- 1Ecology and Evolutionary Biology, University of Reading, Reading, United Kingdom
- 2School of Archaeology, Geography and Environmental Science, University of Reading, Reading, United Kingdom
- 3Quaternary Geology, Department of Environmental Sciences, University of Basel, Basel, Switzerland
The fluctuating climatic conditions of the Saharo-Arabian deserts are increasingly linked to human evolutionary events and societal developments. On orbital timescales, the African and Indian Summer Monsoons were displaced northward and increased precipitation to the Arabian Peninsula which led to favorable periods for human occupation in the now arid interior. At least four periods of climatic optima occurred within the last 130,000 years, related to Marine Isotope Stages (MIS) 5e (128–121 ka BP), 5c (104–97 ka BP), 5a (81–74 ka BP) and 1 (10.5–6.2 ka BP), and potentially early MIS 3 (60–50 ka BP). Stalagmites from Southern Arabia have been key to understanding climatic fluctuations and human-environmental interactions; their precise and high-resolution chronologies can be linked to evidence for changes in human distribution and climate/environment induced societal developments. Here, we review the most recent advances in the Southern Arabian Late Pleistocene and Early Holocene stalagmite records. We compare and contrast MIS 5e and Early Holocene climates to understand how these differed, benchmark the extremes of climatic variability and summarize the impacts on human societal development. We suggest that, while the extreme of MIS 5e was important for H. sapeins dispersal, subsequent, less intense, wet phases mitigate against a simplistic narrative. We highlight that while climate can be a limiting and important factor, there is also the potential of human adaptability and resilience. Further studies will be needed to understand spatio-temporal difference in human-environment interactions in a climatically variable region.
Introduction
The fluctuating palaeoclimate conditions of Southern Arabia are frequently related to broad changes in hominin distribution as well as regional societal developments. Intensifications and expansions of the monsoon domain increased precipitation across Southern Arabia during periods of increased solar insolation, following orbitally-paced cycles (Burns et al., 1998; Burns et al., 2001; Fleitmann et al., 2003b; Fleitmann et al., 2011; Parton et al., 2015b; Jennings et al., 2015; Nicholson et al., 2020). During the last 130 kyrs, at least four prolonged periods of increased precipitation have been identified and dated to MIS 5e (128–121 ka BP), MIS 5c (104–97 ka BP), MIS 5a (81–74 ka BP) and the Early Holocene (10.5–6.2 ka BP), and perhaps early MIS 3 (60–50 ka BP), each lasting for a few millennia (Burns et al., 2001; Fleitmann et al., 2011; Parton et al., 2013; Nicholson et al., 2020). These extreme increases in rainfall permitted the formation of large, deep and perennial lakes and other waterbodies in the now arid interiors (Rosenberg et al., 2011; Rosenberg et al., 2012; Petraglia et al., 2012; Rosenberg et al., 2013; Parton et al., 2013; Parton et al., 2015a). As well as increased surface water availability and refilling of aquifers, increased rainfall led to “greening” events of Arabia, in which grassland environments expanded into the now arid desert interiors and supported the spread of large mammals and human settlement (Rose et al., 2011; Petraglia et al., 2012; Groucutt et al., 2015; Stimpson et al., 2016; Groucutt et al., 2018; Stewart et al., 2020a; Stewart et al., 020b; Groucutt et al., 2021; Scerri et al., 2021).
Speleothems (stalagmites, stalactites and flowstones) have been key sources of terrestrial palaeoclimatic information in Southern Arabia. Unlike other terrestrial archives (e.g., lacustrine and alluvial records), their subterranean location protects them from desert weathering conditions (Burns et al., 1998; Vaks et al., 2010; El-Shenawy et al., 2018; Burstyn et al., 2019; Henselowsky et al., 2021). Additionally, speleothem growth requires a positive precipitation-evaporation balance, and can thus inform the timing of prolonged soil humidity above the cave. Stalagmites are particularly useful for palaeoclimate reconstructions; their laminated growth permits the development of precise climatic records through U-Th dating and analyses of calcite oxygen (δ18Oca) and carbon (δ13Cca) stable-isotopes, which can be linked to archaeological (and historical) records. Since 1998, a series of publications have provided a unique insight into the palaeoclimate of Southern Arabia using stalagmites collected from Hoti (23.08° N, 57.35° E), Mukallah (14.91° N, 48.59° E), and Qunf (17.16° N, 54.3° E) caves (Figure 1A). Specific site descriptions of the caves are available elsewhere (Burns et al., 1998; Neff et al., 2001; Fleitmann et al., 2003b; Fleitmann et al., 2003a; Fleitmann et al., 2007; Fleitmann et al., 2011). Here, we summarize these works and provide a comparison between two of these climatically extreme periods: MIS 5e and the Early-Mid Holocene.
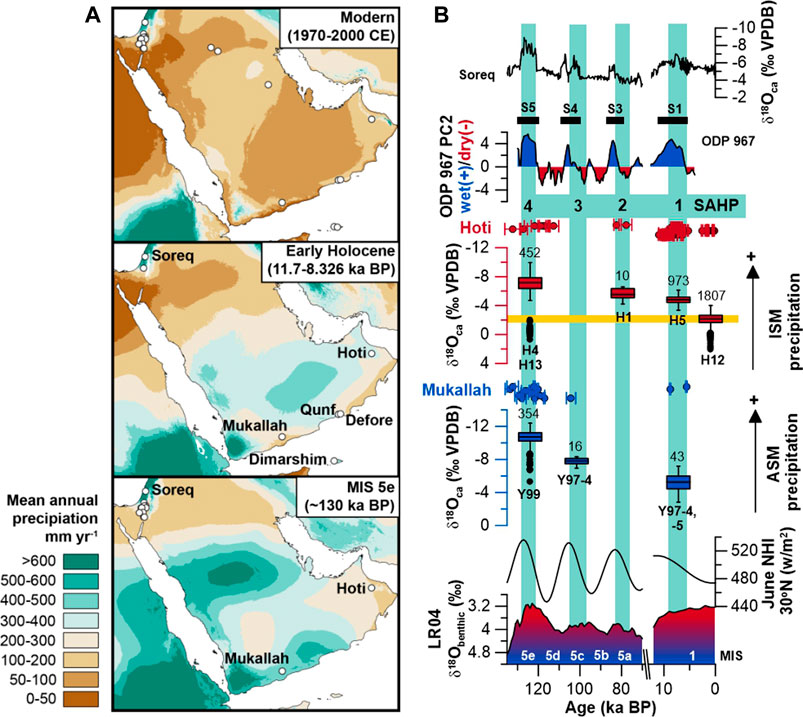
FIGURE 1. (A) simulated mean annual precipitation maps of the Arabian Peninsula for modern (Fick and Hijmans, 2017), Early Holocene (Fick and Hijmans, 2017; Fordham et al., 2017; Brown et al., 2018) and MIS 5e (Otto-Bliesner, 2006; World Clim, 2015) periods. Speleothem cave sites (white circles) show distribution of fossil stalagmites (modern) and their respective growth periods (Early Holocene and MIS 5e). (B) box-whisker plots of stalagmite δ18Oca values from Hoti (red) and Mukallah (blue) caves vs. the Soreq Cave δ18Oca curve (Bar-Matthews et al., 2003; Grant et al., 2012; Grant et al., 2016), ODP 967 PC2 wet/dry index and sapropel layers (Grant et al., 2017), low-latitude (30°N; Berger and Loutre, 1991) and global ice-volume (δ18Obenthic; Lisiecki and Raymo, 2005). Black circles denote statistically extreme values. Sample counts and stalagmite specimens are given above and below boxes, respectively. See Supplementary Material for results of ANOVA and Wilcoxon rank sum test. The yellow bar denotes the range of modern stalagmite δ18Oca values from Hoti Cave (Fleitmann et al., 2003a; Fleitmann et al., 2011). 230Th ages and age uncertainties (2σ) for Mukallah (blue) and Hoti (red) cave speleothems are given above their respective boxplots (Fleitmann et al., 2011; Nicholson et al., 2020). Relevant Marine Isotope Stages are provided using the taxonomy of Railsback et al. (2015).
Timing of Increased Rainfall During the Last 130 kyrs
At least four South Arabian Humid Periods (SAHPs) occurred during the Late Pleistocene and Early Holocene. At Mukallah Cave, stalagmite deposition was recorded during MIS 5e (∼128–121 ka BP; SAHP 4), 5c (∼104–97 ka BP; SAHP 3), and the Early Holocene (∼10–6 ka BP; SAHP 1). At Hoti cave, stalagmite deposition occurred during MIS 5e, 5a (∼85–74 ka BP; SAHP 2) and the Holocene (10–5.2 ka BP and 2.6 ka BP to present) (Fleitmann et al., 2007). Stalagmite growth is also recorded at Qunf Cave (Q5), Defore Cave (S3, S4, S6, S9) and Dimarshim (D1). An almost continuous climatic record (∼10.6–0.3 ka BP) is provided by Q5, whereas S3 and S4 are only active before and after SAHP 1 and D1 grows from ∼4.2 to 0 ka BP. Determination of stalagmite fluid inclusion water δ18O and δD values from Mukallah and Hoti caves have shown that increased precipitation during MIS 5e and the Early-Mid Holocene were delivered by the African Summer Monsoon (ASM) and the Indian Summer Monsoon (ISM) (Fleitmann et al., 2003b; Nicholson et al., 2020). This is in good coherence with other records of ASM and ISM intensity, particularly sapropel layers S5 (128.3–121.5 ka), S4 (107.8–101.8 ka), S3 (85.8–80.8 ka) and S1 (10.5–6.1 ka) from Mediterranean Sea core ODP 967 (Rohling et al., 2015; Grant et al., 2017).
Stalagmite distribution, size and shape can reveal changes in precipitation amounts in arid environments. An estimated annual precipitation >300 mm yr−1 for SAHPs was established using the distribution of active stalagmite growth in the Negev desert (Vaks et al., 2006; Vaks et al., 2010; Vaks et al., 2013), suggesting palaeo-precipitation doubled current amounts at Mukallah and Hoti Caves (Fleitmann et al., 2011; Nicholson et al., 2020; Figure 1A). MIS 5e stalagmites (Y99 and H13) are large (width >30 cm; and height >1 m), suggesting annual rainfall was considerably higher than 300 mm yr−1. This is supported by deposition of a large MIS 5e flowstone at Hoti Cave, which indicates flowing water on the cave floor, deposition fluvio-lacustrine sediments in Northern Arabia (Rosenberg et al., 2013; Parton et al., 2018) and Southern Arabia (Rosenberg et al., 2011; Rosenberg et al., 2012; Matter et al., 2015; Parton et al., 2015a), the deposition of sapropel S5 caused by ∼8 times higher Nile outflow (Amies et al., 2019) and modeled rainfall amounts of 300–600 mm yr−1 during MIS 5e (Otto-Bliesner, 2006; Jennings et al., 2015; Figure 1B). Stalagmites from later growth periods, such as Y97-4 and Y97-5, are comparatively smaller (Fleitmann et al., 2011), suggesting that annual rainfall was less than during SAHP 4. This is consistent with modeled Early Holocene rainfall of 200–300 mm yr−1 over Mukallah Cave (Fordham et al., 2017; Brown et al., 2018).
Differing rainfall amounts between SAHPs are confirmed by stalagmite δ18Oca values, which are influenced by the intensity of ASM (Mukallah) and ISM (Hoti) rainfall (Fleitmann et al., 2011; Nicholson et al., 2020). SAHP 4 (MIS 5e) has the most negative δ18Oca values (increased rainfall), whereas SAHP 1 (Holocene) has the most positive δ18Oca values (drier conditions) (Nicholson et al., 2020). The competing effects of high-latitude glacial-boundary conditions and low-latitude insolation are both considered to control the expansion, contraction and intensity of the monsoon domain (Burns et al., 2003; Cheng et al., 2009a; Beck et al., 2018) and are key differentiating factors of SAHPs (Nicholson et al., 2020). While precipitation intensities of SAHP 4, 3 and 2 follow the declining intensity of glacial-boundary minima, SAHP 1 contradicts this trend, as positive δ18Oca occurred during an interglacial maximum. Instead, SAHP δ18Oca values consistently follow the pattern of declining low-latitude summer Northern Hemisphere Insolation (NHI) maxima, which are regulated on orbital eccentricity (100 kyr) and precession (21 kyr) cycles (Figure 1B). Low-latitude insolation is a key control on the interhemispheric pressure gradient, whereby greater solar heating of the Tibetan Plateau and northern Indian Ocean results in enhanced low pressure and intensification of northern hemisphere cyclones (Burns et al., 2003; Fleitmann et al., 2007; Parton et al., 2015b; Beck et al., 2018). Thus, comparatively low insolation values during SAHP 1 are matched by a weaker response of monsoon intensity compared to preceding SAHPs. Importantly, SAHPs had differing climatic conditions and likely brought unique environmental responses and challenges for human populations.
Rainfall Trends During MIS 5e and the Holocene
MIS 5e
Precise 230Th ages of stalagmites combined with δ18Oca values have provided records of MIS 5e and Holocene climatic variability. The Y99 (Mukallah) δ18Oca and δ13Cca records cover SAHP 4 in Yemen, with onset and termination of stalagmite growth at 127.8 and 121.1 ka BP (Nicholson et al., 2020; Nicholson et al., 2021a). There are four distinct features of the Y99 δ18Oca curve: 1) onset of enhanced rainfall is characterized by negative δ18Oca values, suggesting this was abrupt, perhaps within <500 years as suggested by other ASM records (e.g., Bar-Matthews et al., 2003). 2) There is a clear relationship to the July 30oN isolation curve, demonstrating rainfall intensity was modulated by low-latitude insolation (Figures 1B, 2B). 3) While there is considerable variability, δ18Oca values are consistently more negative (wetter conditions) than succeeding wet phases. 4) There is an abrupt increase in δ18Oca and δ13Cca (drier conditions) at the termination of the wet period as the tropical rain-belt retreated southwards and annual rainfall fell below the threshold for large stalagmite formation (Nicholson et al., 2020). Additionally, sub-annually resolved H13 (Hoti) δ18Oca and δ13Cca records shows MIS 5e was characterised by increased seasonality (wetter summers and drier winters) dominated by a monsoon-driven precipitation regime (Nicholson et al., 2020). This was likely echoed by a seasonal vegetation response, as indicated by the presence of C4 plants (Bretzke et al., 2013; Nicholson et al., 2020), with potentially significant implications for animals and human hunter-gatherers.
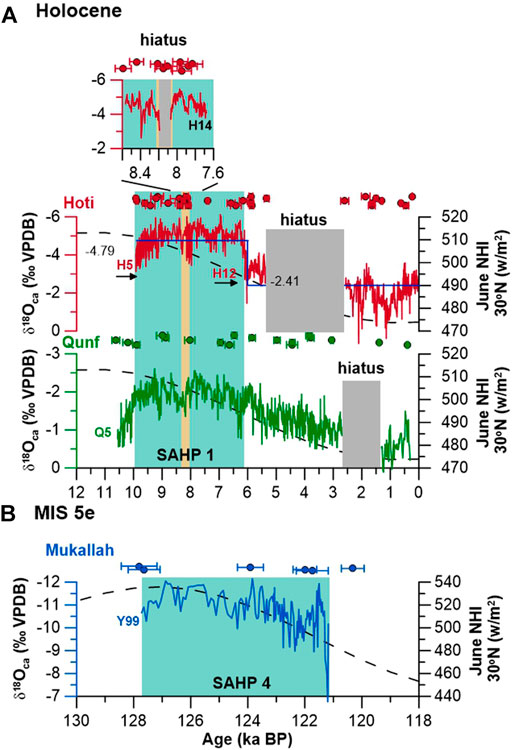
FIGURE 2. (A) High-resolution δ18Oca stalagmite values for the Holocene (H5, H12, H14 and Q5). Blue lines for H5 and H12 represent means before and after the change-point at ∼6.2 ka BP. The change-point was identified using the changepoint package for R (Killick et al., 2016). 230Th ages (cricles) and uncertainty (2σ) bars are given above their respective curves. (B) High-resolution δ18Oca stalagmite values for MIS 5e (Y99). 230Th ages and uncertainties (2σ) from Nicholson et al. (2020) (circles) used to create the StalAge (Scholz and Hoffmann, 2011) age-depth model (Nicholson et al., 2021a).
Early-Mid Holocene
The Early-Mid Holocene is characterized by another period of increased rainfall in Arabia, known as the Holocene Humid Period (HHP), or SAHP 1 in Southern Arabia (Burns et al., 1998; Burns et al., 2001; Fleitmann et al., 2003a, Fleitmann et al., 2007; Fleitmann and Matter, 2009; Lézine, 2009; Rosenberg et al., 2011; Engel et al., 2012; Rosenberg et al., 2013). Stalagmite records from Hoti (H5 and H12), Qunf (Q5) and Defore (S3 and S4) caves provide information of rainfall variability throughout the Holocene. While at Hoti Cave δ18Oca values show shifting dominances of winter (derived from the Mediterranean Sea) vs. summer (derived from the Indian Ocean) precipitation, Qunf Cave δ18Oca values record ISM precipitation intensity. Whereas Hoti Cave δ18Oca values indicate that winter precipitation has been dominant over the last ∼6 kyrs, the Early Holocene is marked by more negative δ18Oca values reflecting increased summer precipitation (Neff et al., 2001; Burns et al., 2003; Fleitmann et al., 2007; Shakun et al., 2007). This is coeval to more negative δ18Oca values at Qunf Cave which indicate an intensification of the ISM. At both caves δ18Oca values show:
1) Intensification of summer precipitation between 10.6 and 9.4 ka BP, which slightly lags low-latitude insolation due to comparatively high glacial-boundary forcing (Fleitmann et al., 2007). 2) Considerable multi-decadal variability within both H5 and Q5, displaying clear relationships with GRIP, NGRIP and DYE-3 ice-core δ18O records (Johnsen et al., 2001; Neff et al., 2001; Fleitmann et al., 2003a; Fleitmann et al., 2007; Fleitmann and Matter, 2009). More negative ice-core δ18O (colder northern-hemisphere conditions) were reflected by more positive (drier conditions) stalagmite δ18Oca values. 3) A distinct increase of δ18Oca values (drier conditions) is observed between ∼8.2–8.0 ka BP and is related to the so-called “8.2-kyr event”; a global climatic event caused by the collapse of Atlantic Overturning Meridional Circulation (AMOC) due to draining of Hudson Bay glacial lakes and freshwater influx into the Atlantic (Barber et al., 1999; Kobashi et al., 2007). δ18Oca values of H14 and H5 (Hoti Cave) show this period was characterised by a weakening of rainfall and led to a hiatus of H14 growth (Cheng et al., 2009b). 4) Summer precipitation declines at ∼6.2 ka BP. At Qunf Cave, this decline is gradual and closely follows the 30°N isolation-curve (for an extended discussion, see Fleitmann et al., 2007). At Hoti Cave, this precipitation decline is more abrupt (identifiable by change point analysis; Figure 2A) and related to winter rainfall becoming the dominant source of precipitation in northern Oman (Fleitmann et al., 2007). As Hoti Cave provides solid timing on the shifting dominance of winter vs. summer precipitation, the H5 record has been used to define the duration of SAHP 1 and is consistent with the 230Th ages of Holocene stalagmites from Mukallah Cave (Fleitmann et al., 2011; Nicholson et al., 2020). Whereas the Y99 record indicates SAHP 4 (during MIS 5e) persisted for ∼6.5 kyrs, the Hoti Cave composite record indicates SAHP 1 lasted for a shorter period of ∼4 kyrs.
These patterns follow established conditions during SAHP 1, which in Southern Arabia are also evidenced by vegetation expansion (Fuchs and Buerkert, 2008), vegetation that requires adequate precipitation (Parker et al., 2004), and palaeolake and river formation (Farraj and Harvey, 2004; Preston, 2011; Berger et al., 2012). Across Arabia, these changes are asynchronous (Preston and Parker, 2013; Preston et al., 2015), with northern Arabia experiencing a truncated period of increased rainfall compared to the south.
Discussion
MIS 5e
What do the varied conditions between SAHPs mean for discussions of human populations and climatic extremes? There is a growing body of evidence which relates Pleistocene human movements between Arabia and Africa to periods of enhanced precipitation. Archaeological remains at Jebel Faya were dated to MIS 5e and may evidence the earliest instance of H. sapiens in the region (Armitage et al., 2011). Outside of Arabia, MIS 5 H. sapiens fossils uncovered at Skhul, Qafzeh (Israel, Millard, 2008) and Fuyan Cave (≥80 ka BP, China; Liu et al., 2015) represent some of the earliest instances of Late Pleistocene humans outside of Africa. MIS 5e saw the most intense enhancement of precipitation, highlighting that this period may have been particularly favorable for hominin occupation and dispersal across the Saharo-Arabian deserts (Larrasoaña et al., 2013; Nicholson et al., 2021b). Such a large increase of precipitation was likely echoed by a greater vegetation response than later SAHPs, as evidenced by Mukallah Cave δ13Cca values (Nicholson et al., 2020) and the Jebel Faya phytolith record (Bretzke et al., 2013). It is thus likely that the carrying capacity of the Arabian Peninsula was greater during SAHP 4 compared to subsequent SAHPs, meaning population expansions and/or dispersals could have been rapid (Nicholson et al., 2021b). Additionally, the longer duration of SAHP 4 indicates that “green” environments were longer-lived than in SAHP 1, offering potentially longer-term occupation of the now arid interior. In this sense, climatic conditions during MIS 5e were at one extreme of Southern Arabia climatic variability and should not be understated as an optimal period for human dispersal.
However, it must be noted that archaeological finds are also dated to MIS 5c, 5a and 3 (Petraglia et al., 2011; Rose et al., 2011; Delagnes et al., 2012; Groucutt et al., 2018). While MIS 5e may therefore be the most extreme period of increased rainfall, other periods were still able to support human populations despite being “less favorable”. Do these climatic differences suggest that strategies of survival differed between SAHPs (e.g., Bretzke and Conard, 2017)? Were subsequent dispersals more limited in terms of numbers of people and other animals? What do statistically significant differences in δ18Oca values translate to in terms of annual rainfall differences, as well as spatio-temporal variance on long (e.g., millennial) and short (e.g., annual) timescales? Or were the additional benefits of SAHP 4 compared to other SAHPs simply not that important for human occupation (i.e., humans could make do with less)? Additionally, the presence of H. sapiens in Arabia within MIS 3 suggests either occupation throughout the MIS 4 glacial or re-entry despite a “drier” climate (Armitage et al., 2011; Delagnes et al., 2012). While these are questions for future research, one message we may take from this is resilience/adaptation despite climatic differences, and that - while the stalagmite record provides useful information on the timing of major climate changes and major H. sapiens biogeographic shifts - providing a climatic “bench-mark” for Late Pleistocene occupations from the stalagmite record is too deterministic and overlooks taphonomical biases and dating uncertainties within the archaeological record.
One thing that is perhaps clearer is that the termination of these wet periods saw a substantial change in environmental conditions. The termination of SAHP 4 likely meant annual rainfall declined to <300 mm yr−1 and was echoed by a decline in vegetation resources. In terms of the “lived” experiences of humans, such a decline would have likely required a shift in survival strategies (Nicholson et al., 2021b). This may have included increased home-range foraging size and mobility patterns, retraction to high-resource retaining areas (such as the Yemeni Highlands; Delagnes et al., 2012; Delagnes et al., 2013) or in some cases dispersal out of Arabia (Nicholson et al., 2021b). Such responses to declining precipitation were also likely variable and not simplistic. Recent archaeological finds in Northern Arabia hint at techno-cultural continuity between Mid-Pleistocene wetter phases (Scerri et al., 2021), perhaps suggesting human resilience to increasingly unfavorable climatic conditions.
Early-Mid Holocene
The key precipitation changes during the HHP/SAHP 1 of gradual intensification of summer precipitation (∼10.6–9.4 ka BP) that only declines following ∼6.2 ka BP, and temporarily during the 9.2-kyr and 8.2-kyr events (Fleitmann et al., 2008), influenced humans and communities living in Arabia (for full summaries, see Parker et al., 2006; Goudie and Parker, 2010; Groucutt et al., 2020; Petraglia et al., 2020).
When compared to MIS 5e however, precipitation increases and associated vegetation response were less intense (Fleitmann et al., 2011; Bretzke et al., 2013; Nicholson et al., 2020). Despite this, and similar to MIS 5c, 5a and 3 (see above), there remains archaeological evidence for human occupation. Mustatils appear in northern Arabia from 9.2 ka BP (Kennedy, 2017; Guagnin et al., 2020; Thomas et al., 2021), and desert kites are evidenced in Jordan from 10 ka BP (Al Khasawneh et al., 2019). In southern Arabia, occupation of Jebel Qara took place ∼10.5–9.5 ka BP (Cremaschi et al., 2015), pastoralism is evidenced by 8.0 ka BP (Drechsler, 2007; Drechsler, 2009; Martin et al., 2009), graves are attested 7.2–6.0 ka BP (Kiesewetter, 2006) and monumental stone platforms are evidenced 6.4 ka BP (McCorriston et al., 2012; Magee, 2014).
Reduced rainfall following ∼6.2 ka BP led to a temporary end of Neolithic herding in the desert interiors, shrinking population numbers, and migration to areas with greater ecological diversity, perhaps suggesting a minimum amount of precipitation is required for human occupation in these marginal environments (Uerpmann, 1992; Vogt, 1994; Uerpmann, 2002; Potts et al., 2003; Goudie and Parker, 2010). However, human communities returned to the interior of Southern Arabia from ∼5.2 ka BP without amelioration of climate, which even aridified further; varied occupation continues until the modern day (Magee, 2014; Petraglia et al., 2020). Therefore, it seems likely that drier climates create challenges for human populations, but these can be overcome by technological (e.g., mustatils, pottery, water management; camels domestication) and strategic (e.g., mobility, pastoralism) adaptations (Petraglia et al., 2020).
Finally, stability and variance of precipitation (which can be hard to detect in palaeoclimate records) may have been more influential to humans than long-term changes in amounts (Thornton et al., 2014). A temporary transition to herding practices occurred in some parts of Arabia during the 8.2 ka event (Drechsler, 2009; Crassard and Drechsler, 2013), whilst more positive δ18Oca values (drier conditions) are observed at Hoti cave (Figure 2A). Conversely, Cremaschi et al. (2015) suggested that—although increasing precipitation ∼10.5–9.5 ka BP facilitated occupation—overly “wet” landscapes at Jebel Qara after 9.5 ka BP led to site abandonment and a preference for coastal settings, hinting at the varied human responses to fluctuating climatic conditions.
Conclusion
Overall, when compared to other periods, stalagmite climate records indicate that the African and Indian Summer Monsoons were most intense during MIS 5e, which was one extreme of climatic variability in the last 130 kyrs. This was likely an important period for the dispersal of H. sapiens from Africa, as well as occupation in the now desert interiors of Arabia, and the subsequent decline back to more arid conditions likely impacted survival strategies in Southern Arabia. A comparably weaker intensification of precipitation (yet long-term trends are comparable) occurred during the Early Holocene. The expansion of human populations into the now arid interior was similar to MIS 5e but the responses to climatic variability and subsequent aridification differed. We emphasize that evidence for human occupation during all periods of insolation maxima, and the varying climates of these drier periods, highlights human resilience/adaptation despite climatic differences and mitigates against a simplistic narrative. Future research will benefit from the addition of climate/environmental proxies (trace-element and perhaps aDNA), increased surveys to advance the spatial-temporal coverage of the speleothem record and development of continuous climate records for SAHP 3 and 2. Understanding the shifting survival strategies in the context of declining rainfall and aridification, as Arabia transitioned from one extreme to another, will be of key importance to future debates of H. sapiens biogeography, behavioral flexibility, and both past and future climate-induced socio-political change.
Author Contributions
SLN acted as primary author for the article, conceptualizing the manuscript, producing the initial draft and figures and ongoing editing of the manuscript. MJ acted as secondary author, assisting with the initial draft, and editing of the manuscript. RH and DF supervised and edited the manuscript.
Funding
This work was supported by the AHRC South, West and Wales Doctoral Training Partnership (Grant AH/L503939/1) the Swiss National Science Foundation (Grant PP002-110554/1 to DF).
Conflict of Interest
The authors declare that the research was conducted in the absence of any commercial or financial relationships that could be construed as a potential conflict of interest.
Publisher’s Note
All claims expressed in this article are solely those of the authors and do not necessarily represent those of their affiliated organizations, or those of the publisher, the editors and the reviewers. Any product that may be evaluated in this article, or claim that may be made by its manufacturer, is not guaranteed or endorsed by the publisher.
Acknowledgments
We thank two reviewers for their constructive feedback on our manuscript.
Supplementary Material
The Supplementary Material for this article can be found online at: https://www.frontiersin.org/articles/10.3389/feart.2021.749488/full#supplementary-material
References
Al Khasawneh, S., Murray, A., Thomsen, K., AbuAzizeh, W., and Tarawneh, M. (2019). Dating a Near Eastern Desert Hunting Trap (Kite) Using Rock Surface Luminescence Dating. Archaeol Anthropol. Sci. 11, 2109–2119. doi:10.1007/s12520-018-0661-3
Amies, J. D., Rohling, E. J., Grant, K. M., Rodríguez‐Sanz, L., and Marino, G. (2019). Quantification of African Monsoon Runoff during Last Interglacial Sapropel S5. Paleoceanography and Paleoclimatology 34, 1487–1516. doi:10.1029/2019pa003652
Armitage, S. J., Jasim, S. A., Marks, A. E., Parker, A. G., Usik, V. I., and Uerpmann, H.-P. (2011). The Southern Route “Out of Africa”: Evidence for an Early Expansion of Modern Humans into Arabia. Science 331, 453–456. doi:10.1126/science.1199113
Bar-Matthews, M., Ayalon, A., Gilmour, M., Matthews, A., and Hawkesworth, C. J. (2003). Sea-land Oxygen Isotopic Relationships from Planktonic Foraminifera and Speleothems in the Eastern Mediterranean Region and Their Implication for Paleorainfall during Interglacial Intervals. Geochimica et Cosmochimica Acta 67, 3181–3199. doi:10.1016/s0016-7037(02)01031-1
Barber, D. C., Dyke, A., Hillaire-Marcel, C., Jennings, A. E., Andrews, J. T., Kerwin, M. W., et al. (1999). Forcing of the Cold Event of 8,200 Years Ago by Catastrophic Drainage of Laurentide Lakes. Nature 400, 344–348. doi:10.1038/22504
Beck, J. W., Zhou, W., Li, C., Wu, Z., White, L., Xian, F., et al. (2018). A 550,000-year Record of East Asian Monsoon Rainfall from 10 Be in Loess. Science 360, 877–881. doi:10.1126/science.aam5825
Berger, A., and Loutre, M. F. (1991). Insolation Values for the Climate of the Last 10 Million Years. Quat. Sci. Rev. 10, 297–317. doi:10.1016/0277-3791(91)90033-q
Berger, J.-F., Bravard, J.-P., Purdue, L., Benoist, A., Mouton, M., and Braemer, F. (2012). Rivers of the Hadramawt Watershed (Yemen) during the Holocene: Clues of Late Functioning. Quat. Int. 266, 142–161. doi:10.1016/j.quaint.2011.10.037
Bretzke, K., Armitage, S. J., Parker, A. G., Walkington, H., and Uerpmann, H.-P. (2013). The Environmental Context of Paleolithic Settlement at Jebel Faya, Emirate Sharjah, UAE. Quat. Int. 300, 83–93. doi:10.1016/j.quaint.2013.01.028
Bretzke, K., and Conard, N. J. (2017). Not Just a Crossroad. Curr. Anthropol. 58, S449–S462. doi:10.1086/694077
Brown, J. L., Hill, D. J., Dolan, A. M., Carnaval, A. C., and Haywood, A. M. (2018). Paleoclim, High Spatial Resolution Paleoclimate Surfaces for Global Land Areas. Scientific Data 5, 180254. doi:10.1038/sdata.2018.254
Burns, S. J., Fleitmann, D., Matter, A., Kramers, J., and Al-Subbary, A. A. (2003). Indian Ocean Climate and an Absolute Chronology over Dansgaard/Oeschger Events 9 to 13. Science 301, 1365–1367. LP–1367. doi:10.1126/science.1086227
Burns, S. J., Fleitmann, D., Matter, A., Neff, U., and Mangini, A. (2001). Speleothem Evidence from Oman for continental Pluvial Events during Interglacial Periods. Geol 29, 623–626. doi:10.1130/0091-7613(2001)029<0623:sefofc>2.0.co;2
Burns, S. J., Matter, A., Frank, N., and Mangini, A. (1998). Speleothem-based Paleoclimate Record from Northern Oman. Geol 26, 499–502. doi:10.1130/0091-7613(1998)026<0499:sbprfn>2.3.co;2
Burstyn, Y., Martrat, B., Lopez, J. F., Iriarte, E., Jacobson, M. J., Lone, M. A., et al. (2019). Speleothems from the Middle East: An Example of Water Limited Environments in the SISAL Database. Quaternary 2, 16. doi:10.3390/quat2020016
Cheng, H., Edwards, R. L., Broecker, W. S., Denton, G. H., Kong, X., Wang, Y., et al. (2009a). Ice Age Terminations. Science 326, 248–252. doi:10.1126/science.1177840
Cheng, H., Fleitmann, D., Edwards, R. L., Wang, X., Cruz, F. W., Auler, A. S., et al. (2009b). Timing and Structure of the 8.2 Kyr B.P. Event Inferred from δ18O Records of Stalagmites from China, Oman, and Brazil. Geology 37, 1007–1010. doi:10.1130/g30126a.1
Crassard, R., and Drechsler, P. (2013). Towards New Paradigms: Multiple Pathways for the Arabian Neolithic. Arabian Archaeol Epigr 24, 3–8. doi:10.1111/aae.12021
Cremaschi, M., Zerboni, A., Charpentier, V., Crassard, R., Isola, I., Regattieri, E., et al. (2015). Early-Middle Holocene Environmental Changes and Pre-neolithic Human Occupations as Recorded in the Cavities of Jebel Qara (Dhofar, Southern Sultanate of Oman). Quat. Int. 382, 264–276. doi:10.1016/j.quaint.2014.12.058
Delagnes, A., Crassard, R., Bertran, P., and Sitzia, L. (2013). Cultural and Human Dynamics in Southern Arabia at the End of the Middle Paleolithic. Quat. Int. 300, 234–243. doi:10.1016/j.quaint.2012.12.012
Delagnes, A., Tribolo, C., Bertran, P., Brenet, M., Crassard, R., Jaubert, J., et al. (2012). Inland Human Settlement in Southern Arabia 55,000 Years Ago. New Evidence from the Wadi Surdud Middle Paleolithic Site Complex, Western Yemen. J. Hum. Evol. 63, 452–474. doi:10.1016/j.jhevol.2012.03.008
Drechsler, P. (2009). The Dispersal of the Neolithic over the Arabian Peninsula. Oxford: BAR International Series 1969Archaeopress.
El-Shenawy, M. I., Kim, S.-T., Schwarcz, H. P., Asmerom, Y., and Polyak, V. J. (2018). Speleothem Evidence for the Greening of the Sahara and its Implications for the Early Human Dispersal Out of Sub-saharan Africa. Quat. Sci. Rev. 188, 67–76. doi:10.1016/j.quascirev.2018.03.016
Engel, M., Brückner, H., Pint, A., Wellbrock, K., Ginau, A., Voss, P., et al. (2012). The Early Holocene Humid Period in NW Saudi Arabia - Sediments, Microfossils and Palaeo-Hydrological Modelling. Quat. Int. 266, 131–141. doi:10.1016/j.quaint.2011.04.028
Farraj, A. A., and Harvey, A. M. (2004). Late Quaternary Interactions between Aeolian and Fluvial Processes: a Case Study in the Northern UAE. J. Arid Environments 56, 235–248. doi:10.1016/s0140-1963(03)00054-5
Fick, S. E., and Hijmans, R. J. (2017). WorldClim 2: New 1‐km Spatial Resolution Climate Surfaces for Global Land Areas. Int. J. Climatol 37, 4302–4315. doi:10.1002/joc.5086
Fleitmann, D., Burns, S. J., Mangini, A., Mudelsee, M., Kramers, J., Villa, I., et al. (2007). Holocene ITCZ and Indian Monsoon Dynamics Recorded in Stalagmites from Oman and Yemen (Socotra). Quat. Sci. Rev. 26, 170–188. doi:10.1016/j.quascirev.2006.04.012
Fleitmann, D., Burns, S. J., Mudelsee, M., Neff, U., Kramers, J., Mangini, A., et al. (2003a). Holocene Forcing of the Indian Monsoon Recorded in a Stalagmite from Southern Oman. Science 300, 1737–1739. doi:10.1126/science.1083130
Fleitmann, D., Burns, S. J., Neff, U., Mangini, A., and Matter, A. (2003b). Changing Moisture Sources over the Last 330,000 Years in Northern Oman from Fluid-Inclusion Evidence in Speleothems. Quat. Res. 60, 223–232. doi:10.1016/s0033-5894(03)00086-3
Fleitmann, D., Burns, S. J., Pekala, M., Mangini, A., Al-Subbary, A., Al-Aowah, M., et al. (2011). Holocene and Pleistocene Pluvial Periods in Yemen, Southern Arabia. Quat. Sci. Rev. 30, 783–787. doi:10.1016/j.quascirev.2011.01.004
Fleitmann, D., and Matter, A. (2009). The Speleothem Record of Climate Variability in Southern Arabia. Comptes Rendus Geosci. 341, 633–642. doi:10.1016/j.crte.2009.01.006
Fleitmann, D., Mudelsee, M., Burns, S. J., Bradley, R. S., Kramers, J., and Matter, A. (2008). Evidence for a Widespread Climatic Anomaly at Around 9.2 Ka before Present. Paleoceanography 23. doi:10.1029/2007pa001519
Fordham, D. A., Saltré, F., Haythorne, S., Wigley, T. M. L., Otto-Bliesner, B. L., Chan, K. C., et al. (2017). PaleoView: a Tool for Generating Continuous Climate Projections Spanning the Last 21 000 Years at Regional and Global Scales. Ecography 40, 1348–1358. doi:10.1111/ecog.03031
Fuchs, M., and Buerkert, A. (2008). A 20 Ka Sediment Record from the Hajar Mountain Range in N-Oman, and its Implication for Detecting Arid-Humid Periods on the southeastern Arabian Peninsula. Earth Planet. Sci. Lett. 265, 546–558. doi:10.1016/j.epsl.2007.10.050
Goudie, A. S., and Parker, A. G. (2010). “Paleoenvironments and Prehistory in the Holocene of SE Arabia,” in Landscapes and Societies: Selected Cases. Editors I.P. Martini, and W. Chesworth (Netherlands, Dordrecht: Springer), 109–120. doi:10.1007/978-90-481-9413-1_7
Grant, K. M., Grimm, R., Mikolajewicz, U., Marino, G., Ziegler, M., and Rohling, E. J. (2016). The Timing of Mediterranean Sapropel Deposition Relative to Insolation, Sea-Level and African Monsoon Changes. Quat. Sci. Rev. 140, 125–141. doi:10.1016/j.quascirev.2016.03.026
Grant, K. M., Rohling, E. J., Bar-Matthews, M., Ayalon, A., Medina-Elizalde, M., Ramsey, C. B., et al. (2012). Rapid Coupling between Ice Volume and Polar Temperature over the Past 150,000 Years. Nature 491, 744–747. doi:10.1038/nature11593
Grant, K. M., Rohling, E. J., Westerhold, T., Zabel, M., Heslop, D., Konijnendijk, T., et al. (2017). A 3 Million Year index for North African Humidity/aridity and the Implication of Potential Pan-African Humid Periods. Quat. Sci. Rev. 171, 100–118. doi:10.1016/j.quascirev.2017.07.005
Groucutt, H. S., Breeze, P. S., Guagnin, M., Stewart, M., Drake, N., Shipton, C., et al. (2020). Monumental Landscapes of the Holocene Humid Period in Northern Arabia: The Mustatil Phenomenon. The Holocene 30, 1767–1779. doi:10.1177/0959683620950449
Groucutt, H. S., Grün, R., Zalmout, I. A. S., Drake, N. A., Armitage, S. J., Candy, I., et al. (2018). Homo sapiens in Arabia by 85,000 Years Ago. Nat. Ecol. Evol. 2, 800–809. doi:10.1038/s41559-018-0518-2
Groucutt, H. S., White, T. S., Clark-Balzan, L., Parton, A., Crassard, R., Shipton, C., et al. (2015). Human Occupation of the Arabian Empty Quarter during MIS 5: Evidence from Mundafan Al-Buhayrah, Saudi Arabia. Quat. Sci. Rev. 119, 116–135. doi:10.1016/j.quascirev.2015.04.020
Groucutt, H. S., White, T. S., Scerri, E. M. L., Andrieux, E., Clark-Wilson, R., Breeze, P. S., et al. (2021). Multiple Hominin Dispersals into Southwest Asia over the Past 400,000 Years. Nature 597, 376–380. doi:10.1038/s41586-021-03863-y
Guagnin, M., Breeze, P., Shipton, C., Ott, F., Stewart, M., Bateman, M., et al. (2020). The Holocene Humid Period in the Nefud Desert: Hunters and Herders in the Jebel Oraf Palaeolake basin, Saudi Arabia. J. Arid Environments 178, 104146. doi:10.1016/j.jaridenv.2020.104146
Henselowsky, F., Eichstädter, R., Schröder-Ritzrau, A., Herwartz, D., Almoazamy, A., Frank, N., et al. (2021). Speleothem Growth Phases in the central Eastern Desert of Egypt Reveal Enhanced Humidity throughout MIS 5. Quat. Int.. doi:10.1016/j.quaint.2021.05.006
Jennings, R. P., Singarayer, J., Stone, E. J., Krebs-Kanzow, U., Khon, V., Nisancioglu, K. H., et al. (2015). The Greening of Arabia: Multiple Opportunities for Human Occupation of the Arabian Peninsula during the Late Pleistocene Inferred from an Ensemble of Climate Model Simulations. Quat. Int. 382, 181–199. doi:10.1016/j.quaint.2015.01.006
Johnsen, S. J., Dahl-Jensen, D., Gundestrup, N., Steffensen, J. P., Clausen, H. B., Miller, H., et al. (2001). Oxygen Isotope and Palaeotemperature Records from Six Greenland Ice-Core Stations: Camp Century, Dye-3, GRIP, GISP2, Renland and NorthGRIP. J. Quat. Sci. 16, 299–307. doi:10.1002/jqs.622
Kennedy, D. (2017). 'Gates': a New Archaeological Site Type in Saudi Arabia. Arab Arch. Epig 28, 153–174. doi:10.1111/aae.12100
Kiesewetter, H. (2006). “Analyses of the Human Remains from the Neolithic Cemetary at Al-Buhais 18,” in Funeral Monuments and Human Remains from Jebel Al-Buhais. Editors H.-P. Uerpmann, M. Uerpmann, and S. A. Jasim (Tübingen: Department of Culture and Information), 103–380.
Killick, R., Haynes, K., Eckley, I., Fearnhead, P., and Lee, J. (2016). Methods for Changepoint Detection. Available at: https://cran.r-project.org/web/packages/changepoint/changepoint.pdf.
Kobashi, T., Severinghaus, J. P., Brook, E. J., Barnola, J.-M., and Grachev, A. M. (2007). Precise Timing and Characterization of Abrupt Climate Change 8200 Years Ago from Air Trapped in Polar Ice. Quat. Sci. Rev. 26, 1212–1222. doi:10.1016/j.quascirev.2007.01.009
Larrasoaña, J. C., Roberts, A. P., and Rohling, E. J. (2013). Dynamics of Green Sahara Periods and Their Role in Hominin Evolution. PLoS ONE 8, e76514. doi:10.1371/journal.pone.0076514
Lézine, A.-M. (2009). Timing of Vegetation Changes at the End of the Holocene Humid Period in Desert Areas at the Northern Edge of the Atlantic and Indian Monsoon Systems. Comptes Rendus Geosci. 341, 750–759. doi:10.1016/j.crte.2009.01.001
Lisiecki, L. E., and Raymo, M. E. (2005). A Pliocene-Pleistocene Stack of 57 Globally Distributed Benthic δ 18O Records. Paleoceanography 20, 1–17. doi:10.1029/2004pa001071
Liu, W., Martinón-Torres, M., Cai, Y.-j., Xing, S., Tong, H.-w., Pei, S.-w., et al. (2015). The Earliest Unequivocally Modern Humans in Southern China. Nature 526, 696–699. doi:10.1038/nature15696
Magee, P. (2014). The Archaeology of Prehistoric Arabia. Adaptation and Social Formation from the Neolithic to the Iron Age. New York: Cambridge University Press.
Martin, L., McCorriston, J., and Crassard, R. (2009). Early Arabian Pastoralism at Manayzah in Wadi Sana, Hadramawt. Proc. Semin. Arabian Stud. 39, 285–296.
Matter, A., Neubert, E., Preusser, F., Rosenberg, T., and Al-Wagdani, K. (2015). Palaeo-environmental Implications Derived from lake and Sabkha Deposits of the Southern Rub' Al-Khali, Saudi Arabia and Oman. Quat. Int. 382, 120–131. doi:10.1016/j.quaint.2014.12.029
McCorriston, J., Harrower, M., Martin, L., and Oches, E. (2012). Cattle Cults of the Arabian Neolithic and Early Territorial Societies. Am. Anthropologist 114, 45–63. doi:10.1111/j.1548-1433.2011.01396.x
Millard, A. R. (2008). A Critique of the Chronometric Evidence for Hominid Fossils: I. Africa and the Near East 500-50ka. J. Hum. Evol. 54, 848–874. doi:10.1016/j.jhevol.2007.11.002
Neff, U., Burns, S. J., Mangini, A., Mudelsee, M., Fleitmann, D., and Matter, A. (2001). Strong Coherence between Solar Variability and the Monsoon in Oman between 9 and 6 Kyr Ago. Nature 411, 290–293. doi:10.1038/35077048
Nicholson, S. L., Hosfield, R., Groucutt, H. S., Burns, S. J., Matter, A., and Fleitmann, D. (2021a). A Climatic Evaluation of the Southern Dispersal Route. Earth Space Sci. Open Archive. Pre-print at Essoar. doi:10.1002/essoar.10508203.2
Nicholson, S. L., Hosfield, R., Groucutt, H. S., Pike, A. W. G., and Fleitmann, D. (2021b). Beyond Arrows on a Map: The Dynamics of Homo sapiens Dispersal and Occupation of Arabia during Marine Isotope Stage 5. J. Anthropological Archaeology 62, 101269. doi:10.1016/j.jaa.2021.101269
Nicholson, S. L., Pike, A. W. G., Hosfield, R., Roberts, N., Sahy, D., Woodhead, J., et al. (2020). Pluvial Periods in Southern Arabia over the Last 1.1 Million-Years. Quat. Sci. Rev. 229, 106112. doi:10.1016/j.quascirev.2019.106112
Otto-Bliesner, B. L., Marshall, S. J., Overpeck, J. T., Miller, G. H., and Hu, A. (2006). Simulating Arctic Climate Warmth and Icefield Retreat in the Last Interglaciation. Science 311, 1751–1753. doi:10.1126/science.1120808
Parker, A. G., Eckersley, L., Smith, M. M., Goudie, A. S., Stokes, S., Ward, S., et al. (2004). Holocene Vegetation Dynamics in the Northeastern Rub' Al-Khali Desert, Arabian Peninsula: a Phytolith, Pollen and Carbon Isotope Study. J. Quat. Sci. 19, 665–676. doi:10.1002/jqs.880
Parker, A. G., Goudie, A. S., Stokes, S., White, K., Hodson, M. J., Manning, M., et al. (2006). A Record of Holocene Climate Change from Lake Geochemical Analyses in Southeastern Arabia. Quat. Res. 66, 465–476. doi:10.1016/j.yqres.2006.07.001
Parton, A., Clark-Balzan, L., Parker, A. G., Preston, G. W., Sung, W. W., Breeze, P. S., et al. (2018). Middle-late Quaternary Palaeoclimate Variability from lake and Wetland Deposits in the Nefud Desert, Northern Arabia. Quat. Sci. Rev. 202, 78–97. doi:10.1016/j.quascirev.2018.10.010
Parton, A., Farrant, A. R., Leng, M. J., Schwenninger, J.-L., Rose, J. I., Uerpmann, H.-P., et al. (2013). An Early MIS 3 Pluvial Phase in Southeast Arabia: Climatic and Archaeological Implications. Quat. Int. 300, 62–74. doi:10.1016/j.quaint.2013.02.016
Parton, A., Farrant, A. R., Leng, M. J., Telfer, M. W., Groucutt, H. S., Petraglia, M. D., et al. (2015a). Alluvial Fan Records from Southeast Arabia Reveal Multiple Windows for Human Dispersal. Geology 43, 295–298. doi:10.1130/g36401.1
Parton, A., White, T. S., Parker, A. G., Breeze, P. S., Jennings, R., Groucutt, H. S., et al. (2015b). Orbital-scale Climate Variability in Arabia as a Potential Motor for Human Dispersals. Quat. Int. 382, 82–97. doi:10.1016/j.quaint.2015.01.005
Petraglia, M. D., Alsharekh, A., Breeze, P., Clarkson, C., Crassard, R., Drake, N. A., et al. (2012). Hominin Dispersal into the Nefud Desert and Middle Palaeolithic Settlement along the Jubbah Palaeolake, Northern Arabia. PLoS ONE 7, e49840. doi:10.1371/journal.pone.0049840
Petraglia, M. D., Alsharekh, A. M., Crassard, R., Drake, N. A., Groucutt, H., Parker, A. G., et al. (2011). Middle Paleolithic Occupation on a Marine Isotope Stage 5 Lakeshore in the Nefud Desert, Saudi Arabia. Quat. Sci. Rev. 30, 1555–1559. doi:10.1016/j.quascirev.2011.04.006
Petraglia, M. D., Groucutt, H. S., Guagnin, M., Breeze, P. S., and Boivin, N. (2020). Human Responses to Climate and Ecosystem Change in Ancient Arabia. Proc. Natl. Acad. Sci. USA 117, 8263–8270. doi:10.1073/pnas.1920211117
Potts, D., Al Naboodah, H., and Hellyer, P. (2003). Archaeology of the United Arab Emirates: Proceedings of the First International Conference. London: Trident Press.
Preston, G. W. (2011). From Nomadic Herder-hunters to Sedentary Famers: The Relationship between Climate, Environment and Human Societies in the United Arab Emirates from the Neolithic to the Iron Age. Oxford, UK: Oxford Brookes University.
Preston, G. W., and Parker, A. G. (2013). Understanding the Evolution of the Holocene Pluvial Phase and its Impact on Neolithic Populations in South-East Arabia. Arabian Archaeol Epigr 24, 87–94. doi:10.1111/aae.12006
Preston, G. W., Thomas, D. S. G., Goudie, A. S., Atkinson, O. A. C., Leng, M. J., Hodson, M. J., et al. (2015). A Multi-Proxy Analysis of the Holocene Humid Phase from the United Arab Emirates and its Implications for Southeast Arabia's Neolithic Populations. Quat. Int. 382, 277–292. doi:10.1016/j.quaint.2015.01.054
Railsback, L. B., Gibbard, P. L., Head, M. J., Voarintsoa, N. R. G., and Toucanne, S. (2015). An Optimized Scheme of Lettered marine Isotope Substages for the Last 1.0 Million Years, and the Climatostratigraphic Nature of Isotope Stages and Substages. Quat. Sci. Rev. 111, 94–106. doi:10.1016/j.quascirev.2015.01.012
Rohling, E. J., Marino, G., and Grant, K. M. (2015). Mediterranean Climate and Oceanography, and the Periodic Development of Anoxic Events (Sapropels). Earth-Science Rev. 143, 62–97. doi:10.1016/j.earscirev.2015.01.008
Rose, J. I., Usik, V. I., Marks, A. E., Hilbert, Y. H., Galletti, C. S., Parton, A., et al. (2011). The Nubian Complex of Dhofar, Oman: An African Middle Stone Age Industry in Southern Arabia. PLoS ONE 6, e28239. doi:10.1371/journal.pone.0028239
Rosenberg, T. M., Preusser, F., Blechschmidt, I., Fleitmann, D., Jagher, R., and Matter, A. (2012). Late Pleistocene Palaeolake in the interior of Oman: A Potential Key Area for the Dispersal of Anatomically Modern Humans Out-Of-Africa?. J. Quat. Sci. 27, 13–16. doi:10.1002/jqs.1560
Rosenberg, T. M., Preusser, F., Fleitmann, D., Schwalb, A., Penkman, K., Schmid, T. W., et al. (2011). Humid Periods in Southern Arabia: Windows of Opportunity for Modern Human Dispersal. Geology 39, 1115–1118. doi:10.1130/g32281.1
Rosenberg, T. M., Preusser, F., Risberg, J., Plikk, A., Kadi, K. A., Matter, A., et al. (2013). Middle and Late Pleistocene Humid Periods Recorded in Palaeolake Deposits of the Nafud Desert, Saudi Arabia. Quat. Sci. Rev. 70, 109–123. doi:10.1016/j.quascirev.2013.03.017
Scerri, E. M. L., Frouin, M., Breeze, P. S., Armitage, S. J., Candy, I., Groucutt, H. S., et al. (2021). The Expansion of Acheulean Hominins into the Nefud Desert of Arabia. Sci. Rep. 11, 10111. doi:10.1038/s41598-021-89489-6
Scholz, D., and Hoffmann, D. L. (2011). StalAge - an Algorithm Designed for Construction of Speleothem Age Models. Quat. Geochronol. 6, 369–382. doi:10.1016/j.quageo.2011.02.002
Shakun, J. D., Burns, S. J., Fleitmann, D., Kramers, J., Matter, A., and Al-Subary, A. (2007). A High-Resolution, Absolute-Dated Deglacial Speleothem Record of Indian Ocean Climate from Socotra Island, Yemen. Earth Planet. Sci. Lett. 259, 442–456. doi:10.1016/j.epsl.2007.05.004
Stewart, M., Clark-Wilson, R., Breeze, P. S., Janulis, K., Candy, I., Armitage, S. J., et al. (2020a). Human Footprints Provide Snapshot of Last Interglacial Ecology in the Arabian interior. Sci. Adv. 6, eaba8940. doi:10.1126/sciadv.aba8940
Stewart, M., Louys, J., Breeze, P. S., Clark-Wilson, R., Drake, N. A., Scerri, E. M. L., et al. (2020b). A Taxonomic and Taphonomic Study of Pleistocene Fossil Deposits from the Western Nefud Desert, Saudi Arabia. Quat. Res. 95, 1–22. doi:10.1017/qua.2020.6
Stimpson, C. M., Lister, A., Parton, A., Clark-Balzan, L., Breeze, P. S., Drake, N. A., et al. (2016). Middle Pleistocene Vertebrate Fossils from the Nefud Desert, Saudi Arabia: Implications for Biogeography and Palaeoecology. Quat. Sci. Rev. 143, 13–36. doi:10.1016/j.quascirev.2016.05.016
Thomas, H., Kennedy, M. A., Dalton, M., McMahon, J., Boyer, D., and Repper, R. (2021). The Mustatils: Cult and Monumentality in Neolithic north-western Arabia. Antiquity 95, 605–626. doi:10.15184/aqy.2021.51
Thornton, P. K., Ericksen, P. J., Herrero, M., and Challinor, A. J. (2014). Climate Variability and Vulnerability to Climate Change: a Review. Glob. Change Biol. 20, 3313–3328. doi:10.1111/gcb.12581
Uerpmann, M. (1992). Structuring the Late Stone Age of Southeastern Arabia. Arab Arch. Epigraphy 3, 65–109. doi:10.1111/j.1600-0471.1992.tb00032.x
Uerpmann, M. (2002). “The Dark Millennium - Remarks on the Final Stone Age in the Emirates and Oman,” in Archaeology of the United Arab Emirates: Proceedings of the First International Conference on the Archaeology of the U.A.E. Editors D. Potts, H. Al Naboodah, and P. Hellyer (London: Trident Press).
Vaks, A., Bar-Matthews, M., Ayalon, A., Matthews, A., Frumkin, A., Dayan, U., et al. (2006). Paleoclimate and Location of the Border between Mediterranean Climate Region and the Saharo-Arabian Desert as Revealed by Speleothems from the Northern Negev Desert, Israel. Earth Planet. Sci. Lett. 249, 384–399. doi:10.1016/j.epsl.2006.07.009
Vaks, A., Bar-Matthews, M., Matthews, A., Ayalon, A., and Frumkin, A. (2010). Middle-Late Quaternary Paleoclimate of Northern Margins of the Saharan-Arabian Desert: Reconstruction from Speleothems of Negev Desert, Israel. Quat. Sci. Rev. 29, 2647–2662. doi:10.1016/j.quascirev.2010.06.014
Vaks, A., Woodhead, J., Bar-Matthews, M., Ayalon, A., Cliff, R. A., Zilberman, T., et al. (2013). Pliocene-Pleistocene Climate of the Northern Margin of Saharan-Arabian Desert Recorded in Speleothems from the Negev Desert, Israel. Earth Planet. Sci. Lett. 368, 88–100. doi:10.1016/j.epsl.2013.02.027
Vogt, B. (1994). “In Search for Coastal Sites in Pre-historic Makkan: Mid-holocene ‘shell-Eaters’ in the Coastal Desert of Ras Al-Khaimah, U.A.E,” in From Sumer to Meluhha: Contributions to the Archaeology of South and West Asia in Memory of George F. Dales, Jr. Wisconsin Arhcaeological Reports. Editor J.M. Kenoyer (Madison: University of Wisconsin), Vol. 3.
World Clim (2015). World Clim-Global Climate Data: Free Climate Data for Ecological Modeling and GISVery High Resolution Interpolated Climate Surfaces for Global Land Areas. Available at: https://www.worldclim.com/.
Keywords: Arabia, monsoon, dispersal, Homo sapiens, stalagmite, isotope, climate
Citation: Nicholson SL, Jacobson MJ, Hosfield R and Fleitmann D (2021) The Stalagmite Record of Southern Arabia: Climatic Extremes, Human Evolution and Societal Development. Front. Earth Sci. 9:749488. doi: 10.3389/feart.2021.749488
Received: 29 July 2021; Accepted: 11 November 2021;
Published: 30 November 2021.
Edited by:
Amy Prendergast, The University of Melbourne, AustraliaReviewed by:
Emma Loftus, Ludwig Maximilian University of Munich, GermanyAndrea Zerboni, University of Milan, Italy
Copyright © 2021 Nicholson, Jacobson, Hosfield and Fleitmann. This is an open-access article distributed under the terms of the Creative Commons Attribution License (CC BY). The use, distribution or reproduction in other forums is permitted, provided the original author(s) and the copyright owner(s) are credited and that the original publication in this journal is cited, in accordance with accepted academic practice. No use, distribution or reproduction is permitted which does not comply with these terms.
*Correspondence: Samuel Luke Nicholson, c2FtLm5pY2hvbHNvbkByZWFkaW5nLmFjLnVr; Dominik Fleitmann, ZG9taW5pay5mbGVpdG1hbm5AdW5pYmFzLmNo