- 1Key Laboratory of Western China’ Environmental System (Ministry of Education), College of Earth and Environmental Sciences, Lanzhou University, Lanzhou, China
- 2Department of Geological and Environmental Sciences, Appalachian State University, Boone, NC, United States
- 3Department of Geological Sciences, California State University Northridge, Northridge, CA, United States
Hyperarid climate and salty lakes prevail in the current Qaidam Basin, but this basin was once a large paleolake until the early Quaternary. However, its evolution history and relationship with climate and tectonics are still elusive. Here we present detailed stratigraphic descriptions combined with total organic carbon content and weight ratio of organic carbon to total nitrogen records from fluvio-lacustrine sediments in the western Qaidam Basin to infer how the lake evolved during the late Pliocene-early Pleistocene. These data reveal a drying trend since 3.3 Ma, which we attribute to low latitude forcing and/or local tectonic activities. However, this trend was interrupted during 2.84–2.48 Ma, in which climate wetting was observed. We attribute the climate wetting during 2.84–2.48 Ma to intensified East Asian summer monsoon as is documented by the Chinese Loess Plateau records. Halite and gypsum content increased dramatically after 2.5 Ma, indicating the formation of salty lake in the western Qaidam Basin. These data improve our understanding of the detailed processes of Qaidam aridification and its potential forcing mechanisms.
Introduction
The Qaidam Basin (QB), with an area of ∼80,000 km2 and an average elevation of ∼2.7 km above sea level, is the largest internally drained basin on the northeastern Tibetan Plateau (Fang et al., 2007). A hyperarid climate prevails with mean annual precipitation <50 mm and evaporation >1,000 mm in this basin (Wu et al., 2011; Cai et al., 2012; Wang et al., 2013). The basin is largely covered by dry salt playas, with only minor desert vegetation and hypersaline lakes (Wang et al., 2012). However, previous studies suggest that a huge Qaidam paleolake might have existed during the Eocene (Wang et al., 2006; Yin et al., 2008a, Yin et al., 2008b; Zhang et al., 2018; Liang et al., 2021), which expanded from the Oligocene to the Miocene (Yang, 1986; Wu and Xue, 1993), and broke into several small lakes likely during the Quaternary (Han et al., 2014; Lu et al., 2015). However, due to the scarcity of continuously dated sediment records (Lu et al., 2015; Fang et al., 2016), how the Qaidam paleolake evolved into its hyperarid condition remains relatively unknown. Furthermore, lack of such records prevents understanding the underlying forcing mechanisms for Qaidam drying and central Asian aridification.
Here we report lithofacies, total organic carbon (TOC), and weight ratio of organic carbon to total nitrogen (C/N) data for the Huatugou (HTG) section in the western Qaidam Basin in order to clarify the above question. TOC coupled with C/N ratio in fluvio-lacustrine sediments is sensitive to lake shrinkage and expansion and has been widely used to reflect variations of terrestrial input relative to lake productivity (Liu et al., 2013; Fan et al., 2017). Normally, >20 C/N ratio indicate terrestrial organic matter in lacustrine setting (Talbot and Lærdal, 2000), therefore a higher TOC coupled with a higher than 20 C/N ratio points toward terrestrial organic matter dominating TOC in lake sediments, indicating more terrestrial input relative to lake productivity (Talbot and Lærdal, 2000).
Materials and Methods
Geological Setting
The Qaidam Basin is located at the northeastern Tibetan Plateau, surrounded by the Altyn Shan to the northwest, the Qilian Shan to the northeast, and the Kunlun Shan to the southwest (Figure 1). It is far from any major moisture source and is in the rain shadow zone of the Tibetan Plateau and central Asian mountain ranges (Fang et al., 2007; Heermance et al., 2016). Its climate is cold (mean annual temperature 0–5°C), windy and dry. Hyperarid conditions combined with internal drainage within the basin have resulted in extensive evaporite and playa deposition throughout the Quaternary (Chen and Bowler, 1986; Fang et al., 2008; Heermance et al., 2016; Zhang et al., 2012; Wang et al., 2013). ∼12,000 m of sedimentary strata has been deposited in the basin since the early Cenozoic (Huang et al., 1996). These strata are divided into seven formations: the Lulehe Formation (Fm.), Xiaganchaigou Fm., Shangganchaigou Fm., Xiayoushashan Fm., Shangyoushashan Fm., Shizigou Fm., and Qigequan Fm. (Fang et al., 2007; Wang et al., 2007; Lu and Xiong, 2009; Zhuang et al., 2011; Chang et al., 2015; Bush et al., 2016; Wang et al., 2017; Nie et al., 2020).
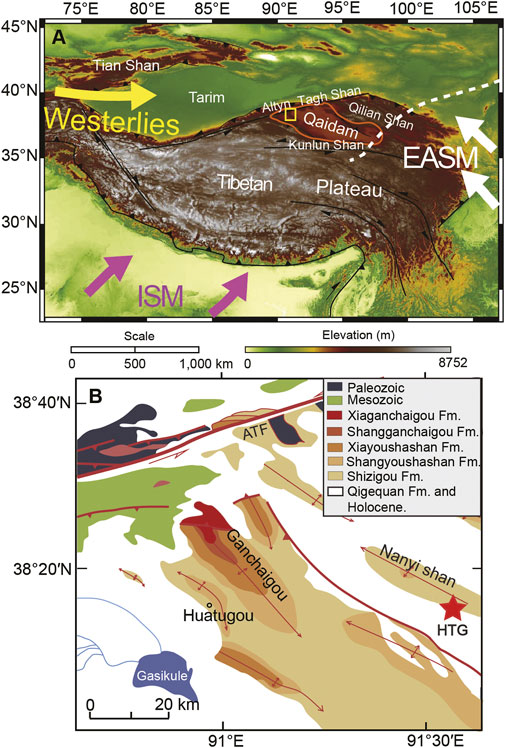
FIGURE 1. (A) Schematic summer atmospheric circulation pattern in Asia and topography of Himalayan-Tibetan orogen (modified from Zhuang et al., 2011). EASM: East Asian summer monsoon, ISM: Indian summer monsoon. (B) Geological map of the western Qaidam Basin and location of the Huatugou section [38°18.16′-38°15.5′N, 91°31.13′−91°34.2′E; labeled with red star, modified from Chang et al. 2015, Luo et al. (2018)]. HTG: Huatugou section, AFT: Altyn Tagh Fault. White dashed line depicts the modern Asian summer monsoon limit (Gao, 1962).
The studied HTG section lies on the southern flank of the Nanyi Shan (Figure 1B). The 476-m sedimentary strata consist of evaporite, shale, siltstone, sandstone, and rare conglomerate corresponding to the fluvio-lacustrine sediment of the Shizigou Fm., and Qigequan Fm. (Luo et al., 2018). The age model of HTG section is ∼3.9–2.1 Ma based on paleomagnetic dating by Luo et al. (2018). Milankovitch cycles are detected in the magnetic susceptibility record (Su et al., 2019a), demonstrating the validity of the paleomagnetic age model.
Methods
For TOC analysis, a total of 298 crushed bulk samples (40–50 mg ea.) were reacted with 3 mol/L HCl to remove carbonates before being wrapped in tin capsules. The wrapped samples were then heated to 1,800°C in a flow of oxygen using a Vario EL Cube elemental analyser (Elementar Analysensysteme, Germany). The combustion of the sample and absorption of the released CO2 were performed in the automated mode. The mass content of carbon in the sample was calculated using the proprietary software provided by the device manufacturer. For each set of analyses, calibration was performed against acetanilide.
Results
Lithofacies of the Huatugou Section
The 476-m strata were divided into three units (Units 1–3) based on lithofacies associations and depositional environments (Figure 2): Unit 1 (0–273 m; playa-fluvial-lacustrine), Unit 2 (273–371 m; playa–fluvial), and Unit 3 (371–476 m; playa–evaporite).
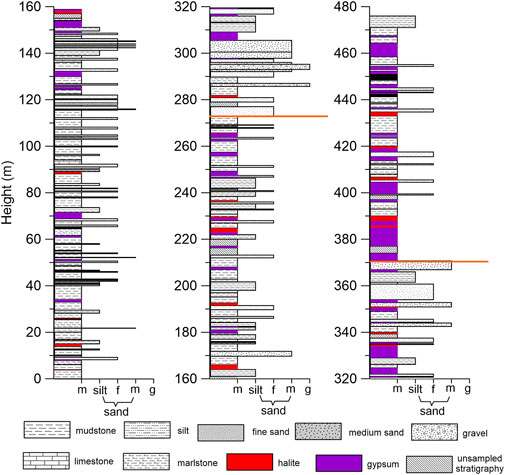
FIGURE 2. Stratigraphic column of the Huatugou section in the western Qaidam Basin. The 476-m strata were subdivided into three units (Units 1–3) based on lithofacies associations and depositional environments.
Unit 1: Playa-Fluvial-Lacustrine (0–273 m)
Unit 1 is characterized by massive mudstone interbedded with fine grained sandstone beds. The evaporite layers started to appear at the base of the section (halite layer at 3.8 m and gypsum layer at 7.9 m). Evaporite layers in Unit 1 are least frequently observed of the entire sequence. Unit 1 is interpreted as littoral to sublittoral lacustrine sedimentation in an internally drained, playa environment (Pietras and Carroll, 2006). We note that fluvial layers are less frequent for the upper portion of this unit (ca. 150–273 m), and this portion is dominated by playa-lacustrine.
Unit 2: Playa–Fluvial (273–371 m)
Unit 2 is dominated by fine-medium grained sandstone beds with more frequent occurrences of evaporite layers (gypsum and halite) than Unit 1, approximately every 2.4 m. Unit 2 is interpreted as a sand flat and perennial shallow lacustrine environment (Gierlowski-Kordesch and Rust, 1994). The overall increase in grain-size and appearance of medium sandstone beds suggest an increase in sediment-input from fluvial erosion.
Unit 3: Playa–Evaporite (371–476 m)
Unit 3 is dominated by massive mudstone with interbedded halite and gypsum beds. This unit has the highest frequency of evaporite beds, occurring every 2.1 m, which implies either regular times of hyper-concentrated brine within the lake or frequent desiccation.
TOC and C/N Ratio Records
The TOC and C/N ratio records show similar patterns and can be divided into four phases for this set of fluvio-lacustrine sediments, with boundaries at 158, 273, and 380 m (corresponding to 3.3, 2.84, and 2.48 Ma, respectively, Figures 3, 4).
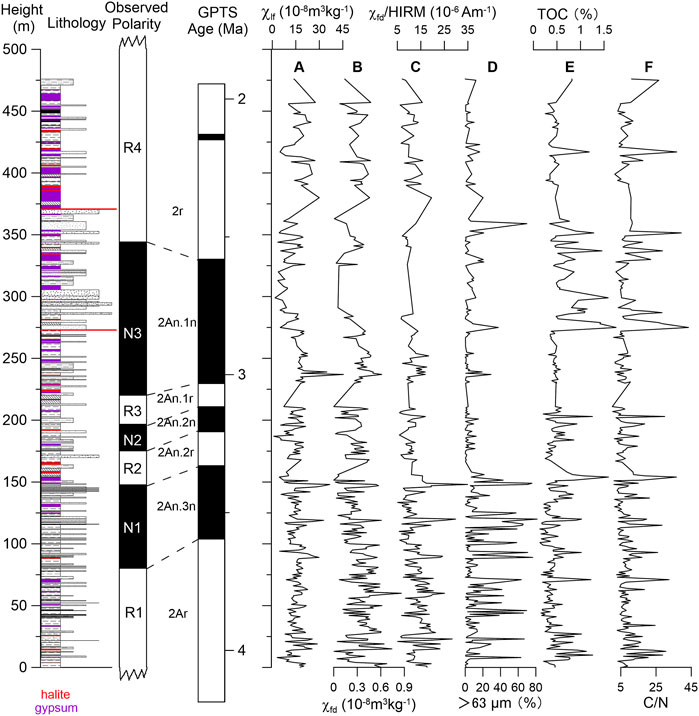
FIGURE 3. Paleoenvironmental proxy records and corresponding stratigraphic column of the Huatugou section in the western Qaidam Basin, NE Tibetan Plateau. (A–C) The χlf, χfd, and χfd/HIRM records (Su et al., 2019a; Su et al., 2019b). (D) The >63 μm fraction grain size record (Su et al., 2019b). (E–F) The TOC and C/N records. Larger TOC values correspond to higher C/N ratio values, suggesting terrestrial organic matter input. The magnetostratigraphy of the Huatugou section was derived from Luo et al. (2018).
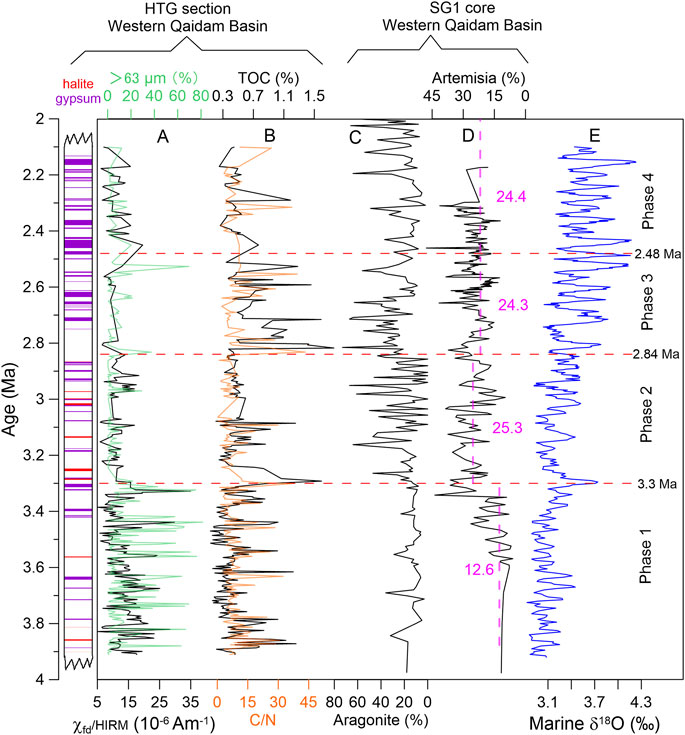
FIGURE 4. Late Pliocene paleoenvironmental records from the Huatugou section and the SG1 core in the western Qaidam Basin. (A) The >63 μm fraction grain size record (green line) and the χfd/HIRM record (black line, Su et al., 2019b) of the Huatugou section in the western Qaidam Basin. (B) The TOC record (black line) and C/N ratio record (yellow line) of the Huatugou section in the western Qaidam Basin. (C–D) The aragonite record (Fang et al., 2016) and the artemisia record (Koutsodendris et al., 2019) from the SG1 core in the western Qaidam Basin. (E) Marine δ18O record (Zachos et al., 2001). Three phase boundaries at ∼3.3, ∼2.84, and ∼2.48 Ma are labeled with red dashed lines.
The TOC content record shows high amplitude fluctuations in Phase 1 (0–158 m, corresponding to 3.9–3.3 Ma; Figure 4) and Phase 3 (273–380 m, corresponding to 2.84–2.48 Ma; Figure 4). The TOC values vary from 0.14–1.59% in Phase 1 and 0.34–1.76% in Phase 3, but almost all the TOC values are below 1% in Phase 2 (158–273 m, corresponding to 3.3–2.84 Ma; Figure 4) and Phase 4 (380–476 m, corresponding to 2.48–2.1 Ma; Figure 4). Similarly, the C/N ratio values show high amplitude fluctuations in Phase 1 and Phase 3 varying between 1.1 and 43.4, but most of the C/N ratio values are below 10 in Phase 2 and Phase 4.
Discussion
Environmental Implications of TOC and C/N Ratio Variations
C/N ration is a useful proxy to distinguish different carbon sources in lacustrine sediments (Talbot and Lærdal, 2000). C/N ratios of aquatic vegetation are generally between 4 and 10, whereas C/N ratios of terrestrial plants are generally greater than 20 (Talbot and Lærdal, 2000). The C/N ratio values in Phase 1 and Phase 3 are frequently greater than 20, suggesting that organic matter in those intervals was mainly derived from terrestrial plants transported by rivers. 83% of C/N ratio values in Phase 2 and Phase 4 are below 10, suggesting a low lake productivity and lack of terrestrial derived organic matter. One may question whether high C/N ratios in the study site are aligned with large grain size and fluvial transport, so that this ratio can not be used to infer terrestrial derived organic matter. However, few large grain size intervals (corresponding to fluvial input) correspond to higher than 20 C/N ratio, suggesting that higher than 20 C/N ratios correspond to lacustrine setting. Following this observation, we interpret the intervals with higher TOC and C/N ratio during Phases 1 and 3 as climate wetting.
Evolution of Western Qaidam Paleolake During 4–2 Ma
The evolution of Qaidam paleolake is divided into four phases based on TOC and C/N ratios during the Plio-Pleistocene: Phase 1 (3.9–3.3 Ma), Phase 2 (3.3–2.84 Ma), Phase 3 (2.84–2.48 Ma), and Phase 4 (2.48 Ma–2.1 Ma).
The lithofacies, TOC and C/N ratio records from the HTG section suggest an open lake and a regularly wetting climate before 3.3 Ma (Phase 1, Figure 4). This inference is consistent with grain size and χfd/HIRM records from the Qaidam Basin (Figure 4, Su et al., 2019b). We interpret that during a wetting climate, terrestrial plants flourished in the surrounding regions, resulting in more terrestrial organic matter being transported to the depositional region.
The TOC and C/N ratio records from HTG section suggest the Qaidam paleolake changed from an open lake to closed, salty lake at ∼3.3 Ma (Figure 4). Previous studies from the Qaidam Basin consistently suggest a phase of drying at ∼3.3 Ma (Heermance et al., 2016; Fang et al., 2016; Su et al., 2019b). For example, in the Northeast Qaidam Basin, a change from wetter fluvial/overbank strata to playa-lacustrine and evaporite strata occurred around 3.3 Ma (Heermance et al., 2016). A drying climate is observed in the western Qaidam Basin as is recorded by mineral composition records suggesting a brackish lake with higher Mg/Ca and higher salinity after 3.3 Ma (Fang et al., 2016). Moreover, our χfd/HIRM and grain size data from the HTG section in northwestern Qaidam Basin reveal a phase of drying in the Qaidam Basin at ∼3.3 Ma (Su et al., 2019b; Figure 4). Coupled with previous studies from the western Qaidam Basin, we interpret the hydrological transformation at ∼3.3 Ma as a result of intensified aridity (Phase 2; Figure 4). Pliocene tectonic uplift in the study region can be one forcing for the observed drying (Lu et al., 2015). Alternatively, closure of the Indonesian Seaway and associated sea surface temperature (SST) decrease of the Indian Ocean is another or additional potential way to explain central Asian drying at ∼3.3 Ma (Su et al., 2019b).
Interestingly, superimposed on the long-term drying trend, a wetting climate in the Qaidam Basin occurred between 2.84 and 2.48 Ma (Figure 4). Sedimentological evidence from the same site also indicates regular occurrences of fluvial system and wetter conditions between 2.84 and 2.48 Ma. Previous studies demonstrate a stepwise drying of central Asia since the Pliocene (Fang et al., 2008; Han et al., 2014; Lu et al., 2015). However, superimposed on the long-term drying trend, a phase of undetected climate wetting did occur in the Qaidam Basin during 2.84–2.48 Ma. This wetting is not unique to this site. For example, averaged artemisia content decreased from 25.3% in Phase 2 to 24.3% in Phase 3 (Figure 4, Koutsodendri et al., 2019), suggesting climate wetting. Interestingly, aragonite content increased from Phase 2 to Phase 3 (Figure 4, Fang et al., 2016), different from the artemisia content variations. We argue that biological proxies may better reflect regional climate than evaporite mineral content. Therefore, we chose to use the biological data.
Global climate was experiencing a cooling trend since 3.6 Ma (Lisiecki and Raymo, 2005), so it is hard to attribute the observed intensified precipitation in the study site during 2.84–2.48 Ma to global forcing. Previous research suggests that stronger East Asian summer monsoon (EASM) precipitation likely penetrated further inland, which could bring more moisture to this inland basin (Liu and Ding, 1998). The magnetic susceptibility records from the Chinese Loess Plateau indicates an intensified Asian summer monsoon during this interval (An et al., 2001; Nie et al., 2014). Thus, we attribute the late Pliocene wetting of the Qaidam Basin to enhancing EASM. Alternatively, increased moisture input from westerly source is another possibility. However, evidence for westerly moisture input increase at this time interval is lacking.
Halite and gypsum content increased dramatically after 2.5 Ma (Figures 3, 4), indicating the formation of salty lake and intensified aridification at ∼2.5 Ma in the western Qaidam Basin. Intensified aridification of the Qaidam Basin at the beginning of Quaternary is consistent with adjacent sections in the Qaidam Basin (Wu et al., 2011; Cai et al., 2012; Wang et al., 2012; Yang et al., 2013). The deep–lake black lamination abruptly disappeared and changed to a shallow–lake gray massive structure (Wang et al., 2012) and a shift in the paleolake nutrient status (Yang et al., 2013) from SG–1 in the western Qaidam Basin at ∼2.5 Ma, indicating rapid aridification at ∼2.6 Ma. Pollen records from SG–3 drill cores and Yahu anticline also support intensified aridity during the Quaternary period (Wu et al., 2011; Cai et al., 2012). The marine benthic δ18O record (Lisiecki and Raymo, 2005) suggests intensified Northern Hemisphere glaciations at 2.7 Ma. The intensified aridification of central Asia at the Pliocene-Quaternary boundary may be in response to intensive Northern Hemisphere glaciations and resulting decreased evaporation from the Pacific and Indian Oceans, which could have provided moisture to the Qaidam Basin during the Pliocene (Su et al., 2019b). Decreased sea surface temperatures in many oceans (Herbert et al., 2016) is in line with this interpretation.
Conclusion
We report new, multiple-proxy records of the western Qaidam Basin lake evolution. These records reveal four phases evolution of the western Qaidam paleolake, with boundaries at ∼3.3, ∼2.84, and ∼2.48 Ma. The data reveal that the Qaidam paleolake change from an open lake to a closed and salty lake at ∼3.3 Ma, likely in response to decreased precipitation from the low latitudes and/or local tectonic uplift. Interestingly, superimposed on the long-term drying trend, a phase of wetting climate was observed in the TOC records in the Qaidam Basin during 2.84–2.48 Ma, which we suggest linked to enhancing East Asian summer monsoon. Halite and gypsum content increased dramatically after 2.5 Ma, indicating the formation of salty lake in the western Qaidam Basin, which may be in response to intensive Northern Hemisphere glaciation and decreased sea surface evaporation. These data improve our understanding of the detailed processes of Qaidam aridification and its potential forcing mechanisms.
Data Availability Statement
The original contributions presented in the study are included in the article/Supplementary Material, further inquiries can be directed to the corresponding authors.
Author Contributions
LG and JN designed the experiment; QS and LG analyzed the data; QS and JN wrote the paper with the help of all authors; HR, LG, and RH participated in the discussion and modification.
Funding
This work was financially supported by the Second Tibetan Plateau Scientific Expedition (grant 2019QZKK0704), National Natural Science Foundation of China (grant 42030505), Science and Technology Department of Gansu Province of China (grant 20JR5RA260), and the 111 Project (grant BP0618001).
Conflict of Interest
The authors declare that the research was conducted in the absence of any commercial or financial relationships that could be construed as a potential conflict of interest.
Publisher’s Note
All claims expressed in this article are solely those of the authors and do not necessarily represent those of their affiliated organizations, or those of the publisher, the editors and the reviewers. Any product that may be evaluated in this article, or claim that may be made by its manufacturer, is not guaranteed or endorsed by the publisher.
Acknowledgments
Thanks to Shunchuan Ji, Wenhan Chen, and Rui Zhang for their field assistance.
Supplementary Material
The Supplementary Material for this article can be found online at: https://www.frontiersin.org/articles/10.3389/feart.2021.736901/full#supplementary-material
References
An, Z. S., Kutzbach, J. E., Prell, W. L., and Porter, S. C. (2001). Evolution of Asian Monsoons and Phased Uplift of the Himalaya-Tibetan Plateau since Late Miocene Times. Nature 411, 62–66. doi:10.1038/35075035
Bush, M. A., Saylor, J. E., Horton, B. K., and Nie, J. (2016). Growth of the Qaidam Basin during Cenozoic Exhumation in the Northern Tibetan Plateau: Inferences from Depositional Patterns and Multiproxy Detrital Provenance Signatures. Lithosphere 8, 58–82. doi:10.1130/L449.1
Cai, M., Fang, X., Wu, F., Miao, Y., and Appel, E. (2012). Pliocene-Pleistocene Stepwise Drying of Central Asia: Evidence from Paleomagnetism and Sporopollen Record of the Deep Borehole SG-3 in the Western Qaidam Basin, NE Tibetan Plateau. Glob. Planet. Change 94-95, 72–81. doi:10.1016/j.gloplacha.2012.07.002
Chang, H., Li, L., Qiang, X., Garzione, C. N., Pullen, A., and An, Z. (2015). Magnetostratigraphy of Cenozoic Deposits in the Western Qaidam Basin and its Implication for the Surface Uplift of the Northeastern Margin of the Tibetan Plateau. Earth Planet. Sci. Lett. 430, 271–283. doi:10.1016/j.epsl.2015.08.029
Chen, K., and Bowler, J. M. (1986). Late Pleistocene Evolution of Salt Lakes in the Qaidam Basin, Qinghai Province, China. Palaeogeogr. Palaeoclimatol. Palaeoecol. 54, 87–104. doi:10.1016/0031-0182(86)90119-74
Fan, J., Xiao, J., Wen, R., Zhang, S., Wang, X., Cui, L., et al. (2017). Organic Geochemical Investigations of the Dali Lake Sediments in Northern China: Implications for Environment and Climate Changes of the Last Deglaciation in the East Asian Summer Monsoon Margin. J. Asian Earth Sci. 140, 135–146. doi:10.1016/j.jseaes.2017.04.011
Fang, X., Li, M., Wang, Z., Wang, J., Li, J., Liu, X., et al. (2016). Oscillation of mineral Compositions in Core SG-1b, Western Qaidam Basin, NE Tibetan Plateau. Sci. Rep. 6, 32848. doi:10.1038/srep32848
Fang, X. M., Wu, F. L., Han, W. X., Wang, Y. D., Zhang, X. S., and Zhang, W. L. (2008). Plio-Pleistocene Drying Process of Asian Inland—Sporopollen and Salinity Records from Yahu Section in the central Qaidam Basin (In Chinese). Quat. Sci. 28, 874–882.
Fang, X., Zhang, W., Meng, Q., Gao, J., Wang, X., King, J., et al. (2007). High-resolution Magnetostratigraphy of the Neogene Huaitoutala Section in the Eastern Qaidam Basin on the NE Tibetan Plateau, Qinghai Province, China and its Implication on Tectonic Uplift of the NE Tibetan Plateau. Earth Planet. Sci. Lett. 258, 293–306. doi:10.1016/j.epsl.2007.03.042
Gao, Y. X. (1962). On Some Problems of Asian Monsoon, in Some Questions about the East Asian Monsoon. Editors Y. X. Gao (Beijing: Chinese Science Press), 1–49.
Gierlowski-Kordesch, E., and Rust, B. R. (1994). The Jurassic East Berlin Formation, Hartford Basin, Newark Supergroup (Connecticut and Massachusetts): a saline lake-playa-alluvial plain System. Spec. Publications 239, 249–265. doi:10.2110/pec.94.50.0249
Han, W., Fang, X., Ye, C., Teng, X., and Zhang, T. (2014). Tibet Forcing Quaternary Stepwise Enhancement of westerly Jet and central Asian Aridification: Carbonate Isotope Records from Deep Drilling in the Qaidam Salt Playa, NE Tibet. Glob. Planet. Change 116, 68–75. doi:10.1016/j.gloplacha.2014.02.006
Heermance, R. V., Pullen, A., Kapp, P., Garzione, C. N., Bogue, S., Ding, L., et al. (2016). Climatic and Tectonic Controls on Sedimentation and Erosion during the Pliocene-Quaternary in the Qaidam Basin (China). Geol. Soc. Am. Bull. 125, 833–856. doi:10.1016/S0899-5362(99)00014-7
Herbert, T. D., Lawrence, K. T., Tzanova, A., Peterson, L. C., Caballero-Gill, R., and Kelly, C. S. (2016). Late Miocene Global Cooling and the Rise of Modern Ecosystems. Nat. Geosci. 9, 843–847. doi:10.1038/NGEO2813
Huang, H. C., Huang, Q. H., and Ma, Y. S. (1996). Geology of Qaidam and Petroleum Prediction. Beijing: Geological Publ. House. (in Chinese).
Koutsodendris, A., Allstädt, F. J., Kern, O. A., Kousis, I., Schwarz, F., Vannacci, M., et al. (2019). Late Pliocene Vegetation Turnover on the NE Tibetan Plateau (Central Asia) Triggered by Early Northern Hemisphere Glaciation. Glob. Planet. Change 180, 117–125. doi:10.1016/j.gloplacha.2019.06.001
Liang, Y., Zhang, B., Zhang, Y., Zhang, Y., Wang, J., and Liu, Z. (2021). Evolution of the Miocene Megalake in the Western Qaidam Basin, Northwestern China. Palaeogeogr. Palaeoclimatol. Palaeoecol. 571, 110384. doi:10.1016/j.palaeo.2021.110384
Lisiecki, L. E., and Raymo, M. E. (2005). A Pliocene-Pleistocene Stack of 57 Globally Distributed Benthic δ18O Records. Paleoceanography 20, PA1003. doi:10.1029/2004PA001071
Liu, T., and Ding, Z. (1998). Chinese Loess and the Paleomonsoon. Annu. Rev. Earth Planet. Sci. 26, 111–145. doi:10.1146/annurev.earth.26.1.111
Liu, W., Li, X., An, Z., Xu, L., and Zhang, Q. (2013). Total Organic Carbon Isotopes: A Novel Proxy of lake Level from Lake Qinghai in the Qinghai-Tibet Plateau, China. Chem. Geol. 347, 153–160. doi:10.1016/j.chemgeo.2013.04.009
Lu, H., and Xiong, S. (2009). Magnetostratigraphy of the Dahonggou Section, Northern Qaidam Basin and its Bearing on Cenozoic Tectonic Evolution of the Qilian Shan and Altyn Tagh Fault. Earth Planet. Sci. Lett. 288, 539–550. doi:10.1016/j.epsl.2009.10.016
Lu, Y., Fang, X., Appel, E., Wang, J., Herb, C., Han, W., et al. (2015). A 7.3-1.6Ma Grain Size Record of Interaction between Anticline Uplift and Climate Change in the Western Qaidam Basin, NE Tibetan Plateau. Sediment. Geol. 319, 40–51. doi:10.1016/j.sedgeo.2015.01.008
Luo, Z., Su, Q., Wang, Z., Heermance, R. V., Garzione, C., Li, M., et al. (2018). Orbital Forcing of Plio-Pleistocene Climate Variation in a Qaidam Basin lake Based on Paleomagnetic and Evaporite Mineralogic Analysis. Palaeogeogr. Palaeoclimatol. Palaeoecol. 510, 31–39. doi:10.1016/j.palaeo.2017.09.022
Nie, J., Ren, X., Saylor, J. E., Su, Q., Horton, B. K., Bush, M. A., et al. (2020). Magnetic Polarity Stratigraphy, Provenance, and Paleoclimate Analysis of Cenozoic Strata in the Qaidam Basin, NE Tibetan Plateau. Geol. Soc. Am. Bull. 132, 310–320. doi:10.1130/B35175.1
Nie, J., Stevens, T., Song, Y., King, J. W., Zhang, R., Ji, S., et al. (2014). Pacific Freshening Drives Pliocene Cooling and Asian Monsoon Intensification. Sci. Rep. 4, 5474. doi:10.1038/srep05474
Pietras, J. T., and Carroll, A. R. (2006). High-Resolution Stratigraphy of an Underfilled Lake Basin: Wilkins Peak Member, Eocene Green River Formation, Wyoming, U.S.A. J. Sediment. Res. 76, 1197–1214. doi:10.2110/jsr.2006.096
Su, Q., Nie, J., Luo, Z., Li, M., Heermance, R., and Garzione, C. (2019a). Detection of Strong Precession Cycles from the Late Pliocene Sedimentary Records of Northeastern Tibetan Plateau. Geochem. Geophys. Geosyst. 20, 3901–3912. doi:10.1029/2019GC008447
Su, Q., Nie, J., Meng, Q., Heermance, R., Gong, L., Luo, Z., et al. (2019b). Central Asian Drying at 3.3 Ma Linked to Tropical Forcing?. Geophys. Res. Lett. 46, 10561–10567. doi:10.1029/2019GL084648
Talbot, M. R., and Lærdal, T. (2000). The Late Pleistocene-Holocene Palaeolimnology of Lake Victoria, East Africa, Based upon Elemental and Isotopic Analyses of Sedimentary Organic Matter. J. Paleolimnol. 23, 141–164. doi:10.1023/a:1008029400463
Wang, E., Xu, F.-Y., Zhou, J.-X., Wan, J., and Burchfiel, B. C. (2006). Eastward Migration of the Qaidam basin and its Implications for Cenozoic Evolution of the Altyn Tagh Fault and Associated River Systems. Geol. Soc. Am. Bull. 118, 349–365. doi:10.1130/B25778.1
Wang, J., Fang, X., Appel, E., and Song, C. (2012). Pliocene-Pleistocene Climate Change at the NE Tibetan Plateau Deduced from Lithofacies Variation in the Drill Core SG-1, Western Qaidam Basin, China. J. Sediment. Res. 82, 933–952. doi:10.2110/jsr.2012.76
Wang, J., Fang, X., Appel, E., and Zhang, W. (2013). Magnetostratigraphic and Radiometric Constraints on Salt Formation in the Qaidam Basin, NE Tibetan Plateau. Quat. Sci. Rev. 78, 53–64. doi:10.1016/j.quascirev.2013.07.017
Wang, W., Zheng, W., Zhang, P., Li, Q., Kirby, E., Yuan, D., et al. (2017). Expansion of the Tibetan Plateau during the Neogene. Nat. Commun. 8, 15887. doi:10.1038/ncomms15887
Wang, X., Qiu, Z., Li, Q., Wang, B., Qiu, Z., Downs, W. R., et al. (2007). Vertebrate Paleontology, Biostratigraphy, Geochronology, and Paleoenvironment of Qaidam Basin in Northern Tibetan Plateau. Palaeogeogr. Palaeoclimatol. Palaeoecol. 254, 363–385. doi:10.1016/j.palaeo.2007.06.007
Wu, C. J., and Xue, S. H. (1993). Petroliferous Basin Sedimentology in China. (Beijing: Petroleum Industry Press).
Wu, F., Fang, X., Herrmann, M., Mosbrugger, V., and Miao, Y. (2011). Extended Drought in the interior of Central Asia since the Pliocene Reconstructed from Sporopollen Records. Glob. Planet. Change 76, 16–21. doi:10.1016/j.gloplacha.2010.11.007
Yang, Y., Fang, X., Appel, E., Galy, A., Li, M., and Zhang, W. (2013). Late Pliocene-Quaternary Evolution of Redox Conditions in the Western Qaidam Paleolake (NE Tibetan Plateau) Deduced from Mn Geochemistry in the Drilling Core SG-1. Quat. Res. 80, 586–595. doi:10.1016/j.yqres.2013.07.007
Yang, Z. L. (1986). “Cenozoic Lithofacies Paleogeography and its Evolution in Qaidam Basin,” in Evolution of Late Cenozoic Geological Environment in Qaidam Basin, Qinghai Province. Qinghai institute of Salt lake, Chinese Academy of Sciences (Beijing: Science Press), 1–18. (in Chinese).
Yin, A., Dang, Y.-Q., Wang, L.-C., Jiang, W.-M., Zhou, S.-P., Chen, X.-H., et al. (2008a). Cenozoic Tectonic Evolution of Qaidam basin and its Surrounding Regions (Part 1): The Southern Qilian Shan-Nan Shan Thrust belt and Northern Qaidam basin. Geol. Soc. Am. Bull. 120, 813–846. doi:10.1130/B26180.1
Yin, A., Dang, Y.-Q., Zhang, M., Chen, X.-H., and Mcrivette, M. W. (2008b). Cenozoic Tectonic Evolution of the Qaidam basin and its Surrounding Regions (Part 3): Structural Geology, Sedimentation, and Regional Tectonic Reconstruction. Geol. Soc. Am. Bull. 120, 847–876. doi:10.1130/B26232.1
Zachos, J., Pagani, M., Sloan, L., Thomas, E., and Billups, K. (2001). Trends, Rhythms, and Aberrations in Global Climate 65 Ma to Present. Sci. 292, 686–693. doi:10.1126/science.1059412
Zhang, T., Han, W., Fang, X., Miao, Y., Zhang, W., Song, C., et al. (2018). Tectonic Control of a Change in Sedimentary Environment at ∼10 Ma in the Northeastern Tibetan Plateau. Geophys. Res. Lett. 45, 6843–6852. doi:10.1029/2018GL078460
Zhang, W., Appel, E., Fang, X., Yan, M., Song, C., and Cao, L. (2012). Paleoclimatic Implications of Magnetic Susceptibility in Late Pliocene-Quaternary Sediments from Deep Drilling Core SG-1 in the Western Qaidam Basin (NE Tibetan Plateau). J. Geophys. Res. 117, B06101. doi:10.1029/2011JB008949
Keywords: total organic carbon, Pliocene, salty lake, Qaidam Basin, Tibetan Plateau
Citation: Su Q, Riegel H, Gong L, Heermance R and Nie J (2021) Detailed Processes and Potential Mechanisms of Pliocene Salty Lake Evolution in the Western Qaidam Basin. Front. Earth Sci. 9:736901. doi: 10.3389/feart.2021.736901
Received: 06 July 2021; Accepted: 17 August 2021;
Published: 03 September 2021.
Edited by:
Yongli Wang, Institute of Geology and Geophysics (CAS), ChinaReviewed by:
Yunfa Miao, Northwest Institute of Eco-Environment and Resources (CAS), ChinaMaodu Yan, Institute of Tibetan Plateau Research (CAS), China
Copyright © 2021 Su, Riegel, Gong, Heermance and Nie. This is an open-access article distributed under the terms of the Creative Commons Attribution License (CC BY). The use, distribution or reproduction in other forums is permitted, provided the original author(s) and the copyright owner(s) are credited and that the original publication in this journal is cited, in accordance with accepted academic practice. No use, distribution or reproduction is permitted which does not comply with these terms.
*Correspondence: Lisha Gong, Z29uZ2xpc2hhQDE2My5jb20=; Junsheng Nie, am5pZUBsenUuZWR1LmNu