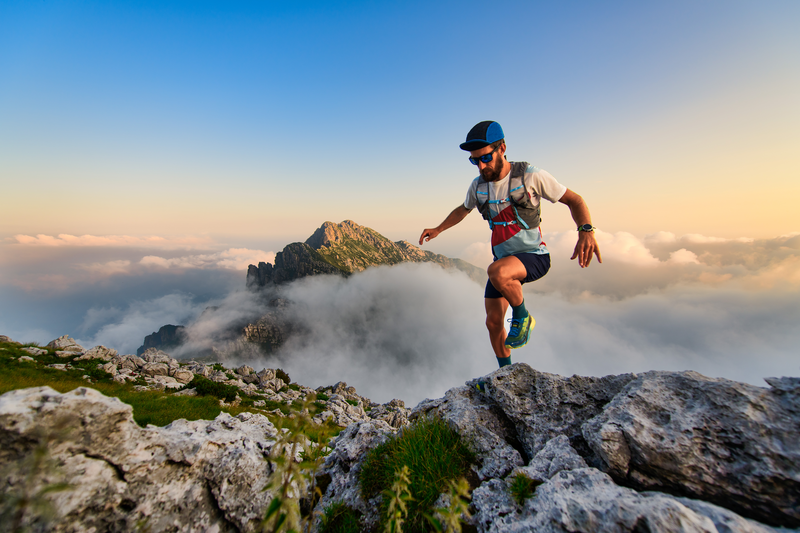
94% of researchers rate our articles as excellent or good
Learn more about the work of our research integrity team to safeguard the quality of each article we publish.
Find out more
ORIGINAL RESEARCH article
Front. Earth Sci. , 04 August 2021
Sec. Quaternary Science, Geomorphology and Paleoenvironment
Volume 9 - 2021 | https://doi.org/10.3389/feart.2021.717720
This article is part of the Research Topic Tibetan Plateau Uplift and Environmental Impacts: New Progress and Perspectives View all 28 articles
Stable isotope analysis is pivotal for investigating the paleodiet and paleoecology of ancient mammals. Recently, a ∼9-Myr-old Hipparion fauna was discovered at an elevation of ∼2,200 m above sea level (a.s.l.) in the Xunhua Basin on the northeastern Tibetan Plateau. These fossils can provide key evidence for the ecosystem structure and regional paleoenvironmental reconstruction. Here we present carbon and oxygen isotopic compositions of 56 bulk and 85 serial tooth enamel samples from this fauna, which includes Hipparion platyodus Selve, Chilotherium sp. and Gazella gaudryi Schlosser. The enamel−δ13°C values display a wide range of variation (−11.4 to −5.0‰), with a mean value of −8.9 ± 1.0‰, indicating that the fauna consumed mainly C3 plants. However, the heaviest δ13C value of Hipparion (−5.0‰) suggests at least some consumption of C4 plants. Combined with pollen records, we infer that the Hipparion fauna in the Xunhua Basin was living in forest-grassland setting at ∼9 Ma. The reconstructed paleo-meteoric δ18O values of the Xunhua Basin at ∼9 Ma are lower than the mean annual δ18O of present-day precipitation in this region, suggesting a wetter climate or stronger monsoonal influence than today. Hipparion fauna in the Xunhua Basin yield significantly higher mean values of δ13C and δ18O than contemporary fossils in the Linxia Basin to the east, which is attributed to rapid uplift of the Jishi Shan, blocking water vapor transport by the East Asian Summer Monsoon and making the climate of the Xunhua Basin relatively drier.
Cenozoic sedimentary basins of the Tibetan Plateau have yielded abundant fossil mammals, whose reconstructed diets can provide valuable information about paleoenvironmental and paleoclimatic changes. Given the resistance of fossil mammal teeth to diagenetic alteration due to their high density and low porosity (Ayliffe et al., 1994; MacFadden and Cerling, 1994; Wang et al., 1994), the stable carbon and oxygen isotopic compositions of tooth enamel have proven to be a useful tool for inferring climate and environmental conditions such as vegetation cover, aridity, precipitation sources, and climate seasonality in the past (Cerling et al., 1993; Cerling et al., 1998; Levin et al., 2006; Wynn et al., 2012; Sun et al., 2021).
In recent decades, many stable isotope studies have been conducted on fossil materials from Miocene to Pliocene strata of the Tibetan Plateau (Wang and Deng, 2005; Biasatti et al., 2010; Biasatti et al., 2012; Biasatti et al., 2018; Zhang et al., 2012a; Deng et al., 2012; Ciner et al., 2015). Previous studies have focused mostly on issues of ecosystem structure and regional paleoenvironmental reconstruction. For instance, Zhang et al. (2012a) inferred that the presence of significant C4 biomass in the Qaidam Basin between 9.5 and 5 Ma signified a wetter and warmer climate. Wang and Deng (2005) suggested that herbivores in the Linxia Basin fed predominantly on C3 plants before 2.5 Ma, and that a positive shift in both horse and rhino bulk δ18O recorded a shift to more arid conditions at 9.5 Ma.
The Xunhua Basin is located on the northeastern Tibetan Plateau in a climatically sensitive area, representing the transitional zone between the monsoonal coastal region to the east and the arid continental interior to the west. Much research has been conducted on climate change in the Xunhua Basin using stable isotopes (Hough et al., 2011), magnetostratigraphy (Ji et al., 2010), palynological data (Xu et al., 2015), ostracod data (Luo et al., 2010), clay mineralogy (Wang et al., 2010), and chemical weathering indices (Ye et al., 2010). Palynological studies in the Xunhua Basin indicate a long-lasting cooling trend beginning at ∼14 Ma. However, clay mineralogy and chemical weathering indices from the Xunhua Basin indicate arid conditions between 14.6 and 12.5 Ma, a relatively wetter stage between 12.5 and 8 Ma, and renewed climatic drying at 8 Ma. Thus, paleoclimate changes in the Xunhua Basin during the Late Miocene remain contentious.
Recently, we discovered an upper Miocene Hipparion fauna (∼9 Ma) in the Xunhua Basin at an elevation of ∼2,200 m a.s.l. Mammal tooth enamel forms incrementally from the crown to the base of the tooth, recording variation in δ13C and δ18O values over time. Such isotopic series can provide excellent records of seasonal variation in diet and climate during tooth growth (Koch et al., 1995; Fricke and O’Neil, 1996; Fricke et al., 1998; Sharp and Cerling, 1998; Nelson, 2005; Sponheimer et al., 2006; Wang et al., 2008a). Stable carbon and oxygen isotopic analyses of our newly discovered fossil mammal teeth in the Xunhua Basin can provide not only useful proxies for reconstructing paleodiets and paleoecology but also a baseline for regional comparison with well-studied Hipparion faunas from the Linxia Basin (Wang and Deng, 2005; Biasatti et al., 2010, 2018) with the goal of better understanding the history of regional environmental and ecosystem changes on the northeastern Tibetan Plateau and their relationship to tectonics and global climate.
The Xunhua Basin, with an elevation between 1870 and 3,000 m, is surrounded by the east-west-trending Laji Shan to the north, the north-south-trending Jishi Shan to the east, and the Zhamazari and West Qinling Shan to west and south, respectively (Figures 1A,B). The basin is located in middle latitudes, in a semi-arid region in which mean annual temperature is ∼8.5°C and mean annual precipitation is 264 mm, of which ∼75% falls as monsoonal rain during the summer (IAEA/WMO, 2018).
FIGURE 1. Geological sketch maps showing the location and tectonic setting of the study area: (A) Location of the study area on Tibetan Plateau (modified from Yin and Harrison, 2000); (B) Tectonic sketch of Xunhua Basin; and (C) Detailed geological map of the Xunhua Basin (modified from Zhang et al., 2010) and the location of the measured section.
The Xigou section (starting coordinates, N 35°49′30″, E 102°30′00″, 1797 m; ending coordinates, N 35°48′18″, E 102°26′10″, 2,344 m), located in the southeastern Xunhua Basin (Figure 1C), has well-exposed Miocene to Pliocene strata with an overall thickness of more than 600 m, deposited without significant hiatuses (Figure 2). Our previous magnetostratigraphic study has determined a depositional age for this section of 14.6 to 5.0 Ma (Ji et al., 2010), with the following approximate formation ages: Dongxiang Formation (14.6–9.6 Ma), Liushu Formation (9.6–7.3 Ma), and Hewangjia Formation (7.3–5.0 Ma) (Figure 2). Many fossil mammals were collected at the base of the Liushu Formation within a 1-m-thick interval at 378–379 m, including Hipparion platyodus Selve (Figures 3A–E), Chilotherium sp. (Figures 3F–J), Gazella gaudryi Schlosser (Figures 3K–N), Gazella paotehensis, Gazella dorcadoides, Gazella sp., and Cervocerus sp. Based on the magnetostratigraphy of the Xigou section (Ji et al., 2010), the Hipparion fauna in the Xunhua Basin was found within chron C4An (Figure 2) and has an estimated age of 8.99–9.06 Ma based on linear interpolation between age tie-points (i.e., assuming a constant sedimentation rate). Because this age range is narrow and beyond the precision of our study, we herein report the age of the Hipparion fauna as ∼9 Ma.
FIGURE 2. Lithology, sedimentary facies and magnetostratigraphic framework of the Xigou section in the Xunhua Basin. Dashed lines show the correlations of the observed magnetic polarities (Ji et al., 2010) with the geomagnetic polarity time scale (GPTS; Ogg, 2012).
FIGURE 3. The segmental cheek teeth for enamel carbon and oxygen isotopic compositions from Xigou section in the Xunhua Basin. Scale bar = 2 mm; 1 = coronal views; 2 = side views. (A)Hipparion platyodus Selve, CUG-XH-1, lower teeth, right M3; (B)Hipparion platyodus Selve, CUG-XH-2, lower teeth, right M1; (C)Hipparion platyodus Selve, CUG-XH-3, upper teeth, left M1; (D)Hipparion platyodus Selve, CUG-XH-4, upper teeth, left M2; (E)Hipparion platyodus Selve, CUG-XH-5, upper teeth, left M2; (F–G) Tooth fragment of Chilotherium sp., and their number are CUG-XH-28, CUG-XH-29, CUG-XH-30, CUG-XH-31, CUG-XH-32, respectively; (K)Gazella gaudryi Schlosser, CUG-XH-39, left mandible; (L)Gazella gaudryi Schlosser, CUG-XH-42, right mandible; (M)Gazella gaudryi Schlosser, CUG-XH-47, left mandible; (N)Gazella gaudryi Schlosser, CUG-XH-51, left mandible.
We selected 56 well-preserved fossils of herbivorous mammals including Hipparion platyodus Selve, Chilotherium sp., and Gazella gaudryi Schlosser for stable carbon and oxygen isotope analyses. We preferentially analyzed molars and premolars to overcome paleoecological bias from juvenile development (Bryant et al., 1996). However, many specimens of the fossil Chilotherium that we analyzed were so fragmentary that to identify them to genus was difficult. The tooth samples were prepared by scraping any dirt, dentine, or other material off the enamel. For bulk enamel samples, fine powder was drilled from a cleaned enamel surface evenly along the entire growth axis to make the sample more representative of the entire period of mineralization. In order to study whether local vegetation exhibited seasonal variations, serial enamel samples were obtained from nine selected Hipparion teeth by drilling in bands perpendicular to the growth axes of each tooth from crown to root with a rotary tool.
The enamel powder was then soaked in 5% sodium hypochlorite (NaOCl) to remove organic matter, followed by treatment with 1 M acetic acid for at least 12 h to remove non-structural carbonate, cleaning with distilled water via centrifugation at least three times, and finally freeze-drying. The enamel samples were the converted to CO2 by reaction with 100% phosphoric acid for approximately 72 h at 25°C; the carbon and oxygen isotope ratios of the resulting CO2 were then analyzed using a Finnigan MAT Delta Plus XP stable isotope ratio mass spectrometer (IRMS) at the University of Rochester’s SIREAL laboratory (Higgins and MacFadden, 2004). The analytical results are reported using the standard ‰ notation where δ13C (or δ18O) = (Rsample/Rstandard–1) × 1,000 and Rsample or Rstandard is the 13C/12C (or 18O/16O) ratio of the sample or standard, respectively. Results are reported relative to the VPDB reference standard. Analytical precision for δ13C and δ18O was better than ±0.1 and ±0.14‰, respectively.
The δ13C and δ18O of bulk enamel from 56 fossil teeth are reported in Figure 4 and Supplementary Table S1. Of the 56 teeth analyzed in this study, nine Hipparion teeth that were analyzed for bulk isotopes were serially sampled (Figure 5; Supplementary Table S2). There is no notable seasonal signal present in the carbon isotope data of the serial samples. The mean intra-tooth variation of enamel-δ13C values in these nine teeth is 0.9 ± 0.2‰. Sample XH-8 has intra-tooth variation of just 0.5‰, the lowest value, and samples XH-5 and XH-13 have intra-tooth variation of 1.2‰, the highest value. Intra-tooth variation is greater for δ18O (up to 4‰) than for δ13C. Serial sampling of Hipparion reveals a strong seasonal signal in oxygen isotope data from the Xunhua Basin, indicating that the enamel samples, and thus the carbon isotope data, have not been strongly affected by diagenesis (Zin Maung Maung Thein et al., 2011). Moreover, comparison of the δ13C and δ18O values of the fossil tooth enamel with those of carbonates in the coexisting matrix (Hough et al., 2011) provides a useful means of determining whether significant alteration of the original isotopic compositions of the enamel has occurred (Wang and Deng, 2005). A significant difference between the isotopic compositions of the enamel and matrix carbonates would argue against strong diagenetic alteration of the samples. Differences in δ13C and δ18O values between the analyzed fossil samples and their coexisting matrix carbonates (Δ13Ce-m and Δ18Oe-m) are plotted in Figure 4. The large Δ13Ce-m and Δ18Oe-m values are consistent with minimal or no diagenetic alteration of the tooth enamel of the Hipparion fauna in the Xunhua Basin.
FIGURE 4. δ13C and δ18O in tooth enamel of Hipparion fauna (including Hipparion, Chilotherium, and Gazzela) and their coexisting matrix carbonates (Hough et al., 2011) in the Xunhua Basin.
FIGURE 5. Intra-tooth isotopic variations in selected Hipparion teeth from Xunhua Basin. Δ13C and Δ18O values indicate the range of carbon and oxygen isotopic variations, respectively, within each tooth.
Bulk enamel−δ13C values range from −11.4 to −5.0‰ with a mean of −8.9 ± 1.0‰ (n = 56): 1) from −11.4 to −9.1‰ with a mean of −9.9 ± 0.75‰ (n = 18) for Gazella; 2) from −9.2 to −8.1‰ with a mean of −8.5 ± 0.35‰ (n = 12) for Chilotherium; 3) from −9.2 to −5.0‰ with a mean of −8.3 ± 0.86‰ (n = 26) for Hipparion. Only one fossil (Hipparion) has bulk δ13C values greater than −6‰, a conservative cutoff for the presence of C4 plants in the diets of fossil mammals (Bowman et al., 2017). When comparing our results with data from Wang and Deng (2005) and Biasatti et al. (2010), Biasatti et al. (2018) (Figure 6), we found that the taxon-specific mean δ13C values in the Xunhua Basin tend to be slightly higher than those in the Linxia Basin (Figure 7). In addition, we found that fossil enamel δ13C was characterized by higher-amplitude variation in the Xunhua Basin than in the Linxia Basin (Figures 4, 7).
FIGURE 6. Correlation of stratigraphic columns for the Xunhua and Linxia basins. Sources: Xunhua Basin (Ji et al., 2010), Linxia Basin (Wang and Deng, 2005).
FIGURE 7. Comparison of enamel δ13C, δ18O and the estimated δ18Ow values of obligate drinkers (Hipparion and Chilotherium) of Xunhua Basin at ∼9 Ma and the Linxia Basin at 9.5 to 9 Ma (Wang and Deng, 2005; Biasatti et al., 2010, 2018).
The fossils have bulk δ18O (VPDB) values of all tooth enamel ranging from −12.2‰ to −1.8‰ with a mean of −8.2 ± 1.8‰ (n = 56): 1) from −11.6 to −1.8‰ with a mean of −7.7 ± 2.52‰ (n = 18) for the Gazella; 2) from −10.7 to −7.4‰ with a mean of −8.4 ± 0.83‰ (n = 12) for the Chilotherium; 3) from −12.2 to −6.5‰ with a mean of −8.4 ± 1.4‰ (n = 26) for the Hipparion. The δ18O of tooth enamel is similar for all specimens of all taxa, with the exception of a single Gazella that yielded a value ∼1.8‰ more positive than other specimens (Figure 4). This difference may be attributable to differences in dietary or drinking behavior between this Gazella specimen and the other fossils (Biasatti et al., 2010). A difference in dietary behavior is distinctly possible as Gazella usually ingest a large amount of water from leaves, and leaf water is strongly affected by relative humidity (Cormie et al., 1994; Koch, 1998). As a result, leaf δ18O values will increase with climatic aridity. Compared to the Linxia Basin, the mean δ18O values of fossil enamel in the Xunhua Basin are higher (by ∼3.4‰) and the most negative δ18O values in the Xunhua Basin is lower than that in the Linxia Basin (Figure 7).
The carbon isotopic composition of mammal teeth closely follows an animal’s diet, reflecting consumption of two types of vegetation with differing isotopic signatures: C3 plants (i.e., trees, most shrubs, and cool-season grasses) with δ13C values of −34 to −23‰ (VPDB; mean −27‰) and C4 plants (i.e., warm-season grasses) with δ13C values of −17 to −9‰ (mean −13‰) (Quade et al., 1992). These differences in carbon isotopic composition are due to use of different photosynthetic pathways: the Calvin-Benson cycle for C3 plants versus the Hatch-Slack cycle for C4 plants. Mammal teeth are enriched in the heavy carbon isotope (13C) by ∼14‰ relative to their dietary source (Cerling and Harris, 1999), based on which enamel δ13C values of < −9‰ and > −2‰ indicate a pure C3 diet and a pure C4 diet, respectively, with mixed feeders yielding intermediate values (Lee-Thorp and van der Merwe, 1987; Koch, 1998; Cerling and Harris, 1999; Koch and Cerling, 2002; Passey et al., 2002). However, in arid and semi-arid (i.e., water-stressed) environments, a maximum enamel−δ13C value for a pure C3 diet could be as high as −8‰ for modern herbivores and −6‰ for fossil herbivores due to changes in the δ13C value of atmospheric CO2 (Bowman et al., 2017). In closed environments such as forests, δ13C values are systematically lower due to the influence of soil respiration and low irradiance (Schleser and Jayasekera, 1985; van der Merwe and Medina, 1989).
The mean δ13C value of enamel samples of the ∼9-Ma Hipparion fauna from the Xunhua Basin is −8.9 ± 1.0‰ (n = 56, Figure 4). The δ13C of atmospheric CO2 in the Late Miocene was approximately −6.4‰ (Tipple et al., 2010), which is higher than the current value of −8.2‰ (Cuntz, 2011). After correction for secular variation in the δ13C of atmospheric CO2 (1.8‰) and biochemical fractionation between diet and enamel (∼14‰), the dietary δ13C of the Hipparion fauna ranged from −27.2 to −20.8‰ (mean −24.7 ± 1.0‰), with the highest value found in a Hipparion specimen and the lowest in a Gazella. These values are consistent with a diet consisting mainly of C3 plants. Further proof of a lack of ingestion of C4 plants in this ecosystem comes from the serial enamel-δ13C data from individual teeth, which show little or no intra-tooth carbon isotopic variation (Figure 5). However, one Hipparion tooth yielded a relatively high dietary-δ13C value (−20.8‰), suggesting the presence of a small amount of C4 plants in the local ecosystem. Thus, the Hipparion fauna in the Xunhua Basin at ∼9 Ma fed mostly on C3 plants, even though the local ecosystem is likely to have contained some C4 grasses, which is consistent with previous observations that C4 plants were already present on the Tibetan Plateau and the Chinese Loess Plateau at 9–8 Ma (Morgan et al., 1994; Zhang et al., 2009, Zhang et al., 2012b).
Variation of dietary-δ13C values among taxa likely reflects differences in the herbivores’ habitats and eating behaviors (Figure 4). Gazella has the lowest δ13C values (range −27.2 to −24.9‰, mean 25.7 ± 0.75‰), suggesting that they had pure C3 diets and preferred forest habitats. In contrast, higher dietary-δ13C values are recorded by Hipparion (range −25.0 to −20.8‰, mean −24.1 ± 0.86‰) and Chilotherium (−25.0 to −23.9‰, mean −24.3 ± 0.35‰). These values are indicative of feeding mainly on C3 plants growing in more open environments, although some Hipparion specimens may have consumed a certain amount of C4 plants. The composition of the Hipparion fauna in the Xunhua Basin reflects an herbivore community adapted to both forest and grassland habitats. Gazella gaudryi Schlosser and Cervocerus sp. represent forest phenotypes, whereas Hipparion platyodus Selve, Chilotherium sp. and Gazella dorcadoides had high-crown teeth and were representative of grassland taxa (Zhang et al., 2002). Moreover, palynological data reveal that the Xunhua Basin contained mainly shrubs and herbs (e.g., Chenopodiaceae, Ephedra and Gramineae), broadleaved trees, and conifers at 12.5–8 Ma (Xu et al., 2015). Combined with enamel-δ13C data, the presence of the Hipparion fauna in the Xunhua Basin at ∼9 Ma is indicative of a mixed forest-grassland setting.
Oxygen isotopes in mammal tooth enamel contain valuable information about climatic conditions (Kohn and Cerling, 2002; Wang et al., 2008b; Wang et al., 2013). The δ18O values of tooth enamel can reflect the isotopic constitution of local meteoric water, which is strongly correlated with local climate conditions, especially for obligate drinkers (>100 kg) that obtain most of their water from meteoric sources (Longinelli, 1984; Luz et al., 1984; Bryant and Froelich, 1995; Wang et al., 2008a). Precipitation is known to display large intra-annual δ18O variations in response to climatic seasonality. Rivers and lakes exhibit a much smaller δ18O range than precipitation as their water is mostly derived from groundwater that integrates precipitation spatially and temporally across a watershed (Clark and Fritz, 1997). Precipitation isotope data from IAEA-GNIP (International Atomic Energy Agency Global Network for Isotopes in Precipitation) stations in the study area show that summer precipitation is more depleted in 18O than winter precipitation (Stacklyn et al., 2017; IAEA/WMO, 2018; Sun et al., 2019). This pattern is characteristic of the Asian Summer Monsoon regime (Johnson and Ingram, 2004). The weighted annual mean δ18O of precipitation in this region shows a decreasing trend with distance from the ocean (the moisture source): −5.8 ± 1.1‰ at Guangzhou, −6.2 ± 0.9‰ at Guilin, −6.5 ± 0.7‰ at Liuzhou, −6.4 ± 2.1‰ at Wuhan, and −7.0 ± 1.1‰ at Chengdu (Stacklyn et al., 2017; IAEA/WMO, 2018; Sun et al., 2019), which reflects a combination of the “continental effect” and the “latitude effect” (Dansgaard, 1964). In summer months, moisture was carried by the Asian summer monsoon from the ocean inland, increasing precipitation over the continent. Because heavy isotopes are preferentially removed from vapor by condensation, the remaining vapor in an air mass and the precipitation subsequently produced from it become progressively more depleted in the heavy isotope (18O) as air masses move further inland and more vapor condenses to precipitation (Dansgaard, 1964). In a given area, the higher the precipitation amount, the lower the δ18O value, owing to the “amount effect” (Dansgaard, 1964). At mid to high latitudes, there is a strong positive correlation (0.58‰/°C) between precipitation δ18O and surface air temperature (Dansgaard, 1964), the so-called “temperature effect”, but this correlation does not apply to the Asian monsoon region or to low-latitude regions in general (Sun et al., 2019).
The δ18O values of structural carbonate in the fossil enamel samples from the Xunhua Basin range from −12.2 to −1.8‰ (mean −8.2 ± 1.8‰; n = 56). The majority of specimens were large mammals (e.g., Hipparion, Chilotherium) that were presumably obligate drinkers. The δ18O values of enamel range from −12.2 to −6.5‰ (mean −8.4 ± 1.4‰) for Hipparion and from −10.7 to −7.4‰ (mean −8.4 ± 0.83‰) for Chilotherium. The similar δ18O values of Hipparion and Chilotherium indicate that they may have had a similar water source. Half of the Hipparion teeth shows limited δ18O variation (range −2.3 to −1.6‰, Figure 5), which likely indicates that these animals drank from isotopically buffered water sources such as rivers or lakes (Clark and Fritz, 1997). The other half displays relatively large intra-tooth variations (up to 4.5‰, Figure 5), possibly reflecting large seasonal variation in the δ18O of ingested water. Enamel samples from fossil Gazella were generally enriched in 18O with a wide range of δ18O values compared to other taxa (Figure 4), implying that Gazella migrated between forested and relatively open habitats and consumed leaf water or highly evaporated lake/pond water (Sun et al., 2021).
Previous studies have shown that the enamel-δ18O values of obligate drinkers are strongly correlated with the δ18Ow of local meteoric water (Kohn and Cerling, 2002; Levin et al., 2006; Wang et al., 2012). Applying the enamel-water δ18O relation for obligate drinkers given in Kohn and Cerling (2002), i.e., δ18Oenamle-CO3 = 0.9 × δ18Owater + 1.245, to enamel samples of Hipparion and Chilotherium from the Xunhua Basin yields a mean paleo-meteoric water δ18Ow value of −10.8 ± 1.38‰ (VSMOW, n = 38). However, the mean enamel-δ18O value may overestimate paleo-meteoric water δ18Ow due to subsequent evaporation induced δ18O enrichment. The best estimate of paleo-meteoric water δ18Ow is based on the lowest measured enamel-δ18O value (−12.2‰), which yields a paleo-meteoric water estimate of −15.1‰. Limited data from the modern Xunhua Basin yield a mean δ18Ow of ∼ −6.5‰ (Hough et al., 2011), which is 8.6‰ higher than the estimated paleo-meteoric water value of −15.1‰ at ∼9 Ma. The present-day Xunhua Basin is within the Asian monsoon region (Figure 8), with climate mainly controlled by the East Asian Summer Monsoon. In summer monsoon regions, summer precipitation has lower δ18O than winter precipitation (Figure 8), primarily due to the “amount effect” (Dansgaard, 1964). The amount of precipitation is mainly controlled by monsoonal intensity (Li et al., 2020). Thus, the lower reconstructed paleo-meteoric water δ18O values at ∼9 Ma are consistent with a wetter climate and possibly stronger influence of the East Asian Monsoon than today. This inference is supported by clay mineralogy and chemical weathering indices, which indicate a relatively warmer and more humid environment in the Xunhua Basin between ∼12.5 Ma and 8 Ma (Wang et al., 2010; Ye et al., 2010).
FIGURE 8. Plots of mean monthly values of temperature, precipitation, and precipitation δ18O for the Xunhua Basin (modified from Hough et al., 2011).
A large number of isotopic paleodiet records from the Upper Miocene of China have been published, yielding data for the Gyirong Basin (Wang et al., 2006; Wang et al., 2012), Zhada Basin (Wang et al., 2013), Kunlun Pass Basin (Wang et al., 2008b), Qaidam Basin (Zhang et al., 2012a), Linxia Basin (Wang and Deng, 2005; Biasatti et al., 2010; Biasatti et al., 2018), Fugu Area (Li et al., 2020), and Yushe Basin (Ciner et al., 2015). These well-dated records from various locations provide a basis for conducting a regional comparison of paleoclimatic changes on the northeastern Tibetan Plateau. The Linxia Basin is close to the Xunhua Basin, separated by the Jishi Shan and located only 50 km to the east. The Upper Miocene strata of the Linxia Basin contain abundant, diverse, and well-preserved mammalian fossils (Figure 6). Here, we chose a nearly contemporaneous Hipparion fauna (including Hipparion and Chilotherium) of the Linxia Basin with an age of ∼9.5–9.0 Ma, that was deposited in mudstones, to compare with the δ13C and δ18O records of the Xunhua Basin at ∼9 Ma (Figures 6, 7).
The bulk enamel δ13C of Hipparion and Chilotherium from the Xunhua Basin ranges from −9.2 to −5.0‰ (mean −8.4 ± 0.74‰), and that of the Linxia Basin ranges from −11.6 to −9.1‰ (mean −10.9 ± 0.74‰) (Wang and Deng, 2005; Biasatti et al., 2010; Biasatti et al., 2018). The difference in mean δ13C values between the Xunhua and Linxia basins is significant (p < 0.00001; t-test) (Figure 6). Kohn (2010) demonstrated that the δ13C values of modern C3 plants are negatively corelated with mean annual precipitation (MAP). The regression equation is: δ13C = −10.29 + 1.9×10−4×Altitude–5.61×log10(MAP+300)–0.0124×Abs(Latitude), where units are m for Altitude and mm/yr for MAP, and the relationship yields r2 = 0.59. The Xunhua and Linxia basins have average elevations of 2,200 m and 1917 m, respectively. The difference of ∼2.5‰ in mammal enamel δ13C values between these basins (Wang and Deng, 2005; Biasatti et al., 2010; Biasatti et al., 2018) is equivalent to a ∼300 mm increase in MAP in the Linxia Basin relative to the Xunhua Basin. This suggests that the Hipparion fauna in the Xunhua Basin may have experienced a drier climate than the Linxia Basin fauna, which is also supported by our oxygen isotope results. δ18O values of Hipparion and Chilotherium from the Xunhua Basin (mean −8.4 ± 1.2‰, range −12.2 to −6.5‰) are somewhat higher than those from the Linxia Basin (mean −9.1 ± 0.75‰, range −9.9 to −7.2‰) (p = 0.08; t-test) (Wang and Deng, 2005; Biasatti et al., 2010; Biasatti et al., 2018), reflecting greater aridity in the former basin (Dettman et al., 2003; Wang and Deng, 2005; Hough et al., 2011).
The primary factors controlling Late Miocene aridification in the Xunhua and Linxia basins were global cooling (Miao et al., 2011) and tectonic uplift of the Tibetan Plateau (Zhisheng et al., 2001; Zhang et al., 2012b; Yang et al., 2021). Many studies have inferred that increasing aridification of the northeastern Tibetan Plateau after 14 Ma was the result of global climatic cooling (Zachos et al., 2001; Ma et al., 2005; Jiang and Ding, 2008; Miao et al., 2011; Xu et al., 2015). Global cooling is likely to have reduced the amount of water vapor in the atmosphere, weakening precession-related moisture cycles (Zachos et al., 2001). Palynological studies in the Jiuxi Basin, Qaidam Basin and Guyuan area indicate a long-lasting cooling trend beginning at ∼13–12 Ma (Ma et al., 2005; Jiang and Ding, 2008; Miao et al., 2011). Sporopollen and geochemical records of paleoclimate in the Xunhua Basin demonstrate a gradual cooling and drying trend from ∼14 Ma to 5 Ma (Xu et al., 2015). However, differences in calculated δ18Ow between the Xunhua and Linxia basins, despite their proximity (Figure 6), suggest that global cooling was not the sole, or even the dominant, control on climate change on the northeastern Tibetan Plateau at ∼9 Ma.
Many lines of evidence confirm that the northeastern Tibetan Plateau experienced strong tectonic activity during the Middle-Late Miocene (Molnar, 2005; Lease et al., 2007; Lin et al., 2010; Lease et al., 2011; Yuan et al., 2011; Lease et al., 2012; Yuan et al., 2013; Fang et al., 2016; Fu et al., 2017; Yang et al., 2017; Saylor et al., 2018). For example, Yuan et al. (2011) inferred initiation of the Ela Shan and Riyue Shan strike-slip faults at 9 ± 1 and 10 ± 3 Ma, respectively, based on estimated Quaternary slip rates of ∼1.1 mm/yr and ∼10 km offset of older geologic contracts. The Laji Shan was first uplifted at 24–22 Ma and underwent accelerated uplift at 14–8 Ma, as indicated by recent studies of paleomagnetism and sedimentary facies of similar deposits in the Xunhua Basin and low-temperature thermochronometry of the Laji Shan (Lease et al., 2011, 2012). The Jishi Shan first began to rise at ∼13 Ma (Hough et al., 2011; Lease et al., 2011; Saylor et al., 2018), likely reflecting a reorientation of contractile deformation from NNE-SSW to ENE-WSW on the northeastern Tibetan Plateau at that time (Lease et al., 2011; Yuan et al., 2013), followed by intense uplift at 9–8 Ma (Lease et al., 2007; Saylor et al., 2018). Magnetostratigraphic correlations and stratigraphic architectures constrained uplift of the Liupan Shan beginning at 9.5 Ma, followed by rapid cooling at 8.2–7.3 Ma as recorded by apatite fission track data (Lin et al., 2010). Moreover, rapid uplift at 9–8 Ma is also evidenced by thick conglomerate layers and increased sedimentation rates in the Mojiazhuang section of the Xining Basin, the Heilingding section of the Linxia Basin, the Xigou section of the Xunhua Basin, and the Jiarang section of the Jianzha Basin (Ji et al., 2010; Fang et al., 2016; Fu et al., 2017; Yang et al., 2017).
Uplift of the Tibetan Plateau had profound effects on local, regional and global climate (Raymo and Ruddiman, 1992; Zhisheng et al., 2001). Based on the regional records of silicate weathering intensity, the Linxia and Xining basins experienced divergent climatic patterns marked by a decrease in (I/S + smectite)/(illite + chlorite) ratios and overall lower values of chemical weathering proxies in the Xining Basin relative to the Linxia Basin, reflecting a drier climate in the former basin since ∼8 Ma due to uplift of the surrounding mountains (Yang et al., 2021). This scenario may apply also to the Xunhua and Linxia basins. The Linxia Basin opens eastward onto the western margin of the Chinese Loess Plateau, allowing monsoonal moisture penetration (Figure 1). The Xunhua Basin is located to the west of the Linxia Basin, separated from it by the Jishi Shan, whose peaks reach 3,500 m (Figure 1). It is thus located in an orographic rainshadow, and as the Jishi Shan rose higher during the Late Miocene (at ∼9–8 Ma; Lease et al., 2007; Saylor et al., 2018), the Xunhua Basin underwent greater aridification than the Linxia Basin (Figure 9).
FIGURE 9. Sketch map illustrating the paleoecological characteristics of the Xunhua Basin in the northeastern Tibetan Plateau at ∼ 9 Ma.
The estimated isotopic composition of meteoric water (δ18Ow) in the Xunhua Basin (−15.1‰) is based on the lowest measured value of enamel-δ18O, which confirms that fossils yielding higher enamel-δ18O values in the Xunhua Basin were influenced by more-evaporated surface waters. This value is ∼3‰ more negative than the δ18Ow estimate for the Linxia Basin (−12.1‰), which is consistent with the aforementioned orographic rainshadow effect, that is, the precipitation across the mountain ranges will be more isotopically depleted. Strong aridification led to intense evaporation within the Xunhua Basin, causing enrichment of surface waters in the heavy isotope of oxygen. This process resulted in higher mean enamel-δ18O of the Xunhua Basin (−8.4 ± 1.2‰) relative to the Linxia Basin (−9.1 ± 0.75‰).
Upper Miocene sediments in the Xunhua Basin of the northeastern Tibetan Plateau yielded a variety of mammal fossils dating to ∼9 Ma, including Hipparion platyodus Selve, Chilotherium sp., Gazella gaudryi Schlosser, Gazella paotehensis, Gazella dorcadoides, Gazella sp., and Cervocerus sp. Stable carbon and oxygen isotope compositions of the fossil tooth enamel allowed significant inferences regarding the paleoenvironment of the Xunhua Basin at ∼9 Ma. First, enamel δ13C data indicate that the Hipparion fauna mostly fed on C3 plants even though the local ecosystem contained a certain amount of C4 grasses. Integrated with pollen records, we infer that the Xunhua Basin at ∼9 Ma contained a mixed forest-grassland ecosystem. Second, the influence of the Asian Summer Monsoon was stronger at ∼9 Ma than today, as reflected by paleo-meteoric water δ18O (as reconstructed from minimum fossil enamel δ18O) lower than that of modern precipitation in the region. Third, higher δ13C and δ18O values for fossil tooth enamel from the Xunhua Basin relative to contemporaneous fossils in the neighboring Linxia Basin indicate a relatively drier climate in the former basin, which can be attributed to development of an orogenic rainshadow following uplift of the Jishi Shan.
The original contributions presented in the study are included in the article/Supplementary Material, further inquiries can be directed to the corresponding authors.
The idea was provided by the corresponding author KZ. First author FH conducted the experiment and wrote the manuscript. Corresponding author BS guided the work and modified this paper. YS and WH gave much advice for this manuscript. TA provided technical input and assistance with English. ML and QL collected the fossils in the field. JJ offered correct age of the Xigou section.
This work was supported by the National Natural Science Foundation of China (Grant Nos. 42072141, 41772107) and the China Geological Survey (No. DD20190370).
The authors declare that the research was conducted in the absence of any commercial or financial relationships that could be construed as a potential conflict of interest.
All claims expressed in this article are solely those of the authors and do not necessarily represent those of their affiliated organizations, or those of the publisher, the editors and the reviewers. Any product that may be evaluated in this article, or claim that may be made by its manufacturer, is not guaranteed or endorsed by the publisher.
We are very grateful to Xiaohu Kou, Fenning Chen, Fang Han, Jianyu Zhang for field assistance in the Xunhua Basin, Higgins Penny for experimental assistance and Chaowen Wang, Ke Yin and Zhao Liu for their valuable suggestions. We greatly thank the editor and reviewers for their careful work and constructive suggestions to this paper.
The Supplementary Material for this article can be found online at: https://www.frontiersin.org/articles/10.3389/feart.2021.717720/full#supplementary-material
Ayliffe, L. K., Chivas, A. R., and Leakey, M. G. (1994). The Retention of Primary Oxygen Isotope Compositions of Fossil Elephant Skeletal Phosphate. Geochimica et Cosmochimica Acta. 58 (23), 5291–5298. doi:10.1016/0016-7037(94)90312-3
Biasatti, D., Wang, Y., and Deng, T. (2010). Strengthening of the East Asian Summer Monsoon Revealed by a Shift in Seasonal Patterns in Diet and Climate after 2-3Ma in Northwest China. Palaeogeogr. Palaeoclimatol. Palaeoecol. 297, 12–25. doi:10.1016/j.palaeo.2010.07.005
Biasatti, D., Wang, Y., and Deng, T. (2018). Paleoecology of Cenozoic Rhinos From Northwest China: a Stable Isotope Perspective. Vertebrata Palasiatica. 56 (1), 45–68. doi:10.19615/j.cnki.1000-3118.10519
Biasatti, D., Wang, Y., Gao, F., Xu, Y., and Flynn, L. (2012). Paleoecologies and Paleoclimates of Late Cenozoic Mammals from Southwest China: Evidence From Stable Carbon and Oxygen Isotopes. J. Asian Earth Sci. 44, 48–61. doi:10.1016/j.jseaes.2011.04.013
Bowman, C. N., Wang, Y., Wang, X., Takeuchi, G. T., Faull, M., Whistler, D. P., et al. (2017). Pieces of the Puzzle: Lack of Significant C4 in the Late Miocene of Southern California. Palaeogeogr. Palaeoclimatol. Palaeoecol. 475, 70–79. doi:10.1016/j.palaeo.2017.03.008
Bryant, D., and Froelich, P. (1995). A Model of Oxygen Isotope Fractionation in Body Water of Large Mammals. Geochim. Cosmochim. Acta. 59, 4523–4537. doi:10.1016/0016-7037(95)00250-4
Bryant, J. D., Froelich, P. N., Showers, W. J., and Genna, B. J. (1996). Biologic and Climatic Signals in the Oxygen Isotopic Composition of Eocene-Oligocene Equid Enamel Phosphate. Palaeogeogr. Palaeoclimatol. Palaeoecol. 126, 75–89. doi:10.1016/S0031-0182(96)00071-5
Cerling, T. E., Harris, J. M., Ambrose, S. H., Leakey, M. G., and Solounias, N. (1997). Dietary and Environmental Reconstruction with Stable Isotope Analyses of Herbivore Tooth Enamel from the Miocene Locality of Fort Ternan, Kenya. J. Hum. Evol. 33, 635–650. doi:10.1006/jhev.1997.0151
Cerling, T. E., and Harris, J. M. (1999). Carbon Isotope Fractionation Between Diet and Bioapatite in Ungulate Mammals and Implications for Ecological and Paleoecological Studies. Oecologia. 120, 347–363. doi:10.1007/s004420050868
Cerling, T. E., Wang, Y., and Quade, J. (1993). Expansion of C4 Ecosystems as an Indicator of Global Ecological Change in the Late Miocene. Nature. 361, 344–345. doi:10.1038/361344a0
Ciner, B., Wang, Y., Deng, T., Flynn, L., Hou, S., and Wu, W. (2015). Stable Carbon and Oxygen Isotopic Evidence for Late Cenozoic Environmental Change in Northern China. Palaeogeogr. Palaeoclimatol. Palaeoecol. 440, 750–762. doi:10.1016/j.palaeo.2015.10.009
Cormie, A. B., Luz, B., and Schwarcz, H. P. (1994). Relationship Between the Hydrogen and Oxygen Isotopes of Deer Bone and Their Use in the Estimation of Relative Humidity. Geochimica et Cosmochimica Acta. 58, 3439–3449. doi:10.1016/0016-7037(94)90097-3
Dansgaard, W. (1964). Stable Isotopes in Precipitation. Tellus. 16, 436–468. doi:10.3402/tellusa.v16i4.8993
Deng, T., Li, Q., Tseng, Z. J., Takeuchi, G. T., Wang, Y., Xie, G., et al. (2012). Locomotive Implication of a Pliocene Three-Toed Horse Skeleton From Tibet and its Paleo-Altimetry Significance. Proc. Natl. Acad. Sci. 109, 7374–7378. doi:10.1073/pnas.1201052109/-/DC
Dettman, D. L., Fang, X. M., Garzione, C. N., and Li, J. J. (2003). Uplift-Driven Climate Change at 12 Ma; a Long δ18O Record From the NE Margin of the Tibetan Plateau. Earth Planet. Sci. Lett. 214 (1-2), 267–277. doi:10.1016/S0012-821X(03)00383-2
Fang, X., Wang, J., Zhang, W., Zan, J., Song, C., Yan, M., et al. (2016). Tectonosedimentary Evolution Model of an Intracontinental Flexural (Foreland) basin for Paleoclimatic Research. Glob. Planet. Change. 145, 78–97. doi:10.1016/j.gloplacha.2016.08.015
Fricke, H. C., Clyde, W. C., and O’Neil, J. R. (1998). Intra-Tooth Variations in δ18O (PO4) of Mammalian Tooth Enamel as a Record of Seasonal Variations in Continental Climate Variables. Geochimica et Cosmochimica Acta. 62, 1839–1850. doi:10.1016/S0016-7037(98)00114-8
Fricke, H. C., and O'Neil, J. R. (1996). Inter- and Intra-Tooth Variation in the Oxygen Isotope Composition of Mammalian Tooth Enamel Phosphate: Implications for Palaeoclimatological and Palaeobiological Research. Palaeogeogr. Palaeoclimatol. Palaeoecol. 126, 91–99. doi:10.1016/S0031-0182(96)00072-7
Fu, C., Qiang, X., Xu, X., Xi, J., Zuo, J., and An, Z. (2017). Late Miocene Magnetostratigraphy of Jianzha Basin in the Northeastern Margin of the Tibetan Plateau and Changes in the East Asian Summer Monsoon. Geol. J. 53 (S1), 282–292. doi:10.1002/gj.3047
Higgins, P., and MacFadden, B. J. (2004). "Amount Effect" Recorded in Oxygen Isotopes of Late Glacial Horse (Equus) and bison (Bison) Teeth From the Sonoran and Chihuahuan Deserts, Southwestern United States. Palaeogeogr. Palaeoclimatol. Palaeoecol. 206, 337–353. doi:10.1016/j.palaeo.2004.01.011
Hough, B. G., Garzione, C. N., Wang, Z., Lease, R. O., Burbank, D. W., and Yuan, D. (2011). Stable Isotope Evidence for Topographic Growth and Basin Segmentation: Implications for the Evolution of the NE Tibetan Plateau. Geol. Soc. America Bull. 123 (1-2), 168–185. doi:10.1130/B30090.1
IAEA/WMO (2018). International Atomic Energy Agency Global Network for Isotopes in Precipitation Database. Available at: http://www.naweb.iaea.org/napc/ih/IHS_resources_gnip.html.
Ji, J., Zhang, K., Qiang, T., Kou, X. H., Chen, F. N., Xu, Y. D., et al. (2010). Magnetostratigraphy of the Neogene Strata in Xunhua Basin, Qinghai Province. Earth Sci. J. China Univ. Geosci. 35, 803–810. (in Chinese). doi:10.3799/dqkx.2010.093
Jiang, H., and Ding, Z. (2008). A 20 Ma Pollen Record of East-Asian Summer Monsoon Evolution from Guyuan, Ningxia, China. Palaeogeogr. Palaeoclimatol. Palaeoecol. 265, 30–38. doi:10.1016/j.palaeo.2008.04.016
Johnson, K. R., and Ingram, B. L. (2004). Spatial and Temporal Variability in the Stable Isotope Systematics of Modern Precipitation in China: Implications for Paleoclimate Reconstructions. Earth Planet. Sci. Lett. 220 (3-4), 365–377. doi:10.1016/S0012-821X(04)00036-6
Koch, P. L., Heisinger, J., Moss, C., Carlson, R. W., Fogel, M. L., and Behrensmeyer, A. K. (1995). Isotopic Tracking of Change in Diet and Habitat Use in African Elephants. Science. 267, 1340–1343. doi:10.1126/science.267.5202.1340
Koch, P. L. (1998). Isotopic Reconstruction of Past continental Environments. Annu. Rev. Earth Planet. Sci. 26, 573–613. doi:10.1146/annurev.earth.26.1.573
Kohn, M. J. (2010). Carbon Isotope Compositions of Terrestrial C3 Plants as Indicators of (Paleo)Ecology and (Paleo)Climate. Proc. Natl. Acad. Sci. 107, 19691–19695. doi:10.1073/pnas.1004933107
Kohn, M. J., and Cerling, T. E. (2002). “12. Stable Isotope Compositions of Biological Apatite,” in Phosphates-Geochemical, Geobiological, and Materials Importance. Reviews in Mineralogy and Geochemistry. Editors M. Kohn, J. Rakovan, and J. Hughes (Washington D.C: Mineralogical Society of America), v. 48, 455–488. doi:10.1515/9781501509636-015
Lease, R. O., Burbank, D. W., Clark, M. K., Farley, K. A., Zheng, D., and Zhang, H. (2011). Middle Miocene Reorganization of Deformation Along the Northeastern Tibetan Plateau. Geology. 39 (4), 359–362. doi:10.1130/G31356.1
Lease, R. O., Burbank, D. W., Gehrels, G. E., Wang, Z., and Yuan, D. (2007). Signatures of Mountain Building: Detrital Zircon U/Pb Ages from Northeastern Tibet. Geol. 35, 239–242. doi:10.1130/g23057a.1
Lease, R. O., Burbank, D. W., Hough, B., Wang, Z., and Yuan, D. (2012). Pulsed Miocene Range Growth in Northeastern Tibet: Insights from Xunhua basin Magnetostratigraphy and Provenance. Geol. Soc. America Bull. 124 (5-6), 657–677. doi:10.1130/B30524.1
Lee-Thorp, J., and van der Merwe, N. J. (1987). Carbon Isotope Analysis of Fossil Bone Apatite. S Afr. J. Sci. 83, 712–715.
Levin, N. E., Cerling, T. E., Passey, B. H., Harris, J. M., and Ehleringer, J. R. (2006). A Stable Isotope Aridity Index for Terrestrial Environments. Proc. Natl. Acad. Sci. USA. 103, 201–205. doi:10.1073_pnas.060471910310.1073/pnas.0604719103
Li, Y. F., Deng, T., Hua, H., Zhang, Y. X., and Wang, J. D. (2020). Isotopic Record of Palaeodiet of a 7.4 Ma Hipparionine Fauna From the Central Loess Plateau, Northern China: Palaeo-Ecological and Palaeo-Climatic Implications. Chem. Geol. 532, 120123. doi:10.1016/j.chemgeo.2019.119353
Lin, X., Chen, H., Wyrwoll, K.-H., and Cheng, X. (2010). Commencing Uplift of the Liupan Shan since 9.5Ma: Evidences from the Sikouzi Section at its East Side. J. Asian Earth Sci. 37, 350–360. doi:10.1016/j.jseaes.2009.09.005
Longinelli, A. (1984). Oxygen Isotopes in Mammal Bone Phosphate: a New Tool for Paleohydrological and Paleoclimatological Research? Geochimica et Cosmochimica Acta. 48, 385–390. doi:10.1016/0016-7037(84)90259-X
Luo, M. S., Lv, X. L., Zhang, K. X., Chen, F. N., Xu, Y. D., Chen, R. M., et al. (2010). Middle Miocene-Early Pliocene Ostracod Assemblages in the Xunhua Basin, Qinghai and Their Geological Significance. Acta Micropalaeontol Sinica. 27 (2), 125–134. (in Chinese).
Luz, B., Kolodny, Y., and Horowitz, M. (1984). Fractionation of Oxygen Isotopes Between Mammalian Bone-Phosphate and Environmental Drinking Water. Geochimica et Cosmochimica Acta. 48, 1689–1693. doi:10.1016/0016-7037(84)90338-7
Ma, Y., Fang, X., Li, J., Wu, F., and Zhang, J. (2005). The Vegetation and Climate Change during Neocene and Early Quaternary in Jiuxi Basin, China. Sci. China Ser. D-earth Sci. 48, 676–688. doi:10.1360/03yd0110
MacFadden, B. J., and Ceding, T. E. (1994). Fossil Horses, Carbon Isotopes and Global Change. Trends Ecol. Evol. 9, 481–486. doi:10.1016/0169-5347(94)90313-1
Miao, Y., Fang, X., Herrmann, M., Wu, F., Zhang, Y., and Liu, D. (2011). Miocene Pollen Record of KC-1 Core in the Qaidam Basin, NE Tibetan Plateau and Implications for Evolution of the East Asian Monsoon. Palaeogeogr. Palaeoclimatol. Palaeoecol. 299 (1-2), 30–38. doi:10.1016/j.palaeo.2010.10.026
Molnar, P. (2005). The Growth of the Tibetan Plateau and Mio-pliocene Evolution of East Asian Climate. Palaeontol. Electro. 8 (1), 1–23. doi:10.1016/j.palaeo.2009.11.002
Morgan, M. E., Kingston, J. D., and Marino, B. D. (1994). Carbon Isotopic Evidence for the Emergence of C4 Plants in the Neogene from Pakistan and Kenya. Nature. 367, 162–165. doi:10.1038/367162a0
Nelson, S. V. (2005). Paleoseasonality Inferred from Equid Teeth and Intra-Tooth Isotopic Variability. Palaeogeogr. Palaeoclimatol. Palaeoecol. 222, 122–144. doi:10.1016/j.palaeo.2005.03.012
Ogg, J. G. (2012). “5. Geomagnetic polarity time scale,” in The geologic time scale 2012. Editors F. M. Gradstein, J. G. Ogg, M. D. Schmitz, and G. M. Ogg (Hoboken, NJ: Elsevier), 85–113.
Passey, B. H., Cerling, T. E., Perkins, M. E., Voorhies, M. R., Harris, J. M., and Tucker, S. T. (2002). Environmental Change in the Great Plains: an Isotopic Record from Fossil Horses. J. Geology. 110, 123–140. doi:10.1086/338280
Quade, J., Cerling, T. E., Barry, J. C., Morgan, M. E., Pilbeam, D. R., Chivas, A. R., et al. (1992). A 16-Ma Record of Paleodiet Using Carbon and Oxygen Isotopes in Fossil Teeth from Pakistan. Chem. Geology. 94, 183–192. doi:10.1016/s0009-2541(10)80003-8
Raymo, M. E., and Ruddiman, W. F. (1992). Tectonic Forcing of Late Cenozoic Climate. Nature. 359, 117–122. doi:10.1038/359117a0
Saylor, J. E., Jordan, J. C., Sundell, K. E., Wang, X., Wang, S., and Deng, T. (2018). Topographic Growth of the Jishi Shan and its Impact on Basin and Hydrology Evolution, NE Tibetan Plateau. Basin Res. 30, 544–563. doi:10.1111/bre.12264
Schleser, G. H., and Jayasekera, R. (1985). δ13C-variations of Leaves in Forests as an Indication of Reassimilated CO2 from the Soil. Oecologia. 65, 536–542. doi:10.1007/BF00379669
Sharp, Z. D., and Cerling, T. E. (1998). Fossil Isotope Records of Seasonal Climate and Ecology: Straight from the Horse's Mouth. Geol. 26, 219–222. doi:10.1130/0091-7613(1998)026<0219:firosc>2.3.co;2
Sponheimer, M., Passey, B. H., de Ruiter, D. J., Guatelli-Steinberg, D., Cerling, T. E., and Lee-Thorp, J. A. (2006). Isotopic Evidence for Dietary Variability in the Early Hominin Paranthropus Robustus. Science. 314, 980–982. doi:10.1126/science.1133827
Stacklyn, S., Wang, Y., Jin, C.-z., Wang, Y., Sun, F., Zhang, C., et al. (2017). Carbon and Oxygen Isotopic Evidence for Diets, Environments and Niche Differentiation of Early Pleistocene Pandas and Associated Mammals in South China. Palaeogeogr. Palaeoclimatol. Palaeoecol. 468, 351–361. doi:10.1016/j.palaeo.2016.12.015
Sun, F., Wang, Y., Jablonski, N. G., Hou, S., Ji, X., Wolff, B., et al. (2021). Paleoenvironment of the Late Miocene Shuitangba Hominoids From Yunnan, Southwest China: Insights from Stable Isotopes. Chem. Geology. 569, 120123. doi:10.1016/j.chemgeo.2021.120123
Sun, F., Wang, Y., Wang, Y., Jin, C.-z., Deng, T., and Wolff, B. (2019). Paleoecology of Pleistocene Mammals and Paleoclimatic Change in South China: Evidence from Stable Carbon and Oxygen Isotopes. Palaeogeogr. Palaeoclimatol. Palaeoecol. 524, 1–12. doi:10.1016/j.palaeo.2019.03.021
Tipple, B. J., Meyers, S. R., and Pagani, M. (2010). Carbon Isotope Ratio of Cenozoic CO2: A Comparative Evaluation of Available Geochemical Proxies. Paleoceanography. 25 (3), 435–461. doi:10.1029/2009PA001851
Van der Merwe, N. J., and Medina, E. (1989). Photosynthesis and Ratios in Amazonian Rain Forests. Geochimica et Cosmochimica Acta. 53, 1091–1094. doi:10.1016/0016-7037(89)90213-5
Wang, F. L., Hong, H. L., Yin, K., Xu, Y. M., Zhang, K. X., and Zhang, J. Y. (2010). Clay Mineralogy and its Paleoclimatic Indicator of the Miocene Sediments of Xunhua Basin. Earth Sci. J. China Univ. Geosci. 35, 828–838. (in Chinese). doi:10.3799/dqkx.2010.096
Wang, Y., Cerling, T. E., and MacFadden, B. J. (1994). Fossil Horses and Carbon Isotopes: New Evidence for Cenozoic Dietary, Habitat, and Ecosystem Changes in North America. Palaeogeogr. Palaeoclimatol. Palaeoecol. 107, 269–279. doi:10.1016/0031-0182(94)90099-x
Wang, Y., and Deng, T. (2005). A 25 m.Y. Isotopic Record of Paleodiet and Environmental Change From Fossil Mammals and Paleosols from the NE Margin of the Tibetan Plateau. Earth Planet. Sci. Lett. 236, 322–338. doi:10.1016/j.epsl.2005.05.006
Wang, Y., Deng, T., and Biasatti, D. (2006). Ancient Diets Indicate Significant Uplift of Southern Tibet after Ca. 7 Ma. Geol. 34, 309–312. doi:10.1130/G22254.1
Wang, Y., Deng, T., Flynn, L., Wang, X., Yin, A., Xu, Y., et al. (2012). Late Neogene Environmental Changes in the Central Himalaya Related to Tectonic Uplift and Orbital Forcing. J. Asian Earth Sci. 44, 62–76. doi:10.1016/j.jseaes.2011.05.020
Wang, Y., Kromhout, E., Zhang, C., Xu, Y., Parker, W., Deng, T., et al. (2008a). Stable Isotopic Variations in Modern Herbivore Tooth Enamel, Plants and Water on the Tibetan Plateau: Implications for Paleoclimate and Paleoelevation Reconstructions. Palaeogeogr. Palaeoclimatol. Palaeoecol. 260, 359–374. doi:10.1016/j.palaeo.2007.11.012
Wang, Y., Wang, X., Xu, Y., Zhang, C., Li, Q., Tseng, Z. J., et al. (2008b). Stable Isotopes in Fossil Mammals, Fish and Shells from Kunlun Pass Basin, Tibetan Plateau: Paleo-Climatic and Paleo-Elevation Implications. Earth Planet. Sci. Lett. 270, 73–85. doi:10.1016/j.epsl.2008.03.006
Wang, Y., Xu, Y., Khawaja, S., Passey, B. H., Zhang, C., Wang, X., et al. (2013). Diet and Environment of a Mid-Pliocene Fauna from Southwestern Himalaya: Paleo-Elevation Implications. Earth Planet. Sci. Lett. 376, 43–53. doi:10.1016/j.epsl.2013.06.014
Wynn, J. G., Sponheimer, M., Kimbel, W. H., Alemseged, Z., Reed, K., Bedaso, Z. K., et al. (2013). Diet of Australopithecus Afarensis From the Pliocene Hadar Formation, Ethiopia. Proc. Natl. Acad. Sci. 110, 10495–10500. doi:10.1073/pnas.1222559110
Xu, Z. L., Zhang, J. Y., Ji, J. L., and Zhang, K. X. (2015). The Mid-Miocene Pollen Record of the Xunhua Basin, NE Tibetan Plateau: Implication for Global Climate Change. Acta Geol. Sin-engl. 89 (5), 1649–1663. doi:10.1111/1755-6724.12571
Yang, R., Fang, X., Meng, Q., Zan, J., Zhang, W., Deng, T., et al. (2017). Paleomagnetic Constraints on the Middle Miocene‐Early Pliocene Stratigraphy in the Xining Basin, NE Tibetan Plateau, and the Geologic Implications. Geochem. Geophys. Geosyst. 18, 3741–3757. doi:10.1002/2017GC006945
Yang, Y., Ye, C., Galy, A., Fang, X., Xue, Y., Liu, Y., et al. (2021). Monsoon‐Enhanced Silicate Weathering as a New Atmospheric CO 2 Consumption Mechanism Contributing to Fast Late Miocene Global Cooling. Paleoceanogr Paleoclimatol. 36, e2020PA004008. doi:10.1029/2020PA004008
Ye, H., Zhang, K. X., Ji, J. L., Zhang, J. Y., Liang, M. Y., Xu, Y. D., et al. (2010). Major and Trace Element Characters of the Sediments and Paleoclimatic Evolvement During About 23.1-5.0 Ma in Xunhua Basin, Qinghai. Earth Sci. J. China Univ. Geosci. 35, 811–820. (in Chinese). doi:10.3799/dqkx.2010.094
Yin, A., and Harrison, T. M. (2000). Geologic Evolution of the Himalayan-Tibetan Orogen. Annu. Rev. Earth Planet. Sci. 28, 211–280. doi:10.1146/annurev.earth.28.1.211
Yuan, D.-Y., Champagnac, J.-D., Ge, W.-P., Molnar, P., Zhang, P.-Z., Zheng, W.-J., et al. (2011). Late Quaternary Right-Lateral Slip Rates of Faults Adjacent to the lake Qinghai, Northeastern Margin of the Tibetan Plateau. Geol. Soc. America Bull. 123, 2016–2030. doi:10.1130/B30315.1
Yuan, D. Y., Ge, W. P., Chen, Z. W., Li, C. Y., Wang, Z. C., Zhang, H. P., et al. (2013). The Growth of Northeastern Tibet and its Relevance to Large‐Scale continental Geodynamics: A Review of Recent Studies. Tectonics. 32, 1358–1370. doi:10.1002/tect.20081
Zachos, J., Pagani, M., Sloan, L., Thomas, E., and Billups, K. (2001). Trends, Rhythms, and Aberrations in Global Climate 65 Ma to Present. Science. 292 (5517), 686–693. doi:10.1126/science.1059412
Zhang, C., Wang, Y., Deng, T., Wang, X., Biasatti, D., Xu, Y., et al. (2009). C4 Expansion in the Central Inner Mongolia during the Latest Miocene and Early Pliocene. Earth Planet. Sci. Lett. 287, 311–319. doi:10.1016/j.epsl.2009.08.025
Zhang, C., Wang, Y., Li, Q., Wang, X., Deng, T., Tseng, Z. J., et al. (2012a). Diets and Environments of Late Cenozoic Mammals in the Qaidam Basin, Tibetan Plateau: Evidence from Stable Isotopes. Earth Planet. Sci. Lett. 333-334, 70–82. doi:10.1016/j.epsl.2012.04.013
Zhang, R., Jiang, D., Liu, X., and Tian, Z. (2012b). Modeling the Climate Effects of Different Subregional Uplifts Within the Himalaya-Tibetan Plateau on Asian Summer Monsoon Evolution. Chin. Sci. Bull. 57, 4617–4626. doi:10.1007/s11434-012-5284-y
Zhang, J. Y., Zhang, K. X., Ji, J. L., Luo, M. S., Chen, F. N., Xu, Y. D., et al. (2010). Oligocene-Pliocene Sedimentary Facies Analysis and Sedimentary Evolution of Xunhua Basin in Northeastern Margin of Qinghai-Tibet Plateau. Earth Sci. J. China Univ. Geosci. 35, 774–788. (in Chinese). doi:10.3799/dqkx.2010.091
Zhang, Y., Yue, L. P., and Cao, H. X. (2002). The Ecological Sequence of Hipparion Fauna in the Middle Reaches of the Yellow River during Neogene. Chin. Sci Bull. 47 (1), 79–82. doi:10.1360/02tb9016
Zhisheng, A., Kutzbach, J. E., Prell, W. L., and Porter, S. C. (2001). Evolution of Asian Monsoons and Phased Uplift of the Himalaya-Tibetan Plateau Since Late Miocene Times. Nature. 411, 62–66. doi:10.1038/35075035
Zin Maung Maung, Thein, Takai, M., Uno, H., Wynn, J. G., Egi, N., Tsubamoto, T., et al. (2011). Stable Isotope Analysis of the Tooth Enamel of Chaingzauk Mammalian Fauna (Late Neogene, Myanmar) and its Implication to Paleoenvironment and Paleogeography. Palaeogeogr. Palaeoclimatol. Palaeoecol. 300, 11–22. doi:10.1016/j.palaeo.2010.11.016
Keywords: carbon isotopes, oxygen isotopes, tooth enamel, late miocene, uplift, Jishi Shan
Citation: Hu F, Song B, Sun Y, Huang W, Luo M, Ji J, Lin Q, Algeo TJ and Zhang K (2021) Paleodietary and Paleoclimatic Reconstruction of Hipparion Fauna at ∼9 Ma From the Xunhua Basin on the Northeastern Tibetan Plateau. Front. Earth Sci. 9:717720. doi: 10.3389/feart.2021.717720
Received: 31 May 2021; Accepted: 23 July 2021;
Published: 04 August 2021.
Edited by:
Yunfa Miao, Northwest Institute of Eco-Environment and Resources (CAS), ChinaCopyright © 2021 Hu, Song, Sun, Huang, Luo, Ji, Lin, Algeo and Zhang. This is an open-access article distributed under the terms of the Creative Commons Attribution License (CC BY). The use, distribution or reproduction in other forums is permitted, provided the original author(s) and the copyright owner(s) are credited and that the original publication in this journal is cited, in accordance with accepted academic practice. No use, distribution or reproduction is permitted which does not comply with these terms.
*Correspondence: Bowen Song, Yndzb25nMTk4NUBjdWcuZWR1LmNu; Kexin Zhang, a3hfemhhbmdAY3VnLmVkdS5jbg==
Disclaimer: All claims expressed in this article are solely those of the authors and do not necessarily represent those of their affiliated organizations, or those of the publisher, the editors and the reviewers. Any product that may be evaluated in this article or claim that may be made by its manufacturer is not guaranteed or endorsed by the publisher.
Research integrity at Frontiers
Learn more about the work of our research integrity team to safeguard the quality of each article we publish.