- 1Key Laboratory of Marine Geology and Metallogeny, First Institute of Oceanography, Ministry of Natural Resources, Qingdao, China
- 2Laboratory for Marine Geology, Pilot National Laboratory for Marine Science and Technology, Qingdao, China
- 3Department of Marine and Coastal Resources, Bangkok, Thailand
- 4Phuket Marine Biological Center, Phuket, Thailand
Coastal lagoons are among the most vulnerable and economically significant ecosystems on Earth. Songkhla Lake, connected with the Gulf of Thailand, is the second largest lake in Southeast Asia and supports the development of the fishery, transportation, and tourism industries in southern Thailand. With increasing anthropogenic disturbances, the lake is facing acute ecological problems and further research is needed. Here, we provide 55-year record of grain size, color reflectance, magnetic susceptibility, total organic carbon, total nitrogen, and trace element (As, Hg, Pb, Cr, Ni, Cu, and Zn) concentrations of sediment core SKL8-2 collected from Songkhla Lake. These records reveal a three-stage sedimentary and input history of trace metals under anthropogenic effects: 1) From 1964 to 1982, it was a natural terrigenous input period with a relative reduction environment when the channel connecting Songkhla Lake and the Gulf of Thailand was closed. 2) Trace metal concentrations, organic carbon content, b* value, and magnetic susceptibility changed abruptly in 1982. During 1982–2000, the sources of trace metals were more complex than during 1964–1982 and mainly came from urban wastewater, industrial effluent, and fishery discharge. 3) From 2000 to 2019, contamination signals of Pb, Hg, As, Zn, and Ni emerged in the first decade because of the rapid development and poor sewage treatment around nearby cities.
Introduction
Coastal lagoons rank among the most productive ecosystems on Earth because they provide a wide range of ecosystem services and resources (Newton et al., 2018; Velasco et al., 2018; Faremi et al., 2021). Their high ecological value encompasses flood control, groundwater recharge, prevention of seawater intrusion, facilitation of shoreline stabilization, storm protection, retention and export of sediment and nutrients, mitigation of climate change, water purification, and providing reservoirs of genetic and/or species biodiversity (Pérez-Ruzafa et al., 2012; Barbier, 2014; Anthony et al., 2016; Hung et al., 2020). Furthermore, lagoons are desirable areas for a variety of anthropogenic activities such as intensive aquaculture, shipping, tourism, and recreation (Pérez-Ruzafa et al., 2007). Consequently, coastal lagoons are highly exposed to various types of contaminants including trace metals, hydrocarbons, and plastics, which result from sources that include, but are not limited to, increased discharge of domestic, municipal, and industrial effluents (Pedro et al., 2016; Sogbanmu et al., 2016; Veiga et al., 2019; Wakkaf et al., 2020; El Zrelli et al., 2021). These contaminants persist for long periods and become bioavailable to living organisms because of their potential to bioaccumulate and biomagnify through the food chain (Bryan and Langston, 1992; Zhou et al., 2008; Bakshi et al., 2018), where sediments serve as their ultimate sink (Gadkar et al., 2019; Jung et al., 2019). Given that such lagoons are only occasionally connected with the open sea, marine influence is limited, and the sedimentary record is relatively continuous and undisturbed. Lagoon sediments can therefore serve as archives of environmental changes through time (Aparecida Leite Silva and Eduardo Rezende, 2002; Liu et al., 2006; Laermanns et al., 2021).
Anthropogenic environmental contamination by trace metals began with the domestication of fire; later, the industrial revolution led to unprecedented demand for metals and an exponential increase in the intensity of metal emissions (Schmidt and Reimers, 1991; Nriagu, 1996). Songkhla Lake, a coastal lagoon, is regarded as one of the most important natural resources in southern Thailand, with fishing and aquaculture as the main economic activities. The lake provides the people of its surroundings with food, water for irrigation and domestic use, and means of transportation and recreation (Kumblad et al., 2001). Increasing human activities, including urbanization, industrialization, and agriculturalization, have led to considerable amounts of trace metal inputs, requiring urgent remediation (Ratanachai et al., 2013).
During the past three decades, a number of studies on trace metals in lacustrine sediments have been carried out in this area, revealing the spatial pattern of trace metals in Songkhla Lake (Nakinchart et al., 2006; Pradit et al., 2010). These studies have shown that canals and their vicinities were associated with abundant trace elements because of municipal, agricultural, and industrial discharges entering the lake through the canals. Furthermore, a recent study, which analyzing the concentrations of As, Cd, Pb, and Zn in mangrove plants (leaves, roots, and bark) and sediments, revealed heavy pollution of As (Pradit et al., 2018). However, research on sedimentary trace metal history, which can help improve understanding of the influence of human activities, remains scarce. Thus, the aims of the present study were to reveal sedimentary records of trace metals in Songkhla Lake for the past several decades, evaluate the contamination status of the lake, and examine the impact of human activities.
Materials and Methods
Study Area
Songkhla Lake (Figure 1), a coastal shallow lagoon, is located in southern Thailand (7°08′-50′N and 100°07′-37′E) and covers an area of 1,042 km2. The lake is divided into four parts: 1) Thale Noi, a freshwater lake surrounded by freshwater swamps with a total area of 28 km2; 2) an inner lake with an average depth of 2 m and an area of 459 km2; 3) a shallow middle lake with an average depth of 1 m and an area of 377 km2; and 4) an outer lake with a surface area of 182 km2, which is connected to the Gulf of Thailand (GoT) through a narrow channel 420 m wide and 9.5 m deep.
Songkhla Lake is dominated by a tropical monsoonal climate. The rainy season, dominated by the southwest monsoon, occurs from mid-May to almost the end of the year and is caused by warm, humid air flowing from the Indian Ocean. Then, the dry season, controlled by the northeast monsoon, is characterized by low precipitation (https://www.tmd.go.th/en/climate.php). The salinity of the water is altered by the tidal currents and varies over a wide range from nearly 0 psu in Thale Noi to 30 psu in the outer section. There are no major rivers in the area; the lake is fed by numerous small streams and man-made canals.
The lake is a vital natural resource for the people living in the surrounding provinces, Songkhla Province and Phatthalung Province, which have populations of 1.25 and 0.50 million people, respectively, as well as some parts of Nakhon Si Thammarat Province. It is a major fishing ground for mollusks, crustaceans, and fish and is especially used for the aquaculture of sea bass. The catchment area of the entire Songkhla Lake Basin (approximately 8,020 km2), besides Songkhla Lake, consists mostly of lowland rice fields, rubber plantations, and forest-covered hills (Kumblad et al., 2001).
Sediment Sampling
Five sediment cores were collected from Songkhla Lake in August 2019 (Figure 1, Table 1) using push corers. The cores were sealed after collection and stored in the repository at a temperature of 4°C. Half of each core was preserved, and the remaining half was photographed and then subsampled at 2-cm intervals for further analysis. In this study, we focused on sediment core SKL8-2.
Laboratory Analysis
This study is based on comprehensive analyses of color reflectance, magnetic susceptibility, grain size, total organic carbon (TOC), total nitrogen (TN), and elements concentrations of core sediment, combined with 210Pb dating chronology.
The sedimentation rate was determined using 210Pb radionuclide dating at the Radioactive Isotopes Analysis Laboratory of East China Normal University, Shanghai, China. Lyophilized samples were stored for at least 3 weeks in sealed containers to reach secular equilibrium prior to analysis. The analysis was then conducted on a well-type HPGe gamma spectroscope (GSW275L).
Sediment color and magnetic susceptibility were measured by MSCL (Multi Sensor Core Logger). In sediment color, L* describes the lightness between black (0) and white (100), while a* and b* denote the red (positive values)–green (negative values) and yellow (positive values)–blue (negative values) chromaticity, respectively. The chlorine content in the sediments was analyzed by an X-ray fluorescence (counting intensity) core scanner (Itrax, Sweden). Grain sizes of the samples were determined with a Mastersizer 3000 instrument, and 10% parallel samples were chosen for repeat measurement to confirm the reliability of the results. The measurement range of the instrument was 0.02–2,000 μm. The error based on repeated measurements was estimated as <3%.
TOC and TN were measured by a VarioEL Ⅲ elemental analyzer (ELementar, Germany). During measurement, 10% parallel samples and GSD-9 standard material were also measured, and the relative measurement error was lower than 0.5%. Inductively coupled plasma optical emission spectrometry (ICP-OES, Thermo iCAP-6300, United States) was used to measure Al2O3 and CaO contents, whereas Cr, Ni, Cu, Zn, and Pb contents were measured by inductively coupled plasma mass spectrometry (ICP-MS, Thermo X-series II, United States). As and Hg were measured using atomic fluorescence spectrometry (AFS-920). Ten percent parallel samples, China Stream Sediment Standards (GBW07309, GBW07313, GBW07314, and GBW07316), and appropriate blanks were used for quality control. The relative standard deviations of the analyses were all <5%. All measurements except for radionuclides were performed at the Key Laboratory of Marine Geology and Metallogeny, Ministry of Natural Resources, China.
Assessment of Trace Metal Contamination in Sediments
To better understand the risk associated with trace metals, contamination indices, including the enrichment factor (EF) and geo-accumulation index (Igeo), and sediment quality guidelines (SQGs) were employed in the present study.
Enrichment Factor of Trace Metals
The EF is a powerful tool used to elucidate the degree of contamination in sediment in relation to the background value. The EF can be calculated according to the following function (Buat-Menard and Chesselet, 1979):
where
EF ≤ 1 indicates no enrichment, 1 < EF ≤ 3 is minor enrichment, 3 < EF ≤ 5 is moderate enrichment, and EF > 5 suggests severe enrichment.
Geo-Accumulation Index of Trace Metals
The Igeo allows evaluation of contamination by correlating the obtained current concentration of metals with their background concentrations. The Igeo values for metals were determined using the following equation (Müller, 1979):
where
As a threshold value, Igeo = 0 is selected. A value of Igeo ≤ 0 suggest uncontaminated, and Igeo > 0 are divided into six different contamination levels.
Sediment Quality Assessment
SQGs are useful to estimate biological effects in contaminated sediments (Long et al., 1995; MacDonald et al., 2000; Filgueiras et al., 2004; Hinkey and Zaidi, 2007; Violintzis et al., 2009). In this study, potential ecological effects were evaluated based on the effects range low (ERL) and effects range median (ERM) guideline values for estuarine and marine environments proposed by the United States National Oceanic and Atmospheric Administration (Table 2). The ERL represents the 10th percentile of the effects database, below which harmful effects on aquatic biota are rarely observed. In contrast, the ERM represents the 50th percentile values of the effects data and indicates concentrations above which harmful effects are often observed.

TABLE 2. ERL (effects range low) and ERM (effects range median) guideline values for trace metals (μg/g in dry weight).
Results and Discussion
Sediment Chronology
From the surface to the bottom of core sediment SKL8-2, 210Pbex activity generally decreased in the pattern of a negative exponential curve, although occasional anomalies fluctuated in certain layers (Figure 2). Constant Flux Constant Sedimentation (CFCS) model was then selected to determine the sedimentation rate (Crozaz et al., 1964; Sanchez-Cabeza and Ruiz-Fernández, 2012). On the basis of 210Pbex values and distinct colors from gray to yellowish brown in core photograph, dividing the SKL8-2 into two stages of sedimentation rates was justified. The bottom part (63–102 cm) indicated a sedimentation rate of 2.3 ± 0.6 cm/year, and the upper layer of 0–63 cm had a lower average sedimentation rate of 1.6 ± 0.6 cm/year. Therefore, this core covered a period of approximately 55 years (1964–2019).
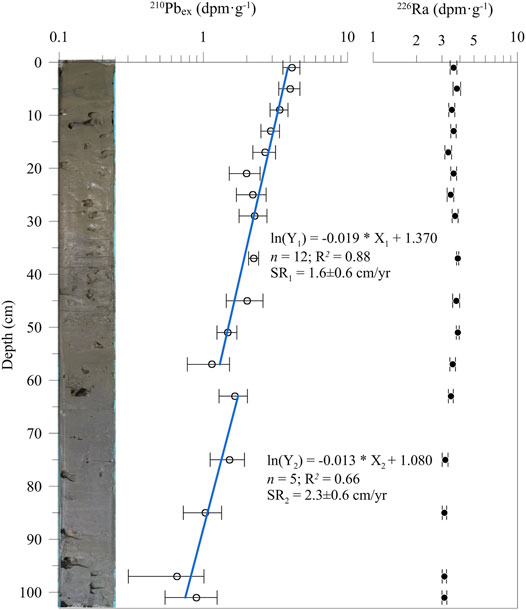
FIGURE 2. Vertical distribution of 210Pbex and 226Ra and a photograph of sediment core SKL8-2. Depth errors are ±1 cm. The blue lines represent the linear fitting results with the detailed information on the right.
Lithology and Sedimentary Environment
Based on the differences of sediment color, magnetic susceptibility, TOC/TN ratio, and calcium content, the core could be divided into three sections (Figure 3): 1) Section A, 63–102 cm corresponding to 1964–1982; 2) Section B, 32–63 cm, spanning from 1982 to 2000; and 3) Section C, 0–32 cm, deposited during 2000–2019.
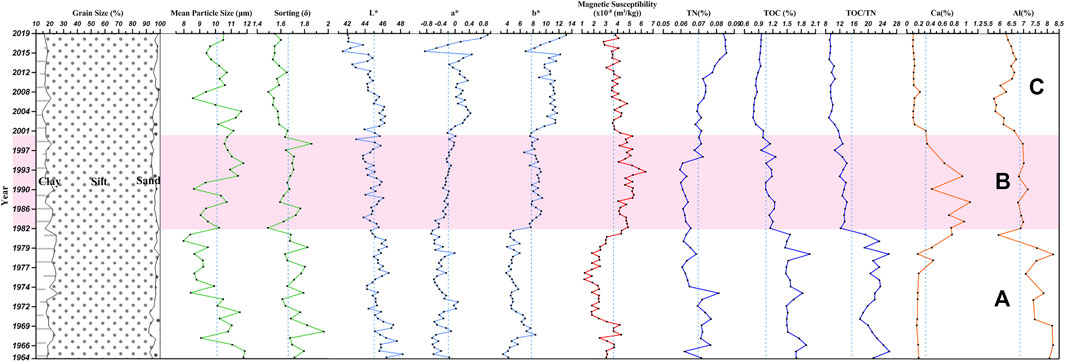
FIGURE 3. Bulk properties of sediment core SKL8-2 collected from Songkhla Lake and its three sections, (A–C). Dashed lines represent the average values.
Section A: 1964–1982
These layers were mainly gray clayey silt with an average silt content of 74.3%, followed by the clay fraction (averaging 21.3%) and sand fraction (averaging 4.4%). The average sorting coefficient was 1.7, indicating poor sorting.
In this section, the sediment was characterized by high TOC content (averaging 1.5%), and there was conspicuous tiny black carbonaceous debris at depths of 70, 73–75, 90, and 95–98 cm. The mean TOC/TN ratio was as high as 21.7, indicating that terrestrial plants prevailed as the source of organic matter (Meyers, 1994). Given the obviously low magnetic susceptibility, b* and a* values, and high TOC and TN, it may be reasonable to conclude that the environment in the lake was relatively reducing (Tian et al., 2011).
The count intensity of chlorine (Cl) was approximately 2,700 cps during 1964–1982 and increased progressively after 1982. The Cl trends in sediment cores SKL7-1 and SKL6-2 were similar to that in SKL8-2 (Figure 4). The period before the rise was consistent with the closure of the GoT channel in order to develop irrigation agriculture by preventing seawater salinity invasion (ONEP, 2005). While the cores collected far away from the channel (e.g., SKL3-2 and SKL1-1) did not show a response to this increasing change of Cl, which further proved the reconnection with the GoT at around 1982.
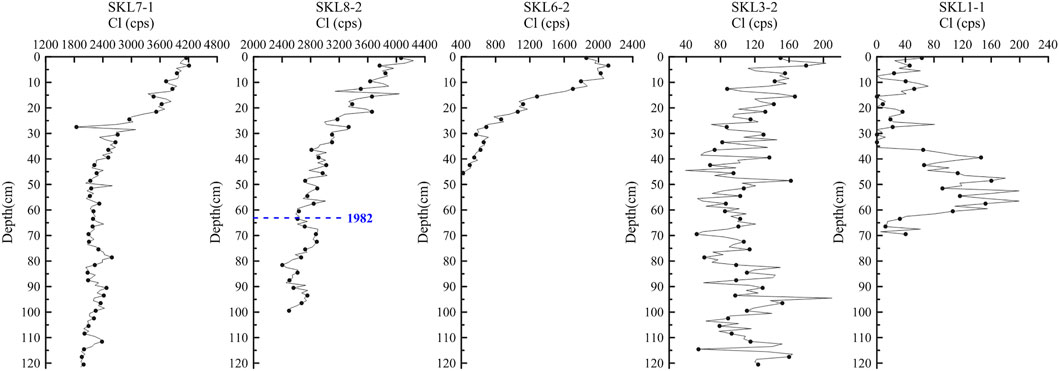
FIGURE 4. Vertical variations of chlorine content at five stations in Songkhla Lake (distance from the channel connected with the Gulf of Thailand: SKL7-1 < SKL8-2 < SKL6-2 < SKL3-2 < SKL1-1).
Section B: 1982–2000
Sediments in Section B were silty sand, slightly coarser than sediments in Section A, with the mean grain size rising from 9.6 to 10.3 μm. The average contents of silt, clay, and sand in this section were 76.7%, 19.2%, and 4.1%, respectively, and the sorting was poor. The mean TOC/TN ratio of 13.2 suggested mixed terrestrial and marine sources for organic matter (Meyers, 1994).
The average calcium content was 0.7%, and Ca content peaked at 1.1% at around 1987. In addition, large amounts of small shells and biological detritus were found in this layer, and pronounced large shells were found at 44-cm depth. We ascribed these findings to the Thai government’s proposal to expand shrimp farming in the Songkhla Lake Basin in 1987 (ONEP, 2005). Since 1987, the area where there previously were rice plantations in Songkhla Lake Basin has been gradually occupied by shrimp ponds. Thus, accelerated shrimp breeding and seafood processing in this period boosted the growth of aquatic shelly organisms and subsequent calcareous deposition in the lake. In addition, this change may also have been caused by the increased productivity of calcareous plankton and/or benthos caused by the opening of the channel with the GoT. As previously reported, nutrients brought by marine plankton can stimulate the growth of calcareous organisms (Yu et al., 2020).
The most intriguing feature of this section was the evident transformation at the bottom interface, where the TOC content, b* value, and magnetic susceptibility all abruptly changed. This was responding to the reconnection of the lake with the GoT. Under marine influence, the hydrodynamic forces in the lake were increased and part of the sediment was transported to the GoT, resulting in the decline in the deposition rate. The dropped TOC and Al contents were considered a consequence of the intensified hydrodynamics as well, rendering it more difficult for fine-grained particulate to sediment. The b* value and magnetic susceptibility increased over their average values, indicating a transition to an oxidizing environment (Tian et al., 2011).
Section C: 2000–2019
Sediments in the upper part of the core (0–32 cm) were gray clayey silt with a mean grain size of 10.2 μm. The average silt content was 79.5%, with 17.1% and 3.4% clay and sand fractions, respectively.
TN and TOC in this section increased considerably, which was consistent with the gradual decrease of brightness. Nevertheless, the organic matter content in this layer was still low, and the average TOC/TN ratio was 9.75, indicating that aquatic plants were the source of the organic matter (Meyers, 1997).
Ca content sharply dropped to below the average of the deeper sections (Figure 3). During this depositional period, local fishery development was contained by either enhanced eutrophication or strengthening regulation; for instance, the use of fishing tools was limited and the area of shrimp aquaculture was reduced. Analysis of satellite images from 2002 previously showed that the shrimp aquaculture area had been reduced to approximately 50.15 km2, 40.2% less than the area in 1995 (Ratanachai et al., 2014). The implementation of environmental regulations led to the decline of nutrients in the lake, which hindered the growth of calcareous organisms and thus caused a decline in sedimentary Ca content. Because there was still marine organism communication during this period, it is reasonable to infer that the plateau of Ca content during 1982–2000 mainly resulted from shrimp farming.
Temporal Variations and Sources of Trace Metals
Trace Metal Concentrations
The ranges of down-core concentrations of As, Hg, Pb, Cr, Ni, Cu, and Zn were 14.82–24.34 (mean 19.04) μg/g, 0.039–0.048 (mean 0.042) μg/g, 45.96–58.55 (mean 49.61) μg/g, 44.11–59.93 (mean 50.05) μg/g, 15.70–25.30 (mean 18.96) μg/g, 9.99–15.94 (mean 11.63) μg/g, and 47.35–53.44 (mean 51.62) μg/g, respectively (Figure 5). In general, the contents of trace metals decreased from the bottom to the top. The downward profiles of Cr, Ni, Cu, and Zn showed similar vertical distributions. Their concentrations exhibited clear declines since 1964 despite peaking at around 1978. In 1981, the turning point year, the decline of trace metal contents slowed considerably, and the values were lower than the mean values. The concentrations of As, Hg, and Pb changed dramatically but presented low values since the 20th century.
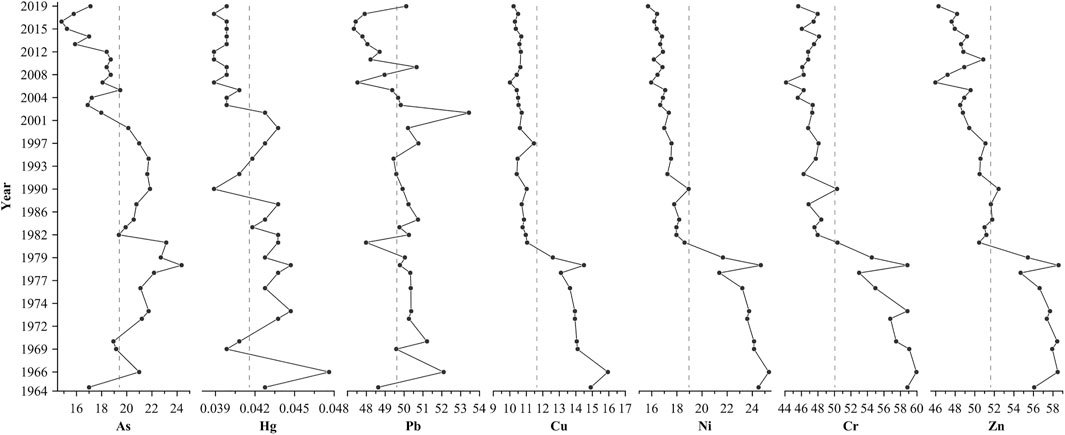
FIGURE 5. Variation of trace metals concentrations (μg/g in dry weight) in sediment core SKL8-2 of Songkhla Lake. Dashed lines represent the average values.
As shown in Table 3, this study revealed that the levels of trace metals in Songkhla Lake were relatively high compared with those of the South China Sea shelf (Cr = 39.3 μg/g, Cu = 7.43 μg/g, Zn = 54.4 μg/g, Pb = 15.6 μg/g, As = 9.71 μg/g, and Hg = 0.02 μg/g) (Zhang and Dui, 2005). However, the concentrations of Cu, Zn, Cr, Ni, and Hg, unlike those of Pb and As, were lower than those in Bangkok Bay (Qiao et al., 2015; Guo et al., 2019), the western GoT (Liu et al., 2016), and the western Sunda Shelf (Zhang et al., 2021). Based on those findings combined with the above results, sediments in the surrounding region of Songkhla Lake were not significantly contaminated by Cu, Zn, Cr, or Ni, while As, Pb, and Hg contents were at moderate contamination levels at many stations in the estuaries primarily because of anthropogenic activities.
Sources of Trace Metals in Sediments
Inter-element correlations were quantified using a correlation matrix. Principal component analysis (PCA) was performed to reduce the dimensionality of the data and determine which elements explained the most variability across the sediment core. PCA generates principal components (PCs), which are uncorrelated variables composed of linear combinations of the original variables. For each PC, a loading is assigned to each of the original variables, determined by correlation between the original variable and the PC. The correlation matrix and PCA were performed on samples from three periods to identify the source of trace metals. The results of sections B and C were similar; therefore, we combined them to obtain the outcomes exhibited in Figure 6 and Table 4.
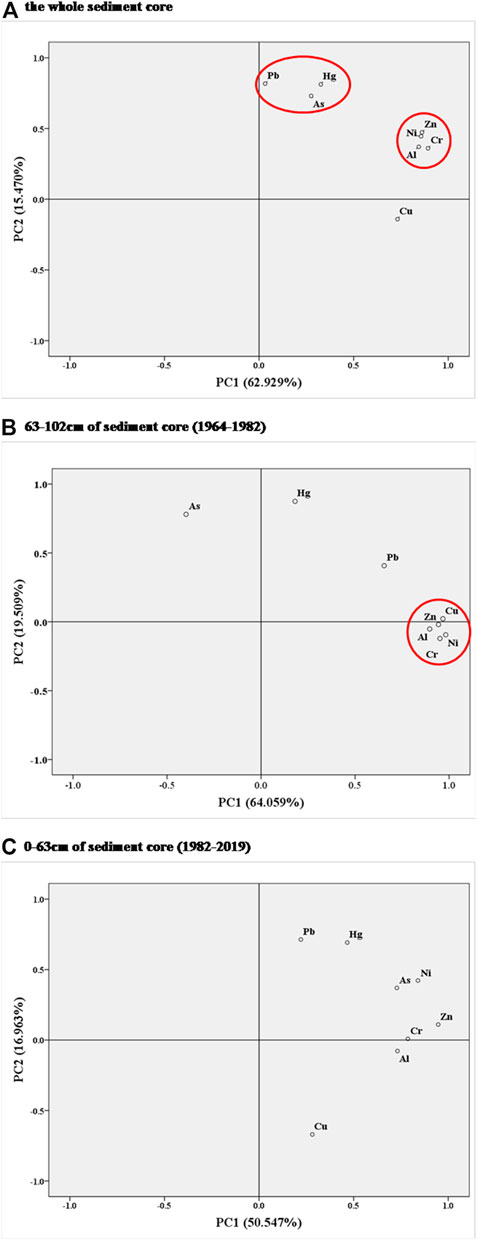
FIGURE 6. Plots of loadings of PC1 and PC2 from principal component analysis (PCA) of sediment core SKL8-2 from Songkhla Lake. (A) The whole sediment core; (B) 63–102 cm of sediment core (1964–1982); (C) 0–63 cm of sediment core (1982–2019).
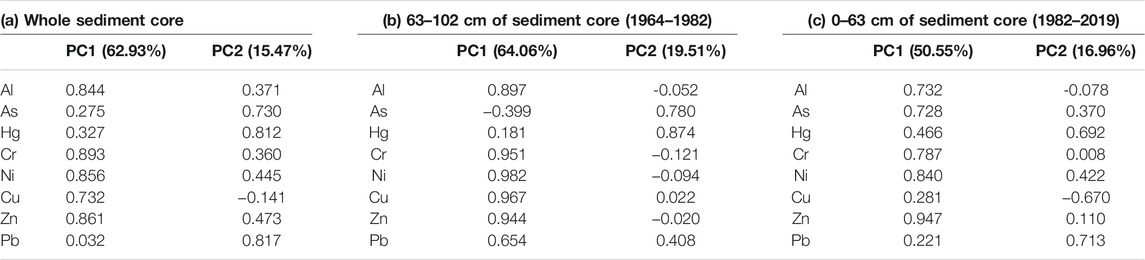
TABLE 4. Explained data variance of the selected principal components (PCs) and their relationships with the individual sediment metals in sediment core SKL8-2.
For the entire sediment core, the first two PCs were responsible for 84% of the total variance, with PC1 accounting for 64% and PC2 explaining 20% of the data variance. In the PCA loading plot (Figure 6A), there are two apparent clusters of trace metals, with Zn, Ni, and Cr having high loadings on PC1 and Pb, Hg, and As having high loadings on PC2. Strong (r > 0.8) and significant (p < 0.01) correlations between trace metals (Zn, Ni, and Cr) and TOC may reflect that organic matter provided more binding sites for metals and thus metals were enriched. Al is generally regarded as a terrestrial proxy; thus, PC1 represented Zn, Ni, and Cr, which are strongly regulated by organic matter and may have originated from lithogenic sources. However, Pb, Hg, and As, represented by PC2, were not correlated with TOC (r <0.5), which may indicate an entirely different regulation pattern from that of the other metals (Figure 6A), likely related to human actions.
Significant correlations between elements may reflect collective sources or similar influencing factors (Zaharescu et al., 2009). During the period from 1964 to 1982, TOC was significantly correlated with TN (r = 0.507; p < 0.01), suggesting a common origin. At the same time, significant correlations between Cr, Ni, Cu, Zn, and Al, as well as high TOC/TN ratio of 21.7, jointly shed light on the proximate natural terrigenous input. Accordingly, PC1 of this part, which encompasses high loading of Cr, Ni, Cu, Zn, and Al, accounted for 64% of the variance. PC2 is distinguished by positive loading of As, Hg, and Pb, indicating greater anthropogenic contribution (Figure 6B).
After 1982, aside from Zn and Ni, the correlations between other indicators weakened (r < 0.5). This indicates that the sources of trace metals after 1982 were more complex than those before 1982 and may have resulted from the following three causes. First, rapid urban and industrial expansion with outdated waste treatment brought large amounts of effluent discharge into the lake (Pradit et al., 2013). This cause may be supported by the striking distinction of the PC loads of Cu and As after 1982 compared with those before 1982. Cu and As have generally been used as wood preservatives, and their material sources were closely related to the rapid development of the wood industry around Songkhla Lake in the 1990s (Sompongchaiyakul and Sirinawin, 2007). Aquaculture may have been the next most important contamination source. The Thai government’s policies stimulated the development of aquaculture starting in 1987. The use of fishing gear and tackle, fertilizer application, food processing, and ultimately transportation may account for the contamination of the lake (Ratanachai et al., 2014). Furthermore, the reconnection of the lake with the ocean exacerbated the complexity of material sources.
Potential Ecological Assessment
The EF and Igeo were used to quantitatively evaluate the degree of anthropogenic pollution in the sediments, and Al was selected as the standardized element because Al2O3 content was stable during the formation of the sediments and was uninfluenced by the surrounding environment (Cheevaporn et al., 1995; Srisuksawad et al., 1997). The background values of Cr, Ni, Cu, Zn, Pb, As, and Hg were 58.5, 13.0, 17.7, 35.5, 20.5, 13.0, and 0.021 μg/g, respectively, with Al content of 5.15%, which was determined through previous studies of Songkhla Lake and the western GoT (Ladachart et al., 2011; Liu et al., 2016).
The EF and Igeo results suggest that trace metal contamination was not severe in Songkhla Lake from 1964 onward (Figure 7). The average EF values were ranked as follows: Pb > Hg > As > Zn > Ni > Cr > Cu. The first five elements exhibited EF > 1, denoting slight contamination. The EF results for Cr and Cu were much lower than 1, indicating natural enrichment. The Igeo values showed the same order as the EF index. Pb and Hg maintained the Igeo > 0 throughout the core, suggesting moderate levels of contamination. The Igeo values of Zn and Ni decreased to less than zero starting approximately in 1981. Cr and Cu showed no enrichment.
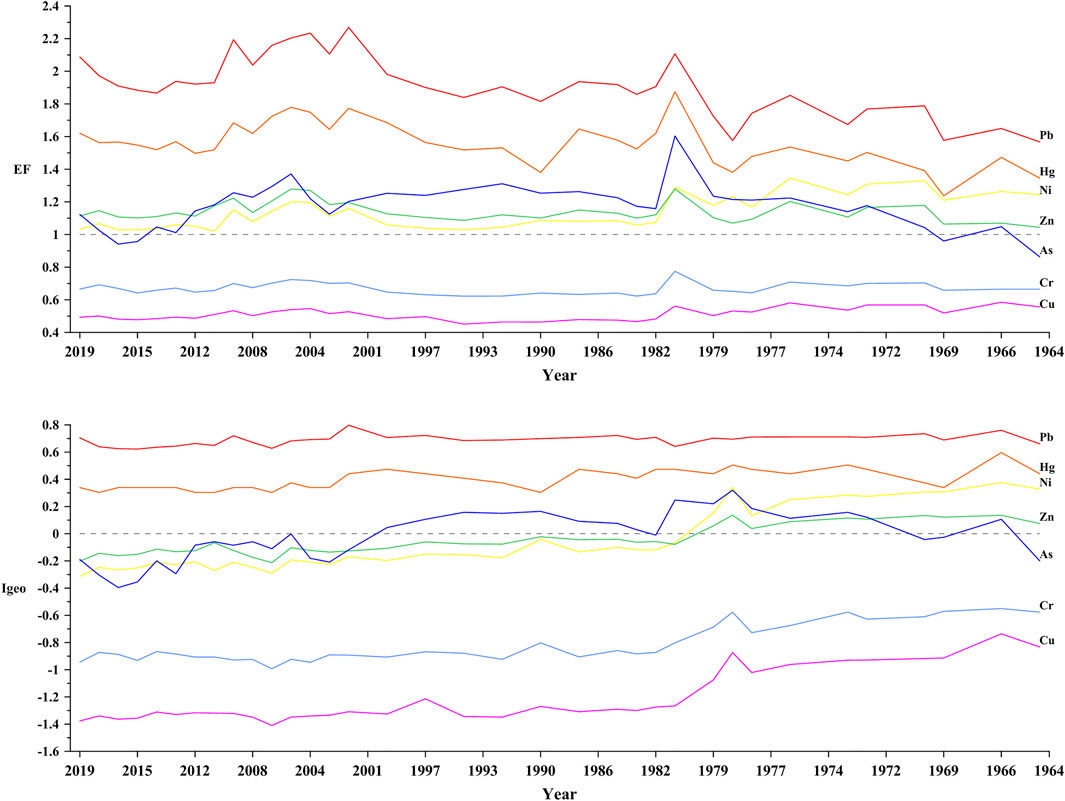
FIGURE 7. Enrichment factor (EF) and index of geo-accumulation (Igeo) of trace metals in sediment core SKL8-2 of Songkhla Lake.
It was notable that except Cr and Cu, trace metals showed contamination signals in the depth range of 15–32 cm, corresponding to 2000–2010. This result was also supported by previous reports (Sompongchaiyakul and Sirinawin, 2007; Pradit et al., 2010; Pradit et al., 2013), which showed that concentrations of As and Pb were rising because of rapid urbanization. The outer section of Songkhla Lake receives municipal waste from two large and rapidly expanding cities, Songkhla and Hat Yai, as well as agricultural and industrial discharge transported by canals. In the 1990s, urbanization accelerated, but the first wastewater treatment plant for Hat Yai City was not built until 1999 and the treatment coverage was limited. Therefore, a large amount of untreated wastewater was still discharged into Songkhla Lake, resulting in a decline of lake water quality and environmental degradation. This poor sewage treatment had led to serious concern with regard to nutrient and metallic pollution in Songkhla Lake. In addition, expansion of Songkhla Port in 2003 (ONEP, 2005) and expansion of rubber planting in Songkhla Lake stimulated by the rising price of rubber from 2003 to 2005 may have facilitated the high enrichment in this period as well. After 2010, pollution has been kept to a low level, which was likely closely related to the concept of “man–land integration and coordinated development” in this period (Ratanachai et al., 2013).
Comparison with the ERL–ERM guideline values (Figure 8) showed that all the trace metals were under the ERM guideline values, reflecting contamination that at present is far from alarming. Nevertheless, As and Pb contents have exceeded the ERL threshold since 1964. Although the As content in Southeast Asia has been found to be elevated relative to global levels, which is considered to result from mining or river enrichment (Jones et al., 2008), the As and Pb contents in SKL8-2 were higher than the 10th percentile of the effects database and are a signal of the sustained influence of human activity, which requires attention. In addition, Ni exceeded the ERL limit in the period during 1964–1982. This finding further indicates terrigenous input in this period, as in nature, Ni mostly occurs in magnetite and sulfide deposits (Guo et al., 2019). Moreover, it was found that the concentrations of Hg, Cr, Cu, and Zn in the sediments did not exceed the ERL limits for sediments during the entire recorded period, indicating that harmful effects on organisms would be rare. In general, these results were in agreement with the obtained EF and Igeo values. However, because the Hg content was relatively low in adjacent areas (Liu et al., 2016; Zhang et al., 2021), the results for this element showed a different contamination status.
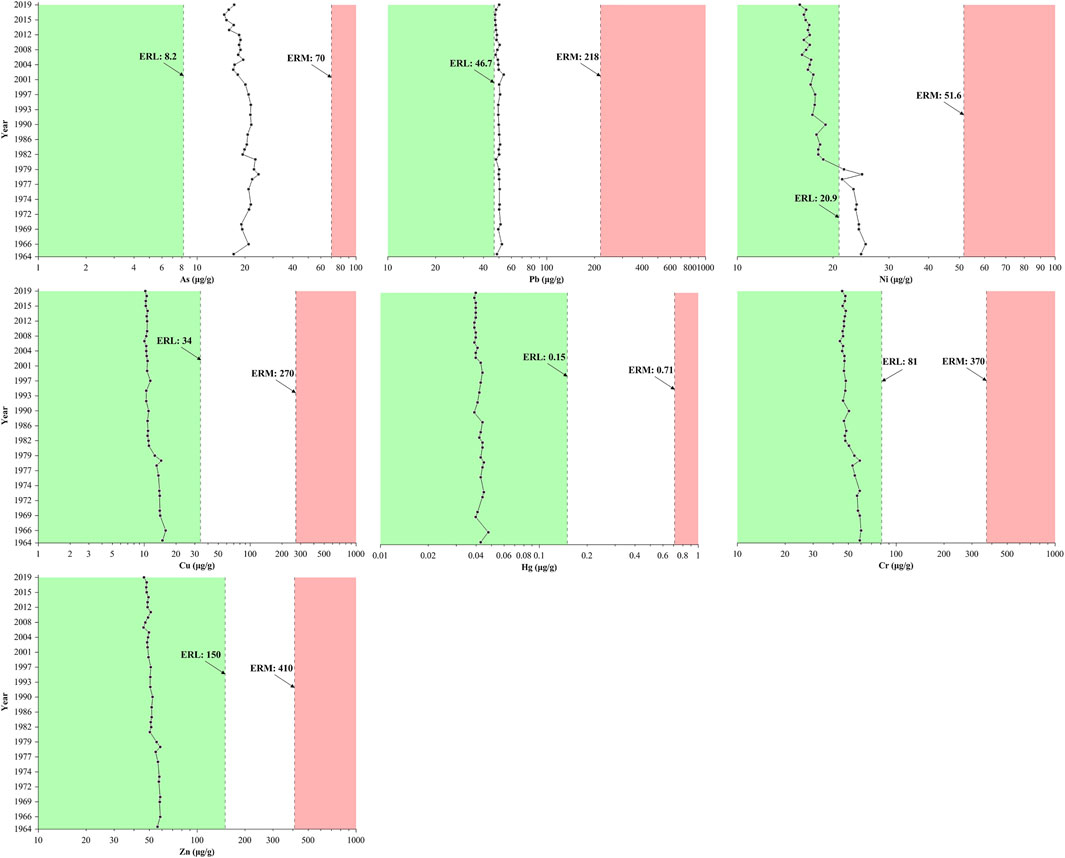
FIGURE 8. Comparison of trace metals concentrations in sediment core SKL8-2 of Songkhla Lake with ERL (effects range low) and ERM (effects range median) guideline values. The green part below the ERL is where harmful effects on aquatic biota are rarely observed. The red area above the ERM indicates severe harmful effects.
Conclusion
This is one of the first studies on the influences of anthropogenic activities and sea–land interactions on the modern sedimentary metal accumulation history of Songkhla Lake. We have provided a comprehensive record of the geochronology, lithology, and geochemistry of metal contamination in lake sediment core SKL8-2. The 55-year record reveals a three-stage input history of sedimentary trace metals: 1) From 1964 to 1982, the channel connecting Songkhla Lake and the GoT was closed, and there was a natural terrigenous input period with a relatively reducing environment. The deposition rate was high during this period, approximately 2.3 ± 0.6 cm/year, trace metal and TOC contents were high with significant correlation, and the mean TOC/TN ratio was as high as 21.7. The b* value and magnetic susceptibility were markedly low. 2) Almost all of the recorded values changed sharply in 1982. During 1982–2000, with the reopening of the channel with the GoT and the intensive development of human activities, the sources of trace metals were more complex than before and mainly came from urban wastewater, industrial effluent, and fishery discharge. 3) From 2000 to 2019, because of the rapid urban development and poor sewage treatment, contamination signals of Pb, Hg, As, Zn, and Ni emerged in the first decade, and this contamination was then alleviated with increasing environmental management efforts. Generally, based on trace metal element analysis, the sediment of Songkhla Lake was at a low contamination level, with the enrichment order Pb > Hg > As > Zn > Ni > Cr > Cu. Herein, Pb, Hg, As, Zn, and Ni showed moderate enrichment, while Cr and Cu exhibited no enrichment. The obtained results will be useful for future assessment of changes in the coastal environment under anthropogenic influences. Although there are signs of contamination mitigation from reduced emissions, trace metal contamination in Songkhla Lake should be closely observed in the long term, as intensified human activities and natural processes enhance trace elements release into the environment.
Data Availability Statement
The raw data supporting the conclusions of this article will be made available by the authors without undue reservation.
Author Contributions
KD, SQ, and BW designed the study, synthesized, analyzed the data, and wrote the article, with contributions from all authors. NK and SK provided background information of the study area. BW, YC, XS, SL, NK, and SK helped to collect the core samples. XS, YC, and KD subsampled and photographed the cores. KD and YC completed all the experiments. SQ and XS analyzed the data related to the sedimentology. KD, BW, and XS analyzed the data of geochemical parameters. All authors contributed to the article and approved the submitted version.
Conflict of Interest
The authors declare that the research was conducted in the absence of any commercial or financial relationships that could be construed as a potential conflict of interest.
The handling Editor declared a past co-authorship with one of the authors (XS).
Publisher’s Note
All claims expressed in this article are solely those of the authors and do not necessarily represent those of their affiliated organizations, or those of the publisher, the editors, and the reviewers. Any product that may be evaluated in this article, or claim that may be made by its manufacturer, is not guaranteed or endorsed by the publisher.
Acknowledgments
The authors are grateful to Dr. Peng Cao and Yuan Dang of First Institute of Oceanography, Ministry of Natural Resources, China, for sample collection. This work was jointly supported by the National Program on Global Change and Air-Sea Interaction (GASI-GEOGE-03), National Nature Science Foundation of China (U1606401), and the cooperative research program between China and Thailand “Research on Vulnerability of Coastal Zones.”
References
Anthony, A., Atwood, J., August, P., Byron, C., Cobb, S., Foster, C., et al. (2016). Coastal Lagoons and Climate Change: Ecological and Social Ramifications in U.S. Atlantic and Gulf Coast Ecosystems. ECOL SOC 14, 1–5. Available at: http://www.ecologyandsociety.org/vol14/iss1/art8/.
Aparecida Leite Silva, M., and Eduardo Rezende, C. (2002). Behavior of Selected Micro and Trace Elements and Organic Matter in Sediments of a Freshwater System in South-East Brazil. Sci. Total Environ. 292, 121–128. doi:10.1016/S0048-9697(02)00034-7
Bakshi, M., Ghosh, S., Chakraborty, D., Hazra, S., and Chaudhuri, P. (2018). Assessment of Potentially Toxic Metal (PTM) Pollution in Mangrove Habitats Using Biochemical Markers: A Case Study on Avicennia officinalis L. In and Around Sundarban, India. Mar. Pollut. Bull. 133, 157–172. doi:10.1016/j.marpolbul.2018.05.030
Barbier, E. B. (2015). Valuing the Storm Protection Service of Estuarine and Coastal Ecosystems. Ecosystem Serv. 11, 32–38. doi:10.1016/j.ecoser.2014.06.010
Bryan, G. W., and Langston, W. J. (1992). Bioavailability, Accumulation and Effects of Heavy Metals in Sediments with Special Reference to United Kingdom Estuaries: a Review. Environ. Pollut. 76, 89–131. doi:10.1016/0269-7491(92)90099-V
Buat-Menard, P., and Chesselet, R. (1979). Variable Influence of the Atmospheric Flux on the Trace Metal Chemistry of Oceanic Suspended Matter. Earth Planet. Sci. Lett. 42, 399–411. doi:10.1016/0012-821X(79)90049-9
Cheevaporn, V., Jacinto, G. S., and San Diego-Mcglone, M. L. (1995). Heavy Metal Fluxes in Bang Pakong River Estuary, Thailand: Sedimentary vs Diffusive Fluxes. Mar. Pollut. Bull. 31, 290–294. doi:10.1016/0025-326X(95)00195-S
Crozaz, G., Picciotto, E., and De Breuck, W. (19641896-1977). Antarctic Snow Chronology with Pb210. J. Geophys. Res. 69, 2597–2604. doi:10.1029/JZ069i012p02597
Doungsuwan, N., Ratanachai, C., Somgpongchaiyakul, P., and Sangganjanavanich, P. (2013). Impacts of the National Economic and Social Development Plan on Songkhla Lake Basin Development Thailand. Iber 12, 895. doi:10.19030/iber.v12i8.7987
Doungsuwan, N., Ratanachai, C., Somgpongchaiyakul, P., and Sangganjanavanich, P. (2014). Sustainability Indicators for Fishery Management in Songkhla Lake, Thailand. Jsm 1, 5–12. doi:10.19030/jsm.v1i1.8388
El Zrelli, R., Yacoubi, L., Wakkaf, T., Castet, S., Grégoire, M., Mansour, L., et al. (2021). Surface Sediment Enrichment with Trace Metals in a Heavily Human-Impacted Lagoon (Bizerte Lagoon, Southern Mediterranean Sea): Spatial Distribution, Ecological Risk Assessment, and Implications for Environmental protection. Mar. Pollut. Bull. 169, 112512. doi:10.1016/j.marpolbul.2021.112512
Faremi, O. E., Sogbanmu, T. O., and Adeyemo, O. K. (2021). How Sawmill Wastes Impact Surface Water, Sediment, Macrobenthic Invertebrates, and Fish: a Case Study of the Lagos Lagoon, Okobaba Area, South-Western Nigeria. Environ. Monit. Assess. 193, 235. doi:10.1007/s10661-021-09006-0
Filgueiras, A., Lavilla, I., and Bendicho, C. (2004). Evaluation of Distribution, Mobility and Binding Behaviour of Heavy Metals in Surficial Sediments of Louro River (Galicia, Spain) Using Chemometric Analysis: a Case Study. Sci. Total Environ. 330, 115–129. doi:10.1016/j.scitotenv.2004.03.038
Gadkar, N. S., Nayak, G. N., and Nasnodkar, M. R. (2019). Assessment of Metal Enrichment and Bioavailability in Mangrove and Mudflat Sediments of the Tropical (Zuari) Estuary, West Coast of India. Environ. Sci. Pollut. Res. 26, 24998–25011. doi:10.1007/s11356-019-05733-7
Guo, Y., Qiao, S., Shi, X., Wu, B., Yuan, L., Ren, Y., et al. (2019). Variation Trend and Contamination Source of Heavy Metals in Sediments from Estuary Area of Bangkok Bay in the Past century. Mar. Geol. Quatern Geol. 39, 61–69. doi:10.16562/j.cnki.0256-1492.2018031901
Hinkey, L. M., and Zaidi, B. R. (2007). Differences in SEM-AVS and ERM-ERL Predictions of Sediment Impacts from Metals in Two US Virgin Islands Marinas. Mar. Pollut. Bull. 54, 180–185. doi:10.1016/j.marpolbul.2006.09.009
Hung, J.-J., Hung, C.-S., Wann, C.-K., Hung, P.-Y., and Kuo, F. (2020). Mercury Distribution and Speciation in Two Lagoons with Different Pollution and Eutrophication Conditions in Taiwan. Mar. Pollut. Bull. 156, 111096. doi:10.1016/j.marpolbul.2020.111096
Jones, H., Visoottiviseth, P., Khoda Bux, M., Földényi, R., Kováts, N., Borbély, G., et al. (2008). “Case Reports: Arsenic Pollution in Thailand, Bangladesh, and Hungary,” in Reviews of Environmental Contamination Volume 197: International Perspectives on Arsenic Pollution and Remediation (New York, NYNew York: Springer), 163–187. doi:10.1007/978-0-387-79284-2_6
Jung, J.-M., Choi, K.-Y., Chung, C.-S., Kim, C.-J., and Kim, S. H. (2019). Fractionation and Risk Assessment of Metals in Sediments of an Ocean Dumping Site. Mar. Pollut. Bull. 141, 227–235. doi:10.1016/j.marpolbul.2019.02.041
Kumblad, L., Olsson, A., Koutny, V., and Berg, H. (2001). Distribution of DDT Residues in Fish from the Songkhla Lake, Thailand. Environ. Pollut. 112, 193–200. doi:10.1016/S0269-7491(00)00118-4
Ladachart, R., Suthirat, C., Hisada, K.-i., and Charusiri, P. (2011). Distribution of Heavy Metals in Core Sediments from the Middle Part of Songkhla Lake, Southern Thailand. J. Appl. Sci. 11, 3117–3129. doi:10.3923/jas.2011.3117.3129
Laermanns, H., Pint, A., Bellanova, P., Feist, L., Wagner, B., Frank, S., et al. (2021). The Santo André Lagoon at the Atlantic Coast of Portugal - Holocene Evolution and Event History. Palaeogeogr. Palaeoclimatol. Palaeoecol. 571, 110366. doi:10.1016/j.palaeo.2021.110366
Liu, E., Shen, J., Liu, X., Zhu, Y., and Wang, S. (2006). Variation Characteristics of Heavy Metals and Nutrients in the Core Sediments of Taihu Lake and Their Pollution History. Sci. China Ser. D 49, 82–91. doi:10.1007/s11430-006-8108-6
Liu, S., Shi, X., Yang, G., Khokiattiwong, S., and Kornkanitnan, N. (2016). Concentration Distribution and Assessment of Heavy Metals in the Surface Sediments of the Western Gulf of Thailand. Environ. Earth Sci. 75, 346. doi:10.1007/s12665-016-5422-y
Long, E. R., Macdonald, D. D., Smith, S. L., and Calder, F. D. (1995). Incidence of Adverse Biological Effects within Ranges of Chemical Concentrations in marine and Estuarine Sediments. Environ. Manage. 19, 81–97. doi:10.1007/BF02472006
Macdonald, D. D., Ingersoll, C. G., and Berger, T. A. (2000). Development and Evaluation of Consensus-Based Sediment Quality Guidelines for Freshwater Ecosystems. Arch. Environ. Contam. Toxicol. 39, 20–31. doi:10.1007/s002440010075
Meyers, P. A. (1997). Organic Geochemical Proxies of Paleoceanographic, Paleolimnologic, and Paleoclimatic Processes. Org. Geochem. 27, 213–250. doi:10.1016/S0146-6380(97)00049-1
Meyers, P. A. (1994). Preservation of Elemental and Isotopic Source Identification of Sedimentary Organic Matter. Chem. Geology. 114, 289–302. doi:10.1016/0009-2541(94)90059-0
Müller, G. (1979). Schwermetalle in den Sedimenten des Rheins-Veränderungen seit. Umschav 79, 133–149.
Nakinchart, P., Sompongchaiyakul, P., and Sirinawin, W. (2006). Trace Metal Levels in Surficial Sediments of Songkhla Lake. Bangkok, Thailand: The Internation Conference on Hazardous Waste Management for Sustainable Future.
Newton, A., Brito, A. C., Icely, J. D., Derolez, V., Clara, I., Angus, S., et al. (2018). Assessing, Quantifying and Valuing the Ecosystem Services of Coastal Lagoons. J. Nat. Conservation 44, 50–65. doi:10.1016/j.jnc.2018.02.009
Nriagu, J. O. (1996). A History of Global Metal Pollution. Science 272, 223. doi:10.1126/science.272.5259.223
Office of Natural Resources and Environmental Policy and Planning, OPEN (2005). Master Plan for Songkhla Lake Basin Development. Hat Yai, Thailand: Final Report by Prince of Songkla University, Taksin University and Songkhla Rajabhat University.
Pedro, C. A., Santos, M. S. S., Ferreira, S. M. F., and Gonçalves, S. C. (2016). The Presence of Cadmium in the Intertidal Environments of a Moderately Impacted Coastal Lagoon in Western Portugal (Óbidos Lagoon)-Spatial and Seasonal Evaluations. Environ. Sci. Pollut. Res. 23, 1960–1969. doi:10.1007/s11356-015-5847-y
Pérez-Ruzafa, A., Marcos, C., Bernal, C. M., Quintino, V., Freitas, R., Rodrigues, A. M., et al. (2012). Cymodocea Nodosa vs. Caulerpa Prolifera: Causes and Consequences of a Long Term History of Interaction in Macrophyte Meadows in the Mar Menor Coastal Lagoon (Spain, Southwestern Mediterranean). Estuarine, Coastal Shelf Sci. 110, 101–115. doi:10.1016/j.ecss.2012.04.004
Pérez-Ruzafa, A., Mompeán, M. C., and Marcos, C. (2007). “Hydrographic, Geomorphologic and Fish Assemblage Relationships in Coastal Lagoons,” in Lagoons and Coastal Wetlands in the Global Change Context: Impacts and Management Issues. Editors P. Viaroli, and P. Lasserre (Campostrini: Springer Netherlands), 107–125.
Pradit, S., Pattarathomrong, M. S., and Panutrakul, S. (2013). Arsenic Cadmium and Lead Concentrations in Sediment and Biota from Songkhla Lake: A Review. Proced. - Soc. Behav. Sci. 91, 573–580. doi:10.1016/j.sbspro.2013.08.456
Pradit, S., Shazili, N. A. M., Pattaratumrong, M. S., Chotikarn, P., Kobkeatthawin, T., Yucharoen, M., et al. (2018). Accumulation of Trace Metals in Mangrove Plant Soneratia Caseoralis in Songkhla Lake, Thailand. Appl. Ecol. Env. Res. 16, 4081–4095. doi:10.15666/aeer/1604_40814095
Pradit, S., Wattayakorn, G., Angsupanich, S., Baeyens, W., and Leermakers, M. (2010). Distribution of Trace Elements in Sediments and Biota of Songkhla Lake, Southern Thailand. Water Air Soil Pollut. 206, 155–174. doi:10.1007/s11270-009-0093-x
Qiao, S., Shi, X., Gao, J., Zhu, A., Narumol, K., Hu, L., et al. (2015). Enrichment and Bioavailability of Heavy Metals in Sediments of the Bangkok Bay. China Environ. Sci. 35, 3445–3451.
Sanchez-Cabeza, J. A., and Ruiz-Fernández, A. C. (2012). 210Pb Sediment Radiochronology: An Integrated Formulation and Classification of Dating Models. Geochimica et Cosmochimica Acta 82, 183–200. doi:10.1016/j.gca.2010.12.024
Schmidt, H., and Reimers, C. E. (1991). The Recent History of Trace Metal Accumulation in the Santa Barbara Basin, Southern California Borderland. Estuarine, Coastal Shelf Sci. 33, 485–500. doi:10.1016/0272-7714(91)90086-Q
Sogbanmu, T. O., Nagy, E., Phillips, D. H., Arlt, V. M., Otitoloju, A. A., and Bury, N. R. (2016). Lagos Lagoon Sediment Organic Extracts and Polycyclic Aromatic Hydrocarbons Induce Embryotoxic, Teratogenic and Genotoxic Effects in Danio rerio (Zebrafish) Embryos. Environ. Sci. Pollut. Res. 23, 14489–14501. doi:10.1007/s11356-016-6490-y
Sompongchaiyakul, P., and Sirinawin, W. (2007). Arsenic, Chromium and Mercury in Surface Sediment of Songkhla Lake, Thailand. Asian J. Water Environ. Pollu. 4, 17–24.
Srisuksawad, K., Porntepkasemsan, B., Nouchpramool, S., Yamkate, P., Carpenter, R., Peterson, M. L., et al. (1997). Radionuclide Activities, Geochemistry, and Accumulation Rates of Sediments in the Gulf of Thailand. Continental Shelf Res. 17, 925–965. doi:10.1016/S0278-4343(96)00065-9
Tian, Q., Yang, T., Zhang, S., Shi, P., Zhang, J., and Fan, Z. (2011). Magnetic Susceptibility and its Environmental Significance of Lake Sediments in Tibet Plateau. Acta Sedimentol Sin 29, 143–150. doi:10.14027/j.cnki.cjxb.2011.01.011
Veiga, K., Pedro, C. A., Ferreira, S. M. F., and Gonçalves, S. C. (2019). Monitoring Metal Pollution on Coastal Lagoons Using Cerastoderma Edule-A Report from a Moderately Impacted System in Western Portugal (Óbidos Lagoon). Environ. Sci. Pollut. Res. 26, 2710–2721. doi:10.1007/s11356-018-3705-4
Velasco, A. M., Pérez-Ruzafa, A., Martínez-Paz, J. M., and Marcos, C. (2018). Ecosystem Services and Main Environmental Risks in a Coastal Lagoon (Mar Menor, Murcia, SE Spain): The Public Perception. J. Nat. Conservation 43, 180–189. doi:10.1016/j.jnc.2017.11.002
Violintzis, C., Arditsoglou, A., and Voutsa, D. (2009). Elemental Composition of Suspended Particulate Matter and Sediments in the Coastal Environment of Thermaikos Bay, Greece: Delineating the Impact of Inland Waters and Wastewaters. J. Hazard. Mater. 166, 1250–1260. doi:10.1016/j.jhazmat.2008.12.046
Wakkaf, T., El Zrelli, R., Kedzierski, M., Balti, R., Shaiek, M., Mansour, L., et al. (2020). Characterization of Microplastics in the Surface Waters of an Urban Lagoon (Bizerte Lagoon, Southern Mediterranean Sea): Composition, Density, Distribution, and Influence of Environmental Factors. Mar. Pollut. Bull. 160, 111625. doi:10.1016/j.marpolbul.2020.111625
Yu, Z., Li, B., Li, H., Zhang, J., and Chen, J. (2020). Response of Modern Planktonic Foraminifera to the Upwelling Activity in the Southwestern South China Sea. QUATERN SCI. 40, 801–810. doi:10.11928/j.issn.1001-7410.2020.03.17
Zaharescu, D. G., Hooda, P. S., Soler, A. P., Fernandez, J., and Burghelea, C. I. (2009). Trace Metals and Their Source in the Catchment of the High Altitude Lake Respomuso, Central Pyrenees. Sci. Total Environ. 407, 3546–3553. doi:10.1016/j.scitotenv.2009.02.026
Zhang, H., Liu, S., Wu, K., Cui, J., Zhu, A., Zhang, Y., et al. (2021). Distribution and Assessment of Heavy Metal Contents in Surface Sediments of the Western Sunda Shelf. Mar. Pollut. Bull. 168, 112433. doi:10.1016/j.marpolbul.2021.112433
Zhang, Y., and Dui, J. (2005). Background Values of Pollutants in Sediments of the South China Sea. Acta Oceanol Sin 27, 161–166. doi:10.1525/aa.1958.60.5.02a00220
Keywords: lagoon, Songkhla Lake, Gulf of Thailand, modern sedimentation, anthropogenic activities, trace metal contamination
Citation: Dong K, Qiao S, Wu B, Shi X, Chen Y, Shan X, Liu S, Kornkanitnan N and Khokiattiwong S (2021) Sedimentary History of Trace Metals Over the Past Half-Century in Songkhla Lake, Western Coast of the Gulf of Thailand: Anthropogenic Impacts and Contamination Assessment. Front. Earth Sci. 9:767899. doi: 10.3389/feart.2021.767899
Received: 31 August 2021; Accepted: 21 October 2021;
Published: 29 November 2021.
Edited by:
Xiting Liu, Ocean University of China, ChinaCopyright © 2021 Dong, Qiao, Wu, Shi, Chen, Shan, Liu, Kornkanitnan and Khokiattiwong. This is an open-access article distributed under the terms of the Creative Commons Attribution License (CC BY). The use, distribution or reproduction in other forums is permitted, provided the original author(s) and the copyright owner(s) are credited and that the original publication in this journal is cited, in accordance with accepted academic practice. No use, distribution or reproduction is permitted which does not comply with these terms.
*Correspondence: Shuqing Qiao, cWlhb3NodXFpbmdAZmlvLm9yZy5jbg==