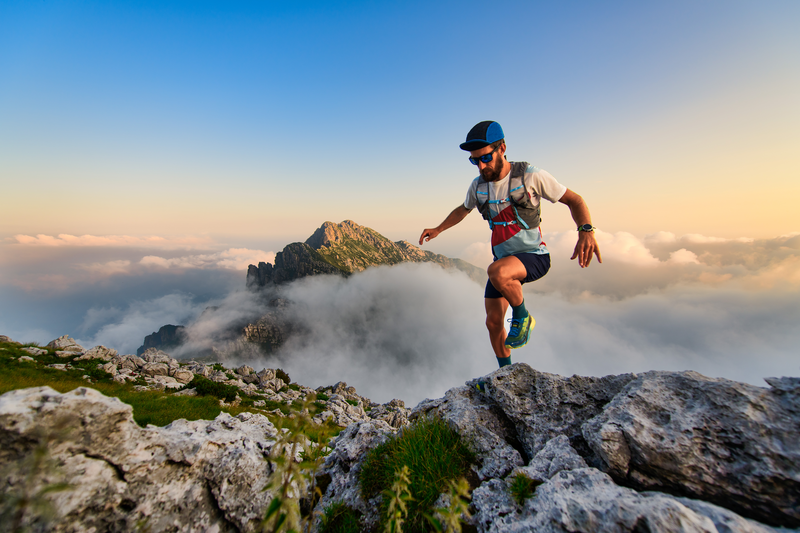
95% of researchers rate our articles as excellent or good
Learn more about the work of our research integrity team to safeguard the quality of each article we publish.
Find out more
ORIGINAL RESEARCH article
Front. Earth Sci. , 27 October 2021
Sec. Cryospheric Sciences
Volume 9 - 2021 | https://doi.org/10.3389/feart.2021.718904
This article is part of the Research Topic Yedoma Permafrost Landscapes as Past Archives, Present and Future Change Areas View all 27 articles
The study focuses on authigenic carbonates that are widespread in different deposition environments and are a component part of the terrestrial biogeochemical cycle of carbon. Samples from the Kolyma Yedoma Ice Complex that formed during the Sartan Cryochrone (MIS 2), the coldest period of the Late Pleistocene, in the northeastern Siberian lowlands, have been studied utilizing scanning electron microscopy and energy-dispersive spectroscopy with replica technique. The samples bear signatures of irreversible multistage cryogenic changes in structure and composition, with the formation of authigenic minerals. Authigenic carbonates as secondary phases in the Ice Complex deposits are remarkable by local changes in chemical, physical, and other properties, which induce gradual changes in the lattice and conversion of one mineral species to another. As a result, the sediments may contain stable and metastable minerals. Crystalline species like calcite or aragonite precipitate from aqueous solutions and their presence are restricted to free pore space in segregation ice. Metastable phases may be produced as an initial reaction product between the CO2 and the aqueous phase, while mineral surfaces and small pores act as possible nucleation sites. Organic matter is also an important agent in the cryometamorphism of sediments, including precipitation of authigenic phases due to the freezing of colloids and high-molecular compounds.
Authigenic minerals in sediments are formed by physicochemical and biochemical reactions during and after deposition in various environmental settings. The mineralogical alteration of sediments soon after the deposition (early diagenesis) is controlled primarily by their original compositions and relative percentages of four main components: chemically passive and active minerals, organic matter, and pore fluids. Chemically passive phases are commonly predominant and mostly include primary minerals (quartz, feldspar, garnet, and ilmenite) except for a few relatively reactive Fe- and Ca-bearing silicates. Chemically active minerals encompass such inorganic phases as Fe, Al, and Mn hydroxides, mix gels, amorphous silica, carbonates, and water-soluble salts, which are in equilibrium with pore fluids only at the time of precipitation (Curtis, 1990; Buggle et al., 2011).
The decay of organic matter produces СО2 and Н2S, NH3, CH4, and other reactive components, which become involved in the alteration of sediments and control the chemical environment, though are as low as a few percents or less (Davidson and Janssens, 2006). As for pore fluids, they commonly acquire higher pH during early diagenesis (Tranter, 2003).
The rate of physicochemical changes and the redistribution of components differ in subaquatic and subaerial settings. The authigenic minerals that precipitate in subaquatic conditions, when sediments undergo compaction and pore water is squeezed out, locally cement detrital mineral grains and form concretions accumulating impurities (Zn, Со, Cu, etc.). Precipitation of calcium carbonate in sediments is driven by the generation of subseafloor alkalinity, which is typically the result of microbial respiration of organic carbon in the absence of oxygen; the oxygen penetration depth for the majority of the seafloor is less than 1 m (D’Hondt et al., 2015; Turchyn et al., 2021).
Subaerial diagenesis requires hydration of sediments to maintain ion exchange between surface-active components (clay minerals, collomorphic oxide phases, organic complexes, etc.) and the ensuing formation of soluble and amorphous phases. Calcification as the process of the accumulation of authigenic carbonates in subaerial settings is dominant in many soils in arid or semiarid environments. Both abiogenic sources of Ca (primary minerals ann groundwater) and atmospheric or biogenic (decaying organic matter and rhizomicrobial respiration) sources of CO2 are involved in carbonate authigenesis in soils (Kolesár and Čurlik, 2015). Various types of microscale secondary carbonates are preserved in loess-paleosol sequences. Their abundance and distribution within loess profiles can indicate moisture regime changes, dust accumulation characteristics, and the extent and intensity of leaching processes (Becze-Deák et al., 1997; Barta, 2011; Durand et al., 2018).
Unlike unfrozen areas, the diagenetic reactions in permafrost mostly involve bound water that affects the surface of organic-mineral particles, whereas free water-sediment interactions are restricted to the active layer. Another difference is that the reactions at negative temperatures are slow, but the low rates are compensated by the high contents of agents in limited volume. Chemical reactions in perennially or seasonally frozen sediments occur, respectively, at long-lasting negative temperatures or during freezing and thawing (single brief events or repeated cycles) (Yershov, 1998). The processes during the freezing and thawing cycles in the active layer have been quite well studied (Siegert, 1981; Konishchev and Rogov, 1993; Ostroumov et al., 2001; Rogov, 2009).
Note that it is hard to discriminate between diagenetic processes and soil formation in subaerial settings (Lupachev, Gubin, 2012). Soil formation processes, which reflect the main physicochemical and biochemical reactions during and after deposition, influence the precipitation of authigenic minerals. These minerals are abundant in frozen soils and permafrost of Central Yakutia (Siegert, 1981) and the northeastern Siberian lowlands (Alekseev et al., 2003).
In general, the main aim of this topic is to elucidate authigenic carbonate accumulations in the Yedoma Ice Complex from the Kolyma Lowland, Northeast Siberia i) to document the in situ crystals of carbonates in Yedoma silt, ii) to report shape and characteristics of the crystals deposited in the textural ice and pore space of the frozen sediments, and iii) to discuss the processes involved in the precipitation of cryogenic carbonates. Their features and distribution patterns in permafrost have implications for the formation conditions of genetically different frozen sediments, both inside and outside the present permafrost limits, and for ecological factors that provide the terrestrial biogeochemical cycle of carbon (Strauss et al., 2013).
The microstructure of Yedoma Ice Сomplex was studied in samples from the well-known sites of the Kolyma Lowland: Kolymskoe, Plakhinskii Yar, Duvanny Yar, Zelyony Mys, and Kur’ishka (Figure 1). Currently, a large number of publications mainly by Russian researchers exist that give detailed description of these sites since the 1970s; therefore, in this article, the authors will not describe their lithological and stratigraphic units (e.g., Arkhangelov et al., 1979; Vasil’chuk, 2005; Kaplina, 2011). The distribution of Yedoma in the northeastern Siberian lowlands has been recently mapped (Veremeeva et al., 2021). One of the last summary publications concerning the Yedoma Ice Complex from the Kolyma Lowland (on the base of stratotype site Duvanny Yar) to test the processes and environmental conditions of silt deposition has been given by Murton et al., 2015.
FIGURE 1. Study area (A) and location of the sampling sites at the Kolyma Lowland (B). Sites are numbered: 1 = Kur’ishka; 2 = Zelyony Mys; 3 = Plakhinskii Yar; 4 = Duvanny Yar; 5 = Kolymskoe.
We chose specific sampling sites to study the undisturbed structure of the frozen sediments. The cubic monoliths (15 × 15 × 15 cm) were cut at a certain height (above river level, arl), which allows considering the age of the samples tested as Sartan Cryochrone (Q4III or MIS 2, Marine Isotope Stage) for each site.
The samples are typical for the Yedoma silt and are characterized by uniform fine grain sizes (up to 75% of coarse silt) and high ice contents. The mineral composition consists of rock-forming quartz, orthoclase, and plagioclase of the light fraction (92–97%); accessory rutile, apatite, zircon, and sporadic Cr-spinel; sericite and montmorillonitic clay minerals. Yedoma deposits are non-saline and have 7–10 pH (Lupachev and Gubin, 2012); mean TOC (total organic carbon) is 3.0 + 1.6/–2.2 wt% (Strauss et al., 2013).
Samples were prepared by the replica technique that enables studying the microstructure of finely dispersed deposits and involves minimal disturbance to their morphology by forming a thin plastic film on the sample surface (Bates and Comer, 1955). The authors have modified the replica technique to investigate the frozen ground in the scanning electron microscope (Rogov and Kurchatova, 2013). Currently, replicas for SEM imaging are made as follows. A frozen specimen is split in the cooling chamber at a negative temperature, and its fresh cleavage plane is coated with polystyrol dissolved in dichloroethane. Then, the specimen is exposed to a specified negative temperature until the solvent evaporates and thaws after drying. The plastic film with the surface print (impression replica) is removed mechanically from the thawed specimen, rinsed in distilled water, and examined under optical or scanning electron microscopes in the laboratory (i.e., at room temperature). The replica technique allows for the study shapes and sizes of particles and aggregates, their surface microtopography, and qualitative and quantitative relations among the components of frozen ground in the initial undisturbed state.
The samples were sputter-coated with gold in a Quorum Q150R S an automatic rotary pumped coater and examined with TM3000 (Hitachi) scanning electron microscope equipped with an energy-dispersive X-ray system SwiftED3000 (Oxford). Determination of the composition of authigenic minerals in replicas was carried out in the Earth Cryosphere Institute, Tyumen Scientific Center of the Siberian Branch of the Russian Academy of Sciences (SB RAS).
The microstructure formation in perennially frozen ground results in their deposition and freezing conditions, including grain size, mineralogy, water content, water chemistry, temperature, and time of freezing (during or after deposition). The sediment particles within a seasonal freezing and thawing layer acquire a particular morphology during diagenesis due to the exposition to cryogenic deformation (frost cracking). The types of microstructure in seasonally and perennially frozen ground control their cryostructures.
The sediments commonly freeze in wet conditions and contain mostly basal-type pore ice and waved microlenses of segregated ice that sometimes are difficult to distinguish. Latent lenticular microstructure of samples from the Zelyony Mys site has been observed using SEM (Figure 2A). The well-preserved diatoms valves, which indicated in situ deposition in the polygonal ponds or thermokarst lakes, were found in these samples. Segregated ice can form only due to the expansion of the soil skeleton when the bonds between mineral particles break down (dilatancy). Since the breakage follows weak zones, the resulting voids represent the pattern of particles and their relationships, i.e., the types of microstructure. Ice contents of Yedoma silt usually increase from latent lenticular microstructure to a braided one (Figure 2B). Silt preserves elements of the primary sedimentary structure; however, some mineral grains are reoriented, displaced, and aggregated. The structure patterns are the most prominent in the layers, with high percentages of silt and plant remaining (Figure 2C). The silt unit from the Kolymskoe site has high ice content: lenses of segregated ice formed in diverse ways. Ice crystals are platy in thin lenses and become purer upward in thick bands, indicating freezing from the surface. The silt unit comprises sand-, silt-, or clay-size particles, sometimes cemented with mud and organic material or silt particles cemented with iron and plant remnants. The particle density between ice layers increases by cyclic freezing; their contacts change from volumetric to point-like and by coagulation and cementation, they form circular features (Yershov, 1998). Poorly decomposed fine in situ roots are pervasive.
FIGURE 2. Microstructure of Ice Complex deposits: (A,B) latent lenticular microstructure, Zelyony Mys site, sampled at 35 m arl; (C,D) braided microstructure, Plakhinskii Yar site, sampled at 12 m arl; (E,F) irregular reticulate microstructure, Kolymskoe site, sampled at 25 m arl. arl = above river level.
Different types of water freeze up successively during cyclic freezing of silt sediments and syncryogenic stratum formation; aqueous solutions acquire more complex compositions, and authigenic minerals precipitate. The following types of secondary carbonates have been observed under a SEM-EDX study in the Sartan Yedoma Ice Complex deposits from the Kolyma Lowland:
1) Calcium carbonate (CaCO3) polymorphs are characterized by parallel intergrowth and polysynthetic twinning of scalenohedral to rhombohedral calcite crystals (Figures 3A,B); radiated rosette-like aggregate and twinning of aragonite with a prismatic or acicular (needle-like) habit of crystals (Figures 3C,D). CaCO3 aggregates have a size ranging from 50 to 100 μm on average and are located in ice layers or at contacts with mineral grains, with coarser sizes of crystals in larger aggregates.
2) Isomorphic series of manganocalcite (Ca,Mn)CO3 (Mn: Ca = 1 : 1) and Ca-rhodochrosite (MnCO3, with up to 17% Са) look like columnar intergrowth in ice layers (Figure 3E) or druses on the surface of mineral grains (Figure 3F). Unlike the calcium carbonate polymorphs, the grains in parallel-columnar aggregates are uneven in size (decreasing toward one end), and some platy crystals have granular surfaces. Ca-rhodochrosite aggregates are sporadic and more often appear as small druses on mineral surfaces.
3) Carbonate compounds of alternating compositions have high Fe, Al, and Si (Figures 4A,B) and films around mineral grains, cement in aggregates, or amorphous flakes, often with metal impurities (Zn).
4) Siderite (FeCO3), as colloidal spherules, are commonly located at the contact with grassroots or moss remains and also around fungal hyphae (Figure 4C); early sediment alteration produces siderite concretions (Figure 4D).
FIGURE 3. Morphology of the authigenic carbonates under SEM-EDX. El = element; W% = weight percent; σ% = error. (A) Parallel intergrowth of calcite crystals, Kur'ishka site, sampled at 17 m arl; (B) polysynthetic twinning of rhombohedral calcite crystals, Duvanny Yar site, sampled at 36 m arl; (C) rosette-like aragonite aggregate, Kur'ishka site, sampled at 19 m arl; (D) twinning of acicular and prismatic aragonite crystals, Kolymskoe sampled at 25 m arl; (E) columnar manganocalcite intergrowth, Kur'ishka site, sampled at 21 m arl; (F) Ca-rhodochrosite druse, Kur'ishka site, sampled at 29 m arl
FIGURE 4. Cryometamorphic features: (A) Fe-colloidal film on mineral grain surface, Kur'ishka site, sampled at 15 m arl; (B) aggregate cement, Plakhinskii Yar site, sampled at 12 m arl; (C) colloidal siderite around fungal hyphae, Zelyony Mys site, sampled at 35 m arl; (D) siderite concretion, Zelyony Mys site, sampled at 35 m arl; (E) amorphous iron sulfide (FeSam), Kur'ishka site, sampled at 21 m arl; (F) octahedral greigites coating a silt particle, Kur'ishka site, sampled at 21 m arl.
Iron sulfides in the form of amorphous FeSam and crystalline greigites (FeS∙F2S3) were observed in the samples from the Kur’ishka site as an indicator of anaerobic environments (Figures 4E,F). FeSam has a characteristic microstructure, consisting of several μm large aggregates of curved, wrinkled sheets. These sheets are coated by completely amorphous material, the wavy, fluffy surface of which can also be observed in high-resolution SEM images. Greigite forms irregularly shaped aggregations on the mineral surface coated by the colloidal film.
Mineral grains in subaerial sediments subject to cryogenesis during cyclic freezing and thawing experience physical, chemical, and biochemical changes under the effect of pore moisture phase transitions. The features of deposits in such conditions shape up when mineral grains, especially quartz, break upon freezing (Konishchev, 1982; Konishchev and Rogov, 1993; Schwamborn et al., 2012). Finer sediments have larger surface areas of grain contacts with water and related physical and chemical interactions. Cyclic freezing-thawing accelerates chemical weathering because the migration of bound water toward the freezing front and subsequent melting of segregation ice maintain high water reactivity at low temperatures and generally decrease the contents of free water in pore fluids.
The lower part of the active layer in moistened tundra landscapes has an anaerobic (reducing) composition and low рН. Hydrolysis, which is especially rapid in layered and framework silicates, leads to concurrent chemical weathering of sediments and ionic decomposition of water (Oelkers and Schott, 1995; Tranter, 2003):
anorthite weathered feldspar
The H+ ions released by the water dissociation enter the lattices of newly formed clay particles, while the OH− and silica groups interact to produce silicic acid. Furthermore, some H2O molecules react with dissolved atmospheric carbon dioxide and provide
Stage I: рН increases slightly to neutral values due to the high contents of СО2 at the near-zero temperature. Freezing under a closed system imparts a concentration of solutes in the residual water, leading to an increase in calcite saturation index. Calcium precipitates from the aqueous solution with high contents of СО2 as calcite on the thermodynamic barrier. Precipitation of carbonates upon freshwater freezing (with the ensuing calcitization) is a common process in permafrost: for example, up to 0.5–2.0 kg/m2 of salts (mainly CaCO3) form upon aufeis fields after ice melting (Lacelle et al., 2009; Fotiev, 2009). As noted, calcites are presented by the parallel aggregates often located along the ice layer and also by polysynthetic twins that are typical for them. Crystals are easily recognized by the rhombohedral and scalenohedral habits.
The precipitation of calcite increases relatively the contents of Mg2+ in free water during freezing (Anisimova, 1981), which is favorable for the formation of aragonite, another calcium carbonate polymorph. As calcite growth rates decrease, aragonite growth rates stay constant and this phase becomes the dominant mineral phase in solutions with a high Mg/Ca ratio (up to five against 0.25 in freshwater) and low supersaturation (De Choudens-Sanchez and Gonzalez, 2009). Crystals show a prismatic or acicular (needle-like) habit as is usually displayed for inorganic origin. Rosette- and sheaf-like aragonite clusters prevail.
Furthermore, freezing in a closed system can produce manganocalcite with ∼1 : 1 Са: Mn and finally Ca-rhodochrosite, as Са2+ becomes isomorphically substituted by manganese. The process accelerates as the amount of free water reduces, resulting in smaller sizes of mineral grains. The accumulation of manganese is fairly widespread in the form of authigenic carbonates of complex composition in the Pleistocene-Holocene sediments of shelf-continental lithogenesis (Kuleshov, 2016).
Thus, the calcium carbonates that form at the first stage of freezing make up the series “calcite—aragonite—manganocalcite—Ca rhodochrosite.” The redistribution of soluble compounds takes place during the ice segregation in freezing grounds. The mobile components are accumulated on the interface of a growing ice lens. A part of soluble substances transforms into fixed forms in zones of cryoconcentration.
Stage II: freezing involves pore fluids with complex organic-mineral compounds (low-molecular complexes of iron with fulvic acid, silica gel, Mn hydroxides, and colloidal clay minerals). Migration of bound water and desorption of collomorph phases produce clay films on grain surfaces and cause cementing of clasts with Fe-Al-carbonate material. Locally, Ba, Zn, Co, and other elements become adsorbed on geochemical barriers of clay and, possibly, Mn hydroxides in compositionally complex flake-like amorphous phases. Lower crystallization temperatures of organic-mineral complexes are indicated by the presence of NaCl compounds entrapped from freezing solutions.
The Kolyma Ice Complex deposits contain an assemblage of iron sulfides with manganic calcite cement, which were also discovered in the central Yakutian permafrost (Siegert, 1981). SEM images reveal two varieties (Figures 4E,F, respectively): aggregates of amorphous sulfide (FeSam), possibly, sheet-like features as mackinawite-type structural elements that can form early during precipitation of colloids in reduced conditions (Csákberényi-Malasicsa et al., 2012), and octahedral greigite (FeS∙F2S3) crystals.
Stage III: further freezing affects high-molecular solutions in living cells, especially fungal hyphae. Several fungi can produce organic acids with chelating properties; as a result of the interaction between oxalic acid and cations, crystals of metal oxalates are produced (Baran, 2016). Therefore, fungi usually induce drastic changes in the microenvironment near their hyphal network. For instance, a modification of pH is an essential environmental factor influencing the redox state and the stability of iron ions and their solubility. Indeed, according to laboratory experiments (Varadachari et al., 1994), oxalic acid produces insoluble salts only reacting with divalent cations such as Ca2+, while oxalates from the reaction with trivalent cations result in soluble salts. Only Fe3+ can react with oxalic acid, which could be why fungal iron oxalate is rarely found in soils, even though fungi produce high amounts of oxalic acid and iron is the most abundant metal on Earth (Comensoli et al., 2017).
Authigenic carbonates as secondary phases in the Ice Complex deposits are remarkable by local changes in chemical, physical, and other properties, which induce gradual changes in the lattice and conversion of one mineral species to another. As a result, the sediments may contain stable and metastable minerals. Crystalline species like calcite or aragonite precipitated from solutions and their presence is restricted to free pore space in segregation ice. Metastable phases commonly form aggregates and polysynthetic twins, which reflect the freezing kinetics and the contrasting properties of the growth medium.
1) The Kolyma Yedoma Ice Complex that was formed during the Sartan Cryochrone (MIS 2), the coldest period of the Late Pleistocene, contains authigenic carbonates of several generations: polymorphic (calcite and aragonite) and isomorphic (manganocalcite, rhodokhrosite, and siderite) calcium carbonates and complex Fe-Al compounds.
2) Growth of authigenic mineral aggregates is constrained by the interstitial space and the thickness of ice lenses and is generally consistent with the predominance of silt-size grains. The morphology of new phases depends on the rate of pore moisture freezing; Ca carbonate crystals are the largest (50–100 μm on average). Secondary carbonates are represented by polysynthetic twins, parallel intergrowth, and druses or aggregates.
3) Cryogenesis leads to irreversible changes in the structure and composition (cryometamorphism) of sediments and the formation of metastable hydrates or colloids in anoxic areas of the active layer (closed system). The freezing sediments change in several successive stages: cryoconcentration of the residual solutions of pore water; migration of bound water and desorption of organic-mineral complexes; Ca-rich silicate weathering.
The original contributions presented in the study are included in the article/Supplementary Material; further inquiries can be directed to the corresponding author.
AK and VR were responsible for sample preparation, analysis of the results, and writing of the article. NT contributed to laboratory research and technical support.
The authors declare that the research was conducted in the absence of any commercial or financial relationships that could be construed as a potential conflict of interest.
All claims expressed in this article are solely those of the authors and do not necessarily represent those of their affiliated organizations, or those of the publisher, the editors and the reviewers. Any product that may be evaluated in this article, or claim that may be made by its manufacturer, is not guaranteed or endorsed by the publisher.
The Supplementary Material for this article can be found online at: https://www.frontiersin.org/articles/10.3389/feart.2021.718904/full#supplementary-material
Anisimova, N. P. (1981). Cryological and Hydrochemical Features of Permafrost. Novosibirsk: Nauka, 152. (in Russian).
Arkhangelov, A. A., Rogov, V. V., and Lyanos-Mas, A. V. (1979). Cryostratigraphy and Facies of the Duvan Yar Ice Complex. Problemy Kriolitologii VIII, 145–157. (in Russian).
Baran, E. J. (2016). Natural Iron Oxalates and Their Analogous Synthetic Counterparts: A Review. Geochemistry 76, 449–460. doi:10.1016/j.chemer.2016.06.005
Bates, T. F., and Comer, J. J. (1955). Electron Microscopy of clay Surfaces. Clays Clay Miner 3, 1–25. doi:10.1346/ccmn.1954.0030102
Becze-Deák, J., Langohr, R., and Verrecchia, E. P. (1997). Small Scale Secondary CaCO3 Accumulations in Selected Sections of the European Loess belt. Morphological Forms and Potential for Paleoenvironmental Reconstruction. Geoderma 76, 22. doi:10.1016/S0016-7061(96)00106-1
Buggle, B., Glaser, B., Hambach, U., Gerasimenko, N., and Marković, S. (2011). An Evaluation of Geochemical Weathering Indices in Loess-Paleosol Studies. Quat. Int. 240, 12–21. doi:10.1016/j.quaint.2010.07.019
Butler, I. B., Rickard, D., and Grimes, S. (2000). “Framboidal Pyrite: Self-Organisation in the Fe-S System,” in Journal of Conference Abstracts, 5, 276–277.
Comensoli, L., Bindschedler, S., Junier, P., and Joseph, E. (2017). Iron and Fungal Physiology. Adv. Appl. Microbiol. 98, 31–60. doi:10.1016/bs.aambs.2016.11.001
Csákberényi-Malasics, D., Rodriguez-Blanco, J. D., Kis, V. K., Rečnik, A., Benning, L. G., and Pósfai, M. (2012). Structural Properties and Transformations of Precipitated FeS. Chem. Geology 294-295, 249–258. doi:10.1016/j.chemgeo.2011.12.009
Curtis, C. D. (1990). Aspects of Climatic Influence on the clay Mineralogy and Geochemistry of Soils, Palaeosols and Clastic Sedimentary Rocks. J. Geol. Soc. 147 (2), 351–357. doi:10.1144/gsjgs.147.2.0351
Davidson, E. A., and Janssens, I. A. (2006). Temperature Sensitivity of Soil Carbon Decomposition and Feedbacks to Climate Change. Nature 440, 165–173. doi:10.1038/nature04514
De Choudens-Sanchez, V., and Gonzalez, L. A. (2009). Calcite and Aragonite Precipitation under Controlled Instantaneous Supersaturation: Elucidating the Role of CaCO3 Saturation State and Mg/Ca Ratio on Calcium Carbonate Polymorphism. J. Sediment. Res. 79 (6), 363–376. doi:10.2110/jsr.2009.043
D’Hondt, S., Inagaki, F., Zarikian, C. A., Abrams, L. J., Dubois, N., Engelhardt, T., et al. (2015). Presence of Oxygen and Aerobic Communities from Sea Floor to Basement in Deep-Sea Sediments. Nat. Geosci. 8, 299–304. doi:10.1038/ngeo2387
Fotiev, S. M. (2009). Cryometamorphism of Rocks and Groundwaters: Results and Conditions. Novosibirsk: Geo, 279. (in Russian).
Kaplina, T. N. (2011). Ancient Alas Complexes of Northern Yakutia. Kriosfera Zemli XV (2), 3–13. (in Russian).
Keys, J. R., and Williams, K. (1981). Origin of Crystalline, Cold Desert Salts in the McMurdo Region, Antarctica. Geochimica et Cosmochimica Acta 45 (12), 2299–2309. doi:10.1016/0016-7037(81)90084-3
Kolesár, M., and Čurlik, J. (2015). Origin, Distribution and Transformation of Authigenic Carbonates in Loessic Soils. Ejss 4 (1), 38–43. doi:10.18393/ejss.50910
Konishchev, V. N. (1982). Characteristics of Cryogenic Weathering in the Permafrost Zone of the European USSR. Arctic Alpine Res. 14 (3), 261–265. doi:10.2307/1551158
Konishchev, V. N., and Rogov, V. V. (1993). Investigations of Cryogenic Weathering in Europe and Northern Asia. Permafrost Periglac. Process. 4, 49–64. doi:10.1002/ppp.3430040105
Kuleshov, V. N. (2016). in Isotope Geochemistry: The Origin and Formation of Manganese Rocks and Ores. Editor J. B. Maynard (Amsterdam: Elsevier), 5–62. doi:10.1016/B978-0-12-803165-0.00002-1
Lacelle, D., Lauriol, B., and Clark, I. D. (2009). Formation of seasonal cave ice and associated cryogenic carbonates in Caverne de l'Ours, Quebec, Canada. Kinetic isotope effects and pseudo-biogenic crystal structures. J. Cave Karst Stud. 71 (1), 48–62.
Lupachev, A. V., and Gubin, S. V. (2012). Suprapermafrost Organic-Accumulative Horizons in the Tundra Cryozems of Northern Yakutia. Eurasian Soil Sc. 45, 45–55. doi:10.1134/s1064229312010115
Murton, J. B., Goslar, T., Edwards, M. E., Bateman, M. D., Danilov, P. P., Savvinov, G. N., et al. (2015). Palaeoenvironmental Interpretation of Yedoma Silt (Ice Complex) Deposition as Cold-Climate Loess, Duvanny Yar, Northeast Siberia. Permafrost Periglac. Process. 26 (3), 208–288. doi:10.1002/ppp.1843
Oelkers, E. H., and Schott, J. (1995). Experimental Study of Anorthite Dissolution and the Relative Mechanism of Feldspar Hydrolysis. Geochimica et Cosmochimica Acta 59 (24), 5039–5053. doi:10.1016/0016-7037(95)00326-6
Ostroumov, V., Ostroumova, N., Sorokovikov, V., Hoover, R., Van Vliet-Lanoë, B., and Siegert, Ch. (2001). Redistribution of Soluble Components during Ice Segregation in Freezing Ground. Cold Regions Sci. Tech. 32 (2-3), 175–182. doi:10.1016/S0165-232X(01)00031-3
Rogov, V. V., and Kurchatova, A. N. (2013). Method of Manufacturing Replica for Analyses of Microstructure of Frozen Rocks in Scanning Electron Microscope. RU Patent 2528256 C1. Available at: http://www.freepatent.ru/images/img_patents/2/2528/2528256/patent-2528256.pdf.
Schwamborn, G., Schirrmeister, L., Frütsch, F., and Diekmann, B. (2012). Quartz Weathering in Freeze-Thaw Cycles: experiment and Application to the El'gygytgyn Crater lake Record for Tracing Siberian Permafrost History. Geografiska Annaler: Ser. A, Phys. Geogr. 94 (4), 481–499. doi:10.1111/j.1468-0459.2012.00472.x
Siegert, Kh. G. (1981). “Mineral Formation in Permafrost,” in Structure and Thermal Regime of Permafrost (Novosibirsk: Nauka), 14–21. (in Russian).
Strauss, J., Schirrmeister, L., Grosse, G., Wetterich, S., Ulrich, M., Herzschuh, U., et al. (2013). The Deep Permafrost Carbon Pool of the Yedoma Region in Siberia and Alaska. Geophys. Res. Lett. 40 (23), 6165–6170. doi:10.1002/2013GL058088
Tranter, M. (2003). “Geochemical Weathering in Glacial and Proglacial Environments,” in Holland, H.D., Turekian, K.K. (Eds.), Treatise on Geochemistry: Surface and Ground Water, Weathering, and Soils. Elsevier, pp. 189–205. doi:10.1016/b0-08-043751-6/05078-7
Turchyn, A. V., Bradbury, H. J., Walker, K., and Sun, X. (2021). Controls on the Precipitation of Carbonate Minerals within marine Sediments. Front. Earth Sci. 9. doi:10.3389/feart.2021.618311
Varadachari, C., Barman, A. K., and Ghosh, K. (1994). Weathering of Silicate Minerals by Organic Acids II. Nature of Residual Products. Geoderma 61, 251–268. doi:10.1016/0016-7061(94)90052-3
Vasil’chuk, Y. K. (2005). Heterochroneity and Heterogeneity of the Duvanny Yar Edoma. Doklady Earth Sci. 402, 568–573. doi:10.1594/PANGAEA.919525
Veremeeva, A., Nitze, I., Günther, F., Grosse, G., and Rivkina, E. (2021). Geomorphological and Climatic Drivers of Thermokarst Lake Area Increase Trend (1999-2018) in the Kolyma Lowland Yedoma Region, North-Eastern Siberia. Remote Sensing 13, 178. doi:10.3390/rs13020178
Keywords: Yedoma Ice Complex, replica techniques, authigenic carbonates, Sartan Cryochrone (MIS 2), the Kolyma Lowland
Citation: Rogov VV, Kurchatova AN and Taratunina NA (2021) Types and Micromorphology of Authigenic Carbonates in the Kolyma Yedoma Ice Complex, Northeast Siberia. Front. Earth Sci. 9:718904. doi: 10.3389/feart.2021.718904
Received: 01 June 2021; Accepted: 21 September 2021;
Published: 27 October 2021.
Edited by:
Lutz Schirrmeister, Alfred Wegener Institute Helmholtz Centre for Polar and Marine Research (AWI), GermanyReviewed by:
Sebastian Wetterich, Alfred Wegener Institute Helmholtz Centre for Polar and Marine Research (AWI), GermanyCopyright © 2021 Rogov, Kurchatova and Taratunina. This is an open-access article distributed under the terms of the Creative Commons Attribution License (CC BY). The use, distribution or reproduction in other forums is permitted, provided the original author(s) and the copyright owner(s) are credited and that the original publication in this journal is cited, in accordance with accepted academic practice. No use, distribution or reproduction is permitted which does not comply with these terms.
*Correspondence: Anna N. Kurchatova, a2FubmlAbWFpbC5ydQ==
Disclaimer: All claims expressed in this article are solely those of the authors and do not necessarily represent those of their affiliated organizations, or those of the publisher, the editors and the reviewers. Any product that may be evaluated in this article or claim that may be made by its manufacturer is not guaranteed or endorsed by the publisher.
Research integrity at Frontiers
Learn more about the work of our research integrity team to safeguard the quality of each article we publish.