- 1Institute for the History of Material Culture, Russian Academy of Sciences, St. Petersburg, Russia
- 2Arctic & Antarctic Research Institute, St. Petersburg, Russia
Ice-rich syncryogenic (termed “Ice Complex”) deposits are common in northern East Siberia and constitute the most important feature of the Quaternary geology of the region. The Ice Complex formed throughout the Late Pleistocene and not only contains an archive of paleoenvironmental proxies such as Pleistocene faunal remains, but also comprises a record of human habitation spanning ∼50,000 years, beginning with early MIS3. The development of syngenetic permafrost is an important variable in the formation of archaeological contexts in this depositional setting. Excavations of the Yana site complex in the lower Yana River area provide a unique opportunity to study archaeological finds preserved in Ice Complex deposits. Based on long-term field observations and dating results, we present important conclusions concerning the geology of the Yana sites. Taphonomic biases with potential to obscure the archaeological record are discussed. The thawing of frozen primary deposits has distorted depositional sequences, leading to the formation of secondary features and contexts, e.g., ice-wedge casts. Collapsed blocks of frozen sediment with undisturbed fragments of frozen layers containing artifacts and/or paleobiotic remains may become incorporated and refrozen into another depositional sequence and a source of misinterpretation and chronometric error. Furthermore, severe cryoturbation within the polygonal mounds warps the sediment in contact with the ice wedges; as a result, the contents of the sediment is uplifted with important consequences: 1) the hypsometric provenience of datable material is altered, creating chronometry problems; 2) in archaeology, there is an increased potential for misinterpretations with respect to dating, cultural classification, and human behavior; 3) transported material may form secondary concentrations at different hypsometric levels and thus bring further complications for its understanding; 4) in geology, the transportation of geochemical signatures may lead to erroneous interpretation of the geological potential of the area; 5) uplifted deposits contribute to increased Ice Complex thickness, which is thus not a direct function of sedimentation, but a combined result of sedimentation and redistribution of the deposits within an existing polygon deposit. Thus, the analysis of Ice Complex deposits during archaeological excavations at the Yana site complex has wider implications for Quaternary science.
Introduction
In the Northern hemisphere, permafrost underlies approximately 22.79 × 106 km2, or 23.9% of the exposed land surface. Permafrost is a trans-zonal natural phenomenon found in the Arctic and the cold temperate (boreal) climatic belt, spanning several landscape zones. The largest permafrost areas are the Asian (∼13 × 106 km2) and North American (∼7.2 × 106 km2) (Zhang et al., 2008); they extend from the shores of the Arctic Ocean southward, deep into the continents. In Northern Eurasia, the permafrost zone reached its greatest extent during the Late Pleistocene. During the Last Glacial Maximum (LGM), it was almost twice as extensive as at present (Velichko, 1973; Vandenberghe et al., 2014). In this enormous territory, frozen ground has various properties related to its formation and evolution that have been investigated for more than a century.
Siberia contains a variety of frozen deposits. Ice-rich permafrost, or “Ice Complex deposits,” are the most widely known phenomenon of the Siberian permafrost, first characterized by Solovyov (1959). Ice complex deposits, also known as the Yedoma Suite, are common in the arctic coastal lowlands of East Siberia (Murton et al., 2015; Opel et al., 2019; Romanovskii, 1993; Schirrmeister et al., 2011; 2013; 2017, Wetterich et al., 2014; 2020), and in northwestern North America (Froese et al., 2009; Kanevskiy et al., 2011), but also occur in the continental regions of East Siberia (Solovyov, 1959; Ashastina et al., 2017). These deposits accumulated across the Beringian landmass at its greatest extent during MIS3 and MIS2, including the exposed shelf areas, and thus they are present also on the arctic islands (Makeyev et al., 2003; Romanovskii et al., 2004; Schirrmeister et al., 2011; Pavlova and Pitulko, 2020). Stratigraphic profiles studied to date are primarily of MIS3 age (Schirrmeister et al., 2011; Wetterich et al., 2014), among which the Bykovsky Peninsula exposure spanning MIS4 – MIS2 is believed to be the most comprehensive.
Ice Complex deposits are represented by ice-rich silty sandy loams, clayey loams, and sands penetrated by syngenetic wedge ice. Deposits forming the Ice Complex include alluvial, lacustrine, slope, and aeolian sediments accumulated under an extremely cold and arid continental climate. The total ice content is very high, up to 80–95% in the arctic Yana-Indighirka Lowland and on the New Siberian Islands. Observed in profiles, Ice Complex deposits are dozens of meters thick and contain organic-rich deposits, such as inclusions and layers of peat, plant macro-fossils, and paleosol horizons.
The East Siberian Ice Complex deposits represent a unique late Pleistocene paleoenvironmental record comprising pollen, paleobotanical, and insect proxies (e.g., Kienast et al., 2005; Sher et al., 2005; Andreev et al., 2011; Wetterich et al., 2011; Jørgensen et al., 2012; Zimmermann et al., 2017; Pavlova and Pitulko, 2020), bones of Pleistocene animals (Sher et al., 2005; Nikolskiy et al., 2011; Pitulko et al., 2014), human remains (Lee et al., 2018; Sikora et al., 2019), and archaeological materials (Pitul’ko, 1993; Pitulko et al., 2016; 2017; Pitulko, 2019a). Information on past environments and landscape evolution, human behavior, and the development of the faunal communities are based on the taphonomy and chronometry of the deposits containing these remains. Partial or complete thawing of frozen deposits beginning ∼15,000 years ago and continuing throughout the Holocene generated multiple taphonomic biases obscuring the archaeological and environmental record (Katasonov, 1979; Kaplina, 1981, 2009; Shur, 1988; Makeyev et al., 2003; Gavrilov et al., 2006; Morgenstern et al., 2013; Pitulko and Pavlova, 2016).
In the archaeological record of arctic East Siberia, there are some gaps that may be attributed to permafrost degradation (Pitulko et al., 2016; 2017). Sites dating to the Pleistocene-Holocene boundary are most common, but cultural deposits of all the known sites have been significantly transformed due to permafrost degradation of the area, marked by the development of the alas complex 13,000–8,000 years ago. Many sites of that age may have been lost due to massive thermokarst processes and landscape restructuring during the Pleistocene-Holocene transition (Pitul’ko, 2001; Pavlova and Pitulko, 2020). Breaks observed in the archaeological record and/or the small number of known sites dating to specific intervals can be accounted for by a taphonomic bias due to permafrost degradation.
Owing to their nature, ice-rich deposits have significant potential for the formation of such biases. However, breaks in the archaeological record and small numbers of sites for a given period also are observed in continuously frozen and undisturbed geological contexts, as is shown in the study presented here of Ice Complex deposits in the Yana River area, arctic East Siberia.
Here, we present an account of geological observations made in the course of long-term archaeological excavation and geological investigation at the Yana site complex, and provide conclusions and interpretation of the findings with wider implications for Quaternary science, including its geological, cryological, and archaeological branches.
The Yana Site Complex: Location and Spatial Organization
The Yana RHS site, discovered in 2001 (Pitulko et al., 2004), is situated at the lower Yana River at 70°43′N and 135°25′E (Figure 1A) in the westernmost portion of the extensive coastal lowlands between the Yana River in the west to the Kolyma River in the east, within present-day Arctic East Siberia. This area is also known as the westernmost part of late Pleistocene Arctic Western Beringia.
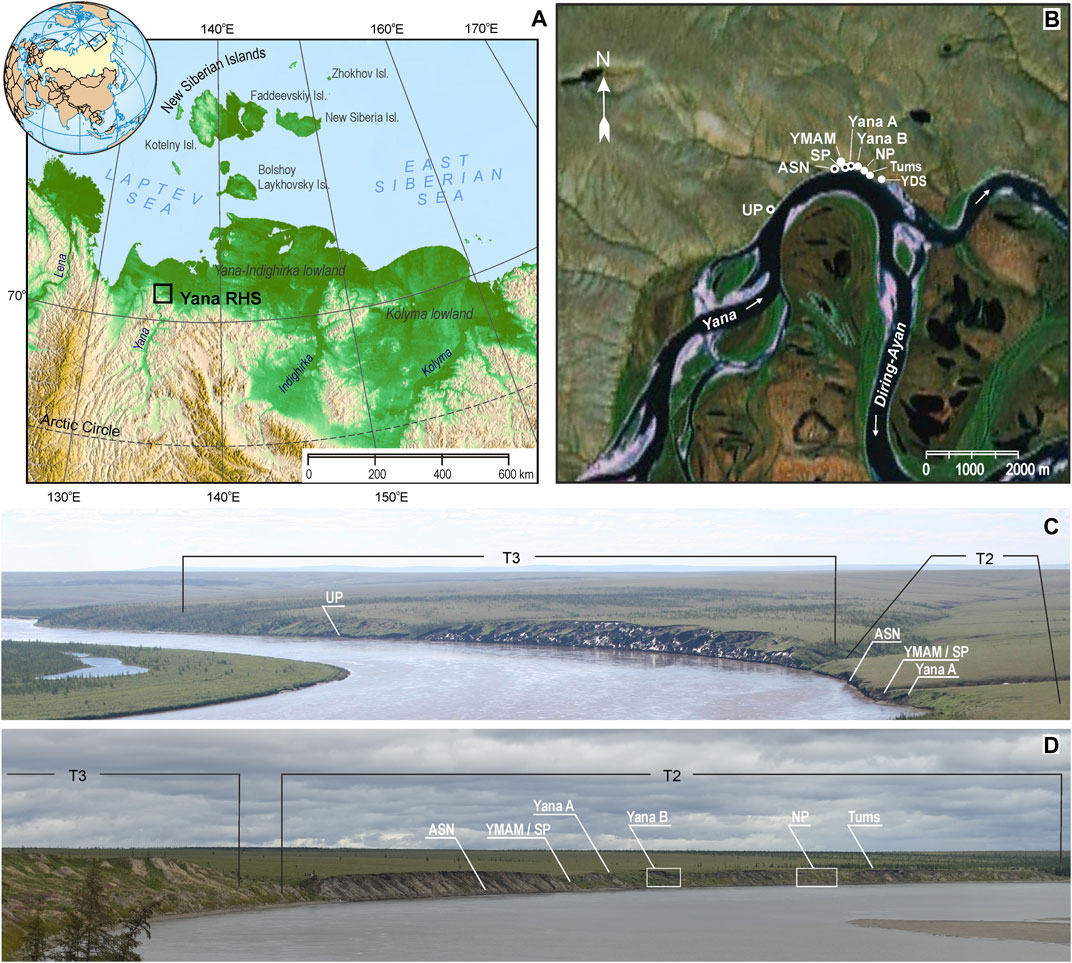
FIGURE 1. The Yana RHS site location and relationship between its structural elements: (A) – westernmost part of the late Pleistocene arctic Western Beringia (a fragment of ETOPO1 Global Relief Model image is used); (B) – schematic map of the area (a fragment of Google Earth satellite image is used. White circles mark localities that retain cultural material in situ – Yana B, Northern Point (NP), Tums 1, Yana Downstream Point (YDS), and Yana mass accumulation of mammoth (YMAM); circles with black center mark localities that yielded surface finds: Upstream Point (UP), ASN, Yana A, and Southern Point (SP); (C) – extent of the second (Т2) and the third (T3) terrace, and location of archaeological objects on the left riverbank of the Yana river viewed from the East and (D) – archaeological objects of the left riverbank viewed from southwest respectively.
Although “Yana RHS” is labeled an archaeological site, it is actually a complex of geoarchaeological sites. The individual localities apparently represent separate but roughly synchronic traces of human occupation (Pituko et al., 2013). The Yana site complex comprises at least seven known separate localities discovered within terrace 2 (T2), and at the Upstream Point locality (UP), which is confined to terrace 3 (T3) deposits (Figures 1B–D). The Southern Point (SP) area and Yana A area are spatially associated with the Yana mass accumulation of mammoth, or YMAM (Basilyan et al., 2011), while ASN locality represents a small concentration of lithic artifacts found roughly 150 m upstream from YMAM. The Yana B area is located approximately 200 hundred meters downstream from this group of sites and occupies a portion of the riverbank that recently has been subject to massive thermodenudation, which led to the formation of a thermoterrace. The Northern Point area (NP) of the Yana site complex is located few hundred meters downstream from the Yana B area (Figures 1B–D). Well described in Pitulko et al. (2004), the Tums1 exposure locates next to the NP area in some 200 m downstream from it. Finally, the Yana Downstream Point (YDP) is situated more than in 1,000 m downstream from the NP area. This locale so far has yielded only a single piece of human-modified mammoth tusk (Pitulko et al., 2017).
The state of preservation of these localities varies considerably. Some of them, including ASN, the Southern Point (SP area), and Tums1, are completely washed out (Pitulko et al., 2013), whereas others comprise extensive portions of extremely rich culture-bearing deposits. The latter are primarily represented by 1) YMAM, although this locality was heavily looted by local residents due to mammoth ivory mining (Pitulko et al., 2015a); 2) the dwelling site uncovered at the NP locality, which was the main excavation area in 2003–2015 (Figures 2A–E) and until it had been fully excavated; and 3) the Yana B area (Figures 3A–E). Yana “B” was first studied in 2003 and 2004. Test excavations continued in 2008 and 2009; since 2014, excavations at the Yana site complex have largely focused on this portion of the site. These two localities at the Yana site complex have yielded the most important archaeological, geological, and paleoenvironmental information (see, e.g., Basilyan et al., 2011; Nikolskiy and Pitulko, 2013; Pavlova and Pitulko, 2020; Pitulko and Pavlova, 2016; 2019; Pitulko et al., 2007; 2013; 2015a; 2017).
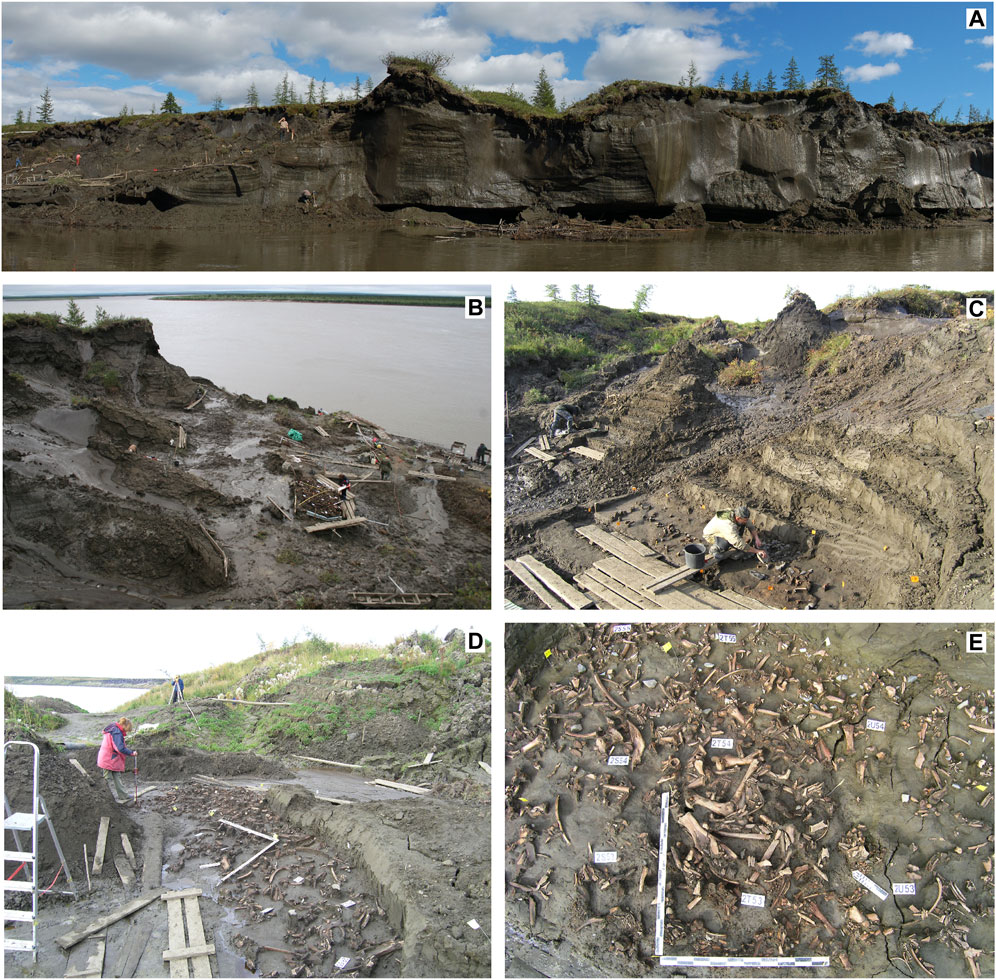
FIGURE 2. The Northern Point area (NP) of the Yana site complex: (A) panoramic view on the exposure of the Ice Complex deposits with the area excavated in 2008; (B) – the Northern Point excavation area viewed from the North-West in 2007 with clearly visible permafrost polygonal patterns including areas covered by culture-bearing deposits isolated inside the polygons walled by the ice-wedge network; (C) – excavations of the cultural layer in neighboring polygons in 2009; (D) – recording of the excavated material during 2012 excavation campaign; (E) – example of the excavated area of the cultural layer bearing dense accumulation of archaeological finds – lithic and bone artifacts, and Pleistocene animal bones.
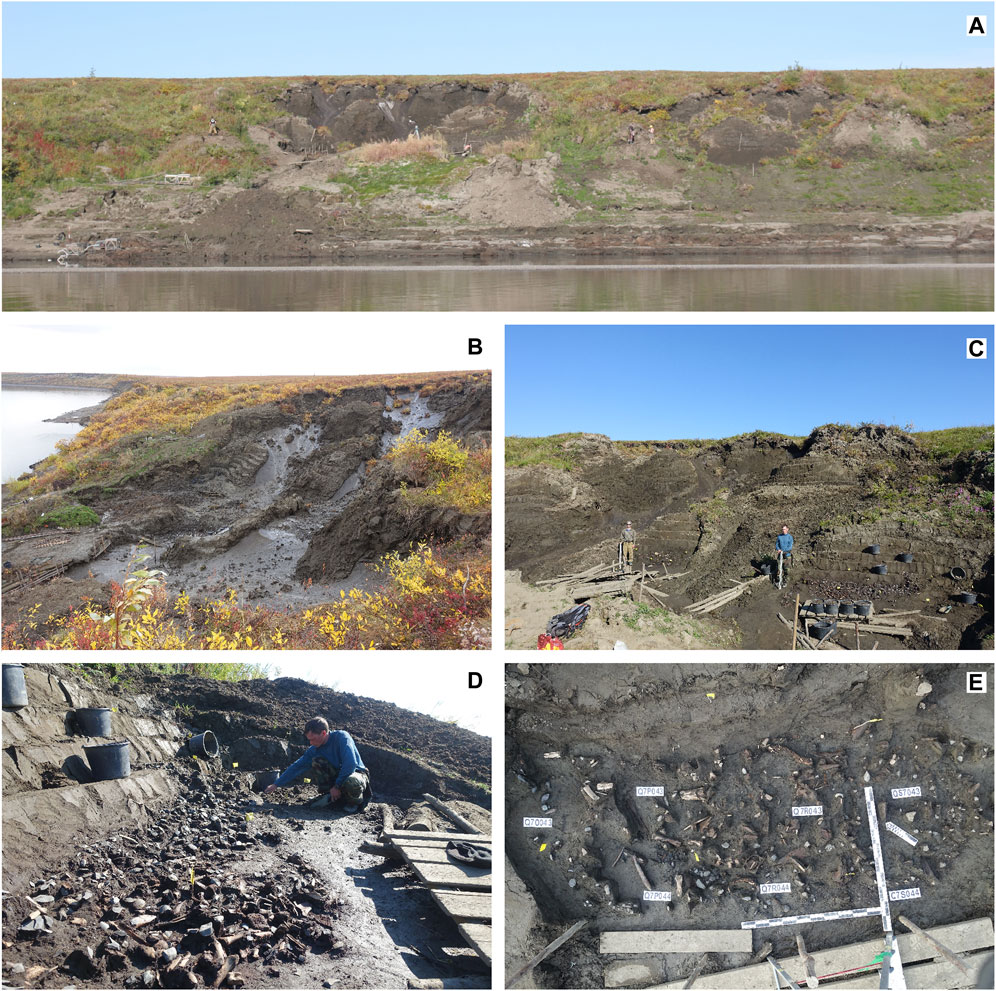
FIGURE 3. The Yana B area of the Yana site complex: (A) left riverbank in the location of the Yana B area of the Yana complex of sites viewed in 2017; (B) excavations in the Ice Complex environment viewed from an Eastern elevation in 2019; (C) excavated areas of the cultural horizon in two polygons located nearby, in 2019; (D) excavation process on one of such polygons performed in 2018; (E) ancient habitation surface displaying numerous finds of lithic artifacts and Pleistocene animal bones and their fragments.
Study Area
The complex of Yana sites is located within the permafrost zone. Vegetation of this area is typical for the transition from southern hypoarctic tundra to open northern woodland (Atlas of the Arctic, 1985; Yurtsev, 1994; CAVM Team, 2003). These tundra landscapes are composed of tussock-sedge, dwarf-shrub, and moss tundra associations. Moist tundra is dominated by tussock cotton grass (Eriophorum vaginatum) and dwarf-shrubs with some Betula exilis shrub vegetation, which coexists with grassy moss bog vegetation and occasional larch (Larix gmelinii) with dwarf shrubs (Vaccinium vitis-idaea, Arctous alpina, Empetrum nigrum, and Ledum palustre), lichen, and mosses in open floodplain woodlands.
The study area is located in the eastern part of a region characterized by a transitional climate: the coastal climate of the Arctic Siberian zone (Gakkel and Korotkevich, 1960; Atlas of the Arctic, 1985). The annual average temperature varies within −13.9 – (−)14.2°C, the mean January temperature is −37.4 – (−)38°С, and the mean July temperature reaches +11 – (+)11.5°С (Izyumenko, 1966; 1968). This is a harsh, cold, and dry environment with a short frostless period (57 days per a year). Average precipitation varies from 200 to 240 mm per year.
Within the study area, the Yana River alters course sharply (almost 90°), flowing roughly from West to East, and then turns again to the North (Figure 1). Along this portion of the river, the terraces are preserved only as fragments. At the present time the 3rd terrace level (T3) is 40–45 m, the 2nd terrace (T2) is 16–18 m, and the 1st terrace (T1) is 10–11 m above the water level (a.w.l.). All geomorphological surfaces lower than 9–10 m a.w.l. are within the modern floodplain.
Ice-rich permafrost deposits with polygonal syngenetic and epigenetic ice wedges are present in all three of the terraces. The ice wedges represent several generations (Pitulko et al., 2007; Basilyan et al., 2011). The ice content of the deposits varies from 30 to 70%. Such ice-rich deposits are termed Ice Complex deposits (Romanovskii, 1993; Romanovskii et al., 2004; Schirrmeister et al., 2011). Archaeological finds such as lithic and bone artifacts and artifact production waste, and Pleistocene animal bone remains, are abundant in the formerly occupied areas and form well-defined culture-bearing layers. Such layers occur in the middle part of T2 at 7–7.5 m a.w.l., and are an important stratigraphic marker for T2 deposits, providing chronological control.
The thickness of the seasonally thawed layer (STL) varies depending on exposure, lithology, and vegetation, but does not exceed 70–80 cm. All deposits at greater depth remain perennially frozen. At the level of the culture-bearing layer, the temperature of the deposits is presumed to be roughly −9°С, based on borehole measurements near Spirka Lake, 8 km northeast of the Yana site complex. As reported by Nekrasov and Devyatkin (1974), temperature values of −9–10°C have been recorded at a depth of 7–8 m below surface; observed temperature next to the village of Kazachie some 30 km downstream from the Yana site complex area was −8.5°C at the same depth. The frozen sediment has ensured excellent artifact preservation required a special excavation strategy (Pitulko 2008). At the same time, these deposits are highly susceptible to natural agents such as running water, summer insolation, and atmospheric heat that cause erosion.
To study them, an annual topographic survey has been conducted on the left bank where T3 and T2 are well expressed. The study area covers 2.5 km of the riverbank, where retreat of the latter comprising Ice Complex deposits is closely associated with erosion caused by the Yana River, as well as thermal denudation and thermokarst processes, and the impact of solar radiation, both direct and indirect (i.e., water-reflected). The rate of riverbank erosion also depends on water discharge. There is a direct connection between mean summer temperatures and riverbank retreat. The latter is accompanied by the development of erosional landforms such as thermoerosional pits, thermokarst, erosion channels, and niches. The combination of all these factors causes rapid destruction of the riverbank.
High water stands in the Yana River reach 7–8 m above the average water level. The ice-rich deposits of the site are subject to lateral river erosion. Retreat of the T3, observed for 17 years (2003 through 2020), averaged 12–13 m/year during 2003–2009, but then slowed to 3–4 m/year. A maximum rate of 22 m/year was observed in 2006. The mean rate of erosion for T2 for the same period was 6–7 m/year, whereas the maximum was 30 m/year, observed in 2014–2016. Within the excavated area of Yana RHS, the mean value for riverbank retreat was 7 m/year with a range between 1.8 m/year and 9.8 m/year (Pitulko et al., 2012).
In 2019, it was discovered that the Northern Point excavation area of the Yana site complex had been almost entirely washed out (Figure 4). Based on a long-term observation, active erosion correlates with gradual migration of the upstream portion of the gravel bar opposite the middle part of T3, which redirects the stream into a westerly direction. This process affected a portion of the bank located further downstream, undercutting the previously stable T2 riverbank slope and eroding culture-bearing deposits of the Yana site complex. Overall, based on both measurements and information provided by local residents, we conclude that in 2005–2006, the Yana River entered new hydrological cycle, following an interval of low activity that began in the early 1990s. The length of such short-term cycles may be roughly 15 years.
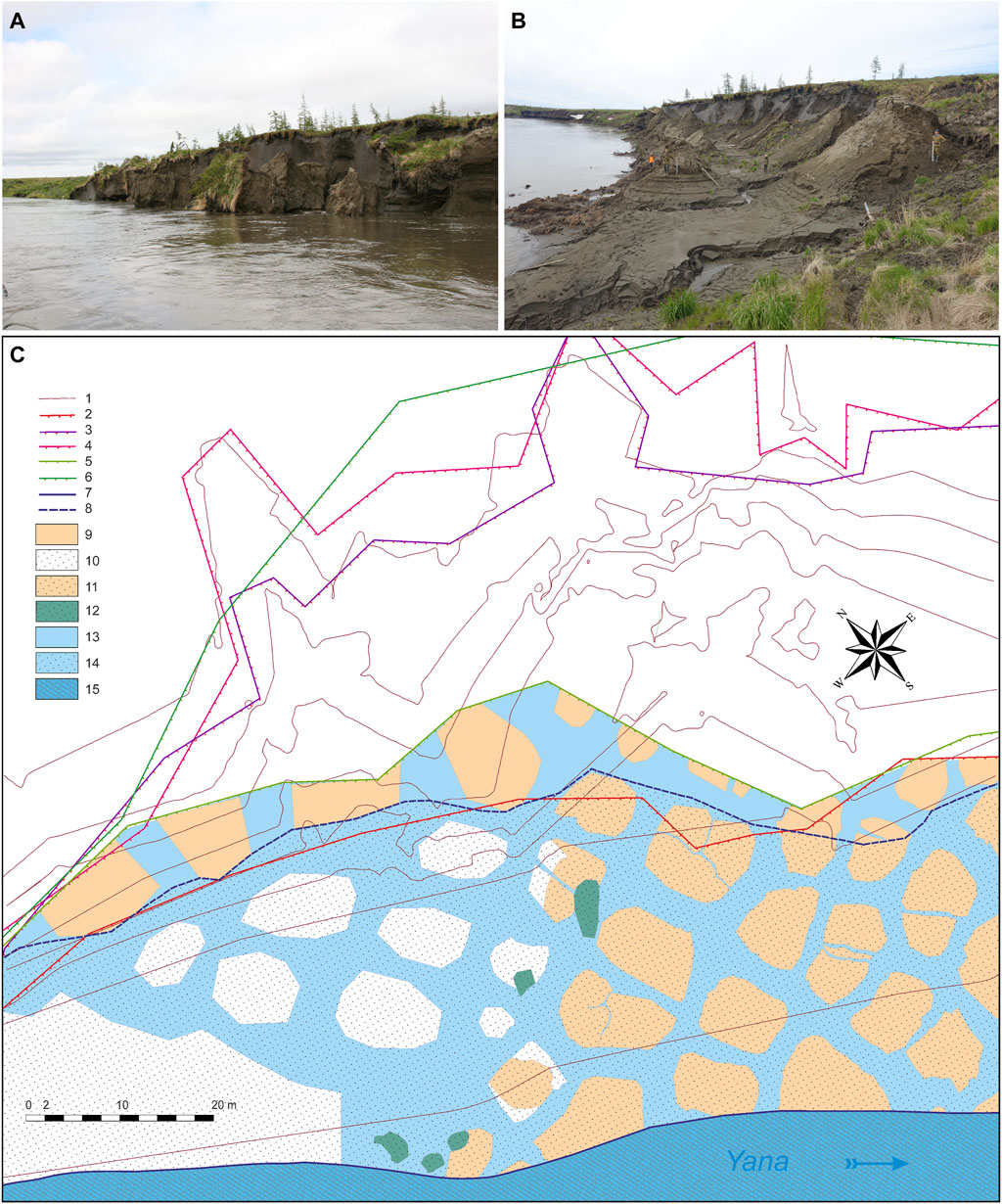
FIGURE 4. Destruction of the left river bank in the area of the Northern Point area of the Yana complex of sites by the thermal erosion: (A) – the catastrophic collapse of large blocks of the bank deposits between Northern Point and Yana B zone in 2016; (B) view of the same area in 2017 during low water stand; (C) – development of the lateral erosion observed on the river bank in the western portion of the excavation at the Northern Point area based on topographic survey. Illustration key: 1—contour lines are given in 2 m increments; 2–6—location of the second terrace brow recorded in different observation years (2—2007; 3—2013; 4—2014; 5—June 2016; 6—September 2016); 7—the lowest water level in 2016; 8—the highest water level of 2016; 9—permafrost polygons holding in situ cultural layer; 10—modern alluvium covering thawed permafrost polygons; 11—modern alluvium covering permafrost polygons, in which the cultural layer was studied during excavations in 2003–2015; 12—collapsed frozen blocks, which contained a cultural layer, studied in a sub-“in situ” position in 2016; 13—polygonal ice wedges in frozen cliffs; 14—thawed polygonal ice wedge covered with modern alluvium; 15— channel alluvium. Compiled by S.G. Kritsuk and E.Y. Pavlova.
Geology and Age of the Yana Site Complex
In the T2 section that contains the cultural layer of the Yana RHS, four geological members can be distinguished (Figure 5A). These members are separated by stratigraphic unconformities and erosion surfaces that correspond to extensive sedimentation breaks. The basal erosional part of the T2 (Figure 5A: Members 1–2) occurs only in the upstream area of the exposure. Member 1 is represented by alluvium-lacustrine and thaw-lake deposits with ice-wedge casts; these deposits were formed during the upper Lower Pleistocene and Middle Pleistocene. Although it is well expressed locally within a portion of T2 near its junction with T3 (Figure 5A), only heavily eroded patches of Member 1 deposits appear sporadically at the base of T2 within the Yana site area. Member 2 consists of alluvium-lacustrine and aeolian deposits of early Late Pleistocene age, which are found only in the area adjacent to the T2/T3 junction. The age of the basal unit of T2 probably lies between the end of the Early Pleistocene and the beginning of the Late Pleistocene (Basilyan et al., 2009; Basilyan et al., 2011). This ancient alluvium is a remnant of the T3 fill that has been laterally eroded and down-cut by the river during the formation of T2.
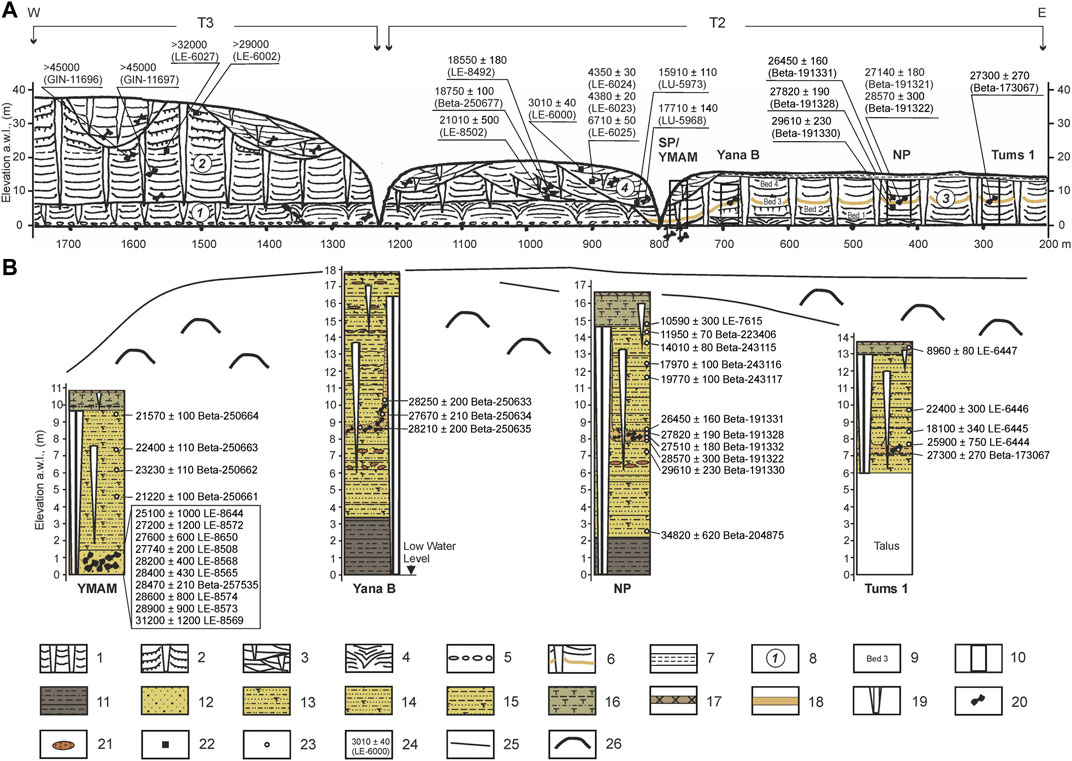
FIGURE 5. Overview of the geology of the Yana complex of sites. Soplivaya Gora (Yana-195) reference profile of Quaternary deposits (A) and cryolithology for different localities within the Yana RHS – YMAM, Yana B, Northern Point, TUMS 1 (B). Illustration key: 1—Ice Complex deposits of different geological age, with syncryogenic ice wedges in alluvial and proluvial deposits; 2—aeolian deposits; 3—cross bedding; 4—ice-wedge cast; 5—conglomerates; 6—position of the Upper Paleolithic Yana RHS cultural layer; 7—deposits of the Holocene cover complex; 8—number for geological Member; 9—bed number within the Member 3; 10—location of cryolithological profiles; 11—interbedding of clays, silts, and clayey silts; 12—sand with small pebbles; 13—sandy silt; 14—clayey-sandy silt; 15—sandy-clayey silt; 16—interbedding of clayey silt bands and sandy-clayey silt with beds and lenses of peat; 17—active layer; 18—cultural layer in situ; 19—polygonal ice wedges; 20—position of Pleistocene animal bones within the deposits; 21—peat lens; 22—position of 14С samples on the geological profile (A) (plant remains dated); 23—position 14C samples within local cryolithological profiles (B); 24—radiocarbon date and lab code; 25—terrace edge (schematic); 26—permafrost mound (baidzharakh) —cone-shaped land forms resulted from partial thawing of polygonal ice-wedge network.
Member 3 (Figures 5A,B: Bed 1–4) and Member 4 represent the fill of T2. Alluvial deposits of T2 started to accumulate shortly after 40,000 years ago. Alluvial deposition ceased around 13,000 14C years ago (when T1 deposits began to accumulate) with the beginning of aeolian sedimentation. Geologically young erosional cuts filled with terminal Pleistocene and Holocene deposits (Member 4) were observed at the top of the T2 sequence. Geological age estimates are based on multiple radiocarbon age determinations, which are consistent throughout the study area (Figures 5A,B). The culture-bearing horizon reflects several human habitation episodes taking place in the late MIS3 (Pitulko et al., 2013; Pitulko et al., 2015a). Although their number and length are not clear, we conclude that humans occupied the Yana site complex for several thousand years at the very end of MIS3 and briefly visited the area during the LGM (Pitulko et al., 2017).
Member 4 (Figure 5) caps the T2 sequence. It is composed of alluvial and proluvial deposits of varying terminal Pleistocene and Holocene age. Deposits of this member consist of fine-grained sand with gray silt – cross-bedded, shoestring/lenticular sands that fill erosion channels. These deposits exhibit numerous unconformities with Member 3. These unconformities represent backfilling of erosional channels and depressions that largely formed in Member 3 deposits during the terminal Pleistocene and middle Holocene, whereas some of them may be relatively recent. These sediments contain a large number of plant remains, such as allochthonous peat concentrations and grass hummocks, roots and twigs of woody plants, and fragments of driftwood. The Member 4 sequence is capped with Holocene surface deposits (after Kaplina, 1981), represented by ice-rich silts with peat inclusions, peat-bog deposits, locally distributed on the surface of the terrace, and proluvial deposits backfilling recent stream channels incised into its surface. The base of the Holocene cover layer truncates the uppermost portion of the ice wedges that belong to the Ice Complex deposits. Member 4 is characterized by epigenetic freezing represented by alternating layers with ataxitic and reticulated cryotextures, and short and wide epigenetic ice wedges, the width of which in the upper part reaches 1.5 m. Member 4 deposits vary 1.0–2.5 m in thickness.
The structure and geocryology of Member 3 are highly germane to the present study, and below we present a detailed description based on the observations of the profiles in the NP area of the Yana site complex (Figures 6A,B). The sequence is uniform along the riverbank. Similar geology is observed for T2 also at the Tums1 locality (Pitulko et al., 2004) and at Yana B as well (Pitulko, 2019a) (Figure 6C).
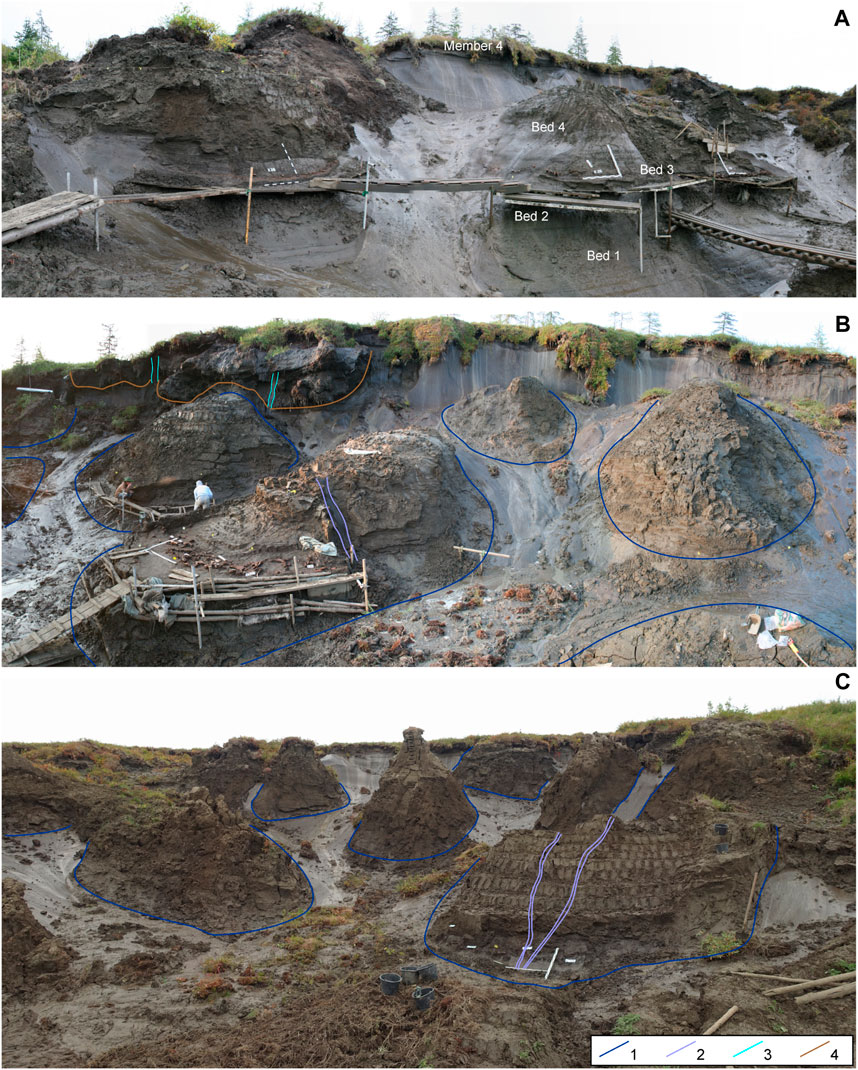
FIGURE 6. Cryology of Member 3 deposits: (A,B) – Northern Point area; (C) – Yana B area of the Yana complex of sites. Illustration key: 1—outlines of the first generation of the late Pleistocene (MIS3) ice wedges; 2—outlines of the second generation of the late Pleistocene (MIS2) ice wedges initiated at the LGM; 3—Holocene ice veins; 4—base of the peaty deposits of Member 4 of Holocene age.
The syngenetically frozen deposits of Member 3 (Figures 5, 6) are composed of several stratigraphic units of varying appearance and thickness. Additionally, it contains the characteristic cultural horizon of the Yana sites, which occurs 7.5 m above the average summer water level (Figures 5A,B). The four clearly distinguishable beds include the following:
Bed 1, represented by a near-channel alluvium facies, occurs in the lower part of Member 3. Bed 1 deposits are composed of fine-grained sands interbedded with sandy silts and layers of gray and dark gray plant detritus. Bed 1 is up to 5–6 m in thickness. The contact with overlying Bed 2 sediments is gradual reflecting the shift to floodplain conditions.
Bed 2 consists of a floodplain alluvium facies, represented by clayey-sandy and sandy-clayey silts with washed plant detritus. The thickness of Bed 2 is 0.5–0.6 m.
Bed 3 is represented by a floodplain alluvial facies of sandy and clayey-sandy silts of gray-brownish and reddish-brownish color, with thin layers of fine-grained sand. The sediments are rich in plant detritus and contain a large number of thin plant roots in situ (i.e., in primary context). Bed 3 contains lithic artifacts (stone tools and lithic debitage pieces), bone and mammoth ivory artifacts, and abundant Pleistocene animal bones and bone fragments (Figures 2D,E, 3D,E). Additionally, there are multiple hearth structures, typically circular, about 1 m wide and 20–25 cm deep (Figures 7A–F). Bed 3 represents the culture-bearing horizon of the Yana site complex, or the ancient habitation surface. The thickness of Bed 3 varies between 0.3 and 0.6 m. The presence of in situ cultural material marking the living surface briefly occupied by late Pleistocene humans also indicates a break in the fluvial sedimentation and a shift to aeolian deposition.
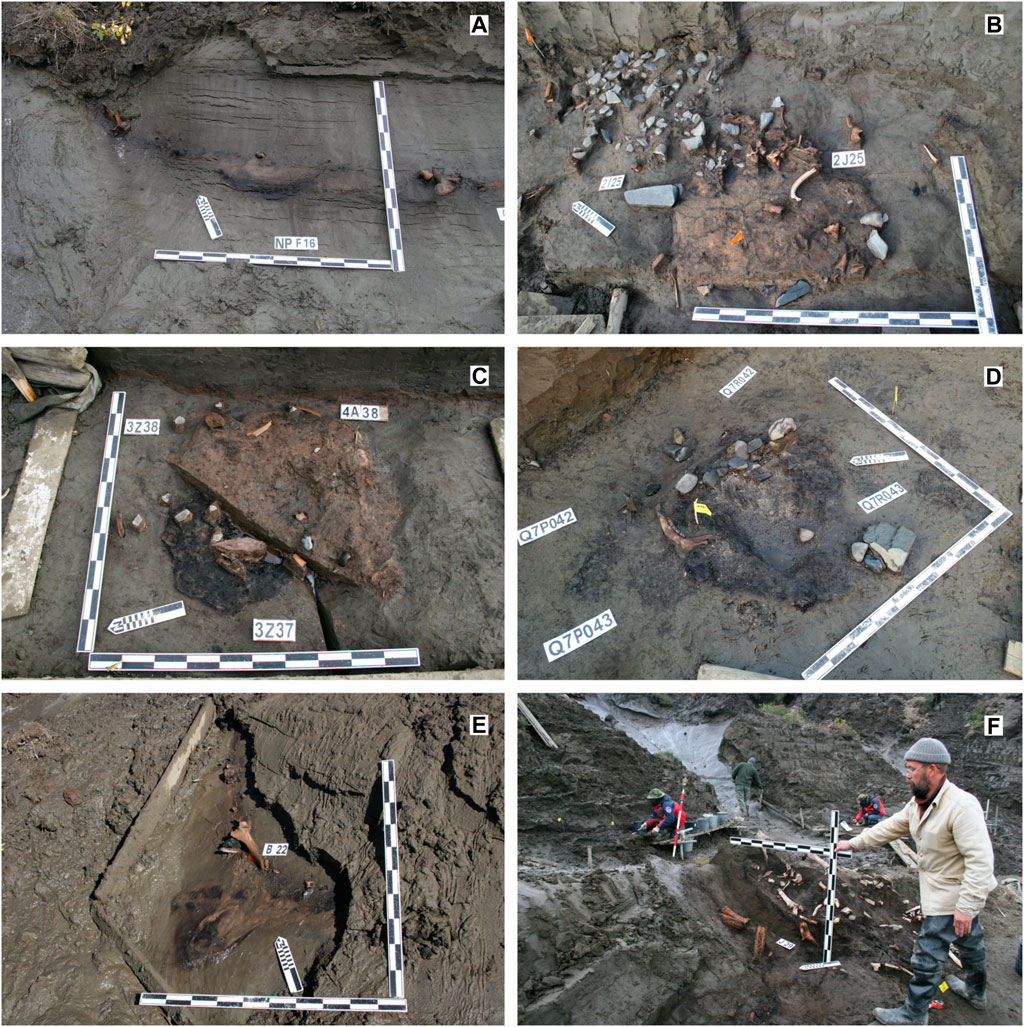
FIGURE 7. Hearth structures opened in the culture-bearing deposits of the Yana site complex: (A) – 20 cm thick cultural layer in the frozen front within the excavation area, depression related to the hearth structure is clearly seen at the crossing of F16 excavation unit of the Northern Point area, excavations of 2004; (B) – excavated habitation surface with stone knapping workshop area near the hearth discovered in 2J25 excavation unit, excavations of the NP area in 2009; (C) – hearth structure in 3Z37 excavation unit at NP area in 2008; (D) – hearth structure in Q7R043 unit, excavations at the Yana B area of the Yana site complex in 2018; (E) – cryogenic deformation of the hearth structure observed in the frozen wall next to the polygon deposit contact with the ice vein observed in В22 unit, Northern Point, in 2005; (F) – cryogenic deformation observed in the frozen wall next to the contact between the ice vein and the polygon deposit in J29 unit, NP area in 2007.
Bed 4 deposits represent a high floodplain facies, with two strata of horizontally layered sandy, clayey-sandy, and sandy-clayey silt containing two paleosol horizons, characterized by a high saturation of in situ plant roots (Bakina et al., 2017). Bed 4 deposits are 5–5.5 m thick.
Generally, Member 3 is composed of sandy-silt and silty sediments. The sand content changes within the profile (Pitulko et al., 2013; Pitulko and Pavlova, 2016), decreasing from bottom to top. Beds 1 and 2, which form the base of the T2 profile, have a higher sand and ice content than Beds 3 and 4, which are composed mostly of fine-grained silt. The sequence reflects a pattern typical of Ice Complex deposits of alluvial origin, exhibiting no visible breaks except for the period of human habitation. It reflects the accumulation of deposits on a floodplain that gradually changed to a high floodplain before the cultural remains were deposited, and then remained a high floodplain until the alluvial sedimentation stopped at around 13,000 years BP. Thus, the accumulation of Member 3 deposits of terrace T2 spans a period between late MIS3 and MIS2, which also is confirmed by the paleoenvironmental proxy record, reflecting relevant temperature and humidity trends (see, e.g., Pitulko et al., 2007; 2017; Pavlova and Pitulko, 2020).
The geocryological properties of Member 3 reveal the freezing of the deposits under varying environmental conditions. The cryotextures of Member 3 deposits represent layered parallel-concave striations, typical for high floodplain topography with low-centered permafrost polygons (Danilov, 1983). Well-expressed ice belts are characteristic of the lower part of the stratigraphic sequence, below the culture-bearing horizon (Figure 6A: Bed 1). Along with these ice belts, the lithological boundaries and the cultural layer exhibit a pronounced warping at the marginal parts of the polygon body. Generally, Beds 1, 2, and 3 are characterized by syngenetic freezing, indicated by the formation of thin micro streaks, and also by massive cryotextures and three generations of ice wedges.
Wide syngenetic ice wedges of the main/oldest generation penetrate Beds 1, 2, and 3 extending below the average water level (Figures 2A, 5A,B). Their vertical length varies 16–18 m. In the lower part of Member 3, their width is 1.5 m, but gradually increases upward to 4 m at the top of the profile, where they are truncated and covered by Holocene deposits. These ice wedges form a regular polygonal pattern (Figure 6); the sides of the polygon vary between 4 and 9 m in length and, thus, the area of the polygon may reach 25–50 sq.m. The width of the ice wedges may be 6–8 m at the junction of two ice wedges. Remarkably, at the elevation of 11–12 m a.w.l., the width of ice wedges of the oldest generation decreases to 1.5 m. This indicates significant environmental change during their development, i.e., reduction in moisture and decline in winter temperatures.
The second generation of ice veins wedges displays an irregular pattern superimposed on the polygonal network formed by the older ice wedges (Figures 6B,C). The ice wedges are 0.2–0.3 m wide. Their upper surfaces are found at an elevation of 11–13 m a.w.l.; from this elevation, they penetrate downward into deposits of Member 3 for roughly 5–7 m and terminate at ∼6–6.5 m a.w.l., extending into Bed 3 (the culture-bearing horizon) and the upper portion of Bed 2. Accordingly, the second generation of ice wedges is epigenetic to Beds 2 and 3, but syngenetic to Bed 4 deposits. As indicated by their spatial distribution, they are often superimposed on the previously formed polygons of the first generation.
The third and youngest generation of the ice wedges is common in the upper part of Member 3 deposits. These ice wedges penetrate T2 deposits to a depth of 3–4 m and do not extend to the culture-bearing deposits.
The above-mentioned geocryological features of the Member 3 deposits were observed during investigations of the Northern Point area (Pitulko et al., 2013). They are typical for the study area within the portion of T2 to which the culture-bearing deposits are confined, as demonstrated by the study of the Tums1 exposure (Pitulko et al., 2004) and the Yana B area (Pitulko, 2019a). The Tums1 sequence shows patterns resembling the NP area; however, the Yana B polygonal ice wedge network is slightly different from that at NP. The difference is manifested by increasing ice wedge width and reduced intra-polygonal area at the Yana B locality compared to Northern Point, where areas within the polygons are larger.
Most likely, this difference is due to differences in available moisture at the time of ice wedge development in various locations on the floodplain; the Yana B area seems to have been saturated, whereas the NP area was dryer. Additionally, second-generation ice wedges are more common at Yana B, whereas almost absent in the central part of the Northern Point locality, but better represented near the margin, where deposits indicate waterlogging.
Because the chronometry of T2 is well established, the development of the syncryogenic deposits of Member 3 can be linked to the paleoclimatic record. Hence, the formation of the oldest generation of ice wedges follows the start of Bed 1 accumulation roughly 40,000 years ago. Generally, this time corresponds to middle MIS3, or middle Karginian in the widely known Siberian geological terminology. Although no significant cooling during this interval was found in Western Beringian paleoenvironmental records (e.g., Wetterich et al., 2014: Figure 11; Pavlova and Pitulko, 2020), the formation of the main generation of ice wedges of T2 probably correlates with the H4/GS 9 stadial of the Greenland ice core climatic record, that is, ∼39,900–38,200 years ago (Svensson et al., 2008; Rasmussen et al., 2014). At that time, near-channel deposits of Bed 1 formed under alternating alluvial and aeolian deposition during warmer months, and clearly remained subaerially exposed over the winter, which is a precondition for initial frost cracking and ice wedge development. It should be stressed that the present-day high floodplain deposits in the study area are perennially frozen and contain ice wedges of recent age, being formed within the past two thousand years and demonstrating that the environmental conditions necessary for ice wedge growth were present in the recent past.
For the next 10–12 thousand years, the accumulation of Bed 1 continued and its surface eventually reached an elevation where it remained above the water level for most of the year including the summer months, as indicated by the seasonality of the occupation at Northern Point, deduced from the composition of faunal remains and reconstruction of human activity (Pitulko et al., 2013; Pitulko and Pavlova, 2019). The Yana B area was wetter and hence could be used during the winter, which is supported by multiple lines of archaeological evidence (Pitulko, 2019a); the preference for winter occupation in this area likely reflects human decision-making based on several factors, including variation in snow cover depth, for sanitary reasons, and/or proximity to mammoth bones concentrated at YMAM and used for fuel at Yana B in large quantities (Pitulko, 2019a). Moreover, summer temperatures at the time of occupation of the Yana sites were warmer than at present (Pitulko et al., 2007), and thus the depth of the STL base should have been greater than at present.
The second generation of ice wedges formed shortly after occupation of the Yana site area ended about 26,000 years ago, most probably due to climate change corresponding to the beginning of the LGM. Frost fissures penetrated through compact deposits of the living surface (Bed 3) and the upper part of Bed 2, and the second generation of ice wedges began forming. Then, both generations of ice wedges continued to grow but the older ice wedges exhibit some reduction in width during the LGM. These events are reflected in the spatial patterns of the archaeological remains at the Yana site.
Excavations of the Northern Point area of the Yana site complex performed in 2003 through 2015 opened more than 3,500 sq.m of the ancient living surface and revealed a dense concentration of occupation debris. The original permafrost polygonal pattern is clearly visible among the mapped cultural features and distribution of artifacts (Figure 8A). Cultural materials and structures are confined to the area within the perimeter of the polygonal bodies, which are separated from each other by the ice wedges. Combined with the latter, which are devoid of cultural materials, the total excavated area equals roughly 8,000 sq.m.
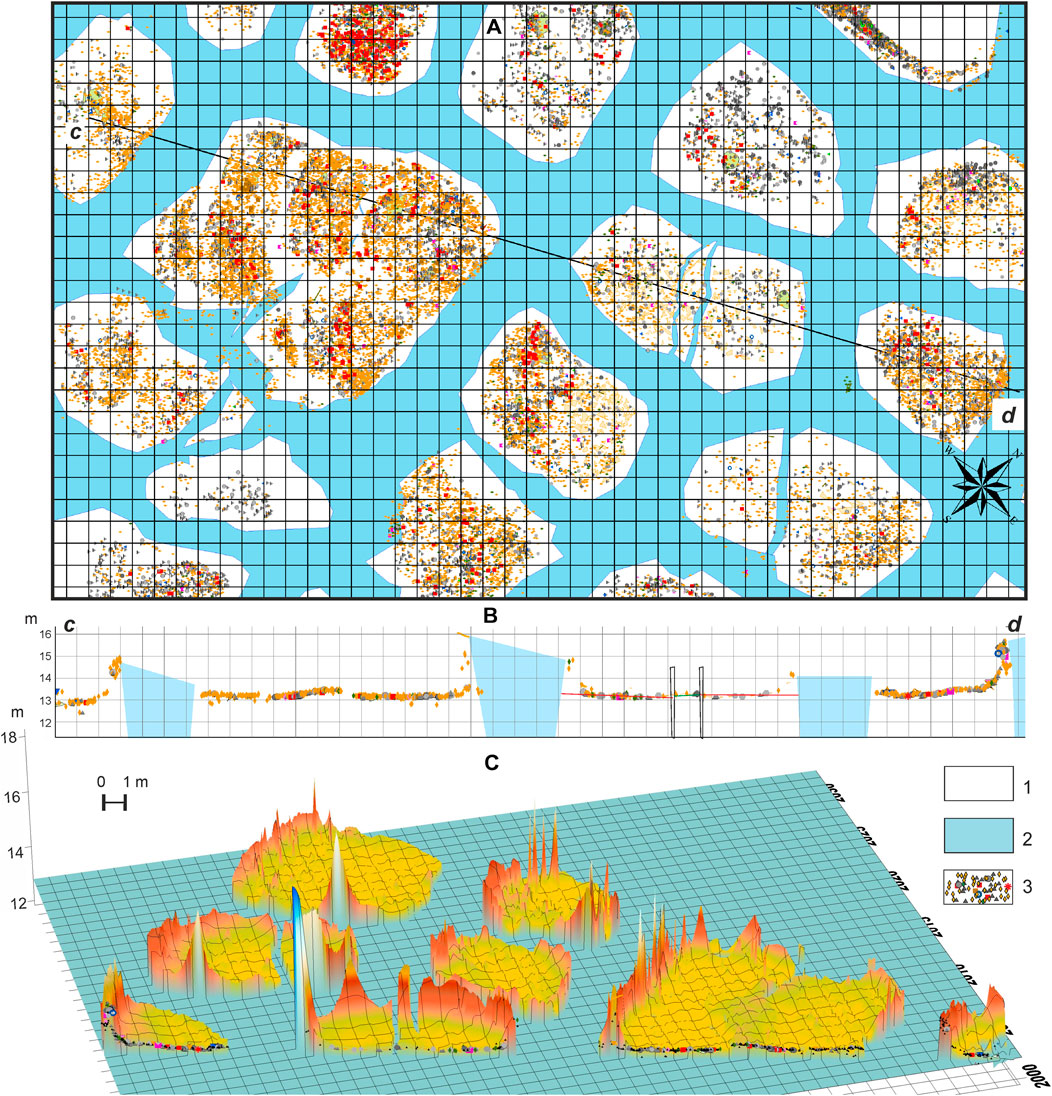
FIGURE 8. Spatial distribution of in situ mapped cultural material of the Northern Point locality: (A) – excavation map; (B) - hypsometric profile along line along line c–d shown in part (A) within 13–16 m elevation in absolute marks viewed from South; (C) - spatial distribution chart for archaeological material along line c–d shown in part (A) viewed from North. Illustration key: 1—polygon ground body deposits; 2—ice wedge; 3—cultural material. After Pitulko and Pavlova (2016).
The excavations yielded an enormous quantity of material, including more than 150,000 artifacts, bones, and bone fragments (Pitulko et al., 2013; Pitulko, 2019a) with a complex spatial distribution, further complicated by the polygonal pattern of the permafrost disturbing the occupation surface (Figures 9A–D, 10A–D) and distorting the spatial arrangement of the cultural remains (Figures 8B,C). Separate pieces of that puzzle, however, may be conjoined by matching portions of the living floor that have been divided by ice wedge formation with their counterparts across the adjacent ice wedge. Remarkably, the former hearths found within many of the polygons appear to be unrelated to the margins of the permafrost polygons (Figures 7B–D); some are located adjacent to an ice wedge margin and have been significantly disturbed by its growth (Figures 7A,E,F).
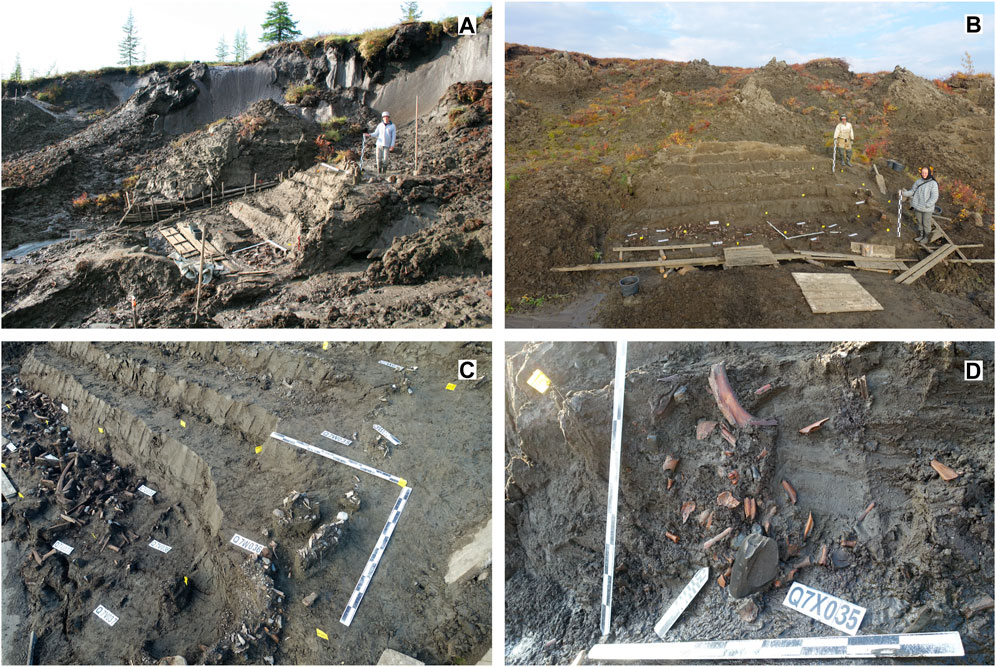
FIGURE 9. The culture-bearing horizon of the Yana complex of sites: (A) excavations of the Northern point area in 2009: in the front of the central part of the polygon, the cultural layer is opened in subhorizontal position while in the rear part of the polygon next to the ice-wedge contact the cultural layer is found 2 m higher due to vertical deformation caused by the ice-wedge growth; (B) excavations of 2020 at the Yana B area: the cultural layer stretches horizontally in the middle part of the polygon deposit but becomes smashed in its marginal zone gradually taking a vertical position seen in bending and archaeological finds that moved upward by ∼1.5 m; (C) the same polygon viewed from the North, with a narrow (1 m wide) strip of cultural material taking a position higher than the source level; (D) excavations of 2019, Yana B area, vertical frozen wall at the polygon boundary near the contact zone between the polygon body and the ice wedge, vertical and nearly vertical distribution of archaeological material in the bended part of the layer occurred due to cryogenic deformation of the cultural layer because of the ice-wedge growth after the formation of perennially frozen deposit which included the cultural horizon.
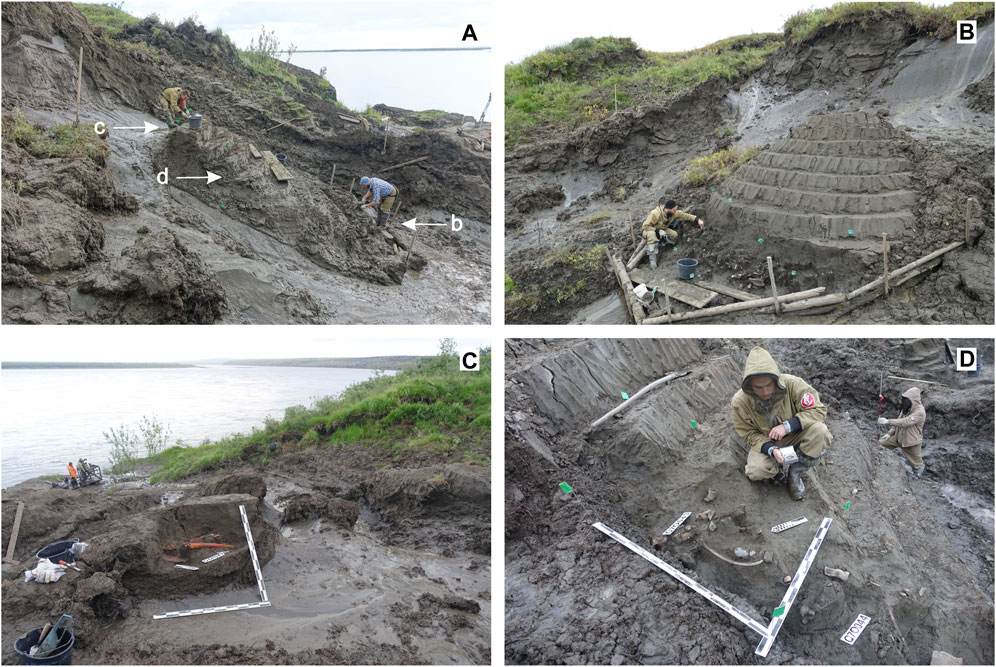
FIGURE 10. Spatial and hypsometric distribution of the cultural layer observed in different parts of the same permafrost polygon revealed by the excavations at the Yana B area in 2017: (A) polygon with cultural horizon viewed from the West, arrows indicate locations of b, c, and d photographs documenting different parts of polygonal mound; (B) – subhorizontal position of the cultural layer in the middle part of the polygon deposit viewed from the East; (C) portion of the cultural layer near the ice-wedge border in the rear part of the polygon lays 3 m higher relative to the source level shown in a, view from the North-East; (D) narrow strip of the cultural layer bended up near the polygon margin, view from the North-West.
The pattern described above suggests that the occupation surface was not subdivided into separate polygon-related areas at the time of human occupation. Instead, the paleo-surface was relatively dry, well drained, level and intact, and unaffected by either a low- or a high-centered polygonal pattern, which would have been avoided by humans as a habitation area. The ice wedge network was in the process of formation, but the uppermost portion of the developing ice wedges remained at least 1 m below the surface, as indicated by analysis of the geology and geomorphology of similar contexts at the Yana site complex, i.e., on the former low and high floodplain surfaces, where observed depth of the permafrost table equals 1 m. Thus, the spatial pattern observable at the culture-bearing horizon is a function of ice wedge development, which continued after the period of human occupation, until the end of the Pleistocene. Portions of the culture-bearing horizon were subdivided by the constant and intense pressure of the growing ice wedges on their sedimentary matrix.
Based on the measurement of individual finds (artifacts, animal bones, and their fragments) in a 3-D coordinate system, performed with a TOPCON GTS-229 total station and a SOKKIA CX-106 instrument, we can estimate the degree of cryogenic deformations of the culture-bearing horizon resulting from ice wedge development (Figures 8B,C, 11A,B). Recorded changes in the hypsometric position of the artifacts adjacent to the margins of the polygons show displacement of 3–5 m relative to the undisturbed portion of the cultural horizon in the center of the polygon. Thus, in polygons containing a portion of the cultural layer, a sterile zone without artifacts can be observed along the perimeter of the polygon at the level of the cultural horizon (Figures 8A,B, 11C). Typically, the artifact-free zone is approximately 1 m wide; here, the cultural deposits are replaced by a yellow-greenish ice-rich sandy silt derived from beneath the cultural horizon (i.e., uplifted from its original stratigraphic provenience).
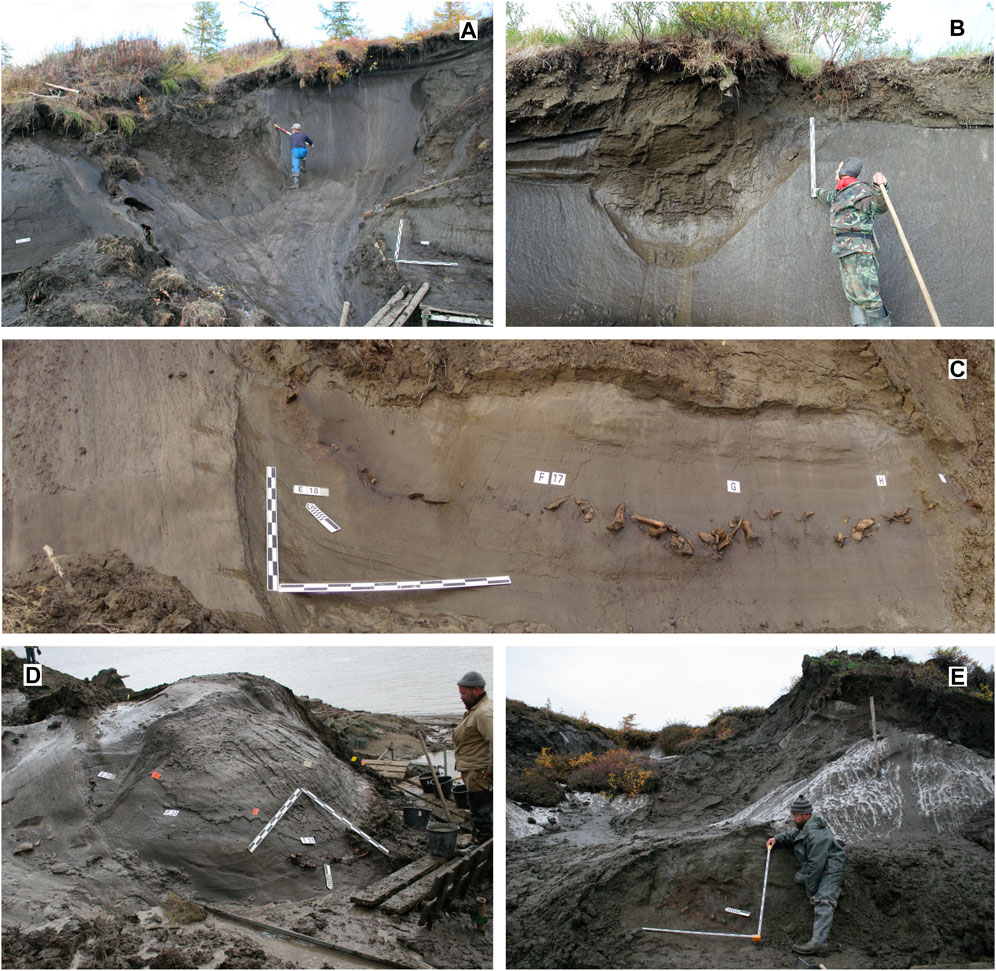
FIGURE 11. Vertical deformations of the cultural layer related to the growth of the ice wedges belonging to the oldest (MIS3) generation dissecting Member 3 deposits: (A) position of frozen in situ cultural layer bended up following the ice-wedge contact (in the right part of the picture) and hypsometric position of bones traveled up from the cultural layer and found 1.5 m below the second terrace (T2) brow (central upper on the picture), viewed from the South, NP area, excavations of 2005; (B) position of the cultural layer with deformations and a bone moved up alongside the ice wedge almost to T2 brow, the bone is found at 1.2 m depth below the present surface, view from the South, Yana B excavation area, excavations of 2016; (C) cultural layer observed in the frozen vertical wall: subhorizontal strip of the cultural layer sharply bends up in 1 m distance from MIS3 ice-wedge margin, view from South-Southwest, NP area, 2004; (D) vertical deformation of the cultural layer alongside the contact of the ice wedge and polygon deposit, viewed from the North, Northern point, 2007; (E) portion of the cultural layer in vertical position near the boundary between MIS3 ice wedge and the polygon deposit, viewed from the West, NP, 2006.
Additionally, there is a pronounced warping of the cultural horizon beginning approximately 1 m from the polygon margin, and significantly uplifted cultural material near the contact of the polygon deposits and the ice wedge (Figures 8B, 11C). The horizontal orientation of the cultural layer is transformed to a nearly vertical one (Figures 11D,E); in some cases, former hearths were found heavily disturbed along the contact zone. The upwarped portions of the cultural layer have been transformed into bands of isolated artifacts and bones which have been uplifted with the increasing thickness of the deposits. The vertical provenience of artifacts found within these cryogenic deformations adjacent to the margins of the polygon is 3–4 m above the cultural layer in the central part of the polygon; isolated bones derived from the ice-wedge contact zone are uplifted even higher. Such displaced finds were recorded whenever possible, but many of them were lost before they could be recorded due to the constraints of excavating as a controlled retrogressive thaw slump (Pitulko, 2008; Pitulko, 2015; Pitulko, 2019b).
The 3-D provenience data allow visualization of spatial patterns as shown in Figure 8 (see also Pitulko, 2008; Basilyan et al., 2011; Pitulko et al., 2013). Each polygon in the profile is surrounded by peaks in the distribution of artifacts indicating the distance of vertical transport of archaeological finds from their original position relative to the concentrations inside the polygon perimeter. In theory, some artifacts and animal bones may have been transported to the upper surface of the ice wedge, or practically to the STL base (Figures 11A,B). Hence, they might end up in a hypsometric position that has no relation to their true geological age. Such cryoturbation occurs due to the continuous pressure of the growing ice wedges on a once intact surface, which is subdivided into separate polygons exposed by the excavations at Yana NP and Yana B. The same pattern of upward movement of material also is noted for the second generation of ice wedges, although the vertical distance of transport is comparatively modest (and proportional to the reduced dimensions of the ice wedges).
The Yana B locality exhibits a similar pattern of cryoturbation, but also illustrates its further development caused by thawing of the deposits. At present, this area of the Yana site complex is associated with a riverbank slope that has been subject to massive thermoerosion. Hence, the slope is largely transformed by a retrogressive thaw slump leading to the formation of thermokarst mounds (baydzharakhs) outlined by a polygon trough network (Figure 3A). After the ice wedges melted, the troughs were filled with younger sediment.
Archaeological material (artifacts and animal bones) was displaced upward by as much as 6–7 m, due to the development of ice wedges, and redeposited in a secondary context by the infilling of thawed ice-wedge troughs (Figures 12A,B). There is no alternative source for these secondary concentrations than the primary culture-bearing horizon in the central portion of T2, 7–8 m below the modern surface. Such concentrations are related to ice wedge casts during their initial formation; further development of these features may lead to full transformation of the primary in situ context to a secondary concentration of archaeological material similar to that investigated at the Zhokhov site in the New Siberian islands (see, e.g., Pitul’ko, 1993; Pitulko et al., 2015b).
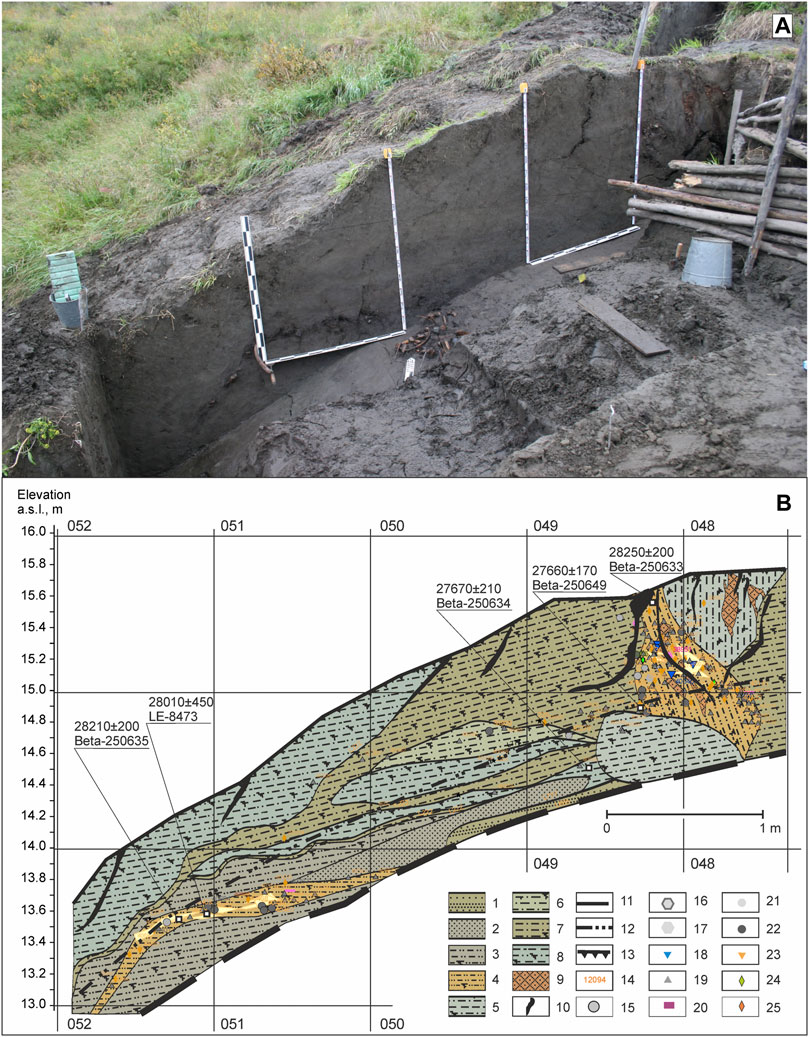
FIGURE 12. Yana site complex, Yana B area, testing trench on the river bank slope at the Dvoinoy polygon unit (A) and lithology sequence observed in the NE excavation wall at grid line Q6F052 - Q6F049 (B). Profile key: 1—unsorted sand of brownish-gray color; 2—fine-grained sand of gray color; 3—sandy silt of gray color; 4—sandy silt of brown color; 5—silt of gray color; 6—sandy-clayey silt of brownish-gray color; 8—clayey silt of light-gray color; 9—peat lenses; 10—drying fissure; 11—profile bottom; 12—STL boundary; 13—profile edge; 14—artifact number; 15—lithic side scraper; 16—core; 17—blank; 18—lithic tool; 19—flake; 20—blade; 21—pebble; 22—burnt rock; 23—bone tool; 24—mammoth ivory fragment; 25—fauna remains. After (Pitulko, 2019a).
Discussion
Within the larger context of Quaternary science, the properties of ice-rich permafrost, or Ice Complex deposits, have important implications for geology, geoarchaeology, and archaeology. Although the cryogenic processes described here are well known, their study is enhanced by insights derived from archaeological excavations in ice-rich permafrost, and geological observations made during the excavations, as concentrations of in situ cultural material serve as an excellent stratigraphic marker. The observations presented above were made over the course of many years of geoarchaeological investigation at the Yana site complex and they may be applied to Quaternary geology. By region, these observations should be applied to East Siberia/Western Beringia first of all; however, they can be extrapolated to any territory of the Northern Hemisphere where syngenetic permafrost deposits are present today or where there is evidence of their presence in the past, that is, mostly Northern Eurasia (see, e.g., Velichko, 1973; Velichko et al., 1997; Vandenberghe et al., 2014).
The most obvious cases are associated with the well-known, generally low resistance of thaw-sensitive Ice Complex deposits to changes in heating and moisture regimes that result in permafrost thawing, which includes thermal erosion and denudation, subsidence of the ground, and a variety of thermokarst processes. In stratigraphic record, they are represented by thaw unconformities and ice-wedge casts. Permafrost melting also entails thaw consolidation of the deposits, resulting in reduced ice component.
It is widely known that thermal erosion generates high rates of riverbank erosion (Kanevskiy et al., 2016; Fuchs et al., 2020; Wetterich et al., 2020; Morgenstern et al., 2021) and the erosion of marine shorelines (Are, 1980; Romanovskii et al., 2004; Günther et al., 2013; Overduin et al., 2016). The process often leads to an enormous loss of ice-rich deposits, followed by accelerated erosion, which involves collapse of frozen blocks undercut by running water, forming erosional niches due to combined thermal and mechanical action. Thus, the riverbank retreats several dozen meters per year. For example, Kanevskiy et al. (2016) calculated an average shoreline retreat of 45.4 m/yr on the Itkilik River, Alaska, with a maximum of 81.1 m/yr recorded at one bank/frozen cliff segment.
Such processes may be observed in the vicinity of the Yana site complex as well (see above) (Figures 4, 13A). Although the erosional rate is slower than that on the Itkilik River, it may reach 20–30 m/yr. Relatively large collapsed blocks may be fully eroded. However, under certain conditions they can be rapidly buried in riverine sediment and incorporated into a new stratigraphic sequence. This phenomenon also may have taken place in the past. It should be stressed that such blocks (Figure 13B) may be buried in a frozen state and contain much information including archaeological finds and even fully datable features containing artifacts and faunal remains. Only the sediment block as a whole may be out of context in its new location (Figures 14A,B). If this is not recognized, the hypsometric position and age of the context may lead to erroneous assumptions, conclusions, and interpretations, which often have occurred in East Siberian Stone Age archaeology (see Pitulko and Pavlova (2016) for details).
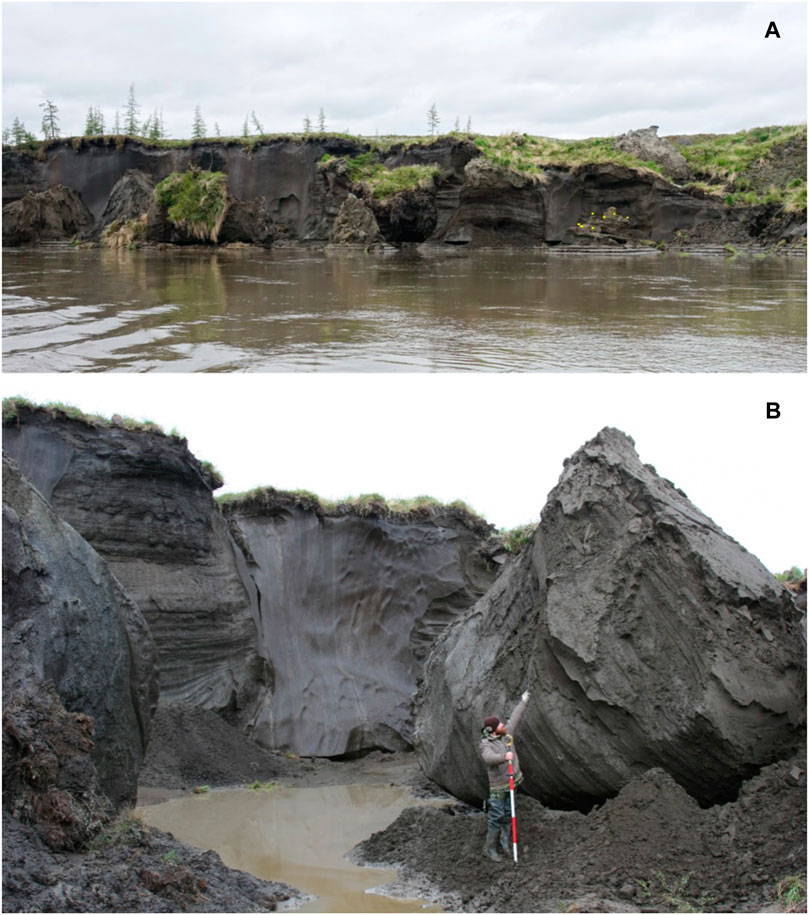
FIGURE 13. Thermal erosion of the area in the Northern Point area of the Yana site complex: (A) - block collapse at the left bank of the Yana River due to continuously high water stand (collapse zone in between Northern Point and Area B of the Yana site; yellow flags seen in the lower right corner mark the western border of the excavation area); (B) - a cultural layer incorporated in the collapsed blocks, its position is indicated by I.G. Kovaltsov (excavations of 2016). Photo: E.Y. Pavlova.
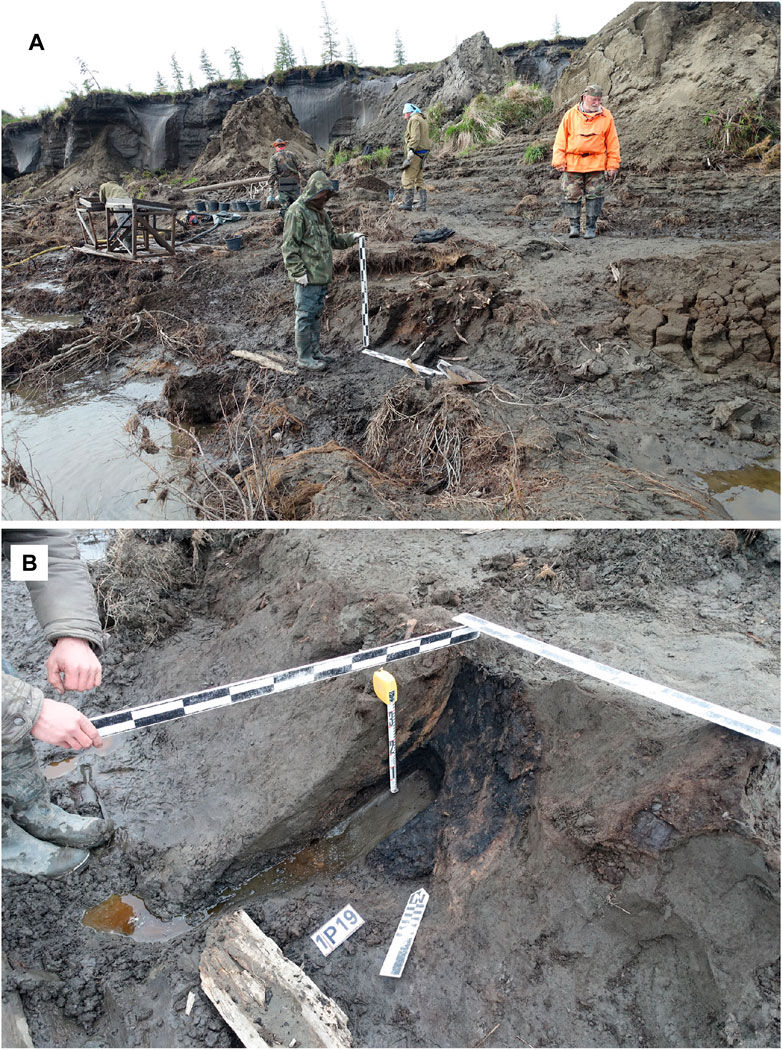
FIGURE 14. Yana site complex, excavations at the Northern Point area. Excavations of frozen deposits from a collapsed block containing a portion of the cultural layer (A) and a hearth structure discovered in this block remnant (B). Photo: Elena Pavlova.
Some cryogenic processes, such as solifluction and landslides, provide an analog to the previously described case. However, the contribution of slope processes to the transformation of culture-bearing deposits can be significantly greater. An impressive example is found at Afontova Gora in Krasnoyarsk, southern Siberia. Despite a hundred years of research, the geology of the site has remained controversial for decades (see review in Astakhov (1999)), inhibiting interpretation of the archaeology. Based on a detailed study of the deposits uncovered in the sections of Afontova, Zolnikov et al. (2017) demonstrated that site formation was a result of a viscoplastic flow of thawed, waterlogged loamy silt deposits containing cultural remains, including massive sediment blocks. It may be regarded as thaw slumping, combining a variety of slope processes, both water-derived and gravitational, that can occur anywhere in ice-rich permafrost regions.
The most noticeable impact on the landscape of thawing frozen deposits is slope (defluction, cryogenic creep) and solifluction processes. Although similar, these processes differ in the degree of water saturation, slope angle, and the rate of sediment transport. Solifluctional and other slope processes can contribute to the formation of a secondary archaeologic context at the base of the slope, as well as in secondary transformations of the cultural horizon in situ. An example is known from the Paleo-Eskimo Tayara Site on Qikirtaq Island, Canadian Arctic. When the site formed, cultural remains became buried in deposits resulting from the solifluction of reworked glacial-marine deposits. This process buried the northern part of the site. The rate of movement of the soliflucted deposit is estimated at roughly 1.68–2.86 cm/yr for approximately 350 years. As a result, three successive “cultural horizons” were formed (Todisco and Bhiry, 2008). Remarkably, this postdepositional process did not alter the spatial distribution of the material associated with human activities, as confirmed by statistical analysis of stone artifact concentrations. At the same time, researchers found that the orientation of bone was modified in part due to the impact of low-energy surface runoff (Todisco et al., 2009). Any natural concentration of fossils (e.g., animal bones, insect and plant fossils, pollen grains) may be reworked in the same way.
In arctic Western Beringia, many sites have been affected by cryogenic processes. The Urez-22 site in the western Yana-Indighirka lowland north-east of the Yana River provides a good example of sequential redepositing due to thermal denudation and slope processes (Pitulko et al., 2016). This small site has been subject to several stages of disturbance since it was originally formed approximately 11,000 years ago. At present, most of the cultural material is found adjacent to the steep slope of a gorge-like depression containing a small stream. Its original setting, however, was on the surface of the watershed. Shortly thereafter, it was redeposited into a thermokarst lake and then gradually transported down slope. As a result, “tertiary” concentrations formed. The same process was attributed by Nikolskiy et al. (2010) for the mass concentration of mammoth remains at Achchaghyi-Allaikha; most probably, the concentration also was human-induced, as it was at Urez-22, Nikita Lake, and other sites (Pitulko et al., 2016; Pitulko et al., 2017; Pitulko V., 2019).
The impact of slope processes on culture-bearing deposits can be significant even without evidence for redeposition, as is evident at such Palaeolithic sites of the Russian Plain as Kostenki (Holliday et al., 2007), Khotylevo (Gavrilov, 2015), and Zaraysk (Amirkhanov et al., 2009), where the culture-bearing deposits were probably disturbed by cryogenic processes. Today there is no permafrost at or near these sites, but it was present during the LGM. For instance, Zaraisk lacks a permafrost polygonal pattern, but there are other, local postdepositional processes contributed to disturbance of the culture-bearing deposits.
Despite a slow rate of transport, measuring only mm/yr, frost creep may have significant effects on cultural horizons, specifically with respect to the vertical distribution of the material. The apparent stratigraphic position of the material may be altered, as the deposit is transported without loss of structural integrity. If locally developed cracks open slowly and are gradually filled with the sedimentary matrix of the cultural horizon, they may form what appear to be cultural features in the form of pits, similar to those found at Zaraysk (Amirkhanov et al., 2009). The fill would contain concentrations of occupation debris, such as ash, charcoal, artifacts, and bones, redeposited in the cracks at a roughly 90° angle to the cultural horizon (indicating the natural origin of the feature as a result of frost creep).
Ice-wedge casts formed due to permafrost thawing are the most spectacular features in cryostratigraphy; this is one of the widely accepted criteria for identifying past permafrost development (Washburn, 1979; 1980). The sediment fill in the trough formed by the thawing of an ice wedge may contain a variety of paleobiotic materials redeposited in the ice-wedge cast. In archaeology, this may result in the formation of a secondary context, sometimes impressive because of the presence of mammoth bones and tusks. Ancient humans are often thought to be tireless builders of mammoth bone dwelling structures. Under certain circumstances, especially in the former permafrost areas, similar concentrations of mammoth bones and tusks may be mis-interpreted by researchers as in situ materials and evidence for human-made constructions, with other conclusions on related human activity and behavior.
A misunderstanding of the processes that underlie the formation of secondary concentrations in ice wedge casts may lead to misconceptions and erroneous interpretations of the cultural material. A good example is the subrectangular “blindage-like” features interpreted as semi-subterranean dwelling structures at the Upper Palaeolithic Timonovka sites on the Russian Plain (Gorodtsov, 1935; Krainov, 1956). As demonstarted by Velichko et al. (1977), the blindage-like structures are in fact large ice-wedge casts mistakenly attributed by Gorodtsov to human activity. In the ice-wedge cast fill, there was archaeological material from the overlying culture-bearing horizon which remained in situ, except for minor changes related to the thaw consolidation of the deposits. Cryogenic deformations of the culture-bearing deposits related to the formation of ice-wedge casts after the LGM are noted also at Eliseevichi and at other archaeological sites of the Russian Plain (Velichko et al., 1997) within the limits of the former permafrost zone (Vandenberghe et al., 2014). In theory, they should be common within the area.
At the Yana site complex, an example of secondary concentrations of archaeological material in ice-wedge casts is found at the Yana B locality. Such concentrations, or secondary contexts, which formed when artifacts and bones were uplifted up by the pressure resulting from ice-wedge growth, may be seen in the geological profiles (Figure 12). If refrozen, such concentrations might be mistakenly regarded as in the primary context, leading to erroneous interpretations. In the case of Yana B, for instance, the cultural layer could be interpreted as three separate horizons at different hypsometric levels, subdivided by thick artifact-free deposits.
As the ice wedges in the study area remain frozen, none of the studied locations at the Yana sites provides evidence for fully formed ice-wedge casts containing redeposited cultural material. By contrast, the Zhokhov site provides evidence for the formation of such features in the East Siberian record. At this site, thick refrozen artifact-bearing deposits filled in troughs formed due to massive degradation of MIS3 ice-rich permafrost during the Terminal Pleistocene and Holocene in a series of formation cycles (Pitulko et al., 2015b; Pitulko, 2019b).
Finally, we turn to the cryogenic disturbance of fossils or, in the case of the Yana sites, the cryogenic disturbance of archaeological material, as a consequence of the pressure of growing ice wedges on frozen deposits within the polygons (Pitulko et al., 2011). During this process, the uppermost portion of the ice wedge grows upward in concert with the increasing thickness of the sediment. It should be emphasized that the width of the ice wedge is also increasing. As shown above (Figures 8–11), as the ice thickness increases, the archaeological material is driven up along the ice-wedge contact zone to a significant degree, in some cases, up to the base of the STL (Figures 11A,B). As a result, radiocarbon-datable materials (Pitulko and Pavlova, 2015), as well as dispersed sediment containing pollen, other microfossils, and geochemical signatures of the source horizon, are uplifted, forming a train of fossils within roughly 1 m from the ice-wedge contact. In their new hypsometric position within the profile, the fossils and artifacts are asynchronic to their sedimentary context. The same mechanism accounts for the transmission of geochemical signatures indicating goldfield deposits near the surface, detected by Victor (Pitulko, 1977) in the 1970s, and adopted as a tool for prospecting deeply buried metal-bearing deposits in the Kular goldfields of the Yana area (Pitulko et al., 1985). It was demonstrated that these characteristic geochemical signatures are transported upward by as much as 40–50 m along the ice-wedge contact zones due to ice-wedge growth.
The uplifted materials preserve in situ characteristics, despite being redeposited from the ice-wedge boundary and from the surface, and they remain frozen. Such material, if used for 14С dating, may lead to erroneous conclusions about the age of the deposits, for example, indicating 28,000 years BP for a level that may be only half that age (Figures 5B, 9A,B, 10, 11). A series of dates obtained on such material at different levels along the ice wedge contact zone will yield ages similar to those obtained on the sediments overlying the source horizon. Such age estimates would be correct for the source horizon, but not for the overlying sediments, as the dated material is asynchronous to sedimentation.
In the Yana site profiles, due to the high density of observable archaeological material in the source horizon, it is relatively easy to avoid such sampling errors. However, it should be kept in mind that the cryogenic disturbance processes described above are common in permafrost areas with polygonal ice wedges. The observations made here may be applied to isolated fossils found in ice-wedge permafrost. In Quaternary geology, this is a common source of dating errors in thawed permafrost deposits, specifically in the former permafrost zone.
Cryogenic disturbance processes may underlie another source of errors in the interpretation of geology and age of the cultural remains and mistakes in the interpretation of past human behavior. This also is illustrated by the excavations at the Yana sites. We observed rich concentrations of cultural material confined to the upper portions of thermokarst mounds studied during test excavations at Yana B in 2003 and 2004 (Figures 15A,B). The concentrations of material exhibited a peculiar spatial pattern: the archaeological materials displayed a ring-like structure parallel to the margins of the thermokarst mound. Cultural material was absent in the center of the mounds but common within an approximately 1 m-wide zone adjoining the margins of the mound.
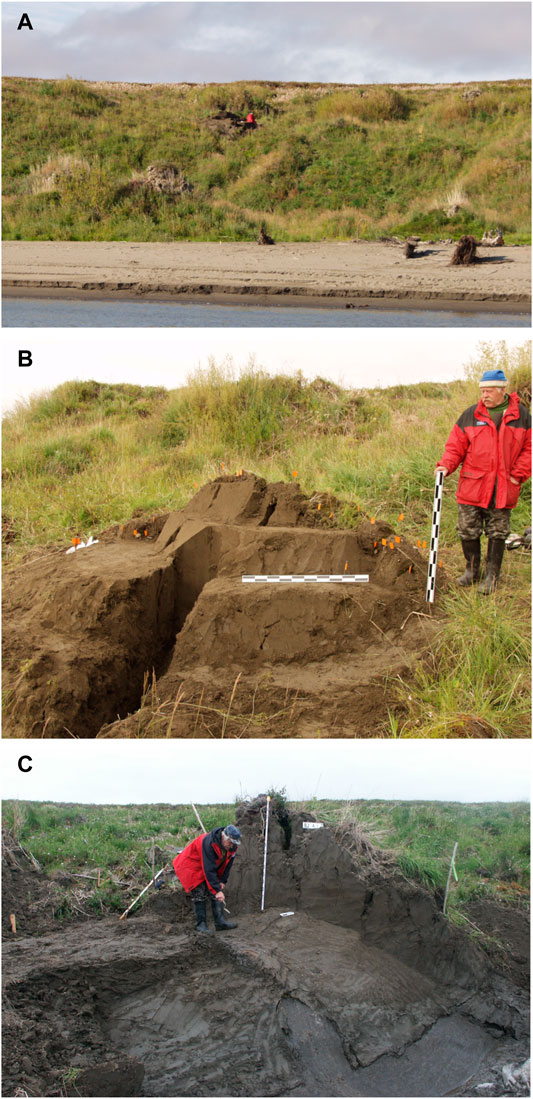
FIGURE 15. Testing excavations in the Yana B area in 2003 and 2004: (A) left bank of the Yana River near the Yana B area of the Yana site complex in 2004 viewed from the South; (B) – excavated upper part of the thermokarst mound with faunistic and lithic finds marked by the flags making a circle, view from the South; (C) excavated part of the thermokarst mound after finds taken away, view from the South-West; it appears to be artifact-free deposit while in fact there is a cultural layer in 7 m depth.
Although the source of the spatial pattern initially was unclear, it became apparent when it was observed that in the upper part of the Yana site geological sequence, the layer containing artifacts often displays a reverse dip. When the uppermost deposits of the polygon containing uplifted faunal remains and artifacts slumped in the course of thawing, a ring-like spatial pattern in the distribution of the material formed (Figures 15B,C). The archaeological material is represented primarily by bone fragments with a mean size class of 10–15 cm. Such spatial patterns appear when frozen deposits begin to thaw from the surface down to subhorizontal features. Although entirely a result of cryogenic processes, the spatial patterns acquire a characteristic signature in a cultural deposit. However, in our case, it is 5–5.5 m above the original cultural context from which the artifacts and bones are derived, as is shown by the age of 28,000 years BP obtained from the source horizon. The patterns reflect a complex interplay of cryogenic processes and archaeological site formation, and it would be a mistake to interpret them as entirely the product of human occupation.
Another feature observed in the Yana Ice Complex relates to bedded and warped pattern of the ice-wedge polygon deposits, which contain peat inclusions, concentrations of plant remains, and the cultural horizons. Alternating ice streaks and sediment layers are warped near the ice-wedges bordering the polygons. The pattern is found at many places bearing MIS3 Ice Complex deposits across arctic East Siberia, including the Yana River (see, e.g., Tomirdiaro and Chernenkiy, 1987; Schirrmeister et al., 2011; 2013; Wetterich et al., 2014; Murton et al., 2015). In the past, it was assumed that the pattern arose from the former microtopography of the polygons, including polygon ponds, in which layered peat/plant remains were deposited, creating the low central depression of the polygons (Romanovskii, 1977; see also Schirrmeister et al., 2013; Wetterich et al., 2014 for overview).
The development of the polygons is thought to be a stepwise transformation of the deposits from seasonally unfrozen to perennially frozen, taking place under climate conditions that also effect changes in the sedimentation regime between aeolian and shallow stream settings (see Vasil’chuk, 2006; 2013; Wetterich et al., 2014). This approach, which is based on actualistic observations, is tied to a hypothesis of a uniform origin of Ice Complex deposits, but there exist various alternative hypotheses [for an overview, see Schirrmeister et al. (2011; 2013)].
Although a general discussion of Ice Complex origins is outside our scope, we note that the polygenetic genesis of the Ice Complex deposits proposed by Sher (1997) is the most compelling one from our perspective, based on our research at the Yana site complex. The concept of polygenetic genesis suggests an accumulation of Ice Complex deposits under various sedimentation regimes, controlled by similar landscape and topography, climate conditions, and periglacial processes. In the Yana area, the Ice Complex deposits of T2 are clearly linked to alluvial deposition, with a succession of changes in the sedimentation environment beginning with alluvial sedimentation gradually changing to subaerial, followed by a return to subaquatic accumulation, which is superseded by a final shift to aeolian deposition before 13,000 years ago. Additionally, in the Yana site complex we have an ideal chrono-stratigraphic marker: an intensively occupied human habitation surface with hundreds of thousands of artifacts and bones, and former hearths.
This culture-bearing horizon, as well as other ice-rich sediments and ice layers present in the profiles, demonstrate the hallmark pattern of the Ice Complex deposits; all of them are warped in contact with the ice wedges, with artifacts, animal bones, and traces of hearths incorporated into the warped layer. The layers exhibit varying angles of warping: the deeper the layer, the larger the angle of warping. The pattern is a consistent one and can be observed throughout the Yana site complex. The culture-bearing horizon displays a pronounced warp at an angle of almost 90° within ∼1 m distance to the ice wedge that may be traced with cultural materials, former hearths, and sedimentary matrix. This suggests that polygonal patterns were not present at the time of occupation, including either low- or high-centered permafrost polygons. A polygonal topography would be incompatible with human habitation. The surface was level at that time, well drained and dry year-round, probably except for occasional flooding events. The warping of the cultural layer took place after abandonment of the site, which is evident in the uplifted margins of the former hearths. The cultural horizon subsequently was buried by river sediments and later became incorporated into the sequence of permafrost deposits of T2, which remain frozen today.
Ice wedges of the oldest generation, formed about 40,000 years ago, continued to grow, resulting in compression of the sediment and causing warping and uplifting. At the time of human habitation, the ice wedges were relatively thin. When the initial frost fissures occurred 40,000 years ago, they were approximately 12 m apart from each other with a diameter of approximately 10–12 m. At present, they are only 5–6 m at the hypsometric position of the culture-bearing horizon. The deposits did not disappear but were displaced by growing ice wedges and partially consolidated, but also partially uplifted and warped. The latter was a function of time in the upper part of the MIS3 sequence of T2.
The continuous growth of ice wedges resulted in the compression of the deposits within the polygons, warping and uplifting of some of the deposits with implications for the rate of accumulation of the Ice Complex. Because uplifted deposits do not disappear but remain in place, they contribute to the increased thickness of the deposits, in conjunction with the continued accumulation of surficial sediment. The increased thickness of Ice Complex deposits within the depositional cycle, thus, is not a function of sedimentation alone, but a combined result of sedimentation and redistribution of existing polygonal deposits. Also, there is positive feedback between ice-wedge growth and increased polygonal sediment thickness: the uplifted deposits result in increased thickness, which promotes further ice-wedge growth. Finally, the frost-related pressure forming the warped pattern of the deposits may account for the formation of a low-centered polygonal pattern on the surface. This explanatory model may be incorporated into the classic theory of Ice Complex development.
It is difficult to determine, whether or not, and to what degree, these observations can be extrapolated to other areas, but they should be taken into account and further explored in other regions of arctic East Siberia. Thus, it is unclear whether peat inclusions can be included in this discussion because they likely reflect the “thaw-lake cycle” hypothesized by Ellis et al. (2008) to explain the formation of arctic wetlands, or the formation of the thermokarst terrain in a broader sense. However, paleosol horizons known in Ice Complex deposits (Gubin, 1994; Lupachev and Gubin, 2008; Zanina et al., 2011) most probably can be regarded as indications of a formerly level surface based on the study of paleosol horizons in the Yana area (Bakina et al., 2017). The current theory of Ice Complex formation may be revised by postulating 1) initial sediment accumulation on level terrain under various depositional regimes, 2) later modified by ice-wedge growth, 3) resulting in warping and uplifting of deposits in contact with ice wedges, as inferred from archaeological investigations at the Yana site complex.
Conclusion
The properties of ice-rich deposits generate a variety of potential taphonomic biases. Most of them have implications for dating the deposits. Generally, such biases are related to the thawing of frozen deposits, which results in the formation of secondary contexts at various scales, which include ice-wedge casts containing thawed material. Such secondary depositional contexts as result of thermokarst processes are found in a lower-lying position than the original hypsometric position. Collapsed blocks of frozen deposits may contain intact fragments of frozen layers with artifacts and/or other fossils and may be incorporated into a new stratigraphic context and subsequently refrozen. If not recognized as such, this can result in erroneous age estimates and overall interpretation of the sequence.
Severe cryoturbation occurs in Ice Complex deposits within the polygons, resulting in warping of the layers in contact with the ice wedge. This process is primarily responsible for the distorted pattern of Ice Complex deposits. The warping of layers is due to the pressure generated by growth of the ice wedges on the polygon structure. As a result, the materials contained in the warped layer are displaced upward, sometimes to a significant degree.
The consequences include the following: 1) chronometry problems, because datable material can be transposed to a different hypsometric position; 2) problems of interpretation in archaeology with respect to dating, culture, and human behavior; 3) material redeposited this way may form secondary concentrations at different hypsometric levels and thus become a basis for multiple misinterpretations (see above); 4) in geology, the transportation of geochemical signatures may lead to erroneous interpretation of the geological potential of the area; 5) uplifted deposits contribute to increased Ice Complex thickness, which is not a direct function of sediment accumulation, but a combined result of sedimentation and redistribution of accumulated matter within an existing polygon deposit.
It should be stressed that these observations can be made only in conjunction with archaeological investigations, which provide an excellent stratigraphic context and a detailed reconstruction of the ancient living surface. We suggest that outstanding questions about Ice Complex deposits can be addressed with the findings presented here. In any case, the results demonstrate the potential of a “geoarchaeological” approach, which therefore deserves further study.
Data Availability Statement
The raw data supporting the conclusions of this article will be made available by the authors, without undue reservation.
Ethics Statement
Written informed consent was obtained from the relevant individual(s) for the publication of any potentially identifiable images or data included in this article.
Author Contributions
Authors have contributed equally to the project both in the fieldwork by data collecting and in the preparation of the manuscript. VP leads the Yana excavation project and designed the present study. Field observations are recorded by EP who also participated in writing of the text and designed all illustrations.
Funding
Authors are more than grateful to the Rock Foundation of New York, U.S., for unlimited support given to Zhokhov/Yana project since 2000 and to the present study. Since 2014, the Yana excavations remain a part of research funded by the Russian Science Foundation (project nos. 16-18-10265P RNF and 21-18-00457 RNF). Unlimited thanks due to all participants of the fieldwork in Yana for their endless patience, labour, and intellectual investment into investigations of Yana’s ice-rich permafrost. Written informed consent was obtained from the Yana project participants S. O. Remizov, I. A. Spiridonov, S. G. Kritsuk, P. I. Pulmanen, VP, S. N. Astakhov, N. O. Chetverikov, E. A. Pushkov, I. G. Kovaltsov, I. V. Chasnyk, M. D. Kupriyanova, A. P. Shubenkin, and A. O. Mashezerskaya for the publication of any potentially identifiable images or data included in this article.
Dedication
We dedicate this article to the memory of Victor M. Pitulko, Vladimir’s father — outstanding scholar, geologist, and explorer of East Siberia.
Conflict of Interest
The authors declare that the research was conducted in the absence of any commercial or financial relationships that could be construed as a potential conflict of interest.
Publisher’s Note
All claims expressed in this article are solely those of the authors and do not necessarily represent those of their affiliated organizations, or those of the publisher, the editors and the reviewers. Any product that may be evaluated in this article, or claim that may be made by its manufacturer, is not guaranteed or endorsed by the publisher.
Acknowledgments
Author’s special thanks due to John F. Hoffecker for kindly provided assistance with the editing of the final version of the article. Finally, we want to thank LS for encouraging us to produce this article, and two reviewers whose valuable comments helped to improve its quality.
References
Amirkhanov, K. A., Akhmetgaleeva, N. B., Buzhilova, A. P., Burova, N. D., Lev, S. I., and Mashchenko, E. N. (2009). Investigation of the Paleolithic in Zaraisk. 1999–2005. Moscow: Paleograf, 466.
Andreev, A. A., Schirrmeister, L., Tarasov, P. E., Ganopolski, A., Brovkin, V., Siegert, C., et al. (2011). Vegetation and Climate History in the Laptev Sea Region (Arctic Siberia) during Late Quaternary Inferred from Pollen Records. Quat. Sci. Rev. 30, 2182–2199. doi:10.1016/j.quascirev.2010.12.026
Ashastina, K., Schirrmeister, L., Fuchs, M., and Kienast, F. (2017). Palaeoclimate Characteristics in interior Siberia of MIS 6-2: First Insights from the Batagay Permafrost Mega-Thaw Slump in the Yana Highlands. Clim. Past 13, 795–818. doi:10.5194/cp-13-795-2017
Astakhov, S. N. (1999). Paleolithic of the Yenisei. The Paleolithic Sites at Afontova Gora at the City of Krasnoyarsk. St. Petersburg: Izdatel’stvo “Evropeiskii Dom”, 207.
Bakina, L. G., Pavlova, E. Y., Kritsuk, S. G., and Pitulko, V. V. (2017). “Humus Content and Fraction-Group Composition of the Ice Complex Deposits of the Yana RHS Site (Western Part of Yana-Indighirka lowland),” in Fundamental problems of the Quaternary: results and perspectives of the research. Proceeding of the X All-Russian Quaternary Conference, Moscow, September 25-29, 2017 (Moscow: GEOS), 33–34.
Basilyan, A. E., Anisimov, M. A., Nikolskiy, P. A., and Pitulko, V. V. (2011). Wooly mammoth Mass Accumulation Next to the Paleolithic Yana RHS Site, Arctic Siberia: its Geology, Age, and Relation to Past Human Activity. J. Archaeological Sci. 38 (9), 2461–2474. doi:10.1016/j.jas.2011.05.017
Basilyan, A. E., Anisimov, M. A., Pavlova, E. Y., Pitulko, V. V., and Nikolskiy, P. A. (2009). “Base Reference Section of Quaternary Deposits for Yana-Indighirka lowland in the Yana River Downstream Area,” in Fundamental Problems of Quaternary: Results and Trends of Further Research. Proceedings of the VI All-Russian Quaternary Conference, Novosibirsk, October 19–23, 2009 (Novosibirsk: SB RAS), 63–65.
CAVM Team (2003). Circumpolar Arctic Vegetation Map. Scale 1:7,500,000Conservation of Arctic Flora and Fauna (CAFF) Map No. 1. Anchorage, Alaska: U.S. Fish and Wildlife Service.
Ellis, C. J., Rochefort, L., Gauthier, G., and Pienitz, R. (2008). Paleoecological Evidence for Transitions between Contrasting Landforms in a Polygon-Patterned High Arctic Wetland. Arctic, Antarctic, Alpine Res. 40 (4), 624–637. doi:10.1657/1523-0430(07-059)[ellis]2.0.co;2
Froese, D. G., Zazula, G. D., Westgate, J. A., Preece, S. J., Sanborn, P. T., Reyes, A. V., et al. (2009). The Klondike Goldfields and Pleistocene Environments of Beringia. GSA Today 19 (8), 4–10. doi:10.1130/gsatg54a.1
Fuchs, M., Nitze, I., Strauss, J., Günther, F., Wetterich, S., Kizyakov, A., et al. (2020). Rapid Fluvio-Thermal Erosion of a Yedoma Permafrost Cliff in the Lena River Delta. Front. Earth Sci. 8, 336. doi:10.3389/feart.2020.00336
Gakkel, Y. Y., and Korotkevich, E. S. (1960). Severnaya Yakutiya (Fiziko-geograficheskaya Kharakteristika [Northern Yakutia (Phisycal Geography)]. Leningrad: Morskoy transport, 280. (In Russian).
Gavrilov, A. V., Romanovskii, N. N., and Hubberten, H.-W. (2006). Paleogeographic Scenario of the Postglacial Transgression on the Laptev Sea Shelf. Earth Cryosphere 10, 39–50.
Gavrilov, K. N. (2015). “The Archaeological Context of the New Radio-Carbon Datings of the Khotylevo 2 - point B (V) Site,” in Ancient Cultures of the Eastern Europe: Important Sites and Reference Complexes in the Context of Modern Archaeological Research. Editor G. A. Khlopachev (St. Petersburg: Peter the Great Museum), 103–112.
Gorodtsov, V. A. (1935). Timonovskaya Palaeolithic Site. Results of Archaeological Excavations of 1933. Moscow and Leningrad: Izdatelstvo AN SSSR Publ., 35. (in Russian).
Gubin, S. V. (1994). Pozdnepleistotsenovoe Pochvoobrazovanie Na Primorskikh Nizmenostyakh Severa Yakutii (Late Pleistocene Pedogenesis in Coastal Lowlands of Northern Yakutia). Pochvovedenie (Soil Sci.) 8, 5–14. (in Russian).
Günther, F., Overduin, P. P., Sandakov, A. V., Grosse, G., and Grigoriev, M. N. (2013). Short- and Long-Term Thermo-Erosion of Ice-Rich Permafrost Coasts in the Laptev Sea Region. Biogeosciences 10, 4297–4318. doi:10.5194/bg-10-4297-2013
Holliday, V. T., Hoffecker, J. F., Goldberg, P., Macphail, R. I., Forman, S. L., Anikovich, M., et al. (2007). Geoarchaeology of the Kostenki-Borshchevo Sites, Don River valley, Russia. Geoarchaeology 22, 181–228. doi:10.1002/gea.20163
Izyumenko, S. A. (1966). Spravochnik Po Klimatu SSSR. Vypusk 24. Yakutskaya ASSR. Chast II. Temperatura Vozdukha I Pochvy [Handbook on the Climate of the USSR. Issue 24. Yakut ASSR. Part II. Air and Soil Temperatures]. Leningrad: Gidrometeoizdat. (In Russian).
Izyumenko, S. A. (1968). Spravochnik Po Klimatu SSSR. Vypusk 24. Yakutskaya ASSR. Chast IV. Vlaznost Vozdukha, Atmosfernye Osadki I Snezhnyi Pokrov [Handbook on the Climate of the USSR. Issue 24. Yakut ASSR. Part IV. Atmospheric Moisture, Precipitation and Snow Cover]. Leningrad: Gidrometeoizdat. (In Russian).
Jørgensen, T., Haile, J., Möller, P., Andreev, A., Boessenkool, S., Rasmussen, M., et al. (2012). A Comparative Study of Ancient Sedimentary DNA, Pollen and Macrofossils from Permafrost Sediments of Northern Siberia Reveals Long-Term Vegetational Stability. Mol. Ecol. 21, 1989–2003. doi:10.1111/j.1365-294X.2011.05287.x
Kanevskiy, M., Shur, Y., Fortier, D., Jorgenson, M. T., and Stephani, E. (2011). Cryostratigraphy of Late Pleistocene Syngenetic Permafrost (Yedoma) in Northern Alaska, Itkillik River Exposure. Quat. Res. 75, 584–596. doi:10.1016/j.yqres.2010.12.003
Kanevskiy, M., Shur, Y., Strauss, J., Jorgenson, T., Fortier, D., Stephani, E., et al. (2016). Patterns and Rates of riverbank Erosion Involving Ice-Rich Permafrost (Yedoma) in Northern Alaska. Geomorphology 253, 370–384. doi:10.1016/j.geomorph.2015.10.023
Kaplina, T. N. (1981). “Late Cenozoic Development of Frozen Deposits in Northern Yakutia,” in Permafrost Development in Eurasia (Based on Examples of Specific Regions). Editors G. D. Dubikov, and V. V. Baulin (Moscow: Nauka), 153–181.
Katasonov, E. M. (1979). Stroenie I Absolyutnaya Geokhronologiya Alasnykh Otlozhenii Tsentral’noi Yakutii [Structure and Absolute Geochronology of Alassy Deposits of Central Yakutia]. Novosibirsk: Nauka. (In Russian).
Kienast, F., Schirrmeister, L., Siegert, C., and Tarasov, P. (2005). Palaeobotanical Evidence for Warm Summers in the East Siberian Arctic during the Last Cold Stage. Quat. Res. 63, 283–300. doi:10.1016/j.yqres.2005.01.003
Krainov, D. A. (1956). Dwellings of Timonovskaya Palaeolithic Site (Based on the Excavations of V.A. Gorodtsov). Soviet Archaeology XXV 1, 13–34. (in Russian).
Lee, E. J., Merriwether, D. A., Kasparov, A. K., Khartanovich, V. I., Nikolskiy, P. A., Shidlovskiy, F. K., et al. (2018). A Genetic Perspective of Prehistoric hunter-gatherers in the Siberian Arctic: Mitochondrial DNA Analysis of Human Remains from 8000 Years Ago. J. Archaeological Sci. Rep. 17, 943–949. doi:10.1016/j.jasrep.2016.06.001
Lupachev, A., and Gubin, S. V. (2008). Role of Pedogenesis in the Formation of Permafrost Transition Layer Structure (In Russian). Earth’ Cryosphere 12, 75–83.
Makeyev, V. M., Ponomareva, D. P., Pitulko, V. V., Chernova, G. M., and Solovyeva, D. V. (2003). Vegetation and Climate of New Siberian Islands for the Past 15 000 Years. Arctic, Antarctic, Alpine Res. 35, 28–35. doi:10.1657/1523-0430(2003)035[0056:vacotn]2.0.co;2
Morgenstern, A., Overduin, P. P., Günther, F., Stettner, S., Ramage, J., Schirrmeister, L., et al. (2021). Thermo-erosional Valleys in Siberian Ice-Rich Permafrost. Permafrost and Periglac Process 32, 59–75. doi:10.1002/ppp.2087
Morgenstern, A., Ulrich, M., Günther, F., Roessler, S., Fedorova, I. V., Rudaya, N. A., et al. (2013). Evolution of Thermokarst in East Siberian Ice-Rich Permafrost: A Case Study. Geomorphology 201, 363–379. doi:10.1016/j.geomorph.2013.07.011
Murton, J. B., Goslar, T., Edwards, M. E., Bateman, M. D., Danilov, P. P., Savvinov, G. N., et al. (2015). Palaeoenvironmental Interpretation of Yedoma Silt (Ice Complex) Deposition as Cold-Climate Loess, Duvanny Yar, Northeast Siberia. Permafrost Periglac. Process. 26, 208–288. doi:10.1002/ppp.1843
Nekrasov, I. A., and Devyatkin, V. N. (1974). Morphology of the Criolithozone of the Yana River basin and Adjacent Regions. Novosibirsk: Nauka, 73.
Nikolskiy, P. A., Basilyan, A. E., Sulerzhitsky, L. D., and Pitulko, V. V. (2010). Prelude to the Extinction: Revision of the Achchagyi-Allaikha and Berelyokh Mass Accumulations of mammoth. Quat. Int. 219, 16–25. doi:10.1016/j.quaint.2009.10.028
Nikolskiy, P. A., Sulerzhitsky, L. D., and Pitulko, V. V. (2011). Last Straw versus Blitzkrieg Overkill: Climate-Driven Changes in the Arctic Siberian mammoth Population and the Late Pleistocene Extinction Problem. Quat. Sci. Rev. 30, 2309–2328. doi:10.1016/j.quascirev.2010.10.017
Nikolskiy, P., and Pitulko, V. (2013). Evidence from the Yana Palaeolithic Site, Arctic Siberia, Yields Clues to the riddle of mammoth Hunting. J. Archaeological Sci. 40, 4189–4197. doi:10.1016/j.jas.2013.05.020
Opel, T., Murton, J. B., Wetterich, S., Meyer, H., Ashastina, K., Günther, F., et al. (2019). Past Climate and Continentality Inferred from Ice Wedges at Batagay Megaslump in the Northern Hemisphere's Most continental Region, Yana Highlands, interior Yakutia. Clim. Past 15, 1443–1461. doi:10.1594/PANGAEA.90410510.5194/cp-15-1443-2019
Overduin, P. P., Wetterich, S., Günther, F., Grigoriev, M. N., Grosse, G., Schirrmeister, L., et al. (2016). Coastal Dynamics and Submarine Permafrost in Shallow Water of the central Laptev Sea, East Siberia. The Cryosphere 10, 1449–1462. doi:10.5194/tc-10-1449-2016
Pavlova, E. Y., and Pitulko, V. V. (2020). Late Pleistocene and Early Holocene Climate Changes and Human Habitation in the Arctic Western Beringia Based on Revision of Palaeobotanical Data. Quat. Int. 549, 5–25. doi:10.1016/j.quaint.2020.04.015
Pitulko, V. (2015). “Digging through Permafrost in Siberia,” in Field Archaeology from Around the World. Ideas and Approaches. Editors M. Carver, B. Gaydarska, and S. Monton-Subias (Springer International Publishing Switzerland), 111–113. doi:10.1007/978-3-319-09819-7_16
Pitulko, V. (2019c). “In Pursuit of the Time: Searching for the Initial Human Settlement of the Siberian Arctic,” in The Past of Humankind as Seen by the Petersburg Archaeologists at the Dawn of the Millenium (To the Centennial of the Russian Academic Archaeology). Editors Y. A. Vinogradov, S. A. Vasiliev, and K. N. Stepanova (St. Petersburg: St. Petersburg: Centre for Oriental Studies Publishers), 103–136. doi:10.31600/978-5-85803-525-1-103-136
Pitulko, V. M., Reznikov, I. N., and Ulyanov, N. K. (1985). Lithochemical Prospecting Methods in Geology. Leningrad: Nedra, 199.
Pitulko, V., Nikolskiy, P., Basilyan, A., and Pavlova, E. (2013). “Human Habitation in the Arctic Western Beringia Prior the LGM,” in Paleoamerican Odyssey. Editors K. Graf, C. V. Ketron, and M. R. Waters (College Station, TX: Center for the Study of the First Americans, Texas A&M University Press), 13–44.
Pitulko, V., Pavlova, E., and Nikolskiy, P. (2017). Revising the Archaeological Record of the Upper Pleistocene Arctic Siberia: Human Dispersal and Adaptations in MIS 3 and 2. Quat. Sci. Rev. 165, 127–148. doi:10.1016/j.quascirev.2017.04.004
Pitul’ko, V. (2001). Terminal Pleistocene—Early Holocene Occupation in Northeast Asia and the Zhokhov Assemblage. Quat. Sci. Rev. 20, 267–275. doi:10.1016/S0277-3791(00)00117-7
Pitul’ko, V. V. (1993). An Early Holocene Site in the Siberian High Arctic. Arctic Anthropol. 30 (1), 13–21. Available at: http://www.jstor.org/stable/40316326.
Pitulko, V. V., Basilyan, A. E., and Pavlova, E. Y. (2014). The Berelekh Mammoth "Graveyard": New Chronological and Stratigraphical Data from the 2009 Field Season. Geoarchaeology 29, 277–299. doi:10.1002/gea.21483
Pitulko, V. V., Ivanova, V. V., Kasparov, A. K., and Pavlova, E. Y. (2015b). Reconstructing Prey Selection, Hunting Strategy and Seasonality of the Early Holocene Frozen Site in the Siberian High Arctic: a Case Study on the Zhokhov Site Faunal Remains, De Long Islands. Environ. Archaeology 20, 120–157. doi:10.1179/1749631414Y.0000000040
Pitulko, V. V., Nikolsky, P. A., Girya, E. Y., Basilyan, A. E., Tumskoy, V. E., Koulakov, S. A., et al. (2004). The Yana RHS Site: Humans in the Arctic before the Last Glacial Maximum. Science 303, 52–56. doi:10.1126/science.1085219
Pitulko, V. V., Pavlova, E. Y., Basilyan, A. E., and Kritsuk, S. G. (2011). “Features of Vertical Distribution of the Materials within the Marginal Zones of Permafrost Polygonal Structures and its Importance for Dating of Quaternary Deposits in Cryolitozone,” in Proceeding of the VII All-Russian Quaternary Conference. The Quaternary in all of its variety. Basic issues, results, and major trends of further research. Apatity, Russia, September 12-17, 2011. Editors O. P. Korsakova, and V. V. Kolka (Apatity and Saint Petersburg: Kola Research Center Geological Institute, RAS), 149–153.
Pitulko, V. V., Pavlova, E. Y., and Basilyan, A. E. (2016). Mass Accumulations of mammoth (mammoth 'graveyards') with Indications of Past Human Activity in the Northern Yana-Indighirka lowland, Arctic Siberia. Quat. Int. 406, 202–217. doi:10.1016/j.quaint.2015.12.039
Pitulko, V. V., and Pavlova, E. Y. (2015). Experience of Mass Radiocarbon Dating of Culture-Bearing Deposits of the Zhokhov Site. Trans. Inst. Hist. Mater. Cult. 12, 27–55.
Pitulko, V. V., and Pavlova, E. Y. (2016). Geoarchaeology and Radiocarbon Chronology of Stone Age Northeast Asia. College Station, TX: Center for the Study of the First Americans, Texas A&M University Press, 334.
Pitulko, V. V., Pavlova, E. Y., and Kritsuk, S. G. (2012). “Alluvial Complex Structure and Development Dynamics in Low-Stream Yana River valley in the Vicinity of Paleolithic Yana RHS Site,” in Proceedings of the Joint Conference “Geomorphology and Quaternary Palaeogeography of Polar Regions”, Symposium “Leopoldina” and the INQUA Peribaltic working group Workshop, Saint Petersburg, 9-17 September 2012. Editors A. Zhirov, V. Kuznetsov, D. Subetto, and J. Thiede (Saint Petersburg: Saint Petersburg State University), 313–316. (In Russian).
Pitulko, V. V., Pavlova, E. Y., Kuzmina, S. A., Nikolsky, P. A., Basilyan, A. E., Tumskoy, V. E., et al. (2007). Natural–Climatic Changes in the Yana–Indigirka Lowland during the Terminal Kargino Time and Habitat of Late Paleolithic Man in Northern Part of East Siberia. Doklady Earth Sci. 417, 1256–1260.
Pitulko, V. V., Pavlova, E. Y., and Nikolskiy, P. A. (2015a). Mammoth Ivory Technologies in the Upper Palaeolithic: a Case Study Based on the Materials from Yana RHS, Northern Yana-Indighirka lowland, Arctic Siberia. World Archaeology 47 (3), 333–389. doi:10.1080/00438243.2015.1030508
Pitulko, V. V., and Pavlova, E. Y. (2019). Upper Palaeolithic Sewing Kit from the Yana Site, Arctic Siberia. Stratum plus 1, 157–224.
Pitulko, V. V. (2019b). “Permafrost Digging,” in Encyclopedia of Global Archaeology. Editor C. Smith. 2nd edition (Cham: Springer). doi:10.1007/978-3-319-51726-110.1007/978-3-319-51726-1_1513-2
Pitulko, V. V. (2008). Principal Excavation Techniques under Permafrost Conditions (Based on Zhokhov and Yana Sites, Northern Yakutia). Archaeology, Ethnology Anthropol. Eurasia 34, 26–33. doi:10.1016/j.aeae.2008.07.003
Pitulko, V. V. (2019a). Yana B Area of the Yana Site: Some Observations Done during the Excavations of 2015 through 2018. PAJIS 1, 64–91. doi:10.31600/2658-3925-2019-1-64-91
Rasmussen, S. O., Bigler, M., Blockley, S. P., Blunier, T., Buchardt, S. L., Clausen, H. B., et al. (2014). A Stratigraphic Framework for Abrupt Climatic Changes during the Last Glacial Period Based on Three Synchronized Greenland Ice-Core Records: Refining and Extending the INTIMATE Event Stratigraphy. Quat. Sci. Rev. 106, 14–28. doi:10.1016/j.quascirev.2014.09.007
Romanovskii, N., Hubberten, H.-W., Gavrilov, A. V., Tumskoy, V. E., and Kholodov, A. L. (2004). Permafrost of the East Siberian Arctic Shelf and Coastal Lowlands. Quat. Sci. Rev. 23, 1359–1369. doi:10.1016/j.quascirev.2003.12.014
Romanovskii, N. N. (1977). Formirovanie Poligonal’no-Zhil’nykh Struktur (Formation of Polygonal-Wedge Systems). Novosibirsk: Nauka, 215. (in Russian).
Romanovskii, N. N. (1993). Fundamentals of Cryogenesis of Lithosphere. Moscow: Moscow University Press, 336. (in Russian).
Schirrmeister, L., Froese, D., Tumskoy, V., Grosse, G., and Wetterich, S. (2013). “Permafrost and Periglacial Features | Yedoma: Late Pleistocene Ice-Rich Syngenetic Permafrost of Beringia,” in The Encyclopedia of Quaternary Science. Editor S. A. Elias. second ed. (Amsterdam: Elsevier), 3, 542–552. doi:10.1016/b978-0-444-53643-3.00106-0
Schirrmeister, L., Kunitsky, V., Grosse, G., Wetterich, S., Meyer, H., Schwamborn, G., et al. (2011). Sedimentary Characteristics and Origin of the Late Pleistocene Ice Complex on north-east Siberian Arctic Coastal Lowlands and Islands - A Review. Quat. Int. 241, 3–25. doi:10.1016/j.quaint.2010.04.004
Schirrmeister, L., Schwamborn, G., Overduin, P. P., Strauss, J., Fuchs, M. C., Grigoriev, M., et al. (2017). Yedoma Ice Complex of the Buor Khaya Peninsula (Southern Laptev Sea). Biogeosciences 14, 1261–1283. doi:10.5194/bg-14-1261-2017
Sher, A. V., Kuzmina, S. A., Kuznetsova, T. V., and Sulerzhitsky, L. D. (2005). New Insights into the Weichselian Environment and Climate of the East Siberian Arctic, Derived from Fossil Insects, Plants, and Mammals. Quat. Sci. Rev. 24, 533–569. doi:10.1016/j.quascirev.2004.09.007
Sher, A. V. (1997). “Yedoma as a Store of Paleoenvironmental Records in Beringida,” in Abstracts and Program of the Beringia Paleoenvironmental Workshop, Colorado, September 20-23, 1997. Editors S. Elias, and J. Brigham-Grette (Florissant, CO), 92–94.
Shur, Y. A. (1988). Upper Stratigraphic Horizon of the Permafrost Amd Thermokarst. Novosiborsk: Nauka, 211.
Sikora, M., Pitulko, V. V., Sousa, V. C., Allentoft, M. E., Vinner, L., Rasmussen, S., et al. (2019). The Population History of Northeastern Siberia since the Pleistocene. Nature 570, 182–188. doi:10.1038/s41586-019-1279-z
Svensson, A., Andersen, K. K., Bigler, M., Clausen, H. B., Dahl-Jensen, D., Davies, S. M., et al. (2008). A 60 000 Year Greenland Stratigraphic Ice Core Chronology. Clim. Past 4, 47–57. doi:10.5194/cp-4-47-2008
Todisco, D., Bhiry, N., and Desrosiers, P. M. (2009). Paleoeskimo Site Taphonomy: An Assessment of the Integrity of the Tayara Site, Qikirtaq Island, Nunavik, Canada. Geoarchaeology 24, 743–791. doi:10.1002/gea.20285
Todisco, D., and Bhiry, N. (2008). Palaeoeskimo Site Burial by Solifluction: Periglacial Geoarchaeology of the Tayara Site (KbFk-7), Qikirtaq Island, Nunavik (Canada). Geoarchaeology 23, 177–211. doi:10.1002/gea.20217
Tomirdiaro, S. V., and Chernenkiy, B. I. (1987). Kriogenno-eolovye Otlozheniya VostochnoiArktiki I Subarktiki [Cryogenic-Aeolian Deposits of the Eastern Arctic and Subarctic]. Moscow: Nauka.
Vandenberghe, J., French, H. M., Gorbunov, A., Marchenko, S., Velichko, A. A., Jin, H., et al. (2014). The Last Permafrost Maximum (LPM) Map of the Northern Hemisphere: Permafrost Extent and Mean Annual Air Temperatures, 25-17 Ka BP. Boreas 43, 652–666. doi:10.1111/bor.12070
Vasil'chuk, Y. K. (2013). “Syngenetic Ice Wedges: Cyclical Formation, Radiocarbon Age and Stable Isotope Records by Yurij K. Vasil 'chuk, Moscow University Press, Moscow, 2006. 404 Pp. ISBN 5-211-05212 -9. Permafrost Periglac. Process., 24, 82–93. doi:10.1002/ppp.1764
Vasil’chuk, Y. K. (2006). Povtorno-zhilnye L’dy: Geterotsiklichnost’, Geterokhronost’, Geterogennost’ (Syngenetic Ice Wedges: Cyclical Formation, Radiocarbon Age and Stable Isotope Records). Moscow: Moscow University Press, 404. (in Russian).
Velichko, A. A., Grekhova, L. V., Gribchenko, Y. N., and Kurenkova, E. I. (1997). Early Man in the Extreme Environmental Conditions. Eliseevich Site. Moscow: Institute of Geography RAS, 192. (in Russian).
Velichko, A. A., Grekhova, L. V., and Gubonina, Z. P. (1977). Environments of the Ancient Man of the Timonovka Sites. Moscow: Nauka, 142. (in Russian).
Washburn, A. L. (1980). Permafrost Features as Evidence of Climatic Change. Earth-Science Rev. 15, 327–402. doi:10.1016/0012-8252(80)90114-2
Wetterich, S., Meyer, H., Fritz, M., Opel, T., and Schirrmeister, L. (2020). Cryolithology of the Sobo-Sise Yedoma Cliff (Eastern Lena Delta). PANGAEA 1, 1. doi:10.1594/PANGAEA.919470
Wetterich, S., Rudaya, N., Tumskoy, V., Andreev, A. A., Opel, T., Schirrmeister, L., et al. (2011). Last Glacial Maximum Records in Permafrost of the East Siberian Arctic. Quat. Sci. Rev. 30, 3139–3151. doi:10.1016/j.quascirev.2011.07.020
Wetterich, S., Tumskoy, V., Rudaya, N., Andreev, A. A., Opel, T., Meyer, H., et al. (2014). Ice Complex Formation in Arctic East Siberia during the MIS3 Interstadial. Quat. Sci. Rev. 84, 39–55. doi:10.1016/j.quascirev.2013.11.009
Yurtsev, B. A. (1994). Floristic Division of the Arctic. J. Vegetation Sci. 5, 765–776. doi:10.2307/3236191
Zanina, O. G., Gubin, S. V., Kuzmina, S. A., Maximovich, S. V., and Lopatina, D. A. (2011). Late-Pleistocene (MIS 3-2) Palaeoenvironments as Recorded by Sediments, Palaeosols, and Ground-Squirrel Nests at Duvanny Yar, Kolyma lowland, Northeast Siberia. Quat. Sci. Rev. 30, 2107–2123. doi:10.1016/j.quascirev.2011.01.021
Zhang, T., Heginbottom, J. A., Barry, R. G., and Brown, J. (2000). Further Statistics on the Distribution of Permafrost and Ground Ice in the Northern Hemisphere1. Polar Geogr. 24 (2), 126–131. doi:10.1080/10889370009377692
Zimmermann, H., Raschke, E., Epp, L., Stoof-Leichsenring, K., Schirrmeister, L., Schwamborn, G., et al. (2017). The History of Tree and Shrub Taxa on Bol'shoy Lyakhovsky Island (New Siberian Archipelago) since the Last Interglacial Uncovered by Sedimentary Ancient DNA and Pollen Data. Genes 8, 273. doi:10.3390/genes8100273
Keywords: Arctic East Siberia, ice complex, syngenetic permafrost development, cryoturbation, permafrost polygon pattern, Yana site complex, geoarchaeology, cultural layer
Citation: Pitulko VV and Pavlova EY (2022) Structural Properties of Syngenetic Ice-Rich Permafrost, as Revealed by Archaeological Investigation of the Yana Site Complex (Arctic East Siberia, Russia): Implications for Quaternary Science . Front. Earth Sci. 9:744775. doi: 10.3389/feart.2021.744775
Received: 20 July 2021; Accepted: 08 December 2021;
Published: 10 February 2022.
Edited by:
Lutz Schirrmeister, Alfred Wegener Institute Helmholtz Centre for Polar and Marine Research (AWI), GermanyReviewed by:
Julie Brigham-Grette, University of Massachusetts Amherst, United StatesFrank Kienast, Senckenberg Research Institute and Natural History Museum Frankfurt, Germany
Copyright © 2022 Pitulko and Pavlova. This is an open-access article distributed under the terms of the Creative Commons Attribution License (CC BY). The use, distribution or reproduction in other forums is permitted, provided the original author(s) and the copyright owner(s) are credited and that the original publication in this journal is cited, in accordance with accepted academic practice. No use, distribution or reproduction is permitted which does not comply with these terms.
*Correspondence: Vladimir V. Pitulko, pitulkov@gmail.com