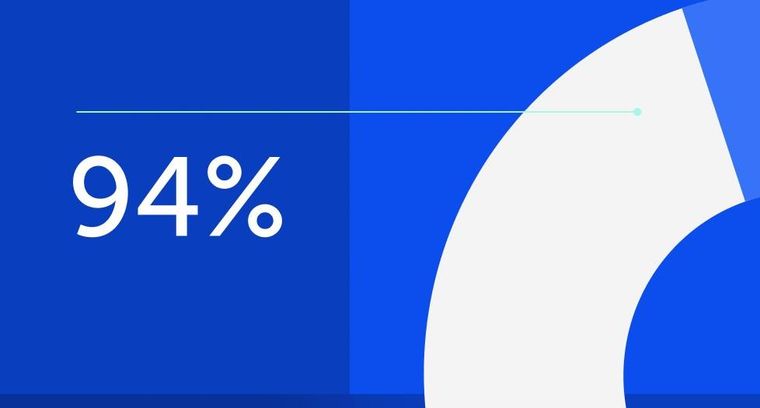
94% of researchers rate our articles as excellent or good
Learn more about the work of our research integrity team to safeguard the quality of each article we publish.
Find out more
ORIGINAL RESEARCH article
Front. Earth Sci., 11 November 2022
Sec. Structural Geology and Tectonics
Volume 10 - 2022 | https://doi.org/10.3389/feart.2022.1018737
We investigated the Late Quaternary activity of a major, crustal fault affecting the southern sector of Central Apennines, i.e., the Roveto Valley Fault (also known as Liri Valley fault). This sector of the chain was hit by numerous M>5 historical seismic events. For some of these, e.g., the 1654 one (Mw 6.33), the causative seismogenic source has never been conclusively defined. Within this seismotectonic framework, the recent activity of the Roveto Valley Fault is still a matter of debate. Some authors defined its activity as ended in the Middle Pleistocene; others considered it as currently active and seismogenic at least in its southern portion. We collected new geologic and geomorphologic data along the eastern (left) flank of the Roveto Valley, where the fault crops out, and we identified evidence of displacement of alluvial fans that we attributed to the Early, Middle, and Late Pleistocene. Moreover, the analysis of the relationship between colluvial/detrital deposits, chronologically constrained by means of radiocarbon dating, allowed us to define the activation of the Roveto Valley fault also during historical times, that is, over the past few centuries. Evidence of this has been collected along a large sector of the fault trace for a length of some tens of kilometres. The results of our studies contribute to improve the knowledge of the seismotectonic setting of a large sector of the Central Apennines. Indeed, proving the current activity of the Roveto Valley fault casts new light on possible seismogenic sources of major seismicity of central Italy, potentially responsible for severe damage over a wide area and to relevant cities, Rome being among them.
The Central Apennines in peninsular Italy have been modeled by Quaternary tectonics manifested in the uplift of the chain and activation of normal faults. Persistency of extensional tectonics is indicated by the displacement of Late Quaternary sediments and landforms. Expression of the current tectonic regime is the rich seismic history reported in the modern catalogues (Rovida et al., 2022), including events of magnitudes larger than 6 (up to 7.1) both in the past (e.g., the 1349, 1703, and 1915 earthquakes) and in the current millennium (the 2009 and 2016 earthquakes).
Recent fault motions have been the object of modern investigations since the 1970s by combining Quaternary geology and geomorphology (Bosi and Bertini, 1970; Bosi, 1975). Paleoseismological investigations have been pervasively made along supposedly active structures since the end of the 1980s (Giraudi, 1989) to collect data on the Late Pleistocene–Holocene activation of faults.
During the past decades, geological studies on the current fault behavior have been dedicated to two interlaced themes of interest for engineering practices: 1) the identification of capable faults, having implications in planning the land use (Commissione tecnica per la Microzonazione Sismica, 2015) and 2) the definition of seismogenic sources, generally having a key role in evaluating potential seismic ground motion through seismic hazard assessment or shaking scenarios for engineering works (Bosi and Galadini, 1995). Within this framework, although databases of capable faults (ITHACA Working Group, 2019) and seismogenic sources (DISS Working Group, 2018) have been produced, available information on these items cannot be considered as conclusive for both 1) methodological aspects (Maceroni et al., 2021) and 2) knowledge on the activity of specific faults/sources. An example of this is the Roveto Valley Fault (hereafter RVF), the object of the present work, as its current activity and seismogenic behavior are still a debated matter. Carrara et al. (1995a, b) stated that displacements along the fault have been supposed only for the Early Quaternary, implying the current fault’s inactivity. By contrast, other authors considered the fault as active during the whole Quaternary (Roberts and Michetti, 2004; Papanikolaou et al., 2005), and more recently, recent activity has been demonstrated for the southern prolongation of the fault (i.e., the Posta Fibreno fault; Saroli et al., 2022), while further investigations defined the inactivity of the northernmost sector (Galadini et al., 2022). These aspects are by no means trivial as for the evaluations on the fault capability, since villages are settled along the fault (Pescosolido, Balsorano, San Vincenzo Valle Roveto, Morrea, Civita d’Antino, Capistrello, etc.) and local constraints on land-use planning may derive from the application of the guidelines on active and capable faults related to seismic microzonations (Figure 1A). Moreover, the attribution of activity or inactivity has paramount implications on the estimation of the seismogenic potential; e.g., a source potentially responsible for large-magnitude earthquakes in Central Italy may or may not be added to the current seismogenic datasets. The expected ground motion has, in turn, implications for the defense against earthquakes, considering that towns such as Avezzano and Sora, having about 40,000 and 25,000 inhabitants, respectively, are in the investigated area. Moreover, a large-magnitude event nucleated in this region might cause severe damage even to Rome, located few tens of km to the west, as already occurred in 1349 and 1915 (I 7–8 and 6–7 MCS, respectively; Locati et al., 2022), just to mention the two major historical earthquakes with the epicenter located in the investigated area.
FIGURE 1. (A) Details of the sheet 151 “Alatri” and sheet 152 “Sora” (mapping scale 1:100.000) (Carta Geologica d’Italia 1:100.000, 1941a, Carta Geologica d’Italia 1:100.000, 1941b) of the geological map of Italy of the southern Abruzzo and Lazio regions where the study area is located and details of the Campoli Appennino fan and the Balsorano Synthem (insets). (B) Digital terrain model (shaded relief) of the sector of the Central Apennines between the southern Abruzzo and the south-eastern Lazio regions; trace of the Roveto Valley–Posta Fibreno Fault, red dash-dotted line.
Starting from these premises, the evidence of Quaternary activity along the RVF is presented and discussed in this article.
Based on the obtained results, in the conclusions, we make inferences on the seismotectonic implications of our analysis, especially as for the possible relationship between the RVF activity and the large-magnitude historical seismic events which struck this region (and, inherently, which can occur in the future) over the past centuries.
The Quaternary activity of the RVF has been investigated by means of geological field analysis, aerial-photograph analysis, and stratigraphic investigations of the Quaternary continental sequences that deposited in the Roveto Valley, focusing on the middle-to-late Quaternary fault activity. With regard to the aero-photograph analysis, several aerial photos of the Italian Military Geographical Institute (IGM), taken in 1954 and 1956, were analyzed from morphotectonic and morphostratigraphic investigations morphotectonic and morphostratigraphic investigations. These allowed us to obtain preliminary information regarding the identification of the main fault trace and of minor splays that characterize the structural framework of the investigated tectonic structure. Furthermore, this analysis allowed us to characterize the morpholithostratigraphic setting of the area in which the fault is located. This is useful for understanding the chronological framework to which the detected continental units relate. Moreover, in order to achieve chronological constraints for the deposition of the detected continental units, especially for the late Quaternary units, we collected, where possible, samples of charcoal and of the organic-rich sediments to be dated by means of the 14C method. Dating was performed by Beta Analytics laboratory, adopting procedures described on the website at link https://www.radiocarbon.com/italiano/archeologia-lab.htm.
The NW–SE trending Roveto Valley, incised by the Liri River, is located in the western sector of the Central Apennines.
The valley is surrounded by mountain reliefs, reaching about 2,000 m in altitude, made of Meso-Cenozoic rocks of carbonate marine sequences. Within the valley, the silicoclastic Miocene turbidite sequence (clayey-arenaceous lithotypes) crops out extensively. Moreover, in few places, a polygenic conglomerate containing allogenic elements attributed to the Late Messinian–Early Pleistocene unconformably overlies the carbonate bedrock. The conglomerate represents the last deposit of a marine environment of the area (Cipollari and Cosentino, 1999; Saroli et al., 2003). Indeed, since the Late Pliocene–Early Pleistocene, the valley hosts continental sedimentary sequences such as slope, alluvial, alluvial fans, lake, and calcareous tufa deposits (Figure 1A).
In terms of tectonic evolution, this sector of the chain developed mainly since the Neogene, through the in-sequence north-eastward propagation of thrust faults, with a piggy-back style (Cipollari and Cosentino, 1999). The Simbruini–Ernici mountainous thrust front, a regional structure that bounds the western flank of the Roveto Valley, first activated in the early Messinian and was then involved during the late Messinian in an out-of-sequence structure, while the paleogeographic domain of the Marsica region was involved in the thrust front propagation (Cipollari et al., 1995; Cipollari and Cosentino, 1999; Saroli et al., 2003; Cosentino et al., 2010).
The Roveto Valley is bounded to the east by a regional fault line, the RVF, part of the “Val Roveto-Atina-Caserta Line” defined by Funiciello et al. (1981).
Several studies (Beneo, 1936; Accordi et al., 1969; Parotto, 1971; Parotto and Praturlon, 1975; Serafini and Vittori, 1995; Saroli et al., 2003) investigated the structural characteristics and evolution of the RVF. The fault has been characterized by different kinematics during time, i.e., the dip–slip motions followed those strike–slip motions (Salvini, 1992; Serafini and Vittori, 1995). Notably, geophysical data showed that the RVF crosses the entire crust, likely displacing the Moho discontinuity (Mostardini and Merlini, 1986; Locardi and Nicolich, 1988; Cavinato et al., 1994; Bernabini et al., 1996).
By analyzing the meso-structures in the Roveto Valley, Sora Plain, and Comino Valley, Serafini and Vittori (1995) concluded that this sector of the Apennine chain experienced several tectonic phases, with progressive rotation of the compressive axis from about SSW–NNE to WSW–ENE. According to the aforementioned authors, the thrust activity here ended in the Pliocene, followed by a transcurrent phase and then by extensional phase. The authors reported that the RVF shows evidence of recent activity with strike–slip left-lateral kinematics, as indicated by the displacement of a Pleistocene alluvial fan, named by the authors as Campoli Appennino Conglomerates.
According to Carrara et al. (1995a), the RVF was responsible for the dislocation of the deposits of the Balsorano fan, named by the authors as “Breccias of the second and third depositional phases” attributed to the Early Pleistocene. However, the authors stated that the fault seems to be sealed by more recent alluvial fan bodies belonging to the “Breccias of the fourth depositional phase” which were deposited during the Middle Pleistocene (Carrara et al., 1995a, b) (Figure 2A).
FIGURE 2. (A) Geological scheme of the study area proposed by Carrara et al., 1995a where the Roveto Valley Fault trace is indicated by a red dash-dotted line. The legend provided in the work of Carrara et al., 1995a: 1) deposits more recent than the breccias of the fourth depositional phase; 2) chaotic deposit; 3) breccias of the fourth depositional phase; 4) breccias of the third depositional phase; 5) alluvial fan; 6) overlapping of the second, third and fourth breccias depositional phase; 7) breccias of the second depositional phase; 8) fluvial and lacustrine deposits; 9) breccias of the first depositional phase; and 10) Meso-Cenozoic carbonate bedrock. (B) Geological scheme of the study area proposed by Saroli et al. (2003) where the Posta Fibreno Fault trace is marked by a red dash-dotted line. Legend provided in the work of Saroli et al. (2003): (dt)- talus material and “red soils”, fluvial-terraced, lacustrine, and travertine deposits; (alt-b)- recent alluvial fans; (fld)- braided fluvial and lacustrine deposits; (af)- ancient cemented alluvial fans; (pc)- poligenic conglomerates with allogenic elements; (caf)- Miocene flysch, (mo)- “Marne a Orbulina” formation (Miocene); and (evu, crtu-a, crtu-b, wmu)- Meso-Cenozoic carbonate bedrock.
Differently from Carrara et al. (1995a, b), Saroli et al. (2006, 2012, 2022) defined fault activity as persistent throughout the Quaternary, at least along the southern fault segment (Figure 2B), named as the Posta Fibreno Fault. According to these authors, the fault activity and the related deformation field conditioned a NW–SE distribution of the dolines, pervasively distributed in the carbonate reliefs of the study area. Moreover, the fault activity induced lowering of the karstic base level, determining the progressive embedding of the karst systems. According to the same authors, this would have occurred during the Early Pleistocene, after the deposition of the first order of the aforementioned Campoli Appennino alluvial fan, when the base groundwater level was close to the surface (Figure 1A). In this framework, Saroli et al. (2022) provides an approximate value of the vertical movement associated with the activity of the Posta Fibreno Fault, supposedly the southern branch of the RVF. Considering that the estimated onset of karst processes is around the Early Pleistocene, the authors obtain a displacement rate in the order of 0.4–0.7 mm/year associated with the fault system.
Finally, Roberts and Michetti, 2004 and Papanikolaou et al., 2005 attribute the current activity to the RVF (referred by the aforementioned authors as the “Liri Fault”) basing on morphotectonic criteria, i.e., the presence of well-preserved bedrock scarps along the fault surficial expression, supposed to be diagnostic of the fault activity. Based just on the height of the scarp in a couple of sites, the authors estimated fault offset, yielding slip rates (0.3–1.1 mm/yr, Roberts and Michetti, 2004; 0.4–0.8 mm/yr, Papanikolaou et al., 2005) averaged over the last 18 ka.
In the whole, the RVF is located in a sector of the Apennines, affected by the main extensional active faults of Central Italy, responsible for historical and instrumental earthquakes even with magnitude in the range M6–7 (Figure 1B).
The investigated area has been struck by strong earthquakes in 1349 (Mw 6.80), 1654 (Mw 6.33), and 1915 (Mw 7.08) (Rovida et al., 2022), and by seismic events with 5 ≤ Mw ≤ 6, as those that occurred in 1922 and 1927. The seismogenic source that generated the 1915 event has been identified in the Fucino Fault, east of the Roveto Valley (e.g., Michetti et al., 1996; Galadini and Galli, 1999, 2000; Gori et al., 2017) (Figure 1B). As for the Lazio–Molise (southernmost) shock of the seismic sequence occurred in 1349 (Figure 1A), a possible causative source was identified in the “Aquae Iuliae Fault” by Galli and Naso (2009). By contrast, in the case of the 1654 earthquake (Figure 1B), detailed geological investigations to define the causative fault have never been made. In the last few years, the earthquake has been tentatively associated to the “Posta Fibreno Fault”, located in the southernmost sector of the investigated area, showing evidence of the late Quaternary activity between Vicalvi and Pescosolido (Saroli et al., 2012, 2022). Seismogenic hypotheses have been recently discussed by Cucci and Cinti (2022), who reviewed the available historical, hydrological, geological, and seismological data related to the 1654 earthquake, suggesting the “Sora Fault” (mapped in the area of Sora by ITHACA Working Group, 2019 and Boncio et al., 2004) as the probable causative source.
Considering the uncertainties and the importance of this open seismogenic issue, casting light on the late Quaternary activity of the RVF appears crucial for the improvement of the knowledge on the Apennine seismotectonic framework.
Field investigations have been performed along the trace of the RVF. The fault scarp has been observed continuously from Civitella Roveto to the NE, to Pescosolido, to the SW, for a length of about 28 km. The fault plane is irregularly exposed at the base of the fault scarp (Figure 3). The observations made are described at specific “sites”, whose locations are reported in Figure 1A.
FIGURE 3. Fault scarps between Pescosolido and Canistro. (A) Panoramic view (view to SE) of the eastern flank of the Roveto Valley between Canistro and Civitella Roveto; red arrows indicate the fault escarpment. (B) The fault plane seen near the site of “Grotta di San Bartolomeo”, described in the main text. (C) The fault plane exposed NE of Civita d’Antino. (D) Panoramic view (view to NE) of the eastern flank of the Roveto Valley, E of San Giovanni Valle Roveto Superiore; red arrows indicate the fault escarpment; a simplified stereonet of the available kinematic data of the fault planes displayed in the photos, inset; the orange arrows indicate the regional extensional direction (E) The fault plane exposed just SE of San Vincenzo Valle Roveto Superiore. (F) The fault plane, affecting Early Pleistocene slope-derived deposits, seen near the “Le Chieie” site, described in the main text.
Geomorphological and geological analyses have been made in the area of Balsorano, where Carrara et al., 1995a indicated the presence of a complex alluvial fan made of superposed orders, composing a sedimentary body up to several tens of meters thick, which the authors referred to the Early Pleistocene, basing on morpholithostratigraphic considerations. Here, we refer to this complex alluvial fan sequence as “Balsorano Synthem” merging the different alluvial fan orders. From a sedimentological point of view, the Balsorano Synthem is largely represented by cemented well-stratified breccias mainly made of carbonate heterometric and angular-to-subrounded clasts. The texture is generally clast-supported, and the sparse cement is mainly sandy–silty pink–orange. The chronological attribution made by Carrara et al., 1995a and the described lithological and sedimentological characteristics suggest that the “Balsorano Synthem” could be comparable to other slope deposits, widespread in the Central Apennines, and referred to the Early Pleistocene “Second Sedimentary Cycle” of Bosi et al., 2003, having the same lithological and sedimentological characteristics and being in comparable geomorphic relation to the landscape.
Aerial photo analysis and field surveys were dedicated to the investigation of an N-140° trending escarpment (about 80 m mean high), that crosses the body of the Balsorano Synthem. This morphological feature was interpreted in the literature as the scarp related to the RVF (Carrara et al., 1995a, b) (Figures 4A,B).
FIGURE 4. (A) Panoramic view (view to NE) of the eastern flank of the Roveto Valley in the Balsorano area, where the deposits pertaining to the “Balsorano Synthem” crop out. The Roveto Valley fault escarpment is indicated by red arrows. (B) Detail of the fault escarpment (red arrows) crosscutting the Balsorano Synthem. (C) Satellite image (from Google Earth) of the Balsorano Synthem. (D) Simplified geological and geomorphological scheme of the Balsorano Synthem. The different colors of the Balsorano Synthem refer to the different facies. (E) Details of the fault escarpments that crosscut the Balsorano Synthem; the yellow dashed line indicates the sub-horizontal attitude of the deposit layers at the fault footwall; the red line indicates the fault scarp that truncates the deposit layers, leaving them hanging over the present valley bottom.
At the footwall of the fault scarp, the deposits of the Balsorano Synthem crop out. The lateral and basal contacts with the carbonate bedrock are seen at places along the slope. The layers of the Balsorano Synthem are clearly truncated by the fault and left perched over the present valley bottom (Figure 4E).
A wide erosional surface has been detected both on the footwall and the hanging wall of the fault, carved on the bedrock and the Balsorano Synthem in the footwall and only on the Balsorano Synthem in the hanging wall. Moreover, a net of small stream incisions affects the erosional surface along both the fault blocks. Comparable to the layers of the Balsorano Synthem, these stream incisions also appear as left-perched in the fault footwall over the correlative ones in the hanging wall. These pieces of evidence thus indicate that the fault not only displaced the deposits of the Balsorano Synthem but also the erosional surface carved on it and the more recent stream incision net (Figures 4C, D).
This implies that the fault activity persisted after the Balsorano Synthem deposition, affecting the geomorphic features that postdate the deposits, i.e., after the Early Pleistocene. A rough estimation of post-Early Pleistocene fault displacement coincides with the height of the fault scarp at this site, that is, about 80 m. This is also confirmed by the evidence we collected along the fault just north of the Balsorano Synthem area, at the “La Stretta-a” site described below.
Here, the fault scarp crosses an alluvial fan, which is morphologically embedded in the Balsorano Synthem.
A stream incision that crosscuts perpendicularly to the fault scarp and the alluvial fan exposes the sub-horizontal basal contact of the alluvial gravel onto the carbonate bedrock in the footwall. In the hanging wall block, instead, the incision is entirely carved into the alluvial sediments. Their basal contact with the bedrock is not visible (that is, it is buried underneath the stream valley bottom), and fault activity placed these deposits in lateral contact with the bedrock of the footwall.
Moreover, the top erosional surface of the fan in the footwall is located at an altitude higher than the top surface in the hanging wall. This evidence points out to the fault activity during and after the deposition of the sedimentary body (Figure 5).
FIGURE 5. The “La Stretta-a” site. (A) View (to the WNW) of the right flank of the incision that exposes the “La Stretta” alluvial fan. (B) Structural and stratigraphic setting of the area, showing the displacement of the alluvial fan due to the Roveto Valley Fault activity.
The exposure of the basal contact of the alluvial deposits on the carbonate bedrock in the footwall and the lack of the correlative feature in the hanging wall define a post-Early Pleistocene minimum fault offset. In fact, the difference in elevation between the basal contact of the alluvial fan onto the bedrock in the footwall and the stream incision, in the hanging wall, provides a minimum fault offset value of about 20 m. This represents a minimum offset value because it does not refer to the correlation across the fault of synchronous stratigraphic/morphological features as the basal contact in the hanging wall is buried and not exposed to allow an actual offset estimation. Instead, further post-Early Pleistocene fault displacement can be also evaluated by considering the difference in elevation between the erosional top surface of the alluvial fan both in the footwall and in the hanging wall that is in the order of 5 m. Nonetheless, as the top surface may have undergone erosion and/or accumulation of younger deposits on it, this makes the correlation of the top surfaces across the fault much less reliable to the estimate fault displacement than the correlation of the base of the correlative sedimentary body across the fault.
The geological evidence previously described indicates that the fault activity persisted even during times more recent than the Early Pleistocene. To verify this hypothesis, we investigated some natural and handmade excavations in some selected sites along different sectors of the RVF, as here described.
We made an excavation on the left flank of the small incision described at the “La Stretta-a” site (Figure 6). The excavation exposed the contact between bedrock and colluvial deposits. The nature of these deposits and their location, with respect to the morphostratigraphic setting of the site, suggest that they resulted from the erosion of the alluvial sediments mentioned for the “La Stretta-a” site. Considering the origin of these unconsolidated deposits, we hypothesized a post-Early Pleistocene age of deposition.
FIGURE 6. Geomorphologic scheme of the “La Stretta-b " and “Le Chieie” sites. Topographic map (scale 1:10.000) from opengeodata of the Abruzzi Region (http://opendata.regione.abruzzo.it/content/dbtr-regione-abruzzo-scala-110000-edizione-2007-formato-ecw).
The colluvial body can be subdivided into two sub-units: the lower one is made of cm-sized, subrounded carbonate clasts in abundant brownish silty–sandy matrix; the upper one is made of cm-to-dm-sized, subangular-to-subrounded carbonate clasts in yellowish–orange sandy matrix.
The bedrock is pervasively affected by shear planes and deformation features. The contact with the colluvial deposit is marked by a fault plane striking N-100° and dipping at 65° (structural data, as fault plane and shear plane attitudes refer to the “Right Hand Rule” notation, henceforth RHR) (Figures 7A,B), whose orientation is consistent with that of the RVF (Figure 3).
FIGURE 7. (A) Handmade excavation made at the “La Stretta-b” site; wall of the excavation that shows the colluvial deposits (in the hanging wall) placed in contact with the carbonate bedrock (in the footwall) caused by the fault; the yellow box shows a detail of the excavation in ‘C’. (B) Stratigraphic scheme of the excavation wall; a and b indicate the sub-units of the colluvial body. (C) Details of the shear zone, marked by red arrows, between the limestone and the colluvial deposits and a detail of the deposit dragged along the fault plane, marked by white dash-dotted line.
The colluvial deposit appears deformed and dragged along the fault plane, suggesting fault activity after its deposition (Figure 7C).
We can rule out that this contact is the consequence of lateral contact between the colluvial deposits and the carbonate bedrock simply due to erosion, based on the following considerations:
(1) The dip angle of the contact appears higher than would be expected if it has been caused by simple erosion, as it would have assumed lower dip angle and it would appeared smoother, with an upward concave shape.
(2) Taking into account the high degree of erodibility of the fault footwall, characterized by intensive fractured carbonate rock losing its primary sedimentary structure, the contact would have not been so vertical (as displayed in Figure 7) but it would appeared with an angle comparable to the slope gradient;
(3) In the fault hanging wall, the deposit layers appear verticalized and dragged against the fault plane, assuming a high dip angle not concordant to the primary repose angle of the deposit (Van Burkalow, 1945; Metcalf, 1966; Carrigy, 1970; Carson, 1977; Wang et al., 2013).
Along the prolongation toward the SE of the fault escarpment observed in the “La Stretta” site area (Figure 6), we performed a handmade excavation that uncovered the contact between the carbonate bedrock, exposed in the fault footwall, and colluvial deposits found in the fault hanging wall (Figures 6, 8). The bedrock appeared intensely fractured and sheared. The colluvial deposit was made of cm-sized angular carbonate clasts in a coarse–sandy yellowish–brownish matrix. Two charcoal shards (samples named as BALS 1 and BALS 2) found in the colluvial unit were dated with the radiocarbon method, and they gave ages of 661–774 cal. A.D. (1280 ± 30 years BP) and 1150–1256 cal. A. D (860 ± 30 years BP), respectively (Figure 9).
FIGURE 8. (A) View of the handmade excavation made at the “Le Chieie” site; the yellow boxes show details of the shear zones. (B) Stratigraphic scheme of the excavation wall.
FIGURE 9. (A) Details of the excavation wall made at the “Le Chieie” site. The close-up view shows the contact between the limestone and the colluvial deposit, marked by white–red arrows; the yellow dots indicate the location of the dated charcoals reported in the main text. (B) Details of the shear zone whose movement involves the carbonate bedrock and the colluvial sediment.
The contact with the colluvial deposits is marked by N-110°, 62° (according to RHR)-oriented fault plane and other secondary structural features, whose orientation was consistent with that of the main plane (Figure 3F). The sediments appeared dragged and deformed along this fault plane (Figure 9A). Moreover, the colluvial deposit is also involved in the movements of secondary shear planes (Figure 9B), located at the footwall of the main fault plane, that locally describe lithons and sigmoidal structures (the sediments are in places “surrounded” by the bedrock through shear planes; Figure 9B).
Comparably to the “La Stretta-b” site described previously, we can rule out that the main fault plane is just the effect of the lateral contact of the colluvial deposits and the bedrock due to erosion and that the secondary features are just open fractures and not fault planes that displaced the colluvial deposits based on the following observations and considerations:
(1) The high dip angle of the contact is not compatible with a simple erosional surface because if the contact has been caused by erosion it would have assumed a lower dip angle and it would have had a smoother shape. In this regard, considering the highly sheared carbonate rock that characterized the fault zone, the sole erosion would have made the contact much less abrupt and much smoother.
(2) Considering the attitude of the secondary planes, that is, they are not simply vertical open fissures but they display a counter-slope opening, it is very difficult to assume that they opened with such an attitude and remained open to allow the colluvial deposit to fill it. In this regard, filling has not occurred vertically, as expected from the opening of a simple fracture, but they appear to have been filled “laterally”, that is, counter-intuitively, against gravity. Hence, the observed features are necessarily the effect of shearing due to the fault activity.
We can also rule out that the described outcrop shows just chaotic deposits (i.e., landslides) found along the fault zone because if they have been blocks contained within an organic-rich sediment (i.e., the colluvial deposit) they would have not remained all standing vertical but they would have toppled and resting on the slope. For the sake of argument, even assuming that they are carbonate blocks in a colluvial matrix, they would have not remained standing vertical “by chance” right along the fault zone, but they would have been subsequently verticalized by the fault movements that involved the colluvial deposit.
The tectonic origin of the observed contact is also corroborated by aerial-photograph analysis and geomorphological field observations that indicate that the observed displacement of the colluvial unit cannot be ascribed to other non-tectonic processes, such as landslides. Indeed, no evidence of gravity-driven phenomena is observed here, such as landslide scarps or counter-slope scarps or tilted trees or any other gravity-related landforms. This is particularly relevant considering the age of the deformation, as recent gravitational slope movements would have left visible evidence in the local landscape which is lacking at this site. The obtained dates define a “post quem” age for the colluvial deposition and thus for the RVF movement, which occurred during or after the late Middle Age.
This site (Figure 10) is located just SE of San Giovanni Valle Roveto Superiore (Figures 3D,E). Here, we performed another excavation across the fault zone that exposed a N-140° striking shear plane that placed the carbonate bedrock of the footwall (intensely fractured and sheared) in contact with a colluvial unit made of brownish sandy clay and sparse mm-to-cm-sized carbonate pebbles. The deposit was displaced by the fault and involved in the shear zone, as shown in Figure 11.
FIGURE 10. Geomorphologic scheme of the “Cornarello” site. Topographic map (scale 1:10.000) from opengeodata of the Abruzzi Region (http://opendata.regione.abruzzo.it/content/dbtr-regione-abruzzo-scala-110000-edizione-2007-formato-ecw).
FIGURE 11. (A) Wall of the excavation made at the “Cornarello” site. (B) Stratigraphic scheme of the excavation wall.
Comparable to the “La Stretta-b” and “Le Chieie” sites described previously, we can rule out that the evidence of faulting is just the effect of erosion that is placed in lateral contact of the colluvial deposit with the carbonate bedrock and that the observed features are just the filling of fractures opened in the bedrock from the following evidence and considerations:
(1) The colluvial deposit is displaced and lowered as in the fault-hanging wall, the basal contact of the sediment with the carbonate bedrock appears lowered and it ends abruptly against the vertical fault shear zone.
(2) Considering that the bedrock in the footwall of the shear zone is characterised by almost pulverized carbonate rock, if the lateral contact between the bedrock and the colluvial deposit has simply been due to erosional processes, taking into account the high degree of erodibility of the fault footwall and the thickness of the colluvial deposit, the contact would have not appeared vertical (even counter-slope dipping) up to the ground surface (as it is), but it would have assumed a lower dip angle and would also have been much smoother (especially in the upper tip, closer to the ground surface).
(3) If the observed features were only open fractures, such a weak lithology of the shear zone (in which the fractures opened) would have not permitted the “lithons” to remain standing vertical but they would have collapsed within the fractures themselves, that is, the fractures would also have been filled with fragments of the pulverized rock itself; instead, the few fragments of the bedrock found within the fractures displayed only a vertical arrangement, parallel to the shear zone, and they were not found with a chaotic arrangement, as expected if they just collapsed within an open fracture without shearing.
Furthermore, as in the case of the “Le Chieie” site, no evidence of gravity-driven phenomena are here observed, such as landslide scarps or counter-slope scarps or tilted trees or any other gravity-related landforms, that could mimic the tectonic displacement. Hence, what can be observed in this site is the evidence of faulting of the colluvial deposit owing to fault movements.
The absence of correlative deposits in the footwall hindered the estimation of the fault offset. Nonetheless, the relation between the colluvial deposit and the local geomorphic setting suggests a very recent age of the sedimentary unit. In fact, the colluvial deposit detected at the “Cornarello” site is located over an alluvial fan which, in turn, appears morphologically embedded in the “Balsorano Synthem”. This morphostratigraphic relationship with other stratigraphic units of the area supports our chronological attribution. Moreover, the colluvial deposit is morphologically in relation to the present slopes, such that it likely represents the product of the last climatic phases that sculptured the Central Apennine slopes and that produced widespread slope deposits along mountain reliefs. These allow to conceivably attribute the colluvial sediment to the late Quaternary, likely to the Late Pleistocene.
The excavation was made NE of the settlement of Civitella Roveto, near the site of “Grotta di San Bartolomeo” (Figure 12), where the plane of the RVF is well-exposed at the base of the main fault scarp (Figure 3B).
FIGURE 12. Geomorphologic scheme of the “Grotta di San Bartolomeo” site. Topographic map (scale 1:10.000) from opengeodata of the Abruzzi Region (http://opendata.regione.abruzzo.it/content/dbtr-regione-abruzzo-scala-110000-edizione-2007-formato-ecw).
Here, starting from the faint evidence of fault displacement in an existing outcrop, we made an excavation that allowed us to investigate the tectonic structure, trending N-120°,70° (according to the RHR), placing the carbonate bedrock exposed in the footwall in contact with a sequence of slope and colluvial deposits accumulated at the base of the fault scarp, and cropping out in the fault-hanging wall.
The analysis of the excavation wall permitted to observe that the sediments have been displaced by the movement of the main fault plane and of at least one synthetic shear plane.
As shown in Figure 13, we distinguished five sedimentary units, here described from the lowermost (the older unit exposed by the excavation) to the uppermost and the more recent one.
FIGURE 13. (A) Wall of the excavation made at the “Grotta di San Bartolomeno” site. (B) Stratigraphic scheme of the excavation walls. (C) Details of the fault zone; evidence of dragging of the deposits along the fault plane is marked by red arrows. The inset shows the basal contact of Unit 2, marked by a white dashed line, cross cutting, and sealing the f2.
Unit 5: colluvial deposits made of sparse carbonate angular-to-subangular, cm-sized clasts in abundant brownish sandy–clayey organic-rich matrix. A charcoal fragment (sample name CIV1) was found and collected for radiocarbon dating, and it gave an age of 517–380 cal. B.C. (2,350 ± 30 years BP) We also collected two samples of the organic-rich matrix (samples were named as CIV BK1 and CIV BK3), which have been radiocarbon dated at 6,474–6,401 cal. B.C. (7,590 ± 30 years BP) and 6,062–5,977 cal. B.C. (7,120 ± 30 years BP), respectively. The discrepancy between the age of the matrix and that obtained from charcoals could be expected. Indeed, the age obtained from the organic-rich matrix of colluvial sediments derives inherently from an average age of the organic parent material plus the possible organic matter brought in the colluvial deposits by underground water circulation. Nonetheless, the obtained dates only define a “post quem” age for the colluvial deposition. Hence, in terms of chronostratigraphic attribution, it has to be considered that the colluvial sediment was deposited after the most recent of the obtained ages.
Unit 4: slope debris made of cm-size angular-to-subangular carbonate clasts, with layers showing different texture (clast- and matrix-supported), and silty brownish-orange matrix. Two sub-units have been distinguished (sub-units 4a and 4b) based on the relative abundance of calcrete remnants, larger in 4b. The layers are generally sub-horizontal, slightly dipping towards the SW.
Unit 3: it unconformably overlies Unit 4 and the related top erosional surface gently dipping towards SW, marked by a line of mm-to-cm size carbonate pebbles. This unit is made of slope debris, angular-to-subangular carbonate clasts in rare and sparse coarse sandy yellowish–brownish matrix (clast-supported texture), alternating with rare silty–sandy layers.
Unit 2: colluvial deposits made of angular carbonate clasts of various sizes in a brown sandy matrix (matrix-supported texture). An erosional surface marks the contact between this deposit and Units 3 and 4.
Unit 1: colluvial deposits made of brown sandy silt with abundant angular-to-subangular carbonate pebbles.
From the analysis of the relationship between the sedimentary sequence and the shear zone, it is possible to distinguish the occurrence of at least two faulting events that involved the deposits (Figures 13B, 14).
FIGURE 14. Palinspastic restoration of the tectonosedimentary evolution of the “Grotta di San Bartolomeo” excavation as derived from the analysis of the excavation wall. The different erosional, depositional, and faulting events are shown starting from the oldest (T0) to the youngest (T10).
The older event, E2, is testified by the displacement of Units 5 and 4 along the fault plane f2. These units end abruptly against the fault plane, being deformed along it. Fault f2, however, is sealed by the erosional surface which separates Units 4 and 3 from Unit 2. Unit 2, conversely, is displaced and dragged along the fault plane f1, testifying to the occurrence of a subsequent faulting event, E1. The analysis of the excavation wall did not allow to definitely infer whether Unit 1 was involved in E1 or not, since the available exposure does not clearly define the relationship between this unit and the fault shear zone.
The occurrence of deformation events occurred after the deposition of Unit 3 is also testified by the attitude of the layers of this unit, which showed a dip angle of about 50° toward the SW (i.e., toward the valley). This value is slightly higher than the primary dip angle of such kind of sediments (e.g. Van Burkalow, 1945; Metcalf, 1966; Carrigy, 1970; Carson, 1977; Wang et al., 2013), suggesting the occurrence of a little rotation as the effect of the dragging.
Despite the absence of correlative deposits at the fault footwall, the thickness of Unit 2, which is placed in contact with the bedrock by E1, allows us to make reasonable hypothesis of the amount of the surface offset caused by E1. Indeed, by observing the geometrical setting of Unit 2 (Figure 14) with respect to the fault plane and by considering that Unit 2 must have been present in the fault footwall (the current absence of Unit 2 in the fault footwall is likely due to erosion) the preserved thickness of Unit 2 in the fault-hanging wall suggests a minimum surface offset caused by E1 in the order of 50 cm. This must be considered as a rough estimation of the minimum offset, considering the limit between Units 2 and 1 corresponds to an erosional surface, so that it is not possible to estimate the actual, original thickness of Unit 2.
Investigations at different sites along the RVF allow making inferences about the activity of this tectonic structure, discussed here in the following points:
(1) Differently from what Carrara et al. (1995a, b) proposed, the fault not only displaced the deposits of the Balsorano Synthem but also the alluvial fan embedded within the Synthem itself (Figure 5). Although no chronological data are available to date to constrain the age of that alluvial fan, the morphostratigraphic relationship with the Balsorano Synthem suggests an age that postdates the Early Pleistocene. We can also estimate in about 20 m the minimum offset affecting the faulted alluvial fan. Further investigations need better constraint to the chronology of the displaced alluvial fans and to obtain reliable long term slip rate estimates of the main fault branches.
(2) The excavations made along the fault at different sites corroborate the fault activity throughout the Quaternary, up to the late Holocene. Specifically, the observations made at the “Le Chieie” and “Grotta di San Bartolomeo” sites permit to identify the occurrence of two faulting events after 517–380 B.C. (calibrated age), that in the past 2,500 years. In particular, the last fault activation event occurred after 1,150–1,256 AD (calibrated age), considering the chronological data obtained at the “Le Chieie” site. Owing the very recent age of the last faulting event detected in both sites (i.e., likely occurred is the past millennium), we can hypothesize that the last event detected at the “Le Chieie” site may correspond to E1 of the “Grotta di San Bartolomeo” site, i.e. the youngest event of the two detected there, following 517–380 B.C. (calibrated age). Therefore, we can likely assume the occurrence of the last episode of the fault activation during or after the late–Middle Ages. Moreover, the observation made at these sites inherently indicates that these events caused significant surface faulting.
(3) The location of the fault and the chronological constraints for the faulting events allow some inferences regarding the possible attribution of major local historical earthquakes to the activation of this tectonic structure. Specifically, the fault location and geometrical features, paleoseismological data, and historical seismicity suggest that the fault may have generated during the the Mw 6.33 ± 0.14, 1654 earthquake (Rovida et al., 2022) whose epicentral area was located in the lower Roveto Valley–Sora Basin (Figure 15). This seismic event is compatible with the RVF in terms of i) intensity datapoint distribution, with respect to the trace and attitude of the fault, ii) age of surface faulting event that we identified, iii) amount of surface offset with respect to the earthquake estimated magnitude, and iv) earthquake magnitude with respect to fault length. Consistency between the 1654 earthquake and a seismogenic fault located in the lower Roveto Valley–Sora Basin, roughly fitting with the southern portion of the RVF, is supported by source modeling proposed by Cucci and Cinti (2022), based on the intensity distribution and other earthquake data. Nonetheless, the authors matched their modeling results with information only derived from the literature concerning the possible active faults and active fault geometries of the area (Boncio et al., 2004; ITHACA Working Group, 2019) which does not include results of recent field investigations. Furthermore, Cucci and Cinti’s modeling does not suggest that a fault in the Balsorano area caused the 1654 earthquake. Nonetheless, our observations indicate rupture of the RVF during a time span was compatible with the 1654 event also in that area. A partial conclusion may be that historical information concerning the 1654 earthquake should be improved in terms of both intensity estimation and intensity distribution. Otherwise, considering the obtained chronological constraints for event E1, we may hypothesize that the RVF originated during an earthquake earlier than 1654, e.g., during the Late–Middle Ages. Considering the proximity of the investigated area with the Fucino Basin, this working hypothesis should also be verified by comparing the available data on the RVF with published (Galadini and Galli, 1999; Galli et al., 2016) and still unpublished (data by some of the authors of this article) evidence of possible medieval earthquake effects collected in that basin. Moreover, RVF activation related to the strong 1349 earthquake (presently dubitatively attributed to the Aquae Iuliae Fault by Galli and Naso, 2009) should also be considered.
(4) As for the segmentation of the RVF in the seismogenic perspective, Pace et al., 2020 defined a source in the northern sector of the valley, named “Liri”, whose trace at the surface coincides with the RVF but whose extension toward south only partially corresponds to the section of the fault along which we found evidence of Holocene activity. Despite the clear structural continuity of the fault toward south, the geological reason why the source proposed by Pace et al., 2020 abruptly ends southward is unclear and similarly unclear is the basis for the proposed fault segmentation. Moreover, most of the trace of the “Liri” seismogenic source coincides with the section of the fault where geological evidence of fault inactivity has been found recently (Galadini et al., 2022).
(5) Regarding the evidence of the recent fault activity, a crucial aspect must be underlined: in many places along the fault trace, the fault plane exposure is due to non-tectonic processes related to the local geological and lithological framework of the slopes affected by the RVF. Indeed, in most of its traces, the fault places the carbonate bedrock of the footwall in contact with the Miocene clayey–sandy sin–orogenic flysch and locally with continental Quaternary deposits overlaying the flysch. Such a setting favors exhumation of the fault plane on the carbonate bedrock due to:
FIGURE 15. Digital terrain model (shaded relief) of the Roveto Valley Fault (red line) area on which the intensity distribution (MCS scale) of the 1654 earthquake (Mw 6.33) is reported (from Rovida et al., 2022).
a) landslides involving flysch and, often, overlying continental deposits, with the fault plane playing the role of a preferential sliding surface in its surficial portion
b) differential erosion between the carbonate bedrock and much more erodible lithotypes the in fault-hanging wall
On these grounds, the evidence of fault activity we collected and the sites we selected and examined have had, as a fundamental pre-condition, the analysis of the sites to verify the absence of the aforementioned surface non-tectonic phenomena, to make our evidence-based inferences reliable. Moreover, this aspect focuses the attention on reliability of defining fault activity, the related kinematic behavior and slip parameters mainly related to morphologic evidence, such as the exposure of fault planes (as in the works by Roberts and Michetti, 2004, and Papanikolaou et al., 2005, on the Roveto Valley–Liri Valley fault), without detailed investigations on the possible non-tectonic causes of the fault plane exposition and of the displacement of recent deposits and landforms. In this regard, other authors have questioned the reliability of bedrock fault plane exposition as a proxy and morphologic element that only by itself testifies to fault activity (e.g., Galadini, 1999; Galadini and Galli, 2000; Fubelli et al., 2009; Kastelic et al., 2017). The investigations along the RVF we made in the last years have fostered our opinion about the misleading potential of the bedrock fault scarps and related fault plane exposures to collect information on active tectonics and to process data in the seismogenic perspective.
(6) The location of the sites investigated in the present work, combined with the information obtained from recent publications (Galadini et al., 2022; Saroli et al., 2022) allow to trace the active portion of the RVF for about 35 Km. This, associated with the geophysical evidence that the fault crosscuts at least the upper crust (Locardi and Nicolich, 1988), indicates that the RVF is the manifestation at the surface of a major active crustal tectonic structure whose movements are able to displace the ground surface. Moreover, as the evidence we collected indicates fault activation characterized by discrete displacement events, it is possible to attribute sudden episodes of activation to the RVF likely associated with the nucleation of earthquakes. Taking into consideration the magnitude threshold for the occurrence of primary-surface faulting in the Apennines, that is Mw 5.5–6 (Michetti et al., 2000; Falcucci et al., 2016), we can hypothesize that strong seismic events may be caused by sudden activation of the RVF. Within this light, by applying the regression proposed by Wells and Coppersmith (1994) or by Galli et al. (2008) for the Italian active faults, a fault length at the surface of about 35 Km (by adding the investigated fault section to that of the Posta Fibreno sector investigated by Saroli et al., 2022; Figure 15) yields a maximum expected magnitude for earthquakes generated by the RVF in the order of Mw 6.9. In addition to the seismogenic implications, the available data have consequences also for the definition of the capable faulting, and therefore for potential surface faulting, in the investigated area. The trace of the fault which can be considered active and capable based on the data presented here is very close to some villages located along the left flank of the Roveto Valley, notably (from north) Morrea, San Vincenzo Valle Roveto, San Giovanni Valle Roveto Superiore, and Ridotti. Evidence of recent fault motion in these areas has implications in processing local seismic microzonations according to the current guidelines (Technical Commission on Seismic Microzonation, 2015). Products of these applied research studies have, in turn, implications in planning land use, through further detailed investigations on recent activity, possibly involving paleoseismological analyses. Therefore, future interventions for engineering purposes may cast further light on the recent historical activity of the RVF.
In this work, we investigated, for the first time, the Late Pleistocene–Holocene activity of the RVF, by means of comprehensive and extensive geological, geomorphological, and paleoseismological investigations along most of the RVF trace that represents one of the major faults of the southern Abruzzo and Lazio regions. The current activity of this tectonic structure is still a matter of debate in the literature. Several sectors of this fault revealed displacement of continental deposits, in particular of alluvial fans probably Middle-to-Late Pleistocene in age and, more importantly, of colluvial deposits dated to the late Holocene. Specifically, we first identified evidence of two Holocene faulting events after 517–380 AD (calibrated age), the most recent of which occurred after 1150–1256 AD (calibrated age), responsible for more than 0.5 m minimum surface offset. The collected geological evidence, the paleoseismological data, and the location of the fault compared to the damage distribution of the major local seismic events suggest that the RVF has been the source of Mw 6.33 ± 0.14, 1654 seismic event (as already hypothesized by Saroli et al. (2022) or of a preceding, still undefined, event possibly occurred during the Late Middle Ages, as only for later periods the seismic catalogues should include complete information as for the destructive earthquakes.
However, considering the maximum expected magnitude of an earthquake generated by the activation of the entire RVF (ca. 35km), to be on order of Mw 6.9, and the magnitude of the 1654 earthquake, the possible association of this seismic event to the fault implies that the fault possibly did not release the maximum potential earthquake in 1654, or that the earthquake did not rupture the whole fault as a consequence of still undefined fault segmentation. This clarifies that further investigations on the RVF should be addressed to solve these open seismogenic issues.
Finally, our work first provides new and crucial parameters for a major active fault of the Central Apennines, located in a region where important urban areas are located such as Sora and Avezzano, and that is close to the urban area of Rome, which suffered severe damage from historical earthquakes that originated in areas adjacent to the RVF, such as the 1915 and 1349 earthquakes.
The raw data supporting the conclusion of this article will be made available by the authors, without undue reservation.
GD, FG, and SG, contributed to the conception and design of the study. GD, DM, EF, SG, and MM performed the aerial-photograph analysis interpretation. GD, FG, DM, and MM performed the in situ surveys. GD, FG, DM, EF, SG, MM, and MS performed and interpreted the handmade excavation. GD wrote the first draft of the manuscript. FG and SG wrote sections of the manuscript. All authors contributed to manuscript revision and read and approved the submitted version.
The authors acknowledge Prof. Federico Sani, Dott. Mikhail Rodkin and Prof. Enrico Miccadei for their helpful comments and suggestions, which definitely improved the overall quality of our work. In particular, they provided very useful comments and in-depth criticism that prompted us both to make the work more rigorous and to better explain some of the concepts and evidence on which our conclusions are based. This work benefited from funding (publication fees) of Istituto Nazionale di Geofisica e Vulcanologia, sezione Roma 1.
GD was employed by Mares S. R. L.
The remaining authors declare that the research was conducted in the absence of any commercial or financial relationships that could be construed as a potential conflict of interest.
All claims expressed in this article are solely those of the authors and do not necessarily represent those of their affiliated organizations, or those of the publisher, the editors, and the reviewers. Any product that may be evaluated in this article, or claim that may be made by its manufacturer, is not guaranteed or endorsed by the publisher.
Accordi, B., Angelucci, A., Avena, G. C., Bernardini, F., Boni, C. F., Bruno, F., et al. (1969). Idrogeologia dell'alto bacino del Liri (Appennino Centrale). Geol. Rom. 8, 177–559.
Beneo, E. (1936). La formazione Miocenica e la tettonica della Valle Roveto (alta Valle del Liri). Boll. dell'Ufficio Geol. d'Italia LXI. Nota VII.
Bernabini, M., Di Bucci, D., Orlando, L., Parotto, M., and Tozzi, M. (1996). Gravimetric evidence of deep structure in mountain building: Subducted adriatic crust beneath the tyrrhenian Moho in central Italy. J. Geodyn. 21, 223–234. doi:10.1016/0264-3707(95)00032-1
Boncio, P., Lavecchia, G., and Pace, B. (2004). Defining amodel of 3D seismogenic sources for seismic hazard assessment applications: The case of central Apennines (Italy). J. Seismol. 8, 407–425. https://doi. org/. doi:10.1023/B:JOSE.0000038449.78801.05
Bosi, C., and Bertini, T. (1970). Geologia della media valle dell'Aterno. Mem. Soc. Geol. It. 9, 719–777.
Bosi, C., Galadini, F., Giaccio, B., Messina, P., and Sposato, A. (2003). Plio-quaternary continental deposits in the latium Abruzzi Apennines: The correlation of geological events across different intermontane basins. Il Quat. 16 (1bis), 55–76.
Bosi, C., and Galadini, F. (1995). La fagliazione di superficie come elemento di fragilità del territorio. Geol. Appl. Idrogeol. 30, 29–43.
Bosi, C. (1975). Osservazioni preliminari su faglie probabilmente attive nell’Appennino centrale. Boll. Soc. Geol. It. 94, 827–859.
Carrara, C., Dai Pra, G., and Giraudi, C. (1995b). “Lineamenti di Tettonica Plio-Quaternaria dell’area,” in Carrara C. “Lazio meridionale. Sintesi delle ricerche geologiche multidisciplinari” E.N.E.A., Dip. Ambiente, Serie Studi e Ricerche, Italy, 151–155.
Carrara, C., Frezzotti, M., and Giraudi, C. (1995a). “Area compresa tra la Valle Latina e la Valle Roveto. stratigrafia Plio-Quaternaria,” in Carrara C. “Lazio meridionale. Sintesi delle ricerche geologiche multidisciplinari”, E.N.E.A., Dip. Ambiente, Serie Studi e Ricerche, Italy, 62–85.
Carrigy, M. A. (1970). Experiments on the angle of repose of granular materials. Sedimentology 14 (3/4), 147–158. doi:10.1111/j.1365-3091.1970.tb00189.x
Carson, M. A. (1977). Angles of repose, angles of shearing resistance and angles of talus slopes. Earth Surf. Process. 2, 363–380. doi:10.1002/esp.3290020408
Carta Geologica d’Italia 1:100.000 (1941a). Cartografia geologica ufficiale, foglio 151 – alatri. Servizio geologico d’Italia. Available at: http://sgi.isprambiente.it/geologia100k/mostra_foglio.aspx?numero_foglio=151.
Carta Geologica d’Italia 1:100.000 (1941b). Cartografia geologica ufficiale, foglio 152 – Sora, servizio geologico d’Italia. Available at: http://sgi.isprambiente.it/geologia100k/mostra_foglio.aspx?numero_foglio=152.
Cavinato, G. P., Cosentino, D., Funiciello, R., Parotto, M., Salvini, F., and Tozzi, M. (1994). Constraints and new problems for a geodynamical modelling of Central Italy (Crop 11 Civitavecchia-Vasto deep seismic line). Boll. Geof. Teor. Appl. 36 (141- 144), 159–174.
Cipollari, P., and Cosentino, D. (1999). Cronostratigrafia dei depositi neogenici del settore Ernico-Simbruino, Appennino Centrale. Boll. Soc. Geol. It. 118, 439–459.
Cipollari, P., Cosentino, D., and Parotto, M. (1995). “Modello cinematico-strutturale dell’Italia centrale,”. «Geodinamica Tettonica attiva del Sist. Tirreno-Appennino», Studi Geologici Camertivol. spec, Editors G. Cello, G. Deiana, and P. P. Piantoni, 1995/1, 135–144.
Cosentino, D., Cipollari, P., Marsili, P., and Scrocca, D. (2010). Geology of the central Apennines: A regional review. J. Virt. Ex. 36, 1441–8142. paper 11. electronic edition, issn, doi:10.3809/jvirtex.2010.00223
Cucci, L., and Cinti, F. (2022). In search of the 1654 seismic source (central Italy): An obscure, strong, damaging earthquake occurred less than 100 km from Rome and naples. Appl. Sci. (Basel). 12 (3), 1150. doi:10.3390/app12031150
DISS Working Group (2018). Database of individual seismogenic sources (DISS), version 3.2.1: A compilation of potential sources for earthquakes larger than M 5.5 in Italy and surrounding areas. Available at: http://diss.rm.ingv.it/diss/, IstitutoNazionale di Geofisica e Vulcanologia. doi:10.6092/INGV.IT-DISS3.2.1
Falcucci, E., Gori, S., Galadini, F., Fubelli, G., Moro, M., and Saroli, M. (2016). Active faults in the epicentral and mesoseismal ml 6.0 24, 2016 amatrice earthquake region, central Italy. Methodological and seismotectonic issues. Ann. Of Geophys. 59. Fast Track 5. doi:10.4401/Ag-7266
Fubelli, G., Gori, S., Falcucci, E., Galadini, F., and Messina, P. (2009). Geomorphic signatures of recent normal fault activity versus geological evidence of inactivity: Case studies from the central Apennines (Italy). Tectonophysics 476, 252–268. doi:10.1016/j.tecto.2008.10.026
Funiciello, R., Parotto, M., and Praturlon, A. (1981). Carta tettonica d’Italia alla scala 1:1.500.000. Roma: Publ. n. 269 del Progetto Finalizzato Geodinamica, C.N.R.
Galadini, F., Ceccaroni, E., Dixit Dominus, G., Falcucci, E., Gori, S., Maceroni, D., et al. (2022). Combining Earth Sciences with Archaeology to investigate natural risks related to the cultural heritage of the Marsica region (central Apennines, Italy). Med. Geosc. Rev. 4 (2), 287–318. doi:10.1007/s42990-022-00078-9
Galadini, F., and Galli, P. (2000). Active tectonics in the central Apennines (Italy)—Input data for seismic hazard assessment. Nat. Hazards 22, 225–268. doi:10.1023/a:1008149531980
Galadini, F., and Galli, P. (1999). The Holocene paleoearthquakes on the 1915 Avezzano earthquake faults (central Italy): Implications for active tectonics in the central Apennines. Tectonophysics 308, 143–170. doi:10.1016/s0040-1951(99)00091-8
Galadini, F. (1999). Pleistocene changes in the central apennine fault kinematics: A key to decipher active tectonics in central Italy. Tectonics 18 (5), 877–894. doi:10.1029/1999tc900020
Galli, P., Galadini, F., and Pantosti, D. (2008). Twenty years of paleoseismology in Italy. Earth-Science Rev. 88, 89–117. doi:10.1016/j.earscirev.2008.01.001
Galli, P., Giaccio, B., Messina, M., and Peronace, E. (2016). Three magnitude 7 earthquakes on a single fault in central Italy in 1400 years, evidenced by new palaeoseismic results. Terra nova. 28 (2), 146–154. doi:10.1111/ter.12202
Galli, P., and Naso, J. (2009). Unmasking the 1349 earthquake source (southern Italy): Paleoseismological and archaeoseismological indications from the Aquae Iuliae fault. J. Of Struct. Geol. 31, 128–149. doi:10.1016/j.jsg.2008.09.007
Giraudi, C. (1989). “Datazione di un evento sismico preistorico con metodi geologici e radiometrici: Piani di Aremogna e delle cinque miglia,” in I terremoti prima del Mille in Italia e nell’area mediterranea. Editor E. Guidoboni (Bologna: ING-SGA), 53–64.
Gori, S., Falcucci, E., Galadini, F., Moro, M., Saroli, M., and Ceccaroni, E. (2017). Geoarchaeology and paleoseismology blend to define the Fucino active normal fault slip history, central Italy. Quat. Int. 451, 114–128. doi:10.1016/j.quaint.2017.01.028
ITHACA Working Group (2019). A database of active capable faults of the Italian territory. ITHACA (ITaly HAzard from CApable faulting)Version December 2019. ISPRA Geological Survey of Italy. Web Portal Available at: http://sgi2.isprambiente.it/ithacaweb/Mappatura.aspx.
Kastelic, V., Burrato, P., Carafa, M. M. C., and Basili, R. (2017). Repeated surveys reveal nontectonic exposure of supposedly active normal faults in the central Apennines, Italy. J. Geophys. Res. Earth Surf. 122, 114–129. doi:10.1002/2016jf003953
Locardi, E., and Nicolich, R. (1988). Geodinamica del tirreno e dell’Appennino centro meridionale: La nuova carta della Moho. Mem. Soc. Geol. It. 41, 121–140.
Locati, M., Camassi, R., Rovida, A., Ercolani, E., Bernardini, F., Castelli, V., et al. (2022). Database macrosismico italiano (DBMI15), versione 4.0. Italy: Istituto Nazionale di Geofisica e Vulcanologia INGV. doi:10.13127/DBMI/DBMI15.4
Maceroni, D., Falcucci, E., Gori, S., Galadini, F., Moro, M., and Saroli, M. (2021). Capable faults and seismogenic sources: Assessing activity and seismogenic behavior for engineering practices. Cases from central Italy. Italian J. Eng. Geol. Environ., 11. pp. doi:10.4408/IJEGE.2021-01.S-12
Metcalf, J. R. (1966). Angle of repose and internal friction. Int. J. Rock Mech. Min. Sci. Geomechanics Abstr. 3 (2), 155–161. doi:10.1016/0148-9062(66)90005-2
Michetti, A. M., Brunamonte, F., Serva, L., and Vittori, E. (1996). Trench investigations of the 1915 Fucino earthquake fault scarps (Abruzzo, central Italy): Geological evidence of large historical events. J. Geophys. Res. 101, 5921–5936. doi:10.1029/95jb02852
Michetti, A. M., Ferreli, L., Esposito, E., Porfido, S., Blumetti, A. M., Vittori, E., et al. (2000). Ground effects during the 9 september 1998, Mw = 5.6 lauria earthquake and the seismic potential of the aseismic pollino region in southern Italy. Seismol. Res. Lett. 71, 31–46. doi:10.1785/gssrl.71.1.31
Mostardini, F., and Merlini, S. (1986). Appennino centro meridionale: Sezioni geologiche e proposta di modello strutturale. Mem. Soc. Geol. It. 35, 177–202.
Pace, B., Valentini, A., Ferranti, L., Vasta, M., Vassallo, M., Montagna, P., et al. (2020). A large paleoearthquake in the Central Apennines, Italy, recorded by the collapse of a cave speleothem. Tectonics 39, e2020TC006289. https://doi.org/. doi:10.1029/2020TC006289
Papanikolaou, I. D., Roberts, G. P., and Michetti, A. M. (2005). Fault scarps and deformation rates in Lazio–Abruzzo, Central Italy: Comparison between geological fault slip-rate and GPS data. Tectonophysics 408 (1), 147–176. doi:10.1016/j.tecto.2005.05.043
Parotto, M., and Praturlon, A. (1975). Geological summary of the central Apennines. Struct. Model Italy 90, 257–300. Quad. De “La Ric. Scient.”, C.N.R.
Parotto, M. (1971). Statigraphy and tectonics of the eastern simbruini and Western Marsica ranges (central Apennines - Italy). Atti Acc. Naz. Lincei, Mem. 10, 91–170.
Roberts, G. P., and Michetti, A. M. (2004). Spatial and temporal variations in growth rates along active normal fault systems: An example from the lazio–abruzzo Apennines, central Italy. J. Struct. Geol. 26 (2), 339–376. doi:10.1016/s0191-8141(03)00103-2
Rovida, A., Locati, M., Camassi, R., Lolli, B., Gasperini, P., and Antonucci, A. (2022). Catalogo Parametrico dei Terremoti Italiani (CPTI15), versione 4.0. Italy: Istituto Nazionale di Geofisica e Vulcanologia INGV. htps://doi.org/. doi:10.13127/CPTI/CPTI15.4
Salvini, F. (1992). Tettonica a blocchi in settori crostali superficiali: Modellizzazione ed esempi da dati strutturali in Appennino centrale studi preliminari all’acquisizione dati del profilo crop 11 civitavecchia - vasto” a cura di Tozzi M., Cavinato G.P & Parotto M. Studi Geol. Camerti 17 (8), 1139–1149. pp.
Saroli, M., Albano, M., Moro, M., Falcucci, E., Gori, S., Galadini, F., et al. (2022). Looking into the entanglement between karst landforms and fault activity in carbonate ridges: The Fibreno fault system (central Italy). Front. Earth Sci. 10, 25,. doi:10.3389/feart.2022.891319
Saroli, M., Biasini, A., Cavinato, G. P., and Di Luzio, E. (2003). Geological setting of the southern sector of the Roveto valley (central appennines, Italy). Boll. Della Società Geol. Ital. 122, 467–481. 9ff, 1 tav. f.t. issn: 0037-8763.
Saroli, M., Moro, M., Cinti, F. R., and Montone, P. (2006). La faglia Val Roveto-Atina (Appennino Centrale): Evidenze di attività tettonica quaternaria. 25° Convegno Nazionale GNGTS, riassunti estesi. Roma: Cnr, 89–90.
Saroli, M., Moro, M., Gori, S., Falcucci, E., and Salvatore, M. C. (2012). Tettonica, idrogeologia e carsismo: Un nuovo approccio multidisciplinare per lo studio della tettonica attiva. L’esempio della faglia di Posta Fibreno (Marsica occidentale - Lazio meridionale). IV congresso nazionale AIGA. EngHydroEnv Geol. 14b (2012), 215–216.
Serafini, S., and Vittori, E. (1995). “Analisi di mesostrutture tettoniche di tipo fragile nella Val Roveto, nella Piana di Sora e nella Val di Comino,” in Carrara C. “Lazio meridionale. Sintesi delle ricerche geologiche multidisciplinari” Italy, E.N.E.A., Dip. Ambiente, Serie Studi e Ricerche, 93–107.
Technical Commission on Seismic Microzonation (2015). Land use guidelines for areas with active and capable faults (ACF). Roma, Conference of the Italian Regions and AutonomousProvinces – Civil Protection Department, 55–VII. pp, Available at: https://www.centromicrozonazionesismica.it/it/download/summary/20-seismic-microzonation-land-use-guidelines-for-areas-with-active-and-capable-faults-acf/66-seismic-microzonation-land-use-guidelines-for-areas-with-active-and-capable-faults-acf.
Van Burkalow, A. (1945). Angle of repose and angle of sliding friction: An experimental study. Geol. Soc. Am. Bull. 56 (6), 669–707. doi:10.1130/0016-7606(1945)56[669:aoraao]2.0.co;2
Wang, J. J., Zhao, D., Liang, Y., and Wen, H. B. (2013). Angle of repose of landslide debris deposits induced by 2008 Sichuan earthquake. Eng. Geol. 156, 103–110. doi:10.1016/j.enggeo.2013.01.021
Keywords: central Apennine, Quaternary geology, geomorphology, Roveto Valley fault, active tectonic, paleoseismology, seismotectonics
Citation: Maceroni D, Dixit Dominus G, Gori S, Falcucci E, Galadini F, Moro M and Saroli M (2022) First evidence of the Late Pleistocene—Holocene activity of the Roveto Valley Fault (Central Apennines, Italy). Front. Earth Sci. 10:1018737. doi: 10.3389/feart.2022.1018737
Received: 13 August 2022; Accepted: 25 October 2022;
Published: 11 November 2022.
Edited by:
Steven L. Forman, Baylor University, United StatesReviewed by:
Federico Sani, University of Florence, ItalyCopyright © 2022 Maceroni, Dixit Dominus, Gori, Falcucci, Galadini, Moro and Saroli. This is an open-access article distributed under the terms of the Creative Commons Attribution License (CC BY). The use, distribution or reproduction in other forums is permitted, provided the original author(s) and the copyright owner(s) are credited and that the original publication in this journal is cited, in accordance with accepted academic practice. No use, distribution or reproduction is permitted which does not comply with these terms.
*Correspondence: Stefano Gori, c3RlZmFuby5nb3JpQGluZ3YuaXQ=
Disclaimer: All claims expressed in this article are solely those of the authors and do not necessarily represent those of their affiliated organizations, or those of the publisher, the editors and the reviewers. Any product that may be evaluated in this article or claim that may be made by its manufacturer is not guaranteed or endorsed by the publisher.
Research integrity at Frontiers
Learn more about the work of our research integrity team to safeguard the quality of each article we publish.