- 1Department of Geophysics, Institute of Astronomy, Geophysics and Atmospheric Sciences, University of São Paulo, São Paulo, Brazil
- 2Department of Sedimentary and Environmental Geology, Institute of Geosciences, University of São Paulo, São Paulo, Brazil
- 3Geology Lab, School of Technology, University of Campinas, Limeira, Brazil
- 4Departament of Geology, Federal University of Ouro Preto – DEGEO/UFOP, Ouro Preto, Brazil
- 5Department of Astronomy, Institute of Astronomy, Geophysics and Atmospheric Sciences, University of São Paulo, São Paulo, Brazil
- 6Department of Zoology, Institute of Biosciences, University of São Paulo, São Paulo, Brazil
- 7Laboratory of Porous Media and Thermophysical Properties, University Federal of Santa Catarina, Florianópolis, Brazil
- 8EDEM, Empresa De Desenvolvimento em Mineração e Participações Ltda., Goiânia, Brazil
- 9Department of Geophysics, Institute of Astronomy, Geophysics and Atmospheric Sciences, University of São Paulo, São Paulo, Brazil
Acritarchs, a polyphyletic group of acid-resistant organic-walled microfossils, dominate the eukaryotic microfossil record in the Proterozoic (2500–541 Ma) yet exhibit significant reduction in diversity and size at the transition to the Phanerozoic (541–520 Ma). Despite the difficulty of tracing phylogenetic relationships among acritarchs, changes in their complexity and diversity through time have allowed their use in paleoecological and biostratigraphic schemes. The Doushantuo-Pertatataka Ediacaran acritarch assemblage, for example, is usually considered as restricted to the early Ediacaran between 635 and 580 Ma. But similar, diverse acritarchs have been recovered from younger rocks in Mongolia and Arctic Siberia and are now reported here from phosphatized horizons of the upper Bocaina Formation (ca. 555 Ma), Corumbá Group, SW Brazil. In the overlying black limestones and shales of the latest Ediacaran Tamengo Formation (542 Ma) acritarch diversity is low, but the skeletal metazoans Cloudina and Corumbella are abundant. The Bocaina acritarch assemblage shares forms referable to the genera Leiosphaeridia, Tanarium, Asseserium and Megasphaera with the Doushantuo-Pertatataka assemblage, but also includes specimens similar to the Phanerozoic genus Archaeodiscina in addition to two new complex acritarchs. The first is covered by rounded low conical bumps, similar to Eotylotopalla but differs in having a distinct opening suggestive of greater (multicellular?) complexity. The second, identified here as Morphotype 1, is a double-walled acanthomorph acritarch with scattered cylindrical processes between the walls. The contrast in acritarch diversity and abundance between the Bocaina and Tamengo formations is likely due in part to paleoenvironmental and taphonomic differences (absence of the phosphatization window in the latter), as well as to the appearance of both suspension-feeding skeletal metazoans (Cloudina and Corumbella). The occurrence of Doushantuo-Pertatataka acritarchs in SW Brazil, northern Mongolia, and Arctic Siberia extend the biostratigraphic range of this assemblage up to the terminal Ediacaran Cloudina biozone.
Introduction
Acritarchs represent a polyphyletic group of resistant organic-walled eukaryotic unicellular organisms (Evitt, 1963), fundamental to our understanding of early eukaryotic evolution and biostratigraphy (Knoll, 2003; Grey, 2005). They dominate the Proterozoic fossil record of plankton from 2500 to 541 Ma, having successfully endured the “Boring Billion” and survived Neoproterozoic icehouse intervals and bolide impacts (Grey et al., 2003; Huntley et al., 2006; Moczydłowska, 2008). Yet, they waned at the end of the Ediacaran and beginning of the Cambrian, between 541 and 520 Ma, as invertebrates began to flourish (Downie et al., 1963; Huntley et al., 2006).
From the Paleoproterozoic to the Early Neoproterozoic, the acritarch record is dominated by simple spheroids (Huntley et al., 2006) of limited biostratigraphic utility. During the rest of the Neoproterozoic, palynomorphs are often large, complex, process-bearing acritarchs having more restricted ranges and thus greater biostratigraphic usefulness, especially in the Ediacaran (Grey et al., 2003).
Ediacaran acritarch biozones were first established in Australia (Grey, 2005; Grey and Calver., 2007), where an older one dominated by simple spheroidal acritarchs is referred to as the Ediacaran Leiosphere Palynoflora (ELP) zone, and a younger one, characterized by ornamented, spheroidal microfossils, is called the Ediacaran Complex Acritarch-dominated Palynoflora (ECAP) zone (Grey, 2005; Grey and Calver, 2007; Gaucher and Sprechmann, 2009). Within the ECAP zone, larger ornamented acanthomorphs ranging in size from 100 to 700 µm are collectively known as the Doushantuo-Pertatataka-assemblage (Zang and Walter 1992; Grey, 2005), originally recognized in the <580 Ma Pertatataka Formation, Australia (Grey et al., 2003; Grey, 2005; McKirdy et al., 2006; Grey and Calver, 2007) and the 635 ± 0.6 to 551.1 ± 0.7 Ma Doushantuo Formation, China (Chen and Liu, 1986; Condon et al., 2005). Attempts to extend these zones to other regions have met with limited success, but, in general, complex large acanthomorphs are largely limited to strata older than the Gaskiers glaciation at about 580 Ma (see discussion in Xiao and Narbonne, 2020).
Even without definitive geochronological constraints, the ELP zone has been considered earliest Ediacaran and the ECAP zone younger, but probably not younger than 560 Ma (Grey 2005; Grey and Calver., 2007; Gaucher and Sprechmann, 2009; Golubkova et al., 2015; Rud’ko et al., 2017; Anderson et al., 2017), making it the last explosive acritarch radiation of the Proterozoic (Moczydłowska, 1991; Vidal and Moczydłowska-Vidal, 1997; Gaucher and Sprechmann, 2009). The decline of the ECAP has usually been attributed to the rise of metazoans within the Nama Assemblage (550–541 Ma) (Fedonkin and Waggoner, 1997; Huntley et al., 2006; Gaucher and Sprechmann, 2009; Xiao and Narbonne, 2020).
Recently, diverse Doushantuo-Pertatataka-like acritarchs have been reported from in the latest Ediacaran of northern Mongolia (Anderson et al., 2017, 2019) and latest Ediacaran to early Cambrian of Arctic Siberia (Grazhdankin et al., 2020). Here, we describe a similar assemblage from shale-hosted phosphatic nodules and phosphatic microbialites of the upper Ediacaran Bocaina Formation (555.18 ± 0.30 Ma; Parry et al., 2017), Corumbá Group, SW Brazil. Overlying this unit is the Cloudina- and Corumbella-bearing, leiosphaerid-dominated (Gaucher et al., 2003) Tamengo Formation (542 ± 0.75 Ma; Parry et al., 2017), also within the Corumbá Group.
The acritarchs in the Bocaina Formation consist of single- and double-walled vesicles, with or without processes, representing sphaeromorphs, acanthomorphs, netromorphs and pteromorphs (Downie et al., 1963). The great variation in shape and dimensions of the acanthomorph vesicles observed in the Bocaina Formation is a feature shared with Doushantuo-Pertatataka-like Mongolian and Siberian acanthomorphs of similar age (Chen and Liu, 1986; Zang and Walter, 1992; Grey, 2005; Anderson et al., 2017; Grazhdankin et al., 2020).
Our results provide paleogeographic, stratigraphic and chronologic constraints for the first occurrence of Doushantuo-Pertatataka-like acritarchs described from South America and further corroborate the extension of the ECAP into Ediacaran rocks just below the terminal Ediacaran Cloudina biozone.
Geological Setting
The Corumbá Group is part of the southern Paraguay Belt, cropping out in the Urucum District and the Serra da Bodoquena, Mato Grosso do Sul, Brazil, central South America (Figure 1) (Almeida, 1946; 1965). In the Urucum District, the Corumbá Group comprises a carbonate-dominated succession deposited upon Paleoproterozoic granite and gneiss-dominated basement of the Rio Apa Block, as well as upon Cryogenian successions of the Jacadigo Group (Freitas et al., 2011; 2021) and the Puga Formation (Maciel, 1959; Freitas et al., 2011). There are two main carbonate sequences in the Corumbá Group: the lower, dominated by light grey dolostone enriched in phosphate near its top - the Bocaina Formation; and the upper, dominated by dark grey limestone with organic-rich shale intercalations and the metazoan fossils Cloudina and Corumbella—the Tamengo Formation (Figure 1A; Gaucher et al., 2003). Zircon crystals from ash beds near the top of both units have provided U-Pb ID-TIMS (CA-ID-TIMS) minimum ages of 555.18 ± 0.30/0.34/0.70 Ma for the Bocaina Formation and 541.2 to 548 Ma for the Tamengo Formation (Figure 1A; Parry et al., 2017). Conformably overlying the Tamengo Formation and finalizing the Corumbá Group are fine-grained siliciclastic deposits of the <541 Ma Guaicurus Formation (Figure 1A; Parry et al., 2017). This same succession and bounding stratigraphic sequences of the Corumbá Group are also recognized in the Serra da Bodoquena, overlying the siliciclastic-dominated succession of the Puga, and Cerradinho formations (Figures 1A,B; Almeida, 1965; Campanha et al., 2011). Geochronology of detrital zircon from phyllite of the Guaicurus Formation in the Serra da Bodoquena provides a maximum depositional age of 543 Ma ± 11 (McGee et al., 2018), consistent with results obtained by Parry et al. (2017) in the Urucum District (Figure 1A).
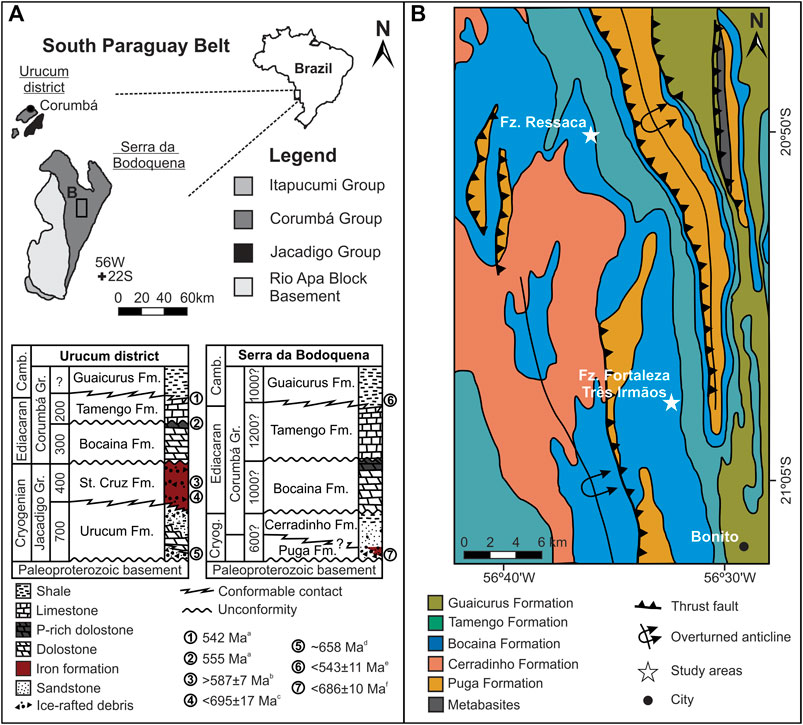
FIGURE 1. Geological setting. (A) Neoproterozoic units, main outcropping areas and simplified stratigraphic charts of the South Paraguay Belt, western Mato Grosso do Sul, Brazil. Numbers in the stratigraphic charts are maximum estimated stratigraphic thickness in meters, for each formation, mostly based on composite sections. For the Urucum district area, maximum thickness estimates are from Gaucher et al. (2003), Boggiani et al. (2010) and Freitas et al. (2021). For Serra da Bodoquena, maximum stratigraphic thicknesses are estimates presented in the pioneer work of Almeida (1965). Time constraints: aAsh bed zircon, U-Pb, ID-TIMS (CA-ID-TIMS) (Parry et al., 2017); bCriptomelane, Ar-Ar (Piacentini et al., 2013); cDetrital zircon, U-Pb, LA-ICP-MS (Frei et al., 2017); dSequence and chemostratigraphic correlation with Neoproterozoic cryochrons (Freitas et al., 2021); eDetrital zircon, U-Pb, SHRIMP (McGee et al., 2018); fDetrital zircon, U-Pb, LA-ICP-MS (McGee et al., 2018). (B) Geological sketch map of study area, after Campanha et al. (2011).
The Bocaina Formation records shallow marine conditions as indicated by abundant ooid grainstone and stromatolitic dolostone, as well as by phosphate-rich horizons, observed in both the Urucum District and the Serra da Bodoquena (Almeida 1945; Gaucher et al., 2003; Freitas et al., 2011). The unit is truncated at the top by a regional erosional unconformity (Figure 1B), overlain by polymictic breccia having clasts of crystalline basement, dolostone and phosphorite at the base of the Tamengo Formation (Gaucher et al., 2003; Campanha et al., 2011; Amorim et al., 2020). The overlying Tamengo Formation was deposited in a shallow to relatively deep storm-influenced carbonate ramp, as interpreted from cross-bedded fine grainstones, mudstones and shales associated with metazoan fossils and meiofaunal trace fossils (Zaine and Fairchild, 1987; Gaucher et al., 2003; Van Iten et al., 2014; Pacheco et al., 2015; Adorno et al., 2017; Parry et al., 2017; Amorim et al., 2020).
The microfossils reported here were recovered from the upper Bocaina Formation at Serra da Bodoquena (Figure 1B) in samples from a drill core (ALW-DD004) and three trenches cutting phosphate-rich horizons mined by EDEM (Empresa de Desenvolvimento em Mineração e Participações Ltda) at Fazenda Ressaca and Fazenda Fortaleza Três Irmãos, between Bonito and Bodoquena, Mato Grosso do Sul (Figures 1, 2).
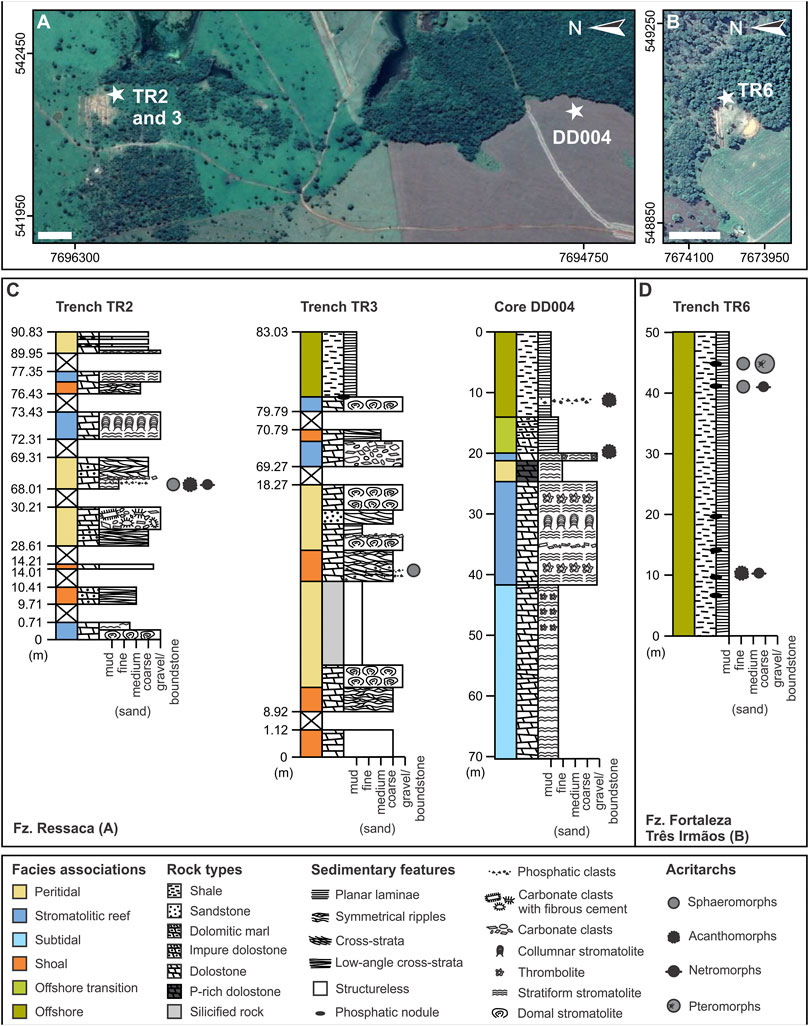
FIGURE 2. Studied successions. (A) Satellite image (Google Earth Pro v. 7.3.4.8248) of Fazenda (Fz.) Ressaca with approximate locations of trenches TR2 and TR3, and drill hole DD004. (B) Satellite image (Google Earth Pro v. 7.3.4.8248) of Fazenda Fortaleza Três Irmãos area displaying the approximate location of trench TR6. (C) Simplified columnar sections measured along trenches TR2 and TR3, and drill core DD004. (D) Simplified columnar section measured along trench TR6. UTM zone: 21K. White scale bars: 100 m.
Materials and Methods
Stratigraphic sections are shown in Figure 2 for drill core DD004, trenches TR2 and TR3 at Fazenda Ressaca and TR6 at Fortaleza Três Irmãos, in areas of phosphate prospection carried out by EDEM mining company in the Serra da Bodoquena. Thirty samples were collected from phosphate-rich intervals mainly composed of dolomitic microbialite and shale in the drill core and trenches, 12 of which yielded the microfossils described here (Table 1).
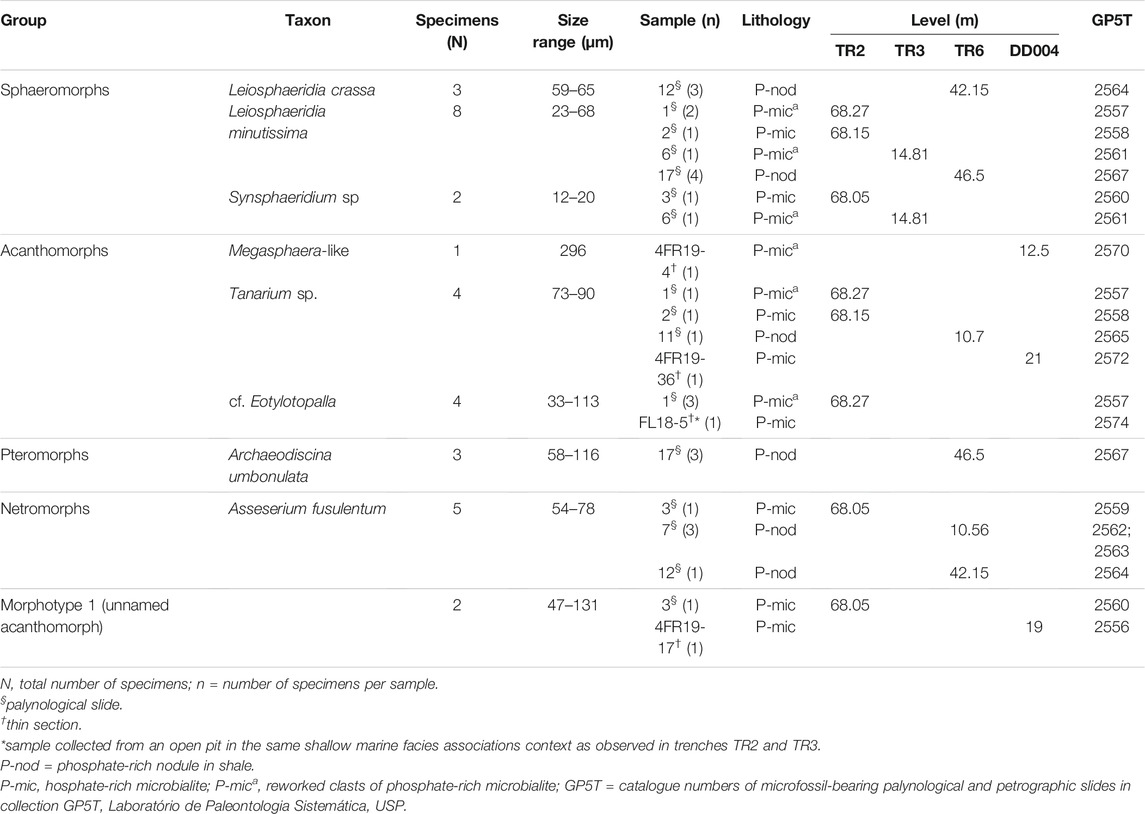
TABLE 1. Summary of basic facts on the size and distribution of acritarchs of the Bocaina Formation recognized in this study.
Samples of P-rich dolomitic microbilite were examined in petrographic thin sections (4FR19-4, 4FR19-17, FL18-5 and 4FR19-36, Table 1) using a Leica DM750 P microscope equipped with a Leica MC 170 HD camera. Some images were obtained using a simplified version of the “white-card” technique of Folk (1987), by placing a small piece of white paper beneath the thin section to diffuse the incoming light beam, thereby softening the visual effect of carbonate crystal boundaries and permitting a clearer view of individual rock components, especially, those made of or containing organic matter.
Palynological preparations were made from P-rich dolomitic microbialite (samples 1, 2 and 3), P-rich nodules in shale (samples 7, 11, 12 and 17) (Table 1). About 100 g of fresh samples were reacted with HCl (37%) and HF (30%) at the Centro de Pesquisa Leopoldo Américo Miguez de Mello (CENPES—Petrobrás, Rio de Janeiro), initially with HCl for 2 days, neutralized, and then with HF for 1 h on a hot plate, and then repeatedly rinsed with distilled water until neutralized. A portion of the organic slurry was withdrawn with a micropipette under a binocular microscope and preserved as palynological slides.
Also, at CENPES, three-dimensional Confocal Laser Scanning Microscope (CLSM) images were obtained from selected specimens of palynological slides from samples 1, 3 and 5 (Table 1) with lasers of different wavelengths using a ZEISS® Imager.Z1mTM microscope, equipped with a ZEISS® LSM 700TM confocal laser scanning system. ZEISS® Zen© BlackEditionTM software was used to capture images with a ×100 oil immersion lens. The submicron-scale resolution of the fluorescence in the vesicles obtained by CLSM allowed reconstruction of their three-dimensional volume, thereby providing insight into their morphology and fidelity of preservation (Figures 5F,H,S).
Rotatable 3D CLSM images rendered for two specimens are deposited in the public repository Sketchfab profile LMPT (https://skfb.ly/opV9p; https://skfb.ly/opVXX). Reconstruction by Sketchfab can produce artifacts in these images, such as voids where the surface is deeply shaded. True holes, however, may be seen clearly in the confocal images (Figures 5F,H).
Dark brown to black carbonaceous acritarch vesicles were investigated by Raman spectroscopy at the Brazilian Astrobiology Research Unit (NAP/Astrobio), using a Renishaw inVia micro-Raman, with 532 nm excitation wavelength, diode laser (500 mW) and 2400-groove/mm grating. Spectra were collected with a ×50 objective at 1, 5 or 10% laser power. This technique is broadly used to determine graphitic composition of organic-walled microfossils (Schopf et al., 2005).
The material is identified as “Doushantuo-Pertatataka-Bocaina fossils, Corumbá Group, Brazil,” and reposited in paleontological collection GP5T, Palynology, in the Laboratório de Paleontologia Sistemática, USP, under catalogue numbers GP5T/2556, 2570, 2572 and 2574 (all petrographic thin sections) and GP5T/2557 to 2565, 2567 and 2570 (palynological sections).
Results
Sedimentary Succession
The base of the Bocaina Formation in the study area (Figure 1B) is a sequence boundary truncating siliciclastic-dominated deposits of the Cerradinho and Puga Formations, whereas its top is limited by the sequence boundary associated with the basal polymictic breccia of the Tamengo Formation. These units often dip between 20 and 60 degrees to eastern quadrants, comprising the normal flank of asymmetrical folds with westward vergence (Figure 1B). Metamorphism is incipient, and primary sedimentary structures and mineralogy are well preserved. The thickness of the Bocaina Formation in this part of the Serra da Bodoquena (Figure 1B) exceeds 500 m.
More than 70 m of continuous stratigraphic thickness of the upper Bocaina Formation are evident in core DD004, and more than 50 m are exposed in Trench TR6 (Figure 2). Trenches TR2 and TR3 offer sparser, meter-scale exposures scattered over about 80–90 m of stratigraphic thickness of the upper Bocaina Formation (Figure 2). The succession represented by these sections shows an upward transition from dolostone to shale (Figure 2).
The dolostone consists mainly of grainstone (Figures 3A,B,F) and boundstone (Figures 3A–E,G,H) with minor rudstone (Figures 3A,B,G) and mudstone (Figure 3B). Grainstones are composed of fine to medium ooids and peloids, with symmetrical ripples (Figure 3G), low-angle and trough cross-strata, as well as sparse quartz sand grains and coarse carbonate clasts (Figures 3A,B). This facies is organized in decimeter-thick beds often intercalated with microbial mats associated with centimeter-thick granule to pebble breccia (Figures 3A,B,G). Parts of the succession are dominated by microbialites, including thrombolites (Figure 3D), columnar and domal stromatolites (Figures 3C,H), and breccias. Phosphate-rich horizons are associated with boundstones (Figure 3G) or breccias with phosphate-rich clasts (Figure 3B). Marl and sandstone intercalations also occur along the carbonate-dominated successions, especially near the top of core DD004, below the shales (Figures 2C, 3E,I). The shale-dominated section reaches more than 50 m in thickness and locally displays phosphate-rich nodules (Figure 3I), seams and clasts (Figures 2C,D).
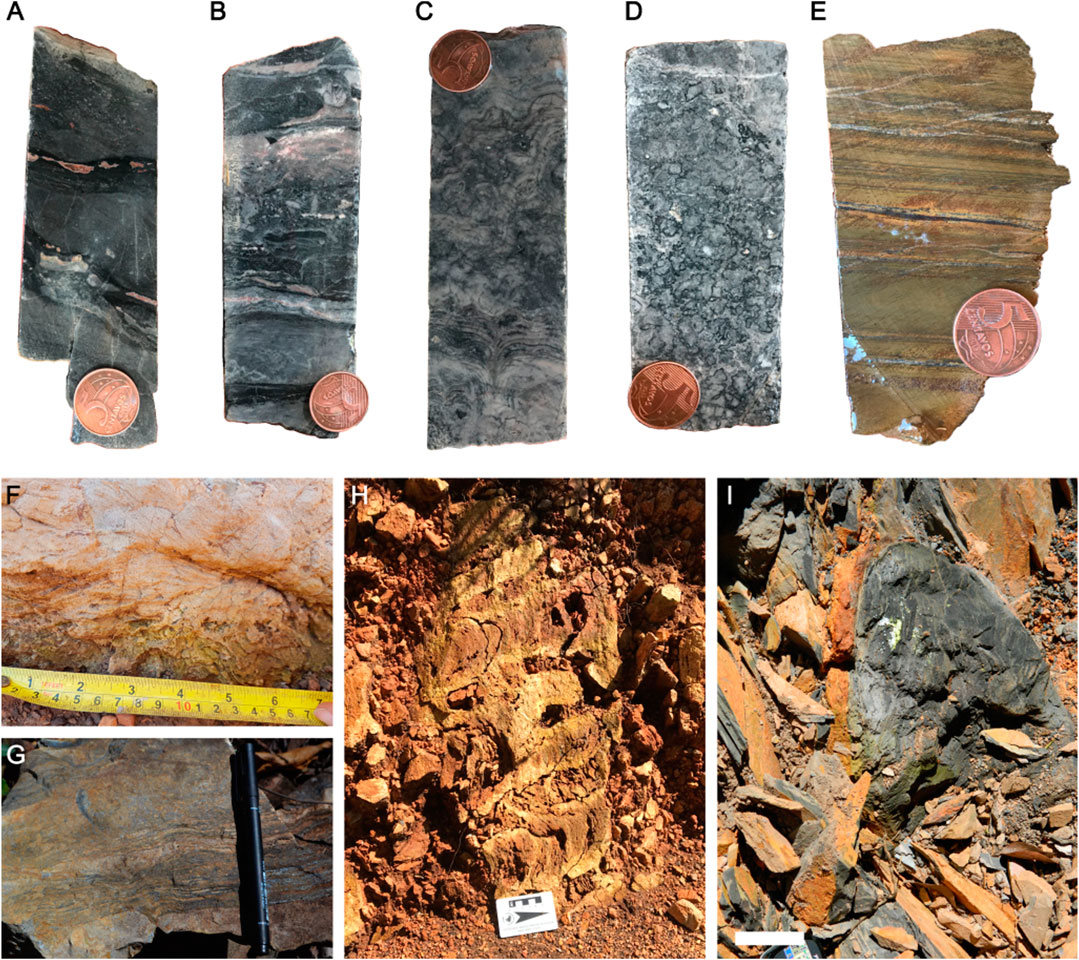
FIGURE 3. Representative facies examples from the studied successions. (A) Dolomitic grainstone and boundstone association, with minor rudstone. Note two P-rich darker beds made up predominantly by irregularly laminated boundstone with dispersed coarse-grained carbonate clasts and irregular vugs. The intermediate grainstone bed appears cross-stratified. (B) Fine-grained dolostone, rudstone and grainstone, from base to top, with subordinate boundstone intercalations, locally P-rich (black). (C) Columnar to domal stromatolites, from base to top. (D) Thrombolitic boundstone. (E) Incipiently foliated fine-grained siliciclastic facies. (F) Cross-section of impure dolomitic grainstone displaying chevron-type upbuilding of bidirectional cross-laminae. (G) P-rich stratiform boundstone with subordinate grainstone. (H) Cross-section of columnar stromatolites in near vertical altered dolostone bed. (I) Cross-section of P-rich nodule in shale. Five-cent piece (A–E): 22 mm; pen (G): 14 cm; White scale bar (I): 10 cm.
The studied part of the Bocaina Formation presents retrogradational stratigraphic architecture in which shallow-water carbonates are succeeded by offshore shales (Figure 2). Phosphate-rich grainstone deposits predominantly displaying oscillation ripples and rip-up clast concentrations, locally with fibrous cements, comprise peritidal facies associations (Figure 2). Diverse boundstone facies with subordinate breccia are interpreted as stromatolitic reefs, whereas successions dominated by cross-bedded grainstone represent carbonate shoals (Figure 2). Subtidal deposits include fine-grained grainstone intercalated with microbial mats and associated breccia (Figure 2). Intercalated marl and mudstone below the shaly succession mark the offshore transition to thick shale deposits in a deeper offshore setting (Figure 2).
Acritarchs
The Bocaina assemblage consists of moderately to very well-preserved organic-walled microfossils present in 12 of the 30 analyzed samples and represented by acid-released palynomomorphs from four samples of shale-hosted phosphatic nodules (samples 7, 11, 12 and 17) and eight samples of phosphatic microbialites (samples 1, 2, 3, 6, 4FR19-4, 4FR19-17, FL18-5, and 4FR19-36) from the upper Bocaina Formation (Figure 1, Table 1).
The Bocaina assemblage is diverse and includes specimens attributed to five known taxa: Assesserium fusulentum (Figures 5B,C), Leiosphaeridia crassa (Figure 5I), L. minutissima (Figure 5J), Synsphaeridium sp. (Figure 5L), Tanarium sp (Figures 5M,N), plus three morphotypes comparable to Archaeodiscina ? (Figure 5A), Eotylotopalla (Figures 5D–H), and Megasphaera (Figure 5K). Additionally, one unnamed form described here as Morphotype 1 (unnamed doubled-walled acanthomorphs) is also presented (Figures 5O–Q).
The acritarchs described here vary in diameter from 12 to 296 µm as well as in shape and morphology of processes. They include three species of sphaeromorphs, four taxa of acanthomorphs, including the new morphotype 1 and one each of pteromorphs and netromorphs (Downie et al., 1963). Of the 30 samples examined, 12 were fossiliferous. Of these, six yielded sphaeromorphs (n = 13); eighth, acanthomorphs (n = 11); three, netromorphs (n = 5) and one, pteromorphs (n = 3) (Table 1).
Microfossils vary in color regardless of their facies association. They can be light brown, opaque brown to black or translucent grey. Thick-walled vesicles are usually dark to opaque brown, while thin vesicles are generally translucent grey (Figure 5). Evaluation of the thermal alteration of the organic vesicles by Raman spectroscopy showed D and G bands (at ∼1350 cm−1 and ∼1600 cm−1, respectively) indicative of mature kerogen in all microfossils analyzed. The presence of kerogen in all the specimens is consistent with the syngenicity of the microfossils and their host-rocks (Schopf et al., 2005). Raman spectra produced no evidence of co-occurring silica, carbonate, or phosphate in their composition (Figure 4). Detailed descriptions of the microfossils are provided in the Systematic Paleontology section below.
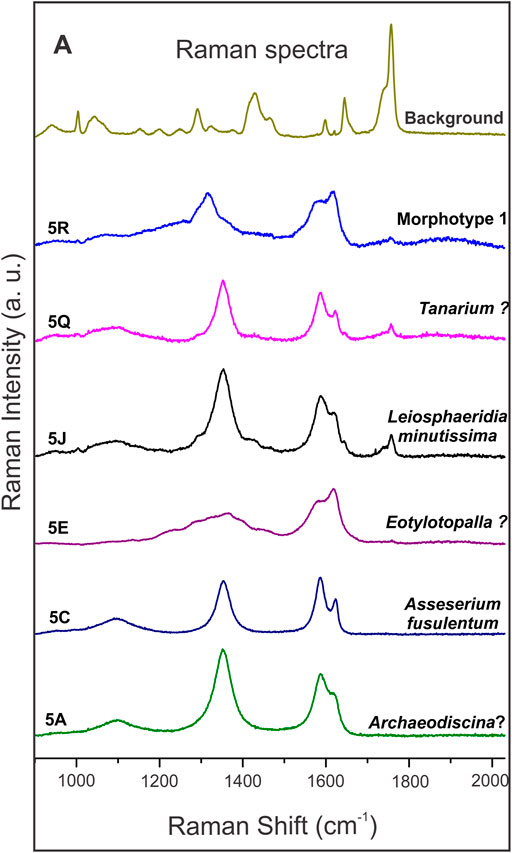
FIGURE 4. Raman point spectra of microfossils from the Bocaina Formation. Number followed by a letter in each spectrum indicates the figure where the analyzed specimens may be found. The bumpy “D” band (at ∼1350 cm−1) and the “G” band (at ∼ 1600 cm−1) indicate matured kerogen in all spectra. Background spectrum refers to the resin used to fix specimens on the palynological slides.
Discussion
The Bocaina Formation has already been tentatively correlated to other Neoproterozoic units by means of litho-, bio- and chemostratigraphy (Gaucher et al., 2003). However, until now, no biozones have been recognized. Here, we present a new diversified acritarch assemblage, including representatives from the Doushantuo-Pertatataka assemblage, as Asseserium fusulentum, cf. Eotylotopalla, Tanarium sp, a Megasphaera-like vesicle, Leiosphaeridia crassa, L. minutissima and Synsphaeridium sp, plus the Cambrian representative Archaeodiscina ? and one morphotype unknown in the literature (Figure 5).
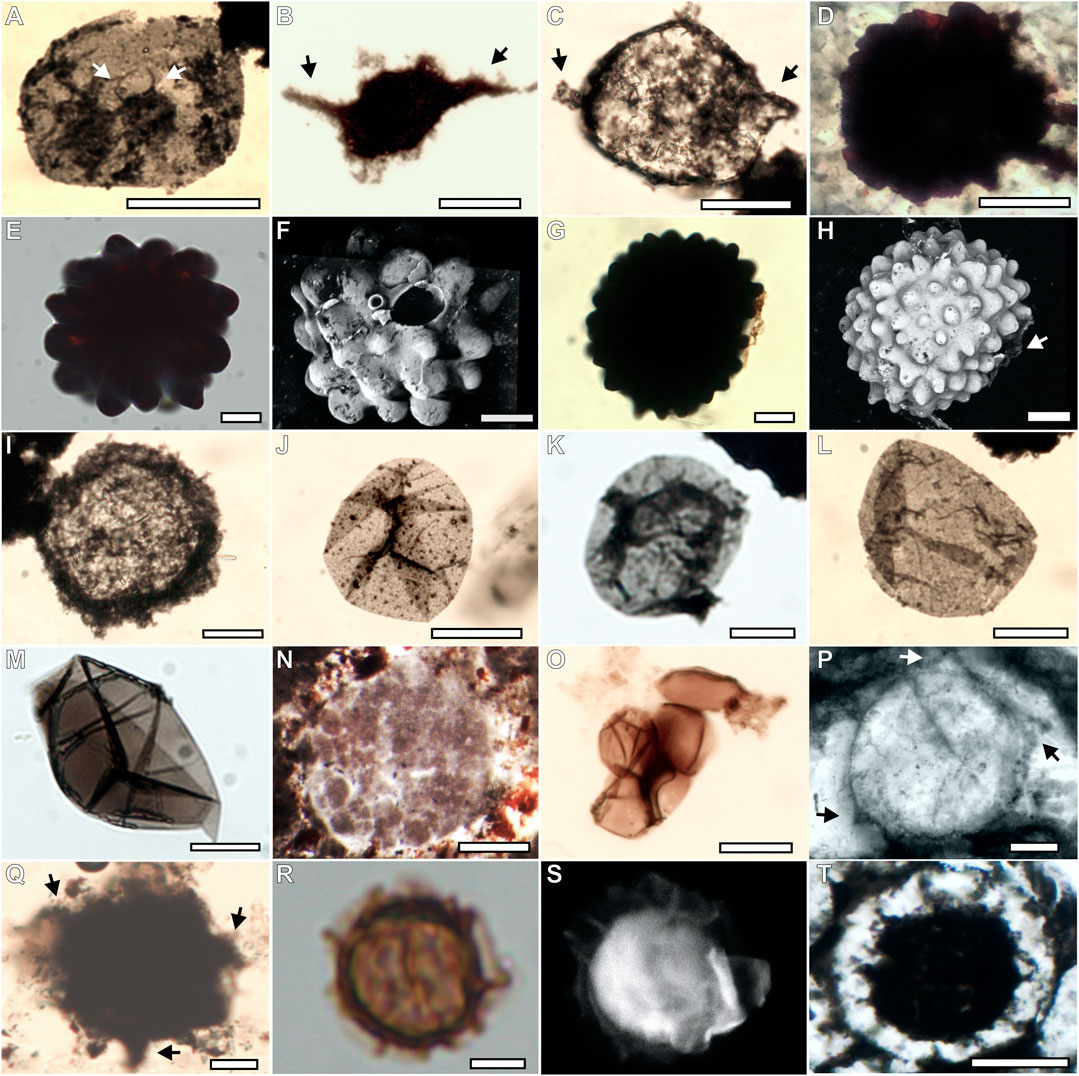
FIGURE 5. Acritarch assemblage from the Bocaina Formation in palynological slides, confocal 3D reconstructions and thin sections. (A; GP5T/2567) Archaeodiscina ? in a palynological slide, characterized by relatively small central body bearing a few relatively long, very narrow spines (indicated by white arrows) within a thin-walled membrane or vesicle. (B,C; GP5T/2559, 2562) Asseserium fusulentum in a palynological slide, showing a roughly fusiform acritarch with irregular opposite spines indicated by black arrows. (D; GP5T/2574) cf. Eotylotopalla in thin section, showing a spheroidal acanthomorph vesicle, ornamented with generally broader than high, rounded to conical processes or verrucae. (E–H; GP5T/2557) (E,G) Cf. Eotylotopalla in palynological slide, showing a spheroidal acanthomorph vesicle, ornamented with generally broader than high, rounded to conical processes or verrucae. (F) Confocal image of specimen shown in (E), with large and small elliptical openings. (H) Confocal image of specimen shown in (G) with white arrow highlighting opening. (I; GP5T/2564) Thick walled Leiosphaeridia crassa in palynological slide. (J–M; GP5T/2561; 2567, 2558, 2570, respectively) Thin walled Leiosphaeridia minutissima in palynological slide. (N; GP5T/2570) Cross-section through a Megasphaera-like acritarch in a thin section. (O; GP5T/2560) Thick-walled aggregate of Synsphaeridium sp in palynological slide. (P; GP5T/2572) Tanarium sp., spheroidal acanthomorph acritarch ornamented with sparse obtusely conical processes indicated by arrows in thin section. (Q; GP5T/2565) Tanarium ? spheroidal acanthomorph acritarch ornamented with sparse conical processes indicated by arrows in palynological slides. (R; GP5T/2560) morphotype 1 consisting of a double-walled spheroidal vesicle with processes in the inner wall. Clear area defines original limits of outer membrane. (S) Confocal image of specimen shown in (R). (T; GP5T/2556) Morphotype 1 consisting of a double-walled spheroidal vesicle with processes in the inner wall in thin section. Scale bars: 5 µm = R, S; 20 µm = A, C–M; 25 µm = B; O–Q; 100 µm = N, T.
The microfossils described here present Raman spectra typical of amorphous carbon, consistent with the degradation of organic matter to kerogen. Contemporary organic matter bands (e.g., C–N, N–H, C=O) were not observed (Figure 4). Spectra indicative of carbonate, silica and phosphate were not present at the surface of these specimens (Figure 4).
Of the 12 fossiliferous samples studied from the Bocaina Formation, six were obtained from the shale-dominated succession here interpreted as offshore deposits near the top of the studied interval. Of these six samples, four come from phosphate-rich nodules within the shale succession and one is from reworked phosphatic microbialite clasts within shale (Figure 2; Table 1). Six other fossiliferous samples were collected within the carbonate-dominated succession. Four correspond to in situ phosphate-rich microbialites and two to reworked clasts of phosphate rich microbialites within shallow marine facies associations (Figure 2, Table 1). Hence, we interpret that most of the Ediacaran complex acritarchs (ECAP, sensu Zhang et al., 1998; Moczydłowska and Nagovitsin, 2012; Xiao et al., 2014; Moczydłowska, 2016) recognized here were entombed in both shallow proximal (n = 17) as well as relatively deeper distal settings (n = 17) (Figure 2; Table 1).
Facies interpreted as deposited in shallow water settings host Synsphaeridium sp, cf. Eotylotopalla, Megasphaera-like structures, Tanarium sp. and Asseserium fusulentum. Distal deposits present Leiosphaeridia crassa and Archaeodiscina ? as well as rare specimens of Tanarium sp. and Asseserium fusulentum. L. minutissima is the only species common in both settings. Morphotype 1 was found in shallow water settings.
Two unusual new acritarch forms resembling Eotylotopalla (Yin, 1987), are designated here as cf. Eotylotopalla (Yin, 1987) (Figures 5D–H). Unlike previously described specimens of this species, they possess large openings (more obvious in Figure 5F and less visible in Figure 5H) and just beside them much smaller openings at the top of an unusually small process (only visible in Figure 5F). Either or both may be artifacts of preservation or sample preparation. But we interpret the larger opening as an original feature of the acritarch, because the border of the opening is evenly elevated above the surrounding surface and very even, neither torn nor irregular. Moreover, no other processes of similar size show signs of breakage.
Accepting that the hole is a biogenic structure, two possible interpretations suggest themselves. The verrucate, aperturate raspberry-like resistant organic vesicle is comparable to tests of the extant testate amoebae Netzelia muriformis and N. tuberculata (Gauthier-Lièvre and Thomas, 1960; Owen and Jones, 1976). Testate amoebae were already described in Neoproterozoic rocks from Brazil (Morais et al., 2017), however, no similar specimen was described until now. Additionally, tests in Netzelia muriformis and N. tuberculata are constructed of autogenous siliceous elements and possess a lobate opening with 3–8 indentations (Gomaa et al., 2017). Despite these discrepancies, phylogenomic reconstructions suggest that major lineages of testate amoebae were already diversified during the Neoproterozoic, which may suggest that the specimens reported here could be related to hypothetical ancestors for Netzelia (Lahr et al., 2019).
The new acritarch also resembles sexually produced zygospores in fungi of the Zygomycota (Hermann and Podkovyrov, 2006), that is, it could represent a stage in the life cycle of an early multicellular organism. More specifically, the opening may have been the point of insertion of the peduncular hyphae or served as the exit for the germinating fungus. However, structures resembling fungi structures (e.g., peduncular hyphae) were not found until now, which prevented the establishment of this biological affinity. New aperturate raspberry-like resistant organic vesicles can help to answer questions about the biological affinity for cf. Eotylotopalla.
Two double-walled acanthomorphic acritarchs described here share similarities with Distosphaera speciosa (Zhang et al., 1998), as a spheroidal vesicle characterized by two wall layers (Figures 5O–Q). However, D. speciosa hosts processes in both vesicles, while the specimens described here have processes only in the inner vesicle. Due to this fact, they were interpreted in open nomenclature as morphotype 1.
The quality of preservation of the acritarchs described here is due to their preservation in phosphatized microbialites and phosphatic nodules in shales in the study area within the so-called phosphatization taphonomic window (Porter, 2004), best epitomized by the exceptional preservation of Doushantuo microfossil assemblage in phosphate (Xiao et al., 2014). The reduced diversity and size of acritarchs in the overlying Tamengo Formation reflects the general trend for acritarchs worldwide at the end of the Ediacaran (Gaucher et al., 2003), while the closure of the phosphatization window accounts in part for their paucity.
Conclusion
Microfossils in the latest Ediacaran Bocaina Formation are diverse, contrasting sharply with the simple, leiosphaerid-dominated assemblage of the overlying Cloudina- and Corumbella-bearing Tamengo Formation. The Bocaina assemblage hosts acritarch taxa typical of the supposedly older Doushantuo-Pertatataka assemblage, specifically, Asseserium, Leiosphaeridia, Tanarium, the acanthomorphic vesicle described here as morphotype 1 and a vesicle resembling Megasphaera, yet it also includes forms comparable to younger acritarch genera of Cambrian age Archaeodiscina. Moreover, two unusual and important new acritarch taxa are also present. The first, cf. Eotylotopalla, possesses a raspberry-like form and distinct opening that permit comparison with some modern testate amoebae Netzelia and less with fungal zygospores. The second, Morphotype 1 resembles Distosphaera speciosa Zhang et al., 1988. However, the absence of diagnostic features prevents this interpretation, leading us to interpretate these specimens in open nomenclature.
The diversity of the Bocaina acritarch assemblage, with its supposedly “older” Doushantou-Pertatataka elements, complex new forms, and Cambrian look-alikes, suggests that, at c. 555 Ma, the plankton in the Ediacaran ecosystem, at least in the Bocaina Formation, was stable and thriving. Initiation of the end-Ediacaran plankton crisis responsible for the depauperate acritarch assemblages of the latest Ediacaran may well be lost in the hiatus represented by the erosional unconformity between the Bocaina and Tamengo formations.
The acritarchs reported here bode well for the potential of the c. 555-Ma-old Bocaina phosphorites and phosphate-rich dolostones to provide further important data on paleobiology and biostratigraphy of the latest Ediacaran as the Neoproterozoic biosphere gave way to that of the Phanerozoic.
Systematic Paleontology
Group Acritarcha Evitt, 1963.
Genus Archaeodiscina Naumova, 1960 emend. Volkova, 1968.
Type species.--Archaeodiscina granulate Naumova.
Archaeodiscina ? Volkova, 1968 (Figure 5A).
Holotype.--Archaeodiscina umbonulata Volkova, Volkova, p. 27, pl. 5, Figures 1–8; pl. 11, Figure 8 (holotype pl. 5, Figure 1).
Material.--Three compressed specimens recovered in the palynological residue of a phosphatic nodule within shale (GP5T/2567).
Description.--Outer membrane irregularly circular to elliptical (compressed), translucent, 58–116 µm in diameter, with few folds; inner body circular to elliptical, 16–36 µm in greatest dimension with a few very narrow ray-like processes or folds 4–14 µm length that give it a “star-like” character.
Occurrence.-- All specimens came from a single phosphatic nodule in shale in TR6 (46.5 m) in the Bocaina Formation (Table 1).
Remarks.--Archaeodiscina has double membranes. The outer membrane diameter usually ranges from 25 to 50 µm and the inner range from 22 to 42 µm. The inner star-like spheroidal body range from 2 to 7 µm and radial folds length 4–10 µm (Downie, 1982; Moczydlowska, 2011). Specimens from the Bocaina Formation are larger (>50 µm in diameter) than specimens belonging to this genus. As Archaeodiscina, they are distinguished from other pteromorphs by the presence of a morphologically regular surficial organic body consisting of a spheroidal center hosting processes. The illustrated specimen presents a central vesicle with a few very fine processes and also presents opaque organic grains adhered to their surfaces. Other dark features may be interpreted to be linear folds of the outer vesicle. The specimen shown in Figure 5A is the most similar to Archaeodiscina umbonulata (see Moczydłowska 2011, Plate 1), showing clearly, at least, two processes (indicated by white arrow in Figure 5A). However, the other two specimens have organic material adhered to the surface that prevents a clear view of the central body and processes. Due to this fact, specimens described here were interpreted with reservations.
Genus Asseserium Moczydlowska and Nagovitsin, 2012.
Type species.--Asseserium diversum Moczydlowska and Nagovitsin, 2012.
Asseserium fusulentum Moczydlowska and Nagovitsin, 2012 (Figures 5B,C).
Holotype.--PN8/16-3, Moczydlowska and Nagovitsin, 2012, Figure 5F, p. 13.
Material.--Five specimens recovered, one recovered in palynological residues from one level of phosphatic microbialites from TR2 (GP5T/2559) and four recovered in two levels of phosphatic nodules within shale (GP5T/2562; 2563; 2564).
Description.--Oval to elongate bipolar vesicles ranging from 54 to 78 µm length and 25–37 µm width. Processes located at opposite sites, conical of varied length (6–27 µm) and basal width or bulbous (Figures 5B,C), and may differ in shape at opposite poles.
Occurrence.--In the Bocaina Formation, one specimen occurs in one level of phosphatic microbialites from TR2 (68.05 m) and four occur in two levels of phosphatic nodules from TR6 (10.56 and 42.15 m; Table 1). A. fusulentum is common in the Ediacaran Vychegda and Doushantuo formations of Russia and China, respectively (Vorob'eva et al., 2007; Liu and Moczydlowska, 2019); early Ediacaran Ura Formation and East Siberia (Moczydłowska and Nagovitsin, 2012).
Remarks.--A. fusulentum is characterized by a medium-sized, bipolar in symmetry vesicle and spindle-shaped in outline (originally three-dimensional spindle) with smooth surface and bearing two processes gradually extending from the central portion of the vesicle. The wall of the vesicle and processes is uniform. Processes are conical in shape and taper towards slightly rounded tips, being hollow inside and communicate with the vesicle cavity. Specimens described here fit the original description of Asseserium fusulentum described by Moczydlowska and Nagovitsin (2012), which ranges from 60 to 124 μm in length and 24–67 μm in width with processes up to 20 μm long. Organic-walled acritarch with two processes that arise from opposite poles similar to A. fusulentum was described in early to middle Ediacaran siliciclastic deposits in northern Russia, in the Vychegda Formation (their Figure 4I), and were not attributed to any recognized species (Vorob'eva et al., 2009).
Genus Eotylotopalla Yin, 1987.
Type species.--Eotylotopalla delicata Yin, 1987, p. 442, pl. 23, Figure 15; pl. 24, Figures 8, 9.
cf.Eotylotopalla Yin, 1987 (Figures 5D–H).
Material.--Four specimens, one observed in a thin section of a phosphatic microbialite and three recovered in palynological residues from a similar interval (GP5T/2574; 2557).
Description.-- Opaque spheroidal vesicles, 33–113 µm in diameter, densely and evenly covered by rounded to bluntly conical processes and verrucae 5–16 µm in diameter and 8–10 µm high. Openings evident in two larger specimens, measurable in one (26 × 16 μm, Figures 5E,F), but unknown in two smaller specimens because of their opacity. A second smaller opening (7 × 6 µm) of unknown origin (taphonomic?) appears just below the larger one in Figure 5F. The larger opening exhibits a uniform, slightly raised border.
Occurrence.--One specimen observed in a thin section of a phosphatic microbialite horizon without stratigraphic control, collected in a pit opened in the area of trenches TR2 and TR3 (Figure 5D). Two additional specimens (Figures 5F–H) in palynological slides from phosphatic microbialites in TR2 (68.27 m, Table 1). This taxon was first described in the upper Doushantuo Formation, China (Yin, 1987; Zhang et al., 1998), and later found in the early Neoproterozoic Wynniat Formation, Canadá (Butterfield, 2015, p. 9, Figure 3E) and the late Ediacaran Kessyusa Group, Arctic Siberia (Grazhdankin et al., 2020, p. 711, Figure 4D).
Remarks.--Specimens from the Bocaina Formation are slightly larger than the type material, which ranges from 35 to 45 µm in diameter (Zhang et al.,1998), and the vesicle described by Grazhdankin et al. (2020), which reaches 83 μm, but are smaller than the vesicles described by Xiao et al. (2014), which reach 200 µm in diameter. Specimens shown in Figures 5D–F, resembles Eotylotopalla dactylos Zhang et al., 1998, mainly by the regularly spaced cylindrical processes, openly communicating with the interior vesicle. Specimen shown in Figures 5G,H is more similar to E. strobilata (Faizullin, 1998) Sergeev, Knoll and Vorobo’eva, 2011, hosting small, hemispherical, nearly equidimensional processes, densely and evenly distributed over vesicle wall.
Openings differentiate at least two of the Bocaina specimens from previously described material and justify their identification as cf. Eotylotopalla, pending further research. The elliptical shape of the openings in the only specimen in which they are clearly visible is likely due to distortion of originally circular structures during compaction.
The two larger specimens are reminiscent of the small hollow aperturate spherical tests of the extant species of testate amoebae Netzelia muriformis and N. tuberculata (Gauthier-Lièvre and Thomas, 1960; Owen and Jones, 1976), which have small regular rounded protuberances giving them a raspberry-like outline. The extant organisms differ, however, in being composed of endogenous siliceous elements (Gomaa et al., 2017) and having lobate openings with three to eight indentations (Gauthier-Lièvre and Thomas, 1960).
Cf. Eotylotopalla is also similar to fungal zygospores of multicellular fungi of the Zygomycota (Hermann and Podkovyrov, 2006), in which case the openings might represent the exit point upon germination or the area of insertion of a peduncular hypha.
Genus Leiosphaeridia Eisenack, 1958, emend. Downie and Sarjeant, 1963, emend. Turner, 1984.
Type species.--Leiosphaeridia baltica Eisenack, 1958.
Leiosphaeridia crassa (Naumova, 1949); Javaux and Knoll: Figure 4(4–6), 2017 (Figure 5I).
Holotype.--No holotype was designated by Naumova (1949). A specimen of Leiotriletes minutissimus from Naumova (1949, pl. 1, Figure 1) was designated as lectotype by Jankauskas et al. (1989, p. 75), but this was later synonymized with L. minutissima (Porter and Riedman, 2016).
Material.--Three specimens found in palynological residues of phosphatic nodules in shale from a single level in TR6 (GP5T/2564).
Description.--Leiosphaeridia with thick-walled, relatively dark walls, ranging from 59 to 65 µm in diameter.
Occurrence.--In the Bocaina Formation, L. crassa occurs in a single level of phosphatic nodules in shale in TR6 (42.15 m; Table 1). L. crassa is very widespread in Proterozoic and lower Paleozoic rocks (Knoll et al., 2020).
Remarks.--Jankauskas et al. (1989) proposed species identification within this polyphyletic genus based on size, wall color and texture, which we have adopted here. Leiosphaeridia crassa is recognized by the smooth, pliant walls with lanceolate folds and a modal diameter of less than 70 µm.
Leiosphaeridia minutissima (Naumova, 1949), emend. Jankauskas et al., 1989, emend. Javaux and Knoll, 2017 (Figures 5J–M).
Lectotype.--No holotype was designated by Naumova (1949). A specimen of Leiotriletes minutissimus from Naumova (1949, pl. 1, Figure 1) was designated as lectotype by Jankauskas et al. (1989, p. 75).
Emended diagnosis.--Species of Leiosphaeridia characterized by thin, smooth walls, with sinuous folds and a modal diameter less than 70 µm.
Material.--Eight specimens, four found in palynological residues of phosphatic microbialites in two levels from TR2 (GP5T/2558; 2570), one found in one level of phosphatic microbialites in TR3 (GP5T/2561) and three from a single level of phosphatic nodules in shale from TR6 (GP5T/2567).
Description.--As for diagnosis. Specimens range from 23 to 68 µm in diameter.
Occurrence.--In the Bocaina Formation, L. minutissima occurs in phosphatic microbialites in two levels of TR2 (68.27 and 68.15 m, Table 1), one level of phosphatic microbialites in TR3 (14.81 m, Table 1) and in one level of phosphatic nodules in shale from TR6 (46.5 m; Table 1). L. minutissima generally dominates Upper Ediacaran deposits (575–542 Ma) (Grey et al., 2003; Huntley et al., 2006; Gaucher and Sprechmann, 2009). It is also abundant in the overlying Tamengo Formation (Gaucher et al., 2003) and widespread in the Nama Group (Germs et al., 1986) and Arroyo del Soldado Group (Uruguay) in Western Gondwana.
Remarks.--Species of Leiosphaeridia minutissima are characterized by thin, smooth walls, with sinuous folds and a modal diameter less than 70 µm. L. minutissima from the Bocaina Formation is more common in phosphatic microbialites in TR2 and TR3 and less common in phosphatic nodules in shale from TR6 (Table 1). Specimens from phosphatic nodules in shale have a translucent wall, but dark spots randomly dispersed over the surface that appears to be adherent granular amorphous organic matter (Figure 5J).
Genus Megasphaera Chen and Liu, 1986, emend. Xiao et al., 2014.
Type species.--Megasphaera inornata Chen and Liu, 1986, emend. Xiao et al., 2014.
Megasphaera-like (Figure 5N).
Material.--One vesicle classified as aff. Megasphaera in a petrographic thin-section of phosphatic microbialite (GP5T/2570).
Description.--A single specimen consisting of a large spheroidal vesicle, 296 µm in diameter, without processes, enclosing well-defined to diffuse spheroidal structures from 35 to 41 µm in diameter interpreted as internal cells.
Occurrence.--In the Bocaina Formation, the specimen occurs only in a phosphatic microbialite in drill-core DD004 (12.5 m, Table 1). Megasphaera inornata was recognized first in the mid-to late Ediacaran Doushantuo Formation, China (Chen and Liu, 1986; Xiao et al., 2014), and more recently in younger deposits immediately preceding the transition to the Cambrian, in the Khensen Formation, Mongolia (Anderson et al., 2019) and Kessyusa Group, central Arctic Siberia (Grazhdankin et al., 2020).
Remarks.--Megasphaera is recognized by the large spherical vesicles, smooth or sculptured, but without long processes. One or more cells may be enclosed within the vesicle. The only specimen in the Bocaina Formation is degraded and found in a thin section of phosphatic microbialite. It would not have survived, had the rock been subjected to acid maceration. While the vesicle margin and several internal cells are well defined at its lower border (Figure 5K), the other cells are more diffuse with less clearly defined borders. The poor preservation of the specimen does not permit speculation about its biological affinities.
Genus Synsphaeridium Eisenack, 1965.
Synsphaeridium sp. (Figure 5O).
Material.--Two well-preserved clusters of five and six specimens each in palynological residues of phosphatic microbialites, one in TR2 and other in TR3 (GP5T/2560; 2561).
Description.--Two small compact clusters of five contiguous psilate cells that range from 12 to 20 µm in diameter. Clusters are 29.6 and 51.8 µm in greatest dimension.
Occurrence.-- Synsphaeridium is rare in the Bocaina Formation, occurring only in one level of phosphatic microbialites in TR2 and TR3 (68.05 and 14.1 m, respectively; Table 1). They are widespread in Proterozoic deposits from the mid-Neoproterozoic (Knoll et al., 2020) and early to late Cambrian (Prasad et al., 2010; Slater and Willman, 2019).
Remarks--Individual cells in the cluster are like small Leiosphaeridia (18–30 µm), but are smaller than specimens of L. crassa and L. minutissima described here. The cells are clearly tightly packed, suggesting that they were held tightly together in life.
Genus Tanarium Kolosova, 1991, emend. Moczydlowska et al., 1993, emend. Grey, 2005.
Type species.--Tanarium conoideum Kolosova, 1991.
Holotype.--PMU-Sib.4-J/30/3, Moczydlowska et al., 1993, Figure 10C–D, p. 514.
Tanarium sp. (Figures 5P–Q).
Material.--Four specimens, one in a thin section (GP5T/2572) of phosphatic microbialite from drill-core DD004 (21 m), two from the palynological residues of two levels of phosphatic microbialites in TR2 (GP5T/2557; 2558) and one in a single level of phosphatic nodule in shale from TR6 (GP5T/2565).
Description.--Spheroidal vesicles 73–90 µm in diameter, bearing sparse (3-7 along perimeter) prominent simple processes with hook-like terminations, ranging from 5 to 14 µm long and 11–20 µm wide at base, which may be hidden within degraded granular to amorphous and dark marginal organic matter.
Occurrence.--In the Bocaina Formation, one specimen occurs in a phosphatic microbialite in drill-core DD004 (21 m), two occur in two levels of phosphatic microbialites from TR2 (68.27 and 68.15 m) and one in phosphatic nodules in shale from TR6 (10.07 m). Tanarium is widely distributed in the Ediacaran Khamaka Formation, Siberian Platform (Moczydłowska, 2016), lower Ediacaran Ura Formation, East Siberia (Moczydłowska and Nagovitsin, 2012), middle Ediacaran Vychegda Formation, East European Platform, Russia, Ediacaran Ungoolya Group, Australia (Willman et al., 2006), Ediacaran Dey Dey Mudstone and Tanana Formation (Willman and Moczydłowska, 2008), and Ediacaran Doushantuo Formation (Xiao et al., 2014).
Remarks.--The genus Tanarium hosts acanthomorphs characterized by generally long (process length 12–50% and typically >20% of vesicle diameter) and more variable or somewhat heteromorphic processes (Moczydłowska et al., 1993; Grey, 2005; Moczydłowska, 2005). Specimens described here are usually dark (Figure 5Q), with the exception of the specimen shown in the petrographic thin section (Figure 5P). The poor preservation observed in some recovered specimens led us to identify them as Tanarium only at the level of the genus. Degraded specimens from the Bocaina Formation are similar to the published figures in Moczydłowska 2016 (Plate 3.1-2). However, the ornamentation of the specimen shown in our Figure 5N, preserved in 3 dimensions, clearly resembles specimens of T. tuberosum shown in Moczydłowska 2016 (Plate 3.3-6). For these reasons, the specimens described here are identified as Tanarium sp. All the specimens recovered via palynological maceration are mostly opaque with degraded granular to amorphous, translucent organic matter around the vesicles.
Incertae Sedis
Morphotype 1 (Figures 5R–T).
Material.--Two specimens, one in a thin-section of phosphatic microbialite (GP5T/2556) and the other from a palynological residue of a phosphatic microbialite (GP5T/2560).
Description.--Double-walled spheroidal vesicle. Outer walls are 231 and 16 µm in diameter and the inner walls are 140 and 12.5 µm, respectively. Scattered cylindrical processes from 3 to 22 µm long on the inner wall are recognizable in both palynological slide and petrographic thin section.
Occurrence.--In the Bocaina Formation, one specimen was recovered from a phosphatic microbialite from drill core DD004 (19 m) and the other from a phosphatic microbialite in TR2 (68.05 m) (Table 1).
Remarks.--Distosphaera speciosa Zhang et al., 1998 are recognized by the spheroidal vesicles characterized by two wall layers, both of which bear processes. The outer wall has sparsely but evenly distributed conical processes, which are sharply pointed at the apex, hollow and open at the base. The inner wall bears long, slender, solid processes, which connect to and support the outer wall. Specimens from the Bocaina Formation vary in size, but still closely comparable to D. speciosa from the Doushantuo Formation (Zhang et al., 1998) having an outer wall, easily visible in a confocal image in Figure 5P. However, scattered sharply conical processes in the outer vesicles in specimens from the Bocaina Formation are not recognizable, preventing this identification. More specimens may help to interpret these specimens within a new genus.
Data Availability Statement
The original contributions presented in the study are included in the article/supplementary material, further inquiries can be directed to the corresponding author.
Author Contributions
LM: Conceptualization; LM, BF, IR, ES, AM, and EA: Data collection; LM, TF, BF, DL, and JL Data analysis and interpretation; JL and RT: Supervision Funding acquisition and Project administration. All authors discussed the results and contributed to the final manuscript.
Funding
The study was funded by the São Paulo Research Foundation (FAPESP) (grants #2015/16235-2; #2016/05937-9; #2016/06114-6; #2017/22099-0) and financed in part by the Coordenação de Aperfeiçoamento de Pessoal de Nível Superior–Brasil (CAPES—Finance Code 001).
Conflict of Interest
Author EA was employed by EDEM, (Empresa De Desenvolvimento em Mineração e Participações Ltda).
The remaining authors declare that the research was conducted in the absence of any commercial or financial relationships that could be construed as a potential conflict of interest.
Publisher’s Note
All claims expressed in this article are solely those of the authors and do not necessarily represent those of their affiliated organizations, or those of the publisher, the editors and the reviewers. Any product that may be evaluated in this article, or claim that may be made by its manufacturer, is not guaranteed or endorsed by the publisher.
Acknowledgments
The authors thank the EDEM mining company (Empresa de Desenvolvimento em Mineração e Participações Ltda.) for access to the drill-cores and trenches, specially L. Nery, the Research Unit in Astrobiology (NAP/Astrobio–PRP/USP), the Centro de Pesquisas, Desenvolvimento e Inovação Leopoldo Américo Miguez de Mello (CENPES/PETROBRÁS) for support with the Confocal images acquisitions, and the Institute of Geoscience (University of São Paulo) for institutional support. We also thanks the insightful discussion with S. Xiao (Virgínia-Tech University) and the reviewers Q. Ouyang and S. Willman.
References
Adorno, R. R., do Carmo, D. A., Germs, G., Walde, D. H. G., Denezine, M., Boggiani, P. C., et al. (2017). Cloudina Lucianoi (Beurlen & Sommer, 1957), Tamengo Formation, Ediacaran, Brazil: Taxonomy, Analysis of Stratigraphic Distribution and Biostratigraphy. Precambrian Res. 301, 19–35. doi:10.1016/j.precamres.2017.08.023
Almeida, F. F. M. (1965). Geologia da Serra da Bodoquena (Mato Grosso), Brasil. Boletim da Divisão de Geologia e Mineralogia, Departamento Nacional de Produção Mineral. DNPM 219, 1–96.
Almeida, F. F. M. (1945). Geologia Do sudoeste mato-grossense: Boletim da Divisão de Geologia e Mineralogia, Departamento Nacional de Produção Mineral. DNPM 116, 1–118.
Almeida, F. F. M. (1946). Origem dos minérios de ferro e manganês de Urucum: Boletim da Divisão de Geologia e Mineralogia. DNPM 119, 1–58. Departamento Nacional de Produção Mineral.
Amorim, K. B., Afonso, J. W. L., Leme, J. d. M., Diniz, C. Q. C., Rivera, L. C. M., Gómez‐Gutiérrez, J. C., et al. (2020). Sedimentary Facies, Fossil Distribution and Depositional Setting of the Late Ediacaran Tamengo Formation (Brazil). Sedimentology 67 (7), 3422–3450. doi:10.1111/sed.12749
Anderson, R. P., Macdonald, F. A., Jones, D. S., McMahon, S., and Briggs, D. E. G. (2017). Doushantuo-type Microfossils from Latest Ediacaran Phosphorites of Northern Mongolia. Geology 45 (12), 1079–1082. doi:10.1130/G39576.1
Anderson, R. P., McMahon, S., Macdonald, F. A., Jones, D. S., and Briggs, D. E. G. (2019). Palaeobiology of Latest Ediacaran Phosphorites from the Upper Khesen Formation, Khuvsgul Group, Northern Mongolia. J. Syst. Palaeontology 17 (6), 501–532. doi:10.1080/14772019.2018.1443977
Boggiani, P. C., Alvarenga, C. D., and Mantesso-Neto, V. (2004). “Faixa Paraguai. Geologia Do Continente Sul-Americano,” in Evolução da Obra de Fernando Flávio Marques de Almeida: São Paulo (São Paulo: Beca), 113–120.
Boggiani, P. C., Gaucher, C., Sial, A. N., Babinski, M., Simon, C. M., Riccomini, C., et al. (2010). Chemostratigraphy of the Tamengo Formation (Corumbá Group, Brazil): A Contribution to the Calibration of the Ediacaran Carbon-Isotope Curve. Precambrian Res. 182 (4), 382–401. doi:10.1016/j.precamres.2010.06.003
Butterfield, N. J. (2015). Early Evolution of the Eukaryota. Palaeontology 58 (1), 5–17. doi:10.1111/pala.12139
Campanha, G. A. d. C., Boggiani, P. C., Sallun Filho, W., Sá, F. R. d., Zuquim, M. d. P. S., and Piacentini, T. (2011). A faixa de dobramento Paraguai na Serra da Bodoquena e depressão Do Rio Miranda, Mato Grosso Do Sul. Geol. Usp, Sér. Cient. 11 (3), 79–96. doi:10.5327/Z1519-874X2011000300005
Chen, M., and Liu, K. (1986). The Geological Significance of Newly Discovered Microfossils from the Upper Sinian (Doushantuo Age) Phosphorites. Chin. J. Geology. 21 (1), 46–53.
Condon, D., Zhu, M., Bowring, S., Wang, W., Yang, A., and Jin, Y. (2005). U-pb Ages from the Neoproterozoic Doushantuo Formation, China. Science 308 (5718), 95–98. doi:10.1126/science.1107765
Dorr, J. V. N. (1945). Manganese and Iron Deposits of Morro Do Urucum, Mato Grosso, Brazil. Bull. United States Geol. Surv. 946A, 1–47.
Downie, C., Evitt, R., and Sarjeant, W. A. S. (1963). Dinoflagellates, Hystrichospheres and the Classification of Acritarchs. Stanford Univ. Publications Geol. Sci. 7 (3), 1–16.
Downie, C. (1982). Lower Cambrian Acritarchs from Scotland, Norway, Greenland and Canada. Trans. R. Soc. Edinb. Earth Sci. 72 (4), 257–285. doi:10.1017/S0263593300010051
Downie, C., and Sarjeant, W. A. S. (1963). On the Interpretation and Status of Some Hystrichosphere Genera. Palaeontology 6 (1), 83–96.
Eisenack, A. (1965). Mikrofossilien aus dem Silur Gotlands. Hystrichosphären Problematika. Neues Jahrb. Geol. Paläontol. Abh. 122, 257–274.
Eisenack, A. (1958). Tasmanites Newton 1975 und Leiosphaeridia n.g. als Gattungen der Hystrichosphaeridia. Paleontographica Abt. A. 110, 1–19.
Evitt, W. R. (1963). A Discussion and Proposals Concerning Fossil Dinoflagellates, Hystrichospheres, and Acritarchs, I. Proc. Natl. Acad. Sci. 49 (2), 158–164. doi:10.1073/pnas.49.2.158
Fedonkin, M. A., and Waggoner, B. M. (1997). The Late Precambrian Fossil Kimberella Is a Mollusc-like Bilaterian Organism. Nature 388 (6645), 868–871. doi:10.1038/42242
Folk, R. L. (1987). Detection of Organic Matter in Thin-Sections of Carbonate Rocks Using a white Card. Sediment. Geology. 54 (3), 193–200. doi:10.1016/0037-0738(87)90022-4
Frei, R., Døssing, L. N., Gaucher, C., Boggiani, P. C., Frei, K. M., Bech Árting, T., et al. (2017). Extensive Oxidative Weathering in the Aftermath of a Late Neoproterozoic Glaciation - Evidence from Trace Element and Chromium Isotope Records in the Urucum District (Jacadigo Group) and Puga Iron Formations (Mato Grosso Do Sul, Brazil). Gondwana Res. 49, 1–20. doi:10.1016/j.gr.2017.05.003
Freitas, B. T., Rudnitzki, I. D., Morais, L., Campos, M. D. R., Almeida, R. P., Warren, L. V., et al. (2021). Cryogenian Glaciostatic and Eustatic Fluctuations and Massive Marinoan-Related Deposition of Fe and Mn in the Urucum District, Brazil. Geology 49, 1478–1483. doi:10.1130/G49134.1
Freitas, B. T., Warren, L. V., Boggiani, P. C., De Almeida, R. P., and Piacentini, T. (2011). Tectono-sedimentary Evolution of the Neoproterozoic BIF-Bearing Jacadigo Group, SW-Brazil. Sediment. Geology. 238 (1-2), 48–70. doi:10.1016/j.sedgeo.2011.04.001
Gaucher, C., Boggiani, P. C., Sprechmann, P., Sial, A., and Fairchild, T. R. (2003). Integrated correlation of the Vendian to Cambrian Arroyo del Soldado and Corumbá Groups (Uruguay and Brazil): palaeogeographic, palaeoclimatic and palaeobiologic implications. Precambrian Res. 120 (3-4), 241–278. doi:10.1016/S0301-9268(02)00140-7
Gaucher, C., and Sprechmann, P. (2009). Chapter 9.1 Neoproterozoic Acritarch Evolution. Dev. Precambrian Geology. 16, 319–326. doi:10.1016/S0166-2635(09)01622-3
Gauthier-Lièvre, L., and Thomas, R. (1960). Le Genre Cucurbitella Pènard. Arch. Protistenk 104, 569–602.
Germs, G. J. B., Knoll, A. H., and Vidal, G. (1986). Latest Proterozoic Microfossils from the Nama Group, Namibia (South West Africa). Precambrian Res. 32 (1), 45–62. doi:10.1016/0301-9268(86)90029-X
Golubkova, E. Y., Zaitseva, T. S., Kuznetsov, A. B., Dovzhikova, E. G., and Maslov, A. V. (2015). Microfossils and Rb-Sr Age of Glauconite in the Key Section of the Upper Proterozoic of the Northeastern Part of the Russian Plate (Keltmen-1 Borehole). Dokl. Earth Sc. 462 (2), 547–551. ISSN 1028334X. doi:10.1134/s1028334x15060045
Gomaa, F., Lahr, D. J. G., Todorov, M., Li, J., and Lara, E. (2017). A Contribution to the Phylogeny of Agglutinating Arcellinida (Amoebozoa) Based on SSU rRNA Gene Sequences. Eur. J. Protistology 59, 99–107. doi:10.1016/j.ejop.2017.03.005
Grazhdankin, D., Nagovitsin, K., Golubkova, E., Karlova, G., Kochnev, B., Rogov, V., et al. (2020). Doushantuo-Pertatataka-type Acanthomorphs and Ediacaran Ecosystem Stability. Geology 48 (7), 708–712. doi:10.1130/G47467.1
Grey, K., and Calver, C. R. (2007). Correlating the Ediacaran of Australia. Geol. Soc. Lond. Spec. Publications 286 (1), 115–135. doi:10.1144/SP286.8
Grey, K. (2005). Ediacaran Palynology of Australia, 31. (Hornsby: Memoir, Association of Australasian Palaeontologists), 439.
Grey, K., Walter, M. R., and Calver, C. R. (2003). Neoproterozoic Biotic Diversification: Snowball Earth or Aftermath of the Acraman Impact. Geol 31 (5), 459–462. doi:10.1130/0091-7613(2003)031<0459:nbdseo>2.0.co;2
Hermann, T. N., and Podkovyrov, V. N. (2006). Fungal Remains from the Late Riphean. Paleontol. J. 40 (2), 207–214. ISSN 0031-0301. doi:10.1134/s0031030106020122
Hofmann, H. J. (1971). Polygonomorph Acritarch from the Gunflint Formation (Precambrian), Ontario. J. Paleontol. 45 (3), 522–524. https://www.jstor.org/stable/1302699.
Huntley, J., Xiao, S., and Kowalewski, M. (2006). 1.3 Billion Years of Acritarch History: An Empirical Morphospace Approach. Precambrian Res. 144 (1-2), 52–68. doi:10.1016/j.precamres.2005.11.003
Jankauskas, T., Mikhailova, N., and Hermann, T. N. (1989). Precambrian Microfossils of the USSR. Leningrad: Nauka, 1–191. [in Russian].
Javaux, E. J., and Knoll, A. H. (2017). Micropaleontology of the Lower Mesoproterozoic Roper Group, Australia, and Implications for Early Eukaryotic Evolution. J. Paleontol. 91 (2), 199–229. doi:10.1017/jpa.2016.124
Knoll, A. H. (2003). Biomineralization and Evolutionary History. Rev. mineralogy Geochem. 54 (1), 329–356. doi:10.2113/0540329
Knoll, A. H., Germs, G. J. B., Tankard, A., and Welsink, H. (2020). Tonian Microfossils from Subsurface Shales in Botswana. Precambrian Res. 345, 105779. doi:10.1016/j.precamres.2020.105779
Kolosova, S. P. (1991). Late Precambrian Spiny Microfossils from the Eastern Part of the Siberian Platform. Algologia 1 (1991), 53–59. [in Russian].
Lahr, D. J. G., Kosakyan, A., Lara, E., Mitchell, E. A. D., Morais, L., Porfirio-Sousa, A. L., et al. (2019). Phylogenomics and Morphological Reconstruction of Arcellinida Testate Amoebae Highlight Diversity of Microbial Eukaryotes in the Neoproterozoic. Curr. Biol. 29 (6), 991–1001. doi:10.1016/j.cub.2019.01.078
Liu, P., and Moczydlowska, M. (2019). Ediacaran Microfossils from the Doushantuo Formation Chert Nodules in the Yangtze Gorges Area, South China, and New Biozones. John Wiley & Sons, 172.
Maciel, P. (1959). Tilito Cambriano (?) no Estado de Mato Grosso. Boletim da Sociedade Brasileira de Geologia. São Paulo 8 (1), 31–39.
McGee, B., Babinski, M., Trindade, R., and Collins, A. S. (2018). Tracing Final Gondwana Assembly: Age and Provenance of Key Stratigraphic Units in the Southern Paraguay Belt, Brazil. Precambrian Res. 307, 1–33. doi:10.1016/j.precamres.2017.12.030
McKirdy, D. M., Webster, L. J., Arouri, K. R., Grey, K., and Gostin, V. A. (2006). Contrasting Sterane Signatures in Neoproterozoic marine Rocks of Australia before and after the Acraman Asteroid Impact. Org. Geochem. 37 (2), 189–207. doi:10.1016/j.orggeochem.2005.09.005
Moczydłowska, M. (1991). Acritarch Biostratigraphy of the Lower Cambrian and the Precambrian–Cambrian Boundary in southeastern Poland. Fossils and Strata 29, 127.
Moczydłowska, M. (2016). Algal Affinities of Ediacaran and Cambrian Organic-Walled Microfossils with Internal Reproductive bodies:Tanariumand Other Morphotypes. Palynology 40 (1), 83–121. doi:10.1080/01916122.2015.1006341
Moczydłowska, M., and Nagovitsin, K. E. (2012). Ediacaran Radiation of Organic-Walled Microbiota Recorded in the Ura Formation, Patom Uplift, East Siberia. Precambrian Res. 198, 1–24. doi:10.1016/j.precamres.2011.12.010
Moczydłowska, M. (2005). Taxonomic Review of Some Ediacaran Acritarchs from the Siberian Platform. Precambrian Res. 136, 283–307. doi:10.1016/j.precamres.2004.12.001
Moczydłowska, M. (2011). The Early Cambrian Phytoplankton Radiation: Acritarch Evidence from the Lükati Formation, Estonia. Palynology 35 (1), 103–145. doi:10.1080/01916122.2011.552563
Moczydlowska, M. (2008). The Ediacaran Microbiota and the Survival of Snowball Earth Conditions. Precambrian Res. 167, 1–15. doi:10.1016/j.precamres.2008.06.008
Moczydłowska, M., Vidal, G., and Rudavskaya, V. A. (1993). Neoproterozoic (Vendian) Phytoplankton from the Siberian Platform, Yakutia. Palaeontology 36, 495–521.
Morais, L., Fairchild, T. R., Lahr, D. J. G., Rudnitzki, I. D., Schopf, J. W., Garcia, A. K., et al. (2017). Carbonaceous and Siliceous Neoproterozoic Vase-Shaped Microfossils (Urucum Formation, Brazil) and the Question of Early Protistan Biomineralization. J. Paleontol. 91 (3), 393–406. doi:10.1017/jpa.2017.16
Naumova, S. N. (1949). Spores of the Lower Cambrian. Izvestiya Akademii Nauk SSSR, Seriya Geologicheskaya 4, 49–56.
Naumova, S. N. (1960). “Sporogo-pyltsevye Kompleksy Rifejskikh I Nizhnekembrijskikh Otlozhenij SSSR (Spore-Pollen Assemblages of the Riphean and Lower Cambrian Deposits in the USSR),” in Stratigrafiya Pozdnego Dokembriya I Kembriya, Problem 8 (Stratigraphy of the Late Precambrian and Cambrian, Problem 8, International Geological Congress 21 Session. Reports of the Soviet Geologists) (Moscow: Akademia Nauk SSSR), 109–117.
Owen, G., and Jones, E. E. (1976). Nebela Tuberculatacomb. Nov. (Arcellinida), its History and Ultrastructure. The J. Protozoology 23 (4), 485–487. doi:10.1111/j.1550-7408.1976.tb03819.x
Pacheco, M. L. A. F., Galante, D., Rodrigues, F., de M. Leme, J., Bidola, P., Hagadorn, W., et al. (2015). Insights into the Skeletonization, Lifestyle, and Affinity of the Unusual Ediacaran Fossil Corumbella. PLoS One 10 (3), e0114219. doi:10.1371/journal.pone.0114219
Parry, L. A., Boggiani, P. C., Condon, D. J., Garwood, R. J., Leme, J. d. M., McIlroy, D., et al. (2017). Ichnological Evidence for Meiofaunal Bilaterians from the Terminal Ediacaran and Earliest Cambrian of Brazil. Nat. Ecol. Evol. 1 (10), 1455–1464. doi:10.1038/s41559-017-0301-9
Porter, S. M. (2004). Closing the Phosphatization Window: Testing for the Influence of Taphonomic Megabias on the Pattern of Small Shelly Fossil Decline. Palaios 19, 178–183. doi:10.1669/0883-1351(2004)019<0178:ctpwtf>2.0.co;2
Porter, S. M., and Riedman, L. A. (2016). Systematics of Organic-Walled Microfossils from the Ca. 780-740 Ma Chuar Group, Grand Canyon, Arizona. J. Paleontol. 90 (5), 815–853. doi:10.1017/jpa.2016.57
Prasad, B., Asher, R., and Borgohai, B. (2010). Late Neoproterozoic (Ediacaran)-Early Paleozoic (Cambrian) Acritarchs from the Marwar Supergroup, Bikaner-Nagaur Basin, Rajasthan. J. Geol. Soc. India 75 (2), 415–431. doi:10.1007/s12594-010-0038-4
Rud’ko, S. V., Petrov, P. Y., Kuznetsov, A. B., Shatsillo, A. V., and Petrov, O. L. (2017). Refined δ13С Trend of the Dal'nyaya Taiga Series of the Ura Uplift (Vendian, Southern Part of Middle Siberia). Dokl. Earth Sc. 477 (2), 1449–1453. doi:10.1134/S1028334X17120182
Schopf, J. W., Kudryavtsev, A. B., Agresti, D. G., Czaja, A. D., and Wdowiak, T. J. (2005). Raman Imagery: a New Approach to Assess the Geochemical Maturity and Biogenicity of Permineralized Precambrian Fossils. Astrobiology 5 (3), 333–371. doi:10.1089/ast.2005.5.333
Slater, B. J., and Willman, S. (2019). Early Cambrian Small Carbonaceous Fossils (SCF S) from an Impact Crater in Western Finland. Lethaia 52 (4), 570–582. doi:10.1111/let.12331
Turner, R. E. (1984). Acritarchs from the Type Area of the Ordovician Caradoc Series, Shropshire, England. Palaeontographica Abteilung B Paläophytologie 190 (4-6), 87–157.
Van Iten, H., Burkey, M., Leme, J. D. M., and Marques, A. C. (2014). Cladistics and Mass Extinctions: the Example of Conulariids (Scyphozoa, Cnidaria) and the End Ordovician Extinction Event. Gff 136 (1), 275–280. doi:10.1080/11035897.2014.880506
Vidal, G., and Moczydłowska-Vidal, M. (1997). Biodiversity, Speciation, and Extinction Trends of Proterozoic and Cambrian Phytoplankton. Paleobiology 23 (2), 230–246. doi:10.1017/S0094837300016808
Volkova, N. A. (1968). “Acritarchs from the Precambrian and Lower Cambrian Deposits of Estonia,” in Problematic Riphean-Cambrian Layers of the Russian Platform, the Urals and Kazakhstan. Trudy Geologischeskogo Instituta. Editors N. A. Volkova, Z. A. Zhuravleva, V. E. Zabrodin, and B. Klinger (Moscow: Akademia Nauk SSSR, Nauka), 188, 8–36.
Vorob'eva, N. G., Sergeev, V. N., and Knoll, A. H. (2007). “Microfossil Assemblages from the Vychegda Formation of the East European Platform Passive Margin—A Biostratigraphic Model for the Upper Riphean (Cryogenian)/Vendian (Ediacaran) Boundary,” in The Rise and Fall of the Vendian (Ediacaran) Biota Origin of the Modern Biosphere Transaction of the International Conference on the IGCP Project, Moscow GEOS, 493. 42–46.
Vorob'eva, N. G., Sergeev, V. N., and Knoll, A. H. (2009). Neoproterozoic Microfossils from the Margin of the East European Platform and the Search for a Biostratigraphic Model of Lower Ediacaran Rocks. Precambrian Res. 173, 163–169. doi:10.1016/j.precamres.2009.04.001
Willman, S., and Moczydłowska, M. (2008). Ediacaran Acritarch Biota from the Giles 1 Drillhole, Officer Basin, Australia, and its Potential for Biostratigraphic Correlation. Precambrian Res. 162 (3-4), 498–530. doi:10.1016/j.precamres.2007.10.010
Willman, S., Moczydłowska, M., and Grey, K. (2006). Neoproterozoic (Ediacaran) Diversification of Acritarchs-A New Record from the Murnaroo 1 Drillcore, Eastern Officer Basin, Australia. Rev. Palaeobotany Palynology 139 (1-4), 17–39. doi:10.1016/j.revpalbo.2005.07.014
Xiao, S., and Knoll, A. H. (2000). Phosphatized Animal Embryos from the Neoproterozoic Doushantuo Formation at Weng'an, Guizhou, South China. J. Paleontol. 74 (5), 767–788. doi:10.1017/S002233600003300X10.1666/0022-3360(2000)074<0767:paeftn>2.0.co;2
Xiao, S., and Narbonne, G. M. (2020). “The Ediacaran Period,” in Geologic Time Scale 2020 (Elsevier), 41. doi:10.1016/b978-0-12-824360-2.00018-8
Xiao, S., Zhou, C., Liu, P., Wang, D., and Yuan, X. (2014). Phosphatized Acanthomorphic Acritarchs and Related Microfossils from the Ediacaran Doushantuo Formation at Weng'an (South China) and Their Implications for Biostratigraphic Correlation. J. Paleontol. 88 (1), 1–67. doi:10.1666/12-157R
Yin, L. (1987). Microbiotas of Latest Precambrian Sequences in China. Stratigr. Palaeontology Systemic Boundaries China, Precambrian-Cambrian Boundary 1, 415–494.
Zaine, M. F., and Fairchild, T. R. (1987). “Novas considerações sobre os fósseis da Formação Tamengo, Grupo Corumbá, SW Brasil,” in Congresso Brasileiro de Paleontologia, 10, Resumos das comunicações (Rio de Janeiro: Sociedade Brasileira de Paleontologia), 54. [in Portuguese].
Zang, Z. W., and Walter, M. R. (1992). Late Proterozoic and Cambrian Microfossils and Biostratigraphy, Amadeus basin, central Australia, 12. (Hornsby: Memoir, Association of Australasian Palaeontologists), 132.
Zhang, Y., Yin, L., Xiao, S., and Knoll, A. H. (1998). Permineralized Fossils from the Terminal Proterozoic Doushantuo Formation, south China. J. Paleontol. 72 (4), 1–52. https://www.jstor.org/stable/1315592.
Keywords: acanthomorph acritarchs, phosphatized microfossils, Neoproterozoic, Paraguay belt, Ediacaran
Citation: Morais L, Fairchild TR, Freitas BT, Rudnitzki ID, Silva EP, Lahr D, Moreira AC, Abrahão Filho EA, Leme JM and Trindade RIF (2021) Doushantuo-Pertatataka—Like Acritarchs From the Late Ediacaran Bocaina Formation (Corumbá Group, Brazil). Front. Earth Sci. 9:787011. doi: 10.3389/feart.2021.787011
Received: 30 September 2021; Accepted: 23 November 2021;
Published: 09 December 2021.
Edited by:
Haijun Song, China University of Geosciences Wuhan, ChinaReviewed by:
Qing Ouyang, Nanjing Institute of Geology and Palaeontology (CAS), ChinaSebastian Willman, Department of Earth Sciences, Uppsala University, Sweden
Copyright © 2021 Morais, Fairchild, Freitas, Rudnitzki, Silva, Lahr, Moreira, Abrahão Filho, Leme and Trindade. This is an open-access article distributed under the terms of the Creative Commons Attribution License (CC BY). The use, distribution or reproduction in other forums is permitted, provided the original author(s) and the copyright owner(s) are credited and that the original publication in this journal is cited, in accordance with accepted academic practice. No use, distribution or reproduction is permitted which does not comply with these terms.
*Correspondence: L. Morais, Moraisluanamoraiss@usp.br