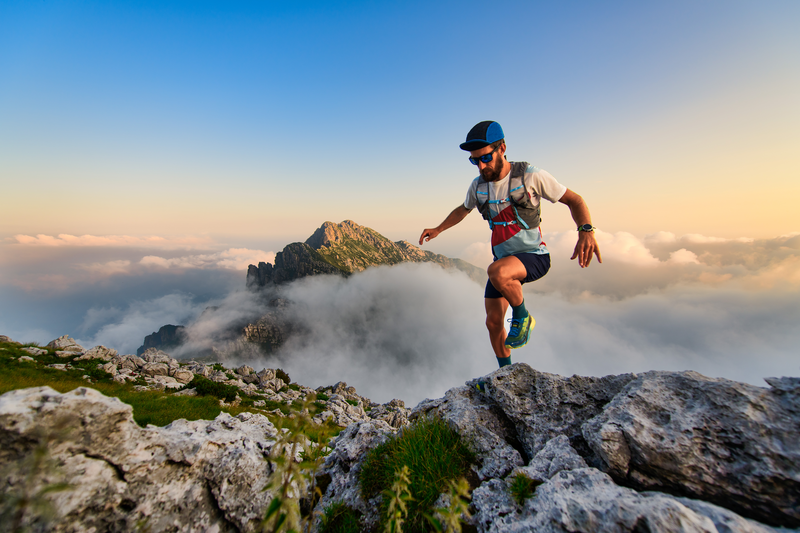
94% of researchers rate our articles as excellent or good
Learn more about the work of our research integrity team to safeguard the quality of each article we publish.
Find out more
REVIEW article
Front. Earth Sci. , 14 December 2021
Sec. Volcanology
Volume 9 - 2021 | https://doi.org/10.3389/feart.2021.777548
This article is part of the Research Topic External Forcing on Volcanoes and Volcanic Processes: Observations, Analysis and Implications View all 12 articles
In this paper, we examine the origins and the history of the hypothesis for an influence of tidal forces on volcanic activity. We believe that exploring this subject through a historical perspective may help geoscientists gain new insights in a field of research so closely connected with the contemporary scientific debate and often erroneously considered as a totally separated niche topic. The idea of an influence of the Moon and Sun on magmatic processes dates back to the Hellenistic world. However, it was only since the late 19th century, with the establishment of volcano observatories at Mt. Etna and Vesuvius allowing a systematic collection of observations with modern methods, that the “tidal controversy” opened one of the longest and most important debates in Earth Science. At the beginning of the 20th century, the controversy assumed a much more general significance, as the debate around the tidal influence on volcanism developed around the formulation of the first modern theories on the origins of volcanism, the structure of the Earth’s interior and the mechanisms for continental drift. During the same period, the first experimental evidence for the existence of the Earth tides by Hecker (Beobachtungen an Horizontalpendeln über die Deformation des Erdkörpers unter dem Einfluss von Sonne und MondVeröffentlichung des Königl, 1907, 32), and the Chamberlin–Moulton planetesimal hypothesis (proposed in 1905 by geologist Thomas Chrowder Chamberlin and astronomer Forest Ray Moulton) about the “tidal” origin of the Solar System, influenced and stimulated new researches on volcano-tides interactions, such as the first description of the “lava tide” at the Kilauea volcano by Thomas Augustus Jaggar in 1924. Surprisingly, this phase of gradual acceptance of the tidal hypothesis was followed by a period of lapse between 1930 to late 1960. A new era of stimulating and interesting speculations opened at the beginning of the seventies of the 20th century thanks to the discovery of the moonquakes revealed by the Apollo Lunar Surface Experiment Package. A few years later, in 1979, the intense volcanism on the Jupiter’s moon Io, discovered by the Voyager 1 mission, was explained by the tidal heating produced by the Io’s orbital eccentricity. In the last part of the paper, we discuss the major advances over the last decades and the new frontiers of this research topic, which traditionally bears on interdisciplinary contributions (e.g., from geosciences, physics, astronomy). We conclude that the present-day debate around the environmental crisis, characterized by a large collection of interconnected variables, stimulated a new field of research around the complex mechanisms of mutual interactions among orbital factors, Milankovitch Cycles, climate changes and volcanism.
In the present review, we will see how the tidal controversy reflected not only the level of contemporary scientific debate but it also stimulated interest and new perspectives on a variety of fields of research including geosciences, climatology, physics and astronomy. In fact, since the beginning, arguments either in favor or against an influence of tidal forces on volcanism were intertwined with important scientific “revolutions”, which greatly influenced man’s view about the origin of the Earth and of the Solar System. In ancient times, during the VI century BC, Greek philosophers from the Ionian school, beyond the mythological and religious beliefs of their times, attempted a more rational interpretation of natural phenomena, including tides and volcanic eruptions. Under the influence of Anaxagoras (V century BC), Plato in his dialogue Phaedo (IV century BC) hypothesized the existence of great fire rivers and streams of mud under the Earth, flowing together with hot and cold water (Bluck, 2014). It is likely that the Plato’s idea of a network of subterranean channels feeding eruptions of mud and fire raised from the direct observation of an eruption at Mount Etna during his first travel in Sicily, in 388-387 BC. Interestingly, he recognized in the fluid nature of “the rivers of mud that flow before the lava in Sicily, and the lava itself” an explanation for a common sensitivity to “a kind of oscillation within the Earth moving all these up and down”. Here, the word “oscillation” (gr. Αiώρα, aiòra; Bluck, 2014) can be translated into “like a twist”, thus evidencing the periodic nature of the fluids motion. This is likely the first documented attempt of relating volcanic phenomena to tidal motions, thus assuming a common tidal “sensitivity” for both the hydrosphere and volcanoes. Plato’s idea was further reconsidered by Aristotle, who proposed that the periodic oscillation of subterranean fire and water may produce the compaction and the violent movement of air within subterranean channels, causing tremors, eruptions and earthquakes (a translation of Aristotle Meteorologica in Lee, 1952). The idea for a pneumatic origin of earthquakes and volcanic eruptions, due to the sea that enters and moves within hot caves, causing wind storms (Williams, 2006), was later on revised by Lucretius (I century BC) in his De rerum natura (Hine, 2002). Lucretius explained the eruptions of Mount Etna as follows: “First, the mountain’s nature is all under-hollow, propped about with caverns of basaltic piers. In all its grottos be there wind and air […] When this air is heated through and through, and, raging round, hath made the earth and all the rocks it touches horribly hot, and hath struck off from them fierce fire of swiftest flame, it lifts itself and hurtles thus straight upwards through its throat into high heaven, and thus bears on afar its burning blasts and scattereth afar its ashes, and rolls a smoke of pitchy murk and heaveth the while boulders of wondrous weight- Leaving no doubt in thee that “tis the air”st tumultuous power. Besides […] the sea there at the roots of that same mount breaks its old billows and sucks back its surf.” (translation of De rerum natura, VI 680–702 in Godwin, 1991). Later on, after a destructive earthquake occurred in the Mt. Vesuvius area in 62 or 63 CE (Hine, 1984), Seneca in his Natural Questions (for translation see e.g., Hine, 2010) interpreted the observation of “sudden tidal waves” inundating settlements and lands after earthquakes—a tsunamis event?- as an evidence for the periodic movements of a subterranean sea; the subterranean, periodic movements of this sea would cause violent movements of unhealthy air, plague, inundations, thus reaffirming the pneumatic origin of seismicity and eruptions. In support of this hypothesis, Seneca interpreted the dead of “hundreds of sheeps […] in the Pompeii area” as an evidence for the fact “that a plague commonly occurs after major earthquakes, and this is not surprising. For many causes of death are lurking deep below: the air itself can be unhealthy for those who breathe it, either through a defect in the earth, or because the air is stagnating inertly in perpetual darkness, or because of contamination by the corrupting effects of subterranean fires”. Certainly, to our modern scientific eyes, the “pneumatic” hypothesis for the origin of earthquakes and volcanic eruptions appears eccentric and deeply inadequate. However, the idea of a twisting subterranean world, where the movements of fire and water, and the related expansion of the vapor, may produce hot and unhealthy winds, earthquakes and rumbling volcanic eruptions was one of the most influencing and long-lasting views at least since the second half of the 18th century. In fact, the atmospheric and environmental effects of the Icelandic 1783–1784 Laki flood lava, which emitted ∼122 megatons of SO2 into the atmosphere and maintained a sulfuric aerosol veil that hung over the Northern Hemisphere for >5 months (Thordarson and Self, 2003), was attributed to the exhalations following the destructive Calabrian (South Italy) seismic sequences occurred in 1783 (Hamilton, 1783). Sir William Hamilton, in 1783, wrote: “Several fishermen assured me, that during the earthquake of the 5th of February at night, the sand near the sea was hot, and that they saw fire issue from the earth in many parts. The circumstance has been often repeated to me in the plain; and my idea is, that exhalations which issued during the violent commotions of the earth were full of electrical fire, just as the smoke of volcanoes is constantly observed to be during volcanic eruptions; for I saw no mark, in any part of my journey, of any volcanic matter having issued from the fissures of the earth; and I am convinced, that the whole damage has been done by exhalations and vapours only.” Yet, in the early 19th century, the Plato’s idea of subterranean fire rivers and hot currents communicating with the exterior of the globe was reported by Alexander von Humboldt (von Humboldt, 1866). However, the beginning of the 19th century was also marked by important changes in the interpretations of volcanism and seismicity. The debate between physicians, early geologists and natural philosophers about the internal state of the Earth will be fed by observations made on Vesuvius, as the early 19th century eruption was “not so small as to be contemptible not so great as to be unapproachable”, as remarked by Humphry Davy in the correspondence with Michael Faraday in 1819 (James, 1991).
In a few years, the dispute about the influence of tides on the molten interior of the Earth will be at the centre of the concomitant debate on the origin of volcanoes. The origin of this dispute will be the subject of the next paragraph.
During the 19th century, the debate around the inner structure of the Earth was fed by conflicting arguments proposed by physicians, geologists and astronomers (Brush, 1979). Within this debate, the mainstream idea for the origin of volcanic ‘fire’ and igneous rocks by combustion of coal or sulphur contrasted with the early experiments of lava remelting conducted by Lazzaro Spallanzani around 1780 (Spallanzani, 1795) who also argued that water vapor is the dominant magmatic gas causing explosions (Sigurdsson, 1999).
The idea of a burning subterranean environment appeared now inadequate, as the origin of the internal heat of the Earth required new hypotheses (Brush, 1979). These include:
• a chemical cause, produced by the reaction of oxidation of metals at depth,
• a solar origin, as the earth’s central fire would represent an ejectum from the solar furnace,
• an effect of the compression of materials by the huge pressures at the center of the Earth,
• the transit of the Earth through a hotter region of the space.
Whatever the origin of the Earth’s internal heat, the dispute during the 19th century opened two main positions as fluidists, primarily geologists, argued for a fluid interior of the Earth, whilst solidists believed that the Earth is completely solid (Brush, 1979). In this dispute, investigating the influence of tidal forces on volcanism will be of central importance to provide evidence against or in favor of the fluidist theory. André-Marie Ampère, in 1833, evidenced how tidal forces exerted by the Moon on the Earth’s enormous liquid interior would render the surface unstable (Brush, 2014):
“Those who assume the liquidity of the interior nucleus of the Earth seem to have forgotten the action which the moon would exert on this enormous liquid mass, an action which would result in tides similar to those of our oceans but much worse, as much by their extent as by the density of the liquid. It is hard to imagine how the envelope of the earth could resist the incessant beating by a kind of hydraulic lever 1,400 leagues in length.”
In 1841, the foundation of the world’s first volcano observatory at Mt. Vesuvius by King Ferdinand II of Bourbon allowed the collection of systematic observations on volcanic eruptions, which opened a new era in the scientific debate between solidism and fluidism. In 1854, Luigi Palmieri, a physicist and meteorologist, was appointed as the second Director of the Vesuvian Observatory where he developed the first model of electro-magnetic seismograph. During the 1872 eruption at Mt. Vesuvius, Palmieri observed that “precursory signals of eruptions” registered by his seismograph before and during the onset of lava flow episodes, often occurred at sygyzies (Palmieri, 1873). Interestingly, the report of the “Eruption of Vesuvius in 1872” by Palmieri is introduced by an overview on “The present state of knowledge of terrestrial volcanicity” by the fluidist Robert Mallet. In his introduction, Mallet proposes the existence, below a fifteen miles thick solid and cooled crust, of a “universal ocean of liquid material” feeding volcanoes. Also, Mallet contests the solidists’ argument (e.g., by William Hopkins, 1837) that the claimed internal fluidity of the Earth would be inconsistent with the observed precessional motion and nutation of its axis of rotation due to the combined gravitational attractions of the Moon and the Sun (Brush, 1979). Kelvin hypothesized that Earth started to cool from its original molten state, and based on the Second Law of Thermodynamics, he calculated the age of the birth of the Earth at no more than 400 million years (Burchfield, 2009). Interestingly, Kelvin added a term due to the effects of tidal friction to its thermodynamic balance of the Earth’s heat (Burchfield, 2009). On their side, solidists, by rejecting the “central fire” fluidists’ hypothesis, opposed a new paradigm: Hopkins, hypothesized the existence of “isolated lakes of liquid rock, existing at comparatively small depths beneath the Earth’s surface within the solid and relatively cold crust, each supplying its own volcano” (Palmieri, 1873). By the end of the 19th century, ancient and emerging theories about the feeding mechanisms of volcanoes, i.e., whether the result of communication between the interior and exterior of the globe or the consequence of the drainage of isolated lakes of molten material, will be tested on the grounds of systematic observations of eruptions. In 1892, Annibale Riccò, astronomer and first director of the Astronomic Observatory in Catania (Sicily), noticed a certain synchronism in the main eruptive crises at Stromboli and Etna volcanoes and hypothesized a common cause in the lunisolar action (Riccò and Arcidiacono, 1904). This hypothesis for a common feeding system shared by the two volcanoes was rejected by Giuseppe Mercalli, who also noticed nearly synchronous eruptive crises at Stromboli and Etna in 1865, 1874, 1879, and 1883, although he attributed this phenomenon to the action of a common, endogenous cause (Mercalli, 1892). Later on, in 1907, Mercalli in “The volcanoes of the Earth” confirmed his skepticism about the existence of the “central fire”, as the persistence of volcanism in a given location for “only” thousands of years suggests the progressive depletion of local feeding systems (Mercalli, 1907). The 20th century opened new perspectives, as the debate among geoscientists around the origin of volcanism will be fed by innovative monitoring techniques of active volcanoes and will be influenced by new hypothesis on the origins of Earth and Solar System. In the next paragraph, we will see how the debate around the influence of tides on volcanism will benefit from the interdisciplinary contributions of geosciences, astronomy and experimental physics.
The 20th century opened with the exposition of new theories on the origin of the Solar System with implications on the hypotheses about the formation and the inner structure of the Earth. In 1906, the geologist T. C. Chamberlin and the astronomer F. R. Moulton published the “planetesimal” or “eruptotidal” hypothesis, according to which the formation of the planets was explained by the gravitational effects of a close encounter of the Sun with another star (Brush, 1978). The new hypothesis by Chamberlin and Moulton, by replacing the nebular hypothesis for the origin of Solar System proposed, among others, by Lord Kelvin, had also a major impact in geosciences. In fact, the birth and growth of Earth was attributed by Chamberlin and Moulton to a slow process of accretion, thus explaining the terrestrial internal heat in terms of a slow “gravitational contraction”. The time-scale for Earth’s cooling hypothesized by Chamberlin, relatively longer than that predicted by the competing theories, was compatible with the presence of a fluid layer below the crust. Among other inferences on the structure of the Earth, Chamberlin hypothesized a differential motion of parts of the crust, leading, for example, to the building of mountain chains (Brush, 1978). The Chamberlin’s model was rejected by physicists like Lord Kelvin, who argued that “the average substance of the Earth is more rigid than steel” and the tidal effects on a fluid layer would have been much higher than those observed (Thomson, 1863).
From a theoretical point of view, the planetesimal theory by Chamberlin and Moulton required quantitative data on the elasticity and plasticity of the Earth to support their hypothesis with convincing arguments against the nebular hypothesis. In this perspective, Chamberlin also proposed an experimental approach to acquire new data about the elasticity of the Earth: these include the experimental determination of the Earth’s elastic properties and investigations about the effects of Earth tides on volcanism and seismicity (Gale, 1914). Interestingly, in the same period, Oskar Hecker, a German geodesist and seismologist, presented the first systematic Earth tides observations by means of a horizontal pendulum, thus determining the effective shear modulus for the Earth by direct instrumental observations (Hecker, 1907). Concerning the hypothesized effects of Earth tides on volcanism, the theory of Chamberlin and Moulton intertwined with the formation -and influenced the activity of-the volcanologist Thomas Augustus Jaggar. In 1902, Jaggar was sent by the US government to study the effects of the worst volcanic disasters of the 20th century at Soufrière and Mont Pelée. In his biography, Jaggar annotated “As I look back on the Martinique experience I know what a crucial point in my life it was....I realized that the killing of thousands of persons by subterranean machinery totally unknown to geologists...was worthy of a life work” (Jaggar, 1956). The Jaggar’s life worthy work will be the construction of the Hawaiian Volcano Observatory (HVO) where, in 1912, he started systematical measurements of the oscillations of the Kilauea lava lake. The results of the analysis of the lava lake oscillations were published in 1924 (Jaggar, 1924). Jaggar opened the introduction of his manuscript as follows: “Chamberlin has written “pronounced tidal movements might be expected in the necks of volcanoes, if they were connected with large reservoirs of lava below…”, thus acknowledging the influence of the contemporaneous scientific debate in astronomy and astrophysics on his work. Jaggar also provided a quantitative evidence for the existence of ‘lava tides’ (i.e., semidiurnal fluctuation of the Kilauea lava lake level of 2–7 feet) by the direct measurement of the fluctuation of the lava lake surface trough a telescopic alidade (Jaggar, 1924).
In the same period, since the early 1920s, the debate within the Earth Sciences was dominated by the hypotheses on the structure and motion of the Earth’s outer surface and by the controversy about the mechanism of continental drift. A comprehensive treatment of the wide scientific debate around the development of the mobilist theory (see e.g., Frankel, 2012) is beyond the aim of the present paper. For our purposes, it is worth attention that one of the main criticisms by the “fixists” to the Wegener’s hypothesis on the continental drift was actually focused on the weakness of the causal mechanism. In fact, Wegener postulated that the extensive horizontal displacements of continents can be explained by tidal forces (Frankel, 2012). Surprisingly, one of the strongest objections to Wegener’s theory was moved by a non-fixist like Chamberlin, as he stated that tidal forces are too weak to cause continental drift (Chamberlin, 1928).
Mobilists like Van der Gracht, Daly, Joly, and Holmes replied to these objections by modifying Wegener’s mechanism of continental drift or by replacing it. Among them, Daly (1911) in his “The Nature of Volcanic Action” wrote: “...if the volcanic mechanism is nicely balanced, a minute effect, like tidal strain, may pull the trigger and renew activity, for which the essential conditions have been long preparing.” Here, for the first time, Daly formulated the idea of a “trigger” mechanism by tidal forces on the activity of volcanoes. However, the search for an endogenous mechanism of continental drift seemed to exile definitively the role of tides on volcanism to a background theme. The 1930–1970 was a period of remarkable lapse in the scientific debate around the tidal effects on terrestrial volcanism, with a very few exceptions as, for example, studies about the effects of Earth tides on Atlantic volcanoes (Hekla, La Palma, Fogo, Tristan da Cunha, Askja, Surtsey and San Jorge) by Machado (1967), where tidal forces are described in terms of “a mere trigger-force”.
In the next paragraph, we will illustrate how the exploration of the Moon, with the first landing in 1969, and the Voyager missions in the outer Solar System opened a new era of stimulating debate among geoscientists, astronomers and physicists and how it fed interesting speculations around the role of gravitational forces on terrestrial and extra-terrestrial volcanism.
After the 1930–1970 lapse, many papers, between 1970 and 1975, focused on the effects of tidal stresses on seismicity and eruptive activity, both at individual volcanoes e.g., at Galapagos (Filson et al., 1973), Stromboli (Johnston and Mauk, 1972), St. Augustine (Mauk and Kienle, 1973), Mt. Ngauruhoe (Michael and Christoffel, 1975) and Kilauea (Shimozuru, 1975) and at a global scale (Hamilton, 1973; Mauk and Johnston, 1973). Interestingly, the early studies by Jaggar (1924) on “lava tides” at Kilauea, apparently forgotten in the previous decades, were acknowledged in the new scientific literature and reinterpreted in light of new data, as for example the Kilauea 1968 eruption (Shimozuru, 1975). This renewed interest on tides and volcanoes emerged during the Moon race which offered both a broad range of advanced space and technology developments and a period of exceptionally stimulating debate around the origin of seismicity and volcanism on planets without plate tectonics. In particular, between 1969 and 1972, during the missions Apollo 11, 12, 14, 15, and 16, a network of seismic stations were installed by astronauts within the ALSEP (Apollo Lunar Surface Experiment Package) and recorded lunar seismicity until September 1977. The early results were published in November 1971 as the seismic network recorded episodes of frequent, small moonquakes (Latham et al., 1971). The seismic energy release, with a few exceptions caused by man-made and meteoroid impacts, appeared modulated by the lunar tidal cycle, with the main peaks occurring at monthly intervals near the time of perigee and a secondary peak near apogee. The exploration of the Moon and the discovery of moonquakes stimulated new hypothesis on the present dynamics of the lunar interior and re-opened a fruitful discussion about the role of tidal stresses on terrestrial seismicity and volcanism.
Overall, it appears that this renewed debate about the role of tides on volcanism is hinged around the idea of a tidal “trigger” mechanism acting on volcanic systems close to a critical state, as already formulated by Daly (1911). To note, among the new hypotheses to explain the trigger mechanism by Earth tides, Mauk and Kienle (1973) explained the tidal modulation of seismicity at St. Augustine volcano in terms of interaction between the orientation of tidal stresses -including the horizontal components- and the stress field of stress related to local structural and geological settings. This new approach will be largely adopted and implemented in the following years, both in terms of structural and geological field studies and theoretical modeling on active volcanoes (Patanè et al., 1994; Jentzsch et al., 2001; Sottili et al., 2007).
A second important discover, stimulating and feeding interesting speculations, occurred in March 1979, when an image captured during the Voyager 1 flyby revealed an eruption on the surface of the Jupiter’s moon Io, the most volcanically active body in the Solar System. A few months later, the Voyager 2 provided a new set of images revealing the existence of hundreds of active volcanoes on the Io’s surface. This unexpected discovery required the formulation of new hypotheses as, unlike Earth, Io’s source of internal heat cannot been explained by radioactive isotope decay. A new model evidenced how the tides induced by Jupiter, enhanced by Io’s orbital eccentricity and resonance with Europa and Ganymede, may lead to tidal dissipation and melting of a hypothetical liquid mantle (Peale et al., 1979): the volcanism on Io was the first documented example of volcanism exclusively caused by tidal forces.
In the following years, the tidal heating in subsurface oceans of icy satellites of the outer Solar System (i.e., Enceladus and Europa) has been proposed as a long-term energy source generating geysers of water vapor, seismicity (icequakes) and tectonic processes (Crawford and Stevenson, 1988; Greenberg et al., 1998; Hoppa et al., 1999). Once again, new models developed in astrophysics during the seventies influenced and stimulated–up to the present times-the debate around the relationship between tides, volcanism and seismicity within the Moon-Earth system. For example, recently, the tidally modulated icequakes on icy satellites has been modeled by investigating as analog the effects of tidal stresses on icequakes of the Ross Ice Shelf, Antarctica (Olsen et al., 2021). Also, the tidal heating during the early evolution of the Earth-Moon system has been modeled in light of evidence from the tidally generated Io’s volcanism; in fact, the tidal heating, partly enhanced by a period of large lunar eccentricity, has been proposed as a potential heat source for the early lunar magma ocean (Touma and Wisdom, 1998).
The following decade opened with the May 1980 Mt St. Helens eruption, the first catastrophic eruption investigated by geoscientists using modern volcanology. The occurrence of the eruption underscored the importance of monitoring precursory activities as a tool to decipher unrest phenomena and eruptive crisis. In this context, the study of geophysical signals jump-started interest in monitoring active volcanoes to improve early warning systems. McNutt and Beavan (1984), noticed that “…the pattern of stress release at Mount St. Helens prior to May 18 was for the most part too rapid to be significantly affected by the Earth tides.“. For the first time, the time-varying sensitivity of seismic activity to tidal stresses, as evidenced by volcanic unrest monitoring, was interpreted as indicator of “changing magma movement in the shallow crust beneath the volcano.” In the last few decades, many studies focused on the role of tidal stresses in influencing volcanic activity (e.g., Kasahara et al., 2001; Custodio et al., 2003; Sottili et al., 2007; Cigolini et al., 2009; van Manen et al., 2010; Sottili and Palladino, 2012; Bredemeyer and Hansteen, 2014; De Lauro et al., 2018; Girona et al., 2018; Petrosino et al., 2018; Dinger et al., 2019; Pering et al., 2019; Caputo et al., 2020; Dumont et al., 2020; Dumont et al., 2021). On the other hand, the optimism about the interpretation of the time-varying sensitivity of seismic and volcanic activity to tidal stresses as a monitoring tool was partly mediated by critical and skeptical positions (e.g., Sparks, 1981; Hurwitz et al., 2014). Concerning the possible mechanisms of interaction between tidal forces and volcanism, recent works focused on phases of caldera unrest, when the quasi-diurnal and semi-diurnal tidal modulation of long-period seismicity and degassing rates has been explained in terms of fluid charge/discharge in the fractures of hydrothermal systems and/or by tidally induced changes in crustal permeability (e.g., at the Campi Flegrei caldera, Petrosino et al.,2018). Other studies focused on effusive activity, as the modulation of the growth rate of lava fields by Earth tides has been associated with the effects of tidal stresses on the movements of magmatic fluids - gas, melt and lava - from the upper crust to the Earth’s surface, e.g., during the 2014 eruption of Holuhraun, central Iceland (Dumont et al., 2020) and during the 2014–2015 eruption of Fogo volcano, Cape Verde (Dumont et al., 2021). Also, persistent open-conduit phases of explosive volcanic activity modulated by the fortnightly tides have been explained by cycles of compression–decompression of wall rocks influencing the escape of magmatic volatiles and promoting fluid-driven cracking of the country rocks, e.g., at Stromboli volcano, (Sottili and Palladino, 2012) and at Mount Etna (Sottili et al., 2007).
In summary, since the beginning of eighties, a lot of studies contributed to further investigate the influence of tidal forces on volcanic activity and, as in the past two centuries, the new models bear on interdisciplinary contributions from geosciences, physics and astronomy. A full review of the recent literature on this rapidly evolving subject is beyond the aim of the present historical overview. For an exhaustive, critical review of the present state-of-the art see for example Dumont et al. (2022). In the conclusive section we will see how the present-day debate around the environmental crisis, characterized by a large collection of interconnected variables, stimulated a new field of research around the complex mechanisms of mutual interactions among orbital factors, Milankovitch cycles, climate changes and volcanism.
Since its beginning, the attention of the scientific community around the tidal controversy appears remarkably influenced by the course of other disputes, as for example the early development of the continental drift theory and the lack of a convincing hypothesis for its causal mechanisms (Chamberlin, 1928). Investigating the origin of tides and their effects on the present state of the Earth influenced the mankind’s general understanding of his origin and place in the Universe, either as the result of a series of catastrophic events or, as Kelvin postulated in light of the 2nd Law of Thermodynamics, as a consequence of a slow cooling where the action and intensity of geological forces must decrease with time (Burchfield, 2009). On the other hand, after long periods of lapse, the renewed interest in studying the effects of tidal forcing on volcanism was inspired by new explorations and discoveries from apparently distant fields of research, as astronomy and astrophysics.
Overall, it appears that the tidal controversy was continuously influenced and greatly stimulated by the contemporary scientific debate. The present-day new frontier of the research around the tidal controversy does not represent an exception. In fact, the ongoing environmental crisis, ruled by a complex interconnection of many variables, emphasizes the importance of evaluating quantitatively the contribution of either natural or anthropogenic factors to climate changes. Many studies dealing with palaeoenvironmental and palaeoclimatic reconstructions reveal millennial to million-year timescales of variability due to orbital forcing, based on high-resolution chronologies of sedimentary successions through tephrochronology (Albert et al., 2018; Giaccio et al., 2019; Leicher et al., 2019; Wulf et al., 2020). From a regional to a global scale, increasing evidence for common cycles in the volcanological, biological and astronomical-orbital events includes a remarkable synchronism with Milankovitch cycles, which strongly influenced the Earth’s climatic patterns (Kutterolf et al., 2013; Kutterolf et al., 2019; Puetz et al., 2014). On a broader perspective, beyond the high-frequency (from semi-diurnal to fortnightly) tidal forcing of volcanic and seismic activities, low-frequency rotational tides (i.e., pole tide and fluctuations in the Length of Day, LOD) are claimed as major controlling factors for a variety of tectonic and volcanic processes acting both at a local (Lambert and Sottili, 2019) and at a global scale (Palladino and Sottili, 2014; Sottili et al., 2015). Specifically, a recent field of research is devoted to understanding how long-period rotational tide (i.e, monthly to multiyear pole tides and LOD changes) acts on volcanoes, as short-periodic (commonly semi-diurnal to fortnightly tides) forcing influences shallow volatile-saturated magma reservoir or hydrothermal environments (Sottili et al., 2007; Sottili and Palladino, 2012; Petrosino et al., 2018) whilst low-frequency, rotational tidal oscillations are compatible with the resonance time of the lithosphere (Zaccagnino et al., 2020) and with differential stress changes on wall rocks of magma chambers located within the shallow 20 km of the Earth’s crust (Sottili et al., 2015; Lambert and Sottili, 2019). Moreover, over the last years, the detection of Milankovitch periodicities in volcanic explosive activity through the Pleistocene-Holocene (Kutterolf et al., 2013, 2019; Praetorius et al., 2016) led to the formulation of a variety of hypotheses on the possible cause-and-effect relationships among orbital forcing, climate changes and volcanism. For instance, the glacio-eustatism hypothesis links the observed Milankovitch periodicities in volcanic activity to crustal stress changes associated with ice age mass redistribution and sea level changes (Rampino and Self, 1993; Ryan et al., 2004; Watt et al., 2013; Praetorius et al., 2016; Rawson et al., 2016). This hypothesis seems to be corroborated by evidence for a subaerial volcanism increase during the last deglaciation phase (Huybers and Langmuir, 2009; Jull and Mc Kenzie, 1996) and during the Messinian salinity crisis in the Mediterranean area (Sternai et al., 2017) whilst the impact of Milankovitch cycle-caused sea level fluctuations on the volcanic construction process at mid-ocean ridges remains an area of active research (e.g., Olive et al., 2015; Goff, 2020).
An alternative hypothesis focuses on the role of crustal stress changes due to orbital oscillations of the inclination of the Earth causing a periodic increase in explosive volcanic activity (Jegen-Kulcsar et al., 2010; Bezverkhnii, 2017) and a perturbation in greenhouse gas emissions from the mantle (Bezverkhnii, 2014; Gilabert et al., 2021). On its turn, the orbital forcing of volcanism and volcanic gas output would play a significant role in determining the 41,000 years variations of the ocean water temperature (Bezverkhnii, 2019). This hypothesis also tries reconciling several difficulties related to the Milankovitch theory in explaining the 100 kyr cycles, since glacial–interglacial oscillations during the pre-mid-Pleistocene transition are significantly asymmetric, thus contradicting a straightforward linear Milankovitch explanation (e.g., Ashkenazy and Tziperman, 2004).
In conclusion, the article collection in the present volume highlights the enduring interest in external processes interacting at various timescales with volcanic systems. Although progresses in specific instrumental developments and physical modeling have improved our capability to detect tidal signals in volcanic records, the new frontier of the tidal controversy appears -as already occurred in the past- greatly influenced by the contemporary scientific debate across a wide spectrum of research fields including geosciences, climatology, physics and astronomy Auer et al., 2015, Lirer et al., 2009.
All authors listed have made a substantial, direct, and intellectual contribution to the work and approved it for publication.
The authors declare that the research was conducted in the absence of any commercial or financial relationships that could be construed as a potential conflict of interest.
All claims expressed in this article are solely those of the authors and do not necessarily represent those of their affiliated organizations, or those of the publisher, the editors and the reviewers. Any product that may be evaluated in this article, or claim that may be made by its manufacturer, is not guaranteed or endorsed by the publisher.
we thank R. Cioni, T. Mittal and the Editors DP and Valerio Acocella for their helpful suggestions. This review was partly developed within the Tidal Interplate Lithospheric Deformation of Earth (TILDE) project, funded by ESA.
Albert, P. G., Smith, V. C., Suzuki, T., Tomlinson, E. L., Nakagawa, T., McLean, D., et al. (2018). Constraints on the Frequency and Dispersal of Explosive Eruptions at Sambe and Daisen Volcanoes (South-West Japan Arc) from the Distal Lake Suigetsu Record (SG06 Core). Earth-Science Rev. 185, 1004–1028. doi:10.1016/j.earscirev.2018.07.003
Ashkenazy, Y., and Tziperman, E. (2004). Are the 41kyr Glacial Oscillations a Linear Response to Milankovitch Forcing. Quat. Sci. Rev. 23, 1879–1890. doi:10.1016/j.quascirev.2004.04.008
Auer, G., Piller, W. E., Reuter, M., and Harzhauser, M. (2015). Correlating Carbon and Oxygen Isotope Events in Early to Middle Miocene Shallow marine Carbonates in the Mediterranean Region Using Orbitally Tuned Chemostratigraphy and Lithostratigraphy. Paleoceanography 30, 332–352. doi:10.1002/2014PA002716
Bay, R. C., Bramall, N., and Price, P. B. (2004). Bipolar Correlation of Volcanism with Millennial Climate Change. Proc. Natl. Acad. Sci. 101 (17), 6341–6345. doi:10.1073/pnas.0400323101
Bezverkhnii, V. A. (2014). Correlation between 41000-year Rhythms in Variations in the Inclination of Earth, Violent Volcanic Eruptions, and Temperature of Deep Ocean Waters. Izv. Atmos. Ocean. Phys. 50 (6), 657–659. doi:10.1134/S0001433814040124
Bezverkhnii, V. A. (2017). Earth’s Obliquity Oscillations Can Influence Climate Change by Driving Global Volcanic Activity. Geosci. Res. 2 (1), 22–26. doi:10.22606/gr.2017.21004
Bezverkhnii, V. A. (2019). On the 100 000-Year Rhythmicity in Geodynamics and the Paleoclimate. Izv., Phys. Solid Earth 55, 488–495. doi:10.1134/s1069351319030017
Bluck, R. S. (2014). Plato's Phaedo: A Translation of Plato's Phaedo. London and New York: Taylor and Francis Group. Routledge.
Bredemeyer, S., and Hansteen, T. H. (2014). Synchronous Degassing Patterns of the Neighbouring Volcanoes Llaima and Villarrica in South-central Chile: the Influence of Tidal Forces. Int. J. Earth Sci. (Geol Rundsch) 103 (7), 1999–2012. doi:10.1007/s00531-014-1029-2
Brush, S. G. (2014). A History of Modern Planetary Physics, 7. Cambridge, United Kingdom: Cambridge University Press.
Brush, S. G. (19781978). A Geologist Among Astronomers: The Rise and Fall of the Chamberlin-Moulton Cosmogony, Part 1. J. Hist. Astron. 9 (1), 1–41. doi:10.1177/002182867800900101
Brush, S. G. (1979). Nineteenth-century Debates about the inside of the Earth: Solid, Liquid or Gas. Ann. Sci. 36, 225–254. doi:10.1080/00033797900200231
Burchfield, J. D. (2009). Lord Kelvin and the Age of the Earth. Chicago: University of Chicago Press. doi:10.7208/9780226080260
Caputo, T., Cusano, P., Petrosino, S., Sansivero, F., and Vilardo, G. (2020). Spectral Analysis of Ground thermal Image Temperatures: what We Are Learning at Solfatara Volcano (Italy). Adv. Geosci. 52, 55–65. doi:10.5194/adgeo-52-55-2020
Chamberlin, R. T. (1928). “Some of the Objections to Wegener’s Theory,” in Theory of Continental Drift: A Symposium on the Origin and Movement of Land Masses Both Inter-continental and Intracontinental, as Proposed by Alfred Wegener. Editor W. van Waterschoot van der Gracht (Tulsa: American Association of Petroleum Geologists), 83–87. doi:10.1306/sv2329c3
Cigolini, C., Poggi, P., Ripepe, M., Laiolo, M., Ciamberlini, C., Delle Donne, D., et al. (2009). Radon Surveys and Real-Time Monitoring at Stromboli Volcano: Influence of Soil Temperature, Atmospheric Pressure and Tidal Forces on 222Rn Degassing. J. Volcanology Geothermal Res. 184 (3–4), 381–388. doi:10.1016/j.jvolgeores.2009.04.019
Crawford, G. D., and Stevenson, D. J. (1988). Gas-driven Water Volcanism and the Resurfacing of Europa. Icarus 73 (1), 66–79. doi:10.1016/0019-1035(88)90085-1
Custodio, S. I., Fonseca, J. F., d'Oreye, N. F., Faria, B. V., and Bandomo, Z. (2003). Tidal Modulation of Seismic Noise and Volcanic Tremor. Geophys. Res. Lett. 30 (15), 1816. doi:10.1029/2003gl016991
Daly, R. A. (1911). The Nature of Volcanic Action. Proc. Am. Acad. Arts Sci. 47, 48–119. doi:10.2307/20022712
De Lauro, E., Petrosino, S., Ricco, C., Aquino, I., and Falanga, M. (2018). Medium and Long Period Ground Oscillatory Pattern Inferred by Borehole Tiltmetric Data: New Perspectives for the Campi Flegrei Caldera Crustal Dynamics. Earth Planet. Sci. Lett. 504, 21–29. doi:10.1016/j.epsl.2018.09.039
Dinger, F., Bredemeyer, S., Arellano, S., Bobrowski, N., Platt, U., and Wagner, T. (2019). On the Link between Earth Tides and Volcanic Degassing. Solid Earth 10, 725–740. doi:10.5194/se-10-725-2019
Dumont, S., Custódio, S., Petrosino, S., Thomas, A., and Sottili, G. (2022). “Tides, Earthquakes and Volcanic Eruptions,” in A Tidal Journey through Earth’s History. Editors M. Green, and J. C. Duarte (Elsevier).
Dumont, S., Le Mouël, J. L., Courtillot, V., Lopes, F., Sigmundsson, F., Coppola, D., et al. (2020). The Dynamics of a Long-Lasting Effusive Eruption Modulated by Earth Tides. Earth Planet. Sci. Lett. 536, 116145. doi:10.1016/j.epsl.2020.116145
Dumont, S., Silveira, G., Custódio, S., Lopes, F., Le Mouël, J.-L., Gouhier, M., et al. (2021). Response of Fogo Volcano (Cape Verde) to Lunisolar Gravitational Forces during the 2014-2015 Eruption. Phys. Earth Planet. Interiors 312, 106659. doi:10.1016/j.pepi.2021.106659
Filson, J., Simkin, T., and Leu, L.-k. (1973). Seismicity of a Caldera Collapse: Galapagos Islands 1968. J. Geophys. Res. 78, 8591–8622. doi:10.1029/jb078i035p08591
Gale, H. G. (1914). On an Experimental Determination of the Earth's Elastic Properties. Science 39 (1017), 927–933. doi:10.1126/science.39.1017.927
Giaccio, B., Leicher, N., Mannella, G., Monaco, L., Regattieri, E., Wagner, B., et al. (2019). Extending the Tephra and Palaeoenvironmental Record of the Central Mediterranean Back to 430 Ka: A New Core from Fucino Basin, central Italy. Quat. Sci. Rev. 225, 106003. doi:10.1016/j.quascirev.2019.106003
Gilabert, V., Batenburg, S. J., Arenillas, I., and Arz, J. A. (2021). Contribution of Orbital Forcing and Deccan Volcanism to Global Climatic and Biotic Changes across the Cretaceous-Paleogene Boundary at Zumaia, Spain. Geology 2021, 15. doi:10.1130/G49214.1
Girona, T., Huber, C., and Caudron, C. (20182018). Sensitivity to Lunar Cycles Prior to the 2007 Eruption of Ruapehu Volcano. Sci. Rep. 8, 1476. doi:10.1038/s41598-018-19307-z
Godwin, J. (1991). “De Rerum Natura IV with An Introduction, Translation and Commentary by John Godwin, ” in Aris & Phillips Classical Texts (Liverpool University Press). doi:10.2307/j.ctv10tq4r7
Goff, J. A. (2020). “Empirical Prewhitening” Spectral Analysis Detects Periodic but Inconsistent Signals in Abyssal hill Morphology at the Southern East Pacific Rise. Geochem. Geophys. Geosyst. 21, e2020GC009261. doi:10.1029/2020GC009261
Greenberg, R., Geissler, P., Hoppa, G., Tufts, B. R., Durda, D. D., Pappalardo, R., et al. (1998). Tectonic Processes on Europa: Tidal Stresses, Mechanical Response, and Visible Features. Icarus 135, 64–78. doi:10.1006/icar.1998.5986
Hamilton, W. (1783). An Account of the Earthquakes in Calabria, Sicily, &c. London: As Communicated to the Royal Society. Colchester: J. Fenno. doi:10.1098/rstl.1783.0012
Hamilton, W. L. (1973). Tidal Cycles of Volcanic Eruptions: Fortnightly to 19 yearly Periods. J. Geophys. Res. 78, 3363–3375. doi:10.1029/jb078i017p03363
Hecker, O. (1907). Beobachtungen an Horizontalpendeln über die Deformation des Erdkörpers unter dem Einfluss von Sonne und MondVeröffentlichung des Königl, 32. Berlin: Preuszischen Geodätischen Institutes.
Hine, H. M. (2002) Seismology and Vulcanology in Antiquity? in C. J. Tuplin, and T. E. Rihll (eds), Science and Mathematics in Ancient Greek Culture, 64–65.
Hine, H. M. (1984). The Date of the Campanian Earthquake. A.D. 62 or A.D. 63 or Both. L’antiquité classique 53, 266–269. doi:10.3406/antiq.1984.2130
Hoppa, G., Randall Tufts, B., Greenberg, R., and Geissler, P. (1999). Strike-Slip Faults on Europa: Global Shear Patterns Driven by Tidal Stress. Icarus 141 (2), 287–298. doi:10.1006/icar.1999.6185
Hurwitz, S., Sohn, R. A., Luttrell, K., and Manga, M. (2014). Triggering and Modulation of Geyser Eruptions in Yellowstone National Park by Earthquakes, Earth Tides, and Weather. J. Geophys. Res. Solid Earth 119, 1718–1737. doi:10.1002/2013JB010803
Jaggar, T. A., Finch, R. H., and Emerson, O. H. (1924). The Lava Tide, Seasonal Tilt and the Volcanic Cycle. Month. Weather Rev. 52, 142–145. doi:10.1175/1520-0493(1924)52<142:tltsta>2.0.co;2
Jaggar, T. A. (1956). My Experiments with Volcanoes. Honolulu: Hawaiian Volcano Research Association.
James, F. A. J. L. (1991). The Correspondence of Michael Faraday, Vol. 1. London: Institution of Electrical Engineers - Engineering and Technology. 1811 to December 1831. Letters 1 to 524.
Jegen-Kulcsar, M., Kutterolf, S., Kwasnitschka, T., and Freundt, A. (2010). Cyclic Volcanism at Convergent Margins: Linked to Earth Orbital Parameters or Climate Changes. Vienna: EGU General Assembly, 13373.
Jentzsch, G., Haase, O., Kroner, C., and Winter, U. (2001). Mayon Volcano, Philippines: Some Insights into Stress Balance. J. Volcanology Geothermal Res. 109, 205–217. doi:10.1016/s0377-0273(00)00312-7
Johnston, M. J. S., and Mauk, F. J. (1972). Earth Tides and the Triggering of Eruptions from Mt Stromboli, Italy. Nature 239, 266–267. doi:10.1038/239266b0
Kasahara, J., Nakao, S., and Koketsu, K. (2001). Tidal Influence on the 2000 Miyake Jima Eruption and its Implications for Hydrothermal Activity and Volcanism. Proc. Jpn. Acad. Ser. B: Phys. Biol. Sci. 77 (6), 98–103. doi:10.2183/pjab.77.98
Kutterolf, S., Jegen, M., Mitrovica, J. X., Kwasnitschka, T., Freundt, A., and Huybers, P. J. (2013). A Detection of Milankovitch Frequencies in Global Volcanic Activity. Geology 41 (2), 227–230. doi:10.1130/G33419.1
Kutterolf, S., Schindlbeck, J. C., Jegen, M., Freundt, A., and Straub, S. M. (2019). Milankovitch Frequencies in Tephra Records at Volcanic Arcs: The Relation of Kyr-Scale Cyclic Variations in Volcanism to Global Climate Changes. Quat. Sci. Rev. 204, 1–16. doi:10.1016/j.quascirev.2018.11.004
Lambert, S., and Sottili, G. (2019). Is There an Influence of the Pole Tide on Volcanism? Insights from Mount Etna Recent Activity. Geophys. Res. Lett. 46, 13730–13736. doi:10.1029/2019GL085525
Latham, G., Ewing, M., Dorman, J., Lammlein, D., Press, F., Toksoz, N., et al. (1971). Moonquakes. Science 174, 687–692. doi:10.1126/science.174.4010.687
Lee, H. D. P. (1952). Aristotle. Meteorologica (With an English Translation by H.D.P.Lee). Cambridge, Mass. & London: Harvard University Press & Heinemann. (reprinted 1978).
Leicher, N., Giaccio, B., Zanchetta, G., Wagner, B., Francke, A., Palladino, D. M., et al. (2019). Central Mediterranean Explosive Volcanism and Tephrochronology during the Last 630 Ka Based on the Sediment Record from Lake Ohrid. Quat. Sci. Rev. 226, 106021. doi:10.1016/j.quascirev.2019.106021
Lirer, F., Harzhauser, M., Pelosi, N., Piller, W. E., Schmid, H. P., and Sprovieri, M. (2009). Astronomically Forced Teleconnection between Paratethyan and Mediterranean Sediments during the Middle and Late Miocene. Palaeogeogr. Palaeoclimatol. Palaeoecol. 275 (1-4), 1–13. doi:10.1016/j.palaeo.2009.01.006
Machado, F. (1967). Activity of the Atlantic Volcanoes, 1947-1965. Bull. Volcanol. 30, 29–34. doi:10.1007/bf02597652
Mauk, F. J., and Johnston, M. J. S. (1973). On the Triggering of Volcanic Eruptions by Earth Tides. J. Geophys. Res. 78, 3356–3362. doi:10.1029/jb078i017p03356
Mauk, F. J., and Kienle, J. (1973). Microearthquakes at St. Augustine Volcano, Alaska, Triggered by Earth Tides. Science 182, 386–389. doi:10.1126/science.182.4110.386
McNutt, S. R., and Beavan, R. J. (1984). Patterns of Earthquakes and the Effect of Solid Earth and Ocean Load Tides at Mount St. Helens Prior to the May 18, 1980, Eruption. J. Geophys. Res. 89 (B5), 3075–3086. doi:10.1029/jb089ib05p03075
Mercalli, G. (1892). Sopra 1’eruzione dell’Etna cominciata il 9 luglio 1892 Atti della Società italiana di Scienze Naturali. Milano.
Michael, M. O., and Christoffel, D. A. (1975). Triggering of Eruptions of Mt Ngauruhoe by Fortnightly Earth Tide Maxima, January 1972-June 1974. New Zealand J. Geology. Geophys. 18, 273–277. doi:10.1080/00288306.1975.10418198
Olive, J.-A., Behn, M. D., Ito, G., Buck, W. R., Escartín, J., and Howell, S. (2015). Sensitivity of Seafloor Bathymetry to Climate-Driven Fluctuations in Mid-ocean ridge Magma Supply. Science 350, 310–313. doi:10.1126/science.aad0715
Olsen, K. G., Hurford, T. A., Schmerr, N. C., Huang, M. H., Brunt, K. M., Zipparo, S., et al. (2021). Projected Seismic Activity at the Tiger Stripe Fractures on Enceladus, Saturn, from an Analog Study of Tidally Modulated Icequakes within the Ross Ice Shelf, Antarctica. J. Geophys. Res. Planets 126, e2021JE006862. doi:10.1029/2021JE006862
Palladino, D. M., and Sottili, G. (2014). Earth's Spin and Volcanic Eruptions: Evidence for Mutual Cause-And-Effect Interactions. Terra Nova 26, 78–84. doi:10.1111/ter.12073
Palmieri, L. (1873). in The Eruption of Vesuvius in 1872. With Notes and an Introductory Sketch, &c. Editor F. R. Robert Mallet (.S, (London: Asher and Co.).
Patanè, G., Frasca, A., Agodi, A., and Imposa, S. (1994). Earth Tides and Etnean Volcanic Eruptions: an Attempt at Correlation of the Two Phenomena during the 1983, 1985 and 1986 Eruptions. Phys. Earth Planet. Interiors 87, 123–135. doi:10.1016/0031-9201(94)90026-4
Peale, S. J., Cassen, P., and Reynolds, R. T. (1979). Melting of Io by Tidal Dissipation. Science 203, 892–894. doi:10.1126/science.203.4383.892
Pering, T. D., Ilanko, T., and Liu, E. J. (2019). Periodicity in Volcanic Gas Plumes: A Review and Analysis. Geosciences 9 (9), 394. doi:10.3390/geosciences9090394
Petrosino, S., Cusano, P., and Madonia, P. (2018). Tidal and Hydrological Periodicities of Seismicity Reveal New Risk Scenarios at Campi Flegrei Caldera. Sci. Rep. 8 (1), 13808–13812. doi:10.1038/s41598-018-31760-4
Praetorius, S., Mix, A., Jensen, B., Froese, D., Milne, G., Wolhowe, M., et al. (2016). Interaction between Climate, Volcanism, and Isostatic Rebound in Southeast Alaska during the Last Deglaciation. Earth Planet. Sci. Lett. 452, 79–89. doi:10.1016/j.epsl.2016.07.033
Puetz, S. J., Prokoph, A., Borchardt, G., and Mason, E. W. (2014). Evidence of Synchronous, Decadal to Billion Year Cycles in Geological, Genetic, and Astronomical Events. Chaos, Solitons & Fractals 62-63, 55–75. doi:10.1016/j.chaos.2014.04.001
Rampino, M. R., and Self, S. (1993). Climate-Volcanism Feedback and the Toba Eruption of ∼74,000 Years Ago. Quat. Res. 40 (3), 269–280. doi:10.1006/qres.1993.1081
Rawson, H., Pyle, D. M., Mather, T. A., Smith, V. C., Fontijn, K., Lachowycz, S. M., et al. (2016). The Magmatic and Eruptive Response of Arc Volcanoes to Deglaciation: Insights from Southern Chile. Geology 44, 251–254. doi:10.1130/G37504.1
Riccò, A., and Arcidiacono, S. (1904). L’eruzione Dell’Etna Nel 1892. Atti Acc. Gioenia Sc. Nat. Catania 416 (15), 17.
Shimozuru, D. (1975). Lava lake Oscillations and the Magma Reservoir beneath a Volcano. Bull. Volcanol. 39, 570–580. doi:10.1007/bf02596977
Sigurdsson, H. (1999). Melting the Earth; the Evolution of Ideas about Volcanic Eruptions. New York: Oxford University Press, 250.
Sottili, G., Martino, S., Palladino, D. M., Paciello, A., and Bozzano, F. (2007). Effects of Tidal Stresses on Volcanic Activity at Mount Etna, Italy. Geophys. Res. Lett. 34, L01311. doi:10.1029/2006GL028190
Sottili, G., Palladino, D. M., Cuffaro, M., and Doglioni, C. (2015). Earth's Rotation Variability Triggers Explosive Eruptions in Subduction Zones. Earth Planet. Sp 67, 208. doi:10.1186/s40623-015-0375-z
Sottili, G., and Palladino, D. M. (2012). Tidal Modulation of Eruptive Activity at Open-Vent Volcanoes: Evidence from Stromboli, Italy. Terra Nova 24 (3), 233–237. doi:10.1111/j.1365-3121.2012.01059.x
Sparks, R. S. J. (1981). Triggering of Volcanic Eruptions by Earth Tides. Nature 290, 448. doi:10.1038/290448a0
Sternai, P., Caricchi, L., Garcia-Castellanos, D., Jolivet, L., Sheldrake, T. E., and Castelltort, S. (2017). Magmatic Pulse Driven by Sea-Level Changes Associated with the Messinian Salinity Crisis. Nat. Geosci 10, 783–787. doi:10.1038/ngeo3032
Thomson, W. (1863). On the Rigidity of the Earth, 153. London: Philosophical Transactions of the Royal Society.
Thordarson, T., and Self, S. (2003). Atmospheric and Environmental Effects of the 1783-1784 Laki Eruption: A Review and Reassessment. J. Geophys. Res. 108 (D1), 4011. doi:10.1029/2001JD002042
Touma, J., and Wisdom, J. (1998). Resonances in the Early Evolution of the Earth-Moon System. Astron. J. 115, 1653–1663. doi:10.1086/300312
van Manen, S. M., Kervyn, M., Blake, S., and Ernst, G. G. J. (2010). Apparent Tidal Influence on Magmatic Activity at Oldoinyo Lengai Volcano, Tanzania, as Observed in Moderate Resolution Imaging Spectroradiometer (MODIS) Data. J. Volcanology Geothermal Res. 189 (1-2), 151–157. doi:10.1016/j.jvolgeores.2009.11.003
von Humboldt, A. (1866). Cosmos: Sketch of a Physical Description of the Universe. New York: Harper & Brothers.
Watt, S. F. L., Pyle, D. M., and Mather, T. A. (2013). The Volcanic Response to Deglaciation: Evidence from Glaciated Arcs and a Reassessment of Global Eruption Records. Earth-Science Rev. 122, 77–102. doi:10.1016/j.earscirev.2013.03.007
Williams, G. D. (2006). Greco-Roman Seismology and Seneca on Earthquakes in Natural Questions 6. J. Rom. Stud. 96, 124–146. doi:10.3815/000000006784016206
Wulf, S., Keller, J., Satow, C., Gertisser, R., Kraml, M., Grant, K. M., et al. (2020). Advancing Santorini's Tephrostratigraphy: New Glass Geochemical Data and Improved marine-terrestrial Tephra Correlations for the Past ∼360 Kyrs. Earth-Science Rev. 200, 102964. doi:10.1016/j.earscirev.2019.102964
Keywords: volcanic eruptions, orbital forcing, history of tides, Tidal forces, orbital (Milankovitch) cycles
Citation: Sottili G, Lambert S and Palladino DM (2021) Tides and Volcanoes: A Historical Perspective. Front. Earth Sci. 9:777548. doi: 10.3389/feart.2021.777548
Received: 15 September 2021; Accepted: 29 November 2021;
Published: 14 December 2021.
Edited by:
David M. Pyle, University of Oxford, United KingdomReviewed by:
Raffaello Cioni, University of Florence, ItalyCopyright © 2021 Sottili, Lambert and Palladino. This is an open-access article distributed under the terms of the Creative Commons Attribution License (CC BY). The use, distribution or reproduction in other forums is permitted, provided the original author(s) and the copyright owner(s) are credited and that the original publication in this journal is cited, in accordance with accepted academic practice. No use, distribution or reproduction is permitted which does not comply with these terms.
*Correspondence: Gianluca Sottili, Z2lhbmx1Y2Euc290dGlsaUB1bmlyb21hMS5pdA==
Disclaimer: All claims expressed in this article are solely those of the authors and do not necessarily represent those of their affiliated organizations, or those of the publisher, the editors and the reviewers. Any product that may be evaluated in this article or claim that may be made by its manufacturer is not guaranteed or endorsed by the publisher.
Research integrity at Frontiers
Learn more about the work of our research integrity team to safeguard the quality of each article we publish.