- 1Center for Advanced Marine Core Research, Kochi University, Kochi, Japan
- 2Graduate School of Integrated Arts and Sciences, Kochi University, Kochi, Japan
- 3College of Education, Yokohama National University, Yokohama, Japan
Oxygen isotopic compositions (δ18O) of benthic foraminifer tests are widely used for reconstructing paleoceanographic changes, such as global ice volumes during glacial–interglacial cycles. Although deep-sea benthic foraminifers have been well characterized and are considered reliable indicators, little attention has been paid to the geochemistry of shallow-water benthic foraminifers. In this study we evaluated δ18O in the shallow-water benthic foraminifer Hanzawaia nipponica Asano, which lives in surface sediments on continental shelves and upper slopes under the influence of two warm currents, the Kuroshio and Tsushima currents, in the East China Sea, northwest Pacific, and southwestern Japan Sea. To evaluate oxygen isotope equilibrium, we analyzed δ18O of H. nipponica and ambient seawater on the continental shelf in Tosa Bay, southwest Japan. Seawater δ18O and salinity in Tosa Bay are similar to those of surface and subsurface waters in the Kuroshio region in the Okinawa Trough and the northwest Pacific. Vertical profiles of seawater δ18O show no variation with water depth (0–200 m) in Tosa Bay. However, tests of living H. nipponica (as determined by staining with Rose Bengal) and fossil (non-stained) H. nipponica, picked from samples of the top centimeter of seafloor sediment, yielded carbonate δ18O values that clearly increase with water depth, suggesting a temperature-dependent relationship. A comparison of carbonate δ18O values in living H. nipponica and those predicted on the basis of seawater δ18O and annual mean bottom temperature shows that H. nipponica tests are in oxygen isotopic equilibrium with ambient seawater. We determined the linear equations of δ18O–temperature relationship, and the slope of −5.26 (0.19‰°C−1) for living and −4.50 (0.22‰°C−1) for the fossil H. nipponica, respectively. The carbon isotopic compositions (δ13C) of H. nipponica also closely match seawater δ13C. Thus, we propose that the carbonate δ18O and δ13C of H. nipponica are useful proxies to reconstruct shallow-water paleoenvironmental changes in the northwest Pacific and its marginal seas.
Introduction
The oxygen isotopic composition (δ18O) of the carbonate tests of benthic foraminifers is widely used in studying the geologic history of the oceans, especially the deep ocean. For example, early researchers argued that the deep-sea benthic foraminifer Uvigerina spp. appears to record δ18O of calcite in isotopic equilibrium with seawater (Shackleton, 1974) and that Cibicidoides wuellerstorfi deviates from equilibrium by −0.64‰ in the Pacific (Shackleton and Opdyke, 1973). Bemis et al. (1998) countered their argument by compiling published δ18O data for Uvigerina and Cibicidoides species from core tops in the Atlantic, Pacific, and Indian oceans and comparing them with predictions from the paleotemperature equation established by experimental results from planktic foraminifers, finding that Cibicidoides δ18O data were in excellent agreement with the equation whereas Uvigerina data were scattered. They concluded that the relative 18O enrichment of Uvigerina over Cibicidoides tests reflects the habitat differences of the genera: Uvigerina is infaunal and experiences low pH and decreased [CO32−] in pore waters, whereas Cibicidoides inhabits the sediment surface where bottom water pH and [CO32−] are higher (Bemis et al., 1998). As a result of these and other advances, much paleoceanographic research has made use of δ18O analyses of epifaunal deep-sea benthic foraminifers. In contrast, shallow-water benthic foraminifers have seen little application in paleoceanographic studies because it is assumed that δ18O in these species would be affected by freshwater input and larger temperature variations in the shallow-water environment. Although δ18O records from shallow-water settings are very important evidence of past environmental changes of the coastal ocean, there is presently no useful species of shallow-water benthic foraminifer to aid in reconstructing past δ18O changes.
Hanzawaia nipponica is a species of benthic foraminifer that was first reported from Pliocene strata in Kawasaki, on the Pacific coast of Japan, by Asano (1944) and has been subsequently reported from Pliocene to Pleistocene sedimentary sequences around Japan, such as in Okinawa (LeRoy, 1964), Kochi (Katto et al., 1953), and Shizuoka (Aoshima, 1978). Outside Japan, it has been recorded from upper Pliocene to lower Pleistocene shallow-marine deposits on Jeju Island in the Korea Strait (Li et al., 1999; Kang et al., 2010) and from a Holocene marine core in the Yellow Sea (Xiang et al., 2008). The living species is abundant in surface sediment on the continental shelf in the East China Sea (Inoue, 1989), off the San’in district in the southwest Japan Sea (Inoue, 1989), and in the Pacific Ocean near Japan off Kyushu Island (Akimoto and Torii, 1996), off Shikoku Island (Ishiwada, 1964; Inoue, 1989), off the Kii Peninsula (Uchio, 1968; Akimoto, 1990), in Suruga Bay (Akimoto, 1990), and off the Boso Peninsula (Kuwano, 1963). Living H. nipponica have been recorded at water depths ranging from 23 to 235 m (mainly at ∼40 m) off the Pacific coast of western Japan (Aoshima, 1978). These records suggest that the main habitat of this species is the shallow continental shelf along southwestern Japan, where the seafloor is influenced by two major warm currents, the Kuroshio and Tsushima currents (Ishiwada, 1964; Matoba, 1976; Matoba and Honma, 1986; Inoue, 1989; Hasegawa, 1993).
Oxygen and carbon isotope compositions have been reported for fossil specimens of H. nipponica from one horizon of middle Pleistocene age in the lower Seogwipo Formation on Jeju Island (Li et al., 1999); however, there is no published evidence bearing on the oxygen isotope equilibrium of this species with seawater. In this study, we analyzed the δ18O of ambient seawater on the continental shelf in Tosa Bay, off Shikoku Island, Japan, and contemporary H. nipponica tests from seafloor surface sediment in Tosa Bay, and we determined that the tests were in isotopic equilibrium with seawater. Our findings indicate that isotopic analyses of H. nipponica, a regionally abundant species recorded from the late Neogene to the present, can be valuable for paleoceanographic studies in shallow-marine environments.
Methods
Sediment was collected with a Smith–McIntyre grab sampler from three sites (sites 5, 25, and 26) on the continental shelf of Tosa Bay (Table 1; Figure 1) by T/S Toyohata-maru and T/S Neptune operated by Kochi University. Sediment samples for this study were collected from the uppermost centimeter of the sediment in the grab sampler. These were stored in a refrigerator and stained with Rose Bengal to identify living individuals of Hanzawaia nipponica Asano. After 2 days, the stained sediments were washed with water through a 150-µm mesh sieve and the fractions remaining on the sieve were dried at 50°C in an oven. Stained tests larger than 150 μm, representing mature living foraminifers, were then picked for isotope analysis (Figure 2). These tests (7–10 specimens) were baked at 450°C in an electric oven to remove organic matter from the tests (e.g. Erez and Luz, 1983). Fossil individuals of H. nipponica (50 specimens) were also picked for isotope analysis. All tests were cleaned with methanol by ultrasonication, slightly crushed in a glass vial and weighed, and then cleaned carbonate fragments weighing approximately 100 µg were reacted with 100% phosphoric acid at 90°C in a vacuum. The released CO2 was purified and analyzed for δ18O in carbonate (δ18Oc) and carbon isotopic composition (δ13C) using the isotope ratio mass spectrometer (IsoPrime, GV Instruments Ltd.), with its MultiPrep automated sample preparation module, at the Center for Advanced Marine Core Research, Kochi University. The results are expressed in relation to the Vienna Pee Dee Belemnite (VPDB) standard. The estimated analytical precisions were better than 0.1‰ for both δ18O and δ13C measurements.
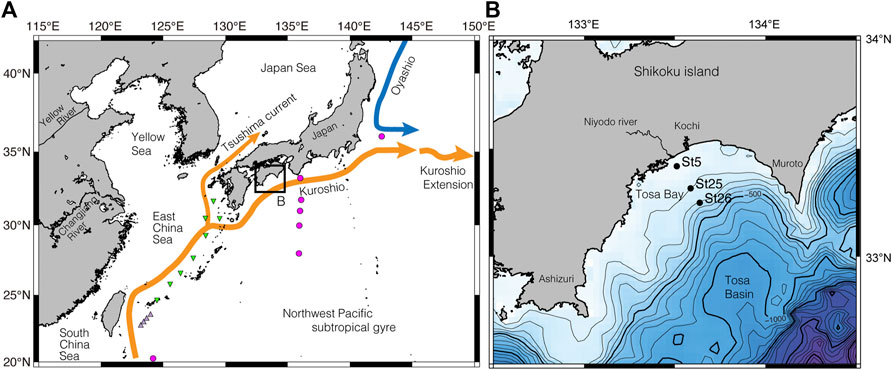
FIGURE 1. Site location maps. (A) Index map with major surface currents in the northwest Pacific and marginal seas. The red circles indicate the location of the isotope data in Horibe and Ogura (1968), and light purple triangles show the location of the isotope data at Kuroshio sites in Horikawa et al. (2015). Green triangles show same at Okinawa Trough sites in Horikawa et al. (2015). (B) Bathymetric map showing site locations of surface sediment and seawater samples on the continental shelf in Tosa Bay; map location shown in (A). Contour interval, 100 m.
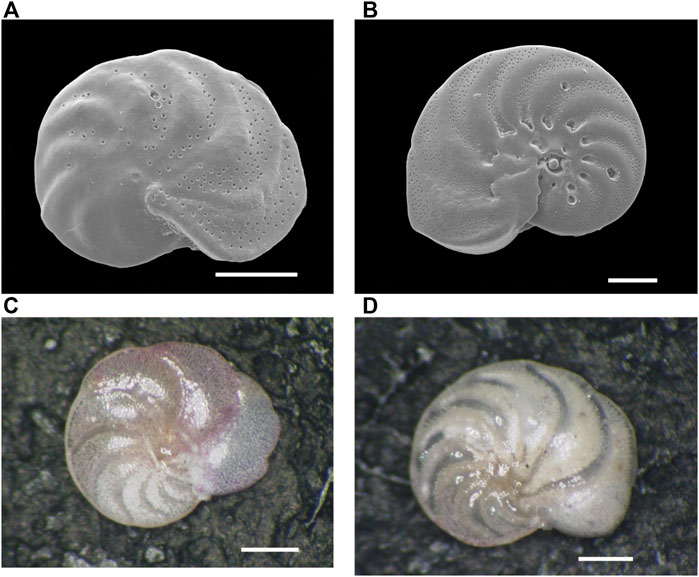
FIGURE 2. Scanning Electron Microscope (SEM) and stereo microscopic images of the benthic foraminifer Hanzawaia nipponica (Asano) from surface sediment in Tosa Bay. (A) SEM image of living (stained) H. nipponica from site 25, (B) SEM image of living H. nipponica from site 5, (C) photomicrograph of living H. nipponica from site 5, (D) photomicrograph of fossil (non-stained) H. nipponica from site 25. Scale bars are 100 µm in (A) and (B) and 200 µm in (C) and (D).
Seawater samples were collected at depths representing the entire water column (Table 2) at sites 5, 25, and 26 using a conductivity–temperature–depth (CTD)/rosette multi-sampler system with Niskin bottles on November 20, 2009 during a cruise by T/S Toyohata-maru. These samples were placed in 6-ml glass vials without bubbles and stored in a refrigerator until isotopic measurements were made. Subsamples measuring 200 µL were transferred into 10-ml vials containing CO2 gas (99.999%) and allowed to reach CO2–H2O exchange equilibrium at 25°C. The equilibrium CO2 gas was purified and analyzed for seawater δ18O (δ18Osw) using the IsoPrime system at Kochi University. The results are expressed in relation to Vienna Standard Mean Ocean Water (VSMOW). The estimated analytical precision was better than 0.1‰.
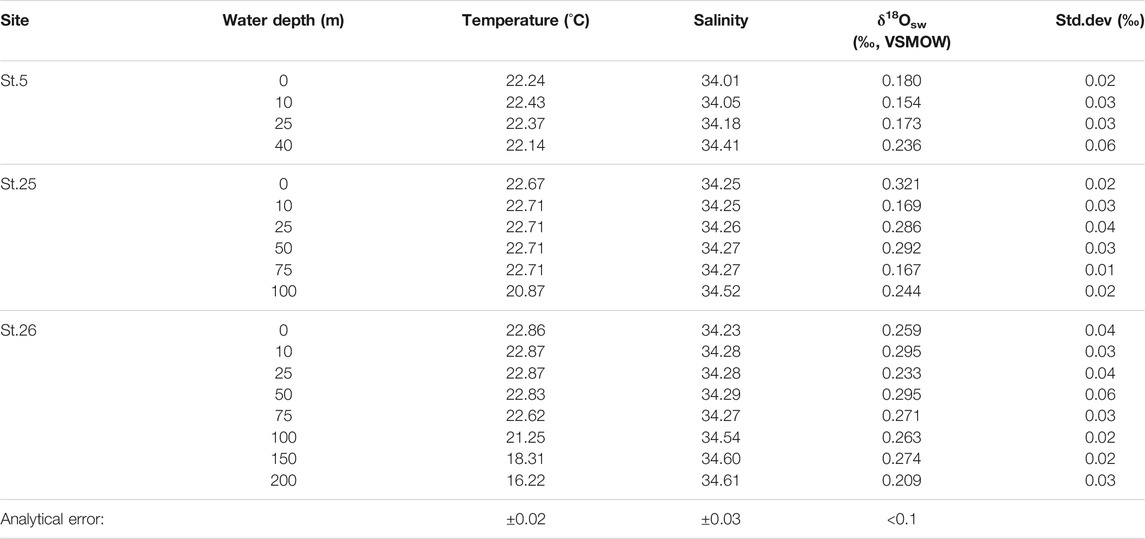
TABLE 2. Temperature, salinity, and oxygen isotopic composition in seawater (δ18Osw) from three sites in Tosa Bay (November 20, 2009).
Profiles of water temperature and salinity were measured at 0.5-m depth intervals from the surface to the seafloor during the same cruise using a Compact-CTD system (Alec Electronics Ltd.). CTD profiles at these three sites were also measured each month from April 2009 to February 2010 by the research group of Usa Marine Biological Institute, Kochi University (Table 3). In this study, the nominal depths of the seafloor were 40 m at site 5, 100 m at site 25, and 200 m at site 26, although actual depths varied slightly from month to month. Accuracy of temperature and salinity measurements are ±0.02°C and ±0.03, respectively.

TABLE 3. Seasonal maximum, minimum, and annual mean value of bottom water temperature and salinity from three sites in Tosa Bay.
Results and Discussion
Oceanic Structure and δ18Osw in Shallow Tosa Bay
Vertical profiles of monthly seawater temperature and salinity on the continental shelf in Tosa Bay show typical seasonal variations (Figure 3). Sea surface temperatures are about 28°C in summer and 17°C in winter at the three study sites (Figure 3). The surface water is thermally stratified during spring to summer, vertical mixing begins in October to November, and the temperature profile is almost uniform during January to March (Ichikawa and Hirota, 2004). The vertical temperature gradients on the day of sampling (November 20, 2009) were steeper than that of the annual mean temperature (Figure 3). The annual mean salinity in surface water was approximately 34.0 at site 5 and approximately 34.2 at sites 25 and 26 (Figure 3). Although the salinity of surface water in Tosa Bay decreased from July to September as a result of freshwater input from the Asian summer monsoon, the low-salinity layer was limited to the upper 80–90 m of the water column at sites 25 and 26. The sea surface salinity on the sampling day was slightly lower at site 5 (34.0) than at sites 25 (34.2) and 26 (34.2), but near the seafloor the salinity at site 5, the shallowest site (34.4), was similar to those at sites 25 (34.5) and 26 (34.6).
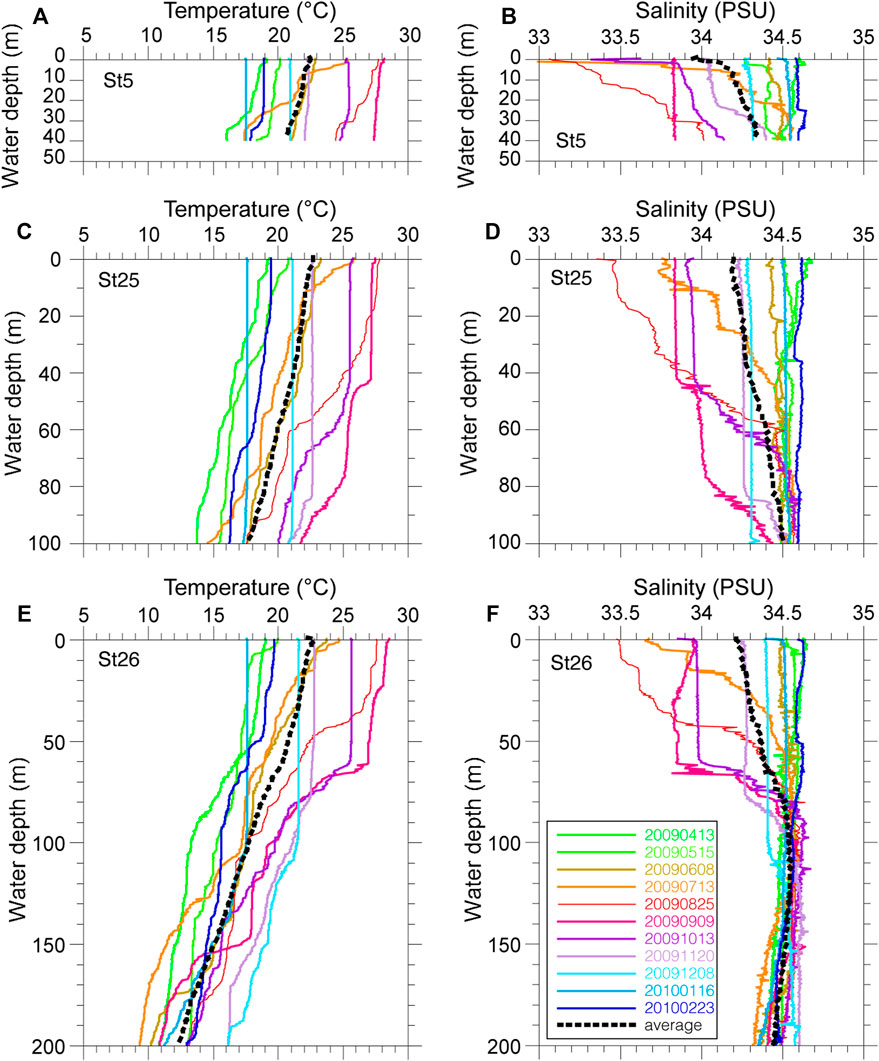
FIGURE 3. Measured temperature and salinity profiles at three sites in Tosa Bay. (A) temperature and (B) salinity at site 5, (C) temperature and (D) salinity at site 25, (E) temperature and (F) salinity at site 26. Thin colored lines are monthly values, heavy dashed lines are annual mean values (April 2009 to February 2010).
Although Tosa Bay is an open bay with a semicircular shelf–slope topography (Figure 1), the warm water of the Kuroshio does not flow directly through the bay. The most dominant current pattern is a counterclockwise circulation interacting with a cold eddy (Fujimoto, 1987; Kuroda et al., 2008). This circulation appears to be induced by the combined effect of changes in the position of the Kuroshio axis and the irregular topography of the continental margin (Awaji et al., 1991). The surface current, subsurface temperature, and vertical thermal structure on the continental slope in Tosa Bay are markedly influenced by the position of the Kuroshio axis, the counterclockwise circulation being enhanced as the Kuroshio axis moves near shore south of the Ashizuri and Muroto peninsulas (Kuroda et al., 2008). Therefore, the seafloor environment is affected by low-salinity coastal water only at depths shallower than 80–90 m in Tosa Bay, and the seafloor below 100 m is predominantly influenced by water originating from the Kuroshio.
On the date of sampling, the three sites in Tosa Bay had δ18Osw ranging from 0.15 to 0.32‰ (Table 2; Figure 4). Although not all of the Tosa Bay data lie on the regression lines of surface water from the East China Sea to the Kuroshio region (Oba, 1990; Horikawa et al., 2015) and their δ18Osw values appear relatively high (Figure 4), the δ18Osw–salinity data appear to be consistent with previously published results. These δ18Osw values and salinity values in Tosa Bay are very close to those of Kuroshio surface water from east of Taiwan (salinity = 34.2–34.4 and δ18Osw = 0.1–0.2‰) and Kuroshio subsurface water (40–100 m) in the Okinawa Trough (salinity = 34.4–34.7 and δ18Osw = 0.15–0.35‰) (Horikawa et al., 2015). The δ18Osw values in Tosa Bay are also consistent with those in surface and subsurface waters (0.25‰–0.4‰; 0–200 m) from the Kuroshio Extension and subtropical gyre (Oba and Yasuda, 1992). There is little available δ18Osw–salinity data of typical Kuroshio waters, but data from the Kuroshio region south of Japanese Islands show that Kuroshio water has higher salinity and δ18O there than in the localities just described (Horibe and Ogura, 1968) (Figure 4). The δ18O data from Horibe and Ogura (1968) were converted from δD data via the formula δ18O = δD/8.03. Slope 8.03 is derived from the relationship between δ18O and δD of observed global data (Mathieu et al., 2002).
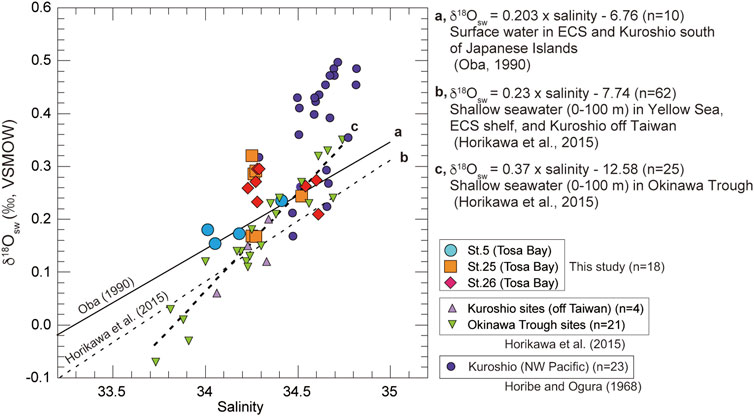
FIGURE 4. Plot of seawater δ18Osw against salinity (0–200 m) in Tosa Bay during November and published data from other northwest Pacific and East China Sea sites. Regression line (A) is derived from surface-water data in the East China Sea and the Kuroshio south of the Japanese Islands (Oba 1990). Regression line (B) is from shallow water (0–100 m) data in the Yellow Sea, East China Sea, and the Kuroshio off Taiwan (Horikawa et al., 2015). Regression line (C) is derived from shallow water (0–100 m) data in the Okinawa Trough (Horikawa et al., 2015). Equations from the literature are also shown. Equation of regression line c is calculated from data in the Okinawa Trough sites in Horikawa et al. (2015). End-member data for the Kuroshio are from northwest Pacific (Horibe and Ogura 1968).
The δ18Osw values averaged over the water column are 0.19‰ at site 5, 0.25‰ at site 25, and 0.26‰ at site 26. These results suggest that site 5 might be affected by freshwater with lower δ18O values from the Niyodo River. The δ18O values of surface water (river, stream, and spring water) in southern Shikoku are ranging from −5.9‰ to −7.9‰ (calculated average value −6.6‰) (Mizota and Kusakabe, 1994), indicating that the freshwater δ18O from Shikoku is almost consistent with the intercept of regression line a (Figure 4), which was derived from surface water in East China Sea and Kuroshio region in south of Japanese Islands (Oba, 1990). However, the discrepancy at site 5 is much smaller in water near the seafloor (Table 2), which suggests that freshwater influences only the surface mixed layer near the mouth of the Niyodo River. We lack data to assess the impact of seasonal changes, but because the seasonal salinity variation in bottom-water is small (Figure 3), we assume that the seasonal variation of δ18Osw in bottom water is negligible.
Oxygen Isotope Equilibrium of H. nipponica
The δ18Oc values of living and fossil H. nipponica increase with water depth in Tosa Bay, even though the vertical profile of δ18Osw is almost constant (Table 4; Figure 5). The differences in δ18Oc with depth may depend on water temperature, given that δ18Osw varies so little with depth (Figure 5A). However, δ18Oc in many foraminifer species differs from the equilibrium state (e.g., Shackleton, 1973; Bemis et al., 1998), and a specific offset value should be calculated to assess the temperature dependence of δ18Oc in H. nipponica. Although the biology of H. nipponica is not documented in detail, the life span of shallow-water benthic foraminifers is typically from several months to a year (Murray, 1991). For example, the life cycle of Planoglabratella opercularis, a benthic inhabitant of rocky shore environments, is completed in 40–65 days (Tsuchiya et al., 2014). We assumed that the life span of H. nipponica is more than 3 months, and on that basis we estimated the effect on calcification in the shallow ambient environment in Tosa Bay. Predicted δ18Oc values were calculated, assuming oxygen isotopic equilibrium in foraminiferal calcite, from

TABLE 4. Oxygen and carbon isotopic compositions of living (stained) and fossil (non-stained) H. nipponica from three sites in Tosa Bay.
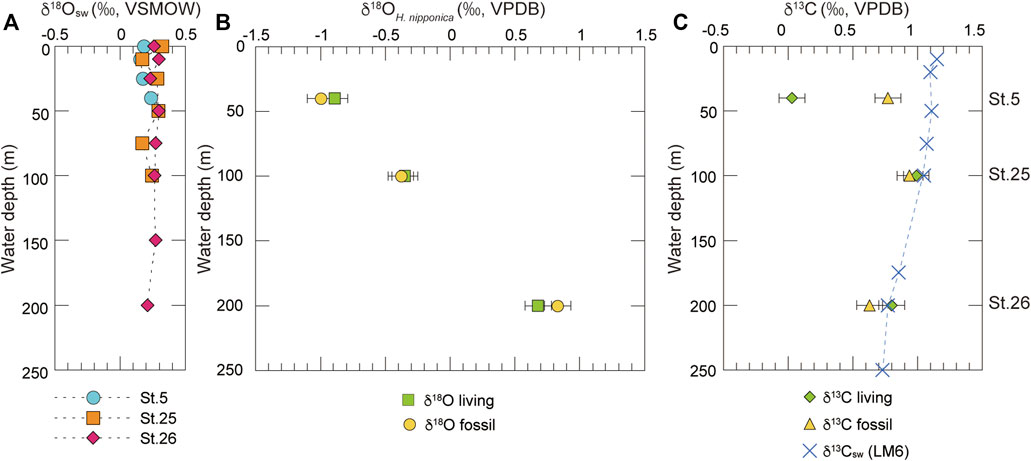
FIGURE 5. Oxygen and carbon isotopic compositions of seawater and H. nipponica at three sites in Tosa Bay. (A) Vertical profiles of oxygen isotopic compositions of seawater. (B) Oxygen and (C) carbon isotopic compositions of living and fossil H. nipponica specimens. Horizontal bars represent analytical error of 0.1‰. The δ13C values of seawater at station LM6 (34°67’N, 142°05’E) in the Kuroshio region off the Boso Peninsula (Oba et al., 2006) are also plotted in (C).
This equation was determined from culturing experiments with a symbiont-bearing planktic foraminifer, Orbulina universa, grown under low-light conditions (Bemis et al., 1998). The adjustment of −0.27‰ to the seawater term compensates for the δ18O difference between the VSMOW and PDB standards. We calculated δ18Oc from the δ18Osw values at each of our three study sites and various bottom-water temperatures for each site: monthly values, the annual mean value, and average values for the 3-month period September to November, based on measurements from April 2009 to February 2010.
The results (Figure 6) show that the δ18Oc values of living H. nipponica lie well within the seasonal variation of predicted δ18Oc at every site and are nearly equal to the predicted δ18Oc values based on the annual mean bottom temperature. They also suggest that the δ18Oc values of living H. nipponica are heavier than the predicted δ18Oc values from the 3-months average. Because we assumed a life span of 3 months for this species, the discrepancy indicates that H. nipponica has a much longer life cycle. This finding is also supported by the fact that δ18Oc values of living and fossil H. nipponica are very close to each other (Figure 5B). From these results, we conclude that the carbonate tests of H. nipponica are in oxygen isotopic equilibrium with the ambient seawater. Although there are only three sites in this study, it is possible to evaluate the relationship between δ18Oc of benthic foraminifer and bottom water temperature. Figure 7 shows the relationship δ18Oc of living and fossil H. nipponica and annual mean bottom water temperature in Tosa Bay. It is clear that the relationship is linear in both cases. The slope of the linear function is −5.26 (0.19‰°C−1) for the living and −4.50 (0.22‰°C−1) for the fossil H. nipponica, respectively (Figure 7).
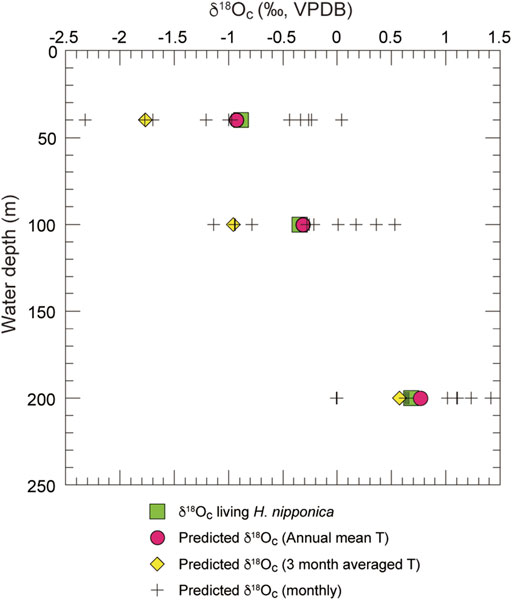
FIGURE 6. Predicted and measured δ18Oc of living H. nipponica plotted against water depth. Plus symbols show the monthly predicted δ18Oc values at sites 5 (40 m), 25 (100 m), and 26 (200 m). Red filled circles and yellow filled diamond symbols show the predicted δ18Oc values for annual mean temperatures and 3-months average temperature (September to November), respectively. The predicted δ18Oc values are calculated from water temperature and δ18Osw in Tosa Bay using a calcite equilibrium equation (Bemis et al., 1998).
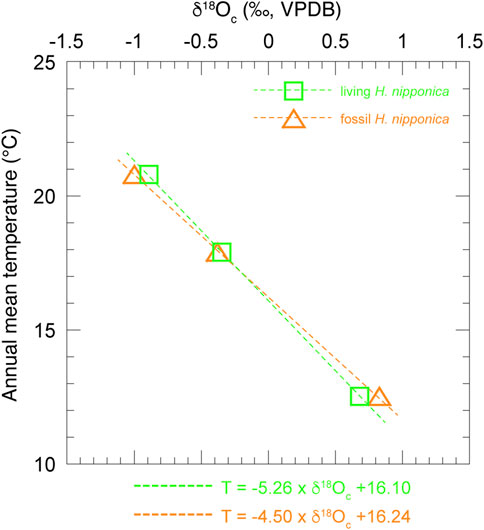
FIGURE 7. Relationship between δ18Oc of living and fossil H. nipponica and annual mean bottom water temperature at three sites in Tosa Bay. Green squares show data from living H. nipponica and orange triangles indicate data from fossil H. nipponica.
Although we applied the δ18Oc–temperature equation of Bemis et al. (1998) to our data, there are other δ18Oc–temperature relationships that yield various estimates for calcification of benthic foraminifers. For instance, Shackleton (1974) used core-top data to calibrate a δ18Oc–temperature relationship for the benthic foraminifer Uvigerina spp., and Zahn and Mix (1991) proposed that δ18Oc in Uvigerina peregrina at water depths greater than 2 km could be described best by the equation of Erez and Luz (1983), derived from culture experiments with the planktic foraminifer Globigerinoides sacculifer. Both of these studies assumed that Uvigerina precipitates its test in oxygen isotope equilibrium with ambient seawater. Bemis et al. (1998) compared Eq. 1 to an analysis of a compilation of published δ18O data for the benthic foraminifers Uvigerina and Cibicidoides from core tops in the Atlantic, Pacific and Indian oceans, the Arabian Sea, and the Gulf of Mexico. They found that the δ18O data for Cibicidoides were in excellent agreement with Eq. 1, which was based on culture experiments in low-light conditions with the planktic foraminifer Orbulina universa. Our data for H. nipponica show that the relationship between δ18Oc and ambient temperature closely fits Eq. 1 (Figure 8). The δ18O data also show a similar trend with temperature in equations based on benthic foraminifers (Shackleton, 1974) and inorganic calcite (Kim and O’Neil, 1997). We conclude that the shallow-water benthic foraminifer H. nipponica precipitates its test close to oxygen isotopic equilibrium with seawater, as does the deep-sea benthic foraminifer Cibicidoides. The equation of Bemis et al. (1998) appears to be widely applicable to foraminifers, including benthic foraminifers in shallow and deep-sea environments. Although H. nipponica is restricted to continental shelves and upper slopes, this species may be very useful for elucidating paleoenvironmental changes in shallow-water settings affected by the Kuroshio and Tsushima current in the northwest Pacific and adjoining seas. Planktic foraminifer, which have been used in many isotope studies, have relatively large depth habitat (e.g. Schiebel and Hemleben, 2017), in contrast, benthic foraminifer have the advantage of being able to extract information on limited water depths, thus our new result is very important to advance paleoceanographic research in shallow water of past ocean.
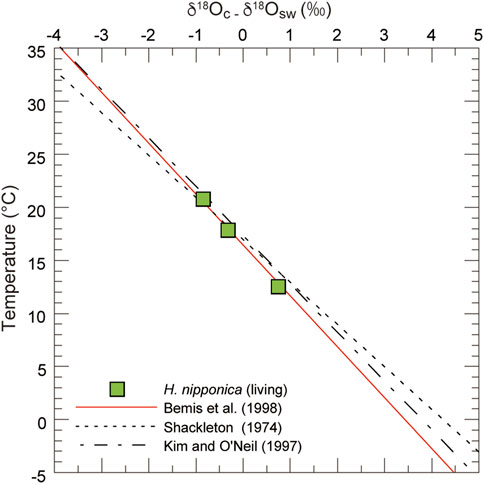
FIGURE 8. Predicted δ18O values for living H. nipponica from surface sediments on the continental shelf in Tosa Bay. Published relations between temperature and δ18O are also plotted (Shackleton 1974; Kim and O’Neil 1997; Bemis et al., 1998). The H. nipponica data show a good fit to the equation of Bemis et al. (1998).
Carbon Isotopes of H. nipponica
Our determinations showed that δ13C of living H. nipponica ranged from 0.03 to 0.99‰, and those of fossil H. nipponica ranged from 0.63 to 0.94‰ (Table 4; Figure 5C). The differences between living and fossil specimens were small at site 25 (0.05‰) and site 26 (0.17‰) and much greater (0.74‰) at site 5, the shallowest site, where living specimens exhibited relatively depleted values (Figure 5C). Although δ13C values of seawater in Tosa Bay have not been reported, values in the Kuroshio off the Boso Peninsula have been reported as a gradation from ∼1.2‰ in surface water to ∼0.8‰ in subsurface water at ∼200 m depth (Figure 5C; Oba et al., 2006). Similarly, Kroopnick (1985) reported δ13C values of 1.2‰ in surface water in the Kuroshio region off central Japan (station 224; 34°25′N, 142°00′E). Our results show that the δ13C of H. nipponica is very close to these values in bottom water at site 25 (100 m) and site 26 (200 m) (Figure 5C), suggesting that δ13C in H. nipponica reflects the δ13C of ambient seawater. The depleted δ13C values of H. nipponica at site 5 suggest that the δ13C of benthic foraminifers in the surface mixed layer are greatly influenced by factors such as photosynthetic processes, local river input, and contributions of depleted δ13C from terrestrial organic materials.
In general, the distribution of δ13C in the ocean is controlled by the interaction of biological uptake at the sea surface, air–sea gas exchange, and decomposition in deeper water masses. Photosynthesis in shallow waters preferentially extracts 12C from the carbon-bearing ions in seawater, enriching the ΣCO2 of surface water in 13C. The δ13C value in seawater after primary producers have removed all nutrients, then, is controlled by the mean δ13C and the mean nutrient concentration of the ocean (Broecker, 1982). Because the carbon taken up by phytoplankton has a δ13C value of approximately −20‰, the surface reservoir is enriched by 2.0‰ relative to the mean δ13C of deep water (Curry et al., 1988). Therefore, δ13C data of fossil benthic foraminifers can be used to reconstruct past properties of deep water and infer past changes in ocean ventilation (e.g., Curry and Oppo, 2005; Ullerman et al., 2016).
Although further research will be necessary to better specify the dominant factors of δ13C in H. nipponica, the δ13C of this species is nearly identical to that of seawater. This similarity means that δ13C of H. nipponica can be used to reconstruct the history of carbon circulation in subsurface to intermediate waters in the northwest Pacific and its marginal seas.
Conclusion
The main findings of this study are as follows:
1) The oxygen isotopic composition (δ18Osw) and salinity of seawater samples from Tosa Bay generally overlap with those of the Kuroshio, which flows from the Okinawa Trough past Tosa Bay to the northwest Pacific. Although the surface and subsurface waters at site 5, the shallowest site, are influenced by freshwater input, the bottom water at all sites (40–200 m) is minimally influenced by less saline coastal water.
2) The δ18Oc values increased with water depth in living and fossil specimens of the benthic foraminifer Hanzawaia nipponica, whereas δ18Osw did not vary with water depth in Tosa Bay. The δ18Oc values of living H. nipponica were predicted well by the calcite equilibrium equation of Bemis et al. (1998) using the annual mean bottom-water temperature, indicating that the carbonate tests of H. nipponica form in oxygen isotopic equilibrium with ambient seawater. This result reinforces the utility of the equation of Bemis et al. (1998) for calculating δ18Osw and temperature for foraminifers, including benthic foraminifers in shallow and deep marine environments.
3) Tests of H. nipponica faithfully record the δ13C of ambient seawater, although the δ13C of this species in the surface mixed layer is difficult to interpret owing to the influence of various factors.
4) Data on δ18Oc and δ13C of H. nipponica are useful proxies to reconstruct paleoenvironmental changes in the shallow waters of the northwest Pacific and its marginal seas.
Data Availability Statement
The original contributions presented in the study are included in the article/Supplementary Material, further inquiries can be directed to the corresponding author.
Author Contributions
MI proposed the topic and conceived and designed the study. SKi carried out the sampling and the experimental study. SKa collaborated with the corresponding author in the identification of benthic foraminifers. MI and SKi analyzed the data and helped in their interpretation. All authors read and approved the final manuscript.
Funding
This work was supported by a research project grant of Kochi University.
Conflict of Interest
The authors declare that the research was conducted in the absence of any commercial or financial relationships that could be construed as a potential conflict of interest.
Publisher’s Note
All claims expressed in this article are solely those of the authors and do not necessarily represent those of their affiliated organizations, or those of the publisher, the editors and the reviewers. Any product that may be evaluated in this article, or claim that may be made by its manufacturer, is not guaranteed or endorsed by the publisher.
Acknowledgments
We thank Professor Emeritus Hiroshi Ueda, Makoto Yano, and Zenji Imoto (Usa Marine Biological Institute, Kochi University) and Daiki Kawata (Kochi University) for support during seawater and sediment sampling in Tosa Bay. We also acknowledge Michiyo Kobayashi and Sayaka Sakaguchi (Center for Advanced Marine Core Research, Kochi University) for analytical support. Discussions with Hitomi Wakaki-Uchimura (Kochi University), Hidetaka Nomaki (JAMSTEC), and Masashi Tsuchiya (JAMSTEC) on benthic foraminiferal habitats were helpful in improving the manuscript. We are grateful to Professor Emeritus Tadamichi Oba and one reviewer for their constructive comments.
References
Akimoto, K. (1990). Distribution of Recent Benthic Foraminiferal Faunas in the Pacific off Southwest Japan and Around Hachijojima Island. Sci. Rep. Tohoku Univ. Sendai, 2nd Ser. (Geology) 60 (2). 139–223.
Akimoto, K., and Torii, M. (1996). Distribution of Recent Benthic Foraminiferal Assemblages in the Surface Sediments of the Hyuganada Area, off Kyushu and Shikoku, Japan. Bull. Geol. Surv. Jpn. 47, 41–59.
Aoshima, M. (1978). Depositional Environment of the Plio-Pleistocene Kakegawa Group, Japan —A Comparative Study of the Fossil and the Recent Foraminifera—. J. Fac. Sci. Univ. Tokyo, Section Geology. mineralogy, Geogr. Geophys. 19 (5). 401–441.
Asano, K. (1944). Hanzawaia, a New Genus of Foraminifera, from the Pliocene of Japan. Jour. Geol. Soc. Jpn. 51, 97–99. doi:10.5575/geosoc.51.97
Awaji, T., Akitomo, K., and Imasato, N. (1991). Numerical Study of Shelf Water Motion Driven by the Kuroshio: Barotropic Model. J. Phys. Oceanogr. 21, 11–27. doi:10.1175/1520-0485(1991)021<0011:nsoswm>2.0.co;2
Bemis, B. E., Spero, H. J., Bijma, J., and Lea, D. W. (1998). Reevaluation of the Oxygen Isotopic Composition of Planktonic Foraminifera: Experimental Results and Revised Paleotemperature Equations. Paleoceanography 13, 150–160. doi:10.1029/98pa00070
Broecker, W. S. (1982). Glacial to Interglacial Changes in Ocean Chemistry. Prog. Oceanography 11, 151–197. doi:10.1016/0079-6611(82)90007-6
Curry, W. B., Duplessy, J. C., Labeyrie, L. D., and Shackleton, N. J. (1988). Changes in the Distribution of δ13C of Deep Water ΣCO2 between the Last Glaciation and the Holocene. Paleoceanography 3, 317–341. doi:10.1029/pa003i003p00317
Curry, W. B., and Oppo, D. W. (2005). Glacial Water Mass Geometry and the Distribution of δ13C of ΣCO2 in the Western Atlantic Ocean. Paleoceanography 20. doi:10.1029/2004PA001021
Erez, J., and Luz, B. (1983). Experimental Paleotemperature Equation for Planktonic Foraminifera. Geochimica et Cosmochimica Acta 47, 1025–1031. doi:10.1016/0016-7037(83)90232-6
Fujimoto, M. (1987). On the Flow Types and Current Stability in Tosa Bay and Adjacent Seas. Umi to Sora 62, 15–27. (in Japanese with English abstract).
Hasegawa, S. (1993). Distribution of Recent Benthic Foraminifers as an Indicator of thermal Conditions of the Seas Around the Japanese Islands - an Approach to Reconstruction of Cenozoic Oceanic Condition. Fossils 55, 17–33. (in Japanese with English abstract).
Horibe, Y., and Ogura, N. (1968). Deuterium Content as a Parameter of Water Mass in the Ocean. J. Geophys. Res. 73, 1239–1249. doi:10.1029/jb073i004p01239
Horikawa, K., Kodaira, T., Zhang, J., and Murayama, M. (2015). δ18Osw Estimate for Globigerinoides ruber from Core-Top Sediments in the East China Sea. Prog. Earth Planet. Sci. 2, 19. doi:10.1186/s40645-015-0048-3
Ichikawa, T., and Hirota, Y. (2004). Seasonal Changes of Primary Productivity in Tosa Bay, Japan. Oceanography Jpn. 13, 259–269. (in Japanese with English abstract). doi:10.5928/kaiyou.13.259
Inoue, Y. (1989). Northwest Pacific Foraminifera as Paleoenvironmental Indicators, Sci. Rep. Inst. Geosci. Univ. Tsukuba, Section B 10, 57–162.
Ishiwada, Y. (1964). Benthonic Foraminifera off the Pacific Coast of Japan Referred to Biostratigraphy of the Kazusa Group. Rep. Geol. Surv. Jpn. 205, 1–45.
Kang, S., Lim, D., and Kim, S.-Y. (2010). Benthic Foraminiferal Assemblage of Seogwipo Formation in Jeju Island, South Sea of Korea: Implication for Late Pliocene to Early Pleistocene Cold Episode in the Northwestern Pacific Margin. Quat. Int. 225, 138–146. doi:10.1016/j.quaint.2010.04.009
Katto, J., Nakamura, J., and Takayanagi, Y. (1953). Stratigraphical and Paleontological Studies of the Tonohama Group, Kochi Prefecture, Japan, Res. Rep. Kochi Univ. 2 (32). 1–15.
Kim, S.-T., and O'Neil, J. R. (1997). Equilibrium and Nonequilibrium Oxygen Isotope Effects in Synthetic Carbonates. Geochimica et Cosmochimica Acta 61, 3461–3475. doi:10.1016/s0016-7037(97)00169-5
Kroopnick, P. M. (1985). The Distribution of 13C of ΣCO2 in the World Oceans. Deep Sea Res. A. Oceanographic Res. Pap. 32, 57–84. doi:10.1016/0198-0149(85)90017-2
Kuroda, H., Shimizu, M., Hirota, Y., Ambe, D., and Akiyama, H. (2008). Surface Current and Vertical thermal Structure on the continental Slope in Tosa Bay. J. Oceanogr 64, 81–91. doi:10.1007/s10872-008-0006-4
Kuwano, Y. (1963). Foraminiferal Biocoenosis of the Seas Around Japan: A Survey of Pacific Side Biocoenoses, III. Biocoenoses off Boso Peninsula. Miscellaneous Rep. Res. Inst. Nat. Resour. 60, 29–51.
LeRoy, L. W. (1964). Smaller Foraminifera from the Late Tertiary of Southern Okinawa. Prof. Pap. U.S. Geol. Surv. 454-F, 1–58. doi:10.3133/pp454f
Li, B., Park, B.-K., Kim, D., and Woo, H. J. (1999). The Geological Age and Paleoenvironment of the Lower Seogwipo Formation, Cheju Island, Korea. Geosci. J. 3, 181–190. doi:10.1007/bf02910489
Mathieu, R., Pollard, D., Cole, J. E., White, J. W. C., Webb, R. S., and Thompson, S. L. (2002). Simulation of Stable Water Isotope Variations by the GENESIS GCM for Modern Conditions. J. Geophys. Res. 107. doi:10.1029/2001JD900255
Matoba, Y. (1976). “Foraminifera from off Noshiro, Japan, and Postmortem Destruction of Tests in the Japan Sea,” in Progress on Micropaleontology. Editors Y. Takayanagi, and T. Saito (New York: Micropaleontology Press), 169–189.
Matoba, Y., and Honma, N. (1986). “Depth Distribution of Recent Benthic Foraminifera off Nishitsugaru, Eastern Sea of Japan,” in Studies on Cenozoic Benthic Foraminifera in Japan. Editors Y. Matoba, and M. Kato (Mining College, Akita University), 53–78. (in Japanese with English abstract).
Mizota, C., and Kusakabe, M. (1994). Spatial Distribution of δD-δ18O Values of Surface and Shallow Groundwaters from Japan, south Korea and east China. Geochem. J. 28, 387–410. doi:10.2343/geochemj.28.387
Murray, J. W. (1991). “Ecology and Distribution of Benthic Foraminifera,” in Biology of Foraminifera. Editors J. J. Lee, and O. R. Anderson (Academic Press), 285–334.
Oba, T., Irino, T., Yamamoto, M., Murayama, M., Takamura, A., and Aoki, K. (2006). Paleoceanographic Change off central Japan since the Last 144,000 Years Based on High-Resolution Oxygen and Carbon Isotope Records. Glob. Planet. Change 53, 5–20. doi:10.1016/j.gloplacha.2006.05.002
Oba, T. (1990). “Paleoceanographic Information Obtained by Isotopic Measurement of Individual Foraminiferal Specimens,” in Proceedings of the First International Conference on Asian Marine Geology. Editors P. Wang, Q. Lao, and Q. He (Beijing: China Ocean Press), 169–180.
Oba, T., and Yasuda, H. (1992). Paleoenvironmental Change of the Kuroshio Region since the Last Glacial Age. Daiyonki-kenkyu 31, 329–339. (in Japanese with English abstract). doi:10.4116/jaqua.31.329
Schiebel, R., and Hemleben, C. (2017). Planktic Foraminifers in the Modern Ocean. Berlin: Springer-Verlag.
Shackleton, N. J. (1974). Attainment of Isotopic Equilibrium between Ocean Water and the Benthonic Foraminifer Genus Uvigerina: Isotopic Changes in the Ocean during the Last Glacial. C.N.R.S. Colloquium 219, 203–209.
Shackleton, N. J., and Opdyke, N. D. (1973). Oxygen Isotope and Palaeomagnetic Stratigraphy of Equatorial Pacific Core V28-238: Oxygen Isotope Temperatures and Ice Volumes on a 105 Year and 106 Year Scale. Quat. Res. 3, 39–55. doi:10.1016/0033-5894(73)90052-5
Tsuchiya, M., Takahara, K., Aizawa, M., Suzuki-Kanesaki, H., Toyofuku, T., and Kitazato, H. (2014). “How Has Foraminiferal Genetic Diversity Developed? A Case Study of Planoglabratella Opercularis and the Species Concept Inferred from its Ecology, Distribution, Genetics, and Breeding Behavior,” in Approaches to Study Living Foraminifera. Environmental Science and Engineering. Editors H. Kitazato, and J. M. Bernhard (Tokyo: Springer), 133–162. doi:10.1007/978-4-431-54388-6_9
Uchio, T. (1968). Foraminiferal Assemblages in the Vicinity of the Seto Marine Biological Laboratory, Shirahama-Cho, Wakayama-Ken, Japan (PART 1). Publications Seto Mar. Biol. Lab. 15 (5), 399–417. doi:10.5134/175480
Ullermann, J., Lamy, F., Ninnemann, U., Lembke‐Jene, L., Gersonde, R., and Tiedemann, R. (2016). Pacific‐Atlantic Circumpolar Deep Water Coupling during the Last 500 Ka. Paleoceanography 31, 639–650. doi:10.1002/2016PA002932
Xiang, R., Yang, Z., Saito, Y., Fan, D., Chen, M., and Guo, Z. (2008). Paleoenvironmental Changes during the Last 8400 Years in the Southern Yellow Sea: Benthic Foraminiferal and Stable Isotopic Evidence. Mar. Micropaleontology 67, 104–119. doi:10.1016/j.marmicro.2007.11.002
Keywords: benthic foraminifer, Hanzawaia nipponica Asano, oxygen isotopic equilibrium, Tosa Bay, continental shelf, Kuroshio
Citation: Ikehara M, Kita S and Kawagata S (2021) Oxygen Isotope Equilibrium of the Shallow-Water Benthic Foraminifer Hanzawaia nipponica Asano in Tosa Bay, Southwest Japan. Front. Earth Sci. 9:708578. doi: 10.3389/feart.2021.708578
Received: 12 May 2021; Accepted: 02 September 2021;
Published: 20 September 2021.
Edited by:
Min-Te Chen, National Taiwan Ocean University, TaiwanReviewed by:
Tadamichi Oba, Hokkaido University, JapanHong-Chun Li, National Taiwan University, Taiwan
Copyright © 2021 Ikehara, Kita and Kawagata. This is an open-access article distributed under the terms of the Creative Commons Attribution License (CC BY). The use, distribution or reproduction in other forums is permitted, provided the original author(s) and the copyright owner(s) are credited and that the original publication in this journal is cited, in accordance with accepted academic practice. No use, distribution or reproduction is permitted which does not comply with these terms.
*Correspondence: Minoru Ikehara, ikehara@kochi-u.ac.jp