- 1School of Geoscience and Technology, Southwest Petroleum University, Chengdu, China
- 2Department of Railway Engineering, Sichuan College of Architectural Technology, Chengdu, China
- 3Shale Gas Research Institute, PetroChina Southwest Oil and Gas Field Company, Chengdu, China
To investigate the geological characteristics and exploration potential of shale gas in the southern Sichuan Basin, we analyze the coupling relationship between the hydrocarbon generation and storage conditions of the Longmaxi Formation and discuss the preservation conditions from the lateral and vertical migration mechanisms of shale gas. According to the results, the organic-rich shale at the bottom was formed in a strongly euxinic and anoxic reducing environment, the oxygen content increased in the upper water body in which the Longmaxi Formation was deposited, and the water body became oxidized. The organic matter type in the shale is dominantly type I kerogen and a small amount of type II1 kerogen. The organic matter content is more than 3.0% and is in the high-to postmature stage. The enrichment of siliceous organisms increases the organic matter and enhances the brittleness of shale, resulting in “superior hydrocarbons and a favorable reservoir”. Pyrolysis of organic matter promotes the formation of organic matter pores and dissolution pores, resulting in a coupled “source-reservoir” accumulation control system. The high vertical formation pressure guarantees the sealing of shale and restrains the lateral escape of shale gas. The high-angle intersection of the highly filled fractures and the current crustal stress can effectively enhance the fracture sealing and inhibit the vertical escape of shale gas, forming a three-dimensional effective closure. Hence, the area featuring a short tectonic uplift time, small amplitudes, large-scale underdeveloped fractures, and a high formation pressure coefficient is a favorable area for shale gas exploration, according to the analysis of three-dimensional preservation conditions.
1 Introduction
The USA (United States of America) was the first to implement the commercial development of shale gas. Its successful development of shale gas verified that the shale that is rich in organic matter has tremendous oil and gas exploration potential, which can be used for relieving energy pressure, adjusting the energy structure, and broadening the field of oil and gas. Since then, the United States has become a major natural gas exporter due to the commercial development of shale gas and simultaneous creation of the worldwide popularity of “shale gas” (Jarvie et al., 2007; Curtis et al., 2012; Christophe et al., 2013; Borjigin et al., 2017). An increasing number of countries have been engaged in the exploration of shale gas. Additionally, the subsequent technological innovation has fueled the efficient development of shale gas, and the supporting fundamental geological surveys and development techniques have been advanced (Fan et al., 2020a; Fan et al., 2020b; Hou et al., 2020; Wang H et al., 2020; He et al., 2021). Nevertheless, the commercial development of shale gas has been rarely achieved despite some countries acquiring shale gas via exploration (He et al., 2018).
China possesses multiple sets of shale formations and has natural advantages in shale gas exploration (Wang et al., 2013; Gu et al., 2018; Li et al., 2020). Inspired by international exploration of shale gas, China began to carry out shale gas geological surveys and resource assessments nationwide in 2005 and conducted the first exploration of shale gas in some areas (Ma and Xie, 2018; Nie et al., 2020a; Li Y. et al., 2020). By referring to the geological conditions and exploration of commercial shale gas reservoirs in the west, China has rapidly carried out research on efficient hydrocarbon generation, enrichment, and differential preservation with the lower Paleozoic shale in the southern part of the country as the main target strata. Moreover, the black shale of the Silurian Wufeng-Longmaxi Formation in the Sichuan Basin was considered the main target of shale gas exploration in China according to hydrocarbon, reservoirs, and preservation conditions (Li Y. et al., 2020). Moreover, shale gas exploration was conducted in the Jiaoshiba area of Chongqing according to favorable accumulation conditions by successively arranging several shale gas development wells, of which the JY1 well features a long stable production period and large gas production with a tested capacity of 20.3 × 104 cubic meters per day (“cubic meters per day” is represented by the abbreviation “m3/d" for the rest of the manuscript). After the successful exploration of shale gas at Jiaoshiba, commercial shale gas flows were also found in Nanchuan, Dingshan, and Changning area, suggesting that the Wufeng-Longmaxi Formation in the southern and southeastern Sichuan Basin has great potential for shale gas exploration (Long et al., 2018; Nie et al., 2020b; Ma et al., 2020; Qie et al., 2021).
Compared with conventional gas reservoirs, the low porosity and permeability of shale gas reservoirs determine their characteristics of in situ accumulation and continuous distribution. Shale gas accumulation barely relies on traps and migration conditions (Wang 2017; Li et al., 2021a, 2021b; Song et al., 2021). High organic matter contents, continuous thickness, a high thermal evolution degree, stable accumulation and effective preservation are essential conditions for the formation of shale gas reservoirs.
Although commercial shale flows have been found in different blocks in the southern Sichuan Basin, due to differences in sedimentation and structure, different blocks and different shale gas wells in the same block have shale gas and single-well gas production (Ma et al., 2021). These large differences seriously restrict the next stage of shale gas development in this area. To further clarify the geological conditions and exploration potential of shale gas reservoirs in the southern Sichuan Basin, the Silurian Wufeng-Longmaxi Formation organic-rich shale in the Luzhou, Changning and Weiyuan areas in southern Sichuan was selected as the research object. The sedimentary environment of the shale, three-dimensional reservoir-forming geological conditions and differential preservation have been analyzed to deepen the understanding of the shale gas reservoir-forming geological conditions and show the differences in the exploration potential of different blocks. The research findings are of theoretical and guiding significance in promoting the exploration and efficient development of the next stage of shale gas in the southern Sichuan Basin and similar areas.
2 Geological Setting
Located in Southwest China, the Sichuan Basin is a large petroliferous basin overlying other strata that developed on the Sinian crystalline basement; the Sichuan Basin has always been an important oil and gas production area in China. Moreover, it is also a major area of shale gas development at the current stage in China due to its complete stratigraphic and marine stratigraphic development. The shale of the Silurian Wufeng-Longmaxi Formation is the main stratum for shale gas exploration at this stage due to its wide distribution, large sedimentary thickness, and rich organic matter (Guo et al., 2014; Li et al., 2015). Southern Sichuan is located in the southern Sichuan Basin, which extends over the low steep tectonic belts in southern and southwestern Sichuan (Huang et al., 2012; Guo et al., 2020) and has complicated tectonic deformation. Moreover, a “brush” structure is present; this structure is oriented northward, specifically northeast and north-northeast, and a southward structure is oriented east-west and northwest, which formed in southern Sichuan under the combined effect of the Huayingshan fault and Ziyun-Luodian fault, as shown in Figure 1. The shale in the Wufeng-Longmaxi Formation formed in association with the changes in the ground-surface environment between the Ordovician and the Silurian. After the end of the Ordovician ice age, the southern Sichuan area was surrounded by the Chuanzhong paleouplift, Central Guizhou paleouplift, and Jiangnan-Xuefeng Uplift, all of which formed a large sedimentary depression. As the sedimentary water body in the surrounding area deepened with the occurrence of large-scale transgressions (Long et al., 2018), the open-sea sediment changed into euxinic deep-water sediment, forming an anoxic reducing water body. Moreover, underwater paleouplifts were locally developed, leading to differences in the regional sedimentary water environment. In summary, it is characterized by deep water shelf deposition and transition from uplift to shallow water shelf deposition. The thickness of high-quality shale in the Wufeng-Longmaxi Formations is within 20–80 m. After the deposition of the Longmaxi Formation, superimposed transformations of multiple tectonic movements, such as the Indosinian movement, Yanshan movement, and Himalayan movement, occurred in this study area. Consequently, the tectonic pattern in southern Sichuan witnessed a tremendous transformation. The deformation intensity was remarkably distinct in different regions. Ejective folds arrays arranged alternately between the enclosed anticline and the syncline that were scattered in the south in a wide syncline were present in the northern part of the study area. Among the multiple tectonic movements, the Yanshan movement and Himalayan movement played decisive roles in the tectonic pattern of the southern Sichuan Basin owing to their strong tectonic strengths and large-scale influences. The fault distribution, burial depth and tectonic pattern of shales have a significant impact on the enrichment and preservation of shale gas.
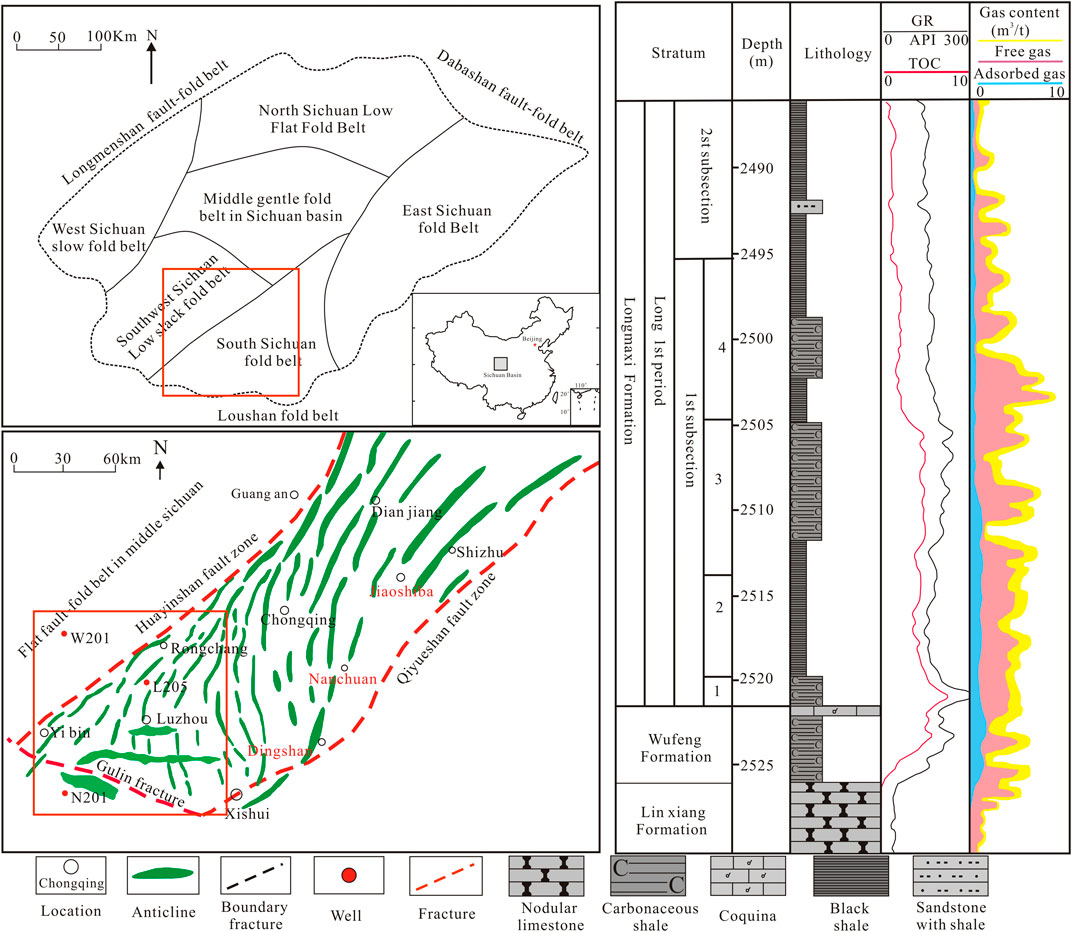
FIGURE 1. Comprehensive histogram of the regional geological setting and stratigraphy in southern Sichuan.
3 Sample and Methods
3.1 Samples
In this study, cores of the Wufeng-Longmaxi Formation from Well L205 in the Luzhou area, Well N201 in the Changning area, and Well W201 in the Weiyuan area were sampled to perform TOC (TOC means total organic carbon content and the “total organic carbon content” is represented by the abbreviation “TOC” for the rest of the manuscript), thermal evolution degree (Ro), whole-rock “X-ray diffraction”, microscopic pore structure and shale porosity experimental tests, as shown in Table 1.
3.2 Method
3.2.1 Natural Gamma Spectroscopy Logging
Natural gamma spectroscopy logging can measure gamma rays of different energies radiated from different radioactive elements. Th and U radioactive elements were measured using the proposed ECLIPS-5700 imaging logging system and Log-IQ fast platform logging system. Specifically, quantitative values in the energy windows of 1764 keV (214Bi) and 2,615 keV (208TI) were changed into U (ppm) and Th (ppm) concentrations with a spectrometer equipped with a NaI detector (Che, 2018). The logging and data processing were conducted in accordance with the petroleum industry standard SY/T5132-2003 “Quality Requirements for Logging Raw Data” (Yin et al., 2018).
3.2.2 Determination of Geochemical Parameters
3.2.2 1 Analysis of Total Organic Carbon Content
The TOC contents of the samples were determined by the solid-state infrared absorption method with an organic sulfur carbon analyzer produced by Leco, and the instrument model was CS230SH. In addition, 99.5% oxygen was used as the carrier, and nitrogen was used as the power gas. Ultrasonic decontamination treatment was conducted on the samples prior to the tests. After drying, the treated shale samples were placed in an agate grinder and ground to smaller than 200 mesh. Then, carbonate mineral components were removed via acid washing before drying at low temperatures. To ensure the stability and reliability of the test results, a standard sample was tested after every 20 experimental samples.
3.2.2 2 Analysis of the Thermal Evolution Degree of Organic Matter
The measured asphalt reflectance was adopted and converted into the equivalent vitrinite reflectance through calculation due to the lack of kerogen vitrinite in the shale in the Wufeng-Longmaxi Formation in southern Sichuan. In the experiment, a combination of a DM4500P polarizing microscope and a QDI302 microphotometer was adopted to measure the asphalt reflectance of the sample on the objective table under nonpolarized light.
3.2.3 Whole-Rock Mineral Characteristics
The mineral contents of the shale were tested with whole-rock “X-ray” diffraction. An X'Pert MPD PRO X-ray diffractometer produced by the Netherlands-based PANalytical Company was adopted as the analytical instrument with tube pressures ranging from 15 to 60 kV, tube flows ranging from 5 to 60 mA, and a maximum power of 3.3 kV. In addition, a fresh shale sample was placed in an agate grinder and ground to smaller than 300 mesh prior to the experiment. In the experiment, a Cu target was used to set the pressure to 45 kV and the current was set to 40 mA. Subsequently, continuous scanning measurements were performed with a minimum diffraction angle of 2° at room temperature. The entire experimental process complied with the petroleum industry’s X-ray diffraction analysis method for clay minerals and common nonclay minerals in sedimentary rocks (SY/T5163-2010).
3.2.4 Scanning Electron Microscopy
A Quanta 450 model environmental scanning electron microscope produced by the FEI company was employed to observe the microscopic pore structure of the shale with acceleration voltages ranging from 200 V to 30 kV, a maximum resolution of 3 nm, and a maximum current of 200 nA. Additionally, 10 kV and 15 nA beam currents were adopted in the experimental process. Before the experiment, the shale sample was first cut to a size of 5 mm × 5 mm×3 mm. Additionally, the accelerating voltage and vacuum treatment were set. Then, argon ion polishing was performed on the surface of the shale sample using an argon ion light beam. Next, the polished sample was placed in an ion grinding mill for grinding to eliminate the unregular shapes on the surface formed by argon ion polishing. Moreover, a carbon coating of 10–20 nm was applied to the surface of the sample to increase its conductivity after the grinding treatment. Then, the treated sample was placed on the sample table for pore type, size, and structure observations of the polished surface using a high-resolution field emission scanning electron microscope.
4 Results
4.1 Analysis of Paleo-Oxygenation Facies and the Euxinic Environment in the Water Body
A large number of graptolite fossils and layered pyrite are developed in the deep black shale at the bottom of the Wufeng-Longmaxi Formation. These are important markers for judging the sedimentary environment and indicate that the sedimentary water body that formed the bottom of the Wufeng-Longmaxi Formation, which had a low oxygen content, was a deep-water anoxic reducing environment. The decreased development of pyrite and graptolite upsection and the concentrated development of laminated silt show that the water shallowed with increased input of terrigenous detritus.
On this basis, the differences in paleo-oxygenated facies and the degree of euxinia of the water that formed the vertical shale were analyzed via geochemical well logging. The results show that the difference between the valence state and the existing pattern of U, Th, V, Cr and other elements in seawater is directly related to the oxidation-deoxidation environment of the water body (Kump and Arthur, 1999; Chen et al., 2006; Melchin and Holmden, 2006; Yan et al., 2009; Chen et al., 2017). Moreover, corresponding research on the analysis of the sedimentary environment of the water body was also conducted for southern and southeastern Sichuan in the Sichuan Basin by utilizing a variety of element combinations (Li et al., 2015; He et al., 2021). Among them, V/Cr, V/(V + Ni), and U/Th and similar elements that are sensitive to oxidation-reduction ratios have been extensively applied analyses of sedimentary environments of paleowater bodies. Specifically, the U/Th ratio is the most frequently applied. A larger ratio indicates a stronger reduction in the water body. Typically, a U/Th ratio greater than 1.25 indicates an anoxic reducing environment; a U/Th ratio between 0.75 and 1.25 indicates an anoxic environment; and a U/Th ratio less than 0.75 indicates an oxidizing environment.
According to the results, an overall trend of increasing and then decreasing is detected in the U/Th ratio of the Wufeng-Longmaxi Formation in the Luzhou, Changning, and Weiyuan areas in southern Sichuan. In general, the U/Th ratio at the bottom of the Wufeng-Longmaxi Formation is greater than 1.25; the U/Th ratio of Well L205 reaches 2.85 in the 1 small layer of the Long 1 sub section submember and then gradually decreases. When the U/Th ratio is between 0.75 and 2.0, the ratio is slightly higher in the 3 small layer, and the U/Th ratio of the 4 small layer is less than 0.75. The U/Th ratio of Well N227 peaks in the 1 small layer and the 4 small layer, reaching 4.2. The U/Th ratio of Well W202 increases dramatically at the top of the Wufeng Formation, reaching 5. Then, it abruptly decreases in the 2 layer, showing that the redox environment of the water body underwent rapid change. The U/Th ratio in the upper part of the Longmaxi Formation is smaller than 0.75, indicating that the water body is rich in oxygen. Evidently, the water body in which the Wufeng-Longmaxi Formation formed changed from an anoxic reducing environment to an oxygen-rich oxidizing environment (Figure 2).
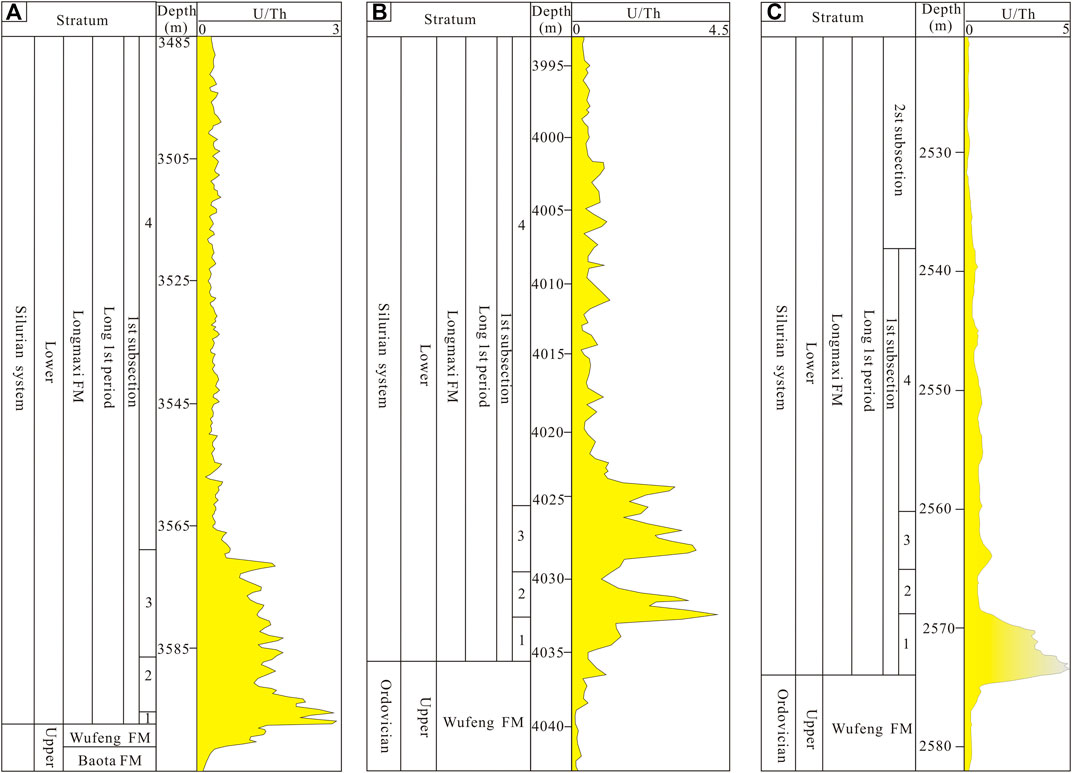
FIGURE 2. U/Th curve of natural gamma spectroscopy logging of the Wufeng-Longmaxi Formation in the southern Sichuan area. (A) Well L205 in the Luzhou area, 3,609 m–3485 m and (B) Well N227 in the Changning area, 4,000 m–4050 m and (C) Well W202 in the Weiyuan area, 2,530 m-2580 m.
The degree of euxinia of the anaerobic basin water can be determined according to the Mo/TOC ratio and the U-Mo covariant relation. Based on the vertical variation in elemental logs in combination with the U-Mo covariant relation, the Mo/TOC ratios of the Wufeng Formation samples are less than 9, showing that the water body in which the Wufeng Formation formed was a strongly euxinic environment. When the TOC contents of the Longmaxi Formation are less than 2 and the Mo/TOC ratios are between 9 and 25, it indicates a moderately euxinic environment. When the TOC contents are greater than 2, the Mo/TOC ratios range from 4.5 to 9, partially ranging from 9 to 25 (Figure 3A). Additionally, taking the Mo/U molar concentration ratio in normal seawater as a reference (1×SW = 7.9) (“the Mo/U molar concentration ratio in seawater” is represented by the abbreviation “SW” for the rest of the manuscript), the Mo/U ratios are mainly between 0.3×SW and 1×SW (Figure 3B), indicating that the sedimentary environment was anoxic and strongly euxinic.
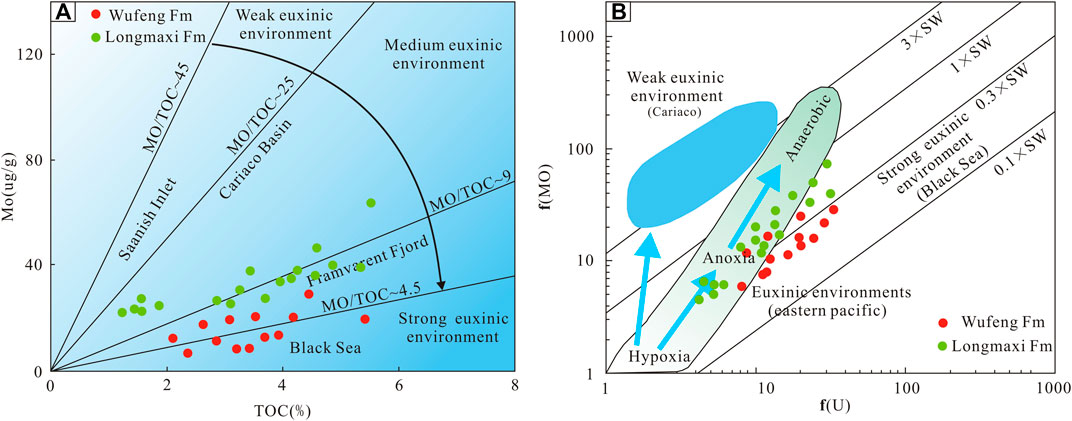
FIGURE 3. Mo/TOC relationship of the Wufeng-Longmaxi Formation in southern Sichuan (A) and U-Mo covariant model; (B). SW represents the Mo/U molar concentration ratio in seawater, SW = 7.9.
4.2 Geochemical Characteristics
4.2.1 Types of Organic Matter
Since kerogen inherits the carbon isotopes of biological precursors, kerogen elemental differences can be adopted when analyzing the types of organic matter. The type of organic matter was studied in this paper using two methods: analyzing the carbon isotopes of organic matter and kerogen element analysis.
4.2.1.1 Organic Matter Carbon Isotope Test
Organic carbon isotopes (δ13C) are a commonly used method for analyzing kerogen types. Specifically, the δ13C (the ratio of 13C and 12C) values of type I kerogen are smaller than −26.5‰, the δ13C values of type II kerogen range from −26.5‰ to −25‰, and the δ13C values of type III kerogen are greater than −25‰. According to the organic carbon isotope analysis in the shale of the Changning, Weiyuan and Luzhou areas, the kerogen 13C values of the Wufeng-Longmaxi Formation shale in the Luzhou area range from −29.98‰ to −31.77‰, indicating type I kerogen. The kerogen 13C values in the Changning and Weiyuan areas range from −27.68 to 31.64‰, indicating that type I kerogen dominated in the main area with the presence of type II 1 kerogen (Table 2).
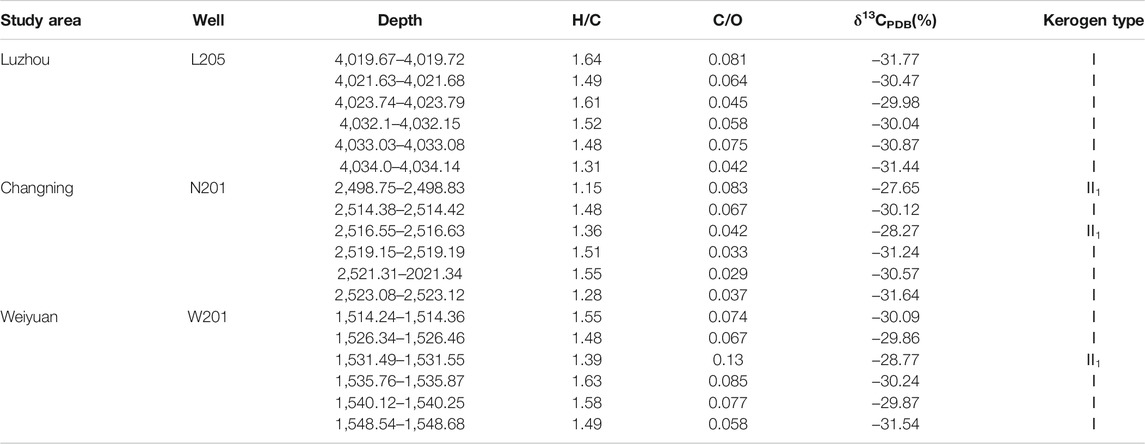
TABLE 2. Isotopic analysis of shale kerogen in the Wufeng-Longmaxi Formations in the Weiyuan, Luzhou, and Changning areas. All experiments were performed at room temperature (26.5 ± 1°C).
4.2.1.2 Analysis of Kerogen Elements
The results of organic kerogen analysis in the shales of the Wufeng-Longmaxi Formation in the Changning and Luzhou areas are displayed in Table 2. The ratio of hydrogen to carbon (“the ratio of hydrogen to carbon” is represented by the abbreviation “H/C″ for the rest of the manuscript) and the ratio of carbon to oxygen (“the ratio of carbon to oxygen” is represented by the abbreviation “C/O″ for the rest of the manuscript) in the Changning area range from 1.31 to 1.64 and from 0.042 to 0.081, respectively; the H/C and C/O ratios in the Luzhou area range from 1.15 to 1.55 and from 0.029 to 0.083, respectively; and the H/C and C/O ratios in the Weiyuan area range from 1.39 to 1.63 and from 0.058 to 0.13, respectively. In addition, the cross-plots of C/O and H/C ratios reveal that the Wufeng-Longmaxi Formation in the Luzhou area is dominated by type I organic matter, while the formations in the Changning and Weiyuan areas are dominated by type I organic matter, with a small amount of type II1 organic matter (Figure 4).
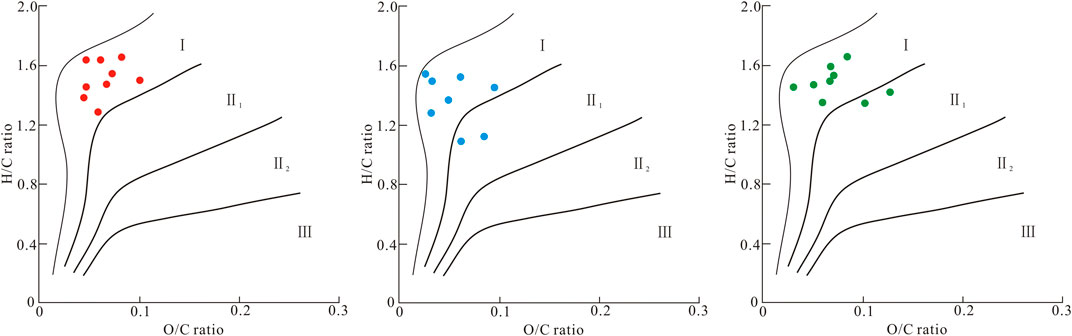
FIGURE 4. Types of organic matter in the shale of the Wufeng-Longmaxi Formation in southern Sichuan.
4.2.2 Organic Matter Content and Thermal Evolution Degree
The organic matter contents of the high-quality shales of the Wufeng-Longmaxi Formation in the Changning, Weiyuan and Luzhou areas of southern Sichuan vary in the horizontal and vertical directions. Specifically, similar organic matter contents can be observed at the bottom of the Wufeng and Longmaxi Formations, while the organic matter content in the upper part of the Longmaxi Formation in the Luzhou area is slightly smaller than that in the Changning and Weiyuan areas. The thermal evolution degree of organic matter is in the high-to postmature stage. The organic matter contents in the Changning area range from 2.54 to 5.34%, with an average of 3.6%. The equivalent vitrinite reflectance values range from 2.77 to 2.92%, with an average of 2.71%. In addition, the organic matter contents of the shale of the Wufeng-Longmaxi Formation in the Weiyuan area range from 2.94 to 5.12%, presenting an average of 3.62%, and the equivalent vitrinite reflectance values range from 2.46 to 2.63%, with an average of 2.57%. The organic matter contents of the shale in Luzhou range from 2.69 to 2.95%, with an average of 2.78%. Vertically, the organic matter content at the bottom of the Long 1 sub section submember of the Wufeng Formation is high, whereas it gradually decreases from upsection. The thermal evolution degrees of organic matter reach the high-to postmature stage (Table 3). In summary, the thermal evolution degree of the organic matter in the Wufeng Formation is slightly higher than that in the Longmaxi Formation. High organic matter abundance and a high degree of thermal evolution guarantee a sufficient supply of hydrocarbon sources.
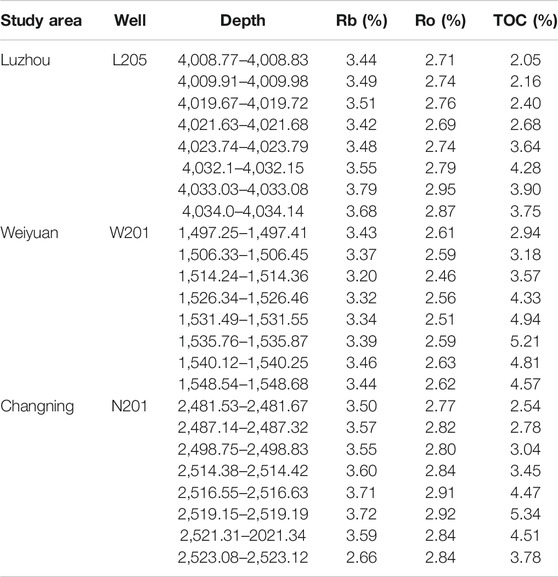
TABLE 3. Organic geochemical characteristics of shales in the Wufeng-Longmaxi Formation in southern Sichuan. All experiments were performed at room temperature (26.5 ± 1°C).
4.3 Whole-Rock Mineral Compositional Characteristics
The Wufeng-Longmaxi Formation shale is composed of various minerals, including quartz, feldspar, carbonate minerals, clay minerals and pyrite, according to whole-rock X-ray results. of these minerals, quartz and clay minerals are in the greatest amounts, and there is a small amount of feldspar (Table 4).
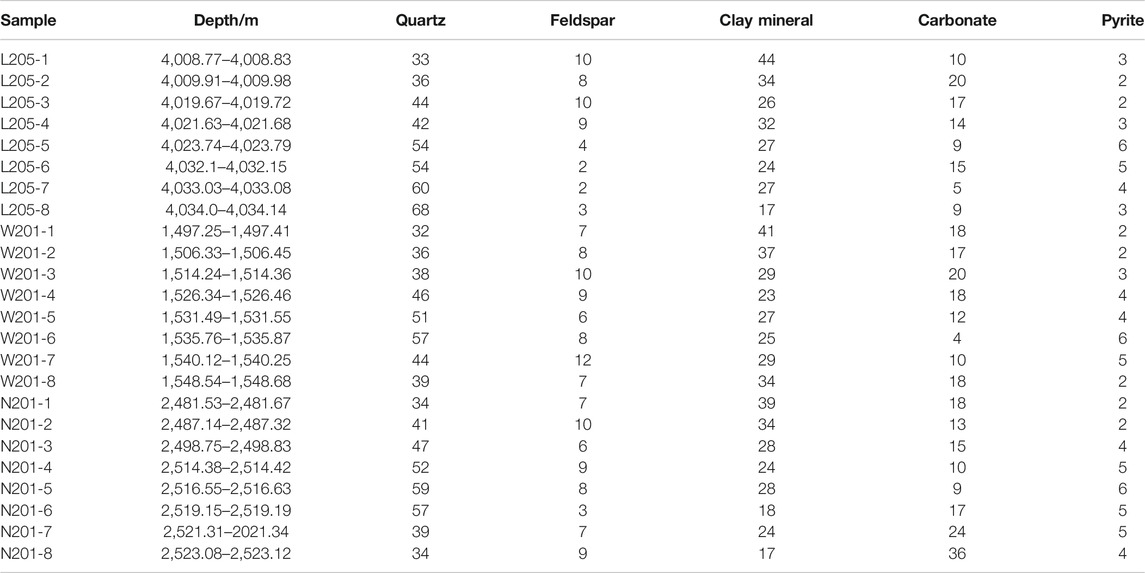
TABLE 4. Mineral composition of shales in the Wufeng-Longmaxi Formation in southern Sichuan. All experiments were performed at room temperature (26.5 ± 1°C).
The mineral compositions of different blocks are similar, but there are certain differences in both mineral contents and the vertical change in mineral contents, especially the vertical change in quartz minerals. Based on these findings, in the Luzhou area, the contents of quartz, feldspar, and clay minerals range from 33 to 68%, with an average of 48.87%; from 2 to 10%, with an average of 5.3%; and from 17 to 44%, with an average of 28.87%, respectively. Additionally, the carbonate mineral and pyrite contents range from 5 to 20%, with an average of 12.37%, and from 32 to 57%, respectively. Furthermore, in Well W201, the feldspar contents range from 6 to 12%, the clay mineral contents range from 23 to 41%, the carbonate rock contents range from 4 to 20%, and the pyrite contents range from 2 to 6%. Moreover, in Well N201, the quartz contents range from 34 to 59%, the feldspar contents range from 3 to 10%, the clay mineral contents range from 17 to 39%, the carbonate rock contents range from 9 to 36%, and the pyrite contents range from 2 to 6%.
4.4 Characteristics of Pore Development in Shale
In general, generated shale gas exists in a free state in pores without a large transport distance and in an adsorbed state on the surfaces of mineral particles and inner walls of microscopic pores. Based on the differences in pore development, shale pores can be divided into organic and inorganic pores. In addition, certain numbers of hydrocarbon-generating shrinkage joints and fractures at the clay edges also develop as pores are developed. Differences in the spatial compositions of shale pores exert a remarkable influence on the gas contents in shale.
4.4.1 Characteristics of Organic Pore and Fracture Development
Organic matter can provide hydrocarbon sources for generating hydrocarbons in pyrolysis, while pores inside organic matter are also vital spaces for gas storage. In addition, organic matter consists of primitive organic matter pores and pyrolysis hydrocarbon-generating pores. The type of hydrocarbon-generating material, burial depth, and thermal evolution degree are the main factors affecting the development and distribution of organic matter pores.
The organic matter in southern Sichuan is distributed among various minerals in spot, dot and irregular banded shapes, which are dark brown with defined boundaries with mineral particles (Figure 5A), according to the observation results of the argon ion-polished scanning electron microscope analysis. There are certain differences in the morphologies of organic pores in different regions. Scanning electron microscopy (SEM) results showed that the pore shapes in organic matter were round, oval and flat. The morphologies of organic pores in the Weiyuan and Changning areas are mainly circular and elliptical, and the overall shape is honeycomb (Figure 5B), which indicates that the organic pores were weakly deformed by compaction and structural extrusion. In the Luzhou area, in addition to the development of a large number of circular and elliptical pores (Figure 5B), in deeply buried wells, the organic matter pores are flat and irregular, which were caused by the extrusion of surrounding minerals. The pore size distribution of organic matter is typically between 2 and 800 nm, of which the pore sizes of honeycomb macropores are between 200 and 800 nm, and the pore sizes of round/oval organic mesopores are between 20 and 50 nm. Moreover, large numbers of edge fractures at the contacts between the organic matter edges and inorganic minerals can be observed under the microscope, which are connected to the pores and not only increase the porosity of the shale but also effectively improve the permeability of the reservoir.
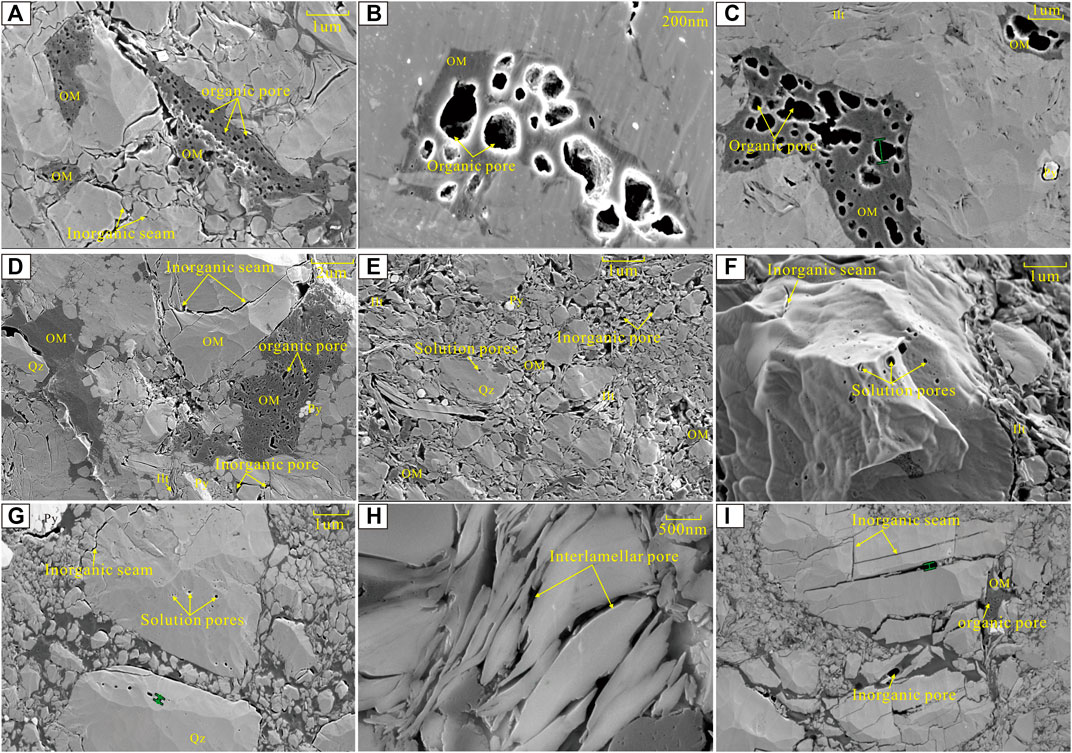
FIGURE 5. Types of microscopic pore spaces in the shales of the Wufeng-Longmaxi Formation in southern Sichuan. (A) Well L205; organic matter pores: 4028.52–4028.66 m. (B) Well L205; organic matter pores; 4033.82–4033.86 m. (C) Well L203; organic matter hole; 3802.15–3802.20 m. (D) Well L207; organic holes, inorganic fractures; 3548.22–3458.31 m. (E) Well L207; dissolved pores, intercrystalline pores; 3456.34–3456.37 m. (F) Well L207: dissolution hole; 3432.58–3432.62 m. (G) Well L207: dissolution hole; 3432.58–3432.62 m. (H) Well L207: Holes between clay slices; 3453.66–3453.69 m. (I) Well L203; mesh fracture; 3804.44–3804.52 m.
4.4.2 Development and Distribution of Inorganic Pores and Fractures
Diagenetic shale minerals are carriers for inorganic pores. Since the mineral compositions of shales in the Wufeng-Longmaxi Formation in the study area are complicated, the types and characteristics of pores developed in different minerals are also varied. Inorganic pores involve intercrystalline pores, transgranular pores, and dissolution holes. Quartz and feldspar featuring strong brittleness possess great compaction resistance in sedimentation and burial processes. Large numbers of intergranular pores with unregular shapes and sizes of 50 nm-1.5 µm form via the mutual support of mineral particles. In addition, as pyrite is characterized by a high elastic modulus, pyrite in shale mostly exists in the form of framboids with different crystal sizes, thereby forming a certain number of intercrystalline pores (Li et al., 2019a). The pores in a single pyrite framboid crystal have good connectivity and are often filled with organic matter. Multiple pyrite framboids are isolated and not connected to each other (Figure 5E). The organic acid formed during the hydrocarbon generation process of organic matter dissolves the crystals, causing the crystals to fall off to form mold pores. The pore sizes are between 200 and 500 nm. Owing to the selective corrosion of organic acids formed in later hydrocarbon generation, carbonate minerals and quartz minerals developed a certain amount of dissolution pores, the dissolution pores were isolated from each other, the shape of the pores was mainly circular, and the pore sizes were between 50 and 200 nm (Figure 5F). As clay minerals have weak compaction resistance, certain numbers of intergranular residual pores and interlaminar pores with pore sizes between 100 and 600 nm develop in the deep burial compaction process (Figure 5G). Moreover, clay minerals are mostly schistose, and the pores between the clay flakes are mostly crescent-shaped and flocculent, with a large number and good connectivity (Figure 5H). Additionally, a large number of granular edge fractures are developed at the contacts between organic matter and shale minerals (Figure 5I); these fractures are mesh-like and show good connectivity under the microscope (Fan et al., 2018; Li et al., 2019b; Xie et al., 2019; Shan et al., 2021; Wang et al., 2021; Wang and Wang, 2021; Zhu et al., 2022).
5 Discussion
5.1 Deepwater Anoxic Depositional Control “Source”
In the Late Ordovician-early Silurian, southern Sichuan underwent strong tectonic deformation due to the impact of the Caledonian tectonic movement. A sustained sedimentary depression area was formed due to the encompassment of the Motianling Ancient Land, the Jiangnan-Xuefeng Uplift, the Chuanzhong paleouplift, and the Central Guizhou paleouplift. Ice covers largely melted by the end of the ice age. Large-scale transgressions resulting from sea level rise increased the water body in the study area, which was a deep-water shelf environment that was low-energy, undercompensated, and hypoxic. Southern Sichuan inherited the Ordovician geographic pattern at the beginning of the Silurian, and continuous transgression resulted in the deepening of the water body (Xu et al., 2020; Zhang et al., 2020). Furthermore, affected by the sealing effect of ancient pericline land, deep-water shell deposits expanded, causing the Longmaxi Formation to be in a euxinic-semi-euxinic sea basin sedimentary environment during its early depositional period (Figure 6).
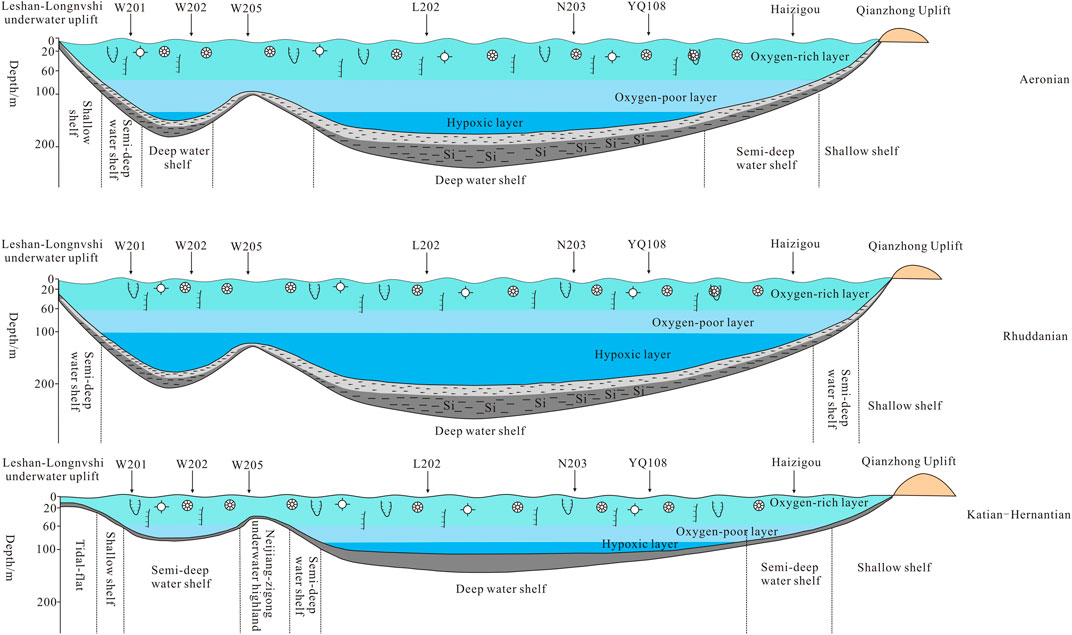
FIGURE 6. Evolutionary model of the sedimentary environment in the depositional stage of the lower Silurian Longmaxi Formation in the southern Sichuan Basin (modified according to Pu et al., 2020).
In the Hirnantian-Rhuddanian ages, a warm climate after the ice age facilitated lower plankton to flourish. Organic detritus formed from the deaths of organisms was deposited in the form of “marine snow” in a deep-water anoxic environment. In particular, low-grade organisms with siliceous shells such as sponge spicules, radiolarians and graptolites effectively improved the brittleness of shale and provided much organic matter, which also accumulated in the quiet anoxic water body, forming a set of organic-rich siliceous shales.
After entering the Aeronian age, regressions occurred as the sedimentation center migrated under the effects of global sea-level decline and regional tectonic uplift. Differential uplifts occurred in southern Sichuan due to tectonic movement. Significant differences in regressive rates occurred. Moreover, differential uplift of underwater paleouplifts in central Sichuan and central Guizhou was caused by sustained compression.
As the sea area confined by the paleouplift shrank, the depth of the water body became shallower, and the sedimentation center shifted northward to the Longchang-Yongchuan area. The maximum thickness of the Wufeng-Longmaxi Formation shale is more than 400 m because the Changning area developed shallow-water shelf facies and semideep-water shelf facies by receiving sedimentation from the Central Guizhou PaleoUplift. However, the Weiyuan area and the Longchang-Yongchuan area are in deep-water environments. The tectonic movement was ongoing in the Telychian period. At the same time, the Chuanzhong paleouplift was further uplifted. Consequently, sedimentation ended due to the exposed surface of the Changning area in southern Sichuan. In addition, the Weiyuan and northern Luzhou areas developed silty shales by receiving sediment from the Chuanzhong paleouplift.
Moreover, the reducing deep-water shelf water body that was euxinic in the early stage accelerated the sedimentation, burial and preservation of bio-organic detritus to form high-quality shale. In the later stage, apparent changes in the shale thickness in the Wufeng-Longmaxi Formation shale in different areas occurred due to the differential uplift and the migration of the sedimentation center. In summary, the thickness of the high-quality shale is distributed in an irregularly circular form along Chongqing-Yongchuan-Luzhou-Yibin (Figure 7), and the thickness of the high-quality shale in the Rongchang-Luxian areas is normally more than 60 m. Specifically, the Luzhou area was a center of sedimentation during the migration of several sedimentation centers. The maximum shale thickness in the Wufeng-Longmaxi Formation is approximately 75 m, which is far greater than that in the Weiyuan and Changning areas.
5.2 Coupled Control of “Source and Storage” Accumulation
In general, gas is contained in mature shale. However, commercial shale gas reservoirs are formed by the effective allocation of multiple spatiotemporal factors instead of a single factor (Pan et al., 2016; Wang et al., 2018). Shale is a special rock type that integrates the source, storage and coverage. Hence, a variety of factors affecting shale gas accumulation occur, of which hydrocarbon source and storage conditions are two important factors. Specifically, hydrocarbon source conditions consist of organic matter type, TOC content, thermal evolution degree of organic matter, and the thickness of high-quality shale, while storage conditions consist of pore type, porosity, permeability and mineral composition. TOC is closely related to the brittle mineral content, thermal evolution degree, and development of organic porosity in shale. Although hydrocarbon sources and storage conditions play different roles in the formation of shale gas reservoirs, they are correlated and interdependent. The spatiotemporal coupling of hydrocarbon sources and storage conditions is essential for the formation of shale gas reservoirs.
An abundant supply of hydrocarbon sources is the foundation for the formation of shale gas reservoirs. Factors that determine the hydrocarbon generation conditions incorporate the type of hydrocarbon generation parent material, TOC content, thickness of high-quality shale, and thermal evolution degree of organic matter. The type of organic kerogen directly leads to differences in the hydrocarbon generation potential. Type I kerogen is primarily dominated by the involvement of a few type II kerogens in the Wufeng-Longmaxi Formation shale in the Changning, Luzhou and Weiyuan areas in southern Sichuan, which indicates that organic matter mainly originated from plankton and algae sediments, presenting a great hydrocarbon generation potential. The TOC content and thickness of high-quality shale codetermine the total amount of hydrocarbon-generating parent material, constituting the material foundation for the formation of shale gas reservoirs. Based on the vertical variation pattern of TOC in the Wufeng-Longmaxi Formation shale in southern Sichuan, the thickness of the high-quality shale at the bottom of the Wufeng and Longmaxi Formations is normally greater than 30 m, with TOC contents reaching more than 2.0%. To be precise, the organic matter content of the high-quality shale in the Wufeng-Longmaxi Formation and at the bottom range from 0.28 to 7.5% and from 1.9 to 7.5%, respectively, presenting an average of 3.97% in the Changning area. The organic matter contents of the high-quality shale in the Wufeng-Longmaxi Formation range from 0.42 to 5.98% and from 2.89 to 5.98%, respectively, with an average of 3.88% in the Weiyuan area. An overall downward trend in the vertical TOC content occurs in the Weiyuan and Changning areas. Additionally, a “decreasing-increasing-decreasing” pattern is observed vertically in the Luzhou area, as shown in Figure 8. TOC content peaked in the 1 small layer of the Long 1 sub section submember and the 3 small layer of the Long 1 sub section submember, respectively. The Wufeng-Longmaxi Formation in southern Sichuan has the foundation for the formation of commercial gas reservoirs, according to analyses of the minimum high-quality shale thickness and TOC content in developing commercial shale gas reservoirs at home and abroad.
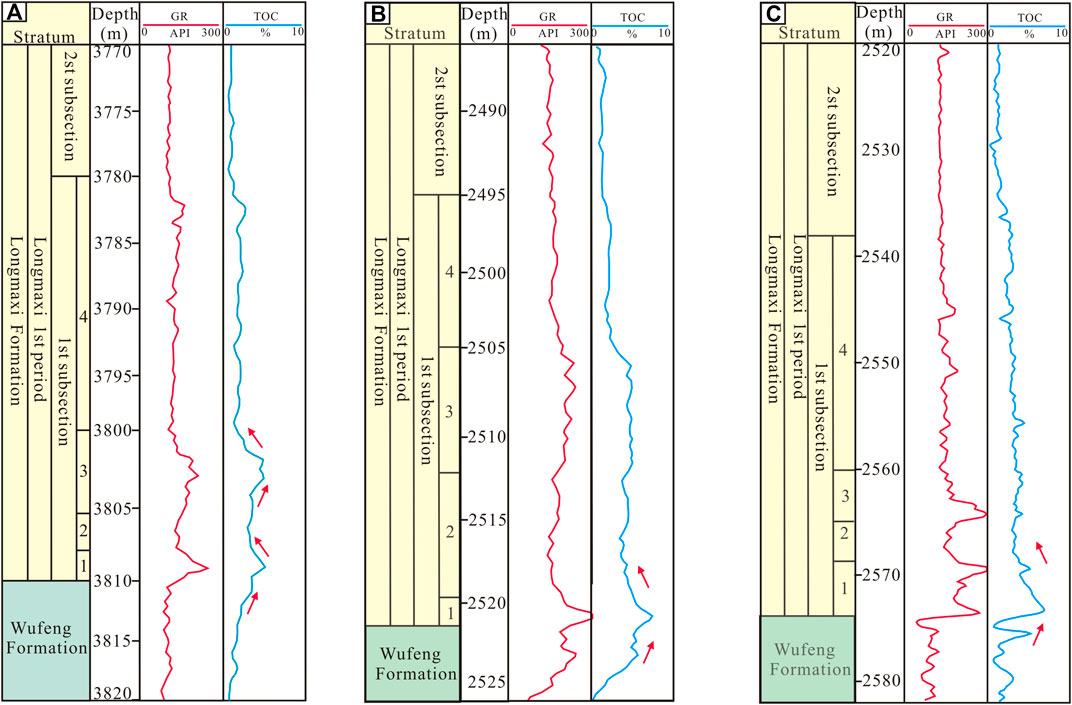
FIGURE 8. Longitudinal variation in TOC contents of shales in the Wufeng-Longmaxi Formation in southern Sichuan. (A) Well L207, 3,770 m–3820 m; (B) Well N201, 2,486 m–2525 m; (C) Well W202, 2,520 m–2582 m.
A certain thermal evolution is the “key” to starting the conversion of organic matter to hydrocarbons, whereas excessive thermal evolution might lead to a decline in the potential for hydrocarbon generation. Hence, the thermal evolution degree is one of the main factors restricting shale gas accumulation. In general, the thermal evolution degree of organic matter is quantitatively characterized by the vitrinite reflectance (Ro). In this regard, a moderate thermal evolution degree stimulates the enrichment of shale gas. The thermal evolution degree of organic matter in southern Sichuan is typically greater than 2.0, with a maximum of 2.95, based on the results of the organic matter equivalent vitrinite reflectance test conducted on shale samples in the Changning, Luzhou and Weiyuan areas. This, together with the differences in the thermal evolution degrees of hydrocarbon-generating products of organic matter, proves that the organic matter in southern Sichuan is in the high-to postmature stage of producing dry gas via pyrolysis. In this way, the conversion of organic matter to hydrocarbon generation can be guaranteed. Moreover, the supply of hydrocarbon sources for shale gas reservoirs is jointly guaranteed by a high organic abundance, moderate thermal evolution, and highly effective thickness.
Generated shale gas is adsorbed on the surfaces of organic matter and mineral particles and stored in microscopic pore spaces and fractures in a free state. The more developed the shale pores and microfractures are, the more space that is available for gas accumulation. Hence, pores and fractures in shales have direct impacts on the free gas content in shale. In general, the degree of shale pore development is quantitatively characterized by porosity. Therefore, porosity is one of the important evaluation indicators for reservoirs and geological conditions of accumulation. As can be observed from the porosity analysis of shale samples from different wells in the Changning, Weiyuan and Luzhou areas, the porosities of shale in the Wufeng-Longmaxi Formation and of high-quality shale in the Wufeng Formation in the 1 small layer of the Long 1 sub section submember range from 0.47 to 9.83% and from 3.3 to 9.83%, respectively, with an average of 4.86% in the Luzhou area. The porosities of shale and high-quality shale at the bottom range from 0.53 to 6.63% and from 2.98 to 6.63%, respectively, with an average of 3.74% in the Changning area. The porosities of shale and of high-quality shale at the bottom range from 0.68 to 7.9% and from 3.9 to 7.9%, respectively, with an average of 4.25% in the Weiyuan area. Overall, favorable shale porosity is witnessed in the Wufeng-Longmaxi Formation. Pore spaces in shales in various wells are composed of inorganic pores, inorganic fractures, organic pores and organic fractures. Among them, the ratios of organic pores and fractures in the Luzhou area range from 32 to 60%, and inorganic pores and fractures range from 34 to 69%. The proportion of organic pores and fractures is equivalent to that of inorganic pores and fractures overall. The proportions of organic pores and fractures in the shale in the Changning area range from 35 to 71%, and those of inorganic pores and fractures range from 29 to 66%. The contribution rate of organic pores and fractures in the Weiyuan area is lower than that of inorganic pores and fractures, whereas the contribution rate of organic pores and fractures in the Changning area is higher than that of inorganic pores and fractures (Figure 9).
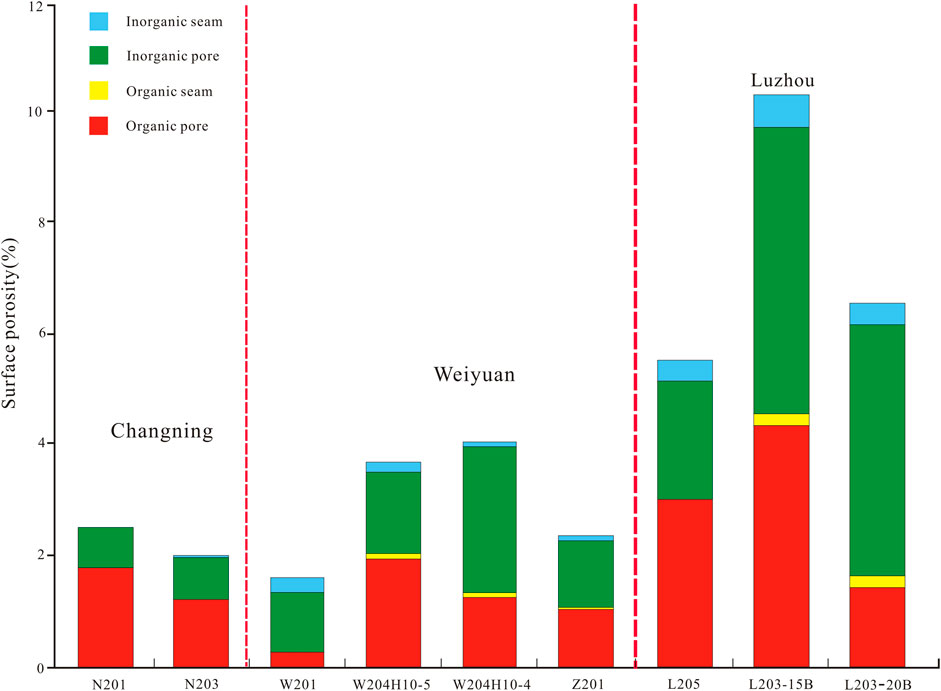
FIGURE 9. Composition of shale pore space in the Wufeng Longmaxi Formation in the Changning, Weiyuan and Luzhou areas in southern Sichuan.
Organic matter and diagenetic minerals are susceptible to pore development. Although organic pores and inorganic pores develop differently, they are closely related in the relationship between the abundance of organic matter and the content of brittle minerals, as well as the thermal evolution process of organic matter and the formation of inorganic pores. Moreover, the development of inorganic pores is inherently connected to organic matter based on the material source and pore composition of marine facies organic-rich shale. The biogenic silica in siliceous minerals is rooted in the siliceous shells of lower microorganisms. In addition, the biogenic silica content is controlled by the TOC content. The anoxic reducing environment provides a foundation for the formation of pyrite on the premise of ensuring the preservation of organic matter. In addition, soluble minerals such as quartz are selectively dissolved by organic acids that are formed via pyrolysis and hydrocarbon generation of organic matter, thereby forming dissolution pores. Apparently, the TOC content is correlated to the brittle mineral content. Since large numbers of low-grade algae and plankton developed in the water body in southern Sichuan during the shale sedimentation period of the Longmaxi Formation, dead plankton with siliceous shells were sedimented, resulting in an increase in brittle minerals, and the pyrite content shows that the TOC content is positively correlated with the shale pore size, content of brittle minerals, and pyrite content. Specifically, the TOC content is highly correlated with the content of brittle minerals in the shale (Figure 10), indicating that the development of organic matter is inherently connected to the development of shale brittleness and porosity.
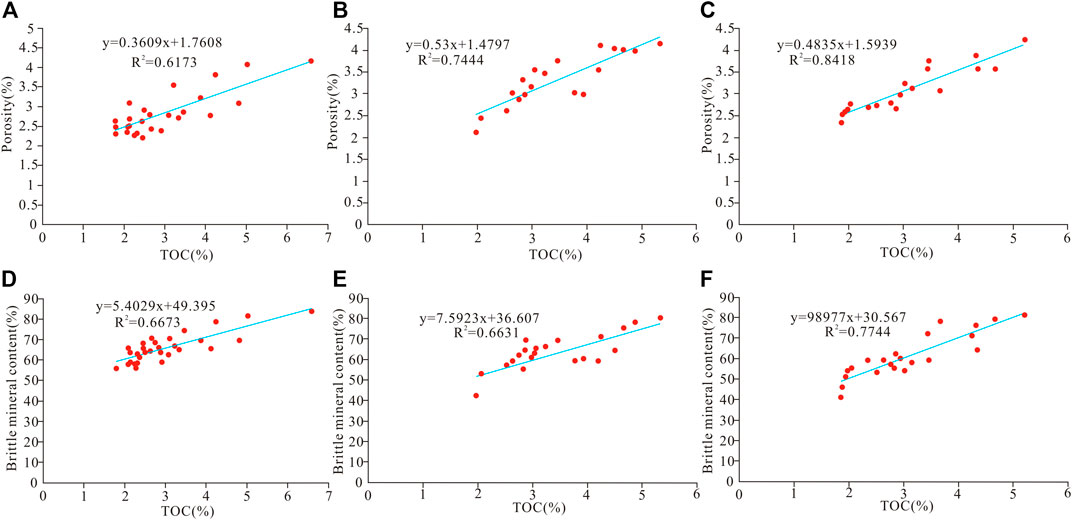
FIGURE 10. Relationship among shale organic matter content, shale porosity, and brittleness in southern Sichuan. (A–C) represent the relationship curve between TOC content and porosity repectively in Luzhou area, Changning area and Weiyuan area. (D–F) correspond to the relationship curve between TOC content and brittle mineral content respectively in Luzhou area, Changning area and Weiyuan area.
In summary, the high-quality hydrocarbon source conditions are constituted by the organic matter type, the thickness of high-quality shale and the TOC content of the shale in the Wufeng-Longmaxi Formation in southern Sichuan. Moreover, the occurrence of shale gas is safeguarded by the content of brittle minerals, thermal evolution degree of organic matter, and shale porosity. Additionally, the brittleness and pore development of the shale are accelerated owing to the enrichment and thermal evolution of organic matter. On this basis, a coupled spatiotemporal accumulation system is constituted by the TOC content, thermal evolution degree, and the intrinsic connection of the development of pores and brittle minerals, contributing to the formation of shale gas reservoirs.
5.3 Three-Dimensional Differential Enrichment of Effective Sealing
The separation of favorable preservation areas results in the shale gas reservoirs of marine sedimentary formations in southern China being characterized by discontinuous distributions. Differences in tectonic deformation immediately affect the preservation conditions. Moreover, lamellar development, the vertical sealing of faults, tectonic uplift time, tectonic strength, and formation pressure coefficients are essential for the preservation of shale gas. They are different but correlate in affecting the preservation of shale gas.
5.3.1 Horizontal Lamellar Sealing
Many lamellar fractures and slip-bedding fractures that are typically developed in organic-rich shale are the main channels for the lateral migration of shale gas. As can be observed from early exploration, the lateral permeability of organic-rich shale in the Wufeng-Longmaxi Formation in the Sichuan Basin is much greater than the vertical permeability under the impact of lamellar fractures.
With the strong stress sensitivity of shale permeability, the horizontal escape strength of shale gas is dependent on the magnitude of the positive pressure suffered by shale fractures. However, no unified quantitative standard is available for evaluating the magnitude of the positive pressure of shales and the closure of lamellation. Studying the influence of pressure on shale porosity and permeability using the overburden pressure test reveals that the shale permeability is almost unchanged as the pressure increases in a low pressure environment (< 3.5 MPa); the permeability of shale decreases abruptly as the pressure increases when the pressure ranges from 3.5 to 15 MPa; and the permeability decreases slowly as the pressure increases in the presence of slow decline. The declining range of permeability decreases under sustained pressurization when the pressure ranges from 15 to 50 MPa. The sealing property is judged in accordance with the magnitude of the shale sealing pressure and the relative magnitude of the shale surface pressure. In general, the compressive strength of shale ranges from 19.61 to 68.65 MPa as per the difference in mineral composition. When the vertical pressure on the lamellation surface is greater than the compressive strength, the lamellation is sealed. In addition, the magnitude of the sealing pressure varies slightly under the influence of tectonic differences in various areas. Normally, the high formation pressure tightly seals the lamellation, which is more effective in inhibiting the migration of shale gas. The difference in the sealing properties of lateral shales is analyzed in combination with the production capacity of typical wells in the Weiyuan, Changning and Luzhou areas and the measured vertical pressure of the formation. The shale of the Wufeng-Longmaxi Formation in Well W201 in the Weiyuan area is buried at a depth of approximately 1,500 m, and its vertical formation stress of 30 MPa is smaller than the shale sealing pressure with a pressure coefficient of 0.92. Hence, the production capacity of a single well is merely 0.26 × 104 m3/d. In addition, the shale of the Wufeng-Longmaxi Formation in Well W204 is buried at a depth of approximately 3,600 m, with a vertical formation pressure of 82.32 MPa and a formation pressure coefficient of 1.96, and the production capacity of a single well is 16.5 × 104 m3/d. The shale of the Wufeng-Longmaxi Formation in Well N203 in the Changning area is buried at a depth of approximately 2,100 m, with a vertical formation pressure of 61.4 MPa, which is slightly lower than the lamellar sealing pressure and a formation pressure coefficient of 1.96, and the production capacity of a single well is 5.10 × 104 m3/d. The burial depth of Well N213 is 2,300 m, with a vertical formation pressure of 67.4 MPa, a formation pressure coefficient of 1.43, and a production capacity of a single well of 21.94 × 104 m3/d. The shale of Well L203 in the Wufeng-Longmaxi Formation in the Luzhou area is buried at approximately 3,800 m with a vertical formation stress of 101.3 MPa and a tested production capacity of 137.9 × 104 m3/d. In addition, the vertical pressure and the test production capacity of Well L207 are 89.8 MPa and 30.63 × 104 m3/d, respectively. The vertical pressure and the test production capacity of Well L204 are 99.9 MPa, and the test productivity is 14.4 × 104 m3/d. The differences in single-well production capacity under various burial depths are compared in the Changning and Weiyuan areas. According to the results, when the vertical formation pressure approaches or exceeds the compressive strength of the rock, a high single-well production capacity of shale gas can be obtained, indicating that a high sealing degree of lateral shale contributes to the preservation of shale gas.
5.3.2 Longitudinal Fault Sealing
The stable floor of the high-quality shale bed of the Wufeng-Longmaxi Formation in southern Sichuan, the hydrocarbon sealing effect in the upper segment of the Longmaxi Formation, and the association of the longitudinal escape of shale gas with differential fracture sealing are the major causes of preservation differences of complicated tectonic areas. The fracture sealing capacity is principally related to the fault occurrence, the filling process characteristics, and the matching relationship between the magnitude and direction of the ground stress and fracture strike (Wang, 2020; Wang X et al., 2020; Yu et al., 2021, 2022; Zhu et al., 2022). Specifically, the small inclination suffers from greater stress superimposed by the static pressure of the superincumbent bed, and the horizontal crushing stress makes it easier for the fracture to be sealed more easily. Under current conditions, previous studies have shown that the best sealing can be achieved when the included angle of the fracture strike and the ground stress is greater than 67.5°. More specifically, a favorable sealing property can be achieved when the angle is between 45° and 67.5°, while a poor sealing property occurs when the angle is between 22.5° and 45.0°. The fracture has nearly no sealing property when the angle is less than 22.5°. Hence, vertical fault sealing properties in the Changning, Weiyuan and Luzhou areas in southern Sichuan are analyzed based on the above conditions. Additionally, the preservation of shale gas is discussed (Figure 11).
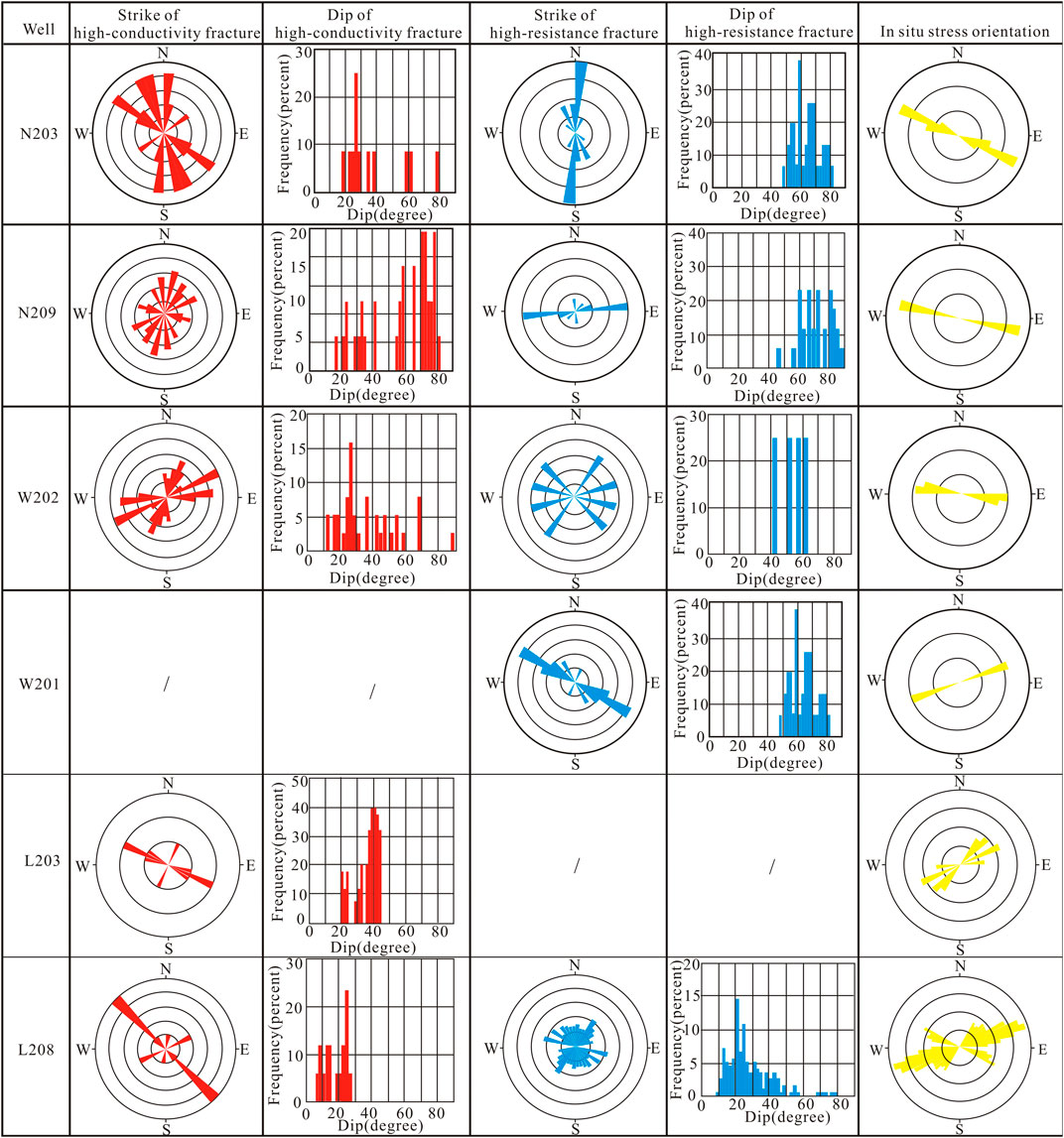
FIGURE 11. Relationship among the orientation of the high-resistance shale gas fractures, the high-resistance fractures and the ground stress.
Image log interpretation results show that the fracture strikes in the Well N203 in the Changning area are NS, NNW and NW. The inclinations of high-resistance fractures range from 20° to 35°. The strike of high-resistance fractures is NS, with inclinations ranging from 50° to 75°. In addition, the ground stress is oriented WNW, which intersects with fractures oriented NNW and NS at high angles and obliquely intersects in the NW at a small angle. The sealing is medium, and the gas content of high-quality shale ranges from 2.9 to 5.0 m3/t, with an average of 3.8 m3/t. NNE, NS, NW and minor NNW are the preferred strike directions of the high-conductivity fractures in Well N209. The inclinations of the fractures mainly range from 60° to 85°. The strike of the high-resistance fracture is ENE. The ground stress is close to WE, which intersects with the high-conductivity fracture at a vertical or large angle and intersects with the high-resistance fracture at a small angle, indicating that an excellent sealing property occurs with the fracture. Additionally, the gas content of high-quality shale ranges from 3.88 to 4.29 m3/t, with an average of 4.08 m3/t. Hence, the high overall gas content shows the favorable sealing property of the shale. The fracture strikes of the shale gas reservoir bed in Well W201 in the Weiyuan area are mainly NW, and they are high-resistance fractures at angles of approximately 310°. The ground stress is approximately 275°, which intersects with the preferred orientation of high-resistance fractures at a small angle. Moreover, the formation pressure coefficient is merely 0.92, and the gas contents of the high-quality shale bed in the Wufeng-Longmaxi Formation range from 0.45 to 2.4 m3/t, with an average of 1.33 m3/t. A poor sealing property is presented. The orientation of the high-conductivity fracture in Well W202 are NNE and ENE. The inclinations of the fractures range from 20° to 40° and from 70° to 90°. There is no obvious preferred orientation for high-resistance fractures. The orientation of the ground stress approaches the WE direction, and the angle of the high-conducting fracture is more than 45°. The gas contents of the high-quality shale bed in the Wufeng-Longmaxi Formation range from 2.42 to 4.4 m3/t, with an average of 3.15 m3/t. The fracture is moderately closed. The preferred orientation of high-resistance fractures in Well L203 at Luzhou is NNW (295° ± 5°), and the inclinations of fractures are between 20° and 50°, which are dominantly low-angle fractures. In addition, the orientation of the ground stress is NE (45° ± 5°), and the angles of the fractures are between 70° and 80°. The gas contents of one segment of shale in the Wufeng-Longmaxi Formation are between 3.4 and 5.3 m3/t, with an average of 4.6 m3/t. The production capacity in a single-well test is 137.9 × 104 m3/d, demonstrating favorable fracture sealing. The preferred orientation of high-conductivity fractures in Well L208 of Luzhou is NW, and the high-resistance fractures have no obvious preferred orientation. In addition, the inclinations of the fractures are between 10° and 30°. The angles of the high-conductivity fractures are between 60° and 80°. The gas contents of high-quality shale in the Wufeng-Longmaxi Formation are between 3.7 and 6.1 m3/t, with an average of 4.2 m3/t. The production capacity in a single well is 137.9 × 104 m3/d, showing favorable fracture sealing.
5.3.3 Formation Pressure Coefficient
The primary state of shale might be destroyed by tectonic movement in a later stage. Fold deformation formed by tectonic movement, uplift and fracture development can lead to changes in the state and energy of the formation fluid, thereby adjusting and destroying the formation pressure system, which can be immediately embodied in the formation pressure coefficient. Undoubtedly, the formation pressure coefficient is one of the comprehensive indicators for evaluating the quality of shale gas preservation. Specifically, favorable shale gas preservation conditions can be found in areas with high formation pressure coefficients. Previous studies in the Dingshan, Jiaoshiba and Nanchuan areas show that the formation pressure coefficient is significantly and positively correlated with the production of shale gas.
Formation pressure coefficients of the Wufeng-Longmaxi Formation shale in various blocks in southern Sichuan and the corresponding gas production of shale gas wells are analyzed. Specifically, the formation pressure coefficients of the Weiyuan and Changning areas have large spans; that is, the formation pressure coefficients in most parts of the Changning area are more than 1.2, and only in the area close to the Changning upper bend area are they less than 1.0 (Figure 12). Wells N208, N212, and N210 are near the denudation zone, and the shallow formation burial and formation pressure coefficient are less than 1. Moreover, featuring high degrees of fracture development around the wells, the gas contents of the shale are 2.4 m3/t, 2.69 m3/t, and 3.78 m3/t, respectively. In addition, Wells N216, N217, N209, and N201 in the Changning areas are located in slope and syncline areas that are far from the denudation zone, which features deep burial formation and a low degree of fracture development, with formation pressure coefficients of 1.71, 1.94, 2.0, and 2.03, respectively. The gas contents of shales are 6.7 m3/t, 5.0 m3/t, 4.82 m3/t, and 5.3 m3/t, respectively. The Weiyuan area is near the Chuanzhong paleouplift, with rapid changes in the formation pressure coefficient. The formation pressure coefficient, single-well gas production, and shale gas content of Well W201 are 0.92, 0.26 × 104 m3/d, and 2.6 m3/t, respectively; the formation pressure coefficient, single-well gas production, and shale gas content of Well W202 are 1.4, 2.75 × 104 m3/d, and 4.0 m3/t, respectively; the formation pressure coefficient, single-well gas production, and shale gas content of Well W204 are 1.96, 16.5 × 104 m3/d, and 6.6 m3/t, respectively. Favorable preservation conditions of shale gas in areas with high formation pressure coefficients can be proven in conjunction with the corresponding relationship between the single-well energy difference, gas content and formation pressure coefficient in various blocks in southern Sichuan (Figure 13).
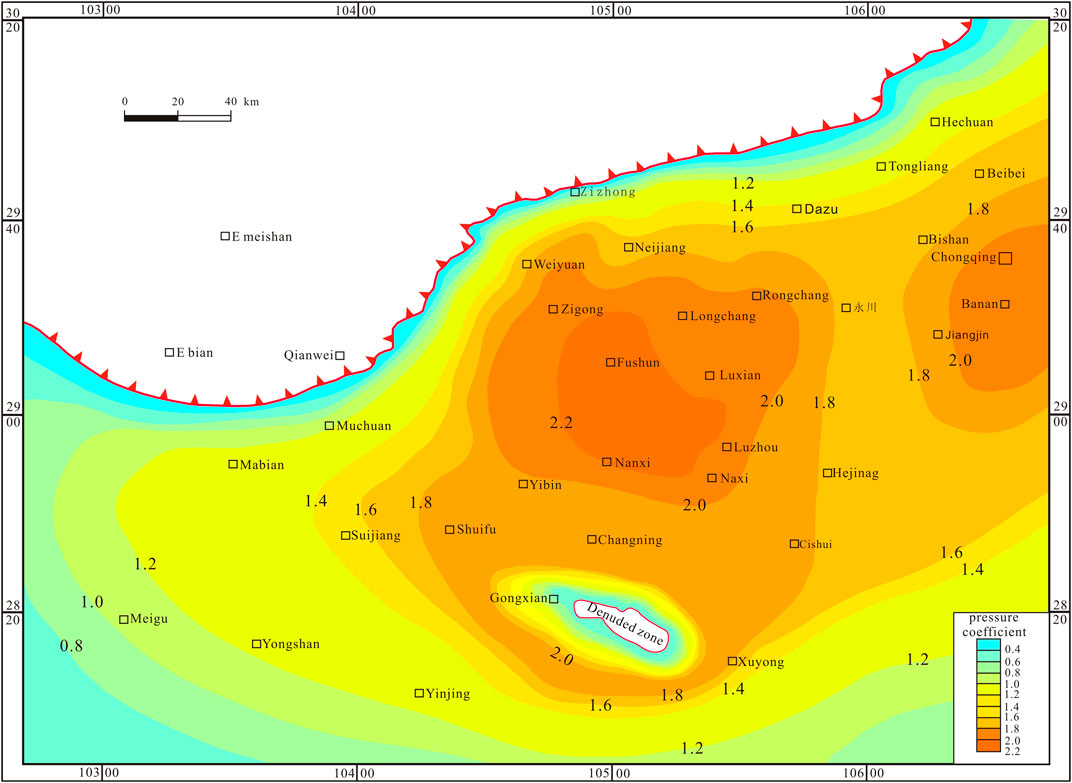
FIGURE 12. Distribution of formation pressure coefficients of the Wufeng-Longmaxi Formation in southern Sichuan.
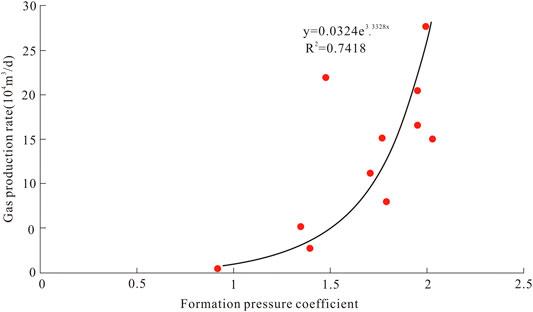
FIGURE 13. Relationship between the formation pressure coefficient and shale gas production in southern Sichuan.
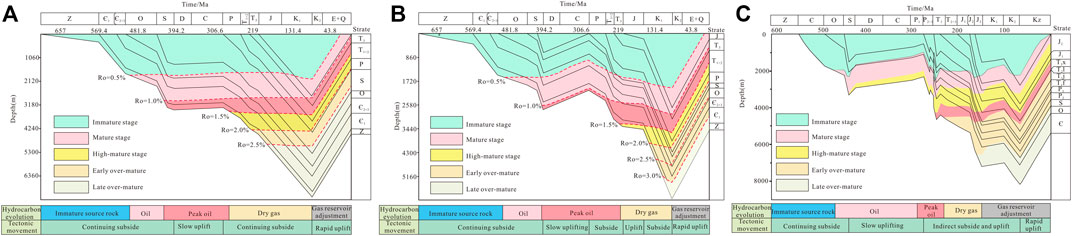
FIGURE 14. Aggregative model of the shale burial history, hydrocarbon generation and structural evolution of the Wufeng-Longmaxi Formation in the (A) Weiyuan, (B) Luzhou, and (C) Changning areas in southern Sichuan.
5.3.4 Differences in Uplift Time and Intensity
The formation of shale gas reservoirs experienced continuous deep burial in the early stage and then structural uplift and reformation in the later stage. Sustained burial in the early stage of burial contributed to thermogenic hydrocarbon generation of organic matter. With a small uplift range, shale gas was formed and well preserved with minor damage. When the Wufeng-Longmaxi Formation was tectonically uplifted after the greatest burial depth, tectonic uplift adjusted the shale gas reservoir and controlled the escape and preservation of shale gas. The earlier the uplift time is, the longer the lost time of shale gas, and the more unfavorable the preservation of shale gas.
By analyzing the times and amplitudes of tectonic uplift through the burial thermal history, tectonic differences are found in the Changning, Weiyuan and Luzhou areas in southern Sichuan (Figure 14). The formation uplift history varied significantly from the southern edge of the basin to the center of the basin. Moreover, the stratigraphic uplift time gradually increased. Specifically, Wells N201, N216, and N215 in the Changning areas began to uplift in the middle of the Late Cretaceous, with uplift times ranging from 80 to 134 Ma. In addition, the tectonic uplift time of Wells W201, W202, and W203 in the Weiyuan area was approximately 80 Ma, while the uplift times of Well HS1 and Well Y101 in the shale of the Wufeng-Longmaxi Formation ranged from 65 Ma to 74 Ma.
The maximum uplift of Well W201 in the Longmaxi Formation is 4,900 m, with a daily gas production of 0.26 × 104 m3/d and a shale gas content of 2.60 m3/t, of which adsorbed gas and free gas are 0.78 m3/t and 1.82 m3/t, respectively. The maximum uplift of Well W202 is 3,926 m, with a daily gas production of 2.75 × 104 m3/d and a shale gas content of 4 m3/t, of which adsorbed gas and free gas are 1.20 m3/t and 2.80 m3/t, respectively. The maximum uplift of W204 in the formation is 2,950 m, with a daily gas production of 16.50 × 104 m3 and a shale gas content of 6.6 m3/t, of which adsorbed gas and free gas are 1.96 m3/t and 4.44 m3/t, respectively. Evidently, the greater the magnitude of the formation uplift is, the lower the shale gas content. The uplift timing of the shale in the Luzhou area is late, with deeply buried formations and a small formation uplift magnitude. For instance, the uplift timing, formation uplift magnitude, and daily gas production of Well Y101 are 55 Ma, 2,923 m, and 43 × 104 m3, respectively. In addition, the Changning area across, inside and outside the basin have denuded areas. By comparison, the uplift timing inside the basin is earlier than that outside the basin, with obvious differences in the uplift magnitude. In particular, the uplift timing has a remarkable impact on the shale gas content. Specifically, the uplift timing, formation uplift amplitude, daily gas production, and shale gas content of Well N201 are 65 Ma, 2,710 m, 14 × 104 m3/d, and 5.3 m3/t, respectively. In other words, the closer to the denudation zone, the greater the formation uplift magnitude is, and the worse the shale gas content is. Specifically, Well N208 is 2.8 km away from the denudation area, and its tested production capacity has a small amount of gas content; Well N201 is 3.5 km away from the denudation area, and its tested production capacity is 0.99 × 104 m3/d; Well N203 is 9.5 km away from the denudation area, and its tested production capacity is 1.29 × 104 m3/d; and Well N209 is 18.3 km away from the denudation area, and its tested production capacity is 18.54 × 104 m3/d.
6 Conclusion
The U/Th and Mo/U ratios of the high-quality shale at the bottom of the Wufeng-Longmaxi Formation range from 0.75 to 4.5 and from 0.3×SW to 1×SW, respectively. The covariant relationship of U/Th and Mo/U reveals a strongly euxinic reducing water body. In addition, as the U/Th ratio of the upper part of the Longmaxi Formation is less than 0.75, the covariant relationship of U and Mo indicates an oxygen-rich water body featuring a higher oxygen content and a lower degree of euxinia. Type I organic matter is dominated by a small amount of type II1 organic matter. An organic matter content greater than 2.5% and a thermal evolution degree greater than 2.5 are observed in the high-quality shale bed. In other words, the shale bed is in the stage of high-to postmature dry production, presenting great potential for hydrocarbon generation. The surface porosity of the shale ranges from 2 to 10%, while the surface porosity of the high-quality shale bed is greater than 5%. That is, the development of microfractures contributes to effectively improving the permeability of the reservoir.
The thickness of the high-quality shale in southern Sichuan is distributed in a crescent shape along the Yibin-Luzhou area. Among them, the maximum thickness of high-quality shale in the Luzhou area reaches as high as 70 m, and the thicknesses of the Weiyuan and Changning areas are greater than 30 m. A high TOC content and high thermal evolution degree are fundamental for the hydrocarbon generation from organic matter. A high porosity provides space for shale gas accumulation. Low-grade siliceous biological accumulation provides abundant organic matter while greatly increasing the brittleness of shale. A high Ro value promotes the conversion of hydrocarbons, not only affecting the development of hydrocarbon-generating pores and dissolution pores but also increasing the porosity of shale. Moreover, “hydrocarbon source” and “storage” conditions are mutually affected and promoted. Based on this, a coupled “source-storage” accumulation system controls the formation of shale gas reservoirs.
Effective preservation is critical for shale gas accumulation. Fracture development is crucial for the preservation of shale gas. Three-dimensional effective sealing conditions include a late tectonic uplift timing in the basin, a small uplift range, a short time of shale gas loss, low strength, the inhibition of the vertical formation pressure on the lateral migration of shale gas along the lamellation, the fracture sealing property effectively enhanced by the large-angle intersection of high-filling fractures with the current ground stress, and the inhibition of the vertical escape of shale gas. Hence, a stable burial area featuring a late tectonic uplift time, small amplitude, large-scale underdeveloped faults, and high formation pressure is a favorable area for shale gas exploration.
Data Availability Statement
The original contributions presented in the study are included in the article/Supplementary Material, further inquiries can be directed to the corresponding author.
Author Contributions
SH contributed in writing, reviewing, and editing, data curation, writing-original draft preparation; QQ and HL contributed in formal analysis, validation, and methodology, supervision, project administration, and funding acquisition; SZ contributed to visualization and investigation.
Funding
This research was supported by Open Fund (G5800-20-ZS-KFZY002) of State Key Laboratory of Oil and Gas Enrichment Mechanisms and Effective Development and the National Science and Technology Major Project (2017ZX05036003-003) of the 13th Five-Year Plan Period.
Conflict of Interest
SZ is employed by Shale Gas Research Institute, PetroChina Southwest Oil and Gas Field Company.
The remaining authors declare that the research was conducted in the absence of any commercial or financial relationships that could be construed as a potential conflict of interest.
The handling editor declared a shared affiliation with one of the authors SH, QQ, and HL at time of review.
Publisher’s Note
All claims expressed in this article are solely those of the authors and do not necessarily represent those of their affiliated organizations, or those of the publisher, the editors, and the reviewers. Any product that may be evaluated in this article, or claim that may be made by its manufacturer, is not guaranteed or endorsed by the publisher.
References
Borjigin, T., Shen, B., Yu, L., Yang, Y., Zhang, W., Tao, C., et al. (2017). Mechanisms of Shale Gas Generation and Accumulation in the Ordovician Wufeng-Longmaxi Formation, Sichuan Basin, SW China. Pet. Exploration Develop. 44 (1), 69–78. doi:10.1016/S1876-3804(17)30009-5
Che, S. Q. (2018). Shale Lithofacies Identification and Classification by Using Logging Data:a Case of Wufeng-Longmaxi Formation in Fuling Gas Field, Sichuan Basin. Lithologic Reservoirs 30 (01), 121–132. (in Chinese). doi:10.3969/j.issn.1673-8926.2018.01.012
Chen, C., Wang, J., Algeo, T. J., Wang, Z., Tu, S., Wang, G., et al. (2017). Negative δ13Ccarb Shifts in Upper Ordovician (Hirnantian) Guanyinqiao Bed of South China Linked to Diagenetic Carbon Fluxes. Palaeogeogr. Palaeoclimatol. Palaeoecol. 487 (3), 430–446. doi:10.1016/j.palaeo.2017.09.030
Chen, X., Rong, J., Fan, J., Zhan, R., Mitchell, C. E., Harper, D. A. T., et al. (2006). The Global Boundary Stratotype Section and Point (GSSP) for the Base of the Hirnantian Stage (The Uppermost of the Ordovician System). Episodes 29 (3), 183–196. doi:10.18814/epiiugs/2006/v29i3/004
Christophe, M. G., Janie, S., and Steve, S. (2013). Unconventional Gas-A Review of Regional and Global Resource Estimates. Energy 55 (15), 571–584. doi:10.1016/j.energy.2013.01.048
Curtis, M. E., Cardott, B. J., Sondergeld, C. H., and Rai, C. S. (2012). Development of Organic Porosity in the Woodford Shale with Increasing thermal Maturity. Int. J. Coal Geology. 103, 26–31. doi:10.1016/j.coal.2012.08.004
Fan, C. H., He, S., Zhang, Y., Qin, Q. R., and Zhong, C. (2018). Development Phases and Mechanisms of Tectonic Fractures in the Longmaxi Formation Shale of the Dingshan Area in Southeast Sichuan Basin, China. Acta Geol. Sin. Engl. 92 (6), 2351–2366. doi:10.1111/1755-6724.13732
Fan, C., Li, H., Qin, Q., He, S., and Zhong, C. (2020a). Geological Conditions and Exploration Potential of Shale Gas Reservoir in Wufeng and Longmaxi Formation of southeastern Sichuan Basin, China. J. Pet. Sci. Eng. 191, 107138. doi:10.1016/j.petrol.2020.107138
Fan, C., Li, H., Zhao, S., Qin, Q., Fan, Y., Wu, J., et al. (2020b). Formation Stages and Evolution Patterns of Structural Fractures in Marine Shale: Case Study of the Lower Silurian Longmaxi Formation in the Changning Area of the Southern Sichuan Basin, China. Energy Fuels 34 (8), 9524–9539. doi:10.1021/acs.energyfuels.0c01748
Gu, Y., Wan, Q., Yu, W., Li, X., and Yu, Z. (2018). The Effects of clay Minerals and Organic Matter on Nanoscale Pores in Lower Paleozoic Shale Gas Reservoirs, Guizhou, China. Acta Geochim 37, 791–804. doi:10.1007/s11631-018-0295-2
Guo, J. L., Jia, C. Y., He, D. B., Li, L., Zhu, H. Q., Zhou, Y., et al. (2020). Classified Evaluation of Shale Reservoirs in the Ordovician Wufeng to Silurian Longmaxi Formations in the Southern Sichuan Basin. Geol. J. China Universities 26 (03), 323–332. (in Chinese). doi:10.16108/j.issn1006-7493.2019047
Guo, X. S., Li, Y. P., Liu, R. B., and Wang, Q. B. (2014). Characteristics and Controlling Factors of Micropore Structures of the Longmaxi Shale in the Jiaoshiba Area, Sichuan Basin. Nat. Gas Industry B 1 (02), 165–171. doi:10.1016/j.ngib.2014.11.007
He, S., Li, H., Qin, Q., and Long, S. (2021). Influence of Mineral Compositions on Shale Pore Development of Longmaxi Formation in the Dingshan Area, Southeastern Sichuan Basin, China. Energy Fuels 35 (13), 10551–10561. doi:10.1021/acs.energyfuels.1c01026
He, Z., Li, S., Nie, H., Yuan, Y., and Wang, H. (2019). The Shale Gas "sweet Window": "The Cracked and Unbroken" State of Shale and its Depth Range. Mar. Pet. Geology. 101, 334–342. doi:10.1016/j.marpetgeo.2018.11.033
Hou, E. K., Cong, T., Xie, X. S., and Wei, J. B. (2020). Ground Surface Fracture Development Characteristics of Shallow Double Coal Seam Staggered Mining Based on Particle Flow. J. Min. Strata Control. Eng. 2 (1), 013521. doi:10.13532/j.jmsce.cn10-1638/td.2020.01.002
Huang, J. L., Zhou, C. N., Li, J. Z., Dong, D. Z., Wang, S. J., Wang, S. J ., et al. (2012). Shale Gas Generation and Potential of the Lower Cambrian Qiongzhusi Formation in Southern Sichuan Basin, China, Petrol. Explor. Dev. 39 (01), 69–75. doi:10.1016/S1876-3804(12)60017-2
Jarvie, D. M., Hill, R. J., Ruble, T. E., and Pollastro, R. M. (2007). Unconventional Shale-Gas Systems: The Mississippian Barnett Shale of north-central Texas as One Model for Thermogenic Shale-Gas Assessment. Bulletin 91, 475–499. doi:10.1306/12190606068
Kump, L. R., and Arthur, M. A. (1999). Interpreting Carbon-Isotope excursions:Carbonates and Organic Matter. Chem. Geol. 168 (1-3), 181–198. doi:10.1016/S0009-2541(99)00086-8
Li, H., Tang, H. M., and Zheng, M. J. (2019a). Micropore Structural Heterogeneity of Siliceous Shale Reservoir of the Longmaxi Formation in the Southern Sichuan Basin, China. Minerals 9, 548. doi:10.3390/min9090548
Li, H., Tang, H., Qin, Q., Zhou, J., Qin, Z., Fan, C., et al. (2019b). Characteristics, Formation Periods and Genetic Mechanisms of Tectonic Fractures in the Tight Gas Sandstones Reservoir: A Case Study of Xujiahe Formation in YB Area, Sichuan Basin, China. J. Pet. Sci. Eng. 178, 723–735. doi:10.1016/j.petrol.2019.04.007
Li, H. T., Peng, R., Du, W. S., Li, X. P., and Zhang, N. B. (2021b). Experimental Study on Structural Sensitivity and Intervention Mechanism of Mechanical Behavior of Coal Samples. J. Min. Strata Control. Eng. 3 (4), 043012. 13532/j.jmsce.cn10-1638/td.20210820.001.
Li, H., Wang, Q., Qin, Q., and Ge, X. (2021a). Characteristics of Natural Fractures in an Ultradeep Marine Carbonate Gas Reservoir and Their Impact on the Reservoir: A Case Study of the Maokou Formation of the JLS Structure in the Sichuan Basin, China. Energy Fuels 35, 13098–13108. doi:10.1021/acs.energyfuels.1c01581
Li, S., Li, Y., He, Z., Chen, K., Zhou, Y., and Yan, D. (2020). Differential Deformation on Two Sides of Qiyueshan Fault along the Eastern Margin of Sichuan Basin, China, and its Influence on Shale Gas Preservationfluence on Shale Gas Preservation. Mar. Pet. Geology. 121, 104602. doi:10.1016/j.marpetgeo.2020.104602
Li, Y. F., Lv, H. G., Zhang, Y., Zhang, X. L., Shao, D. Y., Yan, J. P., et al. (2015). U-mo Covariation in marine Shales of Wufeng—Longmaxi Formations in Sichuan Basin, China and its Implication for Identification of Watermass Restriction. Geochimica 44 (02), 109–116. doi:10.19700/j.0379-1726.2015.02.001
Li, Y., Zhou, D.-H., Wang, W.-H., Jiang, T.-X., and Xue, Z.-J. (2020b). Development of Unconventional Gas and Technologies Adopted in China. Energ. Geosci. 1 (1-2), 55–68. doi:10.1016/j.engeos.2020.04.004
Long, S., Feng, D., Li, F., and Du, W. (2018). Prospect Analysis of the Deep marine Shale Gas Exploration and Development in the Sichuan Basin, China. J. Nat. Gas Geosci. 3 (04), 181–189. doi:10.1016/j.jnggs.2018.11.001
Ma, X. H., and Xie, J. (2018). The Progress and Prospects of Shale Gas Exploration and Exploitation in Southern Sichuan Basin, SW China. Petrol. Explor. Dev. 45 (1), 161–169. doi:10.1016/S1876-3804(18)30018-1
Ma, X. H., Xie, J., Yong, R., and Zhu, Y. Q. (2020). Geological Characteristics and High Production Control Factors of Shale Gas Reservoirs in Silurian Longmaxi Formation, Southern Sichuan Basin, SW China. Pet. Exploration Develop. 47 (05), 841–855. doi:10.1016/S1876-3804(20)60105-7
Ma, X., Wang, H., Zhou, S., Shi, Z., and Zhang, L. (2021). Deep Shale Gas in China: Geological Characteristics and Development Strategies. Energ. Rep. 7, 1903–1914. doi:10.1016/j.egyr.2021.03.043
Melchin, M. J., and Holmden, C. (2006). Carbon Isotope Chemostratigraphy in Arctic Canada: Sea-Level Forcing of Carbonate Platform Weathering and Implications for Hirnantian Global Correlation. Palaeogeogr. Palaeoclimatol. Palaeoecol. 234 (2), 186–200. doi:10.1016/j.palaeo.2005.10.009
Nie, H., He, Z., Wang, R., Zhang, G., Chen, Q., Li, D., et al. (2020b). Temperature and Origin of Fluid Inclusions in Shale Veins of Wufeng-Longmaxi Formations, Sichuan Basin, south China: Implications for Shale Gas Preservation and Enrichment. J. Pet. Sci. Eng. 193, 107329. doi:10.1016/j.petrol.2020.107329
Nie, H., Li, D., Liu, G., Lu, Z., Hu, W., Wang, R., et al. (2020a). An Overview of the Geology and Production of the Fuling Shale Gas Field, Sichuan Basin, China. Energ. Geosci. 1 (3–4), 147–164. doi:10.1016/j.engeos.2020.06.005
Pan, R., Gong, Q., Yan, J., and Jin, J. (2016). Elements and Gas Enrichment Laws of Sweet Spots in Shale Gas Reservoir: A Case Study of the Longmaxi Fm in Changning Block, Sichuan Basin. Nat. Gas Industry B 3 (03), 195–201. doi:10.1016/j.ngib.2016.05.003
Pu, B. L., Dong, D. Z., Wang, F. Q., Wang, Y. M., and Hang, J. L. (2020). The Effect of Sedimentary Facies on Longmaxi Shale Gas in Southern Sichuan Basin. Geology. China 47 (01), 111–120. (in Chinese). doi:10.12029/gc20200109
Qie, L., Shi, Y. N., and Liu, J. G. (2021). Experimental Study on Grouting Diffusion of Gangue Solid Filling Bulk Materials. J. Min. Strata Control. Eng. 3 (2), 023011. doi:10.13532/j.jmsce.cn10-1638/td.20201111.001
Shan, S. C., Wu, Y. Z., Fu, Y. K., and Zhou, P. H. (2021). Shear Mechanical Properties of Anchored Rock Mass under Impact Load. J. Min. Strata Control. Eng. 3 (4), 043034. doi:10.13532/j.jmsce.cn10-1638/td.20211014.001
Song, J. F., Lu, C. P., Li, Z. W., Ou, Y. G. C., Cao, X. M., and Zhou, F. L. (2021). Characteristics of Stress Distribution and Microseismic Activity in Rock Parting Occurrence Area. J. Min. Strata Control. Eng. 3 (4), 043518. doi:10.13532/j.jmsce.cn10-1638/td.20210607.002
Wang, B., Lu, C. L., Huang, Z. K., and Hu, S. Y. (2021). Experimental Study on Damage Evolution Characteristics of Rock under Triaxial Rheological Disturbance. J. Min. Strata Control. Eng. 3 (4), 043028. doi:10.13532/j.jmsce.cn10-1638/td.20210525.001
Wang, G. (2020). Deformation of Organic Matter and its Effect on Pores in Mud Rocks. Bulletin 103, 21–36. doi:10.1306/04241918098
Wang, H., Shi, Z., Zhao, Q., Liu, D., Sun, S., Guo, W., et al. (2020). Stratigraphic Framework of the Wufeng-Longmaxi Shale in and Around the Sichuan Basin, China: Implications for Targeting Shale Gas. Energ. Geosci. 1 (3–4), 124–133. doi:10.1016/j.engeos.2020.05.006
Wang, J., and Wang, X. L. (2021). Seepage Characteristic and Fracture Development of Protected Seam Caused by Mining Protecting Strata. J. Min. Strata Control. Eng. 3 (3), 033511. doi:10.13532/j.jmsce.cn10-1638/td.20201215.001
Wang, K. L., Li, K. Q., Wang, L. K., Gao, H. L., Wang, K. W., and Hu, P. Q. (2018). Mineral Composition and Gas-Bearing Characteristics of Wufeng-Longmaxi Shale in Shizhu Area, Eastern Sichuan Basin. J. Lanzhou University(Natural Sciences) 54 (03), 285–291+302. doi:10.13885/j.issn.0455-2059.2018.03.001
Wang, X., Liu, L., Wang, Y., Sheng, Y., Zheng, S., Wu, W., et al. (2020). Comparison of the Pore Structures of Lower Silurian Longmaxi Formation Shales with Different Lithofacies in the Southern Sichuan Basin, China. J. Nat. Gas Sci. Eng. 81, 103419. doi:10.1016/j.jngse.2020.103419
Wang, Z. (2017). RETRACTED ARTICLE: Characterization of the Microscopic Pore Structure of the Lower Paleozoic Shale Gas Reservoir in the Southern Sichuan Basin and its Influence on Gas Content. Pet. Sci. Technol. 35 (23), 2165–2171. doi:10.1080/10916466.2017.1390682
Xie, J., Qin, Q., Fan, C., Hu, D., and Li, H. (2019). The Quantitative Prediction of Tectonic Fractures of Longmaxi Formation in Dingshan Area, southeastern Sichuan Basin. Earth Sci. Res. J. 23 (2), 157–162. doi:10.15446/esrj.v23n2.79957
Xu, S., Gou, Q., Hao, F., Zhang, B., Shu, Z., Lu, Y., et al. (2020). Shale Pore Structure Characteristics of the High and Low Productivity wells, Jiaoshiba Shale Gas Field, Sichuan Basin, China: Dominated by Lithofacies or Preservation Condition? Mar. Pet. Geology. 114, 104211. doi:10.1016/j.marpetgeo.2019.104211
Yan, D., Chen, D., Wang, Q., Wang, J., and Wang, Z. (2009). Carbon and Sulfur Isotopic Anomalies across the Ordovician-Silurian Boundary on the Yangtze Platform, South China. Palaeogeogr. Palaeoclimatol. Palaeoecol. 274 (1-2), 32–39. doi:10.1016/j.palaeo.2008.12.016
Yin, S., Lv, D., and Ding, W. (2018). New Method for Assessing Microfracture Stress Sensitivity in Tight sandstone Reservoirs Based on Acoustic Experiments. Int. J. Geomechanics 18 (4), 1–16. doi:10.1061/(ASCE)GM.1943-5622.0001100
Yu, X., Bian, J. Q., and Liu, C. Y. (2022). Determination of Energy Release Parameters of Hydraulic Fracturing Roof Near Goaf Based on Surrounding Rock Control of Dynamic Pressure Roadway. J. Min. Strata Control. Eng. 4 (1), 013016. doi:10.13532/j.jmsce.cn10-1638/td.20210908.001
Yu, X. Y., Wang, Z. S., Yang, Y., and Mao, X. W. (2021). Numerical Study on the Movement Rule of Overburden in Fully Mechanized Caving Mining with Thick Depth and High Mining Height. J. Min. Strata Control. Eng. 3 (1), 013533. doi:10.13532/j.jmsce.cn10-1638/td.20200715.001
Zecheng, W., Shipeng, H., Deyu, G., Wei, W., and Chong, Y. (2013). Geochemical Characteristics of Natural Gases in the Upper Triassic Xujiahe Formation in the Southern Sichuan Basin, SW China. Int. J. Coal Geology. 120, 15–23. doi:10.1016/j.coal.2013.09.002
Zhang, K., Jia, C., Song, Y., Jiang, S., Jiang, Z., Wen, M., et al. (2020). Analysis of Lower Cambrian Shale Gas Composition, Source and Accumulation Pattern in Different Tectonic Backgrounds: A Case Study of Weiyuan Block in the Upper Yangtze Region and Xiuwu Basin in the Lower Yangtze Region. Fuel 263, 115978. doi:10.1016/j.fuel.2019.115978
Keywords: shale gas, sedimentary environment, accumulation conditions, different preservation conditions, Wufeng-Longmaxi formation, Southern China
Citation: He S, Qin Q, Li H and Zhao S (2022) Geological Characteristics of Deep Shale Gas in the Silurian Longmaxi Formation in the Southern Sichuan Basin, China. Front. Earth Sci. 9:818155. doi: 10.3389/feart.2021.818155
Received: 19 November 2021; Accepted: 20 December 2021;
Published: 20 January 2022.
Edited by:
Kun Zhang, Southwest Petroleum University, ChinaReviewed by:
Shuai Yin, Xi’an Shiyou University, ChinaZhu Baiyu, Yangtze University, China
Yang Wang, China University of Petroleum, Beijing, China
Copyright © 2022 He, Qin, Li and Zhao. This is an open-access article distributed under the terms of the Creative Commons Attribution License (CC BY). The use, distribution or reproduction in other forums is permitted, provided the original author(s) and the copyright owner(s) are credited and that the original publication in this journal is cited, in accordance with accepted academic practice. No use, distribution or reproduction is permitted which does not comply with these terms.
*Correspondence: Hu Li, bGlodTg2MDYyOEAxMjYuY29t