- 1School of Geography and Tourism, Shaanxi Normal University, Xi’an, China
- 2College of Earth and Environmental Sciences, Lanzhou University, Lanzhou, China
Identifying the provenance of aeolian deposits in semi-arid zones of China is beneficial in understanding Earth’s surface processes and helping to alleviate ecological stress. In this paper, we use grain-size, geochemical elements, heavy-minerals, and quartz grain morphology data to investigate the potential source of aeolian sands from the Ordos Deserts (Mu Us Sandy Land and Hobq Desert). Sedimentological, geochemical and geomorphological results indicate that significant provenance differences exist among various parts of the Mu Us Sandy Land, i.e., aeolian sediments from the southwest region are obviously distinct from other areas in the Mu Us Sandy Land but show the same external provenance with the Hobq Desert referring to the sorting, mineralogical maturity, geochemical characteristics, heavy-minerals, and quartz grain morphology. Comparing the samples from the Ordos Deserts with felsic rocks from potential sources via a serious of geochemical methods, we conclude that: 1) Aeolian sands from other regions of the Mu Us are a mixture of binary provenance, i.e., one originated from local lacustrine sediments and underlying sandstones, and another from the Alxa Plateau (AP) carried by northwesterly Asian winter monsoon. 2) The fluvial deposits denuded from the Qilian Orogenic Belt in the Northeastern Tibetan Plateau (NTP) and carried by the Yellow River are likely the initial material source for the southwest region of the Mu Us Sandy Land and the Hobq Desert. 3) The Yellow River plays a significant and critical role in sediment transport for sand seas in arid and semi-arid areas of northern China.
Introduction
Desert sand seas, characterized by various dune types, make up a significant part of the Earth’s surface sediment systems (Goudie and Middleton, 2001). Sand seas and dune fields are widely distributed across China, from semi-arid zones with annual precipitation greater than 400 mm in the northeast to hyper-arid zones with annual precipitation less than 50 mm in the northwest (Dong et al., 2013). Previous studies have demonstrated that extensive sand seas in northern and western China are a significant component of the Asian aeolian dust system (Chen and Li, 2011). Dust from these areas has drawn widespread public attention because of its potential influence on the Asian system including radiation balance, hydrological and biogeochemical cycles, as well as air quality (Arimoto, 2001; Goudie, 2009). Also, no matter what state a dune field is in (active or stable), as well as what sort of the dune forms evolve, a better understanding of the sand source is significant in judging the origin and subsequent evolution of a dune field (Dong et al., 2017). Therefore, tracing the provenance of aeolian sediment is fundamental to understanding the formation and evolution of deserts, the interaction between surface processes and climatic changes, the operation of the Earth system (Molnar, 2004). Also, it is beneficial for establishing effective environmental governance policies for such sensitive areas in China (Du et al., 2018). Diverse methods have been adopted to investigate the origin of desert sediments, such as grain size analysis, geochemical tracing, detrital mineralogy, scanning electron microscopy (SEM), satellite imagery, environmental magnetism, detrital zircon, and numerical modeling (Honda et al., 2004; Howari et al., 2007; Pan et al., 2016). For example, the grain-size characteristics of dune sands contain a mass of information about the transport pathways and potential sources of the sediment (Li et al., 2019). Geochemical analysis, especially of trace and rare Earth elements (REE), has great potential in identifying the provenance and transport pathways of sediments since they are less fractionated during weathering, transport, and sedimentation (Dehghani et al., 2018). Examining the provenance of heavy minerals has been used because their composition directly reflects the parent lithologies and primary provenance signals (Garzanti et al., 2008). Furthermore, some peculiar morphologies related to specific sedimentary processes and environments can be discriminated by the analysis of quartz grains using SEM, which provides new insights into the provenance of the sediments (Moral Cardona et al., 2005).
The Ordos Deserts, including the Mu Us Sandy Land and the Hobq Desert, are located in a transitional climatic zone in northern China (Figure 1). However, aeolian landforms are differentiated between them, i.e., the Mu Us is characterized by fixed and semi-fixed dunes, whereas active dunes are dominant in the Hobq Desert. In recent years, diverse techniques have been employed to explore the provenance of aeolian sands in the Mu Us, including geochemical indices (Rao et al., 2011b; Rao et al., 2014; Liu and Yang, 2018), zircon U-Pb, heavy mineral analysis, framework petrography (Stevens et al., 2013; Nie et al., 2015; Zhang et al., 2016; Wang et al., 2019; Sun et al., 2020), quartz crystallinity and magnetic susceptibility (Sun et al., 2013; Hällberg et al., 2020). Detailed results from the above provenance analyses have demonstrated that there exist distinct provenance differences between the western and eastern regions of the Mu Us, and diverse opinions about the region’s provenance remain. Furthermore, previous studies have either ignored provenance with respect to regional variation or are based on a small number of samples of the Mu Us, which may bias interpretations of the provenance in terms of its regional variation.
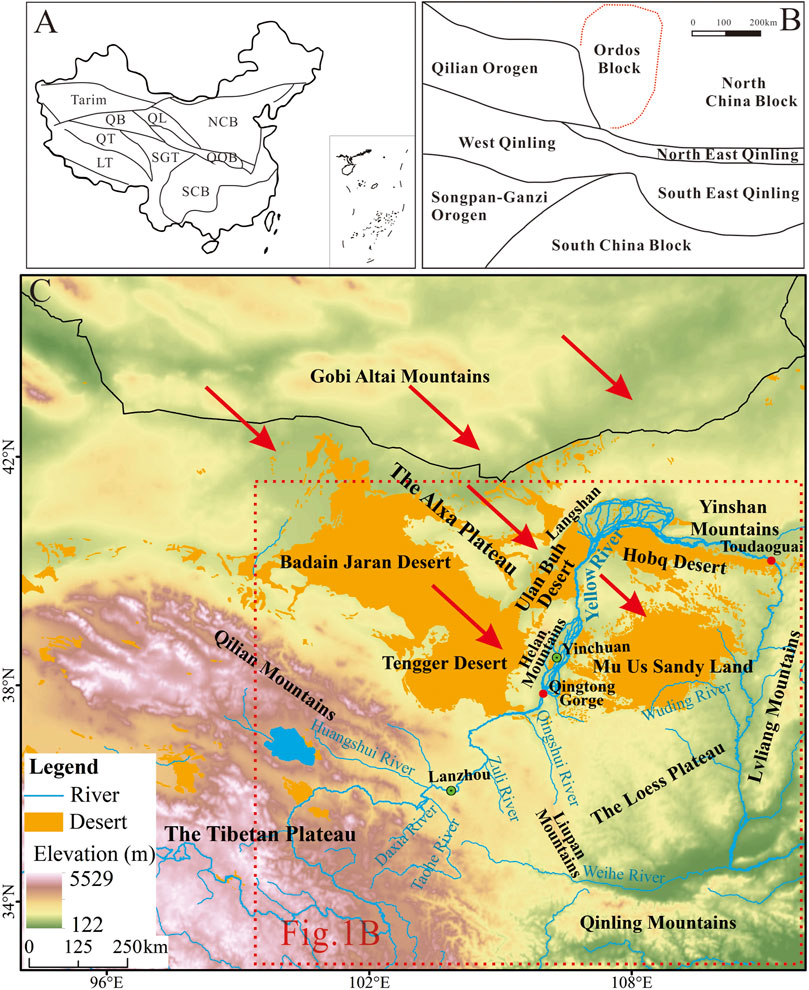
FIGURE 1. (A) Simplified geological map of China. (NCB: North China Block; SCB: South China Block; SGT: Songpan-Ganzi terrane; QB: Qaidam Basin; QT: Qiangtang terrane; LT: Lhasa terrane; QOB: Qinling Orogenic Belt). (B) Simplified regional geological map of study area (modified after Zhu et al., 2011). (C) Geographical location of the Ordos Deserts. The red arrows refer to the dominant wind direction.
Therefore, to better reflect the regional variation in the provenance of these desert sediments, we conducted detailed fieldwork and collected aeolian sand samples from different parts in the Ordos Deserts. Furthermore, we used an integrated approach to identify the provenance of the aeolian sediments in the Ordos Deserts, including grain size, geochemical tracing, mineralogy, and SEM analysis. Based on sedimentological and geomorphological data, this paper aims to present a more complete understanding of the regional variations in the provenance of the sediments that make up the Ordos Deserts and to further ascertain the critical role of the Yellow River in supplying sediments to the sand sea.
Field Site, Material, and Methods
The Study Area
The Ordos Plateau, tectonically situated in the western part of the North China Plate, is enclosed by the Yellow River on its western, northern, and eastern boundaries and is mainly covered by the Ordos Deserts. The Alxa Plateau (AP) and the Chinese Loess Plateau (CLP) are distributed in the upwind and downwind areas of the Ordos Desert, respectively (Figure 1). The Alxa Desert is mainly composed of the Badain Jaran Desert (BJD), the Tengger Desert (TD), the Ulan Buh Desert (UBD), and some small deserts in north-central China. The bedrock of the Ordos Plateau are composed of Archaean and Proterozoic metamorphic rocks (Rao et al., 2011b).
Located in the transitional zone between agriculture farming and animal grazing in the Ordos Plateau, the Mu Us Sandy Land (37°27′-39°22′N, 107°20′-111°30′E) is bordered by the Hobq Desert in the north, and the Yellow River in the east and west, and covers an area of 3.2 × 104 km2 (Figure 1). It lies on the margin of the area impacted by the East Asian monsoon, and the mean annual precipitation ranges from 440 mm in the southeast to 250 mm in the northwest, of which 60–80% is concentrated during the period from June to August (Shang et al., 2001). The annual mean temperature is about 6–9°C, and the annual mean evaporation and aridity are 1,800–2,500 mm and 1.0–2.5, respectively (Liu et al., 2015). The ground surface is characterized by semi-fixed, fixed, and mobile dunes, i.e., honeycomb dunes, parabolic dunes, vegetated dunes, crescent dunes, dune networks, etc. The present surface is dominated by substantial aeolian, lacustrine, and fluvial landforms (Wang et al., 2019). Owing to the elevation increasing gradually from the southeast to the northwest, the majority of the rivers flow from the northwest to the southeast. According to the calculation of the resultant drift direction, the wind system that controls aeolian transport in the Mu Us is from the northwest to the southeast (Figure 2).
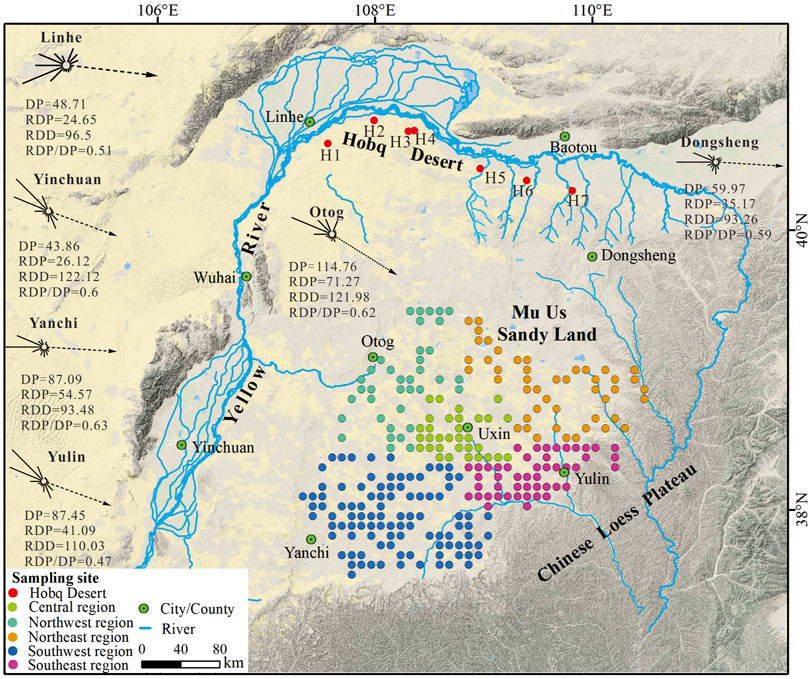
FIGURE 2. Locations of sand dune samples in the Ordos Deserts. Sand transport roses are calculated based on the descriptions of the wind environment proposed by (Fryberger, 1979) with data from the U.S. National Climate Data Center (NCDC). Abbreviations: DP, drift potential; RDP, resultant drift potential; RDD, resultant drift direction; RDP/DP, directional variability.
The Hobq Desert, with an area of 1.64 × 104 km2, is located on the margin of the northern Ordos Plateau. The mean annual precipitation ranges from 360 mm in the southeast to 240 mm in the northwest. Compared to the Mu Us, the majority of dunes in the Hobq Desert are active (Zhu et al., 1980). One prominent characteristic is that ten tributaries of the Yellow River flow through the desert northwards (Figure 2).
Material and Methods
The Mu Us Sandy Land was evenly divided into a 10 km × 10 km grid. Sand samples were collected from the dune crest (to a depth of 2 cm in a 20 cm × 20 cm area), with a total of 261 surface samples collected from 261 grid cells covering different regions (solid circles with different colors in four different regions, Figure 2) of the Mu Us and 7 samples (marked by H1 to 7) were collected from the Hobq Desert (Figure 2).
Samples were oven-dried and then analyzed using a Mastersizer 2000 (Malvern Instruments, Malvern, United Kingdom) at the School of Geography and Tourism of Shaanxi Normal University. Gain-size parameters, i.e., mean grain size (Mz) and sorting (σI) were calculated based on the method of Folk and Ward (1957).
Given that different sediment particles reflect different transport processes and sources, we separated the fine fractions (<125 μm) from the coarse fractions by dry sieving. The concentrations of major and trace elements (except REE) were analyzed using X-ray fluorescence (XRF) spectrometry at the School of Geography and Tourism of Shaanxi Normal University. The procedures for sample preparation are the same as described in Liang et al. (2019). The estimated error for repeat measurements was less than 5%. The chemical weathering intensity evaluation index (CIA) was calculated using the following formula (Nesbitt and Young, 1982):
where the values for each component are given in molecular units. This calculation assumes that CaO* refers to the amount of CaO incorporated only in silicate fraction. Here, the CIA values were calculated assuming CaO* = Na2O (in moles), following McLennan (1993). In addition, the plagioclase index of alteration (PIA) was calculated by the following formula (Fedo et al., 1995):
The concentrations of REE were determined by inductively coupled plasma-mass spectrometry (ICP-MS) at the Institute of Geochemistry, Chinese Academy of Sciences. The preparation of samples strictly comply with the pretreatment procedures of Yang et al. (2007). Analytical uncertainties (relative standard deviation) were less than ±1% for REE.
Heavy-mineral analyses were performed at the Hebei Central Laboratory of Geology and Mineral Resources. Dried samples were flushed in a washing pan for removing all impurities and then were separated with bromoform (2.89 g/cm3) from a settling tube via gravity. The types and volume of heavy-minerals were determined by using the “area method” under a binocular microscope and a polarizing microscope (Mange and Maurer, 1992). Given that the experimental conditions and the representativeness of the samples, we selected five representative samples for heavy minerals analysis from each region of the Mu Us.
The analysis of grain-morphology was conducted by using Hitachi Desktop Scanning Electron Microscope TM3000 at the School of Geography and Tourism of Shaanxi Normal University. The preparation of quartz grains strictly complies with the pretreatment procedures of Pan et al. (2016). We selected fifteen to twenty quartz grains from each sample randomly using a binocular microscope and then was sprayed gold film for ensuring electrical conductivity in an ion plating machine before testing. The surface micro-textures from each studied grain were recognized and interpreted according to the concepts of previous studies (Krinsley and Doornkamp, 1973).
Results
Grain-Size Parameters
Figure 3 shows the parameters of the sediments in the Mu Us. Here, the main grain size (Mz) for the Mu Us samples averaged 186.86 μm (2.42Φ), which is finer than sediments from the Hobq Desert, which averaged 201.66 μm (2.31Φ). Most regions in the Mu Us show moderate sorting, whereas the southwest region shows better sorting (Figure 3). Similarly, the sorting of the Hobq Desert samples includes two gradations: moderately sorted and better sorted (Table 1).
Geochemical Elements
The spatial variation of all major and trace elements in the Mu Us Sandy Land and the Hobq Desert are listed as supplementary materials (Supplementary Figures S1, S2, and Appendix A). Key features are described below.
Major Elements
Except for SiO2, the major elements of the bulk fractions are depleted in the Mu Us and the Hobq Desert, compared to the Upper Continental Crust (UCC) (Figure 4). What stands out in Figure 4 is the markedly higher SiO2 content, but lower content of Fe2O3, MgO, P2O5, TiO2, and MnO of the bulk fractions when compared with the fine fractions of aeolian sands, indicating that the bulk fractions have a higher amount of quartz and feldspar. One prominent characteristic is that Ti in the fine sands is enriched, which means that the Ti-bearing minerals (ilmenite, rutile, and titanite) can be found in the Ordos Deserts. Additionally, aeolian sands collected from the southwest region of the Mu Us have higher SiO2 but lower Al2O3, K2O, Na2O, and P2O5 when compared with other areas (Figure 4). According to the UCC-normalized diagram of major elements, both bulk and fine fractions of aeolian sands from the Hobq Desert are relatively uniform, whereas this trend is not found in the Mu Us, as the southwest region differs from other parts of the Mu Us (Figure 4).
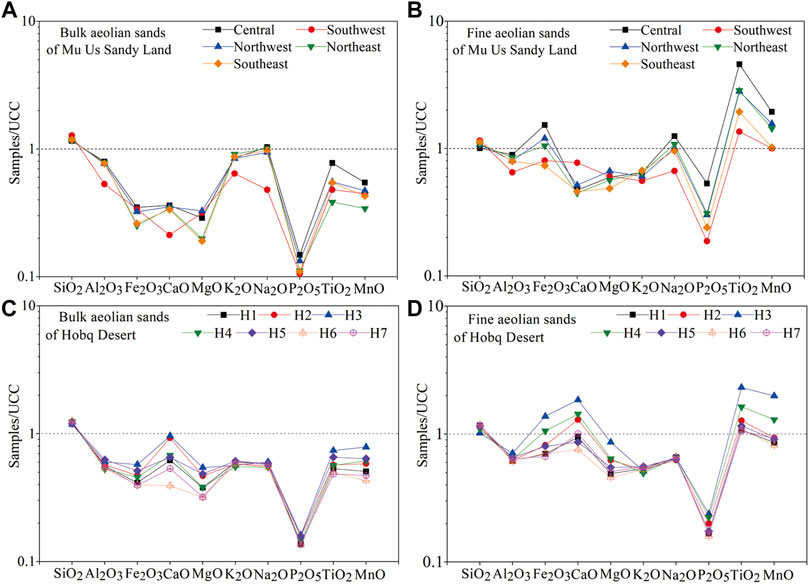
FIGURE 4. UCC-normalized diagrams of major elements. (A,B) are for bulk and fine fraction sands in the Mu Us Sandy Land, respectively. (C,D) are for bulk and fine fraction sands in the Hobq Desert, respectively. UCC values from Taylor and McLennan (1985).
Trace Elements
UCC-normalized plots of trace elements for various grain size fractions are shown in Figure 5. Most trace elements of the bulk fractions experience a varying degree of depletion, except for Co in the Mu Us and Cr in the Hobq Desert. It must be noted, however, that the elements Cr, Zr, and Hf are distinctly enriched in the fine fractions, and the content of most trace elements in the fine fraction sands are higher than in the bulk fractions. Notably, the distribution patterns of fine sands are consistent both in the Mu Us and the Hobq Desert (Figure 5).
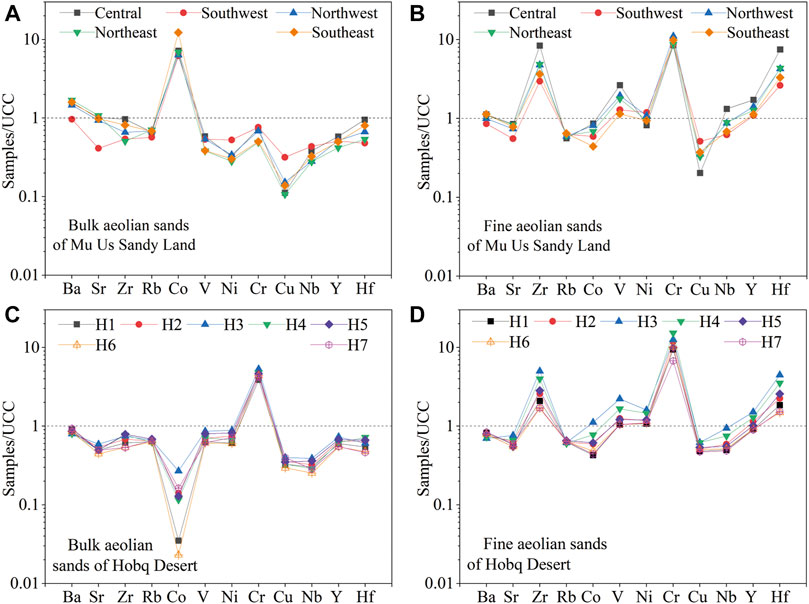
FIGURE 5. UCC-normalized distribution patterns of trace elements. (A,B) are for bulk and fine fraction sands in the Mu Us Sandy Land, respectively. (C,D) are for bulk and fine fraction sands in the Hobq Desert, respectively. UCC values from Taylor and McLennan (1985).
Rare Earth Elements
The mean total REE contents for bulk aeolian sands of different regions in the Mu Us varies from 61.8 to 96 ppm, and the fine fractions range from 180.4 to 270.5 ppm. The content of bulk fractions and fine fractions in the southwest regions is distinctly lower than that in other parts of the Mu Us. Furthermore, the total REE contents varies from 53.4 to 80.9 ppm for bulk fractions and from 114.2 to 217.3 ppm for fine aeolian sands in the Hobq Desert (Appendix A).
The chondrite-normalized REE distribution patterns of both samples from the Mu Us and the Hobq Desert are characterized by steep light-REE (LREE) and smooth heavy-REE (HREE), which is similar to UCC (Figure 6). Compared with bulk aeolian sands, the Eu anomalies show more negative in the fine fractions in both the Mu Us and the Hobq Desert. In addition, Eu anomalies for bulk aeolian sands are not obvious in other areas of the Mu Us except for the southwest (Figure 6).
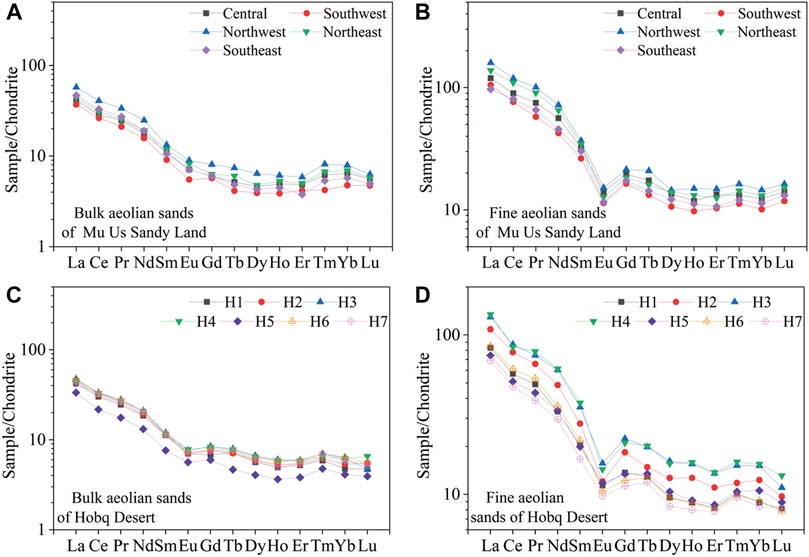
FIGURE 6. Chondrite-normalized REE patterns for different fractions. (A,B) are for bulk and fine fraction sands in the Mu Us Sandy Land, respectively. (C,D) are for bulk and fine fraction sands in the Hobq Desert, respectively. UCC values from Taylor and McLennan (1985).
Mineralogy
Figure 7 provides an overview of the composition of heavy minerals, with significant differences in heavy minerals existing across the Mu Us. Amphibole (24.9–38.4%) and garnet (30.8–39.8%) are the dominant heavy minerals in most areas of the Mu Us, except for the southwest. However, samples from the southwest display significantly higher haematite and limonite (average 18.1%), pyroxene (average 6.7%), and epidote (average 2%) contents, and lower garnet content (average 25.3%) (Figure 7).
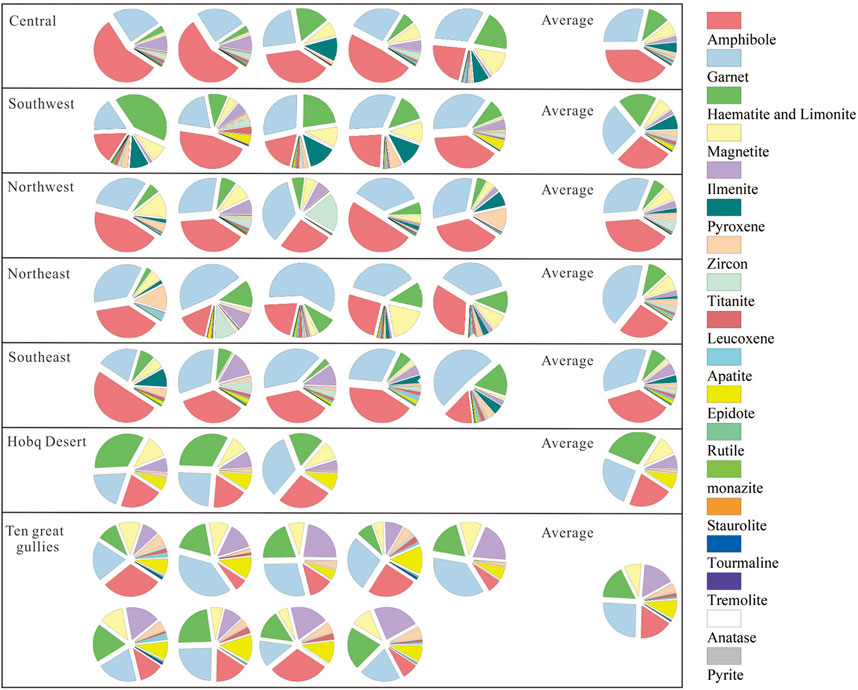
FIGURE 7. Heavy-mineral assemblages of the samples. The data of Hobq Desert and Ten great gullies from Pan et al. (2016).
Quartz Grain Morphology
The quartz grain morphologies of the Mu Us include micro-textures produced by mechanical and chemical actions (Figure 8). Previous studies of quartz grains from different sedimentary environments concluded that butterfly-shaped pits, pitted surfaces, and crescent-shaped pits are commonly shown in aeolian environments, whereas V-shaped fractures, conchoidal fractures, straight/curved grooves, and parallel striations are characteristics of aqueous environments (Mahaney, 2002; Vos et al., 2014). Quartz grains of all regions in the Mu Us display butterfly-shaped (Figure 8B) and crescent-shaped pits (Figure 8D) typical of aeolian environments, which indicates that the sands had suffered intense aeolian activities. In addition, pitted surfaces are found in some grains (Figure 8C). However, some micro-textures such as conchoidal fractures (Figure 8E), V-shaped pits (Figure 8F), straight/curved grooves (Figures 8G,H), and parallel striations (Figure 8I) are also found in the quartz grains, and are especially more pronounced in samples from the southwest region, which suggests that those sands may have been subjected originally to an aqueous environment with intense collisions and frictions between grains. Moreover, other structures, such as silica globules on rounded grains (Figure 8J), silica flowers (Figures 8K,L), solution crevasses (Figures 8M,N), and silicon compounds (Figure 8O) are common, indicating noticeable chemical processes.
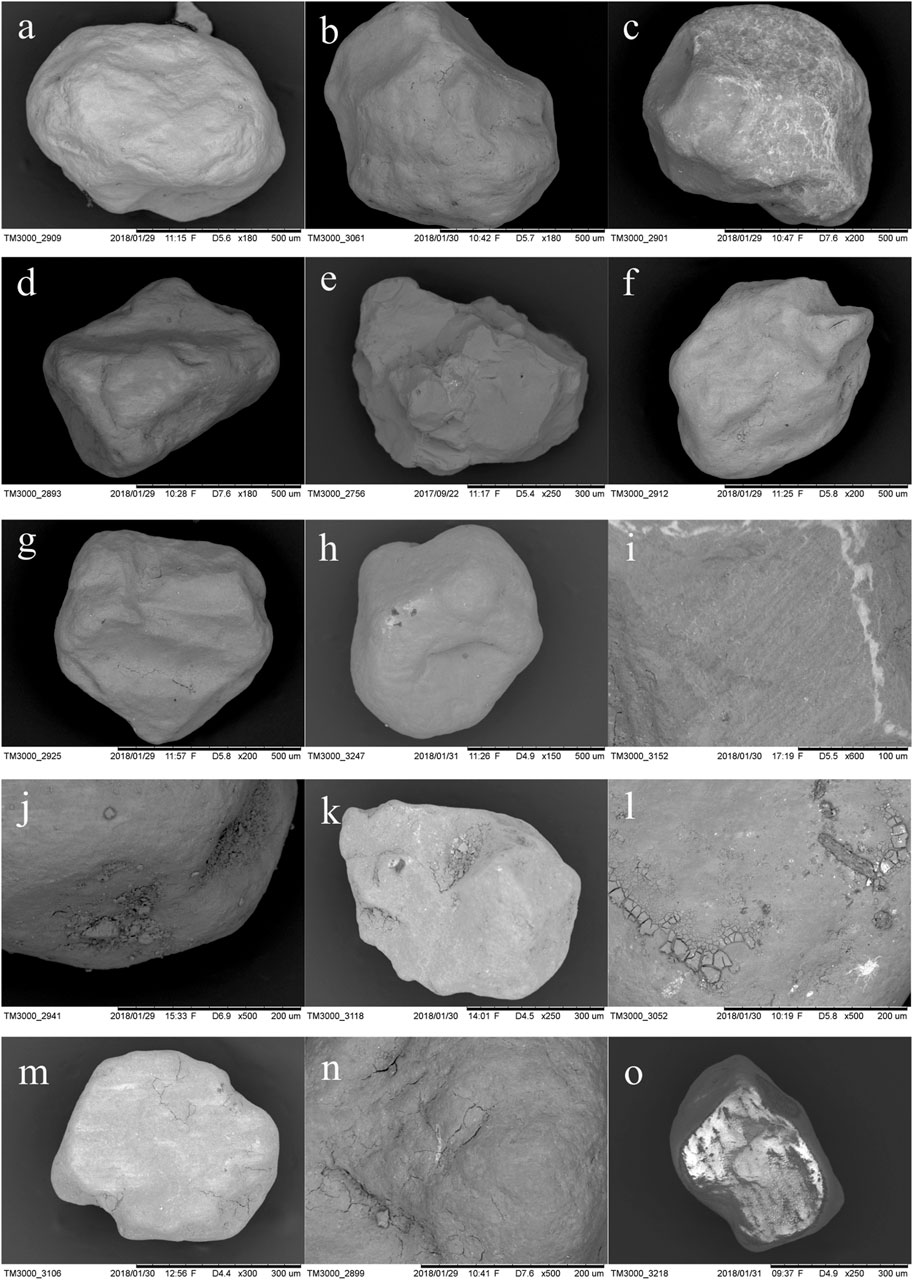
FIGURE 8. Typical surface microstructures for quartz grains in the Mu Us Sandy Land. (A) Rounded grain from the southwest region of the Mu Us Sandy Land. (B) rounded grain showing butterfly-shaped pits. (C) pitted surface. (D) crescent-shaped pits. (E) conchoidal fractures produced in the high-energy subaqueous environment. (F) large V-shaped fractures with high relief indicates a typical subaqueous environment. (G) straight grooves. (H) curved grooves; (I) parallel striations. (J) rounded grains with silica globules. (K) sub-angular grains showing silica flowers. (L) silica flowers. (M) angular grains with solution crevasses. (N) solution crevasses founded around silica globule. (O) silicon compounds.
Discussions
Provenance Differences Over the Ordos Deserts
Generally, the sands in downwind areas tend to be finer and more well sorted than those in upwind areas, and this trend had been validated in many deserts (Lancaster, 1989, 1995; Zhang et al., 2015; Zhang and Dong, 2015). The mean grain size of dunes in the Mu Us clearly shows a regional variation that tends to be finer from the northwest to the southeast (Figure 3), which is consistent with the compound direction of the regional wind system (NW, WNW, Figure 2). However, the best-sorted sands occur in the southwest region, which is located in the upwind direction of the prevailing wind in the Mu Us (Figure 3). It is evident that this trend is not in accord with the Mu Us, as no systematic trend in the variation of sorting was found with decreasing MZ, indicating that the spatial variation of sands in the Mu Us cannot be viewed simplistically as a response to the regional wind patterns. Generally, the distribution of grain size over a large sand sea is primarily controlled by wind and the characteristics of the source material (Livingstone et al., 1999). Hence, we conclude that the sediments in the southwest area of the Mu Us may have undergone long-term transportation, thus showing a better sorting than in other areas, or to go a step further, the provenance of sediments in the southwest area of the Mu Us is likely to be different from other regions.
Mineralogical maturity is considered as a compositional state of the clastic sedimentary body wherein quartz is dominant in its resistant minerals, which can be used to ascertain sediment sources (Muhs et al., 2013). Generally, mineralogical maturity can be portrayed by Log (Na2O/K2O) vs. Log (SiO2/Al2O3) presented by Pettijohn et al. (1972). As illustrated by Figure 9, higher Log (SiO2/Al2O3) values of sands from the southwest region indicate that more quartz and less feldspar existed in this region. Hence, we infer that sands from the southwest region have been subjected to long-distance transport, and their provenance draws the most essential part in terms of their differentiation of mineralogical maturity in the Mu Us. Heavy-mineral assemblages in fluvial deposits are controlled by source-rock lithology, chemical weathering, and the sorting of detrital materials (Garzanti et al., 2007; Garzanti et al., 2013a). However, evidence indicates that sediments in the Mu Us have undergone a low degree of chemical weathering (Figure 10). Garzanti et al. (2012) found that although sorting may give rise to the separation of heavy and light minerals during sediment transport, it would not cover up information about the provenance. According to our analysis of the heavy-mineral assemblages, aeolian sediments in the Mu Us can be classified into two groups (Figure 7). One group is the southwest region, which is dominated by amphibole, garnet, hematite, and limonite, and some pyroxenes, whereas the other group covers other areas of the Mu Us, which are dominated by amphibole and garnet. Therefore, the distinctive heavy-mineral assemblages between these two groups manifest how the source-rock lithology plays the most critical role in their different provenances. In addition, the roundness of the quartz grain in the southwest region of the upwind direction is significantly higher than that for other areas, also indicating that the deposits from the southwest region may have experienced a longer transport before being deposited (Supplementary Figure S3).
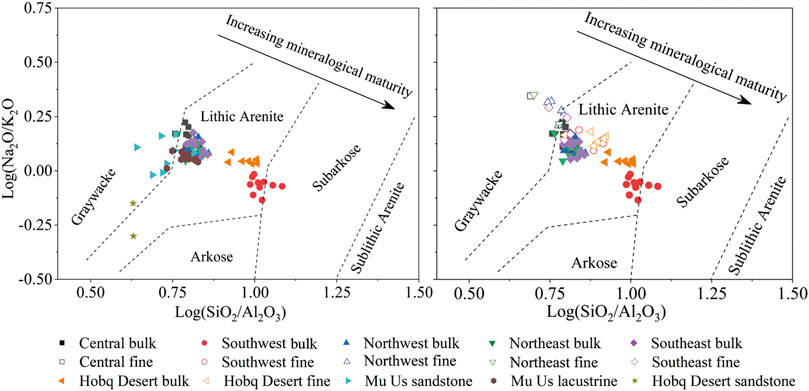
FIGURE 9. Plots of mineralogical maturity for sediments in the Ordos Deserts. Data of sandstone and lacustrine sediments in Mu Us and the Hobq Desert is from Liu and Yang (2018).
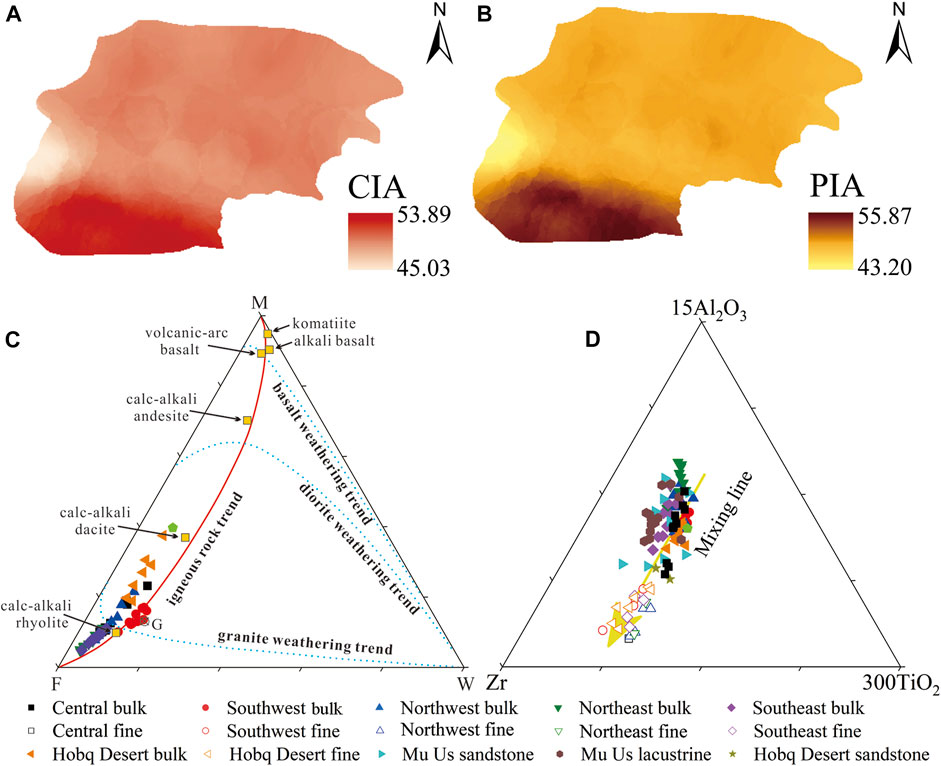
FIGURE 10. Spatial patterns of CIA (A) and PIA (B) for sands in the Mu Us Sandy Land. Plots of MFW (Mafic-Felsic-Weathering) characterizing weathering trends (C) and Al-Zr-Ti diagram (D) for sediments in the Ordos Deserts. M and F denote the mafic and felsic rocks source, respectively, whereas the W used to identify the degree of weathering of these igneous rocks. Data of sandstone and lacustrine sediments in Mu Us and the Hobq Desert is from Liu and Yang (2018).
Taken together, these results suggest that there is a distinct difference of provenance between the southwest region and other areas in the Mu Us, whereas the southwest region of the Mu Us may have the same sources as the Hobq Desert.
Feasibility of Using Chemical Composition to Determine Provenance
Although the geochemical composition of detrital sediments is widely used for tracing the provenance and composition of sediments, the chemical composition of clastic sediments is the combined result of source-rock composition, the weathering of the source area, sorting, and recycling (Fedo et al., 1995; Perri et al., 2013). As an example, the clastic sediments may not reflect the provenance directly due to the depletion of the mobile elements (like alkali and alkaline Earth metals) and the enrichment of non-mobile elements (such as Al2O3, TiO2, REE) resulting from weathering processes (Nesbitt et al., 1996). It is, hence, necessary to first evaluate whether the effects of these factors have hidden potential provenance signatures before identifying the provenance of the Ordos Deserts as inferred from the geochemical composition.
The chemical index of alteration (CIA), proposed by Nesbitt and Young (1982), is an ideal chemical indicator for estimating the chemical weathering intensity of sediments. Generally, higher weathering intensity tends to result in a larger CIA value of the sediment (Taylor and McLennan, 1985). The range of CIA values for the Mu Us is extremely narrow (45.03–53.89, average 50), indicating that aeolian sands are in the initial stage of weathering and have undergone a low degree of weathering (Figure 10A). Also, the Plagioclase Index of Alteration (PIA) is an essential chemical indicator to evaluate the chemical weathering intensity (Fedo et al., 1995). The PIA values of the studied samples are similar to those of the CIA, ranging from 43.2 to 55.87, which also indicates a low degree of chemical weathering (Figure 10B). Sediments from the Hobq Desert also show a low degree of chemical weathering (Appendix A). In addition, the diagram of mafic-felsic-weathering (MFW) can also evaluate the intensity and tendency of weathering (Ohta and Arai, 2007). Compared with the diagram of A-CN-K (where A = Al2O3, CN = CaO*+Na2O, K = K2O), the MFW diagram is more sensitive for characterizing the chemical weathering as a result of it being defined by eight major elements. A linear compositional trend extending from the M apex to the F apex can be defined by igneous rocks ranging from felsic to mafic and ultramafic (Ohta and Arai, 2007). The weathering trend of mafic rocks tends to be closer to the M-W join, which is evidently different from the weathering trend of felsic rocks that is extended from the F apex to the W apex. Samples in the Ordos Deserts near the igneous rock compositional trend (solid red line in Figure 10C) and close to the F-W line (dotted blue line in Figure 10C), but far away from W apex, indicate a low degree of chemical weathering, as illustrated by the CIA and PIA values. Overall, therefore, the CIA, PIA, and MFW values of the sediments from the Ordos Deserts all indicate a low degree of chemical weathering, with the degree of chemical weathering gradually increasing from north to south in the Mu Us (Figure 10).
As a significant sedimentary process, the mechanical sorting of grains occurs in the process of transport and deposition (Perri et al., 2013). As suggested by Garcia et al. (1994), the ternary diagram of Al2O3-TiO2-Zr can highlight the effects of sorting-related fractionations due to Al, Ti and Zr are generally viewed as the least mobile elements in the process of chemical weathering of the major and trace elements, i.e., these elements show little or no change when they are transported from the source areas to the sedimentary areas. Generally, the ratios of TiO2/Zr or Al2O3/Zr of mature sediments show a wide variation, contrary to the immature sediments that exhibit a limited variation. We can see that samples from the Ordos Deserts, especially fine fractions, show a mixing trend toward the Zr apex, indicating that the effect of sedimentary sorting and the increase in zircon results from robust sedimentary recycling (Figure 10D).
It is acknowledged that mechanical sorting may cause the separation of coarse and fine particles, and thus have an effect on the chemical composition of clastic sediments in the process of transport and deposition (Perri et al., 2013). However, evidence shows that mechanical sorting in obscuring sediment provenance is inefficient in fluvial and aeolian environments (Garzanti et al., 2012). In addition, chemical weathering tends to break the source rocks down to clays, which usually occurs in the source area (Sawyer, 1986). Hence, we believe that chemical composition can be used for provenance analysis in the Ordos Deserts since it is rarely affected by the above factors.
Types of Main Source Rocks of the Aeolian Sediments in the Ordos Deserts
Previous studies have illustrated that the chemical composition of sedimentary rocks is closely connected to that of their source regions (Fralick and Kronberg, 1997; Kalsbeek and Frei, 2010). Therefore, many discrimination diagrams related to the major and trace elements, and REE have been put forward to allow the discrimination of the provenance of source rocks (Hayashi et al., 1997).
According to the provenance discrimination diagram proposed by Roser and Korsch (1988), the DF1 and DF2 were calculated by the following equations:
we conclude that the majority of aeolian sands from the Ordos Deserts plotted in the field of felsic igneous, indicating that their source rocks are mainly felsic igneous (Figure 11A). The elements Ti and Zr show little or no change when they are transported from the source areas to the sedimentary areas for they are generally viewed as the least mobile elements in the process of chemical weathering (Garcia et al., 1994). Therefore, the TiO2/Zr ratio can reveal useful information on the chemical nature of parent rock. As suggested by Hayashi et al. (1997), different source rock types, i.e., felsic rocks, intermediate rocks and mafic rocks, can be distinguished by the ratio of TiO2/Zr. The TiO2/Zr ratio in felsic rocks is greater than 200, while in intermediate rocks is between 55 and 200. A TiO2/Zr ratio less than 55 is suggestive of felsic source rocks. Ratio of TiO2/Zr for bulk particle from the Ordos Deserts tends to be less than 55, indicating they may originate from a felsic provenance (Figure 11B). With further analysis of the Zr to Hf ratio (Supplementary Figure S4), we conclude that all samples from the Ordos Deserts (average 33) are close to the ratio of the UCC (32.8), providing evidence for a crustal origin of the zircons and further demonstrating a granitoid source of the zircons in the Ordos Deserts (Batchelor and Bowden, 1985). Furthermore, the trends of weathering and the composition of the source rocks can also be determined by the triangular diagram of A-CN-K and A-CNK-FM (Nesbitt and Young, 1984) where A = Al2O3, CN = CaO*+Na2O, K = K2O, CNK = CaO*+Na2O+K2O, and FM = Fe2O3 + MgO (all in mol%). All samples from the Ordos Deserts when plotted in the A-CN-K diagram (in molecular proportions) show that the weathering trend shown by our data is obviously parallel to the A-CN join, but does not tend toward the K apex (Figure 11C). It can be concluded that the sediments in the Ordos Deserts have not been influenced by potash metasomatism and they are in the early stage of Na and Ca removal. What stands out in Figure 11C is that the intersection between the weathering trend line and the feldspar line (Pl-Ks) appears in the field between granite (G) and granodiorite (Gd), indicating the main source rocks of sands in the Ordos Deserts are probably granite and granodiorite (Hu and Yang, 2016). In the ternary diagram of A-CNK-FM, all samples lie between granite (G) and granodiorite (Gd) and cluster near the feldspar-FM joins, which is commonly recognized as an igneous compositional linear trend (Figure 11D). Therefore, these samples show a low degree of weathering and an igneous source as illustrated by the ternary diagram of A-CN-K, showing that aeolian sands in the Ordos Deserts tend to have a felsic rocks source.
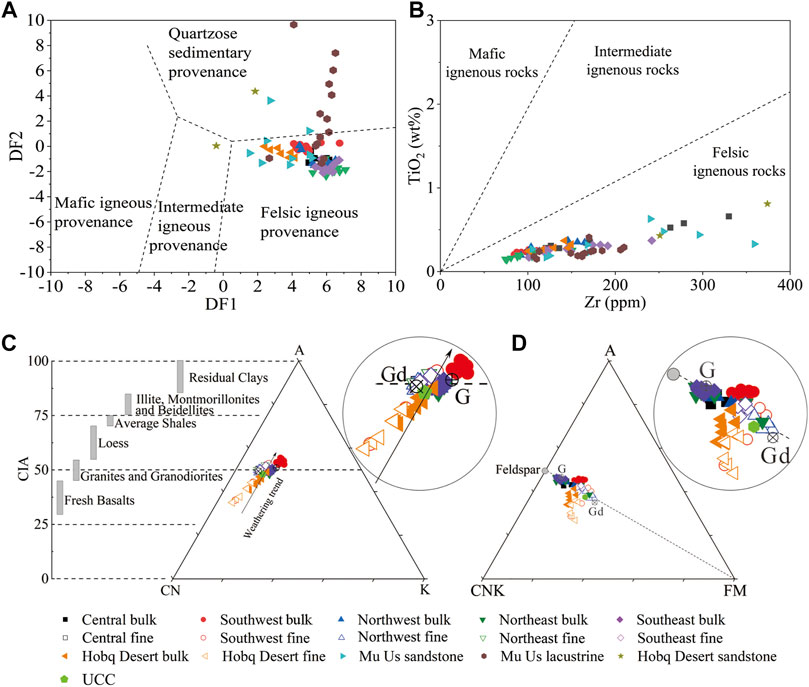
FIGURE 11. Diagrams characterizing primitive source rock nature for the sediments in the Ordos Deserts. (A) DF1 vs. DF2. (B) Zr vs. TiO2 plot. The triangular diagram of A-CN-K (C) and A-CNK-FM (D) for sediments in the Ordos Deserts. Gd and G represent granitoid and granite, respectively. The CIA values of fresh basalts, granitoid and granite, loess, average shales, illites, montmorillonites, beidellites, and residual clays are from Taylor and McLennan (1985) and Nesbitt and Young (1982). Data of sandstone and lacustrine sediments in Mu Us and the Hobq Desert is from Liu and Yang (2018).
Identification of the Provenance of the Ordos Deserts
The Provenance of Aeolian Sands in the Southwest Region of Mu Us and the Hobq Desert
As we discussed above, a series of geochemical diagrams show that sediments in the Ordos Desert are mainly derived from felsic rocks. Therefore, we can determine the provenance of these sediments by comparing the relevant geochemical parameters between the sediments of the Ordos Desert and its potential source rocks.
Clastic sediments, as the source of Quaternary aeolian depositions, are the result of a combination of multiple processes, such as active tectonics, glacial erosion, fluvial washout, chemical, and mechanical weathering, etc. They are then transported, with the aid of wind or rivers (Harvey, 2012; Lancaster et al., 2015). As clearly shown in Figure 1, the east, north, and west of the Ordos Deserts are surrounded by the Yellow River, and to the south, there is the CLP. The Langshan Mountain is an offshoot of the Yinshan Mountain range. Considering that the Helan Mountains and the Langshan Mountain are located in the upwind areas of the Ordos Deserts (Figure 2), we concluded that the Helan Mountains, the Langshan Mountain, and the sediments from the surrounding Yellow River might contribute tremendous amounts of material to the Ordos Deserts.
Although the Helan Mountains and the Langshan Mountain are located in the upwind areas of the Mu Us Sandy Land, their detritus could not be the source for the southwest of the Mu Us because significant differences exist in the elemental composition (Figure 12). It is clear that the samples from the southwest region of the Mu Us did not concentrate in the field of its potential source area, indicating the Helan Mountains and the Langshan Mountain are not the source areas for the Ordos Deserts. Thus, we conclude that the fluvial sediments from the upper Yellow River may be the potential source of the southwest region of the Mu Us for its unique geographic location (located in the upwind direction of the Ordos Plateau). The evidence presented in Figure 12 provides support for the above-mentioned hypothesis. The geochemical characteristics of sediments from the upper reach of Yellow River are similar to the samples from the Hobq Desert and the southwest region of the Mu Us, indicating that fluvial deposits from the upper reach of the Yellow River are the primary sources for the Hobq Desert and the southwest region of the Mu Us.
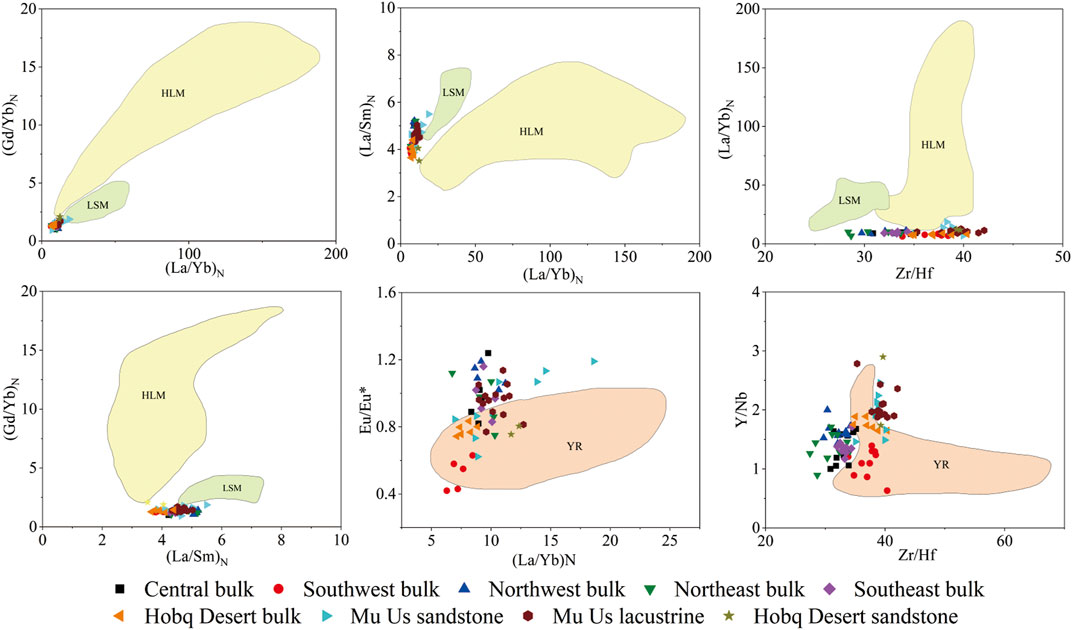
FIGURE 12. Bivariate diagrams of geochemical elements ratios of samples between the Ordos Deserts and their potential source areas. Data from other potential areas including those from the Helan Mountains (Dan et al., 2014; Li W. et al., 2017), the Langshan Mountain (Wang et al., 2017; Wang et al., 2018), the Yellow River (data from Rao et al., 2011b; Liu et al., 2013; Pang et al., 2018). Data of sandstone and lacustrine sediments in Mu Us and the Hobq Desert is from Liu and Yang (2018). Abbreviations: HLM, the Helan Mountains; LSM, the Langshan Mountain; YR, the Yellow River.
As the second-longest river in China, the Yellow River can be divided into three reaches: the upper, middle, and lower reaches. The upper reach of the Yellow River (from the headwater to Toudaoguai) flows through the Northeastern Tibetan Plateau (NTP), CLP, and the Inner Mongolia Plateau, which is characterized by loose riverbed sediments (Figure 1). As the last canyon in the upper reach of the Yellow River, the average gradient of the reach above Qingtong Gorge is 0.001605, and vast valleys and inter-mountainous basins are widely distributed in this area. Therefore, rivers in this section produce tremendous sediments and transport them downstream. In contrast, the downstream of the Qingtong Gorge flows through two plains, i.e., the Yinchuan Plain and the Hetao Plain, characterized by an average gradient of 0.000159 (Wang et al., 2012). Large quantities of deposits from the upper reaches of the Qingtong Gorge and upwind deserts near the Yellow River cause the Ningxia-Inner Mongolian section to appear as a “hanging river”. Studies illustrated that the total accumulated sediments of the Ningxia-Inner Mongolian section were about 20.1×108 t during the period 1954–2000, which supplies abundant material for aeolian activities in the surrounding area (Ta et al., 2003). Hence, the detrital deposits brought from the Yellow River and then deposited in the reach of Ningxia-Inner Mongolian are probably the immediate material source of the Hobq Desert and the southwest region of the Mu Us.
According to previous studies, the sediment feeding the Ningxia-Inner Mongolian section originates from three major sources: the UBD, the Hobq Desert, and the tributaries between Lanzhou and Toudaoguai (the Huangshui, Daxia, Tao, Zuli, and Qinshui Rivers). Among these three major sources, the contribution from the tributaries in the upstream basin is the largest (Ta et al., 2003; Ta et al., 2008; Liu, 2015). As discussed earlier, the UBD, which was blocked by the Helan Mountains, could not provide material for the southwest region of the Mu Us, and new evidence of heavy mineral assemblages in the UBD (Zhang et al., 2020) shows differences with what was found in the Mu Us (Figure 7). The tributaries between Lanzhou and Toudaoguai belong to the Qilian Orogenic Belt and the western Qinling Orogenic Belt according to the geotectonic units of China (Figure 1). Thus, it can be concluded that the detrital deposits from these belts may be source areas for sediments of the reach between Lanzhou and Toudaoguai. However, when geochemical comparisons between the sediments from the Ordos Deserts and the felsic rocks of the potential source areas are conducted, an overlapped field was only found among the Hobq Desert, the southwest region of Mu Us, and the Qilian Orogenic Belt (Figure 13). Therefore, we conclud that the original source area is likely to be the Qilian Orogenic Belt.
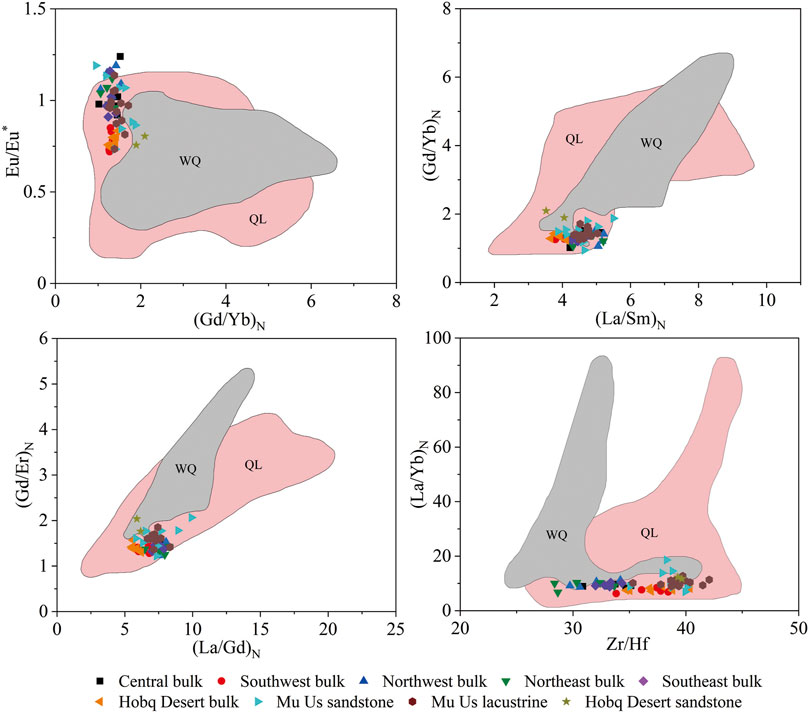
FIGURE 13. Bivariate diagrams of geochemical elements ratios of samples between the Ordos Deserts and their potential source areas. Data from other potential source areas including those from the Qilian Orogenic Belt (Wu et al., 2010; Tung et al., 2013; Li J. et al., 2017), the western Qinling Orogenic Belt (Zhang et al., 2007; Xin, 2014). Data of sandstone and lacustrine sediments in Mu Us and the Hobq Desert is from Liu and Yang (2018). Abbreviations: QL, the Qilian Orogenic Belt; WQ, the western Qinling Orogenic Belt.
The heavy mineral assemblages of the Hobq Desert and the southwest region of the Mu Us is consistent with that in the upper reach of the Yellow River (Pan et al., 2016), which further corroborates the previous views that there is a genetic lineage between the sediments transported from the NTP via the Yellow River and aeolian sediments in the southwest of the Mu Us (Stevens et al., 2013; Nie et al., 2015). The latest published data of heavy mineral assemblages in the southwest of the Mu Us has revealed that the Yellow River is a potential source for this region, which is exactly matched with the results of this article (Wang et al., 2019). Also, the quartz grains in the upper Yellow River displays typical aqueous characteristics such as V-shaped fractures and conchoidal fractures (Pan et al., 2016). Such characteristics resulting from the action of aqueous processes are also found in the southwest region of the Mu Us (Supplementary Figure S3), but the edges of these features tend to be smooth, indicating that the sand has been affected by aeolian activities after being transported to the sedimentary area by fluvial processes (Figure 8). Together, these results provide overwhelming evidence to conclude that the detrital sediments brought by the Yellow River from the NTP provide the sediments for the Hobq Desert and the southwest region of the Mu Us.
Our interpretation of the sediment source is also strongly supported by previous studies of detrital zircons from the Ordos Deserts. Stevens et al. (2013) and Nie et al. (2015) suggested that the U-Pb ages of detrital zircons from the NTP closely matched those from the western Mu Us (referred to as the southwest region in this article), indicating that substantial amounts of sediments were eroded from the NTP and, carried by the upper Yellow River, and then stored in the western Mu Us desert.
The Provenance of Aeolian Sands in Other Areas of the Mu Us
In the 1950s, Petrov (1959) suggested that the mineral characteristics of aeolian sands in the Mu Us inherited the nature of bedrock. That is, the potential source of the Mu Us is its underlying sandstone. However, this conclusion was based on samples from areas other than the southwest of the Mu Us. Zhu et al. (1980) and Wang (2013) also confirmed such conclusion through evidence from sedimentology and geomorphology. In recent years, some studies have confirmed multiple sources areas of the Mu Us by using diverse technologies, which are either from local sediments (Stevens et al., 2013; Liu and Yang, 2018; Wang et al., 2019) or from exterior sediments (Nie et al., 2015; Zhang et al., 2016; Hällberg et al., 2020), or both (Rao et al., 2011a; Rao et al., 2011b). The bedrocks of Cretaceous and Jurassic and lacustrine landforms are widely distributed in the central and western parts of the Mu Us (Wen et al., 2018), which may provide substantial detrital sediments for local aeolian activities (Zhu et al., 1980). Overlapped fields are found among the sandstone and lacustrine sediments, and the bulk and fine aeolian sands of other areas in the Mu Us (Figure 14), illustrating that the bedrock and lacustrine sediments are important potential sources.
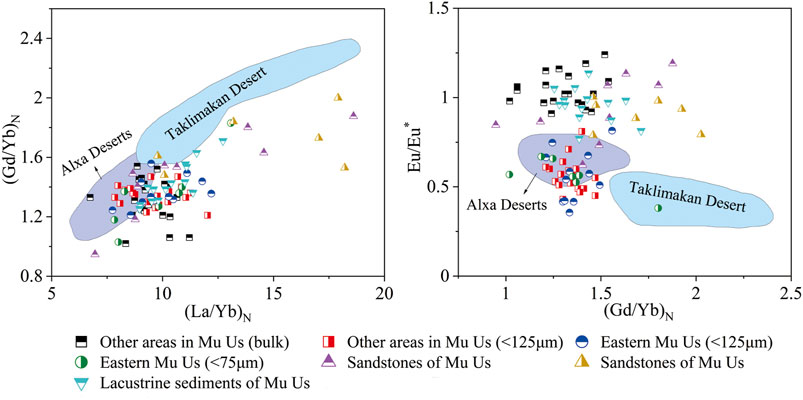
FIGURE 14. Bivariate diagrams of geochemical elements ratios of samples from other areas of the Mu Us Sandy Land and their potential source areas. Data from other studies include those from the eastern Mu Us, sandstone, and lacustrine sediments of the Mu Us (Rao et al., 2011b; Liu and Yang, 2018), the sands of Taklimakan Desert and Alxa Deserts (Hu and Yang, 2016; Jiang and Yang, 2019).
Furthermore, the combined actions of tectonic activities, frost weathering, fluvial comminution, and glacial abrasion have occurred in the Gobi Altay Mts and the AP may provide vast amounts of detrital material for downwind areas, i.e., the CLP (Sun, 2002). The comparisons of elemental ratios of fine sands between the Alxa Deserts and other areas of the Mu Us reveals more or less overlapped fields among them, whereas the fine sands of the Taklimakan Desert show no overlapped fields at all (Figure 14). Although the contribution of the Altay Mountains and the Mongolian Gobi is relatively small, it is one of the significant sources for the BJD (Hu and Yang, 2016). Therefore, we can conclude that the Alxa Deserts may also be a potential source of other areas of the Mu Us, which is supported by previous studies (Zhang et al., 2016; Sun et al., 2018; Hällberg et al., 2020).
The Link Between the Ordos Plateau and Its Surrounding Areas
As the longest and most continuous dust archive known, the CLP is an ideal and critical area for understanding the wind circulation patterns in East Asia. However, substantial issues, especially the source areas and transport pathways, remain controversial. Due to the wide extend of sandy deserts, mountain belts, and playas that lie to the north and northwest of the CLP, these areas are considered as the original dust sources of the CLP (Sun, 2002; Sun et al., 2007; Che and Li, 2013; Bird et al., 2020). Investigations into these areas have used various methods, including zircon U-Pb, isotopes, magnetic susceptibility, major and trace element chemistry, and heavy-mineral assemblage analysis, with each of these techniques providing different interpretations for the dust sources of the CLP. For example, the Ordos Plateau and its upwind areas, including the BJD, the TD, and the UBD, are located in the arid areas in the north of China, and their materials are considered to be the crucial sources of loess on the CLP (Che and Li, 2013; Zhang et al., 2016; Sun et al., 2018). However, some studies have suggested that dust from the northwestern inland basins, such as the Junggar Basin, the Tarim Basin, and the Qaidam Basin, are important dust sources for the CLP (Liu et al., 1994; Pullen et al., 2011). In addition, the north Tibetan Plateau also supplies substantial materials to the CLP through westerly winds, and by the Yellow River as outlined in other studies (Stevens et al., 2013; Nie et al., 2015). Therefore, we can see that the difference and diversity of provenance in the CLP implies a far more complex relationship between the Ordos Plateau and the CLP than previously envisioned.
The Ordos Deserts (the Mu Us Sandy Land and the Hobq Desert) and the Alxa Deserts (the BJD, the TD, and the UBD) are the proximal areas upwind of the CLP (Figure 1). The latest geochemical data has demonstrated that Qilian Mountains of the NTP contributes more to the BJD than the AP, but this does not exclude that the AP is not the provenance area of the BJD (Hu and Yang, 2016), since Che and Li (2013) proposed that the zircons in the Alxa arid lands are mixture of those from NTP and Gobi Altay Mountains, and are better matched with zircons from the CLP. In addition, Liu et al. (2017) suggested that Alxa arid lands and the Mu Us desert were the dominant dust sources of the CLP during interglacial and interstadial warm stages, and this has been confirmed by additional studies (Sun et al., 2018; Zhang et al., 2018; Fan et al., 2019; Hällberg et al., 2020). As a consequence, our findings that the AP may be a potential source for the Mu Us is credible. It can therefore be seen from the complexity of the loess provenances that the identification of aeolian sediment provenances in arid and semi-arid areas of China needs a variety of comprehensive analytical methods.
Sedimentary Process
Generally, wind is considered as the significant transport agent that contributes to the accumulation of sand in arid areas, whereas fluvial processes often play a substantial role in presorting and concentrating the products of weathering before aeolian transport takes place (Pye and Tsoar, 2009). Go to a step further, sediment transport are usually the combined results of fluvial-aeolian systems in some inland sand seas, such as the Great Sand Dunes of Colorado (Pye and Tsoar, 2009), the Namib Sand Sea (Garzanti et al., 2012), the Wahiba Sand Sea (Garzanti et al., 2013b), the Taklamakan Desert (Jiang and Yang, 2019), the Badain Jaran Desert (Hu and Yang, 2016), the Kumtagh Sand sea (Liang et al., 2020), etc. The weathering products were denudated from the NTP and then undergone long-distance fluvial transport by the Yellow River. These detrital sediments deposited in the southwest region of the Mu Us Sandy Land and then reworked by the aeolian activities, which shows better sorting, higher mineralogical maturity, and typical quartz grains characteristics in aqueous environments (Figures 3, 8, 9, Figure 8 and Figure 9). In addition, the weathering products from Altay Mountains and the Mongolian Gobi were transported by fluvial processes and reworked by wind and then deposited in other regions of the Mu Us Sandy Land through westerly winds. It is evident that the role of the fluvial system is significant and must be taken seriously for it may exert considerable control on sediment transport in a sand sea.
Conclusions
We have systematically collected aeolian sediments from Ordos Deserts, to characterize their textural, mineralogical, geochemical, sedimentological signatures and to discriminate their potential sources. The main conclusions are as follows:
1) Provenance differences exist between the southwest region of the Mu Us Sandy Land and other areas. The evidence obtained by other methods also support this conclusion, i.e., the heavy-mineral assemblages and mineralogical maturity, the content of major, trace, and REE elements, the surface micro-textures of quartz grains of southwest region in the Mu Us Sandy Land and the Hobq Desert shows similarity, but their characteristics are obviously different from other regions of the Mu Us.
2) Based on low values of CIA and PIA, as well as MFW diagrams, aeolian sediments from the Ordos Plateau have experienced a low degree of chemical weathering in the source area. The source of aeolian sands in the Ordos Deserts tend to have felsic igneous rocks detected by a series of diagrams, such as DF1 vs. DF2 diagram, Zr vs. TiO2, as well as A-CN-K and A-CNK-FM diagrams.
3) By comparing to geochemical elements of felsic rocks in potential source areas, it is found that aeolian sands from the Hobq Desert and southwest regions of the Mu Us are mainly originated from fluvial sediments denuded from the NTP and deposited in the section of Ningxia-Inner Mongolian of the Yellow River, whereas aeolian sands from other areas of the Mu Us are a mixture of binary provenance, with one sourced from local lacustrine sediments and underlying sandstones, and another from the AP via northwesterly Asian winter monsoon.
4) Fluvial action often plays a significant role in the process of sediment transport and supply for sand seas, and sometimes even plays a decisive role. Therefore, for understanding modern sand seas formation and evolution, we must take the role of fluvial processes seriously.
Data Availability Statement
The original contributions presented in the study are included in the article/Supplementary Material, further inquiries can be directed to the corresponding author.
Author Contributions
Conceptualization: GC and ZD; Data Curation and Methodology: GC and CL; Analysis and Supervision: WS, TS, WN, and JY.
Funding
This study was financially supported by the National Natural Science Foundation of China (41930641) and the Fundamental Research Funds for the Central Universities (2019TS012).
Conflict of Interest
The authors declare that the research was conducted in the absence of any commercial or financial relationships that could be construed as a potential conflict of interest.
Supplementary Material
The Supplementary Material for this article can be found online at: https://www.frontiersin.org/articles/10.3389/feart.2021.711802/full#supplementary-material
Supplement Table 1 | Results of major, trace and rare earth elements for different sediments and grain size fractions in the Mu Us Sandy Land and the Hobq Desert.
References
Arimoto, R. (2001). Eolian Dust and Climate: Relationships to Sources, Tropospheric Chemistry, Transport and Deposition. Earth-Science Rev. 54, 29–42. doi:10.1016/S0012-8252(01)00040-X
Batchelor, R. A., and Bowden, P. (1985). Petrogenetic Interpretation of Granitoid Rock Series Using Multicationic Parameters. Chem. Geology. 48, 43–55. doi:10.1016/0009-2541(85)90034-8
Bird, A., Millar, I., Rodenburg, T., Stevens, T., Rittner, M., Vermeesch, P., et al. (2020). A Constant Chinese Loess Plateau Dust Source since the Late Miocene. Quat. Sci. Rev. 227, 106042. doi:10.1016/j.quascirev.2019.106042
Che, X., and Li, G. (2013). Binary Sources of Loess on the Chinese Loess Plateau Revealed by U-Pb Ages of Zircon. Quat. Res. 80, 545–551. doi:10.1016/j.yqres.2013.05.007
Chen, J., and Li, G. (2011). Geochemical Studies on the Source Region of Asian Dust. Sci. China Earth Sci. 54, 1279–1301. doi:10.1007/s11430-011-4269-z
Dan, W., Li, X.-H., Wang, Q., Wang, X.-C., Liu, Y., and Wyman, D. A. (2014). Paleoproterozoic S-type Granites in the Helanshan Complex, Khondalite Belt, North China Craton: Implications for Rapid Sediment Recycling during Slab Break-Off. Precambrian Res. 254, 59–72. doi:10.1016/j.precamres.2014.07.024
Dehghani, S., Moore, F., Vasiluk, L., and Hale, B. A. (2018). The Geochemical Fingerprinting of Geogenic Particles in Road Deposited Dust from Tehran metropolis, Iran: Implications for Provenance Tracking. J. Geochem. Explor. 190, 411–423. doi:10.1016/j.gexplo.2018.04.011
Dong, Z., Qian, G., Lv, P., and Hu, G. (2013). Investigation of the Sand Sea with the Tallest Dunes on Earth: China's Badain Jaran Sand Sea. Earth-Science Rev. 120, 20–39. doi:10.1016/j.earscirev.2013.02.003
Dong, Z., Hu, G., Qian, G., Lu, J., Zhang, Z., Luo, W., et al. (2017). High-Altitude Aeolian Research on the Tibetan Plateau. Rev. Geophys. 55, 864–901. doi:10.1002/2017RG000585
Du, S., Wu, Y., and Tan, L. (2018). Geochemical Evidence for the Provenance of Aeolian Deposits in the Qaidam Basin, Tibetan Plateau. Aeolian Res. 32, 60–70. doi:10.1016/j.aeolia.2018.01.005
Fan, Y., Li, Z., Wang, F., Ma, J., Mou, X., Li, X., et al. (2019). Provenance Variations of the Tengger Desert since 2.35 Ma and its Linkage with the Northern Tibetan Plateau: Evidence from U-Pb Age Spectra of Detrital Zircons. Quat. Sci. Rev. 223, 1–10. doi:10.1016/j.quascirev.2019.105916
Fedo, C., Nesbitt, H. W., and Young, G. (1995). Unraveling the Effects of Potassium Metasomatism in Sedimentary Rocks and Paleosols, with Implications for Paleoweathering Conditions and Provenance. Geology 23, 921–924. doi:10.1130/0091-7613(1995)023<0921:UTEOPM>2.3.CO;2
Folk, R. L., and Ward, W. C. (1957). Brazos River Bar [Texas]; a Study in the Significance of Grain Size Parameters. J. Sediment. Res. 27, 3–26. doi:10.1306/74D70646-2B21-11D7-8648000102C1865D
Fralick, P. W., and Kronberg, B. I. (1997). Geochemical Discrimination of Clastic Sedimentary Rock Sources. Sediment. Geology. 113, 111–124. doi:10.1016/S0037-0738(97)00049-3
Fryberger, S. G. (1979). “Dune Forms and Wind Regime,” in A Study of Global Sand Seas: United States Geological Survey Professional Paper. Editor E. McKee (Washington: Government Printing Office).
Garcia, D., Fonteilles, M., and Moutte, J. (1994). Sedimentary Fractionations between Al, Ti, and Zr and the Genesis of Strongly Peraluminous Granites. J. Geology. 102, 411–422. doi:10.1086/629683
Garzanti, E., Vezzoli, G., Andò, S., Lavé, J., Attal, M., France-Lanord, C., et al. (2007). Quantifying Sand Provenance and Erosion (Marsyandi River, Nepal Himalaya). Earth Planet. Sci. Lett. 258, 500–515. doi:10.1016/j.epsl.2007.04.010
Garzanti, E., Andò, S., and Vezzoli, G. (2008). Settling Equivalence of Detrital Minerals and Grain-Size Dependence of Sediment Composition. Earth Planet. Sci. Lett. 273, 138–151. doi:10.1016/j.epsl.2008.06.020
Garzanti, E., Andò, S., Vezzoli, G., Lustrino, M., Boni, M., and Vermeesch, P. (2012). Petrology of the Namib Sand Sea: Long-Distance Transport and Compositional Variability in the Wind-Displaced Orange Delta. Earth-Sci. Rev. 112, 173–189. doi:10.1016/j.earscirev.2012.02.008
Garzanti, E., Padoan, M., Andò, S., Resentini, A., Vezzoli, G., and Lustrino, M. (2013a). Weathering and Relative Durability of Detrital Minerals in Equatorial Climate: Sand Petrology and Geochemistry in the East African Rift. J. Geology. 121, 547–580. doi:10.1086/673259
Garzanti, E., Vermeesch, P., Andò, S., Vezzoli, G., Valagussa, M., Allen, K., et al. (2013b). Provenance and Recycling of Arabian Desert Sand. Earth-Sci. Rev. 120, 1–19. doi:10.1016/j.earscirev.2013.01.005
Goudie, A. S., and Middleton, N. J. (2001). Saharan Dust Storms: Nature and Consequences. Earth-Sci. Rev. 56, 179–204. doi:10.1016/S0012-8252(01)00067-8
Goudie, A. S. (2009). Dust Storms: Recent Developments. J. Environ. Manage. 90, 89–94. doi:10.1016/j.jenvman.2008.07.007
Hällberg, L. P., Stevens, T., Almqvist, B., Snowball, I., Wiers, S., Költringer, C., et al. (2020). Magnetic Susceptibility Parameters as Proxies for Desert Sediment Provenance. Aeolian Res. 46, 100615. doi:10.1016/j.aeolia.2020.100615
Harvey, A. (2012). Introducing Geomorphology: A Guide to Landforms and Processes. Edinburgh: Dunedin Academic Press.
Hayashi, K.-I., Fujisawa, H., Holland, H. D., and Ohmoto, H. (1997). Geochemistry of ∼1.9 Ga Sedimentary Rocks from Northeastern Labrador, Canada1.9 Ga Sedimentary Rocks from Northeastern Labrador, Canada. Geochim. Cosmochim. Acta 61, 4115–4137. doi:10.1016/S0016-7037(97)00214-7
Honda, M., Yabuki, S., and Shimizu, H. (2004). Geochemical and Isotopic Studies of Aeolian Sediments in China. Sedimentology 51, 211–230. doi:10.1111/j.1365-3091.2004.00618.x
Howari, F. M., Baghdady, A., and Goodell, P. C. (2007). Mineralogical and Gemorphological Characterization of Sand Dunes in the Eastern Part of United Arab Emirates Using Orbital Remote Sensing Integrated with Field Investigations. Geomorphology 83, 67–81. doi:10.1016/j.geomorph.2006.06.015
Hu, F., and Yang, X. (2016). Geochemical and Geomorphological Evidence for the Provenance of Aeolian Deposits in the Badain Jaran Desert, Northwestern China. Quat. Sci. Rev. 131, 179–192. doi:10.1016/j.quascirev.2015.10.039
Jiang, Q., and Yang, X. (2019). Sedimentological and Geochemical Composition of Aeolian Sediments in the Taklamakan Desert: Implications for Provenance and Sediment Supply Mechanisms. J. Geophys. Res. Earth Surf. 124, 1217–1237. doi:10.1029/2018JF004990
Kalsbeek, F., and Frei, R. (2010). Geochemistry of Precambrian Sedimentary Rocks Used to Solve Stratigraphical Problems: An Example from the Neoproterozoic Volta basin, Ghana. Precambrian Res. 176, 65–76. doi:10.1016/j.precamres.2009.10.004
Krinsley, D., and Doornkamp, J. (1973). Atlas of Quartz Sand Surface Textures. Cambridge: Cambridge University Press.
Lancaster, N., Baker, S., Bacon, S., and McCarley-Holder, G. (2015). Owens Lake Dune fields: Composition, Sources of Sand, and Transport Pathways. Catena 134, 41–49. doi:10.1016/j.catena.2015.01.003
Lancaster, N. (1989). Star Dunes. Prog. Phys. Geogr. Earth Environ. 13, 67–91. doi:10.1177/030913338901300105
Li, J., Zhou, L., Yan, J., Cui, X., and Cai, Y. (2019). Source of Aeolian Dune Sands on the Northern Margin of Qarhan Salt Lake, Qaidam Basin, NW China. Geol. J. 55, 3643–3653. doi:10.1002/gj.3624
Li, J., Niu, Y., Chen, S., Sun, W., Zhang, Y., Liu, Y., et al. (2017). Petrogenesis of Granitoids in the Eastern Section of the Central Qilian Block: Evidence from Geochemistry and Zircon U-Pb Geochronology. Miner Petrol. 111, 23–41. doi:10.1007/s00710-016-0461-3
Li, W., Yin, C., Long, X., Zhang, J., Xia, X., and Wang, L. (2017). Paleoproterozoic S-type Granites from the Helanshan Complex in Inner Mongolia: Constraints on the Provenance and the Paleoproterozoic Evolution of the Khondalite Belt, North China Craton. Precambrian Res. 299, 195–209. doi:10.1016/j.precamres.2017.07.0097
Liang, X., Niu, Q., Qu, J., Liu, B., Liu, B., Zhang, C., et al. (2019). Geochemical Analysis of Yardang Strata in the Dunhuang Yardang National Geopark, Northwest China, and Implications on its Palaeoenvironment, Provenance, and Potential Dynamics. Aeolian Res. 40, 91–104. doi:10.1016/j.aeolia.2019.06.003
Liang, A., Dong, Z., Su, Z., Qu, J., Zhang, Z., Qian, G., et al. (2020). Provenance and Transport Process for Interdune Sands in the Kumtagh Sand Sea, Northwest China. Geomorphology 367, 107310. doi:10.1016/j.geomorph.2020.107310
Liu, Q., and Yang, X. (2018). Geochemical Composition and Provenance of Aeolian Sands in the Ordos Deserts, Northern China. Geomorphology 318, 354–374. doi:10.1016/j.geomorph.2018.06.017
Liu, C.-Q., Masuda, A., Okada, A., Yabuki, S., and Fan, Z.-L. (1994). Isotope Geochemistry of Quaternary Deposits from the Arid Lands in Northern China. Earth Planet. Sci. Lett. 127, 25–38. doi:10.1016/0012-821X(94)90195-3
Liu, J., Lai, Z., and Liu, Y. (2013). Study on Speciation and Fractionation of Rare Earth Eleinents in Surface Sediments in Gansu, Ningxia and Inner Mongolia Sections of Yellow River. Spectroscopy Spectral Analy. 33, 798–803. doi:10.3964/j.issn.1000-0593(2013)03-0798-06
Liu, B., Jin, H., Sun, L., Sun, Z., and Zhao, S. (2015). Geochemical Evidence for Holocene Millennial-Scale Climatic and Environmental Changes in the South-Eastern Mu Us Desert, Northern China. Int. J. Earth Sci. (Geol Rundsch) 104, 1889–1900. doi:10.1007/s00531-015-1161-7
Liu, X.-J., Xiao, G., E, C., Li, X., Lai, Z., Yu, L., et al. (2017). Accumulation and Erosion of Aeolian Sediments in the Northeastern Qinghai-Tibetan Plateau and Implications for Provenance to the Chinese Loess Plateau. J. Asian Earth Sci. 135, 166–174. doi:10.1016/j.jseaes.2016.12.034
Liu, C. (2015). Analysis of Sediment Source of the Inner Mongolia Section of the Yellow River. Lan Zhou: University of Lanzhou. doi:10.2495/feem140411
Livingstone, I., Bullard, J. E., Wiggs, G. F. S., and Thomas, D. S. T. (1999). Grain-Size Variation on Dunes in the Southwest Kalahari, Southern Africa. J. Sediment. Res. 69, 546–552. doi:10.1306/D4268A31-2B26-11D7-8648000102C1865D10.2110/jsr.69.546
Mahaney, W. (2002). Atlas of Sand Grain Surface Textures and Applications. New York: Oxford University Press.
Mange, M., and Maurer, H. (1992). Heavy Minerals in Colour. London, UK: Chapman & Hall. doi:10.1007/978-94-011-2308-2
McLennan, S. M. (1993). Weathering and Global Denudation. J. Geology. 101, 295–303. doi:10.1086/648222
Molnar, P. (2004). Late Cenozoic Increase in Accumulation Rates of Terrestrial Sediment: How Might Climate Change Have Affected Erosion Rates?. Annu. Rev. Earth Planet. Sci. 32, 67–89. doi:10.1146/annurev.earth.32.091003.143456
Moral Cardona, J. P., Gutiérrez Mas, J. M., Sánchez Bellón, A., Domínguez-Bella, S., and Martínez López, J. (2005). Surface Textures of Heavy-mineral Grains: a New Contribution to Provenance Studies. Sediment. Geology. 174, 223–235. doi:10.1016/j.sedgeo.2004.12.006
Muhs, D. R., Roskin, J., Tsoar, H., Skipp, G., Budahn, J. R., Sneh, A., et al. (2013). Origin of the Sinai-Negev Erg, Egypt and Israel: Mineralogical and Geochemical Evidence for the Importance of the Nile and Sea Level History. Quat. Sci. Rev. 69, 28–48. doi:10.1016/j.quascirev.2013.02.022
Nesbitt, H. W., and Young, G. M. (1982). Early Proterozoic Climates and Plate Motions Inferred from Major Element Chemistry of Lutites. Nature 299, 715–717. doi:10.1038/299715a0
Nesbitt, H. W., and Young, G. M. (1984). Prediction of Some Weathering Trends of Plutonic and Volcanic Rocks Based on Thermodynamic and Kinetic Considerations. Geochim. Cosmochim. Acta 48, 1523–1534. doi:10.1016/0016-7037(84)90408-3
Nesbitt, H. W., Young, G. M., McLennan, S. M., and Keays, R. R. (1996). Effects of Chemical Weathering and Sorting on the Petrogenesis of Siliciclastic Sediments, with Implications for Provenance Studies. J. Geology. 104, 525–542. doi:10.1086/629850
Nie, J., Stevens, T., Rittner, M., Stockli, D., Garzanti, E., Limonta, M., et al. (2015). Loess Plateau Storage of Northeastern Tibetan Plateau-Derived Yellow River Sediment. Nat. Commun. 6, 8511. doi:10.1038/ncomms951110.1038/ncomms9511
Ohta, T., and Arai, H. (2007). Statistical Empirical index of Chemical Weathering in Igneous Rocks: A New Tool for Evaluating the Degree of Weathering. Chem. Geology. 240, 280–297. doi:10.1016/j.chemgeo.2007.02.017
Pan, B., Pang, H., Gao, H., Garzanti, E., Zou, Y., Liu, X., et al. (2016). Heavy-mineral Analysis and Provenance of Yellow River Sediments Around the China Loess Plateau. J. Asian Earth Sci. 127, 1–11. doi:10.1016/j.jseaes.2016.06.006
Pang, H., Pan, B., Garzanti, E., Gao, H., Zhao, X., and Chen, D. (2018). Mineralogy and Geochemistry of Modern Yellow River Sediments: Implications for Weathering and Provenance. Chem. Geology. 488, 76–86. doi:10.1016/j.chemgeo.2018.04.010
Perri, F., Critelli, S., Martín-Algarra, A., Martín-Martín, M., Perrone, V., Mongelli, G., et al. (2013). Triassic Redbeds in the Malaguide Complex (Betic Cordillera - Spain): Petrography, Geochemistry and Geodynamic Implications. Earth-Sci. Rev. 117, 1–28. doi:10.1016/j.earscirev.2012.11.002
Petrov, M. П. (1959). The mineral Element and Origin of Sand in the Ordos, East Alxa and Middle valley of Yellow River. Acta Geogr. Sinica 5, 1–20.
Pettijohn, F. J., Potter, P. E., and Siever, R. (1972). Sand and Sandstone. Berlin: Springer-Verlag.
Pullen, A., Kapp, P., McCallister, A. T., Chang, H., Gehrels, G. E., Garzione, C. N., et al. (2011). Qaidam Basin and Northern Tibetan Plateau as Dust Sources for the Chinese Loess Plateau and Paleoclimatic Implications. Geology 39, 1031–1034. doi:10.1130/G32296.1
Pye, K., and Tsoar, H. (2009). Aeolian Sand and Sand Dunes. Berlin: Springer. doi:10.1007/978-3-540-85910-9
Rao, W., Chen, J., Tan, H., Jiang, S., and Su, J. (2011a). Sr-Nd Isotopic and REE Geochemical Constraints on the Provenance of fine-grained Sands in the Ordos Deserts, north-central China. Geomorphology 132, 123–138. doi:10.1016/j.geomorph.2011.05.003
Rao, W., Tan, H., Jiang, S., and Chen, J. (2011b). Trace Element and REE Geochemistry of fine- and Coarse-Grained Sands in the Ordos Deserts and Links with Sediments in Surrounding Areas. Geochemistry 71, 155–170. doi:10.1016/j.chemer.2011.02.003
Rao, W., Chen, J., Tan, H., Weise, S. M., and Wang, Y. (2014). Nd-Sr Isotopic and REE Geochemical Compositions of Late Quaternary Deposits in the Desert-Loess Transition, north-central China: Implications for Their Provenance and Past Wind Systems. Quat. Int. 334-335, 197–212. doi:10.1016/j.quaint.2013.06.009
Roser, B. P., and Korsch, R. J. (1988). Provenance Signatures of sandstone-mudstone Suites Determined Using Discriminant Function Analysis of Major-Element Data. Chem. Geology. 67, 119–139. doi:10.1016/0009-2541(88)90010-1
Sawyer, E. W. (1986). The Influence of Source Rock Type, Chemical Weathering and Sorting on the Geochemistry of Clastic Sediments from the Quetico Metasedimentary Belt, Superior Province, Canada. Chem. Geology. 55, 77–95. doi:10.1016/0009-2541(86)90129-4
Shang, K., Dong, G., Wang, S., and Yang, D. (2001). Response of Climatic Change in North China Deserted Region to the Warming of the Earth. J. Desert Res. 21, 387–392. doi:10.3321/j.issn:1000-694X.2001.04.012
Stevens, T., Carter, A., Watson, T. P., Vermeesch, P., Andò, S., Bird, A. F., et al. (2013). Genetic Linkage between the Yellow River, the Mu Us Desert and the Chinese Loess Plateau. Quat. Sci. Rev. 78, 355–368. doi:10.1016/j.quascirev.2012.11.032
Sun, J., Li, S.-H., Muhs, D. R., and Li, B. (2007). Loess Sedimentation in Tibet: Provenance, Processes, and Link with Quaternary Glaciations. Quat. Sci. Rev. 26, 2265–2280. doi:10.1016/j.quascirev.2007.05.003
Sun, Y., Chen, H., Tada, R., Weiss, D., Lin, M., Toyoda, S., et al. (2013). ESR Signal Intensity and Crystallinity of Quartz from Gobi and sandy Deserts in East Asia and Implication for Tracing Asian Dust Provenance. Geochem. Geophys. Geosyst. 14, 2615–2627. doi:10.1002/ggge.20162
Sun, J., Ding, Z., Xia, X., Sun, M., and Windley, B. F. (2018). Detrital Zircon Evidence for the Ternary Sources of the Chinese Loess Plateau. J. Asian Earth Sci. 155, 21–34. doi:10.1016/j.jseaes.2017.10.012
Sun, Y., Yan, Y., Nie, J., Li, G., Shi, Z., Qiang, X., et al. (2020). Source-to-sink Fluctuations of Asian Aeolian Deposits since the Late Oligocene. Earth-Sci. Rev. 200, 102963. doi:10.1016/j.earscirev.2019.102963
Sun, J. (2002). Provenance of Loess Material and Formation of Loess Deposits on the Chinese Loess Plateau. Earth Planet. Sci. Lett. 203, 845–859. doi:10.1016/S0012-821X(02)00921-4
Ta, W., Yang, G., Qu, J., Wang, T., and Dai, F. (2003). The Effect of the Coarse Aeolian Sand on Siltation of the Inner Mongolian Reach of the Yellow River. Env Geol. 43, 493–502. doi:10.1007/s00254-002-0666-0
Ta, W., Xiao, H., and Dong, Z. (2008). Long-term Morphodynamic Changes of a Desert Reach of the Yellow River Following Upstream Large Reservoirs' Operation. Geomorphology 97, 249–259. doi:10.1016/j.geomorph.2007.08.008
Taylor, S. R., and McLennan, S. M. (1985). The Continental Crust: Its Composition and Evolution. United States: Blackwell Press.
Tung, K.-a., Yang, H.-y., Liu, D.-y., Zhang, J.-x., Yang, H.-j., Shau, Y.-h., et al. (2013). The Neoproterozoic Granitoids from the Qilian Block, NW China: Evidence for a Link between the Qilian and South China Blocks. Precambrian Res. 235, 163–189. doi:10.1016/j.precamres.2013.06.016
Vos, K., Vandenberghe, N., and Elsen, J. (2014). Surface Textural Analysis of Quartz Grains by Scanning Electron Microscopy (SEM): From Sample Preparation to Environmental Interpretation. Earth-Sci. Rev. 128, 93–104. doi:10.1016/j.earscirev.2013.10.013
Wang, S., Yan, Y., and Li, Y. (2012). Spatial and Temporal Variations of Suspended Sediment Deposition in the Alluvial Reach of the Upper Yellow River from 1952 to 2007. Catena 92, 30–37. doi:10.1016/j.catena.2011.11.0110.1016/j.catena.2011.11.012
Wang, W., Teng, X., Liu, F., Teng, F., Guo, S., He, P., et al. (2017). Zircon U-Pb Chronology and Geochemical Characteristics of the Wuheertu Granite Mass in the Langshan, Inner Mongolia. J. Geomech. 23, 382–396. doi:10.3969/j.issn.1006-6616.2017.03.006
Wang, W., Teng, X., Liu, Y., Teng, F., Guo, S., He, P., et al. (2018). Geochemical Characteristic, LA-ICP-MS Zircon U-Pb Dating and Hf Isotopic Compositions of the Haorigeshan Monzogranite in the Langshan Region, Inner Mongolia. Acta Geol. Sinica 92, 2227–2247. doi:10.3969/j.issn.0001-5717.2018.11.004
Wang, Z., Wu, Y., Tan, L., Fu, T., Wen, Y., and Li, D. (2019). Provenance Studies of Aeolian Sand in Mu Us Desert Based on Heavy-mineral Analysis. Aeolian Res. 40, 15–22. doi:10.1016/j.aeolia.2019.05.003
Wang, T. (2013). Deserts and Aeolian Desertification in China. Hebei: Science and Technology Press. doi:10.2495/isme132472
Wen, Y., Hao, C., Tan, L., Li, D., Fu, T., Zhang, M., et al. (2018). Compilation of Geomorphic Map of the Mu Us Desert. J. Desert Res. 38, 508–515. doi:10.7522/j.issn.1000-694X.2017.00030
Wu, C., Xu, X., Gao, Q., Li, X., Lei, M., Gao, Y., et al. (2010). Early Palaezoic Grranitoid Magmatism and Tetonic Evolution in North Qilian, NW China. Acta Petrologica Sinica 26, 1027–1044.
Xin, Y. (2014). Geochemical Features of Granites and Tectonic Significance in the North of West Qinling Orogenic belt. Hu Nan: Central South University.
Yang, X., Zhu, B., and White, P. D. (2007). Provenance of Aeolian Sediment in the Taklamakan Desert of Western China, Inferred from REE and Major-Elemental Data. Quat. Int. 175, 71–85. doi:10.1016/j.quaint.2007.03.005
Zhang, Z., and Dong, Z. (2015). Grain Size Characteristics in the Hexi Corridor Desert. Aeolian Res. 18, 55–67. doi:10.1016/j.aeolia.2015.05.006
Zhang, H., Jin, L., Zhang, L., Nigel, H., Zhou, L., Hu, S., et al. (2007). Geochemical and Pb-Sr-Nd Isotopic Compositions of Granitoids from Western Qinling belt: Constraints on Basement Nature and Tectonic Affinity. Sci. China Ser. D 50, 184–196. doi:10.1007/s11430-007-2015-3
Zhang, Z., Dong, Z., and Li, J. (2015). Grain‐size Characteristics of Dune Networks in china's Tengger Desert. Geografiska Annaler: Ser. A, Phys. Geogr. 97, 681–693. doi:10.1111/geoa.12108
Zhang, H., Lu, H., Xu, X., Liu, X., Yang, T., Stevens, T., et al. (2016). Quantitative Estimation of the Contribution of Dust Sources to Chinese Loess Using Detrital Zircon U-Pb Age Patterns. J. Geophys. Res. Earth Surf. 121, 2085–2099. doi:10.1002/2016JF003936
Zhang, W., Zhao, J.-x., Chen, J., Ji, J., and Liu, L. (2018). Binary Sources of Chinese Loess as Revealed by Trace and REE Element Ratios. J. Asian Earth Sci. 166, 80–88. doi:10.1016/j.jseaes.2018.07.017
Zhang, C., Li, Z., Chen, Q., Dong, S., Yu, X., and Yu, Q. (2020). Provenance of Eolian Sands in the Ulan Buh Desert, Northwestern China, Revealed by Heavy mineral Assemblages. Catena 193, 104624. doi:10.1016/j.catena.2020.104624
Zhu, L.-m., Zhang, G.-w., Chen, Y.-j., Ding, Z.-j., Guo, B., Wang, F., et al. (2011). Zircon U-Pb Ages and Geochemistry of the Wenquan Mo-Bearing Granitioids in West Qinling, China: Constraints on the Geodynamic Setting for the Newly Discovered Wenquan Mo deposit. Ore Geology. Rev. 39, 46–62. doi:10.1016/j.oregeorev.2010.10.001
Keywords: sediment provenance, grain size, geochemical element, heavy mineral, quartz grain morphology, Ordos Deserts
Citation: Chen G, Dong Z, Li C, Shi W, Shao T, Nan W and Yang J (2021) Provenance of Aeolian Sediments in the Ordos Deserts and Its Implication for Weathering, Sedimentary Processes. Front. Earth Sci. 9:711802. doi: 10.3389/feart.2021.711802
Received: 19 May 2021; Accepted: 21 June 2021;
Published: 05 July 2021.
Edited by:
Kathryn Elizabeth Fitzsimmons, Max Planck Institute for Chemistry, GermanyReviewed by:
Benli Liu, Northwest Institute of Eco-Environment and Resources (CAS), ChinaXiaodong Miao, Linyi University, China
Copyright © 2021 Chen, Dong, Li, Shi, Shao, Nan and Yang. This is an open-access article distributed under the terms of the Creative Commons Attribution License (CC BY). The use, distribution or reproduction in other forums is permitted, provided the original author(s) and the copyright owner(s) are credited and that the original publication in this journal is cited, in accordance with accepted academic practice. No use, distribution or reproduction is permitted which does not comply with these terms.
*Correspondence: Zhibao Dong, zbdong@snnu.edu.cn