- 1Research Institute of Exploration and Development, Tarim Oil-field Company, Korla, China
- 2Oil and Gas Survey, China Geological Survey, Beijing, China
- 3Department of Geography, Kyung Hee University, Seoul, South Korea
- 4Institute of Geology and Geophysics, Key Laboratory of Petroleum Resource Research, Chinese Academy of Sciences, Beijing, China
The Mesozoic syn- and post-collision structures were revealed in the southern Tarim Basin based on careful seismic interpretation and field investigation. The syn-collision structures are the Late Triassic thrusts in the piedmonts of W. Kunlun and Altun Mountains and the Markit Slope. They include both newly formed thrusts and reactivation of some preexisting thrusts, such as the Qarqan fault in the piedmont of the Altun Mountains. The post-collision structures are the Jurassic–Cretaceous normal faults, with the development of horsts and grabens. In the Late Triassic, the maximum stress was SSW-NNE-directed in the piedmont of the W. Kunlun Mountains and the Markit Slope but SSE-NNW-directed in the piedmont of the Altun Mountains, forming many reverse faults thrusting from the mountains to the Tarim Basin with the development of some back-thrusts in the Markit Slope. They are the syn-collision structures in response to the North Qiangtang–Tarim collision. This Late Triassic collision is an important tectonic event in the complicated closing process of the Paleo-Tethys. The Jurassic–Cretaceous extensional structures are the post-collision structures.
Introduction
The Tarim Basin is located between the Tethysides and Altaids (or Central Asian Orogenic Belt), two super orogenic complexes in the world (Figure 1). Its tectonic history is closely related to these two orogenic systems. Previously, the geologists studied the Tethysides and Altaids mainly within the mountains, few in the neighboring basins. The key reason is that the basins are covered with younger deposits, especially the Cenozoic deposits. It is impossible to investigate the geology of the basins on the Earth's surface. The stratigraphic sections in the basin are relatively complete, from the Upper Precambrian to the Quaternary (Zhou, 2001; Jia et al., 2004a; Sun, 2004). The tectonic events in the surrounding orogenic belt resulted in different structural deformations in and around the basin. As the Tarim Basin is a sedimentary basin with a Precambrian cratonic crystalline basement, the deformation in it is relatively weaker, and the preexisting structures were relatively weakly transformed during the later deformation. Therefore, the deformations of different tectonic events at different times could be well preserved in the basin. The newly collected seismic and drilling data during petroleum exploration in the basin provide a possibility for the geologists to study the underground geology in the basin. So, the pre-Cenozoic structures in the Tarim Basin could be analyzed in detail (Jia, 1997; Jia et al., 2004b; Yang et al., 2007; Li et al., 2013; Li et al., 2015; Yang et al., 2015; Li et al., 2016a; Li et al., 2016b; Li Y. et al., 2016; Li et al., 2019; Li et al., 2020; Wen et al., 2020). These structures of different ages, different types, and different origins are important records of the tectonic evolution process of the Tethysides and Altaids.
Triassic is a key time in the tectonic evolution history of both the Tethysides and Altaids. For the Tethysides, the oceanic crust subduction, the oceanic basin(s) closing, and some collision events occurred in the Paleo-Tethyan realm during the Triassic time, and the Neo-Tethys was opened in Triassic (Hsü and Bernoulli, 1978; ȘengÖr, 1985; Chang et al., 1986; ȘengÖr, 1987; ȘengÖr et al., 1988; ȘengÖr, 1990; ȘengÖr, 1992; ȘengÖr and Nata’in, 1996; Mattern and Schneider, 2000; Jia, et al., 2001; Yang et al., 2002; Xiao et al., 2005; Chen et al., 2009; Wu et al., 2020; Zeng et al., 2020). For the Altaids, the Southern Tianshan Ocean was closed during the Triassic and formed the South Tianshan Mountains at its southernmost margin. The Paleo-Asian Ocean was possibly finally closed after this collision orogeny (ȘengÖr et al., 1993; Li et al., 2002; Xiao et al., 2002; Li et al., 2005; Windley et al., 2006; Xiao et al., 2008; Li et al., 2009; Xiao et al., 2009; Li et al., 2010; Li et al., 2012; Zhao et al., 2012; Li et al., 2013; Huang et al., 2020; Li et al., 2020; Wen et al., 2020). The closing of the Southern Tianshan Ocean led to the Tarim–Yili collision. Its collision-related structures in the northern Tarim Basin have been revealed and analyzed systematically (Zhao et al., 2012; Li et al., 2020; Wen et al., 2020).
The Mesozoic collision-related structures in the southern Tarim Basin imply an important collision event in the Paleo-Tethys closing history. Previously, these collision-related structures were ignored or unnoticed for a long time. Recently, some Late Triassic structures have been discovered in the piedmont of the West Kunlun Mountains (Chen Y.G et al., 2018). Based on several years of continuous field investigation and seismic interpretation, we have revealed more and more Mesozoic collision-related structures, including the Late Triassic syn-collision structures and Jurassic–Cretaceous post-collision structures in the southern Tarim Basin, not only in the piedmont of West Kunlun Mountain but also in the Markit Slope and the piedmont of Altun Mountains. Their related collision should be located south of the Tarim plate. It is the North Qiantang–Tarim collision. This paper reports these collision-related structures preserved in the southern Tarim Basin and discuss their significance for the Paleo-Tethys study.
Seismic Data and Interpretation
Seismic Data Are Used in This Paper, Under Kind Permission of the Tarim Oilfield Company, PetroChina
The Mesozoic collision-related structures are hard to be preserved in the Kunlun and Altun Mountains because of the severe deformation in the Late Cenozoic under the far-field effect of the India–Asia collision (Molnar and Tapponnier, 1975; Tapponnier and Molnar, 1977; Sobel and Dumitru, 1997; Cui et al., 2002; Yang et al., 2002; Chen H. L. et al., 2018). Although the (late) deformation is much weaker and the stratigraphic system is more complete in the Tarim Basin, the pre-Cenozoic structures (including the Mesozoic collision-related structures) could be relatively well preserved within the basin (Jia et al., 1992; Jia et al., 2001; Li et al., 2013; Li et al., 2015; Li et al., 2016a; Wen et al., 2020). However, the Tarim Basin is covered with thick Upper Cenozoic deposits, especially, the Taklamakan Desert. The Mesozoic strata and structures are deeply concealed and are impossible to be directly investigated in the field. Thus, the seismic data and interpretation are essential for this study. They make it possible to investigate and analyze the underground strata and structures.
Seismic interpretation is the key method in this research. It is a method widely used in petroleum exploration and development in the world. The relationship between seismic stratigraphy, chronostratigraphy, and lithostratigraphy is the base of seismic interpretation. After then, the geological meaning of each main seismic reflector is given. In the Tarim Basin, each petroleum exploration well has a drilling report and even a single-well evaluation report for the important exploration wells. Based on the stratigraphy of the drilling reports from representative drilling wells, the correlations between the seismic reflectors and chronostratigraphic horizons are carefully discussed. For horizon calibration, we usually choose the respective seismic profile(s) across the well and carefully define the geological (chronostratigraphic and lithostratigraphic) meanings of the major reflectors on the basis of the single-well stratigraphic study results, synthetic seismogram, and single-well vertical seismic profile data. This work is essential for the following interpretation. At the same time, many special stratigraphic studies were carried out, such as Jia et al. (1992,2004b), Li et al. (1992), and Zhang et al. (2004). Also, now, the relationship between the seismic stratigraphy, chronostratigraphy, and lithostratigraphy in the Tarim Basin is clear and definite. We quote this study result directly in this paper. Also, then, the seismic interpretation is just like structural geology. During the interpretation, we trace the reflectors to identify faults, folds, and unconformities. The deformation is timed according to the variation and termination of seismic reflectors.
Mesozoic Collision-Related Structures in the Southwestern Tarim Basin
The Mesozoic collision-related structures were discovered in the piedmont of the West Kunlun Mountains and the Markit Slope of the Tarim Basin. They are the Late Triassic syn-collision structures and the Jurassic–Cretaceous post-collision structures.
Mesozoic Collision-Related Structures in the Piedmont of the West Kunlun Mountains
The far-field effect of India–Asia collision led to the reviving of many ancient orogenic belts, such as Kunlun, Altun, and Tianshan in Central Asia during the Late Cenozoic (Molnar and Tapponnier, 1975; Tapponnier and Molnar, 1977; Zhang et al., 1996; Yin et al., 1998; Li et al., 2001a; Bullen et al., 2001; Cowgill, 2010; Cao et al., 2015). During this intracontinental orogenic process, the mountain massifs were pushed toward the neighboring basins while uplifted rapidly, forming foreland fold-thrust belts in the piedmont of the mountains (Lu et al., 1994; Sobel and Dumitru, 1997; Wang et al., 2002; Guan et al., 2007; Wang et al., 2009; Yang et al., 2010; Cheng et al., 2012a; Chen H.L et al., 2018).
The previous structural analysis in the piedmont of the West Kunlun mostly focused on the Late Cenozoic fold and thrust deformations (Wu et al., 2004; Cheng et al., 2012a; Xie et al., 2012; Wang, 2014; Xu et al., 2016; Chen H.L. et al., 2018), rare on the pre-Cenozoic structures (Chen Y. G. et al., 2018), which were strongly transformed during the Late Cenozoic deformation and difficult to identify. The Mesozoic collision-related structures in southern Tarim Basin were first discovered in the piedmont of the West Kunlun Mountains (Chen Y. G. et al., 2018) and verified by our following field investigations, seismic interpretations, and drilling data analysis.
The Triassic strata are sparsely outcropped in the piedmont of the West Kunlun. The Jurassic and Cretaceous strata distribute only in a narrow zone at the foot of the mountains and rapidly pinched out toward the basin (Figures 2 and 3).
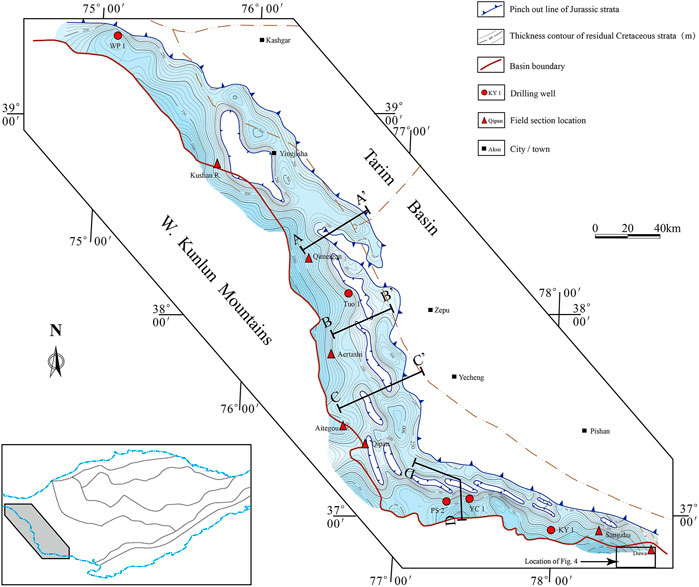
FIGURE 3. Distribution of residual Cretaceous strata in piedmont of western Kunlun (see location also in Figure 1).
The only known Triassic strata in the piedmont of West Kunlun are the Lower Triassic Wuzunsayi Formation in the Duwa area (Figure 4). It is a 200–300-m-thick clastic rock formation, including purple-red feldspathic quartz sandstone, silty mudstone, interbedded with gray-yellow, gray-black, gray-green silty mudstone, and mudstone. The Wuzunsayi Formation is conformably overlying the Upper Permian Duwa Formation and unconformably overlain by the Jurassic and Cretaceous strata (Yang, 1994; Li, 2000; Huang et al., 2012; Chen Y. G. et al., 2018). This is important evidence for the time of the Triassic collision. The Jurassic or Cretaceous strata are directly unconformably overlying the Permian and older strata, missing the entire Triassic in almost the whole piedmont of West Kunlun except the Duwa area (Figures 5 and 6A,B). It is conformity or parallel unconformity between the Jurassic and Cretaceous, indicating a relatively stable tectonic setting without major tectonic events during Jurassic–Cretaceous. There is a major unconformity between the Jurassic–Cretaceous and Lower Triassic strata in the piedmont of West Kunlun. The Lower Triassic (Wuzunsayi Formation) in the Duwa area and its stratigraphic relationship with the underlying and overlying strata indicate an obvious Late Triassic tectonic event (Sobel, 1999; Yang et al., 2002; Cheng et al., 2012b; Chen Y.G et al., 2018). That is the collision between the North Qiangtang and Tarim Basin.
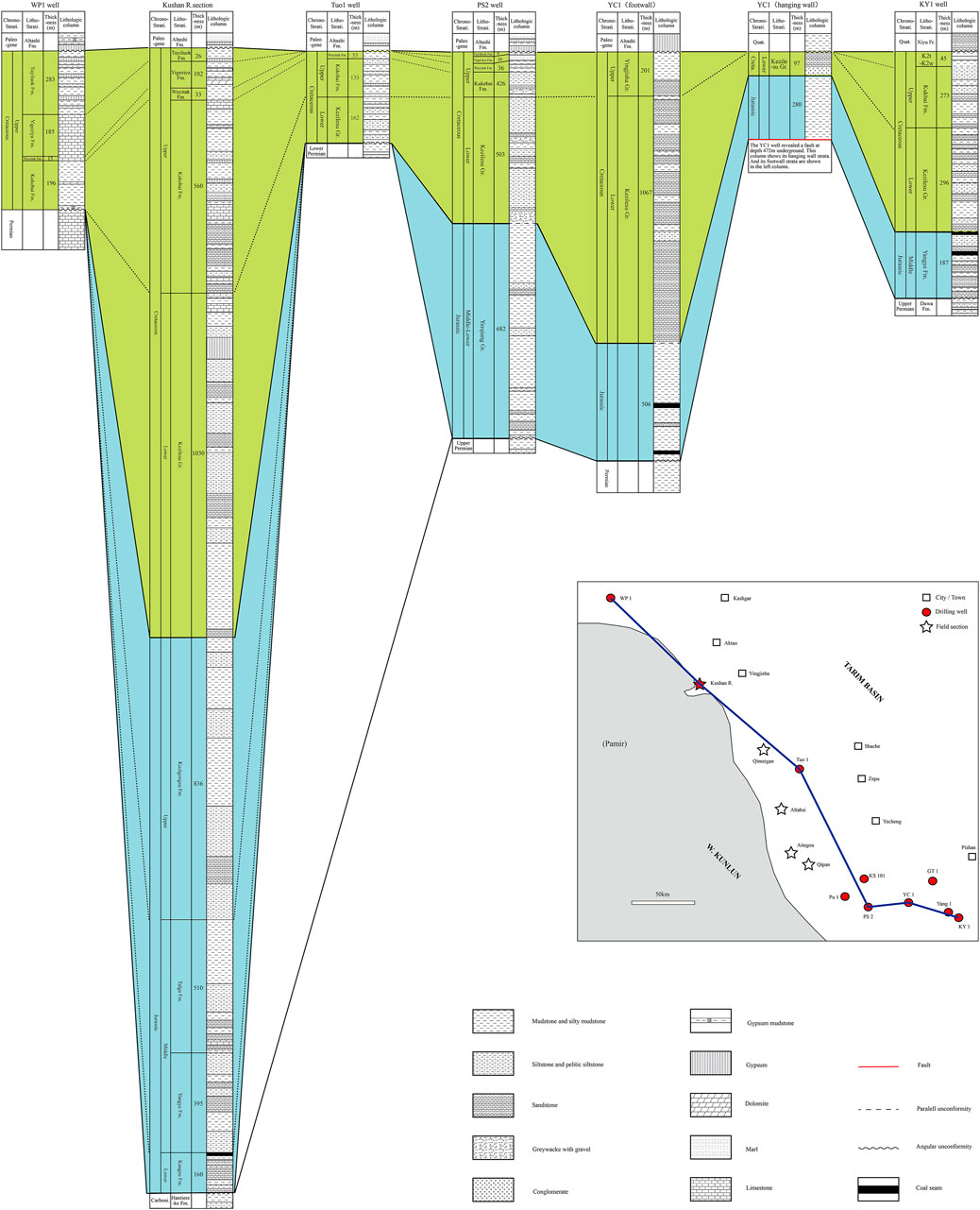
FIGURE 5. Mesozoic stratigraphic columns and correlation in piedmont of western Kunlun (locations of wells and section are also shown in Figures 2 and 3).
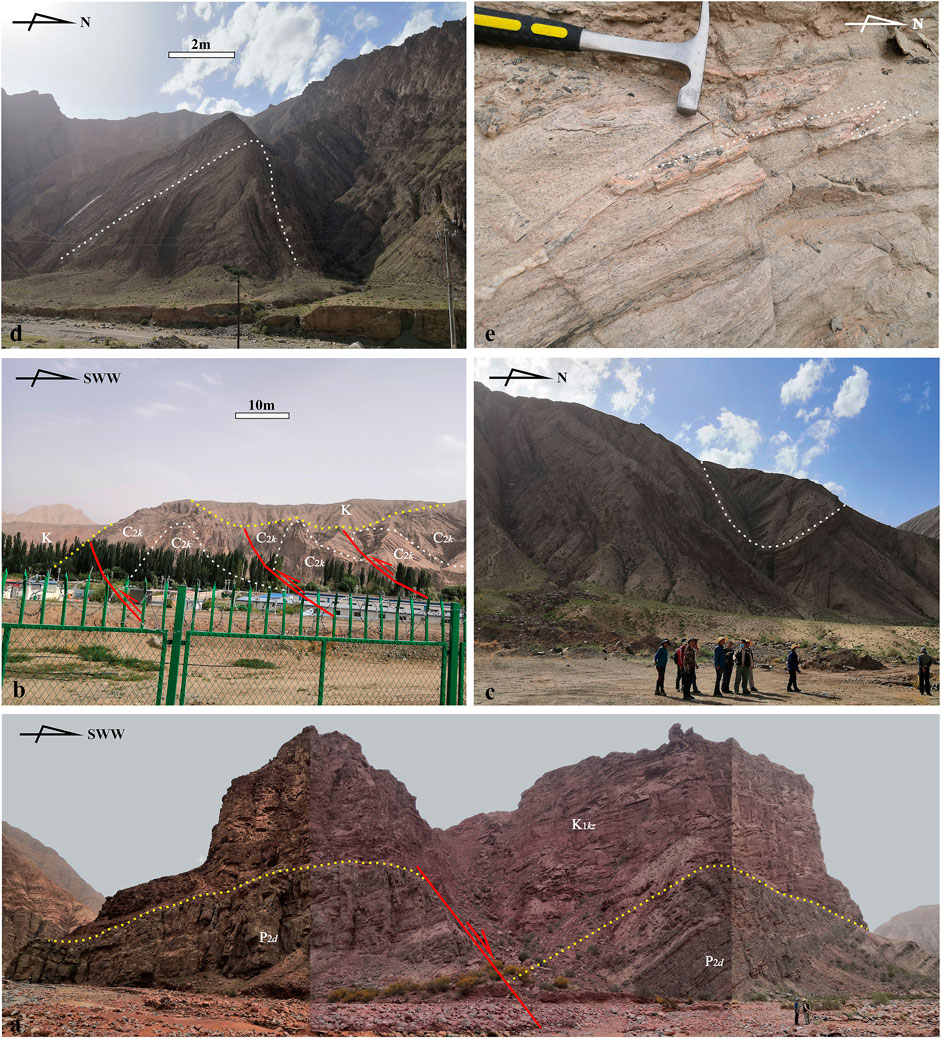
FIGURE 6. Triassic thrusts and bottom unconformity of Jurassic–Cretaceous strata on outcrops in piedmont of West Kunlun (see section locations in Figures 2 and 3). Yellow dotted line: unconformity; white dotted line: stratigraphic level drawn to show structural deformation; red line: fault. (A) Unconformity between Cretaceous Kezilesu Group and Permian Dariyol Formation in Qimeigan section; (B) asymmetric syncline of Permian Keziliqiman Formation in Qipan section; (C) asymmetrical anticline of Azigan Formation of Carboniferous in Qipan section; (D) weakly structurally deformed Cretaceous unconformity over strongly structurally deformed Carboniferous Karawuyi Formation; (E) ductile shear deformation in granite gneiss of Eliankat Group in Sangzhu section.
The Jurassic–Cretaceous strata above this unconformity were slightly deformed. The thrusts are developed in the Permo-Carboniferous and older rocks under the unconformity (Figures 6B–D), and the Jurassic–Cretaceous strata were not involved in the thrust deformation (Figure 6B). The thrusting occurred in the (Late) Triassic. The thrusting direction in the piedmont of West Kunlun is basin-ward, i.e., from Kunlun to Tarim Basin.
The ductile shear deformation in the granite gneiss in the Ailiankat Group on the Sangzhu section is possibly a special type of the Late Triassic thrust, which also indicates the basin-ward thrusting (Figure 6E). In the Early Paleozoic, the Paleo-Tethys oceanic crust subducted under the Tarim continental crust, forming an accretionary wedge, the West Kunlun Early Paleozoic accretionary wedge (Xiao et al., 2002; Xiao et al., 2005). In this accretionary wedge, the main structural deformation should be thrust southward. However, the thrust direction of ductile shear deformation of Sangzhu granite gneiss is northward. Furthermore, the ductile shear deformation in the Sangzhu granite gneiss is a rather deep structure, which is obviously different from the late Cenozoic brittle thrust structures widely distributed in the West Kunlun (Sobel and Dumitru, 1997; Cowgill, 2010; Sobel et al., 2011; Xie et al., 2012; Cao et al., 2015; Xu et al., 2016). Therefore, we can fairly infer that this ductile shear structure is possibly also a Late Triassic syn-collision structure.
The Late Triassic collision-related structures were also be revealed by seismic interpretation (Figures 7 and 8).
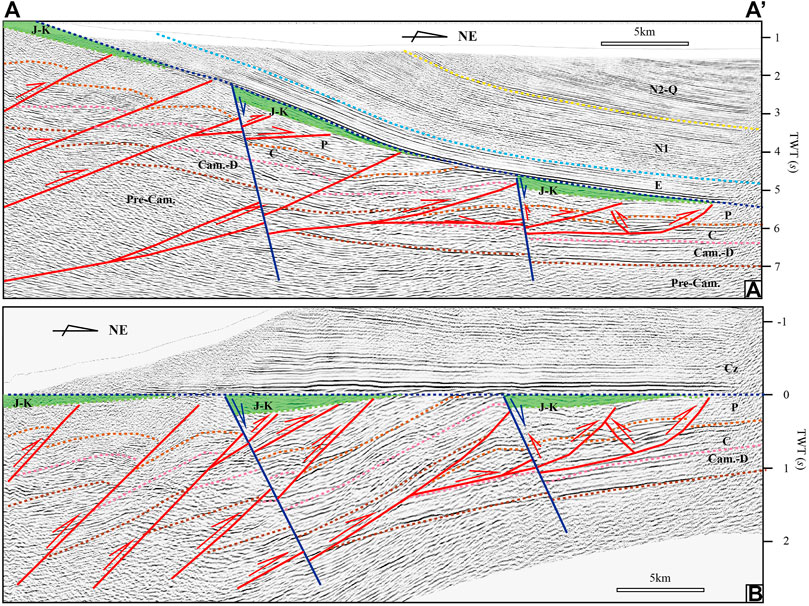
FIGURE 7. A–A′ two-dimensional seismic section in piedmont of W. Kunlun (see locations in Figures 2 and 3). (A) section’s initial appearance; (B) flattened its Paleogene bottom. Red lines represent faults active in Triassic (syn-collision structure); blue lines represent Jurassic–Cretaceous normal faults (post-collision structure, that one on right is a negative inversion structure).
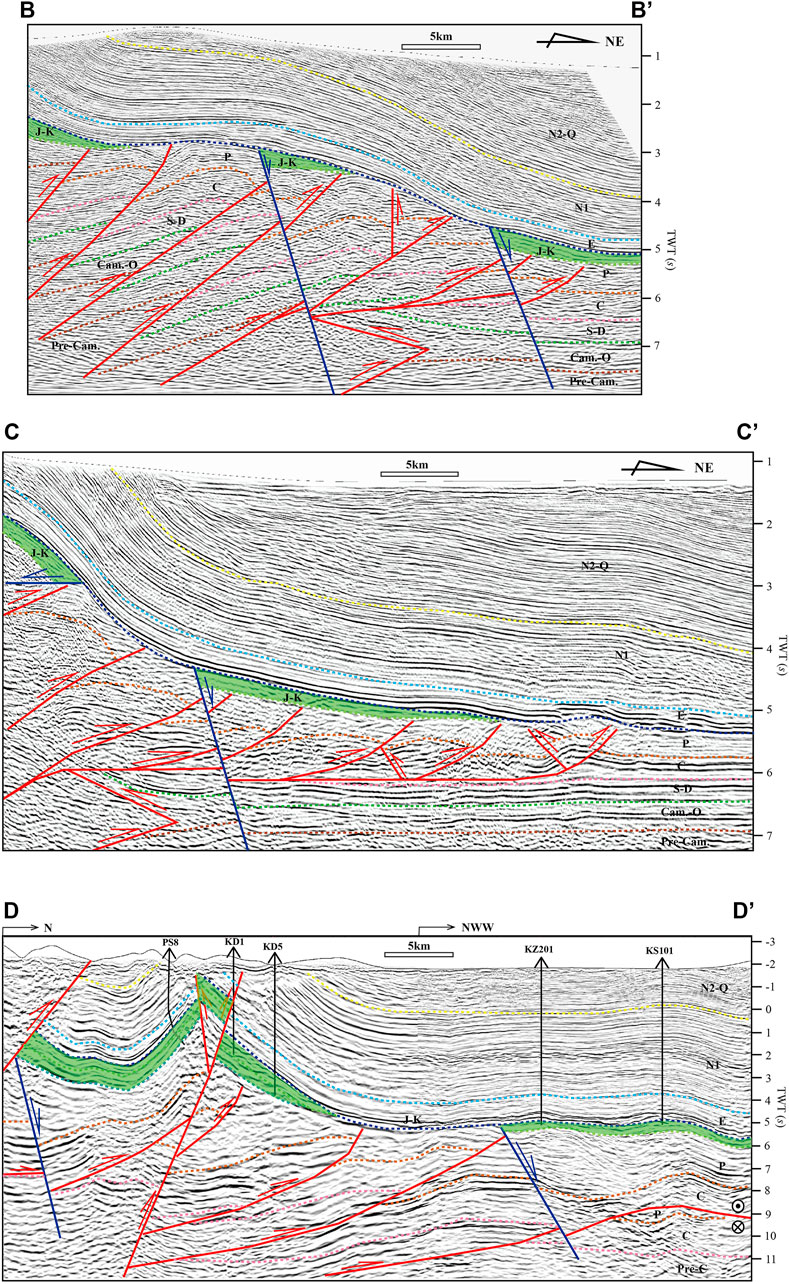
FIGURE 8. B–B′, C–C′, and D–D′ seismic sections (see locations in Figures 2 and 3). B–B′ and C–C′ are two-dimensional seismic sections. D–D′ is a three-dimensional seismic section. Red lines represent faults active in Triassic (syn-collision structure); blue lines represent Jurassic–Cretaceous normal faults (post-collision structure).
On the seismic sections, the Late Triassic thrusts cut through the Paleozoic and stop at the bottom of the Jurassic–Cretaceous. The deformations in the Jurassic–Cretaceous and the overlying Cenozoic are similar. They were not involved in the Late Triassic thrusting (Figures 7 and 8).
The Jurassic–Cretaceous deposited in half grabens and/or grabens are controlled by the normal faults (Figures 7 and 8). The Jurassic–Cretaceous half-graben can also be directly observed in the field (Figure 6A). They were formed subsequently after the Late Triassic syn-collision thrusts.
Drilling and outcrop data also show that the thickness of the Jurassic–Cretaceous strata is laterally various and basin-wardly pinched out rapidly. The YC1 well on the east side of Kekeya Oilfield encountered a large fault at a depth of 472 m (Figure 5). It thrusts from the Kunlun to the Tarim Basin. On its hanging wall, the oldest strata are the 280-m-thick Jurassic strata. Above the Jurassic strata is the 97-m-thick Lower Cretaceous Kezilesu Group, which is unconformably overlaid by the Quaternary strata. On the footwall, the Permian mudstone was revealed at the bottom of the well. Upward is the 506-m-thick Jurassic strata, the 1,067-m-thick Lower Cretaceous Kezilesu Group, and the 201-m-thick Upper Cretaceous Yingjisha Group. There is a large stratigraphic hiatus between the Jurassic and Permian. Also, then, the Paleogene Altash Formation unconformably overlies the Upper Cretaceous Yingjisha Group (Figure 5). The footwall is the in situ stratigraphic system, and the hanging wall of the fault is the allochthonous system that came from the south. The Jurassic strata on the hanging wall are thicker than the footwall, and the (Lower) Cretaceous strata on the hanging wall are much thinner than the footwall. That is to say, the Jurassic–Cretaceous strata are not simply thickened southwardly. They were possibly deposited in many strip-shaped grabens.
The Jurassic–Cretaceous strata deposited in strip-shaped grabens formed on the Triassic thrusts. The initial features of the Late Triassic thrusts and Jurassic–Cretaceous grabens can be shown by the seismic section flattened its Paleogene bottom (Figure 7B).
The Jurassic and Cretaceous strata in the piedmont of the West Kunlun are distributed in strip-shaped (Figures 2 and 3), showing the planar distribution characteristics of graben–horst structures. The strata deposited in the grabens and missing on the horsts.
Mesozoic Collision-Related Structures in the Markit Slope, SW Tarim Basin
The Markit Slope is a secondary tectonic unit of the Southwestern Depression, SW Tarim Basin (Figure 1). Unlike the Kunlun Mountains, the pre-Cenozoic strata and structures are covered by kilometers-thick Cenozoic strata in the Markit. The Mesozoic collision-related structures cannot be directly investigated on the surface. Seismic interpretation is necessary to study these structures. On the basis of careful seismic interpretation, the Late Triassic syn-collision thrusts have been revealed in the Markit Slope, but no Jurassic–Cretaceous post-collision structures are revealed so far.
There are two widely distributed gypsum-salt series in the Markit Slope. They occur at the Middle Cambrian and lower Paleogene. They are not only high-quality regional caprocks but also the stratigraphic horizons where the main regional décollement faults developed (Yang et al., 2007; Yang et al., 2015; Li et al., 2016a; Yang et al., 2016). The Paleogene gypsum-salt series has no influence on the Mesozoic collision-related deformations. The Middle Cambrian gypsum-salt series serves as the main décollement surface of the Late Triassic syn-collision thrusts (Figure 9).
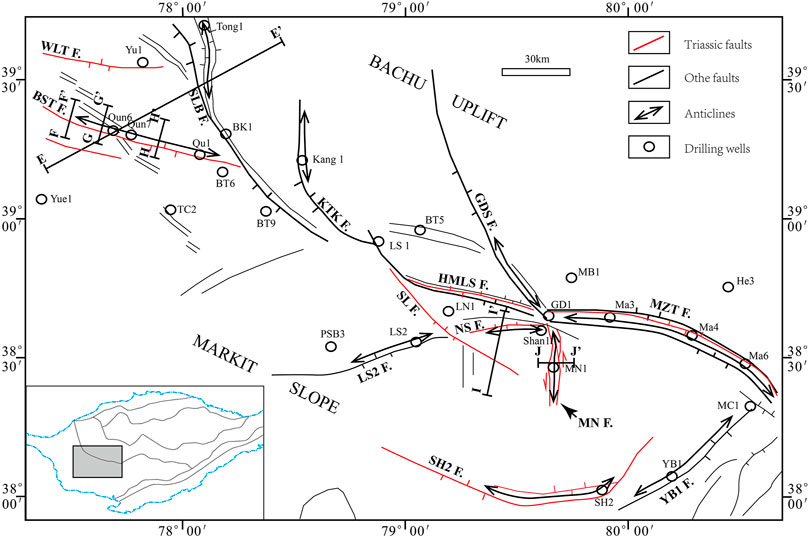
FIGURE 9. Fault distribution in Markit Slope, showing distribution of Triassic thrusts and strike-slip faults (see location also in Figure 1). BST F.—Bashituopu fault; WLT F.—Wolituogelake fault; SLB F.—Selibuya fault; KTK F.—Kangtakumu fault; HMLS F.—Haimiluosi fault; GDS F.—Gudongshan fault; MZT F.—Mazhatage fault; SL F.—Shalong fault; NS F.—Niaoshan fault; MN F—Manan fault; SH2 F.—Shenghe2 fault; YB1 F.—Yubei1 fault.
The Mesozoic strata are completely missing in the Markit Slope, and the Paleogene strata overlie the Permian strata directly (Figures 10 and 11). The Late Triassic syn-collision structures are a series of thrust and some strike-slip faults developed in the Paleozoic strata.
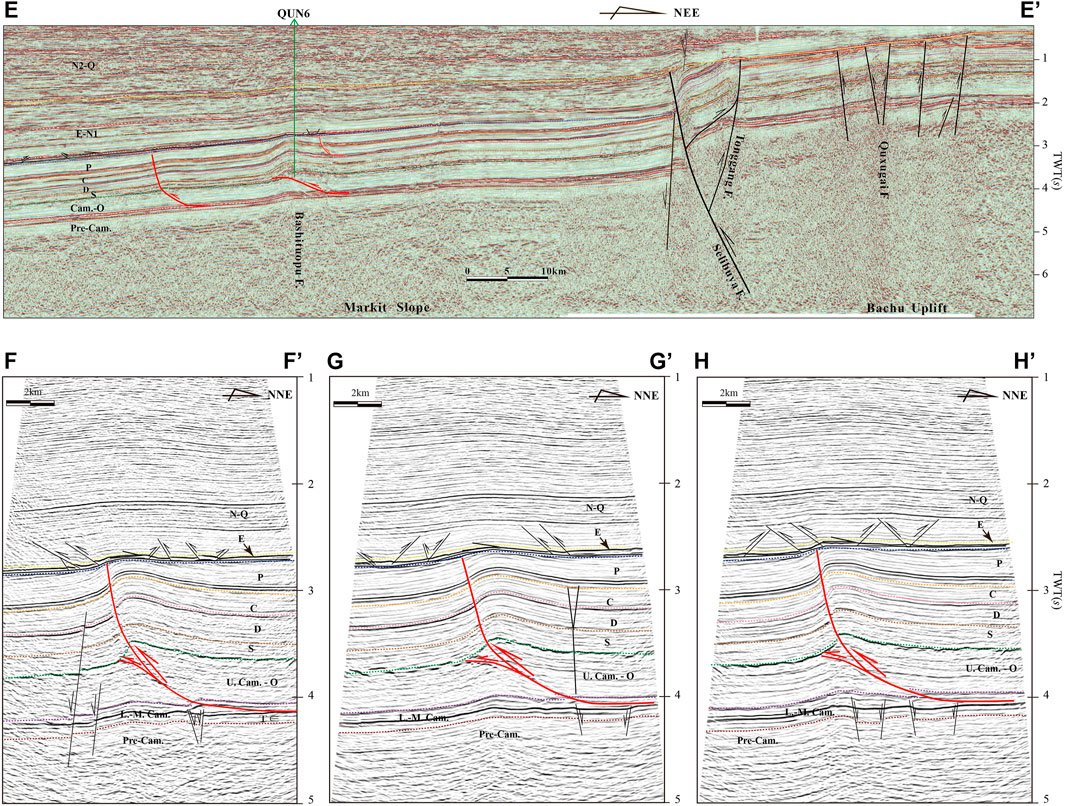
FIGURE 10. Seismic sections across Bashituopu structural belt (see location in Figure 9). E–E′ is a regional two-dimensional seismic section; others are three-dimensional seismic sections. Red lines represent faults active in Late Triassic (syn-collision thrust structures); black lines represent other faults.
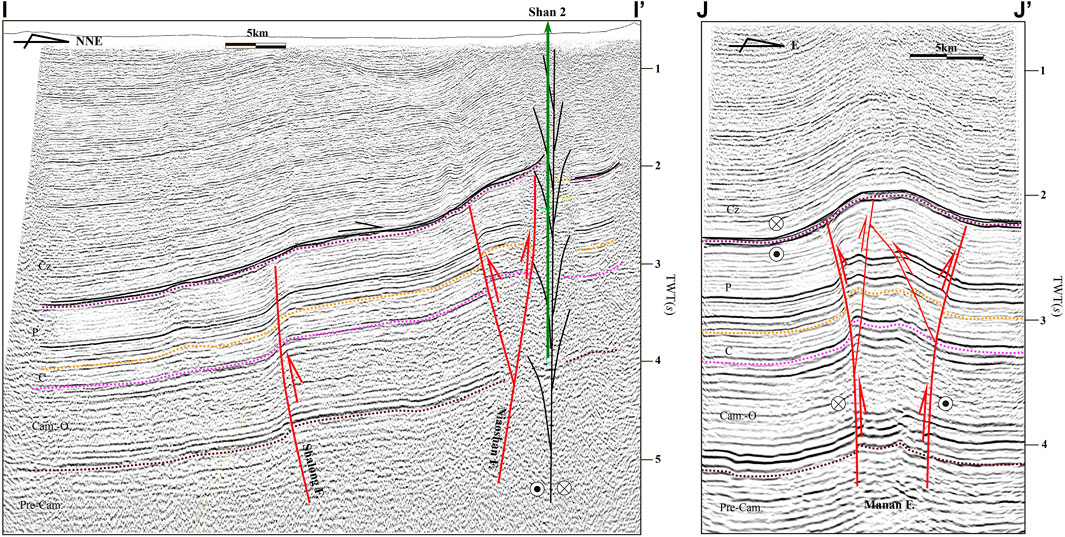
FIGURE 11. I–I′ 2D seismic section across Niaoshan and Shalong faults and J–J′ three-dimensional seismic section across Manan fault. Red lines represent faults active in Triassic (syn-collision thrust structures). Black lines represent other faults.
Among the thrusts, the Bashituopu structural belt in the western Markit Slope is a typical representative (Figures 9 and 10). The initial structural style of the Bashituopu belt is a fault-bend fold. In the late deformation stage, the southern wing of the fault-bend fold (Bashituopu anticline) was broken through and formed a faulted anticline, showing an illusion appearance of the fault-propagation fold. Careful observation reveals that the fault displacement of this breakthrough fault is only approximately 20 ms, and the original fault-bend folds can be basically preserved (G-G′ section on Figure 10). This fault-bend fold was not even broken and maintained its initial structural style on some sections (E-E′ section on Figure 10).
The Bashituopu anticline is mainly developed in the Cambrian–Permian and unconformably overlain by the Paleogene strata. At the top of the anticline, the Permian was more eroded, and the thickness of the eroded strata increased toward the core of the anticline and resulted in an angular unconformity between the Paleogene and the Permian. Away from the top of the anticline, it is a parallel unconformity or micro-angle unconformity between the Paleogene and Permian. Obviously, this anticline was formed after Permian and before Paleogene. Due to the lack of all the Mesozoic strata, its exact deformation time cannot be determined directly from the seismic sections. According to regional geology, from Jurassic to Cretaceous, Tarim Basin was in a regional extensional tectonic setting, and the Bashituopu structure was not likely formed at that time. Combined with the previous analysis on the Mesozoic deformations in the piedmont of the West Kunlun, the Bashituopu should be a Late Triassic thrust.
The Cenozoic strata above the anticline have a relatively slight deformation, forming a wide gentle anticline, indicating that the Bashituopu structure revived in the Cenozoic.
The Late Triassic syn-collision structures such as Shalong, Niaoshan, and Manan faults are also developed in the middle and eastern Markit Slope (Figure 11). The Shalong and Niaoshan faults are basement-involved Late Triassic thrusts. The Manan structural belt has obvious characteristics of a palm tree structure (J-J′ section in Figure 11). It is a shear-compressional strike-slip fault zone formed in the Late Triassic. The obvious deformations of the Cenozoic above the Shalong, Niaoshan, and Manan indicate that all these three structural belts and possibly all the Late Triassic syn-collision structures in the middle and eastern Markit Slope revived obviously in the Cenozoic.
Mesozoic Collision-Related Structures in the SE Tarim Basin
The SE Tarim Basin is also the northwestern piedmont of the Altun Mountains (Figures 1 and 12). The Qarqan fault is the largest fault zone in the Tarim Basin (Figures 12 and 13). The Jurassic–Cretaceous extensional structures in the piedmont of the Altun were once reported and interpreted as post-orogenic structures of the Cimmerides (Chen et al., 2009). Our recent seismic interpretation revealed the Late Triassic thrusts together with the Jurassic–Cretaceous extensional structures in the SE Tarim Basin. The Late Triassic thrusts include newly formed and revived thrusts. The Qarqan fault was revived during the Late Triassic. Several newly formed smaller Late Triassic thrusts were revealed on the hanging wall or the southern side of the Qarqan fault (Figure 13). The Late Triassic thrust structures and Jurassic–Cretaceous normal faults together in the piedmont of the Altun constitute an integrated set of collision-related structures of the North Qiangtang–Tarim collision, which is one of the most important collisions in the Cimmerides.
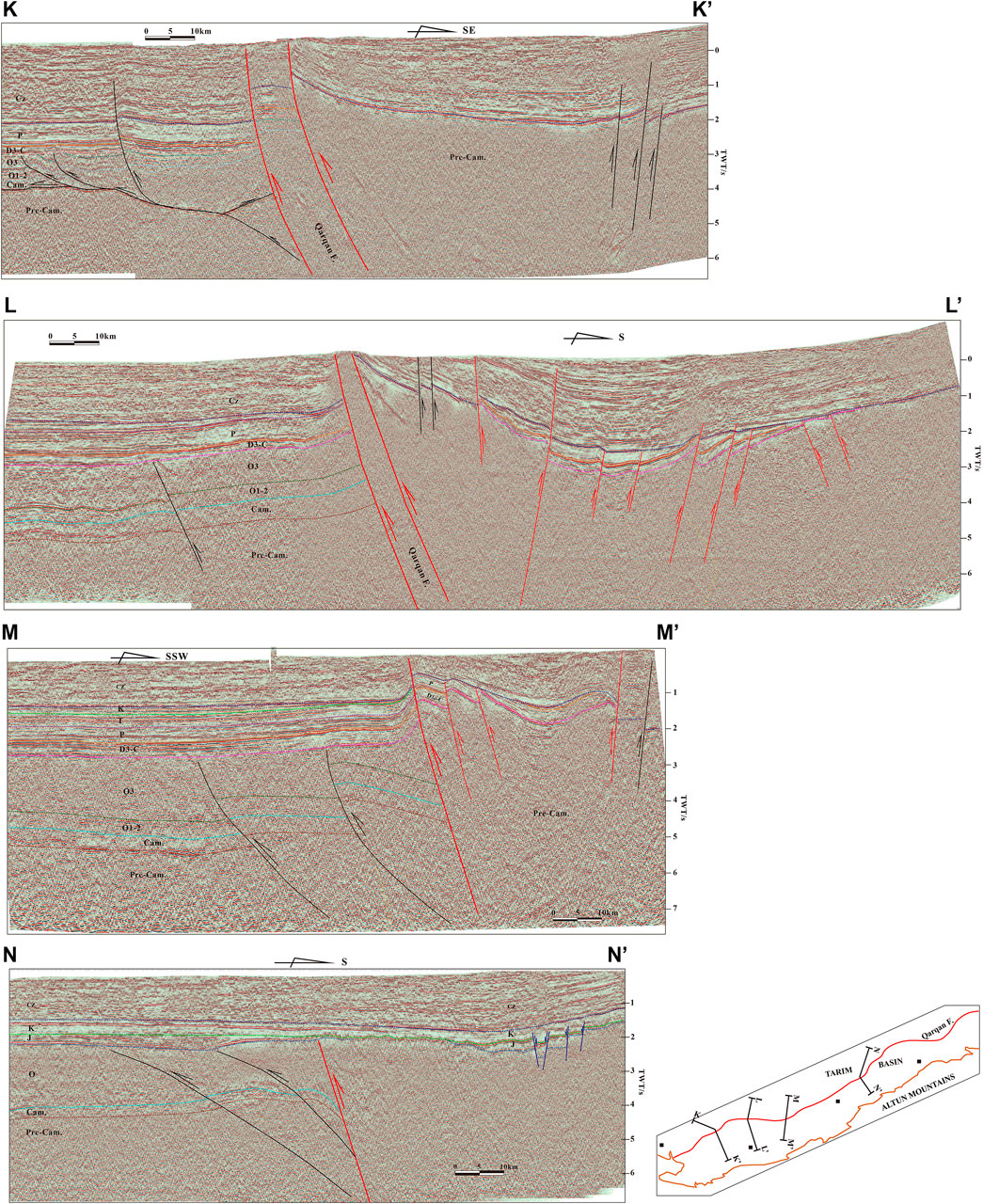
FIGURE 13. K–K′, L–L′, M–M′, and N–N′ 2D seismic sections across Qarqan fault. Red lines represent faults active in Late Triassic (syn-collision thrust structures). Blue lines represent Jurassic–Cretaceous normal faults (post-collision extensional structures). Black lines represent other faults.
The Qarqan fault is a high-angle basement-involved thrust dipping to SE and forming the NW boundary fault of the Tanan Rise. The fault plane distribution is in a wave-like shape. Its overall strike direction is SW–NE, and its breaking length is approximately 1,600 km (Figures 12, 13).
Because the Qarqan Fault is neighboring to the Altun Late Cenozoic strike-slip fault system and their overall trend is basically parallel, many geologists believe that the Qarqan is a branch of this strike-slip system (Cui et al., 2002; Lu et al., 2006). In fact, the Qarqan is not a branch of the Altun Late Cenozoic strike-slip system because they do not match in both deformation time and structural type. The Qarqan Fault is a basement-involved thrust but not a strike-slip fault. It was formed in the Late Ordovician–Early Silurian (Li et al., 2016a; Yang et al., 2016), revived in the Triassic, and partly revived in the Late Cenozoic. The Late Cenozoic faulting only occurred at Qarqan’s SW segment, with no Late Cenozoic reactivation at its other segments.
The Qarqan fault thrusts on a large scale for the second time during the Late Triassic. Together with it are the newly formed Late Triassic smaller thrusts on Qarqan’s hanging wall (Figures 12 and 13). The footwall of the fault developed the Late Ordovician–Early Silurian Tangguzibas fold-thrust belt. After the formation, the fold-thrust belt is relatively stable, and the deformation is weak. It is a well-preserved Early Paleozoic fold-thrust belt (Li et al., 2016a; Yang et al., 2016). A large number of strata are missing on the Qarqan’s hanging wall. The Jurassic–Cretaceous and even the Cenozoic strata often directly unconformably overlie the Precambrian (?) metamorphic rocks. Between the metamorphic rocks and the Jurassic–Cenozoic strata, the Triassic is missing, and the partially developed Permo-Carboniferous strata can only be seen locally. The Permo-Carboniferous strata were involved in the Late Triassic deformation and formed the angular unconformity between the Permo-Carboniferous and the overlying Jurassic–Cenozoic (Figure 13). This unconformity represents the (Late) Triassic tectonic event, and that between the Permo-Carboniferous and the underlying metamorphic rocks represents an Early Paleozoic tectonic event. The existing Permo-Carboniferous is the key to identifying the (Late) Triassic tectonic event.
The Qarqan's NE segment is a basement-involved thrust. The Jurassic–Cretaceous unconformably overlies on the Precambrian (?) metamorphic rocks (hanging wall) and Ordovician (footwall) directly. The Jurassic strata pinch out toward the Qarqan’s top. The Cretaceous, Jurassic, and Cenozoic exhibit similar deformation features. Between them are conformity or parallel unconformity. The fault cuts upward to the bottom of the Jurassic–Cretaceous and then stops. The deformation is mainly seen in the strata below the Jurassic–Cretaceous bottom unconformity. Above this unconformity, the Jurassic–Cenozoic strata were deformed slightly (N-N′ section on Figure 13). Obviously, the fault activity of the Qarqan's NE segment ended before the Jurassic. Its faulting history includes two thrust phases that occurred at the Late Ordovician–Early Silurian and the Late Triassic.
The Qarqan’s SW segment is composed of two to three basement-involved thrusts (K-K′, L-L′, and M-M′ sections on Figure 13). The Permo-Carboniferous strata are not only developed on its footwall but also can be partially seen in the fault zone and on the hanging wall. In the fault zone and on the hanging wall, the Permo-Carboniferous unconformably overlies the metamorphic rocks and underlying the Jurassic–Cenozoic strata (Figures 13, 14). The unconformity between the Permo-Carboniferous and the metamorphic rocks represents the Early Paleozoic collision on the southeastern margin of the Tarim plate (Li et al., 2016a; Yang et al., 2016). The unconformity between the Permo-Carboniferous and the overlying Jurassic–Cenozoic on the hanging wall indicates the Qarqan's reactivation in the Late Triassic. Accompanying with the Qarqan’s reactivation, several Late Triassic thrusts were formed on Qarqan’s hanging wall. On the seismic sections, it is clear that the Permo-Carboniferous strata were involved in this thrust deformation, and the Jurassic–Cretaceous and Cenozoic strata were not involved (Figure 13).
Different from the NE segment, the Qarqan's SW segment does not stop at the bottom of Cenozoic strata (the Jurassic–Cretaceous strata are absent on the top of the Qarqan fault at its SW segment). It cuts through the Cenozoic bottom and upward to the Upper Cenozoic and even near the ground surface. There are also some smaller faults on the Qarqan’s hanging wall that cut upward into the Cenozoic and stopped near the surface (K-K′, L-L′ and M-M′ sections in Figure 13). Evidently, there is a third thrust phase in the Qarqan’s SW segment. It occurred in the Late Cenozoic under the far-field effect of the India–Asia collision (Tapponnier and Molnar, 1977; Sobel and Dumitru, 1997; Li et al., 2016b).
Discussion and Conclusion
Late Triassic Thrusts and Jurassic–Cretaceous Normal Faults Developed in the Southern Tarim Basin
The known Late Triassic thrusts in the southern Tarim Basin distribute in the piedmonts of West Kunlun and Altun Mountains and the Markit Slope in Tarim Basin. The Jurassic–Cretaceous extensional structures are only found in the piedmonts of West Kunlun and Altun Mountains.
The Late Triassic thrusts (including décollements) and related folds developed in the Permian and below strata, indicating that the deformation occurred after the deposition of the Permian strata. In the studied area, almost all of the Triassic systems are missing; the Jurassic–Cretaceous and even the Paleogene strata, which were not involved in the thrust deformation, are directly unconformably overlying the thrusts. These indicate that the deformation ended before the deposition of the Jurassic strata. The existence of the Lower Triassic Wuzunsayi Formation in the Duwa area (Figure 4) and the Jurassic–Cretaceous strata in the piedmonts of the West Kunlun and Altun are significant for accurately timing the deformation. The Wuzunsayi Formation is conformably overlying the Upper Permian strata and unconformably overlaid by the Jurassic–Cretaceous strata. Obviously, the thrusting occurred in the Middle–Late Triassic. Many geologists believe that this thrusting occurred in the Late Triassic (Sobel, 1999; Chen and Wang, 2000; Chen H. L et al., 2009; Chen Y. G. et al., 2018).
The Jurassic–Cretaceous extensional structure is a series of normal faults, forming horst–graben structures that controlled the distribution and deposition process of the Jurassic–Cretaceous strata in the piedmonts of the West Kunlun and Altun. The Jurassic–Cretaceous is the growth-strata of normal faults. In other words, the normal faults are the growth faults of the Jurassic–Cretaceous strata. The normal faulting time is clear and credible.
Late Triassic Thrusts Are Syn-Collision Structures in Response to the North Qiangtang–Tarim Collision
The Late Triassic thrusts in the southern Tarim Basin are distributed in the piedmonts the West Kunlun and Altun. They thrust mainly from the mountains to the basin (Figures 7, 8, 12 and 13). There are also some back-thrusts in the Markit Slope (Figures 10 and 11). These thrusts indicate a nearly N–S maximum (σ1) of the Late Triassic compressional tectonic stress field in the southern Tarim Basin. What tectonic event resulted in this paleo-compressional stress field? The ancient Asian Ocean on the north side of Tarim had been closed, and the collision on the northern margin of the Tarim plate occurred before Late Triassic (Li et al., 2001a; Li et al., 2001b; Li et al., 2002; Xiao et al., 2002; Li et al., 2005; Xiao et al., 2008; Li et al., 2009; Xiao et al., 2009; Li et al., 2010; Zhao et al., 2012; Li et al., 2013; Li et al., 2020; Wen et al., 2020). The Altaids have no relation to the Late Triassic thrusts in the southern Tarim Basin. The related tectonic event must be on the Tarim Basin's southern margin. The thrusting time (Late Triassic) is basically the same as the onset time of the North Qiangtang–Tarim collision (Jia et al., 2001; Yang et al., 2002; Chen et al., 2009). This collision is fairly inferred as the related tectonic event of the Late Triassic thrusts in the southern Tarim Basin. In other words, these Late Triassic thrusts are the syn-collision structures in response to the North Qiangtang–Tarim collision.
Jurassic–Cretaceous Extensional Structures Are the Post-Collision Structure of the North Qiangtang–Tarim Collision
The Jurassic–Cretaceous extensional structures in the southern Tarim Basin are the normal faults and the horst–graben structures controlled by them (Figures 6A, 7, 8 and 13). They were formed immediately after the Late Triassic syn-collision structures and contemporary with the post-collision relaxation stage of the North Qiangtang–Tarim collision (Jia et al., 2001; Yang et al., 2002; Chen et al., 2009). Thus, they should be the post-collision structures of the North Qiangtang–Tarim collision. The collision between North Qiangtang and Tarim blocks onsets at the beginning of the Late Triassic lasted to the end of the Late Triassic and then ceased. The tectonic stage evolved from the Late Triassic syn-collision stage to the Jurassic–Cretaceous post-collision stage and the structural deformation from the Late Triassic thrusting to Jurassic–Cretaceous normal faulting.
Implications of the Mesozoic Collision-Related Structures in the Southern Tarim Basin to the Closing Process of the Paleo-Tethys
The Late Triassic syn-collision structures and Jurassic–Cretaceous post-collision structures in the southern Tarim Basin constitute a complete set of collision-related structures in response to the North Qiangtang–Tarim collision. This collision is an important tectonic event in the Paleo-Tethys closing history.
The closing history of the Tethyan oceanic basins is very complex, resulting in numerous sutures and lasting for a very long time. This complex closing process is also a complex collision process that resulted in the Tethysides, a super orogenic complex between the Laurasia and Gondwana (ȘengÖr, 1987; ȘengÖr et al., 1988; ȘengÖr, 1990; Hsü et al., 1995; ȘengÖr and Nata’in, 1996; Wu et al., 2020; Zeng et al., 2020). The North Qiangtang–Tarim collision was seen as the final collision of the Paleo-Tethysides (Jia et al., 2001; Yang et al., 2002; Chen et al., 2009).
The Tethys is usually divided into Carboniferous–Jurassic Paleo-Tethys and Meso-Cenozoic Neo-Tethys (StÖcklin, 1968; ȘengÖr et al., 1988). Some geologists think that the ancient oceanic basins before the Carboniferous in the Tethys domain and the suture zone formed by their closure do not belong to the category of Tethys (ȘengÖr et al., 1988). Some other geologists named them the Proto-Tethys (Pan, 1989; Zhang et al., 2015; Wu et al., 2020). There are also some geologists who believed that the Tethys came into being during Cambrian or late Precambrian but not limited to Carboniferous–Cenozoic (Tozer, 1989; Scotese, 2002).
This is because many ancient oceanic basins represented by the ancient sutures in the Paleo-Tethys domain were evolved continuously from the Early Paleozoic ancient oceanic basins (Metcalfe, 1988; Tozer, 1989; Yang et al., 1999; Scotese, 2002; Li et al., 2008; Wu, 2013; Zhang et al., 2015) rather than Permo-Carboniferous newly formed oceanic basins. Therefore, we see these pre-Carboniferous oceanic basins as the components of the Paleo-Tethys and the sutures formed by their closure as the components of the Paleo-Tethysides.
Almost all the blocks in the Paleo-Tethys domain are Gondwana-land fragments. They collided with each other due to the closure of the oceanic basins between them and resulted in the Cimmeride orogenic system or Cimmerian continent (ȘengÖr, 1984; ȘengÖr et al., 1988).
In the Tibet Plateau, the Qiangtang terrane is the main part of the Cimmerian continent. It is separated into the North and South Qiangtang by the Longmucuo-Shuanghu Suture (Li, 1987; Metcalfe, 1994; Li et al., 1997; Deng et al., 2000; Kapp et al., 2003). According to the analysis on the chronology of blueschist (Bao et al., 1999; Deng et al., 2000; Kapp et al., 2003; Lu et al., 2006; Zhai et al., 2009; Zhu et al., 2010), subduction–collision-related magmatic activities (Kapp et al., 2003; Zhang et al., 2014; Li et al., 2015; Gao et al., 2019), radiolarian fauna (Li et al., 1997; Zhu et al., 2006), and sedimentary tectonic background (Li et al., 2000), the Longmutuo-Shuanghu ancient oceanic basin was not closed yet in the Late Triassic, and the paleo-oceanic crust is still subducting to the north and forming a volcanic island arc on the southern margin of the North Qiangtang. In other words, the North and South Qiangtang were still separated from the Longmutuo-Shuanghu oceanic basin and had not been united as one terrane in the Late Triassic. Therefore, in the Late Triassic, it was the North Qiangtang that collided with the southern margin of the Tarim plate, and the Paleo-Tethys had not finally closed yet (Figure 15).
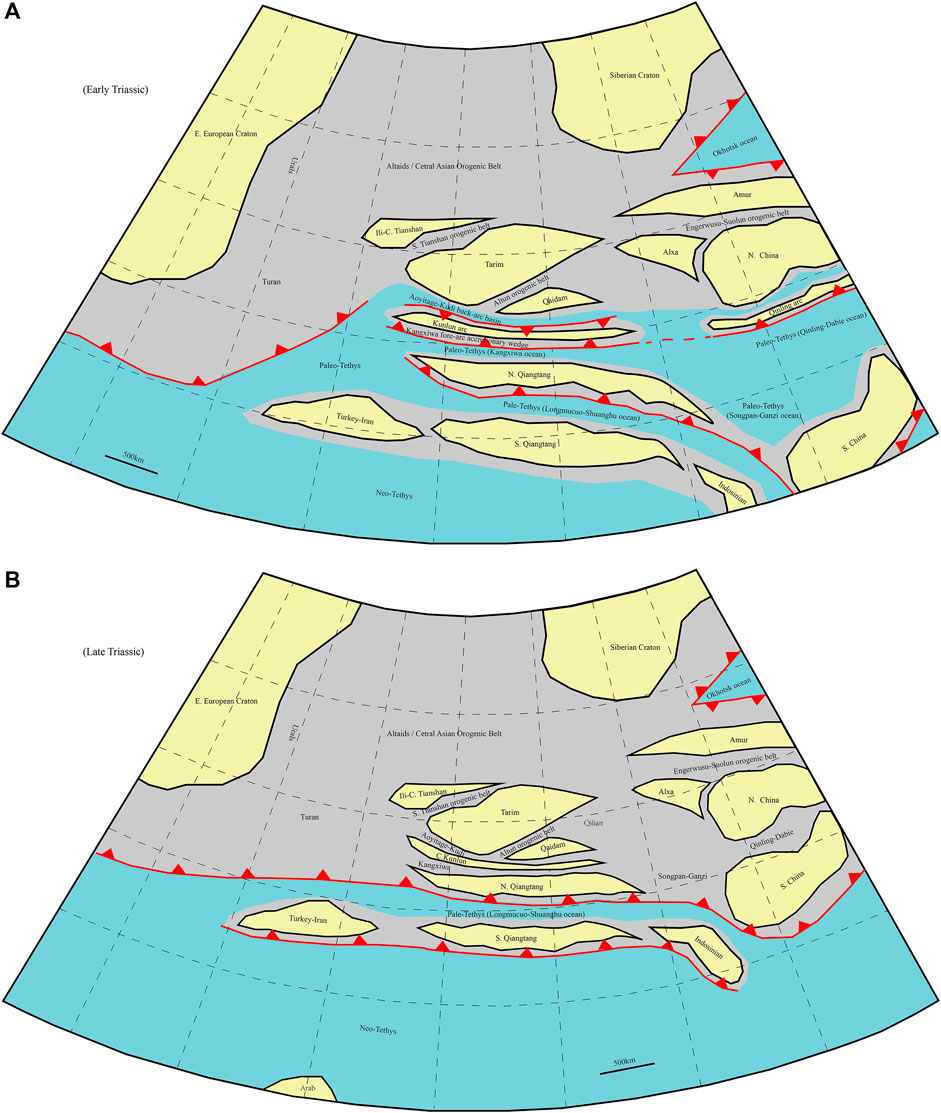
FIGURE 15. A sketch showing tectono-paleogeographic framework of Tarim Basin and its adjacent units in Early (A) and Late (B) Triassic.
Just before the collision, the southern margin of the Tarim block was an active continental margin, including the Kangxiwa oceanic crust subduction zone, Central Kunlun Arc, and Aoyitage-Kudi back-arc basin in the Early Triassic (Figure 15). This Late Paleozoic active margin system was formed from the Early Paleozoic West Kunlun accretionary complex (Xiao et al., 2000; Xiao et al., 2003; Xiao et al., 2005). Just for this reason, there are both Early and Late Paleozoic oceanic crust fragments (ophiolites) in the Aoyitage-Kudi Suture (Yang et al., 1999; He et al., 2001; Xiao et al., 2002; Xiao et al., 2005). It is the Central Kunlun Arc that directly collided with the North Qiangtang block firstly in the Late Triassic. This collision immediately led to the closure of the Aoyitage-Kudi back-arc basin and the arc–continent collision between the Central Kunlun Arc and Tarim continent. To be exact, it is this arc–continent collision that led to the formation of the Mesozoic collision-related structures in the southern Tarim Basin.
Following the North Qiangtang–Tarim collision in the Late Triassic, the Longmutuo-Shuanghu oceanic basin between the North and South Qiangtang closed in the Jurassic. This is possibly the final closing of the Paleo-Tethys and then is the closing history of the Neo-Tethys. There is not a clear demarcation between the Paleo- and Neo-Tethys both in time and space. The evolution history of the Tethys is the process of numerous Gondwana-land fragments moving northward and colliding on the southern margin of the Eurasian plate one by one from Early Paleozoic to Cenozoic. The final collision between the Gondwana and Eurasia in the Late Cenozoic led to the formation of the Alps–Zagros–Himalaya Mountains.
Data Availability Statement
The original contributions presented in the study are included in the article/Supplementary Material; further inquiries can be directed to the corresponding author.
Author Contributions
YL contributed to conception and supervised the project. Y-JL is the lead author, with major contributions from LW, Y-LL, and CL. LW contributed in writing the manuscript draft. X-ZY, LZ, BW and CC provided and drafted Figures 2, 3, 5, 7, 8, 10, 11 and 13. All authors equally revised the manuscript and approved the manuscript.
Funding
This study is funded by the National Science and Technology Major Project (2017ZX05008-004, 2016ZX05004-004), the PetroChina Science and Technology Major Project (kt2018E-1802), and the Science and Technology Project of the Tarim Oilfield Company, PetroChina (no. 041019120072). Gratitudes are due to Tarim Oilfield Company, PetroChina, for providing us the precious seismic data.
Conflict of Interest
Authors YL, XY, LZ, BW, and CC were employed by the company Tarim Oil-Field Company. Authors LW and Y-LL were employed by the company Oil and Gas Survey, China Geological Survey
The remaining authors declare that the research was conducted in the absence of any commercial or financial relationships that could be construed as a potential conflict of interest.
Publisher’s Note
All claims expressed in this article are solely those of the authors and do not necessarily represent those of their affiliated organizations or those of the publisher, the editors, and the reviewers. Any product that may be evaluated in this article, or claim that may be made by its manufacturer, is not guaranteed or endorsed by the publisher.
Acknowledgments
We are grateful to Professors Wang Daoxuan, Wang Zhenyu, Luo Shunshe, and Drs. Huang Shaoying, Yang Zhilin, and Zhao Tianyu for their beneficial help. We would like to express our great appreciation to the reviewers and editors for their constructive comments and suggestions, which significantly improved the quality of our article.
References
Bao, P. S., Xiao, X. C., and Wang, J. (1999). The Blueschist Belt in the Shuanghu Region, Central-Northern Tibet and its Tectonic Implications. Acta Geologica Sinica 73 (4), 302–314. (in Chinese with English abstract).
Bullen, M. E., Burbank, D. W., Garver, J. I., and Abdrakhmatov, K. Y. (2001). Late Cenozoic Tectonic Evolution of the Northwestern Tien Shan: New Age Estimates for the Initiation of Mountain Building. DSR Bull. 113 (12), 1544–1559. doi:10.1130/0016-7606(2001)113<1544:lcteot>2.0.co;2
Cao, K., Wang, G.-C., Bernet, M., van der Beek, P., and Zhang, K.-X. (2015). Exhumation History of the West Kunlun Mountains, Northwestern Tibet: Evidence for a Long-Lived, Rejuvenated Orogen. Earth Planet. Sci. Lett. 432, 391–403. doi:10.1016/j.epsl.2015.10.033
Chang, C. F., Chen, N. S., Coward, M. P., Deng, W. M., Dewey, J. F., Gansser, A., et al. (1986). Preliminary Conclusions of the Royal Society and Academia Sinica 1985 Heotraverse of Tibet. Nature 323 (6088), 501–507.
Chen, F., and Wang, X. (2000). Prototype Analysis of Early-Middle Jurassic basin in Northwestern China. Earth Sci. Front. 7 (4), 459–465.
Chen, H. L., Li, K., Li, Y., Wu, H. X., Cheng, X. G., Zeng, C. M., et al. (2018). The Segmentation Deformation of the Thrust belt in Front of Western Kunlun, Western China, and its Controlling Factors. Acta Petrologica Sinica 34 (7), 1933–1942.
Chen, H. L., Luo, J. C., Guo, Q. Y., Liao, L., Xiao, Z. Y., Cheng, X. G., et al. (2009). Deformation History and Tectonic Evolution of Southeastern Tarim Basin in Mesozoic and Cenozoic. Geotectonica et Metallogenia 33 (1), 38–45. (in Chinese with English abstract).
Chen, Y. G., Wu, H. X., Zhang, L., Cheng, X. G., Chen, C., Zhang, Y. Q., et al. (2018). Characteristics of the Late Triassic Paleostructure in the Mountain Front Region of Western Kunlun and its Control of Jurassic-Cretaceous Deposition. Chin. J. Geology. 53 (4), 1405–1418.
Cheng, X. G., Chen, H. L., and Shi, J. (2012a). Distribution Characteristics and Controlling Factors of Jurassic-Cretaceous in the Front of West Kunlun Mountains. Earth Sci. 37 (4), 635–644.
Cheng, X. G., Huang, Z. B., Chen, H. L., Du, Z. L., Li, K., and Shi, J. (2012b). Fault Characteristics and Division of Tectonic Units of the Thrust belt in the Front of the West Kunlun Mountains. Acta Petrologica Sinica 28 (8), 2591–2601.
Cowgill, E. (2010). Cenozoic Right-Slip Faulting along the Eastern Margin of the Pamir Salient, Northwestern China. Geol. Soc. America Bull. 122 (1), 145–161. doi:10.1130/b26520.1
Cui, J., Zhang, X., and Li, P. (2002). The Altun Fault: Its Geometry, Nature and Mode of Growth. Acta Geosicientia Sinica 23 (6), 509–516.
Deng, X. G., Ding, L., and Liu, X. H. (2000). Blue Amphibole Schist and its 40 Ar/ 39 Ar Chronology in the Gangmari Area, Central Qiangtang, Qinghai-Tibet Plateau. Chin. Sci. Bull. 45 (21), 2322–2326. (in Chinese). doi:10.1007/bf02884983
Gao, X., Li, J. C., Yuan, G. L., Wang, G. H., Liang, X., Zheng, Y. L., et al. (2019). Middle-Late Triassic Magmatic Records for the Accretionary Processes of South Qiangtang Accretionary Terrane: The Mafic Dykes in Mayigangri-Jiaomuri Area, North Tibet. Acta Petrologica Sinica 35 (3), 760–774. (in Chinese with English abstract).
Guan, S. W., Li, B. L., He, D. f., Wang, X., John, S., and Lei, G. l. (2007). Late Cenozoic Active Fold-And-Thrust Belts in the Southern and Northern Flanks of Tianshan. Acta Geologica Sinica 81 (6), 725–744. (in Chinese with English abstract).
He, Z., Luo, C., Gong, M., Xiao, Y., Xu, G., Long, S., et al. (2001). Multicycle basin and Complex Petroleum System in Tarim. China. Wuhan: China University of Geosciences Press, 8–13.
Hsü, K. J., and Bernoulli, D. (1978). Genesis of the Tethys and Mediterranean. Init. Rep. DSDP 42 (1), 143–949.
Hsü, K. J., Pan, G., ȘengÖr, A. M. C., Briegel, U., Chen, H., Chen, C., et al. (1995). Tectonic Evolution of the Tibetan Plateau: a Working Hypothesis Based on the Archipelago Model of Orogensis. Int. Geology. Rev. 37, 473–508.
Huang, S. Y., Zhang, W., Li, Y. J., Zhou, S. Y., Li, H. H., Zheng, D. M., et al. (2020). Timing the Formation of the Lunnan Paleo-Uplift in Tarim Basin, NW China. Chin. J. Geology. 55 (4), 1089–1098.
Huang, Z., Zhong, D., and Du, Z. (2012). 1: 100, 000 Geological Map and Manual of Yecheng Sag and its Surroundings.
Jia, C. (1997). Tectonic Characteristics and Petroleum, Tarim Basin. China. Beijing: Petroleum Industry Press, 1–295.
Jia, C., Wei, G., Xiao, A., Jia, D., and Chen, H. (2004b). The Meso-Cenozoic Tectonics and Petroleum in the Tarim Basin. Beijing: Petroleum Industry Press, 1–167.
Jia, C., Yang, S., Chen, H., and Wei, G. (2001). Structural Geology and Natural Gas of the Group of Basins in the Northern Margin of Tethyes. Petroleum Industry Press, 1–162.
Jia, C., Yao, H., Gao, J., Zhou, D., and Wei, G. (1992). “Stratigraphic System of the Tarim Basin,” in Symposium of the oil and gas exploration in Tarim Basin, Urumqi. Editors X. Tong, and D. Liang (Xinjiang Technology and Medical Publishing House), 34–63.
Jia, C., Zhang, S., and Wu, S. (2004a). Stratigraphy of the Tarim Basin and Adjacent Areas. Beijing: Science Press, 1–547.
Kapp, P., Yin, A., and Manning, C. E. (2003). Tectonic Evolution of the Early Mesozoic Blueschist-Bearing Qiangtang Metamorphic Belt, Central Tibet. Tectonics 22 (4), 1043. doi:10.1029/2002tc001383
Li, C., Dong, Y. S., and Zhai, Q. G. (2008). Discovery of Eopaleozoic Ophiolite in the Qiangtang of Tibet Plateau: Evidence from SHRIMP U-Pb Dating and its Tectonic Implications. Acta Petrologica Sinica 24 (1), 31–36. (in Chinese with English abstract).
Li, C. (1987). The Longmucuo-Shuanghu-Lancangjiang Plate Suture and the North Boundary of Distribution of Gondwana Facies Permo-Carboniferous System in Northern Xizang, China. J. Changchun Coll. Geology. 17 (2), 155–166. (in Chinese with English abstract).
Li, H. H., Li, Y. J., Ma, D. B., Wen, L., Li, C., Zhao, Y., et al. (2020). Syn- and post-collision Structures of the South Tianshan Orogenic belt: Results of the Seismic Interpretation in the Northern Tarim Basin along the South Tianshan. Chin. J. Geology. 55 (2), 322–338.
Li, H., Zhou, D., Feng, Q., and Zhou, D. (1992). “The Establishment of Seimic Stratigraphic Sequence in Eastern Tarim Basin,” in Symposium of the oil and gas exploration in the Tarim Basin, Urumqi. Editors X. Tong, and D. Liang (Xinjiang Technology and Medical Pulishing House), 142–157.
Li, J. C., Zhao, Z. B., Zheng, Y. L., Yuan, G. L., Liang, X., Wang, G. H., et al. (2015). The Magmatite Evidences in Southern Qiangtang for Paleo-Tethys Ocean Subducting Collision: Gangtang-Co Granites in Rongma, Tibet. Acta Petrologica Sinica 31 (7), 2078–2088.
Li, P.-C., Liu, Z.-H., Li, S.-C., Zhao, Q.-Y., Shi, Q., Li, C.-H., et al. (2019). Late Paleocene-Early Eocene Granitoids in the Jiamusi Massif, NE China: Zircon U-Pb Ages, Geochemistry, and Tectonic Implications. Int. Geology. Rev. 61 (1), 1–16. doi:10.1080/00206814.2018.1440646
Li, Y.-J., Wen, L., Li, H.-L., Peng, G.-X., Qiu, B., Zheng, D.-M., et al. (2016a). The Madong Early Paleozoic Fold-Thrust belt in Southern Tarim Basin. J. Asian Earth Sci. 115, 247–256. doi:10.1016/j.jseaes.2015.10.007
Li, Y.-J., Wen, L., Yang, H.-J., Zhang, G.-Y., Shi, J., Peng, G.-X., et al. (2015). New Discovery and Geological Significance of Late Silurian-Carboniferous Extensional Structures in Tarim Basin. J. Asian Earth Sci. 98, 304–319. doi:10.1016/j.jseaes.2014.11.020
Li, Y.-J., Zhang, Q., Zhang, G.-Y., Tian, Z.-J., Peng, G.-X., Qiu, B., et al. (2016b). Cenozoic Faults and Faulting Phases in the Western Tarim Basin (NW China): Effects of the Collisions on the Southern Margin of the Eurasian Plate. J. Asian Earth Sci. 132, 40–57. doi:10.1016/j.jseaes.2016.09.018
Li, Y.-J., Zhao, Y., Sun, L.-D., Song, W.-J., Zheng, D.-M., Liu, Y.-L., et al. (20132013). Meso-Cenozoic Extensional Structures in the Northern Tarim Basin, NW China. Int. J. Earth Sci. (Geol Rundsch) 102, 1029–1043. doi:10.1007/s00531-012-0847-3
Li, Y., Chen, C., Feng, X., Zhang, L., Zeng, C., and Fan, Q. (2016c). New Discovery of Nanhuaian Rift System in Southwestern Tarim basin and its Geological Significance. Acta Petrologica Sinica 32 (3), 825–832.
Li, Y. J., Li, Y. P., Wu, H. R., Hu, J. F., Su, Y. S., and Huang, Z. B. (2001a). Radiolarian Fossils Discovered from the Chert Gravels of Triassic Conglomerate at the NW Margin of Tarim Basin, NW China, and Discussions. Sciencia Geologica Sinica 10 (4), 275–282.
Li, Y. J., Mai, G. R., Peng, G. X., Zeng, D. M., Luo, J. C., and Huang, Z. B. (2000). A Preliminary Study on the Tectonic Setting of Upper Triassic Flysch at Chasang, North Tibet. Acta Petrologica Sinica 16 (3), 443–448.
Li, Y. J., Song, W. J., Mai, G. R., Zhou, L. X., Hu, J. F., and Shang, X. L. (2001b). Kuqa , Northern Tarim Foreland Basins and the Doubling between Them and South Tianshan Orogen. Xinjiang Pet. Geology. 22 (5), 376–381.
Li, Y. J., Sun, L. D., Wu, H. R., Zhang, G. Y., Wang, G. L., and Huang, Z. B. (2005). Permo-Carboniferous Radiolarians from the Wupata’erkan Group, Western South Tianshan, Xinjiang, China. Acta Geologica Sinica 79 (1), 16–23.
Li, Y. J., Wang, Z. M., Wu, H. R., Huang, Z. B., Tan, Z. J., and Luo, J. C. (2002). Discovery of Radiolarian Fossils from the Aiktik Group at the Western End of the South Tianshan Mountains of China and its Implications. Acta Geologica Sinica 76 (2), 146–154.
Li, Y. J., Wu, H. R., Li, H. S., and Sun, D. L. (1997). Discovery of Radiolarians in the Amugang and Chasang Groups and Lugu Formation in Northern Tibet and Some Related Geological Problems. Geol. Rev. 43 (3), 250–256.
Li, Y. J., Yang, H. J., Zhang, G. Y., Zheng, D. M., Liu, Y. X., Zhao, Y., et al. (2012). Redivision of the Tectonic Units of Tabei Rise in Tarim Basin, NW China. Acta Petrologica Sinica 28 (8), 2466–1478.
Li, Y. J., Yang, H. J., Zhao, Y., Luo, J. C., Zheng, D. M., and Liu, Y. L. (2009). Tectonic Framework and Evolution of South Tianshan, NW China. Geotectonic et Metallogenia 33 (1), 96–106.
Li, Y. J., Zhang, H. A., Qian, Y. X., Zhang, Q. R., Zhao, Y., and Liu, Y. L. (2010). The Collision Time of South Tianshan Orogen, NW China. Chin. J. Geology. 45 (1), 57–65.
Lu, H., David, G. H., Jia, D., Cai, D., Wu, S., Chen, C., et al. (1994). Rejuvenation of the Kuqa Foreland basin, Northern Flank of the Tarim basin, Northwest China. Int. Goel. Rev. 36, 1151–1158.
Lu, H., Wang, S., Luo, J., et al. (2006). Fault Systems and Their Tectonic Evolution in the Eastern Tarim Basin. Oil Gas Geology. 27 (4), 433–441.
Mattern, F., and Schneider, W. (2000). Suturing of the Proto- and Paleo-Tethys Oceans in the Western Kunlun (Xinjiang, China). J. Asian Earth Sci. 18 (6), 637–650. doi:10.1016/s1367-9120(00)00011-0
Metcalfe, I. (1994). Gondwanaland Origin, Dispersion, and Accretion of East and Southeast Asian Continental Terranes. J. South Am. Earth Sci. 7 (3-4), 333–347. doi:10.1016/0895-9811(94)90019-1
Metcalfe, I. (1988). “Origin and Assembly of South-East Asian continental Terranes,” in Gondwana and Tethys. Editors M. G. Audley-Charles, and A. Hallam, (Geological Society Special Publication), 37, 119–181. doi:10.1144/gsl.sp.1988.037.01.08
Molnar, P., and Tapponnier, P. (1975). Cenozoic Tectonics of Asia: Effects of a Continental Collision: Features of Recent continental Tectonics in Asia Can Be Interpreted as Results of the India-Eurasia Collision. Science 189 (4201), 419–426. doi:10.1126/science.189.4201.419
Pan, Y. S. (1989). A Preliminary Study on the Regionalization of the Structures in the Kunlun Mountains Region. J. od Nat. Resour. 4 (3), 196–203.
Scotese, C. R. (2002). Paleomap Website. Available at: http://www.scotese.com, chris@scotese.com.
ȘengÖr, A. M. C., Altmer, D., Cin, A., UstaÖmer, T., and Hsü, K. J. (1988). “Origin and Assembly of the Tethyside Orogenic Collage at the Expense of Gondwana Land,” in Gondwana and Tethys. Editors M. G. Audley-Charles, and A. Hallam, (Geological Society Special Publication), 37, 119–181.
ȘengÖr, A. M. C., Nata'in, B. A., and Burtman, U. S. (1993). Evolution of the Altaid Tectonic Collage and Paleozoic Crustal Growth in Eurasia. Nature 364, 209–304.
ȘengÖr, A. M. C., and Nata'in, B. A. (1996). “Paleotectonics of Asia: Fragments of a Synthesis,” in The Tectonic Evolution of Asia. Editors A. Yin, and T. M. Harrison (Cambridge: Cambridge University Press), 486–640.
ȘengÖr, A. M. C. (1990). Plate Tectonics and Orogenic Research after 25 years: a Tethyan Perspective. Eath Sci. Rev. 27, 1–201.
ȘengÖr, A. M. C. (1987). Tectonics of the Tethysides: Orogenic Collage Development in a Collisional Setting. Ann. Rev. Earth Planet. Sci. 15, 213–244.
ȘengÖr, A. M. C. (1984). The Cimmeride Orogenic System and the Tectonics of Eurasia. Geological Society of America Special Paper195, 84p.
ȘengÖr, A. M. C. (1992). The Paleo-Tethyan Suture: A Line of Demarcation between Two Fundamentally Different Architectural Styles in the Structure of Asia. Isl. Arc 1 (1), 78–91.
Sobel, E. R. (1999). Basin Analysis of the Jurassic-Lower Cretaceous Southwest Tarim basin, Northwest China. Geol. Soc. America Bull. 111 (5), 709–724. doi:10.1130/0016-7606(1999)111<0709:baotjl>2.3.co;2
Sobel, E. R., and Dumitru, T. A. (1997). Thrusting and Exhumation Around the Margins of the Western Tarim Basin during the India-Asia Collision. J. Geophys. Res. 102, 1043–1063. doi:10.1029/96jb03267
Sobel, E. R., Schoenbohm, L. M., Chen, J., et al. (2011). Late Miocene-Pliocene Deceleration of Dextral Slip between Pamir and Tarim: Implications for Pamir Orogenesis. Earth Planet. Sci. Lett. 304 (3-4), 369–378. doi:10.1016/j.epsl.2011.02.012
StÖcklin, J. (1968). Structural History and Tectonics of Iran: A Review. AAPG Bull. 52 (7), 1229–1258.
Sun, L. (2004). Sedimentary Facies and Exploration of Petroleum of the Early Cretaceous in Kuqa Depression and Southwest Depression in Tarim Basin. J. Palaeogeogr. 6 (2), 252–260.
Tapponnier, P., and Molnar, P. (1977). Active Faulting and Tectonics in China. J. Geophys. Res. 82 (20), 2905–2930. doi:10.1029/jb082i020p02905
Tozer, E. T. (1989). Tethys, Thetis, Thethys, or Thetys? what, where, and when Was it. Geol 17, 882–884. doi:10.1130/0091-7613(1989)017<0882:tttotw>2.3.co;2
Wang, C. (2014). The Deformation Controlling Factors of Fold-And-Thrust belt in SW Tarim Basin: Physical Analogue Modeling Study (PhD Thesis). Zhejiang: Zhejiang University, 1–97.
Wang, G. L., Li, Y. J., Sun, J. H., Huang, Z. B., Zhao, Y., and Liu, Y. L. (2009). Structural Deformation Characteristics of Keping Thrust belt, NW Tarim. Chin. J. Geology. 44 (1), 50–62.
Wang, X., Jia, C. Z., and Yang, S. F. (2002). Geometry and Kinematics of the Kuqa Fold-And -thrust belt in the Southern Tianshan. Chin. Jornal Geology. 37 (3), 372–384.
Wen, L., Li, C., Li, H. H., Liu, Y. L., Li, Y. J., Zhao, Y., et al. (2020). The Collision‐related Structures Revealed in the Northern Tarim Basin and Their Geological Significance. Geol. J. 55, 3054–3069. doi:10.1002/gj.3561
Windley, B. F., Alexeiev, D., Xiao, W., Kröner, A., and Badarch, C. (2006). Tectonic Models for Accretion of the Central Asian Orogenic Belt. J. Geol. Soc. 164 (12), 31–47.
Wu, C., Shang, X., and Chen, J. (2004). Tectonic Features and Potentials of the Sugaite Structural belt in West Xinjiang. Chin. J. Geology. 39 (4), 571–579.
Wu, F. Y., Wan, B., Zhao, L., Xiao, W. J., and Zhu, R. X. (2020). Tethyan Geodynamics. Acta Petrologica Sinica 36 (6), 1627–1674.
Wu, Y. W. (2013). The Evolution Record of Longmuco-Shuanghu-Lancang Ocean: Cambrian-Permian Ophiolites (PhD Thesis). Changchun: Jilin University.
Xiao, W. J., Hou, Q. L., Li, J. L., Windley, B. F., Hao, J., Fang, A. M., et al. (2000). Tectonic Facies and the Archipelago-Accretion Process of the West Kunlun, China. Sci. China (Series D: Earth Sciences) 43 (Suppl. p), 22–28. doi:10.1007/bf02911939
Xiao, W. J., Windley, B. F., Chen, H. L., Zhang, G. C., and Li, J. L. (2002). Carboniferous-Triassic Subduction and Accretion in the Western Kunlun, China: Implications for the Collisional and Accretionary Tectonics of the Northern Tibetan Plateau. Geol 30 (4), 295–298. doi:10.1130/0091-7613(2002)030<0295:ctsaai>2.0.co;2
Xiao, W. J., Windley, B. F., Huang, B. C., Han, C. M., Yuan, C., Chen, H. L., et al. (2009). End-Permian to Mid-triassic Termination of the Accretionary Processes of the Southern Altaids: Implications for the Geodynamic Evolution, Phanerozoic continental Growth, and Metallogeny of Central Asia. Int. J. Earth Sci. (Geol Rundsch) 98, 1189–1217. doi:10.1007/s00531-008-0407-z
Xiao, W. J., Windley, B. F., Liu, D. Y., Jian, P., Liu, C. Z., Yuan, C., et al. (2005). Accretionary Tectonics of the Western Kunlun Orogen, China: A Paleozoic-Early Mesozoic, Long‐Lived Active Continental Margin with Implications for the Growth of Southern Eurasia. J. Geology. 113 (6), 687–705. doi:10.1086/449326
Xiao, W. J., Zhou, H., Windley, B. F., Yuan, C., Chen, H. L., Zhang, G. C., et al. (2003). Structures and Evolution of the Multiple Accretionay Complexs, Western Kunlun Orogenic belt (China). Xinjiang Geology. 21 (1), 31–36.
Xiao, W., Pirajno, F., and Seltmann, R. (20082008). Geodynamics and Metallogeny of the Altaid Orogen. J. Asian Earth Sci. 32, 77–81. doi:10.1016/j.jseaes.2007.10.003
Xie, H., Wang, C., and Wang, Z. (2012). The Effect of Spatial Distribution of Basement Décollement on Deformation in a Fold and Thrust Belt: an Analogue Modeling Approach an Example of West Kunlun Fold-And-Thrust Belt. Geol. J. China Universities 18 (4), 701–710.
Xu, X., Cai, Z., and He, B. (2016). Thrust System and its Evolution in Eastern Part of the basin-range junction belt between the West Kunlun and Tarim Basin. Acta Petrologica Sinica 32 (12), 3743–3752.
Yang, H. J., Li, Y. J., Feng, X. J., Zheng, M., Zhang, C. L., and Zhao, Y. (2007). Analysis on Thrustings of the Mazhatage Structural belt in the Tarim Basin. Chin. J. Geology. 42 (3), 506–517.
Yang, H. J., Li, Y. J., Li, Y., Chen, C., Zeng, C. M., Wen, L., et al. (2016). Madong Early Paleozoic Fold-Thrust belt in Southern Tarim Basin. Acata Petrologica Sinica 32 (3), 815–824.
Yang, H. J., Li, Y. J., Shi, J., Xiao, A. C., Huang, S. Y., Wu, G. Y., et al. (2010). Tectonic Characteristics of the Late Cenozoic South Tianshan Fold-Thrust Belts. Quat. Sci. 30 (5), 1030–1043.
Yang, H. J., Li, Y. J., Zeng, C. M., Feng, X. J., Chen, C., Zhang, L., et al. (2015). Niaoshan-Gudongshan Structural Joint in Western Tarim Basin and its Formation and Evolution. Chin. J. Geology. 50 (4), 1023–1043.
Yang, K. (1994). The Formation and Evolution of the Western Kunlun continental Margin. Geologicl Rev. 40 (1), 9–18.
Yang, S., Chen, H., Dong, C., Shen, X., Qi, D., Jia, C., et al. (1999). The Characteristics of Kudi Ophiolite Suite and its Tectonic Setting. Sciencia Geologica Sinica 34 (3), 281–288.
Yang, S., Jia, C., Chen, H., Wei, G., Cheng, X., Jia, D., et al. (2002). Tectonic Evolution of Tethyan Tectonic Field, Formation of Northern Margin basin and Explorative Perspective of Natural Gas in Tarim Basin. Chin. Sci. Bull. 47 (Suppl. p), 34–41. doi:10.1007/bf02902816
Yin, A., Nie, S., Craig, P., Harrison, T. M., Ryerson, F. J., Xianglin, Q., et al. (1998). Late Cenozoic Tectonic Evolution of the Southern Chinese Tian Shan. Tectonics 17 (1), 1–27. doi:10.1029/97tc03140
Zeng, Q., Wang, B., Xi, L., Mao, G., Liu, H., and Liu, G. (2020). Suture Zones in Tibetan and Tethys Evolution. Earth Sci. 45 (8), 2735–2763.
Zhai, Q. G., Li, C., Wang, J., Chen, W., Zhang, Y., Zhang, J. X., et al. (2009). Petrology, Mineralogy and 40 Ar/ 39 Ar Chronology for Rongma Blue‐schist from Central Qiangtang, Northern TibetSubduction, Accretion and Closure of Proto-Tethyan Ocean: Early Paleozoic Accretion / Collision Orogeny in the Altun-Qilian -North Qaidam Orogenic System. Acta Petrologica SinicaActa Petrologica Sinica 2531 (912), 22813531–22883554.
Zhang, P. Z., Deng, Q. D., and Yang, X. P. (1996). Late Cenozoic Tectonic Deformation and Mechanism along the Tianshan Mountain, Northwestern China. Earthquake Reserch in China 12 (2), 127–140. ( in Chinese with English abstract).
Zhang, S., Huang, Z., and Zhu, H. (2004). Phanerozoic Strata in Cover Area of Tarim Basin. Xinjiang. Beijing: Petroleum Industry Press, 151–189.
Zhang, X. Z., Dong, Y. S., Li, C., Deng, M. R., Zhang, L., and Xu, W. (2014). Tectonic Setting and Petrogenesis Mechanism of Late Triassic Magmatism in Central Qiangtang, Tibetan Plateau: Take the Xiangtaohu Pluton in the Hongjishan Region as an Example. Acta Petrologica Sinica 30 (2), 547–564.
Zhao, Y., Li, Y. J., Sun, L. D., Zheng, D. M., Liu, Y. L., Wang, D. X., et al. (2012). Mesozoic - Cenozoic Extensional Structure in North Uplift of Tarim basin and its Genetic Discussion. Acta Petrologica Sinica 28 (8), 2557–2568.
Keywords: Late Triassic thrust, Jurassic–Cretaceous normal fault, collision-related structures, S Tarim Basin, N Qiangtang—Tarim collision, Cimmerides, closing of the Paleo-Tethys
Citation: Li Y, Wen L, Yang X-Z, Li C, Zhang L, Wang B, Chen C, Liu Y-L and Li Y-J (2022) Mesozoic Collision-Related Structures in the Southern Tarim Basin, W. China: Implications for the Paleo-Tethys Closing Process. Front. Earth Sci. 9:792049. doi: 10.3389/feart.2021.792049
Received: 09 October 2021; Accepted: 23 December 2021;
Published: 11 March 2022.
Edited by:
Lei Wu, Zhejiang University, ChinaReviewed by:
Junpeng Wang, China University of Geosciences Wuhan, ChinaChen Xinwei, Chengdu University of Technology, China
Hongwei Yin, Nanjing University, China
Copyright © 2022 Li, Wen, Yang, Li, Zhang, Wang, Chen, Liu and Li. This is an open-access article distributed under the terms of the Creative Commons Attribution License (CC BY). The use, distribution or reproduction in other forums is permitted, provided the original author(s) and the copyright owner(s) are credited and that the original publication in this journal is cited, in accordance with accepted academic practice. No use, distribution or reproduction is permitted which does not comply with these terms.
*Correspondence: Yue-Jun Li, eXVlanVubGVlY2hlbmdAc2luYS5jb20=