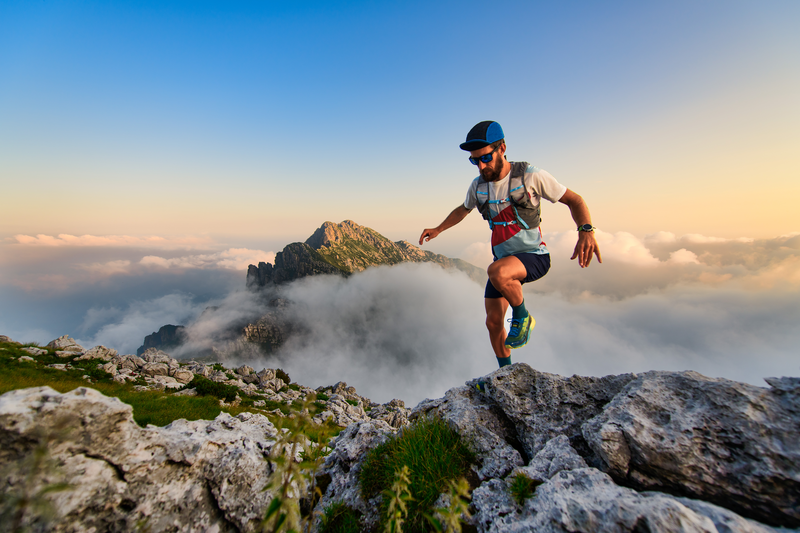
94% of researchers rate our articles as excellent or good
Learn more about the work of our research integrity team to safeguard the quality of each article we publish.
Find out more
ORIGINAL RESEARCH article
Front. Earth Sci. , 03 May 2022
Sec. Structural Geology and Tectonics
Volume 9 - 2021 | https://doi.org/10.3389/feart.2021.825852
This article is part of the Research Topic Continental Basin and Orogenic Processes: Deep Structure, Tectonic Deformation, and Dynamics View all 24 articles
The accretionary processes and the continental growth of the Altaids are still controversial. The Beishan orogen is situated in the southernmost Altaids and is an ideal tectonic site to address these issues. In this study, we report the results of new field-based lithological mapping and structural analysis on the Huaniushan complex in the Beishan orogen, which is composed of blocks of serpentinized ultramafic, gabbro, basalt, chert, limestone, and other rocks within a strongly deformed and cleaved matrix of sandstone and schist. Our new zircon U-Pb date reveal that a gabbro block formed at 504 ± 3 Ma. Our geochemical and isotopic data of gabbroic and basaltic blocks show that they are relics of the Mid-Ocean-Ridge (MORB)-type and Ocean-Island-Basalt (OIB)-type oceanic lithosphere, with high values of εNd(t) (+4.3–+14.5) and εHf(t) (+8.07–+17.74). The maximum depositional ages (MDAs) of two sandstone blocks were dated at 309 ± 5 Ma and 502 ± 11 Ma, respectively. U-Pb ages and Hf isotopes of detrital zircons from the matrix sandstones indicate that they were derived only from the Shuangyingshan–Huaniushan arc to the north. Accordingly, the Huaniushan complex was part of the Liuyuan accretionary complex that fringed the Huaniushan arc, and, therefore, formed by the northward subduction of the Liuyuan oceanic plate. Combined with the basalt yields zircon U-Pb age of 1,071 ± 5 Ma, we concluded that the Huaniushan complex has an age of 1,071 Ma to 309 Ma. Furthermore, the oceanic blocks and sedimentary matrix of the Liuyuan accretionary complex have an age of 1,071–270 Ma and 920–234 Ma, respectively, suggesting that the Liuyuan Ocean was still open at ca. 234 Ma. Thus, the studies reveal that the Liuyuan Ocean, a major branch of the Paleo-Asian Ocean, may have experienced a prolonged tectonic history, starting in the late Mesoproterozoic (1,071 Ma) and terminating later than the late Triassic (234 Ma), with a long subduction and development of a series of seamounts and/or plateaus emplaced into the Liuyuan accretionary complex.
The Altaids or the southern Central Asian Orogenic Belt was a most critical site of juvenile crustal growth, lying among the European craton to the west, the Siberian craton to the north, and the Tarim and North China cratons to the south (Kröner et al., 2007; Windley et al., 2007; Schulmann and Paterson, 2011; Wilhem et al., 2012; Xiao et al., 2013; Safonova and Santosh, 2014; Xiao et al., 2018) (Figure 1A). It experienced long-lived accretion of island arcs, continental arcs, seamounts, microcontinents, and accretionary complexes (Coleman, 1989; Allen et al., 1995; Dobretsov et al., 1995; Buchan et al., 2002; Bazhenov et al., 2003; Kröner et al., 2007; Windley et al., 2007; Xiao et al., 2018; Liu et al., 2021). The Altaids is generally regarded as a result of the final formation of the Kazakhstan and Tuva–Mongol oroclines, accompanying the convergence of the Tarim and North China cratons along the South Tianshan–Solonker suture zone (Şengör et al., 1993; Xiao et al., 2018). The South Tianshan and Solonker belts are confirmed to be the final suture for the eastern and western segments of the southern Altaids. However, the exact position of the final position of the suture in the middle segment is not clear, which hampers our understanding of the accretionary processes of the southern Altaids and continental growth of Central Asia.
FIGURE 1. (A) Schematic tectonic map of Central Asia and adjacent regions, showing the tectonic position of the Beishan orogen (Şengör et al., 1993; Xiao et al., 2018) EST-BS, eastern Tianshan-Beishan orogen. (B) Tectonic map of the Beishan orogenic belt, showing the position of the Liuyuan accretionary complex in relation to the three main arcs (Xiao et al., 2010). (C) Geological map of the Liuyuan accretionary complex and adjacent areas showing the relations with the main stratigraphic units of the Beishan (GSBGMR, 1989; Mao et al., 2012b).
The Beishan orogen is the middle segment of the Tianshan–Solonker suture of the southern Altaids (Windley et al., 2007; Domeier and Torsvik, 2014; Xiao et al., 2018; Liu et al., 2021), which is one of the key areas for unraveling the accretionary processes and continental growth of Central Asia (Zuo et al., 1991; Nie et al., 2002a; Xiao et al., 2010; Mao et al., 2012b). It was formed by episodic amalgamation and accretion of continental margin arcs, island arcs, ophiolites, and accretionary wedges. The Liuyuan-Houhongquan mélange complex belt is located at the southern part of Beishan orogen, represents the ancient position of the Paleo-Liuyuan Ocean and one of the final sutures of the Beishan orogen (Windley et al., 2007; Mao et al., 2012b; Domeier and Torsvik, 2014; Xiao et al., 2010), is one of the key areas to constraint the evolution of the Beishan orogen. However, when the ocean opened and the nature of the Liuyuan Ocean and the early-stage tectonic evolution of the Beishan orogen are rarely discussed and debatable (Zuo et al., 1991; Liu and Wang, 1995; Nie et al., 2002a; Xiao et al., 2010; Mao et al., 2012b; Wang et al., 2016; Saktura et al., 2017). In this study, we report our new discovery of Proterozoic to Cambrian ophiolite fragments in the Huaniushan area in the southern Beishan orogen and present new geological, whole-rock geochemical, and Sr-Nd isotopic data for representative mafic rocks to constrain their genesis and reveal the early geodynamic evolution of the southern Altaids.
The Beishan Orogen is located in a key tectonic position of the southern Altaids, connecting with the eastern Tianshan suture to the west and the Solonker suture to the east (Figures 1A,B). The tectonics of the Beishan collages is characterized by several blocks/arcs separated by several ophiolitic belts which are regarded as suture zones (Zuo et al., 1991; Liu and Wang, 1995; Nie et al., 2002a; Xiao et al., 2010). These major mélange (accretionary complex) belts are the Kanguer-Hongshishan mélange, Xingxingxia-Shibanjin mélange, Hongliuhe mélange and Liuyuan accretionary complex (Figure 1B). A detailed description of these fault zones and terranes can be found in related references (Zuo et al., 1991; Liu and Wang, 1995; Nie et al., 2002a; Zuo et al., 2003; Xiao et al., 2010; Mao et al., 2012b). Here, we mainly introduced the regional geology associated with the Shuangyingshan–Huaniushan arc and Liuyuan accretionary complex and Shibanshan arc (Figures 1B,C).
The Shuangyingshan–Huaniushan arc is a composite arc, similar to the Japan arc (Xiao et al., 2010; Mao et al., 2012b). The northern part of the Shuangyingshan–Huaniushan arc comprises the Precambrian to Ordovician shelf clastic sediments and carbonates on which the Paleozoic Huaniushan arc was built. The late Proterozoic is characterized by a marine sedimentary layer (Zuo et al., 1991; Nie et al., 2002b; Xiao et al., 2010; Song et al., 2013). The Ordovician–Permian Huaniushan arc developed on the southern margin of the Shuangyingshan block. It comprises Ordovician–Permian calc-alkaline basalts, andesites, rhyolites, tuffs, and volcaniclastic rocks with interlayered clastics and carbonate (GSBGMR, 1989; Zuo et al., 1990; Zuo et al., 1991; Nie et al., 2002a; Xiao et al., 2010; Mao et al., 2012a; 2012b; Guo et al., 2014). A suite of metamorphic rocks is distributed discontinuously on the southern margin of the Huaniushan arc, mainly comprising gneisses, migmatites, schists, and marbles that show greenschist-to eclogite-facies metamorphism (Mei et al., 1998; Mei et al., 1999; Liu et al., 2002; Liu et al., 2011; Qu et al., 2011; Yang et al., 2006). The ages of the complex are not well-constrained; for example, the Ordovician–Silurian ages are mainly designated by regional comparison with the rocks nearby (GSBGMR, 1989; Zuo et al., 1990; Zuo et al., 1991; Nie et al., 2002a). Recently, U-Pb zircon dates reveal that the protolith of the Gubaoquan eclogites has an age of 819–1,007 Ma (Yang et al., 2006; Liu et al., 2011; Qu et al., 2011; Saktura et al., 2017), an augen orthogneiss has a zircon U-Pb age of 920 ± 14 Ma (Saktura et al., 2017), and the sandstone, schist, and mylonite have zircon U-Pb ages ranging from 293 to 457 Ma (Tian and Xiao, 2020; Wang et al., 2016). Different type intrusions are extensive and age of Ordovician to Triassic (Figure 2, (Nie et al., 2002a; Nie et al., 2002b; Zhao et al., 2007; Mao et al., 2012a; Li et al., 2012; Wang et al., 2016).
FIGURE 2. Geological map of the Huaniushan complex in the northern part of the Liuyuan accretionary complex.
The late Paleozoic Shibanshan arc is located on the northern margin of the Dunhuang block (Figures 2A,B), containing low-grade and high-grade metamorphic units. The low-grade unit, on the northern margin of the arc, contains low greenschist facies Devonian–Permian calc-alkaline volcanic rocks, volcaniclastics, tuffs, carbonates, and clastic rocks. The high-grade unit is mainly composed of gneisses, migmatites, schists, mylonitic schists, and marbles, and age of 896–294 Ma (Song et al., 2016; Tian and Xiao, 2020). Abundant granitic intrusions are formed from the Carboniferous to Triassic (Zuo et al., 1990; Zuo et al., 1991; Nie et al., 2002a; Zhang et al., 2010; Zhang et al., 2011; Song et al., 2016; Tian and Xiao, 2020; Zheng et al., 2020).
The Liuyuan accretionary complex, located south of the Huaniushan arc (Figure 1C), contains the Liuyuan, Houhongquan, Huaniushan, Zhangfanshan, Huitongshan complex, and Gubaoquan eclogite. These ophiolitic complexes contain metamorphic basalts, gabbros, hornblendites, ultramafic rocks, cherts, limestones, sediments, and metamorphic tectonic blocks (Zuo et al., 1991; Liu & Wang, 1995; Xiao et al., 2010; Mao et al., 2012b; Wang et al., 2016). The Huaniushan complex is located in the northernmost part of the Liuyuan accretionary complex along the Huaniushan fault (Figure 1C and Figure 2).
In order to understand the composition and structure of the Huaniushan complex, we mapped in detail based on the previous map data (GSBGMR, 1989). The main lithologies and structures that are representative of the complex are described below.
The Huaniushan complex has a block-in-matrix structure in which blocks of ultramafic rocks, gabbros, massive basalt, diabase dykes, cherts, limestone, and sandstone/siltstone embedded and imbricated in a matrix of chlorite–phyllite schist and cleaved sandstone (Figures 2–4), which should be renamed as the Huaniushan ophiolitic mélange.
FIGURE 3. Cross-sections of the Huaniushan complex; (A) cross-section (A-A′) in the western part; (C) cross-section (B-B′) in the eastern part.
FIGURE 4. Field photos and microphotos for different rocks of the Huaniushan complex, Beishan. (A) Serpentinized ultramafic; (B) gabbro; (C) schist basalt; (D) chert and basalt; (E) limestone blocks in basalt; and (F) sandstone. (G–I) Microphotos of gabbro, basalt, and sandstone. Px, pyroxene; Pl, plagioclase; Qz, quartz.
The Huaniushan ophiolitic mélange contains oceanic fragments which were thrusted on the calcareous sediments and volcanic and volcaniclastic rocks or emplaced into sedimentary matrix as large blocks (Figures 2–5). The Huaniushan complex mainly comprises basalt blocks and a few blocks of ultramafic rocks, gabbros, basalt, thinly bedded cherts, limestones, and tuff sandstones (Figure 4). Ultramafic blocks are located along the fault on the southwestern margin of the complex (Figures 2, 3), and are cleaved (Figure 4A). A few gabbros develop along the southern and northern boundary fault in the eastern parts of the complex (Figures 2, 3, 4B). We found a NW-trending chert + limestone+ tuff sandstone layer in the middle of the complex (Figures 3, 4D–F), namely, the thin chert cover on the basalts, the laminated limestone cover the cherts, and finally, the tuff sandstone lay on the limestone as shown in Figures 4D, F. The matrix limestone, sandstones, and chlorite–phyllites are highly cleaved (Figures 4, 5). The southern part Huaniushan Formation (O2hn) matrix calcareous sediments and volcanic and volcaniclastic rocks develop folds and EW-trending subvertical cleavage (Figure 5C). The central parts of the complex (containing the basalt, ultramafic gabbro, and sediments) also underwent subvertical cleavage (Figures 4A, C, F), and the gabbro also comprised gneisses (Figure 5D). The EW-trending, subvertical cleavage in the matrix in the northern parts of the complex is penetrative and has overprinted the bedding so strongly that the primary depositional structures are mostly difficult to observe (Figures 5G, H). And, they were intruded by the Huaniushan granite in the late Triassic and formed the skarn-type Pb-Zn mineralization (Figure 2).
FIGURE 5. Structural deformation photos for different rocks of the Huaniushan complex, Beishan. (A) Huaniushan complex thrust on Huaniushan Formation (O2hn) in the southern part; (B) section of the thrust in the southern part; (C) folded sediments in the southern part; (D) deformed gabbro blocks in the Huaniushan complex; (F) Huaniushan complex thrust on sediments in the northern part; (G) section of the thrust in the northern part; (H) strongly deformed and silicified basalt and suggest the dextral shear; (I) strongly deformed, folded, and silicified basalt.
Thirty samples (twenty-two basalt and eight gabbros) were collected from the outcrops (Figures 2, 3) for major and trace element analyses.
Peridotite is dark gray and strong serpentinized and develops EW-trending foliations with network structures. It mainly consists of serpentine, a few pyroxenes, and magnetite (Figure 4A). Gabbro samples are gray and have altered display (Figures 4B,G). The gabbros in the faults are strongly deformed (Figure 5D). They are characterized by fine-to medium-grained hypidiomorphic textures and mainly contain plagioclase (55–65 vol%), clinopyroxene (30–35 vol%), and olivine (2-3 vol%), with minor amphibole and Fe-Ti oxides (Figure 4G). Most basalt are gray, massive, and altered, and the schist basalts are located along the faults (Figures 4C,D). Basaltic rocks are composed of plagioclase, magnetite, ilmentite, minor olivine, and volcanic glass (dis-glass), and some samples have a little amount of clinopyroxene (Figure 4H).
The sandstone samples were collected in the middle (20HNS34) and northern (18HNS15) part of the Huaniushan complex (Figures 2, 3). These samples are well-bedded and develop EW-trending foliations as shown in Figure 4F. They are mainly composed of bedded quartz and tuffs (Figure 4I).
The zircon U-Pb datings were dated using a LA-ICP-MS with an ESI New Wave NWR 193UC (TwoVol2) laser ablation system connected to an Agilent 8900 Inductively Coupled Plasma Mass Spectrometry (ICPMS) at Beijing Quick-Thermo Science and Technology Co., Ltd,. Details of the procedure can be found in the study by Ji et al., (2020). In situ Lu–Hf isotope measurements were performed using a Thermo Finnigan Neptune-Plus MC–ICP–MS fitted with a J-100 femtosecond laser ablation system Applied Spectra Inc. housed at the Beijing Chron Technology Co., Ltd., Beijing, China. The analytical procedures and calibration methods are similar to those described by Wu et al. (2006). Zircons were ablated for 31 s at a repetition rate of 8 Hz at 16J/cm2, and ablation pits were ∼30 μm in diameter. During analysis, the isobaric interference of 176Lu on 176Hf was negligible due to the extremely low 176Lu/177Hf in zircon (normally <0.002). The mean 173Yb/172Yb value of individual spots was used to calculate the fractionation coefficient (βYb) and then to calculate the contribution of 176Yb to 176Hf. An isotopic ratio of 173Yb/172Yb = 1.35274.
Major elements were determined by X-ray fluorescence spectrometry (XRF); trace elements were analyzed by inductively coupled plasma techniques (ICP) at the Geological Test and Analysis Center of the Beijing Research Institute of Uranium Geology. Details of the procedure can be found in the study by Mao et al. (2018). Sr-Nd isotopic analyses were performed in the Institute of Geology and Geophysics (IGG), Chinese Academy of Sciences, Beijing.
Isotopic compositions of Sr and Nd analyses were analyzed on a Thermo Fisher Scientific Neptune Plus MC-ICP-MS at the Institute of Geology and Geophysics, Chinese Academy of Sciences (IGGCAS) in Beijing. The measurements were carried out following the isotope dilution procedures of Yang YH et al., (2010). A static multi-collection mode was used during the measurements, and a traditional caution exchange technique was adopted for the chemical separation. The mass fractionation corrections for Sr and Nd isotopic ratios were based on 88Sr/86Sr = 8.375209 and 146Nd/144Nd = 0.7219.
The zircon U-Pb dates for the gabbro and sediment blocks are shown in Table 1. Major and trace element contents, zircon Hf, and Sr-Nd isotopic data are shown in Tables 1, 2, 3 and 4.
TABLE 1. Zircon U-Pb ages of gabbros and sediment blocks from the Huaniushan complex in the Beishan orogen, NW China.
TABLE 3. Major (wt%) and trace element (ppm) results for the Huaniushan complex in the Beishan orogen, NW China.
A gabbro and two sedimentary samples from the Huaniushan complex (Figures 3, 6) were analyzed. All the U-Pb and Hf isotopic data are shown in Tables 1 and 2.
FIGURE 6. CL images and Concordia U-Pb diagram for gabbros and sandstone of the Huaniushan complex in Beishan. (A and B) are LA-ICP-MS U-Pb age and CL images of gabbro (20HNS27); (C and D) are LA-ICP-MS U-Pb age and CL images of the sandstone in the complex (20HNS34); (E and F) are LA-ICP-MS U-Pb age and CL images of sandstone (20HNS15) in the northern part of the complex.
The zircon grains from the granite sample 20HNS27 show length/width ratios of 1.2–2 with size ranging from 50 to 120 μm. They are euhedral and transparent and show clear magmatic oscillatory zoning in CL images (Figure 6B). The Th/U ratios of analyzed zircons range from 0.92–2.91 and are consistent with a magmatic origin (Hoskin and Schaltegger, 2003). The 20 analyses give concordant 206Pb/238U ages ranging from 493 to 518 Ma, with a weighted mean age of 504.4 ± 3.2 Ma (MSWD = 3.7; Figure 6A). The age records the crystallization time for the gabbro block. Lu-Hf isotopic analyses of the gabbro yielded 176Hf/177Hf values of 0.282691–283097 and high εHf(t) values (+8.07 to +17.74) (Figure 7).
FIGURE 7. εHf(t) vs. age diagram for the Huaniushan complex in the Beishan orogen. The Hf isotope data of the sediments from the Heiyingshan block are after (Song et al., 2013).
A total of 118 analyses of zircon grains from two sedimentary samples (20YY34 and 18HNS15) from the Huaniushan complex (Figure 6) yielded 116 concordant ages [concordance % > 90% or <110%, the age <1,500 Ma used U/Pb age, and the age >1,500 Ma used Pb/Pb age (Spencer et al., 2016)]. Only concordant ages are described and discussed below.
Zircons from sample 20HNS34 are weak rounded structures and are 50–100 μm long with length/width ratios of 1.0–1.5. They have prominent zones in CL images (Figure 6D). They have variable Th/U values of 0.04–1.88. The 89 analyzed zircon grains yield concordant ages ranging from 309 ± 5 Ma to 2,657 ± 29 Ma. The youngest zircon yields concordant Pb206/U238 age of 309 ± 8 Ma (Figures 6C, 12A). Eighty-one zircon grains (91% of the total) have concordant age of 703 Ma to 1903 Ma, and show three peaks at 706 Ma, 1,093 Ma, and 1880 Ma. Five grains are older than 2033 Ma, scattered ages at 2,468 Ma (Figures 6C, 12A). The youngest zircon age (309 Ma) is interpreted as the MDA of the sandstone. Lu-Hf isotopic analyses of the detrital zircons yielded εHf(t) values ranging from −11.7 to +10.5 (Figure 7).
Zircons from sample 18HNS15 are weak rounded structures too. They are 50–90 μm long, with length/width ratios of 1.0–1.5 and have prominent zones in CL images (Figure 6F). They have variable Th/U values of 0.19–1.36. Of 28 analyzed zircon grains, 27 zircon grains yield concordant ages ranging from 501 ± 5 Ma to 2,703 ± 28 Ma (Figures 6E, 12B). Twenty-three zircon grains (85% of the total) have concordant age range from 824 Ma to 1818 Ma and show four peaks at 840 Ma, 1,168 Ma, 1,680 Ma, and 1876 Ma. Three grains show age of 2,481–2,703 Ma (Figure 12B). The youngest zircon yields concordant Pb206/U238 age of 501 ± 5 Ma (Figures 6E, 12B). This age is interpreted as the MDA of the sandstone.
Whole-rock major, trace elements, and Sr-Nd isotope data of the basalts and gabbros are listed in Tables 3 and 4.
The basalts can be separated into MORB and OIB types according to their element contents.
The major element compositions of the MORB-type basalts and gabbros have variable contents of SiO2 (44.08–51.22 wt%), TiO2 (0.55–1.45 wt%), Al2O3 (11.43–15.01 wt%), MgO (6.25–18.17 wt%), and CaO (8.83–13.98 wt%). They are classified as tholeiitic basalts (Figure 8). These basalts exhibit high Cr (104–1,303 ppm) and Ni (71–649 ppm) concentrations. Their depleted to slightly enriched REE patterns (Figure 9A) are like those of MORBs, with (La/Yb)N ratios of 0.68–1.53 and slightly negative to positive Eu anomalies (δEu = 0.90–1.38) (Figure 9A). On the primitive mantle–normalized spider diagrams (Figure 9B), they have a positive Rb, Ba anomalies, and negative to positive Sr anomalies. Four basaltic samples have relatively low (87Sr/86Sr)i values of 0.70354–0.70475, and high εNd(t) values of +4.5 to +14.5 (Figure 10).
FIGURE 8. Geochemical classification diagrams of the Huaniushan complex in the Beishan orogen, NW China. (A) Zr/Ti vs. Nb/Y diagram (Winchester and Floyd, 1977) and (B) FeOt/MgO vs. SiO2 diagram (Myashiro, 1974).
FIGURE 9. Chondrite-normalized REE patterns and Primitive Mantle (PM)–normalized multi-element diagrams for the gabbros from the Huaniushan complex. The chondrite values are given by Boynton, (1984). The PM, N-MORB, E-MORB, and OIB values are given by Sun and McDonough, (1989). Data of dash lines for the Gubaoquan eclogite are given by Qu et al., (2011).
FIGURE 10. εNd(t) vs. initial (87Sr/86Sr)i (Ma) diagram for the Huaniushan complex in Beishan orogen. DM, Depleted mantle; BSE, Bulk silicate earth; EMI and EMII, enriched mantle; HIMU, mantle with high U/Pb ratio; PREMA (Zindler and Hart, 1986). The Sr-Nd isotope data of the Liuyuan complex are after (Mao et al., 2012b).
Two OIB-type basaltic samples have contents of SiO2 (46.97–46.99 wt%), TiO2 (2.48–2.56 wt%), Al2O3 (8.59–9.06 wt%), CaO (10.51–10.74 wt%), and MgO (10.91–12.67 wt%), and relatively high Cr (656–828 ppm) and Ni (447–532 ppm). They are tholeiitic basalts (Figure 8). They are enriched in LREE and comparable to those of OIB with (La/Yb)N ratios between 7.13 and 7.17 on the REE pattern diagram (Figure 9C). They have positive Nb and Ti anomalies and negative Sr anomalies on the primitive mantle–normalized spider diagrams (Figure 9D). One sample has the highest (87Sr/86Sr)i value of 0.70590 and the lowest εNd(t) value of +4.3 (Figure 10).
The gabbroic rocks have similar geochemical compositions to the MORB-type basalts. They are tholeiitic basalts (Figure 8) and have narrow contents of SiO2 (46.57–49.19 wt %), but wide range of TiO2 (0.55–1.47 wt%), Al2O3 (10.46–17.83 wt%), CaO (8.40–12.14 wt%), and MgO (6.84–16.95 wt%), and high contents of Cr (143–1,535 ppm) and Ni (72–623 ppm). They have slightly enriched REE patterns ((La/Yb) N =(1.15–2.17) and positive Eu anomalies (δEu = 0.90–1.46, Figure 9E). They are enriched in Rb and Ba and have slightly negative to positive Sr and Ti anomalies on the primitive mantle–normalized spider diagrams (Figure 9F). The 176Hf/177Hf ratios of these zircons for the gabbros range from 0.281973 to 0.283097, and the high positive εHf (t) values range from +8.2 to +17.8 (Figure 7).
The zircon U-Pb dates reveal the crystallization time for the gabbro block to be 504.4 ± 3.2 Ma (Figure 6A), suggesting that the Huaniushan complex contains the Cambrian oceanic fragments. Yang J. G. et al. (2010) reported that basalts of the Huaniushan complex yielded zircon U-Pb age of 1,071 ± 5 Ma. Thus, available data indicate that the ophiolitic blocks contain Cambrian to Proterozoic oceanic fragments.
Several methods are used for calculating the MDA of sedimentary rocks from their detrital zircon U-Pb ages (Coutts et al., 2019). Here, we use the youngest grain with a 2σ uncertainty. The sandstone samples (20YY34 and 18HNS15) yield minimum ages of 309 ± 5 Ma and 501 ± 5 Ma, respectively (Figures 6C,E). Thus, the MDA of the sandstone 20YY34 is less than 309 ± 5 Ma, while the MDA of the sandstone 18HNS15 is less than 501 Ma. The least MDA of the sandstone matrix of the Huaniushan complex belt deposit in the tholeiitic Carboniferous, which have younger ophiolite fragments (504 Ma), indicates that the northward subduction beneath the Huaniushan arc may have started at least at ca. 501 Ma, and final accretion of the Huaniushan ophiolitic mélange was after the tholeiitic Carboniferous (309 Ma). Thus, the geochronological studies reveal the blocks of the Huaniushan complex age ranging from 309 Ma to 1,071 Ma.
Numerous ophiolite fragments have been reported in the Liuyuan accretionary complex at the southern margin of the Huaniushan arc (Figure 1B and Table 5). The zircon U-Pb dates reveal that the age of the ophiolite blocks of the Liuyuan accretionary complex is from 1,071 Ma to 270 Ma (Table 5), e.g. 1) the basalts and gabbros of the Huaniushan ophiolitic complex have ages of 1,071 ± 5 Ma and 504 ± 3 Ma [(Yang J. G. et al., 2010); this study], respectively; 2) the Gubaoquan eclogites have protolith ages of 819 Ma to 1,007 Ma (Yang et al., 2006; Liu et al., 2011; Qu et al., 2011; Saktura et al., 2017); 3) the gabbro of the Huitongshan ophiolite has an age of 446 ± 3 Ma (Yu et al., 2012); 4) the gabbro of the Zhangfangshan ophiolite has an age of 363 ± 4 Ma (Yu et al., 2012); 5) gabbros of the Liuyuan ophiolite have an age of 270–286 Ma (Mao et al., 2012b; Zheng et al., 2014; Wang et al., 2016); 6) the gabbro of the Yinaoxia ophiolite has an age of 281 ± 11 Ma (Zheng et al., 2014). The detrital zircon LA-ICPMS U-Pb dates for these sediments and metamorphic sediments blocks revealed that the minimum age of clastic sediments blocks is from 457 Ma to 234 Ma (Wang et al., 2016; Tian and Xiao, 2020; this study).
In summary, the geochronological studies suggest the Liuyuan accretionary complex are composed of Neoproterozoic to late Triassic oceanic crust and sedimentary fragments. The Liuyuan Ocean may have been the latest closed branch of the Paleo-Asian Ocean.
The Huaniushan complex has been thrust-imbricated on the Ordovician volcanic sediments in the northern part of the Liuyuan accretionary complex. They display block-in-matrix structures and are intruded by the late Triassic Huaniushan A-type granite (Li et al., 2012). Different degree schists and cleaved fragments in the complex consist of ultramafic rocks, gabbros, basalts, cherts, limestones, and sandstones which are enclosed in a matrix of chlorite–phyllite strong schist and cleaved sandstone (Figures 4, 5). Ordovician sediments developed sub-vertical tight folds, the axes of which strike east–west. All these structures indicate that the Huaniushan complex underwent intense top-to-the-south thrusting and east–west shearing. Although most of the different rock types in the Huaniushan complex are mutually juxtaposed by thrusts, the blocks of basalt, gabbro, serpentinized ultramafic, limestone, and chert with tuff beds are probably fragments of oceanic plate stratigraphy. They would have provided information on the travel history of the oceanic plate from ridge to trench (Kusky et al., 2013). All the mafic blocks in the Huaniushan complex are tholeiitic magma, but as described above, they consist of MORB- and OIB-type geochemical signatures.
The MORB-type basalts and gabbros have slightly depleted to enriched LREE patterns (La/YbN = 0.68–1.53, Figures 9A,E), as also are the similar trace element patterns with their positive Ba and negative to positive Rb and Sr anomalies (Figures 9B,F). Their enrichments in fluid-soluble elements (Rb and Ba) and Sr-Nd isotope plot above the mantle array indicate seawater alteration (Hawkins, 2003; Reagan et al., 2010) (Figure 10). On Ti-V and Cr-Y diagrams, most of the mafic rocks plot in the field of MORB (Figures 11A,B). And, they further plot as MORB and within-plate tholeiites on the Nb-Zr-Y diagram (Figure 11C), and close to the E-MORB basalt field on the Th/Yb-Nb/Yb diagram (Figure 11D). Their εNd(t) values have relatively high and wide range changes (+4.5 to +14.5) and can be subdivided into two groups. Group 1 has higher εNd(t) values (+11.2 to +14.5) which are more depleted than the MORB-type rocks of the Liuyuan ophiolite (+6.6 to +9.0) and the Gubaoquan eclogite (+6.3 to +6.4) (Qu et al., 2011; Mao et al., 2012b). Group 2 has relatively low εNd(t) values (+4.5- +6.0) which are lower than those of the MORB-type rocks of the Liuyuan ophiolite and Gubaoquan eclogite, but higher than those of the E-MORB–type rocks of the Gubaoquan eclogite (−1.6 to −0.1). The gabbro blocks have high εNf(t) values (+8.2–+17.8). These geochemical and isotopic features suggest that the basalts of the Huaniushan complex blocks contain MORB- and E-MORB–type basalts which are derived from the depleted to relatively enriched mantle.
FIGURE 11. (A) Ti vs. V discrimination diagram for the rocks from the Huaniushan complex (Shervais, 1982). (B) Cr vs. Y discrimination diagram for the rocks from the Huaniushan complex, after (Pearce et al., 1981), BON-boninite, IAT-island arc tholeiite, and MORB-mid-oceanic ridge. The fields of the Lau Basin-Axial Ridge, the Tofua Arc, Mariana Trough and Mariana Arc are after (Hawkins, 2003). (C) Nb-Zr-Y discriminant diagrams (Meschede, 1986) and (D) Th/Yb vs. Nb/Yb discriminant diagram [SHO: shoshonite, CA-Calc: alkaline, TH: tholeiite (Pearce, 2008)]. Symbols are the same as those in Figure 6.
The OIB-type basalts have high contents of TiO2 (2.48–2.56 wt%), MgO (10.91–12.67 wt%), and ΣREE (99–103 ppm). Their REE patterns and trace element patterns plot between the MORB and OIB lines on the chondrite-normalized REE diagrams (Figure 9C). They have relatively lower Nd isotopic value (+4.3) than the MORB-type rocks (Figure 10), suggesting relatively enriched mantle sources.
All these geochemical features demonstrate that these mafic blocks in the Huaniushan complex containing the MORB-, E-MORB-, and OIB-type oceanic crust fragments, which have similar REE and trace patterns to the Gubaoquan eclogite (Figures 9, 11), were probably generated in an oceanic plateau/seamount. These results are consistent with the geological fact that they consist of gabbros, basalts, cherts, and limestones. Combined with the regional data, our results and the 1,071–866 Ma MORB and E-MORB–type ocean slab metamorphic genetic Gubaoquan eclogite (Qu et al., 2011) suggest a hot spot in the Liuyuan oceanic from 1,071 Ma to 466 Ma.
The Huaniushan complex is located in the northernmost part of the Liuyuan accretionary complex which is situated between the Shuangyingshan–Huaniushan arc in the north and the Shibanshan arc in the south (Figure 1B). Therefore, the Shuangyingshan–Huaniushan arc was the main potential provenance for the sandstone matrix of the Huaniushan complex.
The two sandstones (18HNS15 and 20HNS 34) have similar and consistent detrital zircon U-Pb age populations (Figures 12A,B) with dominant age peaks in the period 820–1900 Ma (more than 85% of total concordant ages for each sample), and a second peak at 2,500 Ma. This age spectrum of these detrital zircons for the sedimentary blocks is similar to that of the sediments in the Shuangyinshan–Huaniushan arc (Figure 12C) (Song et al., 2013; Ao et al., 2016). Lu-Hf isotopic analyses of these detrital zircons yielded εHf(t) values ranging from −11.7 to +10.5 (Figure 7), which are plotted in the same area to the sediments in the Shuangyinshan–Huaniushan arc (Song et al., 2013). In summary, our detrital zircon LA-ICPMS U-Pb dates and Lu-Hf isotopic analyses for the sandstone blocks of the Huaniushan complex indicate that they are mainly sourced from the Precambrian blocks. Our detrital zircon LA-ICPMS U-Pb dates also find a few Paleozoic zircon grains, suggesting the young sources. As described before, the Shuangyinshan–Huaniushan arc is a Japanese type island arc. Large volume of granitoids and volcanic rocks was formed by the Liuyuan ocean subduction in the Paleozoic to Neoproterozoic to Triassic [673 Ma–217 Ma (Nie et al., 2002a; Nie et al., 2002b; Zhao et al., 2007; Mao et al., 2012a; Li et al., 2012; Wang et al., 2016); Table 5]. Thus, the Carboniferous to Cambrian zircon grains maybe sourced from these magmas. Both the detrital zircon age spectra and εHf(t) values are comparable with the sedimentary and magmatic record in the Shuangyinshan–Huaniushan arc. The sedimentary samples (20HNS15 and 34) were probably derived from the Shuangyinshan–Huaniushan arc in the north, further to constrain the Huaniushan complex probably formed in the forearc of the Shuangyinshan–Huaniushan arc.
FIGURE 12. Comparison of probability plots for zircon U-Pb ages of sedimentary rocks. (A and B) are from the sedimentary rocks of the Huaniushan complex in the Beishan orogen, (C) is from the metasedimentary rocks of Shuangyinshan block in the Beishan orogen (Song et al., 2013; Ao et al., 2016; Song et al., 2016).
The Liuyuan accretionary complex is very essential to understand the evolution of the Paleo-Asian Ocean and the accretionary orogenic processes of the southern Altaids (Zuo et al., 1991; Xiao et al., 2010; Domeier and Torsvik, 2014; Xiao et al., 2018). The compositions and emplaced processes of the Liuyuan accretionary complex are extremely complicated (Zuo et al., 1991; Liu and Wang, 1995; Qu et al., 2011; Mao et al., 2012b; Yu et al., 2012; Zheng et al., 2014; Wang et al., 2016). As discussed above, the oceanic fragments are aged from Neoproterozoic (1,071 Ma) to Middle Permian (270 Ma).
The geochemical and isotopic studies reveal that these ophiolitic mélange blocks have OIB, E-MORB, and MORB geochemical signatures[(Qu et al., 2011; Mao et al., 2012b; Zheng et al., 2014); this study], For example, our studies indicate that the Huaniushan complex consists of OIB, EMORB, and/or MORB fragments; the protolith of the Gubaoquan eclogite is mainly composed of E-MORB and N-MORB fragments (Qu et al., 2011); the Liuyuan ophiolite mainly consists of MORB fragments (Mao et al., 2012b); the fragments of the Yinaoxia ophiolite have an OIB-like mantle source and are metasomatized by fluids and/or melts derived from the subducted slab (Zheng et al., 2014). These data suggest that the oceanic fragments of the Liuyuan accretionary complex are composed of seamounts and the oceanic crust. The 1,071 Ma to 504 Ma E-MORB and OIB fragments indicate that the oceanic island or seamounts is an important component of the Liuyuan oceanic plate. At least, the mantle plume is continuously active from 1,071 Ma to 504 Ma. The clastic sediments and metamorphic clastic blocks of the Liuyuan accretionary complex contain Middle Ordovician to Low strata (GSBGMR, 1989; Wang et al., 2016; Shi et al., 2018; Xu et al., 2019). In summary, the ophiolitic blocks of the Liuyuan accretionary complex consist of Neoproterozoic to Middle Permian seamounts and/or oceanic islands and oceanic crust fragments, Neoproterozoic to Triassic sediments units, and Neoproterozoic to late Triassic granitic and volcanic rocks.
Our data, integrated with published information on the Huaniushan ophiolitic mélange and the Liuyuan accretionary complex in the Beishan orogen, provide new constraints on the tectonic evolution and the geodynamic mechanism of the southern Paleo-Asian Ocean from the late Mesoproterozoic to late Triassic (Figure 13):
FIGURE 13. Schematic tectonic diagrams illustrating the development of the Liuyuan accretionary complex in the Beishan orogen, NW China. (A) Neoproterozic. (B) Cambrian to Middle ordovician. (C) Late carboniferous. (D) Early permian to late triassic.
The Liuyuan ocean, a branch basin of the Paleo-Asian Ocean, may be born in the late Mesoproterozoic (1,071 Ma) by the mantle plume or earlier. Mantle plumes continuously acted from late Mesoproterozoic (1,071 Ma) to late Cambrian (504 Ma) and formed a series of seamounts and/or plateaux (Figure 13A). In the late Cambrian to Middle Ordovician (Figure 13B), the deep subduction of the seamount with oceanic crust formed the Gubaoquan eclogites (Liu et al., 2011; Qu et al., 2011; Saktura et al., 2017). In the late Carboniferous (Figure 13C), the Huaniushan oceanic and seamount ophiolite fragments were docked on the southern margin of the Shuangyinshan–Huaniushan arc. In the early Permian to late Triassic (Figure 13D), large volume of the Liuyuan MORB-type ophiolitic blocks suggest that the Liuyuan Ocean was still growing (Mao et al., 2012b; Zheng et al., 2014; Wang et al., 2016), which is consistent with volume of Middle Permian–Triassic arc-related granites formed in the Shibanshan and Huaniushan arc, for example, high-Mg diorite, Nb-enriched dikes, and adakites (Li et al., 2012; Zheng et al., 2020). Finally, this branch of the Paleo-Asian Ocean was closed after 234 Ma (Ao et al., 2021), and the Liuyuan Ocean may have been the final closed branch of the Paleo-Asian Ocean.
1. The Huaniushan complex, located in the northernmost part of the Liuyuan accretionary complex, Beishan, is composed of blocks of serpentinized ultramafic rocks, gabbros, basalt, cherts, and limestones within a strongly deformed and cleaved matrix of sandstone and schist.
2. A gabbro block yields zircon U-Pb age of 504 ± 3 Ma. The gabbroic and basaltic blocks have Mid-Ocean-Ridge (MORB)-type and Ocean-Island-Basalt (OIB)-type geochemical characters and high values of εNd(t) (+4.3 to +14.5) and εHf(t) (+8.07 to +17.74).
3. The maximum depositional ages of two sandstone samples (20HNS34 and 20HNS15) from the complex matrix were 309 ± 8 Ma and 501 ± 5 Ma, respectively, indicating that the Huaniushan complex contains matrix rocks varying from 504 Ma to 309 Ma.
4. U-Pb ages and Hf isotopes of detrital zircons from the matrix sandstones indicate that they were derived only from the Shuangyingshan–Huaniushan arc to the north.
5. Available geochronological data reveal that the oceanic blocks and sedimentary matrix of the Liuyuan accretionary complex contain ages of 1,071–270 Ma and 920–234 Ma, respectively. These data suggest that the Liuyuan ocean, a major branch of the Paleo-Asian Ocean may have experienced a prolonged tectonic history, starting in the Late Mesoproterozoic (1,071 Ma), through a long subduction with development of a series of seamounts and/or plateaus emplaced into the Liuyuan accretionary complex in the Paleozoic. The Liuyuan ocean may have been closed later than the late Triassic (234 Ma).
The raw data supporting the conclusion of this article will be made available by the authors, without undue reservation.
All authors have been involved in the study. QM and WX initiated the idea and designed the studies. QM, SA, and MW finished the field, petrology, and geochemical experiments. DS and RL performed the zircon dating and Hf isotopic analysis. HW and ZT processed the geochemical data. QM and SA wrote the original manuscript. WX and BW worked with the geological model and finalized the manuscript.
This study was financially supported by the National Natural Science Foundation of China (41888101, 41822204, and 41802067), the One Hundred Talent Program of the Chinese Academy of Sciences (CAS), the National Key Research and Development Program of China (2017YFC0601201), the Chinese Ministry of Land and Resources for the Public Welfare Industry Research (201411026-1), the “Light of West China” Program of the CAS (2017-XBQNXZ-B-013, 2018-XBYJRC-003), and the Project of China–Pakistan Joint Research Center on Earth Sciences of the CAS (131551KYSB20200021). This is a contribution to IGCP 622.
Author QM was employed by the company Redrock Mining CO., Ltd.
The remaining authors declare that the research was conducted in the absence of any commercial or financial relationships that could be construed as a potential conflict of interest.
All claims expressed in this article are solely those of the authors and do not necessarily represent those of their affiliated organizations, or those of the publisher, the editors, and the reviewers. Any product that may be evaluated in this article, or claim that may be made by its manufacturer, is not guaranteed or endorsed by the publisher.
Allen, M. B., Engör, A. M. C., and Natal’in, B. A. (1995). Junggar, Turfan and Alakol Basins as Late Permian to ?Early Triassic Extensional Structures in a Sinistral Shear Zone in the Altaid Orogenic Collage, Central Asia. J. Geol. Soc. 152, 327–338. doi:10.1144/gsjgs.152.2.0327
Ao, S., Xiao, W., Windley, B. F., Mao, Q., Han, C., Zhang, J. E., et al. (2016). Paleozoic Accretionary Orogenesis in the Eastern Beishan Orogen: Constraints from Zircon U-Pb and 40 Ar/39 Ar Geochronology. Gondwana Res. 30, 224–235. doi:10.1016/j.gr.2015.03.004
Ao, S. J., Mao, Q. J., Windley, B. F., Song, D. F., Zhang, Z. Y., and Zhang, J. E. (2021). The Youngest Matrix of 234 Ma of the Kanguer Accretionary Mélange Containing Blocks of N-MORB Basalts: Constraints on the Northward Subduction of the Paleo-Asian Kanguer Ocean in the Eastern Tianshan of the Southern Altaids. Inter. J. Earth Sci. 110, 791–808.
Bazhenov, M. L., Collins, A. Q., Degtyarev, K. E., Levashova, N. M., Mikolaichuk, A. V., Pavlov, V. E., et al. (2003). Paleozoic Northward Drift of the North Tien Shan (Central Asia) as Revealed by Ordovician and Carboniferous Paleomagnetism. Tectonophysics 366, 113–141. doi:10.1016/s0040-1951(03)00075-1
Boynton, W. V. (1984). “Cosmochemistry of the Rare Earth Elements: Meteorite Studies,” in Rare Earth Element Geochemistry. Editor P. Henderson (Amsterdam: Elsevier), 63–114. doi:10.1016/b978-0-444-42148-7.50008-3
Buchan, C., Pfänder, J., Kröner, A., Brewer, T. S., Tomurtogoo, O., Tomurhuu, D., et al. (2002). Timing of Accretion and Collisional Deformation in the Central Asian Orogenic belt: Implications of Granite Geochronology in the Bayankhongor Ophiolite Zone. Chem. Geol. 192, 23–45. doi:10.1016/s0009-2541(02)00138-9
Coleman, R. G. (1989). Continental Growth of Northwest China. Tectonics 8, 521–635. doi:10.1029/tc008i003p00621
Coutts, D. S., Matthews, W. A., and Hubbard, S. M. (2019). Assessment of Widely Used Methods to Derive Depositional Ages from Detrital Zircon Populations. Geosci. Front. 10, 1421–1435. doi:10.1016/j.gsf.2018.11.002
Dobretsov, N. L., Berzin, N. A., and Buslov, M. M. (1995). Opening and Tectonic Evolution of the Paleo-Asian Ocean. Int. Geol. Rev. 37, 335–360. doi:10.1080/00206819509465407
Domeier, M., and Torsvik, T. H. (2014). Plate Tectonics in the Late Paleozoic. Geosci. Front. 5, 303–350. doi:10.1016/j.gsf.2014.01.002
Duan, J., Xu, G., Qian, Z., Zhang, J., Ma, B., Gao, W., et al. (2021). Petrogenesis and Ni-Cu Exploration Potential of Devonian Mafic–Ultramafic Intrusions in the Southern Part of the Central Asian Orogenic Belt, NW China: Constraints From Zircon O Isotopes and Whole-Rock Sr-Nd Isotopes. Int. Geol. Rev. 1–19. doi:10.1080/00206814.2021.1934739
GSBGMR (1989). Regional Geology of Gansu Province. Geological Memoirs. Beijing: Geological Publishing House.
Guo, Q., Xiao, W., Hou, Q., Windley, B. F., Han, C., Tian, Z., et al. (2014). Construction of Late Devonian Dundunshan Arc in the Beishan Orogen and its Implication for Tectonics of Southern Central Asian Orogenic Belt. Lithos 184-187, 361–378. doi:10.1016/j.lithos.2013.11.007
Hawkins, J. W. (2003). Geology of Supra-subduction Zones-Implications for the Origin of Ophiolites. Geol. Soc. Am. Spec. Paper 373, 227–268. doi:10.1130/0-8137-2373-6.227
Hoskin, P. W. O., and Schaltegger, U. (2003). The Composition of Zircon and Igneous and Metamorphic Petrogenesis. Rev. Mineral. Geochem. 53, 27–62. doi:10.1515/9781501509322-005
Ji, W. Q., Wu, F. Y., Wang, J. M., Liu, X. C., and Zhang, C. (2020). Early Evolution of Himalayan Orogenic belt and Generation of Middle Eocene Magmatism: Constraint from Haweng Granodiorite Porphyry in the Tethyan Himalaya. Front. Earth Sci. 8, 236. doi:10.3389/feart.2020.00236
Kröner, A., Windley, B. F., Badarch, G., Tomurtogoo, O., Hegner, E., Jahn, B. M., et al. (2007). Accretionary Growth and Crust Formation in the Central Asian Orogenic Belt and Comparison with the Arabian-Nubian Shield. Geol. Soc. Am. Mem. 200, 181–209. doi:10.1130/2007.1200(11)
Kusky, T. M., Windley, B. F., Safonova, I., Wakita, K., Wakabayashi, J., Polat, A., et al. (2013). Recognition of Ocean Plate Stratigraphy in Accretionary Orogens through Earth History: A Record of 3.8billion Years of Sea Floor Spreading, Subduction, and Accretion. Gondwana Res. 24, 501–547. doi:10.1016/j.gr.2013.01.004
Li, S., Wang, T., Tong, Y., Hong, D. W., and Ouyang, Z. Y. (2009). Identification of the Early Devonian Shuangfengshan A-type Granites in Liuyuan Area of Beishan and its Implications to Tectonic Evolution. Acta Petrol. Mineral. 28, 407–422. (in Chinese with English abstract)
Li, S., Wang, T., Tong, Y., Wang, Y. B., Hong, D. W., and Ouyang, Z. X. (2011). Zircon U-Pb Age, Origin and its Tectonic Significances of Huitongshan Devonian K-Feldspar Granites from Beishan Orogen, NW China. Acta Petrol. Sin. 27, 3055–3070. (in Chinese with English abstract).
Li, S., Wang, T., Wilde, S. A., Tong, Y., Hong, D., and Guo, Q. (2012). Geochronology, Petrogenesis and Tectonic Implications of Triassic Granitoids from Beishan, NW China. Lithos 134-135, 123–145. doi:10.1016/j.lithos.2011.12.005
Liu, X. Y., and Wang, Q. (1995). Tectonics of Orogenic Belts in the Beishan Mountains, Western China and Their Evolution. Geosci. Stud. 28, 37–48. (in Chinese with English abstract).
Liu, X. C., Wu, G. G., Chen, B. L., and Shu, B. (2002). Metamorphic History of Eclogites from Beishan, Gansu Province. Acta Geosci. Sin. 23, 25–29. (in Chinese with English abstract).
Liu, X., Chen, B., Jahn, B. M., Wu, G., and Liu, Y. (2011). Early Paleozoic (Ca. 465 Ma) Eclogites from Beishan (NW China) and Their Bearing on the Tectonic Evolution of the Southern Central Asian Orogenic Belt. J. Asian Earth Sci. 42, 715–731. doi:10.1016/j.jseaes.2010.10.017
Liu, Y., Li, W., Ma, Y., Feng, Z., Guan, Q., Li, S., et al. (2021). An Orocline in the Eastern Central Asian Orogenic Belt. Earth Sci. Rev. 221, 103808.
Mao, Q., Xiao, W., Fang, T., Wang, J., Han, C., Sun, M., et al. (2012a). Late Ordovician to Early Devonian Adakites and Nb-Enriched Basalts in the Liuyuan Area, Beishan, NW China: Implications for Early Paleozoic Slab-Melting and Crustal Growth in the Southern Altaids. Gondwana Res. 22, 534–553. doi:10.1016/j.gr.2011.06.006
Mao, Q., Xiao, W., Windley, B. F., Han, C., Qu, J., Ao, S., et al. (2012b). The Liuyuan Complex in the Beishan, NW China: a Carboniferous-Permian Ophiolitic Fore-Arc Sliver in the Southern Altaids. Geol. Mag. 149, 483–506. doi:10.1017/s0016756811000811
Mao, Q., Yu, M., Xiao, W., Windley, B. F., Li, Y., Wei, X., et al. (2018). Skarn-mineralized Porphyry Adakites in the Harlik Arc at Kalatage, E. Tianshan (NW China): Slab Melting in the Devonian-Early Carboniferous in the Southern Central Asian Orogenic Belt. J. Asian Earth Sci. 153, 365–378. doi:10.1016/j.jseaes.2017.03.021
Mei, H., Yu, H., Li, Q., Lu, S., Li, H., Zuo, Y., et al. (1998). The First Discovery of Eclogite and Palaeoproterozoic Granitoids in the Beishan Area, Northwestern Gansu Province, China. Chin. Sci. Bull. 44, 356–361. (in Chinese).
Mei, H. L., Li, H. M., Lu, S. N., Yu, H. F., Zuo, Y. C., and Li, Q. (1999). The Age and Origin of the Liuyuan Granitoid, Northwestern Gansu. Acta Petrol. Mineral. 18, 14–17. (in Chinese with English abstract).
Meschede, M. (1986). A Method of Discriminating between Different Types of Mid-ocean ridge Basalts and continental Tholeiites with the Nb-1bZr-1bY Diagram. Chem. Geol. 56, 207–218. doi:10.1016/0009-2541(86)90004-5
Myashiro, A. (1974). Volcanic Series in Island Arc and Active continental Margins. Am. J. Sci. 274, 321–355.
Nie, F. J., Jiang, S. H., Bai, D. M., Wang, X. L., Su, X. X., Li, J. C., et al. (2002a). Metallogenic Studies and Ore Prospectiong in the Conjuction Area of Inner Mongolia Autonomous Region, Gansu Province and Xinjiang Uygur Autonomous Region (Beishan Mt.), Northwest China. Beijing: Geological Publishing House.
Nie, F. J., Jiang, S. H., Liu, Y., Chen, W., Liu, X. Y., and Zhang, S. H. (2002b). 40Ar/39Ar Isotopic Age Dating on K-Feldspar Separates from Eastern Huanniushan Granite, Gansu Province, and its Geological Significance. Chin. J. Geology. 37, 415–422. (in Chinese with English abstract).
Pearce, J. A., Alabaster, T., Shelton, A. W., and Searle, M. P. (1981). The Oman Ophiolite as a Cretaceous Arc-basin Complex: Evidence and Implications. Philos. Trans. R. Soc. Lond. ser. A 300, 299–317.
Pearce, J. A. (2008). Geochemical Fingerprinting of Oceanic Basalts with Applications to Ophiolite Classification and the Search for Archean Oceanic Crust. Lithos 100, 14–48. doi:10.1016/j.lithos.2007.06.016
Qu, J. F., Xiao, W. J., Windley, B. F., Han, C. M., Mao, Q. G., Ao, S. J., et al. (2011). Ordovician Eclogites from the Chinese Beishan: Implications for the Tectonic Evolution of the Southern Altaids. J. Metamorp. Geol. 29, 803–820. doi:10.1111/j.1525-1314.2011.00942.x
Reagan, M. K., Ishizuka, O., Stern, R. J., Kelley, K. A., Ohara, Y., Blichert-Toft, J., et al. (2010). Fore-arc Basalts and Subduction Initiation in the Izu-Bonin-Mariana System. Geochem. Geophys. Geosys. 11, Q03X12. doi:10.1029/2009gc002871
Safonova, I. Y., and Santosh, M. (2014). Accretionary Complexes in the Asia-Pacific Region: Tracing Archives of Ocean Plate Stratigraphy and Tracking Mantle Plumes. Gondwana Res. 25, 126–158. doi:10.1016/j.gr.2012.10.008
Saktura, W. M., Buckman, S., Nutman, A. P., Belousova, E. A., Yan, Z., and Aitchison, J. C. (2017). Continental Origin of the Gubaoquan Eclogite and Implications for Evolution of the Beishan Orogen, Central Asian Orogenic Belt, NW China. Lithos 294-295, 20–38. doi:10.1016/j.lithos.2017.10.004
Schulmann, K., and Paterson, S. (2011). Asian continental Growth. Nat. Geosci. 4, 827–829. doi:10.1038/ngeo1339
Şengör, A. M. C., Natal'in, B. A., and Burtman, U. S. (1993). Evolution of the Altaid Tectonic Collage and Paleozoic Crustal Growth in Eurasia. Nature 364, 209–304.
Shervais, J. W. (1982). Ti-V Plots and the Petrogenesis of Modern and Ophiolitic Lavas. Earth Planet. Sci. Lett. 59, 101–118. doi:10.1016/0012-821x(82)90120-0
Shi, J., Lu, J., Wei, J., Niu, Y., Jiang, T., Han, X., et al. (2018). Petrology, Geochemistry and Sedimentary Environment of Permian Siliceous Rocks in Yingen-Ejin basin and its Adjacent Areas. Geol. Bull. China 37, 120–131. (in Chinese with English abstract).
Song, D., Xiao, W., Han, C., Tian, Z., and Wang, Z. (2013). Provenance of Metasedimentary Rocks from the Beishan Orogenic Collage, Southern Altaids: Constraints from Detrital Zircon U-Pb and Hf Isotopic Data. Gondwana Res. 24, 1127–1151. doi:10.1016/j.gr.2013.02.002
Song, D., Xiao, W., Windley, B. F., Han, C., and Yang, L. (2016). Metamorphic Complexes in Accretionary Orogens: Insights from the Beishan Collage, Southern Central Asian Orogenic Belt. Tectonophysics 688, 135–147. doi:10.1016/j.tecto.2016.09.012
Spencer, C. J., Kirkland, C. L., and Taylor, R. J. M. (2016). Strategies towards Statistically Robust Interpretations of In Situ U-Pb Zircon Geochronology. Geosci. Front. 7, 581–589. doi:10.1016/j.gsf.2015.11.006
Sun, S. S., and McDonough, W. F. (1989). “Chemical and Isotopic Systematic of Oceanic Basalts: Implicattions for Mantle Composition and Process,” in Magmatism in the Ocean Basins. Editors A. D. Sauders, and M. J. Norry (London, United Kingdom: Geological Society London Special Publication), 3l3–345.
Tian, Z., and Xiao, W. (2020). An Andean‐type Arc Transferred into a Japanese‐type Arc at Final Closure Stage of the Palaeo‐Asian Ocean in the Southernmost of Altaïds. Geol. J. 55, 2023–2043. doi:10.1002/gj.3700
Wang, L., Yang, J., Wang, X., Xie, X., Jiang, A., Li, W., et al. (2015). Characteristics of Dashantou Basic-Ultrabasic Complex and its Comprehensive Information Prospecting Model. Mod. Mining 2, 72–76. (in Chinese with English abstract).
Wang, Y., Luo, Z., Santosh, M., Wang, S., and Wang, N. (2016). The Liuyuan Volcanic Belt in NW China Revisited: Evidence for Permian Rifting Associated with the Assembly of continental Blocks in the Central Asian Orogenic Belt. Geol. Mag. 154, 265–285. doi:10.1017/s0016756815001077
Wilhem, C., Windley, B. F., and Stampfli, G. M. (2012). The Altaids of Central Asia: A Tectonic and Evolutionary Innovative Review. Earth Sci. Rev. 113, 303–341. doi:10.1016/j.earscirev.2012.04.001
Winchester, J. A., and Floyd, P. A. (1977). Geochemical Discrimination of Different Magma Series and Their Differentiation Products Using Immobile Elements. Chem. Geol. 20, 325–343. doi:10.1016/0009-2541(77)90057-2
Windley, B. F., Alexeiev, D., Xiao, W., Kröner, A., and Badarch, G. (2007). Tectonic Models for Accretion of the Central Asian Orogenic belt. J. Geol. Soc. 164, 31–47. doi:10.1144/0016-76492006-022
Wu, F.-Y., Yang, Y.-H., Xie, L.-W., Yang, J.-H., and Xu, P. (2006). Hf Isotopic Compositions of the Standard Zircons and Baddeleyites Used in U-Pb Geochronology. Chem. Geol. 234, 105–126. doi:10.1016/j.chemgeo.2006.05.003
Xiao, W. J., Mao, Q. G., Windley, B. F., Han, C. M., Qu, J. F., Zhang, J. E., et al. (2010). Paleozoic Multiple Accretionary and Collisional Processes of the Beishan Orogenic Collage. Am. J. Sci. 310, 1553–1594. doi:10.2475/10.2010.12
Xiao, W., Windley, B. F., Allen, M. B., and Han, C. (2013). Paleozoic Multiple Accretionary and Collisional Tectonics of the Chinese Tianshan Orogenic Collage. Gondwana Res. 23, 1316–1341. doi:10.1016/j.gr.2012.01.012
Xiao, W., Windley, B. F., Han, C., Liu, W., Wan, B., Zhang, J. E., et al. (2018). Late Paleozoic to Early Triassic Multiple Roll-Back and Oroclinal Bending of the Mongolia Collage in Central Asia. Earth Sci. Rev. 186, 94–128. doi:10.1016/j.earscirev.2017.09.020
Xie, J., Di, P., Yang, J., Chen, W., Wei, H., and Zhai, X. (2018). LA-ICP-MS Zircon U-Pb Age, Geochemistry and Tectonic Implications of Metamorphic Dacite from Huanjiushan Group in Beishan Area, Gansu, China. Northwestern Geol. 51, 54–64. (in Chinese with English abstract)
Xie, W., Song, X.-Y., Deng, Y.-F., Wang, Y.-S., Ba, D.-H., Zheng, W.-Q., et al. (2012). Geochemistry and Petrogenetic Implications of a Late Devonian Mafic-Ultramafic Intrusion at the Southern Margin of the Central Asian Orogenic Belt. Lithos 144-145, 209–230. doi:10.1016/j.lithos.2012.03.010
Xu, W., Xu, X., Niu, Y., Song, B., Chen, G., Shi, J., et al. (2019). Geochronology and Petrogenesis of the Permian marine basalt in the Southern Beishan Region and Their Tectonic Implications. Acta Geol. Sin. 93, 1928–1953. (in Chinese with English abstract). doi:10.1111/1755-6724.13758
Yang, J. S., Wu, C. L., Chen, S. Y., Shi, R. D., Zhang, J. X., Meng, F. C., et al. (2006). Neoproterozoic Eclogitic Metamorphic Age of the Beishan Eclogite of Gansu, China: Evidence from SHRIMP U-Pb Isotope Dating. Geol. China 33, 317–325. (in Chinese with English abstract).
Yang, J. G., Zhai, J. Y., Yang, H. W., Wang, C. F., Xie, C. L., Wang, X. H., et al. (2010). LA-ICP-MS Zircon U-Pb Dating of basalt and its Geological Significance in Huaniushan Pb-Zn deposit, Beishan Area, Gansu, China. Geol. Bull. China 29, 1017–1023. (in Chinese with English abstract).
Yang, Y. H., Zhang, H.-f., Chu, Z.-y., Xie, L.-w., and Wu, F.-y. (2010). Combined Chemical Separation of Lu, Hf, Rb, Sr, Sm and Nd from a Single Rock Digest and Precise and Accurate Isotope Determinations of Lu-Hf, Rb-Sr and Sm-Nd Isotope Systems Using Multi-Collector ICP-MS and TIMS. Int. J. Mass Spectrom. 290, 120–126. doi:10.1016/j.ijms.2009.12.011
Yang, J., Wang, L., Xie, X., Qi, Q., Jiang, A., and Zhang, Z. (2016). SHRIMP Zircon U-Pb Age and its Signification of Guaishishan Mafic-Ultramafic Complex in Beishan Mountains, Gansu Province. Geotecton. Metallog. 40, 98–108.
Yu, J. Y., Li, X. G., Wang, G. Q., Wu, P., and Yan, Q. J. (2012). Zircon U-Pb Ages of Huitongshan and Zhangfangshan Ophiolite in Beishan of Gansu-Inner Mongolia Border Area and Their Significance. Geol. Bull. China 31, 2038–2045. (in Chinese with English abstract).
Zhang, W., Wu, T. R., He, Y. K., Feng, J. C., and Zheng, R. G. (2010). LA-ICP-MS Zircon U–Pb Ages of Xijian Quanzi Alkali-Rich Potassium-High Granites in Beishan, Gansu Province, and Their Tectonic Significances. Acta Petrol. Mineral. 29, 719–731. (in Chinese with English abstract).
Zhang, W., Feng, J. C., Zheng, R. G., Wu, T. R., Luo, H. L., He, Y. K., et al. (2011). LA-ICPMS Zircon U–Pb Ages of the Granites from the South of Yin’aoxia and Their Tectonic Significances. Acta Petrol. Sin. 27, 1649–1661. (in Chinese with English abstract).
Zhao, Z. H., Guo, Z. J., and Wang, Y. (2007). Geochronology, Geochemical Characteristics and Tectonic Implications of the Granitoids from Liuyuan Area, Beishan, Gansu Province, Northwest China. Acta Petrol. Sin. 23, 1847–1860. (in Chinese with English abstract).
Zheng, R., Wu, T., Zhang, W., Meng, Q., and Zhang, Z. (2014). Geochronology and Geochemistry of Late Paleozoic Magmatic Rocks in the Yinwaxia Area, Beishan: Implications for Rift Magmatism in the Southern Central Asian Orogenic Belt. J. Asian Earth Sci. 91, 39–55. doi:10.1016/j.jseaes.2014.04.022
Zheng, R. G., Wang, Y. P., Zhang, Z. Y., Zhang, W., Meng, Q. P., and Wu, T. R. (2016). Geochronology and Geochemistry of Yinwaxia Acidic Volcanic Rocks in the Southern Beishan: New Evidence for Permian continental Rift Magmatism. Geotecton. Metallog. 40, 1031–1048. (in Chinese with English abstract).
Zheng, R., Li, J., Zhang, J., Xiao, W., and Wang, Q. (2020). Permian Oceanic Slab Subduction in the Southmost of Central Asian Orogenic Belt: Evidence from Adakite and High-Mg Diorite in the Southern Beishan. Lithos 358-359, 105406. doi:10.1016/j.lithos.2020.105406
Zindler, A., and Hart, S. (1986). Chemical Geodynamics. Annu. Rev. Earth Planet. Sci. 14, 493–571. doi:10.1146/annurev.ea.14.050186.002425
Zuo, G. C., Zhang, S. L., Wang, X., Jin, S. Q., He, G. Q., Zhang, Y., et al. (1990). Plate Tectonics and Metallogenic Regularities in Beishan Region. Beijing: Peking University Publishing House.
Zuo, G., Zhang, S., He, G., and Zhang, Y. (1991). Plate Tectonic Characteristics during the Early Paleozoic in Beishan Near the Sino-Mongolian Border Region, China. Tectonophysics 188, 385–392. doi:10.1016/0040-1951(91)90466-6
Keywords: late mesoproterozoic–late triassic, Huaniushan complex, Liuyuan accretionary complex, southern Beishan, altaids
Citation: Mao Q, Xiao W, Wang H, Ao S, Windley BF, Song D, Sang M, Tan Z, Li R and Wang M (2022) Prolonged Late Mesoproterozoic to Late Triassic Tectonic Evolution of the Major Paleo-Asian Ocean in the Beishan Orogen (NW China) in the Southern Altaids. Front. Earth Sci. 9:825852. doi: 10.3389/feart.2021.825852
Received: 30 November 2021; Accepted: 10 December 2021;
Published: 03 May 2022.
Edited by:
Xiubin Lin, Zhejiang University, ChinaCopyright © 2022 Mao, Xiao, Wang, Ao, Windley, Song, Sang, Tan, Li and Wang. This is an open-access article distributed under the terms of the Creative Commons Attribution License (CC BY). The use, distribution or reproduction in other forums is permitted, provided the original author(s) and the copyright owner(s) are credited and that the original publication in this journal is cited, in accordance with accepted academic practice. No use, distribution or reproduction is permitted which does not comply with these terms.
*Correspondence: Qigui Mao, cWdfbWFvQHNpbmEuY24=; Wenjiao Xiao, d2oteGlhb0BtYWlsLmlnZ2Nhcy5hYy5jbg==; Hao Wang, d2FuZ2hhb0Btcy54amIuYWMuY24=
Disclaimer: All claims expressed in this article are solely those of the authors and do not necessarily represent those of their affiliated organizations, or those of the publisher, the editors and the reviewers. Any product that may be evaluated in this article or claim that may be made by its manufacturer is not guaranteed or endorsed by the publisher.
Research integrity at Frontiers
Learn more about the work of our research integrity team to safeguard the quality of each article we publish.