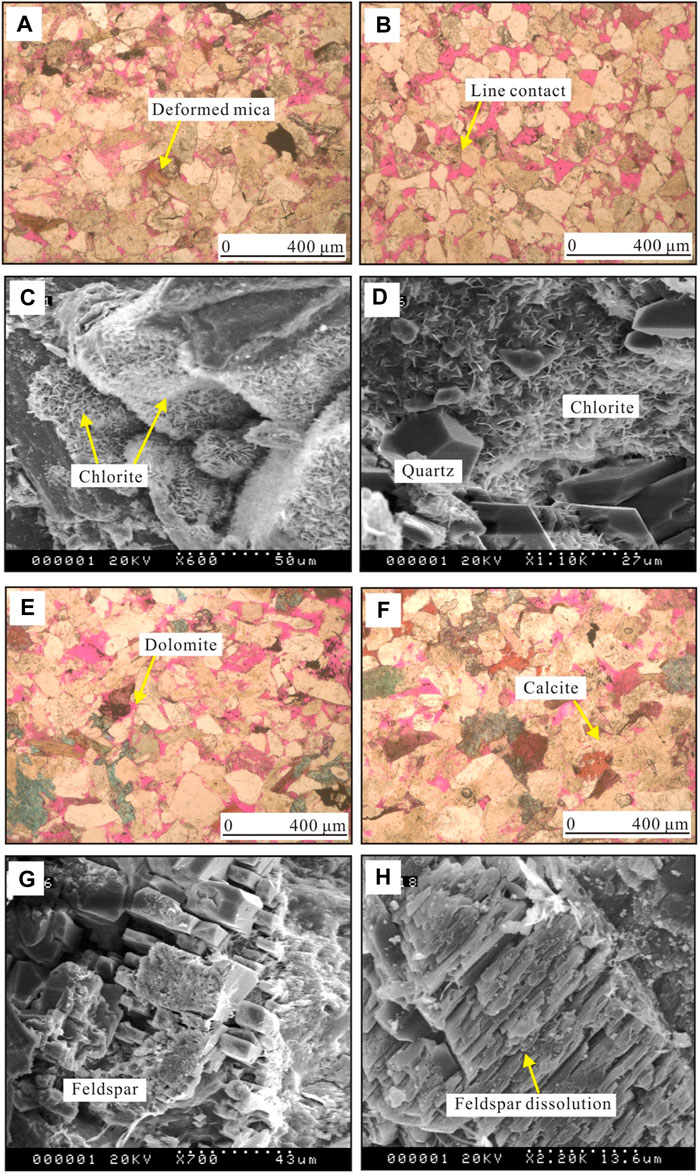
FIGURE 5. Microstructural characteristics of the Chang 1 Member sandstone in the study area. (A) Deformed sheet mica, Well Nan141; (B) Mosaic and linear contact particles, Well Nan128; (C) Chlorite cementation, Well Nan141, 521.97–522.07 m; (D) Quartz-filled and chlorite-cemented intergranular pores, Well Nan128, 715.83–715.93 m; (E) Dolomite and ankerite cements with grain structures are filled in the intergranular pores, Well Nan128, 725.18–725.28 m; (F) Calcite and ankerite cements with grain structures are filled between particles, Well Xi41, 500.89–500.99 m; (G) Feldspar dissolution, Well Nan128, 715.83–715.93 m; (H) Feldspar dissolution, Well Xi41, 500.89–500.99 m.
As the overburden pressure increases, the compaction effect is gradually replaced by the pressure solution. Furthermore, the inter-particles contact changes from linear contact to concave-convex contact and mosaic cementation. Then, the secondary growth of feldspar and quartz leads to the reduction of the primary intergranular pores and the throat radius. Ultimately, the permeability of tight reservoirs is greatly reduced. Overall, the pressure-dissolution of the tight sandstone in the target layer resulted in the secondary increase of quartz and feldspar, and a large number of patchy dense mosaic structures could be observed under the microscope (Figures 5A,B). The porosity of the Chang 1 sandstones was greatly lost due to compaction and solution. The remaining porosity of the reservoir is only about half of the original porosity. Compaction is the main reason for the sharp decrease of rock porosity. The original porosity of the target layer is calculated according to the original porosity model of unconsolidated sandstone proposed by Beard (Wang and Yang., 2017), which is 25%.
4.3.2 Cementation
The cements in the target sandstones mainly include authigenic clay minerals, carbonate minerals, authigenic quartz feldspar and turbidite. The main types of cementation include calcite cementation, chlorite film and chlorite filling cementation, overgrowth of quartz and feldspar. They are precipitated between the detrital particles, or appear in the form of secondary particle enlargement, or in the form of mutual metasomatism and metasomatic detrital particles.
4.3.2.1 Argillaceous cementation
The argillaceous cementation is mainly clay mineral cementation, including authigenic clay minerals and a small amount of terrigenous clay (Abdel-Fattah et al., 2022). Terrigenous clay minerals mainly occur in the early diagenetic stage and are common in siltstone; while the composition of authigenic clay minerals is quite simple, which is common in medium and fine sandstones.
Under the microscope, argillaceous usually fills the intergranular pores in the form of thin films, micrites and scales. Clay cements include chlorite, kaolinite, illite, and imon mixed layer. Among them, the chlorite film is the main cement in the early diagenetic A-stage sandstone. From the X-ray diffraction test results (Table 1), the Chang 1 Member has the highest content of chlorite, followed by kaolinite, illite, and imon mixed layer.
The formation of chlorite is caused by the combination of biotite and feldspar in terrigenous debris after weathering. It was found that the chloriteization of biotite debris in the target layer is very significant. The rich content of mica debris is the main reason for the good development of chlorite film. Moreover, under the microscope, it can be seen that a yellow film is formed around the detrital particles, which grows perpendicular to the detrital wall and has different degrees of metasomatism to the detrital particles. Most chlorites are attached to the grain surface in leaf-like or “pompom-like” aggregates (Figures 5C,D), and the content of chlorite aggregates in the medium and fine sandstones is less than that in siltstones.
In the early stage of diagenesis, the chlorite film can effectively inhibit the regrowth or secondary enlargement of quartz, feldspar, etc., and a large number of primary pores are retained (Figures 5C,D). At the same time, the covering of chlorite film on the particle surface leads to the reduction of the pore throat radius. The thickness of the chlorite films in the target layer is 0–20 μm, which causes the pores and throats to be blocked, and the permeability of the sandstone is reduced. At the same time, the development of a large number of micropores complicates the pore structures inside the sandstone (Figures 5C,D).
Kaolinite is the product of feldspar weathering. They generally appear as pseudo-hexagonal wafers under the microscope, while their aggregates usually exist in the form of book pages or worms. The content of kaolinite in the authigenic clay minerals in the target layer is relatively high (Table 1). In addition, the illite cements can be observed to grow on the pore walls in a bridging form, which results in a significant reduction in rock porosity and permeability.
4.3.2.2 Siliceous cementation
The secondary growth of quartz is formed by the coaxial growth of dissolved SiO2 in the pore water on the surface of the detrital quartz. Early secondary enlarged quartz formed before or during compaction. The quartz overgrowth is mainly controlled by the limitation of the size of the growth space (Khan et al., 2020). It can surround the entire detrital quartz, or distributed only in local quartz grains. Therefore, secondary quartz usually exists in the form of enlarged edges or fills the pores. Type I secondary enlarged quartz is dispersed on the pore surface in the form of point-like aggregates. Type II secondary enlarged quartz has a more continuous distribution on the pore surface. Type II secondary quartz is mainly developed in the target layer.
4.3.2.3 Carbonate cementation
The carbonate cements in the target layer include ankerite, calcite, and iron-bearing calcite cements, among which ankerite cements are the main ones (Figures 5E,F). The calcite cements are divided into early and late stages. Among them, the early calcite cements are not as common as the late ferrocalcite cements under the microscope. In the early calcite-cemented detrital grains, only a very small amount of detrital quartz experienced secondary enlargement at the edges. In the target layer, late-stage calcite cementation is common, which is typically characterized by pore filling and is irregularly distributed among the clastic particles.
4.3.3 Dissolution
According to this study, the dissolution in the Chang 1 Member reservoir is strong, and the dissolution is the main factor for the formation of secondary pores and the improvement of reservoir storage properties and permeability. Dissolution in the target layer occurred in the late stage of diagenesis. At this time, the organic matter is in the low-mature to mature stage, and the organic matter in the source rock begins to decarboxylate and release CO2. When these substances enter the pore fluids, they make the formation water acidic. This type of acidic fluid, rich in organic and inorganic acids, is the main driving force for the dissolution of reservoir detrital components (Haile et al., 2018; Liu et al., 2020). Overall, the intergranular and intragranular dissolved pores formed by dissolution are the most important secondary storage spaces of feldspar sandstones in this area (Figures 5G,H).
According to the observations of the cast thin section and scanning electron microscope in this area, a large number of dissolution-type secondary pores were formed in the sandstones during the middle diagenetic stage, and the pore structures of the sandstone reservoir were effectively improved. Through the analysis of the development characteristics of microscopic mineral components, the Chang 1 Member in the study area has gone through the syngeneic stage→early diagenetic stage→intermediate diagenetic stage A. It is in the middle diagenetic stage A now.
4.4 Physical characteristics
According to the statistical results of the physical property data of 51 samples in the area, the porosity of the Chang 1 reservoir is distributed between 6.6% and 20.1%, with an average value of 12.5%. The permeability of the samples is distributed between 0.05 and 26.9 mD, with an average of 2.4 mD. There is an obvious positive correlation between the porosity and permeability of the Chang 1 reservoir in the study area (Figure 6).
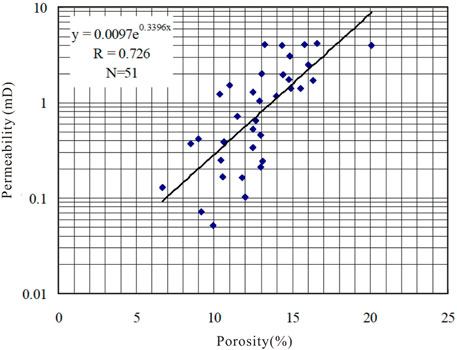
FIGURE 6. Relationship between porosity and permeability of sandstone in the Chang 1 Member of the study area.
The main sedimentary microfacies types of the target layer include distributary channel, estuary bar, interdistributary bay and floodplain. The relationship between sedimentary microfacies and reservoir porosity is compared, and the results are shown in Figure 7. According to statistics, the distribution intervals of the average porosity of the distributary channel, estuary bar, interdistributary bay and floodplain facies are 10%–17%, 12.5%–20%, 6.7%–10%, and less than 7%, respectively. Their average values are 14.2%, 14.9%, 8.6%, and 5.5%, respectively.
4.5 Pore types
In this study, the surface ratios of the samples were obtained from thin section tests. The LEICA 4M research microscope was used to automatically scan the micrographs of the samples. Then, the surface ratio of the samples was calculated. According to the observation and statistics of the cast thin section and electron microscope scanning of the target sandstone, the main types of pores developed in the sandstone of the Chang 1 Member are intergranular dissolved pores and residual intergranular pores (Figure 8). There are 100 samples in total.
The dissolved pores in the target layer are mainly intergranular and feldspar dissolved pores. Intergranular dissolution pores are pores formed by the dissolution of interstitials (clay, carbonate minerals) and the edges of clastic particles such as feldspar and debris. Part of the primary pores were recovered and enlarged or some new secondary pores were formed. Statistics show that the intergranular dissolution pores in the Chang 1 Member reservoir in the study area account for 66.7%–91.7% of the total surface porosity, with an average of 82.9%. Dissolution greatly improves the physical properties of the reservoir.
Residual intergranular pores refer to the pores remaining after the primary intergranular pores have been subjected to mechanical compaction or secondary quartz enlargement. The intergranular pores in the Chang 1 reservoir group in the study area are an important type of pores. They account for 5.0%–24.0% of the total surface porosity, with an average of 10.3%.
5 Discussion
The distribution of crude oil in tight reservoirs is actually the result of the coupling of various geological elements (Enayati-Bidgoli and Saemi., 2019). This study believes that the accumulation of hydrocarbons in the Chang 1 Member is mainly affected by factors of superimposed sand bodies, nose-like structures, sand body thickness, and eroded paleogeomorphology.
5.1 Effects of superimposed sand bodies and fractures on hydrocarbon migration
The oil and gas from the Chang 7 source layer in the oil-generating center in the southwest migrated under the action of buoyancy and capillary force when migrating along the lipophilic residual path network in contact with oil for a long time. Through the effective migration channels in the transport layer, the hydrocarbons migrate upward in a stepwise manner along the main fluid flow direction. When hydrocarbons encounter the top caprock and there is good shielding conditions in its updip direction, hydrocarbons can accumulate on a large scale (Figure 9). Large-scale vertical fractures mainly developed in the Chang 1 Member, and the longitudinal extension of these fractures can reach tens of meters (Figure 9). For the nose-like fold structures, oil and gas first accumulated linearly along the structural ridges where the fracture system was well developed, and then gradually accumulated outward along the areas with good physical properties and low capillary resistance. Finally, a strip-shaped hydrocarbon enrichment belt extending along the axis of the nose-like fold belt is formed on the plane. Based on this study, it is believed that the hydrocarbon migration and accumulation mode of the Chang 1 reservoir belongs to the ladder-like climbing migration + structural ridge accumulation type (Figure 10).
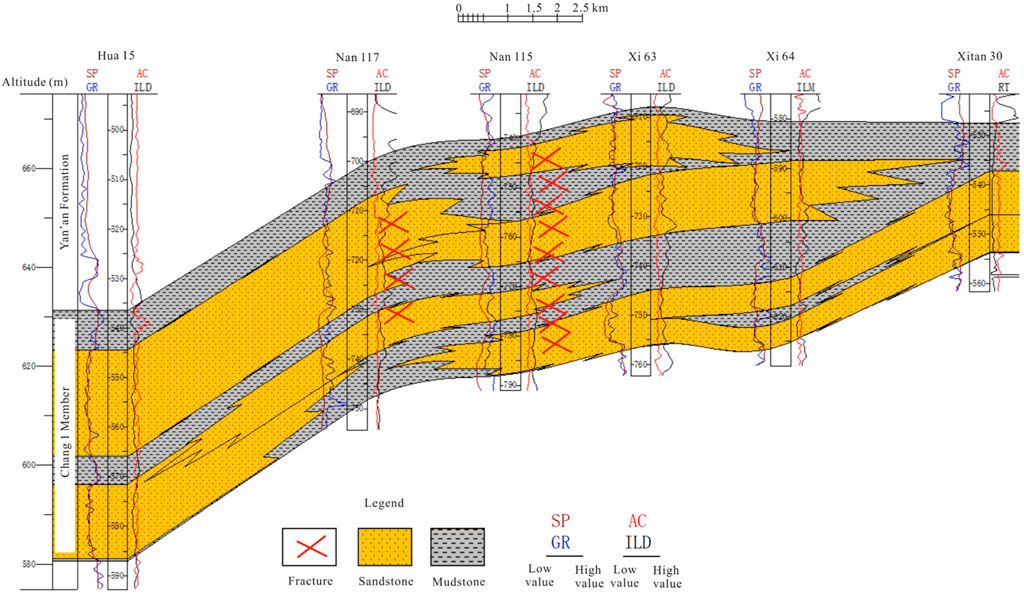
FIGURE 9. Sand body profile of connecting wells in Chang 1 Member of the study area. SP stands for spontaneous potential; GR stands for natural gamma; AC stands for acoustic time difference; ILD stands for deep lateral resistivity; ILM stands for medium induced resistivity; RT stands for true resistivity.
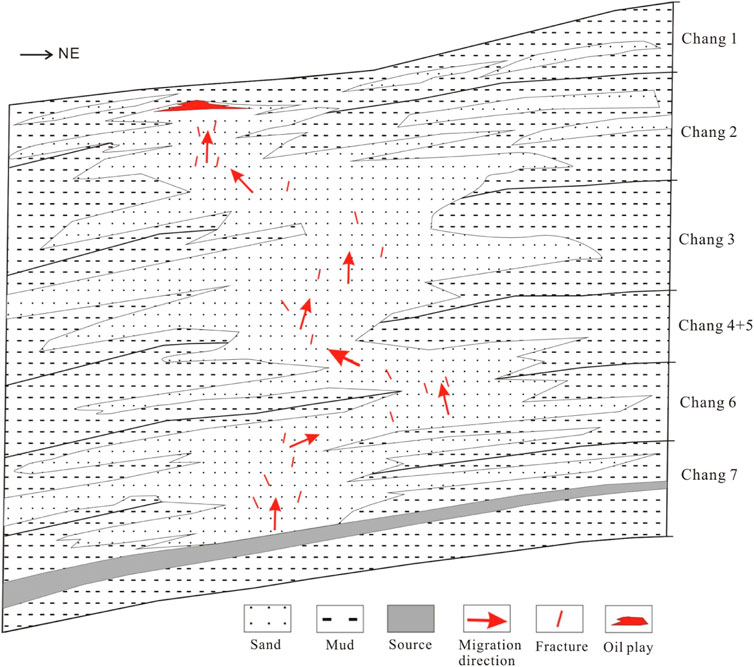
FIGURE 10. Hydrocarbon migration model of the Yanchang Formation in the study area. The superimposed sand bodies and fractures constitute channels for long-term continuous migration of hydrocarbons.
The Ordos Basin is a craton basin, and tectonic activities in the Mesozoic strata are not active. In the past, structural geologists believed that the Yishan Slope in the Ordos Basin was free of any faults and fractures. However, with the in-depth study of a large number of low-amplitude structures in the Ordos Basin, it has been found that the Mesozoic strata of the Yishan Slope have multiple active records of tectonic activities, and a large number of fractures have been discovered.
The study area is located in the southeastern part of the Yishan Slope, the first-level structural unit of the Ordos Basin. In the thin section observations, some micro-fractures were found in the sandstones of the Chang 1 Member (Figure 11). Sand bodies with relatively developed fractures are also important channels for hydrocarbon migration.
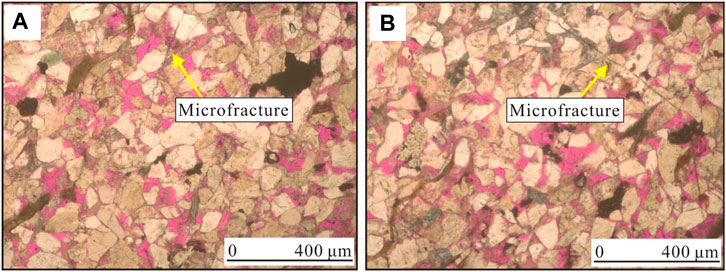
FIGURE 11. Development characteristics of micro-fractures in sandstone of the Chang 1 Member in the study area. Well Nan 141. (A) 521.97 m; (B) 522.07 m.
5.2 Effects of nose-like uplifts on hydrocarbon accumulation
The residual thickness of the Chang 1 oil layer group in the study area varies greatly, ranging from 25 to 90 m, and its distribution direction is NW-SE. Due to the uneven distribution of sand and mudstone in the formation, the Chang 1 oil layer group is prone to differential compaction. Furthermore, the nose-like structures are relatively developed. On the regional west-dipping monoclinic structural background, the NW-SE or nearly EW-striking nose-like uplifts play a very important role in controlling the accumulation of crude oil in the Chang 1 Member. The discovered oil enrichment areas in the Chang 1 Member are all located in the nose-shaped uplifts with large structural amplitude and long extension distance. That is, the oil reservoir is located in the nose-shaped uplifts in the line of Well Xitan27-3 ∼ Well Xitan7 ∼ Well Nan127 ∼ Well Nan128 ∼ Well Xi63. Its structure undulates about 10–40 m, spreads about 5 km wide, and extends about 9.5 km in length. The nose-shaped uplifts are well developed in the top structure of the main sand bodies of the oil reservoir group. In addition, the oil reservoir is mainly distributed along the axial structural ridges of the nose-shaped uplifts. The main reason is that, on the one hand, the fractures formed at the axis of the nose-like fold are favorable for oil and gas migration; on the other hand, there are differences in the hydrocarbon accumulation capacity of different structural units such as monoclinic, nose-like uplift and structural high point. In the case of consistent oil and gas migration, oil and gas molecules will choose the shortest path (ie, the vertical contour direction) when they migrate upwards through buoyancy (Wang et al., 2021). Therefore, in general, the hydrocarbon accumulation capacity of different structural units such as monoclinic, nose-like uplift, and structural high point will change from weak to strong (Figure 12).
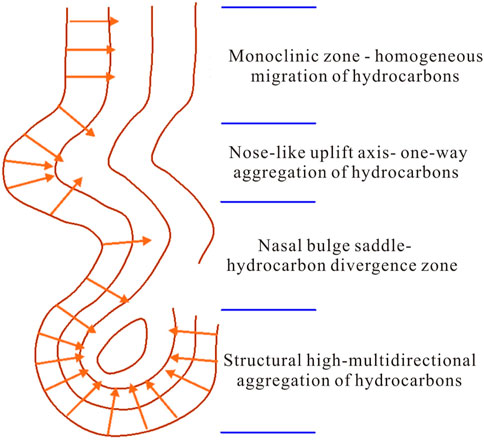
FIGURE 12. Schematic diagram of hydrocarbon migration in different structural units of the Yanchang Formation.
In conclusion, the Chang 1 reservoir in the study area is obviously controlled by the nose-like structures. The nose-like structure is an important factor for the accumulation and high production of oil and gas in the Chang 1 oil layer group in the study area.
5.3 Effects of dominant facies and eroded paleo-highlands on hydrocarbon accumulation
Sand body thickness is an important controlling factor for large-scale hydrocarbon accumulation (Zhang et al., 2017; Yin et al., 2020). At present, the discovered oil layers in the Chang 1 Member are all distributed in the areas with sand body thickness greater than 20 m. Most of the sand bodies have a thickness of 25–40 m, and the corresponding effective thickness is in the range of 2–6 m (Figure 13). In addition, the crude oil in Chang 1 Member is distributed in the area with sandstone ratio greater than 0.5. The sand bodies in these areas are thicker and have better physical properties, and the connectivity between the sand bodies is better as well. The good connectivity of sand bodies ensures good migration channels, so that oil can accumulate in the upper part of the Chang 1 Member where there are good shielding conditions. The river channel sedimentary areas with sandstone ratio greater than 0.5 is the dominant facies areas of the Chang 1 oil layer group in the study area.
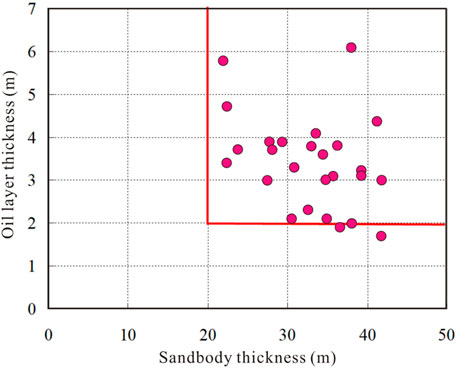
FIGURE 13. Relationship between sandstone thickness and effective thickness of the Chang 1 Member in the study area.
The eroded highlands are the topographical units with the highest topography. The study area is located in the east of the Ganshan ancient river. The flow direction of the Ganshan ancient river is from west to east, so that the upper strata of the Yanchang Formation are less eroded in the east of the ancient river than in the west. The residual formation thickness of the Chang 1 oil layer group in the study area is 25–90 m, so the thickness varies greatly. The distribution direction of the isopachous sand bodies is mainly in NW-SE striking, and the local striking is in NEE-SW and nearly SN directions. It reflects that the main channel of the Ganshan Ancient River in the study area is northwest-southeast.
Previous studies believed that paleotopography had an important influence on the distribution of oil and gas under the erosion surface. The paleogeomorphic units with higher topography are the main areas for oil and gas enrichment. Therefore, the eroded highlands, slope areas and residual hills are the best places for hydrocarbon accumulation in the upper strata of the Yanchang Formation. This study found that the superimposed position of dominant facies and eroded residual hills is more conducive to the accumulation of crude oil in the Chang 1 Member of the study area.
6 Conclusion
1) The Chang 1 Member sandstone in the Wanhua area is a typical ultra-low porosity-ultra-low permeability reservoir. The strong pressure solution caused secondary enlargement of quartz and feldspar and the formation of patchy dense mosaic structures. The original porosity of the target layer is 25%. Statistics show that the intergranular dissolution pores in the Chang 1 Member reservoir in the study area account for 66.7%–91.7% of the total surface porosity, with an average of 82.9%. Meanwhile, the intergranular pores account for 5.0%–24.0% of the total surface porosity, with an average of 10.3%. Dissolution is the main factor for the formation of secondary pores and the improvement of reservoir physical properties.
2) Superimposed sand bodies, nose-shaped uplifts, dominant facies and eroded paleo-highlands have significant effects on the hydrocarbon accumulation. The hydrocarbon migration and accumulation mode of the Chang 1 reservoir belongs to the ladder-like climbing migration + structural ridge accumulation type. In addition, sand body thickness is an important controlling factor for the hydrocarbon accumulation.
(3) At present, the discovered crude oil in the Chang 1 Member is distributed in the areas with thick sand bodies (>20 m), and most of the sand bodies have a thickness in the range of 25–40 m, and the effective thickness is in the range of 2–6 m. In addition, the eroded highlands are the highest topographic units, they are favorable areas for the large-scale accumulation of oil and gas.
Data availability statement
The original contributions presented in the study are included in the article/Supplementary Material, further inquiries can be directed to the corresponding author.
Author contributions
CJ is responsible for the idea and writing of this paper, and CX, MS, XL, and LW are responsible for the experiments and analysis.
Conflict of interest
Authors CJ, MS, and LW were employed by the company Shaanxi Yanchang Petroleum (Group) Corp. Ltd. and authors CX and XL were employed by the Fifth Oil Production Plant of Petrochina Changqing Oil Field Company.
Publisher’s note
All claims expressed in this article are solely those of the authors and do not necessarily represent those of their affiliated organizations, or those of the publisher, the editors and the reviewers. Any product that may be evaluated in this article, or claim that may be made by its manufacturer, is not guaranteed or endorsed by the publisher.
References
Abdel-Fattah, M., Sen, S., Abuzied, S., Abioui, M., Radwan, A., and Benssaou, M. (2022). Facies analysis and petrophysical investigation of the late miocene abu madi sandstones gas reservoirs from offshore baltim east field (nile delta, Egypt). Mar. Petroleum Geol. 137. doi:10.1016/j.marpetgeo.2021.105501105501
Asante-Okyere, S., Ziggah, Y. Y., and Marfo, S. A. (2021). Improved total organic carbon convolutional neural network model based on mineralogy and geophysical well log data. Unconv. Resour. 1, 1–8. doi:10.1016/j.uncres.2021.04.001
Bukar, M., Worden, R., Bukar, S., and Shell, P. (2021). Diagenesis and its controls on reservoir quality of the Tambar oil field, Norwegian North Sea. Energy Geosci. 2 (1), 10–31. doi:10.1016/j.engeos.2020.07.002
Cui, X., and Radwan, A. E. (2022). Coupling relationship between current in-situ stress and natural fractures of continental tight sandstone oil reservoirs. Interpretation 10 (3), SF9–SF21. doi:10.1190/INT-2021-0200.1
El-Gendy, N., Radwan, A. E., Waziry, M., Dodd, T., and Barakat, M. (2022). An integrated sedimentological, rock typing, image logs, and artificial neural networks analysis for reservoir quality assessment of the heterogeneous fluvial-deltaic Messinian Abu Madi reservoirs, Salma field, onshore East Nile Delta, Egypt. Mar. Petroleum Geol. 145. doi:10.1016/j.marpetgeo.2022.105910105910
Enayati-Bidgoli, A., and Saemi, E. (2019). Effects of late diagenesis on primary reservoir quality of a quartz arenite unit: A case study from the lower cretaceous successions of SW Iran. Pet. Sci. 16 (2), 267–284. doi:10.1007/s12182-019-0306-x
Haile, B. G., Klausen, T. G., Czarniecka, U., Xi, K., Jahren, J., and Hellevang, H. (2018). How are diagenesis and reservoir quality linked to depositional facies? A deltaic succession, edgeøya, svalbard. Mar. Petroleum Geol. 92, 519–546.
Huang, Y. G., Chen, Z. Q., Wignall, P. B., Grasby, S. E., Zhao, L. S., Wang, X. D., et al. (2019). Biotic responses to volatile volcanism and environmental stresses over the Guadalupian-Lopingian (Permian) transition. Geology 47, 175–178. doi:10.1130/G45283.1
Jiang, W., Zhang, P., Li, D., Li, Z., Wang, J., Duan, Y., et al. (2022). Reservoir characteristics and gas production potential of deep coalbed methane: Insights from the No. 15 coal seam in shouyang block, Qinshui Basin, China. Unconv. Resour. 2, 12–20. doi:10.1016/j.uncres.2022.06.001
Katz, B., Gao, L., Little, J., and Zhao, Y. R. (2021). Geology still matters – unconventional petroleum system disappointments and failures. Unconv. Resour. 1, 18–38. doi:10.1016/j.uncres.2021.12.001
Khan, E. U., Saleem, M., Naseem, A. A., Ahmad, W., Yaseen, M., and Khan, T. U. (2020). Microfacies analysis, diagenetic overprints, geochemistry, and reservoir quality of the jurassic samanasuk. Formation at the kahi section, nizampur basin, NW himalayas, Pakistan. Carbonates Evaporites 35 (3), 95–96. doi:10.1007/s13146-020-00622-4
Li, S. H., Fang, G. Q., Yang, J. L., Liao, J. B., and Fan, J. M. (2012). Origin of ultra-low permeability reservoirs in Ordos Basin and its significance. Lithol. Reserv. 24 (6), 32–37. doi:10.3969/j.issn.1673-8926.2012.06.008
Li, Z., Xi, S., Feng, S., and Liu, X. (2016). 150 million year history of north China craton disruption preserved in mesozoic sediments of the Ordos Basin. Int. Geol. Rev. 58 (11), 1417–1442. doi:10.1080/00206814.2016.1166351
Liao, J. B., Xi, A. H., Liang, S. J., Zhou, X. P., Li, Z. Y., Di, J., et al. (2020). Genetic mechanisms of deep-water massive sandstones in continental lake basins and their significance in micro–nano reservoir storage systems: A case study of the Yanchang Formation in the Ordos Basin. Nanotechnol. Rev. 9, 489–503. doi:10.1515/ntrev-2020-0040
Liu, B., Yang, Y., Li, J., Chi, Y., Li, J., and Fu, X. (2020). Stress sensitivity of tight reservoirs and its effect on oil saturation: A case study of lower cretaceous tight clastic reservoirs in the hailar basin, northeast China. J. Petroleum Sci. Eng. 184. doi:10.1016/j.petrol.2019.106484106484
Liu, C., Zhao, H., and Sun, Y. (2009). Tectonic background of Ordos Basin and its controlling role for basin evolution and energy mineral deposits. Energy Explor. Exploitation 27 (1), 15–27. doi:10.1260/014459809788708219
Nabawy, B., Abudeif, A., Masoud, M., and Radwan, A. (2022). An integrated workflow for petrophysical characterization, microfacies analysis, and diagenetic attributes of the Lower Jurassic type section in northeastern Africa margin: Implications for subsurface gas prospection. Mar. Petroleum Geol. 140. doi:10.1016/j.marpetgeo.2022.105678105678
Radwan, A. E. (2022). “Chapter Two - three-dimensional gas property geological modeling and simulation,” in Sustainable geoscience for natural gas sub-surface systems. Editors D. A. Wood, and J. Cai (Elsevier), 29–45. Chapter 2 in. doi:10.1016/B978-0-323-85465-8.00011-X
Su, Y., Zha, M., Jiang, L., Ding, X., Qu, J., Jin, J., et al. (2022). Pore structure and fluid distribution of tight sandstone by the combined use of SEM, MICP and X-ray micro-CT. J. Petroleum Sci. Eng. 208, 109241–109245. doi:10.1016/j.petrol.2021.109241
Sun, Y., Chen, C., and Ma, S. Z. (2013). Hydrocarbon migration mechanism and accumulation models of the fuyu oil layer in southern fuxin uplift, songliao basin. Geol. Rev. 59 (3), 501–509.
Sun, Y., Zhang, J. Y., and Ma, S. Z. (2014). Analysis of high-resolution sequence stratigraphy of Fuyu oil layer in the southern Fuxin Uplift. Geol. Rev. 3, 473–480.
Wang, A., Zhong, D. K., Zhu, H. H., Guo, L., Zheng, X., Jiang, Y., et al. (2019). Depositional and diagenetic controls on the reservoir quality of Upper Triassic Chang-7 tight oil sandstones, southwestern Ordos basin, China. Geosci. J. 23 (3), 471–488.
Wang, J., Fu, Y., Yan, Z., Fu, J., Xie, J., Li, K., et al. (2021). Influence of sedimentation and diagenesis on reservoir physical properties: A case study of the funing formation, subei basin, eastern China. Front. Earth Sci. 45, 892–908. doi:10.1007/s11707-020-0836-y
Wang, Z., and Yang, G. Q. (2017). Some research hot spots of tight oil and gas reservoir. Chin. foreign energy 16 (8), 37–42.
Yin, S., Dong, Li., Yang, Xia., and Wang, R. (2020). Experimental investigation of the petrophysical properties, minerals, elements and pore structures in tight sandstones. J. Nat. Gas Sci. Eng. 76 (1), 1–14. doi:10.1016/j.jngse.2020.103189
Zhang, B., Shen, B., and Zhang, J. (2020). Experimental study of edge-opened cracks propagation in rock-like materials. J. Min. Strata Control Eng. 2 (3). doi:10.13532/j.jmsce.cn10-1638/td.20200313.001033035
Zhang, Q., Wu, X., Radwan, A. E., Wang, B., Wang, K., Tian, H., et al. (2022). Diagenesis of continental tight sandstone and its control on reservoir quality: A case study of the quan 3 member of the cretaceous quantou formation, fuxin uplift, songliao basin. Mar. Petroleum Geol. 145. doi:10.1016/j.marpetgeo.2022.105883105883
Zhang, Q., Zhu, X. M., Steel, R. J., Wang, G., and Ji, H. (2014). Fluid inclusions and their application in hydrocarbon history and genesis. Petroleum Sci. Technol. 32, 2911–2920. doi:10.1080/10916466.2014.933976
Zhang, Y., Bao, Z., Zhao, Y., Jiang, L., and Gong, F. (2017). Diagenesis and its controls on reservoir properties and hydrocarbon potential in tight sandstone: A case study from the upper triassic Chang 7 oil group of Yanchang Formation, Ordos Basin, China. Arab. J. Geosci. 10 (11), 234–240. doi:10.1007/s12517-017-3023-z
Keywords: Yanchang formation, reservoir properties, hydrocarbon accumulation, pore structure, sandstone thickness
Citation: Ji C, Xue C, Sun M, Li X and Wang L (2023) Reservoir properties and hydrocarbon enrichment law of Chang 1 oil layer group in Yanchang Formation, Wanhua area, Ordos Basin. Front. Earth Sci. 10:1023844. doi: 10.3389/feart.2022.1023844
Received: 20 August 2022; Accepted: 31 October 2022;
Published: 17 January 2023.
Edited by:
Wenlong Ding, China University of Geosciences, ChinaReviewed by:
Jianguo Zhang, China University of Geosciences, ChinaXinghua Wang, SINOPEC Petroleum Exploration and Production Research Institute, China
Ahmed E. Radwan, Jagiellonian University, Poland
Copyright © 2023 Ji, Xue, Sun, Li and Wang. This is an open-access article distributed under the terms of the Creative Commons Attribution License (CC BY). The use, distribution or reproduction in other forums is permitted, provided the original author(s) and the copyright owner(s) are credited and that the original publication in this journal is cited, in accordance with accepted academic practice. No use, distribution or reproduction is permitted which does not comply with these terms.
*Correspondence: Chaohui Ji, amljaGFvaHVpMzNAMTYzLmNvbQ==, OTgwNjQ4Mzg2QHFxLmNvbQ==