- 1Yunnan Key Laboratory of Earth System Science, Yunnan University, Kunming, China
- 2Key Laboratory of Cenozoic Geology and Environment, Institute of Geology and Geophysics, Chinese Academy of Sciences, Beijing, China
- 3School of Geosciences and Resources, China University of Geosciences, Beijing, China
- 4School of Earth and Environmental Sciences, The University of Queensland, Brisbane, QLD, Australia
Although diatom records from lake sediments have been used for quantitative paleoclimatic reconstruction, their validity and sensitivity have rarely been tested rigorously. At Yunlong Lake, an alpine lake in Southwest China, we studied the seasonal succession of diatom assemblages to produce a mean surface water temperature (MSWT) transfer function. In addition, based on the spatial distribution of surface diatom assemblages with water depth, we produced a diatom-water depth (WD) transfer function. Combined with the analysis of diatom assemblages in a sediment core (YL2013-A), changes in surface water temperature and water level over the last ∼100 years were quantitatively reconstructed using the diatom-based transfer functions. Comparison with records of regional meteorology and reservoir water capacity revealed that the diatom-based lake water level reconstruction is a sensitive indicator of short-term fluctuations in precipitation, and it also reflects a long-term stepwise rise in water level caused by the impounding and large-scale extension of the reservoir. In addition, the diatom-inferred MSWT is consistent with the changes in air temperature prior to large-scale human disturbance of the site. However, after the extension of the reservoir, although the regional air temperature continued to increase, the water temperature decreased substantially. This suggests that the large increase in lake water volume in the short term led to a decrease in the average water temperature, which in turn led to the occurrence of a diatom bloom in the cold season. The results demonstrate that diatom transfer functions based on modern observations of the same lake has a high environmental sensitivity and can be used for the quantitative reconstruction of regional climate change. Overall, our findings provide a foundation for the use of lake diatom records for quantitative paleoclimatic reconstruction on various timescales.
Introduction
Diatom records from lake sediments are important in paleoclimatic reconstruction, for the following three major reasons. First, diatoms are widely distributed in lakes, and they have a high diversity and can be clearly classified (Battarbee et al., 2001). Second, diatoms have a relatively short reproductive cycle and are very sensitive to climate changes (temperature, precipitation, snowfall, wind speed, etc.) (Smol and Douglas, 2007; Wang et al., 2011; Wang et al., 2012; Wang et al., 2018; Rühland et al., 2015). Third, the siliceous valves of diatoms are well preserved in lake sediments, and compared with other algal indicators this makes them uniquely advantageous for paleoclimatic reconstruction on various timescales (Smol and Cumming, 2000; Dong et al., 2016).
Different diatom species in lakes respond differently to climate changes, such as temperature and precipitation. Diatoms in lakes, especially planktonic diatoms, are very sensitive to temperature changes (Smol and Douglas, 2007; Rühland et al., 2015), including direct and indirect responses (Zou et al., 2018). For example, recent global warming has led to the predominance of the warm-preference planktonic diatom Aulacoseira granulata in Douhu Lake in China (Li et al., 2021), and this preference was also evidenced by an investigation on the seasonal diatom succession of Yunlong Lake in China (Zou et al., 2018), which indicated a direct response of diatoms to temperature. Meanwhile, global warming has greatly reduced the length of the ice-cover period of high-latitude lakes, and intensified the thermal stratification of temperate lakes, which has led to blooming of the cyclotelloid diatom taxa (Wang et al., 2012; Rühland et al., 2015), this is an indirect climatic response.
The spatial distribution of planktonic and benthic diatoms within a lake basin, especially of benthic diatoms, is controlled mainly by light penetration and is closely linked to water depth. Therefore, different benthic diatom assemblages and the ratio of planktonic to benthic diatoms are sensitive indicators of past lake water level (Stone and Fritz, 2004; Laird et al., 2010; Laird et al., 2011; Kingsbury et al., 2012). For lakes supplied mainly by atmospheric precipitation, water level is a valuable index of changes in precipitation within the lake catchment (Yang et al., 2003; Magny, 2004), enabling lake diatom assemblages to be used to reconstruct past changes in precipitation.
A major prerequisite of climatic reconstruction using diatoms is a clear understanding of their ecological preferences (Zou et al., 2018). However, because diatom species are numerous, in many cases we still lack a comprehensive understanding of their ecological preferences; moreover, due to site-specific factors, diatom assemblages can vary substantially between lakes. Therefore, arguably the best way of interpreting paleolimnological diatom records is to investigate modern diatom assemblages in specific lakes in order to determine their ecological response to environmental factors.
Determining the quantitative relationship between diatom assemblages and the environmental factors of temperature and water level requires two steps. First, investigating the seasonal succession of modern lake diatom species is an effective means of understanding their response to temperature changes. Seasonality is a major characteristic of diatoms in freshwater ecosystems (Kӧster and Pienitz, 2006; Winder and Cloern, 2010; Zou et al., 2018). Pronounced changes in physical and chemical conditions within a lake can occur on a seasonal basis, resulting in major changes in diatom assemblages (Winder and Cloern, 2010). Therefore, the seasonal succession of diatom assemblages in lakes is often a rich source of information on the response of diatoms to environmental changes. Additionally, large environmental gradients typically accompany seasonal changes, such as in the temperature contrast between summer and winter, which is important for diatom-based temperature reconstruction on various timescales (Zou et al., 2018).
Second, investigating the spatial pattern of diatom assemblages in surface lake sediments can be used to obtain a quantitative relationship between diatom assemblages and water depth, which can then be used to reconstruct past changes in lake water level (Stone and Fritz, 2004; Laird et al., 2010; Laird et al., 2011). This is because diatoms are photosynthetic algae, which requite light for growth, and different diatom species have different requirements for light conditions. Since lakes are seldom entirely translucent, benthic diatoms can often only grow within the shallow-water photic zone, while planktonic diatoms can remain suspended in the upper photic zone of the entire lake. Therefore, the relative abundance of different benthic diatom species, as well as the ratio between planktonic and benthic diatoms, is significantly correlated with water depth (Kingsbury et al., 2012; Zou et al., 2020).
Transfer functions are widely used for diatom-based quantitative paleoclimatic reconstruction. The methodology can be summarized as follows: A dataset of modern diatom samples and environmental variables is collected, which is used to establish a quantitative relationship between diatom assemblages and the main controlling environmental variables (e.g., water temperature and water depth). This enables a transfer function to be constructed which can then applied to fossil diatom assemblages to quantitatively reconstruct the corresponding climatic variable(s) (Lotter and Bigler, 2000; Yang et al., 2003; Laird et al., 2010; Laird et al., 2011). Many advanced data processing methods are available for constructing transfer functions, such as weighted average partial least squares regression (WAPLS) (Birks, 2012), which is statistically robust and simple to calculate, and hence is widely used. Several paleoclimatic reconstructions have been produced using diatom transfer functions (Lotter and Bigler, 2000; Kӧster and Pienitz, 2006); however, the sensitivity and validity of these reconstruction need to be further examined (Juggins, 2013), based for example on comparisons with meteorological observations.
Yunlong Lake, a subtropical alpine lake in Yunnan Province of China which is very sensitive to the regional climate change (Wang et al., 2018; Zou et al., 2018; Zou et al., 2020; Jiang et al., 2019) was selected to produce diatom-based environmental transfer functions. Through a two-year investigation of the modern diatom seasonal succession at the lake (Zou et al., 2018), a diatom-water temperature transfer function was constructed. By investigating spatial pattern of surface diatom assemblages with water depth (Zou et al., 2020), a diatom-water depth transfer function was also constructed. Combined with the analysis of diatom assemblage changes in a sediment core over the last ∼100 years, the two diatom transfer functions were used to quantitatively reconstruct changes in mean surface water temperature and water level at the site. Finally, the sensitivity and validity of the diatom-inferred climate reconstruction were examined by comparing the results with meteorological and reservoir capacity records over the last 60 years.
Overview of the Study Site
Yunlong Lake is located in the Tianchi National Nature Reserve in Yunnan Province, Southwest China, in the core area of the longitudinal ridge valley of the Hengduan Mountains (Figure 1A). The climate of the region is influenced mainly by the Indian Summer Monsoon, and there is a very strong positive relationship between temperature and rainfall (Zou et al., 2018). According to meteorological records for Yunlong Station (25°32′24″ N, 99°13′12″ E; 1,659 m.a.s.l.) for 1981–2010, the average annual air temperature was 16.2°C, and the average monthly air temperature was generally high from april to October (>15°C). The average annual rainfall was 780.5 mm and the rainy season was from May to October (Zou et al., 2018).
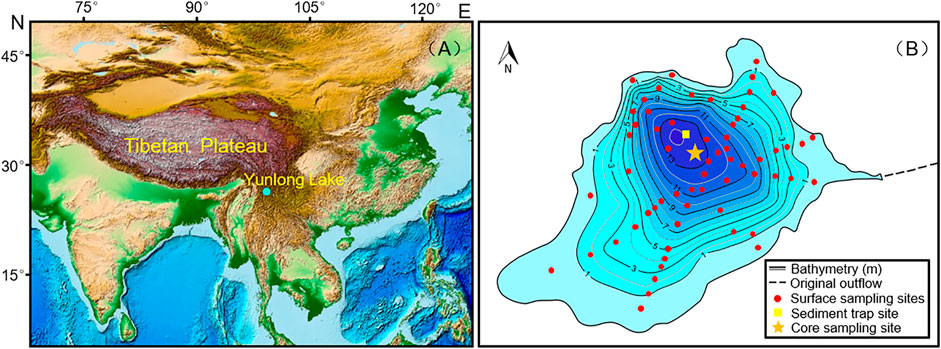
FIGURE 1. Location of Yunlong Lake and sampling sites. (A) Schematic diagram of the geographical location of Yunlong Lake. (B) Bathymetry of Yunlong Lake and the locations of the surface samples, sediment core, and sediment trap.
Yunlong Lake (25°52′12.89″ N, 99°16′49.75″ E, Figure 1B) is at an altitude of 2,551 m.a.s.l.; it has a surface area of ∼1.5 km2, a watershed area of ∼11.25 km2, and a maximum water depth of ∼14.1 m. Yunlong Lake is a typical tectonic faulted lake with no natural inflows, and the lake water is charged mainly by atmospheric precipitation (Zou et al., 2018; Zou et al., 2020). In the past, there was a natural outflow in the east (Figure 1B), which flowed into a branch of the Lantsang River. Prior to the 1950s, the lake was largely unaffected by human activity due to its remoteness and relatively high altitude. However, to alleviate the local scarcity of water, from the 1950s onwards, it was gradually transformed into an artificial reservoir. A dam with a total height of ∼12.74 m was constructed between 1953 and 1985 on the eastern outflow. Later, in October 2003, in response to increasing demand for water for domestic consumption and irrigation, a water input project to increase the reservoir capacity was initiated and completed in July 2009. Subsequently, the lake was enlarged to form a medium-sized reservoir (Zou et al., 2020).
Materials and Methods
Construction of Diatom Transfer Functions
We chose the WAPLS method (Component 1 and Component 2) to construct diatom transfer functions (Birks, 2012). The diatom percentage data were square root transformed, and the error of the climate reconstruction was estimated by the bootstrapping method (conducted 100 times). The transfer function was constructed using C2 software. To construct a diatom-water temperature transfer function, we used the results of a two-year diatom study of the seasonal diatom succession in Yunlong Lake (Zou et al., 2018). Details of the deployment of the sediment trap (Figure 1B), sample collection and diatom analysis are given in Zou et al. (2018). The results showed that water temperature is the most important environmental variable controlling the seasonal succession of diatom assemblages in Yunlong Lake (Figure 2). However, the diatom assemblages postdating November 2014 were significantly influenced by an increase in lake trophic status (Zou et al., 2018), and therefore we only used the 12 samples collected before November 2014 to construct the transfer function. Based on the high correlation between seasonal diatom assemblages and the MSWT (mean surface water temperature at depths of 1, 5, and 7 m), we constructed a diatom-MSWT transfer function for Yunlong Lake in order to quantitatively reconstruct the past changes in surface water temperature.
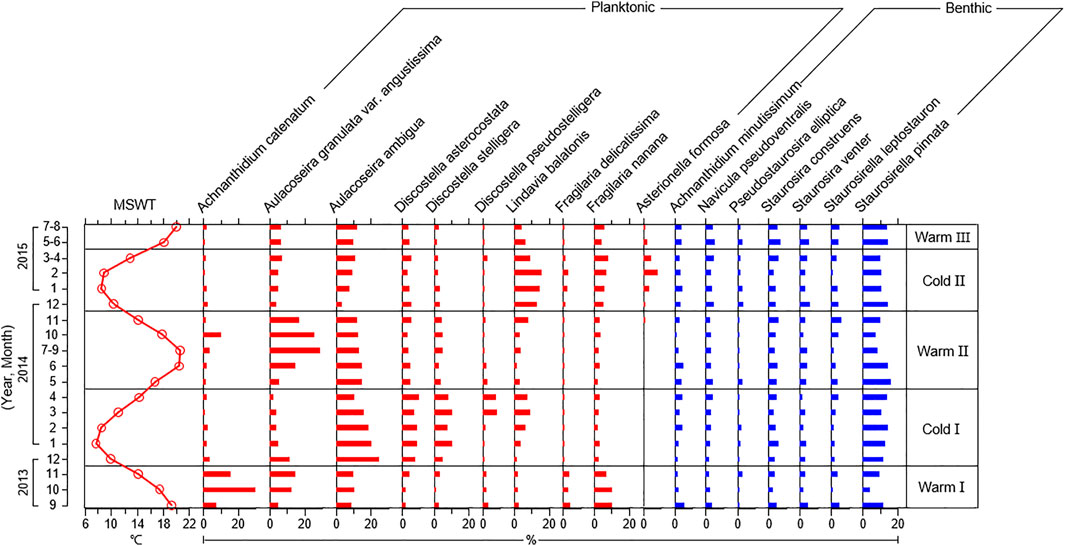
FIGURE 2. Changes in the mean relative abundance of the main diatom species from diatom seasonal investigation of Yunlong Lake. MSWT represents the mean surface water temperature at depths of 1, 5 and 7 m. Three warm and two cold seasons are defined by changes in monthly average water temperature at the four depths within the water column (Zou et al., 2018).
To construct a diatom-water depth transfer function, we used the results of an investigation of the spatial distribution of lake sediment surface diatom assemblages according to water depth (Zou et al., 2020). The results showed that the surface diatom assemblages are highly correlated with water depth: epiphytic and benthic diatoms are dominant in the littoral and shallow water zones, and the percentage of planktonic diatoms increases significantly in the deep profundal zone (Figure 3). Based on this relationship, we constructed a diatom-water depth transfer function, which can be used to quantitatively reconstruct past changes in lake water level and hence regional precipitation.
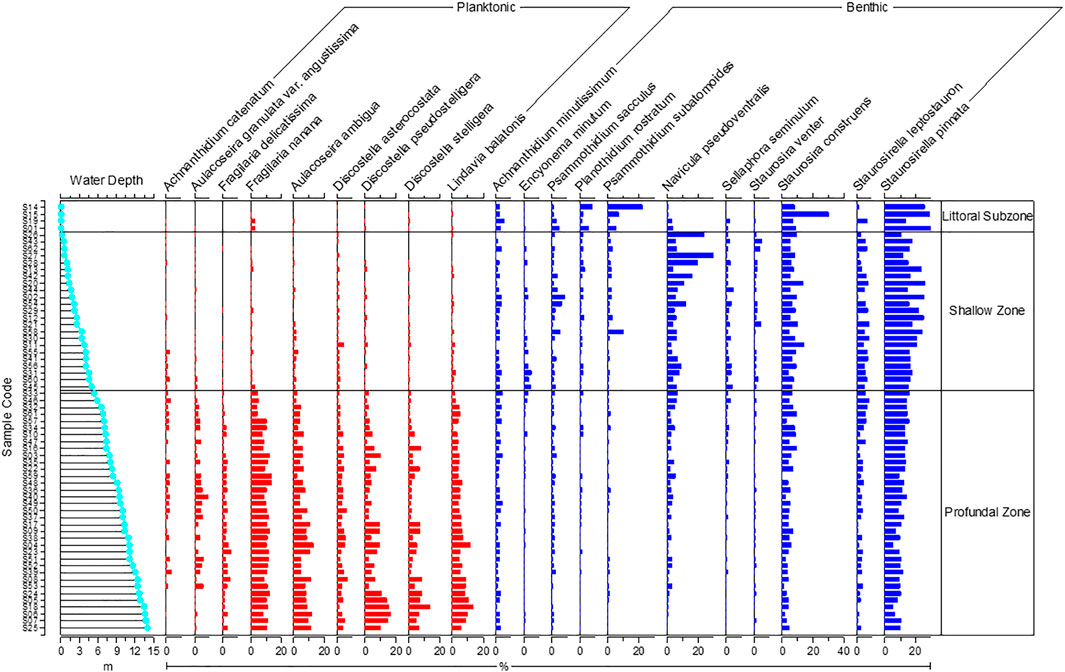
FIGURE 3. Changes in diatom assemblages vs. water depth for the 62 surface samples from Yunlong Lake. Only diatom species with a total relative abundance >1% are shown.
Meteorological Monitoring and Reservoir Capacity Records
To evaluate the sensitivity and validity of the diatom-based transfer functions for temperature and precipitation in the lake catchment, we collected meteorological observations (including mean annual air temperature and mean annual precipitation data) from the nearby Dali Station (covering 1951–2013, ∼ 150 km away from Yunlong Lake) and Yunlong Station (1977–2013, just surrounding Yunlong Lake), and measured changes in the water capacity of the reservoir from engineering records which were used to determine long-term changes in water level.
Chronology and Diatom Analysis :
In July 2013, we retrieved a short sediment core (YL2013-A, Figure 1B) from near the lake center using a UWITEC gravity corer. The chronology of the uppermost 39 cm of the core was determined using the 210Pb distribution calculated using the CRS (Constant Rate of Supply) model, together with the location of peaks in the distribution of 137Cs (Zou et al., 2020). Diatom analysis of all sediment samples was carried out according to standard methods (Battarbee et al., 2001). Diatoms were identified to species level mainly using the taxonomic references of Krammer and Lange-Bertalot (1986-1991), and with reference to algaebase (https://www.algaebase.org/) for the identification of rare species. For all sediment samples, at least 600 diatom valves were counted, and the diatom data were expressed as percentage relative abundance of the total number of valves counted.
Results
The final diatom-mean surface water temperature (MSWT) transfer function was constructed using the screened 12 sediment trap samples. The results of a test of the performance and prediction ability of the transfer function constructed using Component 1 and Component 2 with the WAPLS method are listed in Table 1. It can be seen that predictive ability of the transfer function constructed using Component 2 has a better fit to the observations (R2 = 0.97) and a smaller residual (RMSE = 0.53) (Figure 4). However, it did not pass the random t-test, likely due to the small number of samples involved in the training set.

TABLE 1. Comparison of the performance of diatom mean surface water temperature (MSWT) transfer function constructed using WAPLS-Component 1 and Component 2 in predicting changes in surface water temperature.
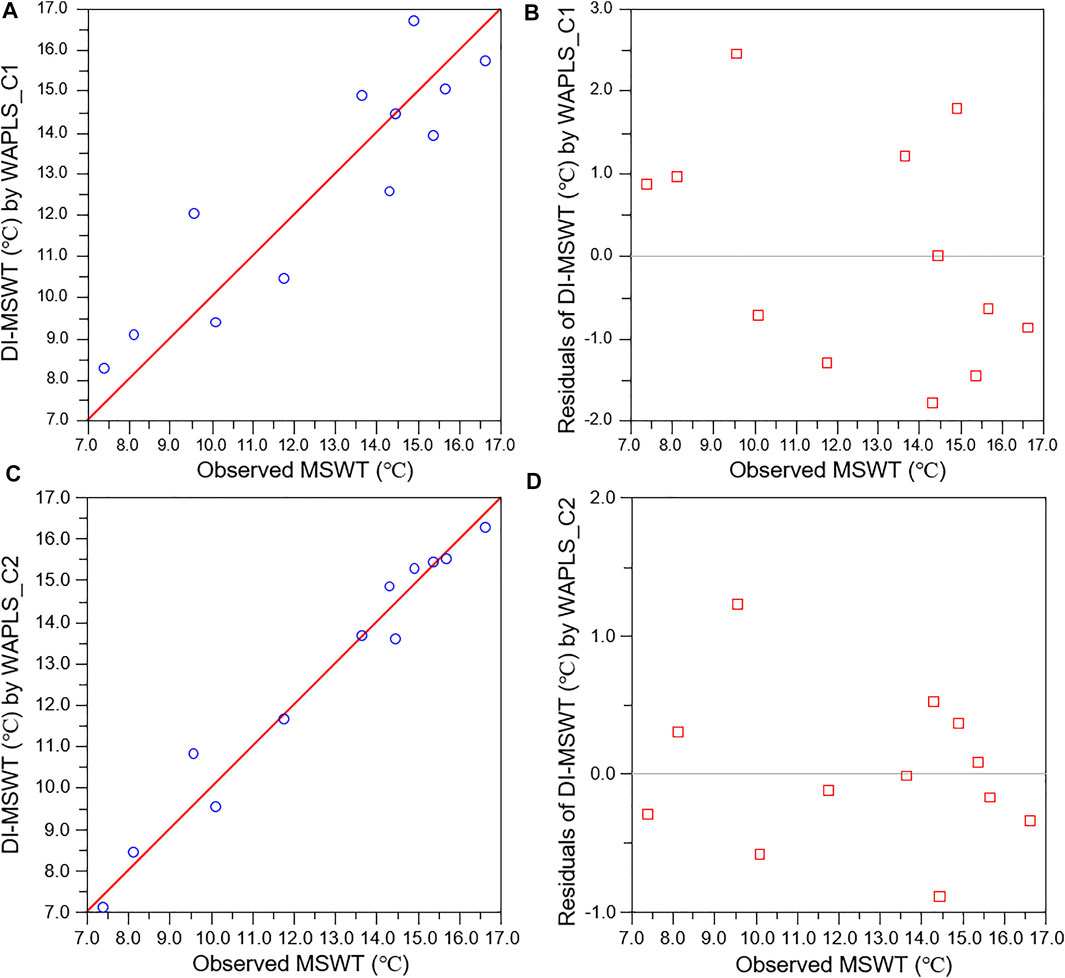
FIGURE 4. Performance of the diatom-inferred mean surface water temperature (DI-MSWT) transfer function for Yunlong Lake, constructed by WAPLS (Components 1, 2) method. (A) Regression analysis results of the DI-MSWT using Component 1 and the measured dataset. (B) Residuals of the DI-MSWT using Component 1. (C) Regression analysis results of the DI-MSWT based on Component 2 and the measured dataset. (D) Residuals of the DI-MSWT using Component 2.
The diatom-water depth (WD) transfer function was constructed using the 62 surface samples. Transfer functions constructed using Component 1 and Component 2 using the WAPLS method were both effective in predicting water depth, with R2 > 0.9 (Table 2); however, the predicted water depth of the transfer function constructed using Component 2 had the better fit to the observations (R2 = 0.95) and a smaller residual (RMSE = 0.96) (Figure 5), which also passed the random t-test (p = 0.04 < 0.05) (Table 2).

TABLE 2. Comparison of the performance of diatom water depth (WD) transfer function constructed using WAPLS-Component 1 and Component 2 in predicting changes in water depth.
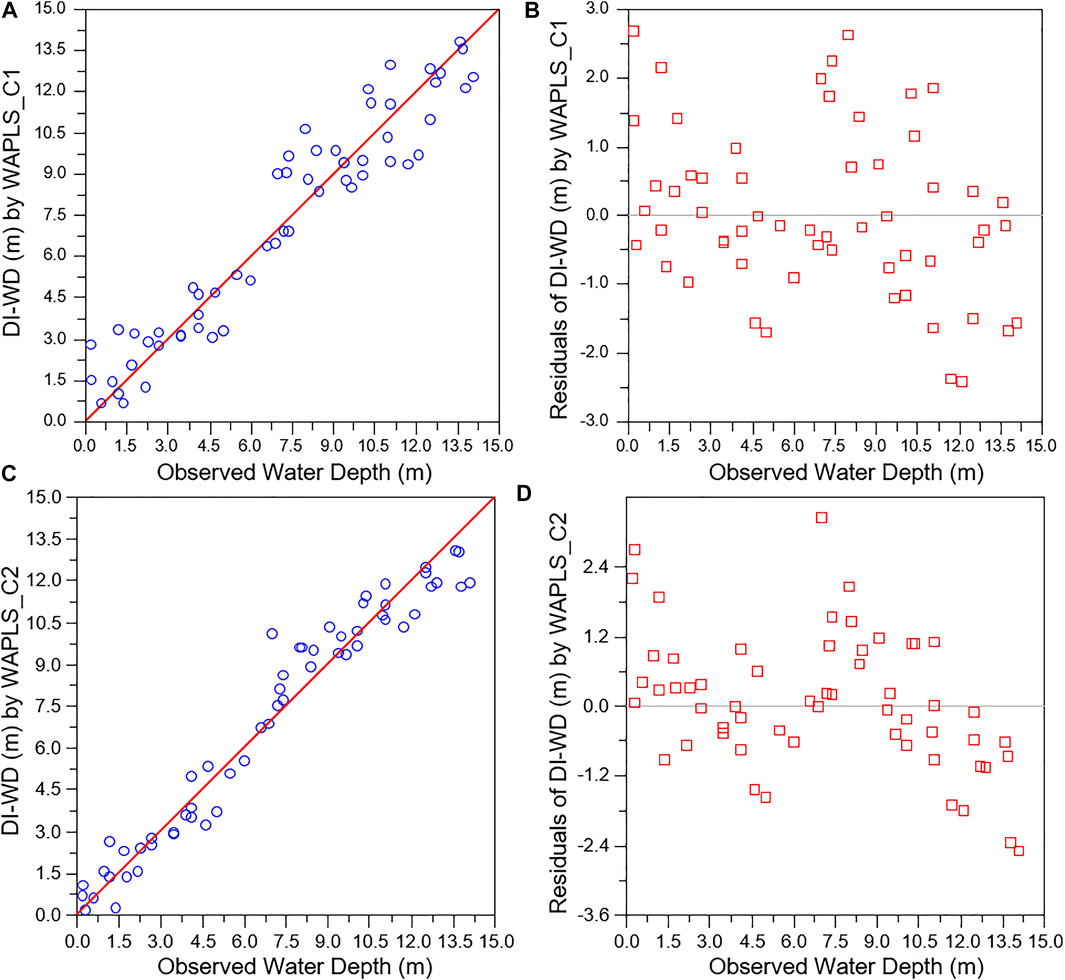
FIGURE 5. Performance of the diatom-inferred water depth (DI-WD) transfer function for Yunlong Lake, constructed by WAPLS (Components 1, 2). (A) Regression analysis results of the DI-WD using Component 1 and the measured dataset. (B) Residuals of the DI-WD using Component 1. (C) Results of regression analysis of the DI-WD using Component 2 and the measured dataset. (D) Residuals of the DI-WD using Component 2.
The age range of the upper 39 cm of sediment core YL2013-A is 1919–2013, nearly 100 years (Zou et al., 2020). Using the constructed diatom-MSWT and diatom-WD transfer functions, combined with the diatom assemblage succession in the core (Zou et al., 2020), we reconstructed the changes in the annual average surface water temperature and the annual average water level of Yunlong Lake over the last ∼100 years using the WAPLS method (Component 1 and Component 2) (Figure 6). The trend of variation of water temperature and water level reconstructed by Component 1 and Component 2 is very similar, with the difference being the range of the reconstructed variables and the estimated errors (Figure 6). The reconstructed MSWT using Component 1 ranges from 9.21 to 13.03°C, with an average of 10.6°C, and the estimated error range is from 0.6 to 1.33°C. The reconstructed MSWT using Component 2 ranges from 8.97 to 12.18°C, with an average of 10.58°C, and the estimated error range is from 0.7 to 1.67°C. The annual average water level of Yunlong Lake over the last ∼100 years reconstructed by Component 1 ranges from 6.23 to 13.51 m, with an average of 9.5 m, and an estimated error ranging from 0.23 to 0.39 m. While the annual average water level reconstructed by Component 2 ranges from 5.47 to 12.52 m, with an average of 8.98 m, and an estimated error ranging from 0.37 to 0.69 m. Because both transfer functions constructed using Component 2 have a better performance (a higher R2 value and a smaller residual), we selected the results reconstructed by Component 2 and compared them with the meteorological and reservoir capacity records (Figure 7) in order to evaluate their sensitivity and validity for reconstructing climate changes.
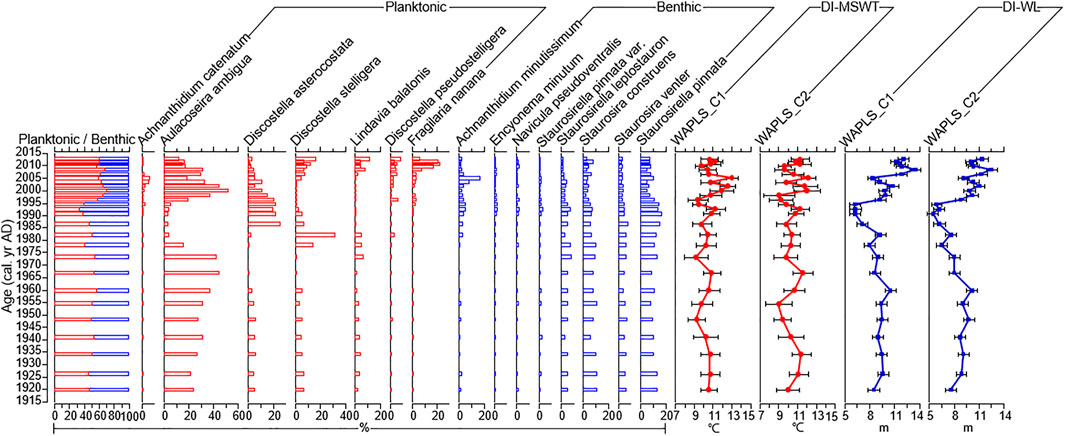
FIGURE 6. The succession of dominant diatom assemblages over the last ∼ 100 years in sediment core YL2013-A from Yunlong Lake and diatom-inferred MSWT and water level (WL) obtained using a transfer function using WAPLS (Components 1, 2).
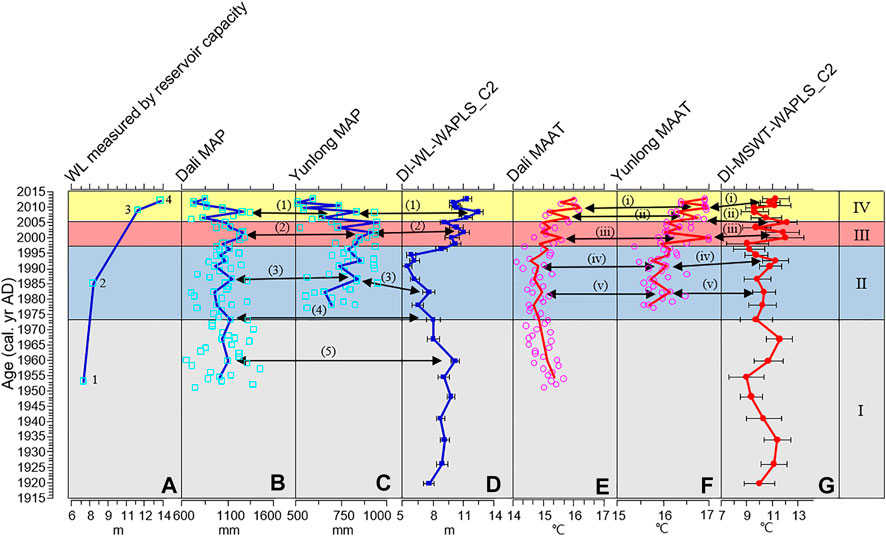
FIGURE 7. Comparison of diatom-inferred lake water level, mean surface water temperature and meteorological and reservoir capacity records over the last ∼100 years in the Yunlong Lake area. (A) Long-term change in lake water level estimated by the reservoir capacity records and the measured water level in 2013. (B) Mean annual precipitation (MAP) during 1950–2013 recorded by Dali Station. The squares represent the measured data points, and the solid line represents the fitted trend of the precipitation corresponding to the diatom sampling points based on sediment dating, and the same below. (C) Mean annual precipitation (MAP) during 1977–2013 recorded at Yunlong Station. (D) Diatom-inferred lake water level obtained using WAPLS-Component 2 and the estimated reconstruction errors. (E) Mean annual air temperature (MAAT) during 1950–2013 recorded at Dali Station. The circles represent the measured data points, and the solid line represents the fitted trend of the air temperature corresponding to the diatom sampling points based on sediment dating, and the same below. (F) Mean annual air temperature (MAAT) during 1977–2013 recorded at Yunlong Station. (G) Diatom-inferred MSWT obtained using WAPLS-Component 2 and the estimated reconstruction errors. 1–4 represents four damming and reservoir extension time interval. (1)–(5) represents five interannual interval of increased precipitation. (i)–(v) represents five interannual interval of increased temperature.
Because the monitoring interval of Yunlong Station is relatively short (since 1977), we conducted Pearson correlation analysis of the mean annual air temperature (MAAT) and the mean annual precipitation (MAP) obtained from Dali and Yunlong Station since 1977. The resulting correlation coefficients for MAAT and MAP are 0.94 and 0.71, respectively, indicating that the patterns of climate change recorded at the two locations are essentially the same, and both reliably reflect the climatic conditions of the Yunlong Lake area (Figure 7).
We estimated the approximate water level during four phases (described below) based on the water capacity of the reservoir recorded by the dam construction project and the measured water level in 2013 (Zou et al., 2020). The results show that since the beginning of dam construction in 1953, the water level of Yunlong Lake exhibited a stepwise increase due to the dam construction and the reservoir extension project: the water level rose from 7 m (the reservoir water capacity was 7.26 × 106 m3) in 1953 to 7.79 m (7.9 × 106 m3) when dam construction was completed in 1985. When the reservoir extension project was completed in 2009, the water level increased to 11.45 m (1.09 × 107 m3), and the final water level measured during field work in 2013 was 13.3 m (Figure 7A).
By comparison, we found that the water temperature and water level change of Yunlong Lake over the last ∼100 years reconstructed by the diatom transfer function correspond well to the meteorological observations of air temperature and precipitation and the long-term stepwise increasing trend of water level estimated by the reservoir capacity record (Figure 7A). Based on these records, the pattern of climate change of the Yunlong region over the last ∼100 years can be divided into four phases, as follows.
Phase I (∼1919–1973): relatively stable temperature and precipitation. Overall, the temperature was relatively high, and the diatom-inferred MSWT ranges from 8.97 to 11.6°C, with an average of 10.36°C. The MAAT recorded by Dali Station for 1951–1973 varied from 14.46 to 15.68°C, with an average 15.05°C. The climate was generally humid during this interval. The MAP recorded by Dali Station during 1951–1973 is 1,080 mm, and the diatom-inferred lake water level ranges from 7.61 to 10.17 m, with an average of 8.84 m.
Phase II (∼1973–1997): decreasing temperature, precipitation and lake water level. The MAAT recorded by Dali Station ranges from 14.12 to 15.3°C, with an average of 14.79°C; and the MAAT recorded by Yunlong Station for 1977–1997 ranges from 15.38 to 16.52°C, with an average of 15.93°C. The diatom-inferred MSWT ranges from 9.04 to 11.27°C, with an average of 10.07°C. All of these values are lower than during Phase I. Precipitation also decreased during this phase. The averaged MAP recorded by Dali Station was 1,024 mm, and the MAP recorded by Yunlong Station is 559–951 mm with an average of 772 mm; thus the climate was relatively dry. The diatom-inferred lake water level has the range of 5.47–10.21 m, with an average of 7.11 m, which is much lower than during Phase I.
Phase III (∼1997–2005): increasing temperature, precipitation and lake water level. The temperature records show that temperatures in the region began to increase, with averaged MAAT recorded by Dali and Yunlong Stations of 15.25 and 16.37°C, respectively. The diatom-inferred MSWT ranges from 9.75 to 12.18°C, with an average of 11.45°C. Regional precipitation also increased. The averaged MAP recorded by Dali and Yunlong Stations during this interval was 1,148 and 814 mm, respectively, which is much higher than during Phase II. The diatom-inferred lake water level ranges from 9.16 to 11.04 m, with an average of 10.09 m, which is much higher than during Phase II.
Phase IV (∼2005–2012): increasing air temperature, decreasing water temperature and rainfall, and increasing lake water level. The records of air temperature and water temperature show opposing trends during this interval. The averaged MAAT recorded by Dali and Yunlong Stations was 15.71 and 16.66°C, respectively; this is higher than during Phase III and indicates the regional air temperature continued to increase. However, the diatom-inferred MSWT ranges from 9.59 to 11.29°C, with an average of 10.51°C, which is much lower than during the preceding phase. The averaged MAP recorded by Dali and Yunlong Stations was 963 and 667 mm, respectively, which is much lower than during Phase III. However, in response to the completion of the reservoir extension project, the lake water level increased, with the diatom-inferred water level ranging from 10.04 to 12.52 m, with an average of 10.96 m.
A total of five intervals of relatively high diatom-inferred water level occurred, which match the interannual pattern of increased precipitation, within the range of the dating error (Figure 7). Additionally, there are five intervals of relatively high diatom-inferred water temperature which correspond to the intervals of observed increases in air temperature (Figure 7).
Discussion
Much research work has been conducted on quantitative paleoclimatic reconstruction using diatom transfer functions, which is a generally accepted method of paleoclimatic reconstruction. For example, the seasonal diatom succession in lakes has been used to reconstruct temperature changes (Lotter and Bigler, 2000; Kӧster and Pienitz, 2006), and the spatial pattern of surface diatom assemblages with water depth has been used to reconstruct changes in lake water level (Moos et al., 2005; Laird et al., 2010; Laird et al., 2011). However, Juggins (2013) suggested that the observed correlation between diatom assemblages and temperature and water depth may be an artifact, and that temperature and water depth may not be the primary environmental variables controlling changes in diatom assemblages. Moreover, the lack of reliable evidence has meant that the validity of these climatic reconstructions is still questioned.
The results from Yunlong Lake demonstrate that a surface sediment diatom-water depth transfer function established within a single lake can effectively be used to reconstruct water level changes. The investigation of the spatial pattern of surface diatom assemblages with water depth at Yunlong Lake shows that water depth is the most important environmental variable determining the pattern of surface diatom assemblages. This is because water depth exerts a strong control on the ratio of planktonic to benthic diatoms and of the relative abundances of different benthic diatoms (Zou et al., 2020). Although this spatial pattern of surface diatom assemblages is in fact controlled by the degree of light penetration, it is directly linked with water depth, which leads to the observed high correlations between surface diatom assemblages and water depth (Kingsbury et al., 2012). Although the spatial pattern of diatom assemblages in a lake may also be affected by factors such as the nutrient content and pH of the lake water, many studies have shown that water depth is the most important environmental factor (Moos et al., 2005; Laird et al., 2010; Laird et al., 2011; Kingsbury et al., 2012; Zou et al., 2020). We have used the observed high correlation between water depth and surface diatom assemblages at Yunlong Lake to construct a diatom-water depth transfer function, which was then used to reconstruct the history of lake water level changes over the last ∼100 years.
Comparison with the meteorological rainfall records and the long-term trend of water level estimated by the record of reservoir water capacity, the diatom-inferred record of lake water level is shown to accurately track changes in precipitation. For example, within the dating error, the diatom-inferred lake level tracks the five interannual-scale intervals of heavy precipitation (Figure 7). Moreover, the diatom-inferred water level is also coupled with the long-term stepwise rise in water level caused by the human activities, which is especially pronounced in Phase IV (Figure 7). The diatom-inferred water level of the lake was generally high, mainly due to the implementation of the reservoir extension project, while the meteorological records show that the MAP during this interval decreased substantially (Figure 7).
In addition, the changes in diatom-inferred MSWT reliably reflect the water temperature fluctuations at Yunlong Lake over the last ∼100 years, which include the signal of natural climate change prior to human disturbance (Phases I, II and III) and the ecological response signal of the lake under strong human disturbance (Phase IV). During Phases I, II and III, the diatom-inferred surface water temperature changes are largely consistent with changes in air temperature. For example, during Phase II both the air temperature and water temperature were relatively low overall; and during Phase III, both the regional air temperature and the water temperature increased substantially (Figure 7). In addition, the diatom-inferred MSWT captured five interannual-scale warming events (Figure 7), which indicates that diatoms respond sensitively and rapidly to regional temperature changes, and that the diatom-inferred water temperature changes are reliable.
During Phase IV, the diatom-inferred MSWT decreased substantially, but the MAAT in the study area continued to increase (Figure 7). The opposing trends of air temperature and water temperature during this period were mainly the result of human disturbance. From October 2003 to July 2009, the local government implemented a large-scale reservoir extension project for Yunlong Lake, which led to a short-term large increase in the reservoir water capacity which may have triggered an immediate response of the lake ecology. When the external heat source (i.e, solar radiation) for the lake remained essentially unchanged or increased slightly (as a result of regional warming), the increased heating of the lake water may not have been sufficient to offset the greatly increased thermal capacity of the reservoir within a short interval, resulting in the observed pronounced decrease in lake water temperature (Zou et al., 2020). Because diatoms respond directly to changing water temperature, the decrease in water temperature finally led to the dominance of diatom assemblages that bloomed in the cold season (Zou et al., 2018), while the relative abundance of warm-preference diatom species, such as Achnanthidium catenatum, did not increase with the further warming of the study area (Figure 6). As a result, the diatom-inferred MSWT decreased substantially during this interval, but the air temperature continued to increase (Figure 7).
Conclusions
1) Diatom-climate reconstruction and the meteorological records aiming at Yunlong Lake over the last ∼100 years show that climatic and environmental change of the Yunlong Lake region experienced four phases. Phase I (∼1919–1973), relatively stable temperature and precipitation; Phase II (∼1973–1997), decreasing temperature, precipitation and lake water level; Phase III (∼1997–2005), increasing temperature, precipitation and lake water level; Phase IV (∼2005–2012), increasing air temperature, decreasing water temperature and rainfall, and increasing lake water level.
2) At a lake site where temperature and water depth are the dominant environmental variables determining the seasonal succession and spatial pattern of diatom assemblages, a diatom-based transfer function was constructed and is shown to provide a reliable quantitative reconstruction of past changes in temperature and precipitation of different timescales in the study area.
3) When using lake diatoms to reconstruct past water temperature changes, the impact of substantial changes in lake water volume on thermal properties and the resulting ecological response should be considered.
Data Availability Statement
The original contributions presented in the study are included in the article/Supplementary Material, further inquiries can be directed to the corresponding author.
Author Contributions
YZ and LW designed research. YZ, LW, JZ, and YY collected samples. YZ and ZG analyzed data. YZ, LW, HH, and GL wrote and revised the article, and HZ provided suggestions. All authors read and approved the final manuscript.
Funding
This study was supported by the National Natural Science Foundation of China (42001077, 41772379, 41991323), China Postdoctoral Science Foundation (2021T140582), and the National Key R&D Program of China (2017YFA0603400).
Conflict of Interest
The authors declare that the research was conducted in the absence of any commercial or financial relationships that could be construed as a potential conflict of interest.
Acknowledgments
We thank Jan Bloemendal for polishing the manuscript.
References
Battarbee, R. W., Jones, V. J., Flower, B. P., Cameron, N. G., Bennion, H., Carvalho, L., et al. (2001). “Diatoms,” in Tracking Environmental Change Using Lake Sediments. Editors JP Smol, HJB Birks, and W M Last (Dordrecht: Kluwer Academic Publishers), 155–202. doi:10.1007/978-94-007-2745-8
Birks, H. H. (2012). Ecological Responses to Late-Glacial Climate Changes in Northern and Western Norway. Quat. Int. 279-280, 52. doi:10.1016/j.quaint.2012.07.227
Dong, X., Yang, X., Chen, X., Liu, Q., Yao, M., Wang, R., et al. (2016). Using Sedimentary Diatoms to Identify Reference Conditions and Historical Variability in Shallow lake Ecosystems in the Yangtze Floodplain. Mar. Freshw. Res. 67, 803–815. doi:10.1071/mf14262
Jiang, W., Leroy, S. A. G., Yang, S., Zhang, E., Wang, L., Yang, X., et al. (2019). Synchronous Strengthening of the Indian and East Asian Monsoons in Response to Global Warming since the Last Deglaciation. Geophys. Res. Lett. 46, 3944–3952. doi:10.1029/2019GL082084
Juggins, S. (2013). Quantitative Reconstructions in Palaeolimnology: New Paradigm or Sick Science?. Quat. Sci. Rev. 64, 20–32. doi:10.1016/j.quascirev.2012.12.014
Kingsbury, M. V., Laird, K. R., and Cumming, B. F. (2012). Consistent Patterns in Diatom Assemblages and Diversity Measures across Water-Depth Gradients from Eight Boreal Lakes from north-western Ontario (Canada). Freshw. Bio. 57, 1151–1165. doi:10.1111/j.1365-2427.2012.02781.x
Krammer, K., and Lange-Bertalot, H. (1986-1991). Bacillariophyceae. Süsswasserflora von Mitteleuropa, Band 2. Berlin: Spektrum Akademischer Verlag Heidelberg.
Kӧster, D., and Pienitz, R. (2006). Seasonal Diatom Variability and Paleolimnological Inferences-A Case Study. J. Paleolimnol. 35, 395–416. doi:10.1007/s10933-005-1334-7
Laird, K. R., Kingsbury, M. V., and Cumming, B. F. (2010). Diatom Habitats, Species Diversity and Water-Depth Inference Models across Surface-Sediment Transects in Worth Lake, Northwest Ontario, Canada. J. Paleolimnol. 44, 1009–1024. doi:10.1007/s10933-010-9470-0
Laird, K. R., Kingsbury, M. V., Lewis, C. F. M., and Cumming, B. F. (2011). Diatom-inferred Depth Models in 8 Canadian Boreal Lakes: Inferred Changes in the Benthic:planktonic Depth Boundary and Implications for Assessment of Past Droughts. Quat. Sci. Rev. 30, 1201–1217. doi:10.1016/j.quascirev.2011.02.009
Li, J., Wang, L., Cao, Q., Rioual, P., Lei, G., Cai, B., et al. (2021). Diatom Response to Global Warming in Douhu Lake, Southeast China. Acta Geologica Sinica ‐ English Edition. 95, 638–647. doi:10.1111/1755-6724.14294
Lotter, A. F., and Bigler, C. (2000). Do diatoms in the Swiss Alps Reflect the Length of Ice-Cover?. Aquat. Sci. 62, 125–141. doi:10.1007/s000270050002
Magny, M. (2004). Holocene Climate Variability as Reflected by Mid-European lake-level Fluctuations and its Probable Impact on Prehistoric Human Settlements. Quat. Int. 113, 65–79. doi:10.1016/s1040-6182(03)00080-6
Moos, M. T., Laird, K. R., and Cumming, B. F. (2005). Diatom Assemblages and Water Depth in Lake 239 (Experimental Lakes Area, Ontario): Implications for Paleoclimatic Studies. J. Paleolimnol. 34, 217–227. doi:10.1007/s10933-005-2382-8
Rühland, K. M., Paterson, A. M., and Smol, J. P. (2015). Lake Diatom Responses to Warming: Reviewing the Evidence. J. Paleolimnol. 54, 1–35. doi:10.1007/s10933-015-9837-3
Smol, J. P., and Cumming, B. F. (2000). Tracking Long‐term Changes in Climate Using Algal Indicators in Lake Sediments. J. Phycology. 36, 986–1011. doi:10.1046/j.1529-8817.2000.00049.x
Smol, J. P., and Douglas, M. S. (2007). From Controversy to Consensus: Making the Case for Recent Climate Change in the Arctic Using lake Sediments. Front. Ecol. Environ. 5, 466–474. doi:10.1890/060162
Stone, J. R., and Fritz, S. C. (2004). Three-dimensional Modeling of Lacustrine Diatom Habitat Areas: Improving Paleolimnological Interpretation of Planktic: Benthic Ratios. Limnol. Oceanogr. 49, 1540–1548. doi:10.4319/lo.2004.49.5.1540
Wang, L., Jiang, W. Y., Jiang, D. B., Zou, Y. F., Liu, Y. Y., Zhang, E. L., et al. (2018). Prolonged Heavy Snowfall during the Younger Dryas. J. Geophys. Res. Atmos. 123, 13748–13762. doi:10.1029/2018JD029271
Wang, L., Li, J., Lu, H., Gu, Z., Rioual, P., Hao, Q., et al. (2012). The East Asian winter Monsoon over the Last 15,000 Years: its Links to High-Latitudes and Tropical Climate Systems and Complex Correlation to the Summer Monsoon. Quat. Sci. Rev. 32, 131–142. doi:10.1016/j.quascirev.2011.11.003
Wang, R., Yang, X., Langdon, P., and Zhang, E. (2011). Limnological Responses to Warming on the Xizang Plateau, Tibet, over the Past 200 Years. J. Paleolimnol. 45, 257–271. doi:10.1007/s10933-011-9496-y
Winder, M., and Cloern, J. E. (2010). The Annual Cycles of Phytoplankton Biomass. Phil. Trans. R. Soc. B. 365, 3215–3226. doi:10.1098/rstb.2010.0125
Yang, X., Kamenik, C., Schmidt, R., and Wang, S. (2003). Diatom-based Conductivity and Water-Level Inference Models from Eastern Tibetan (Qinghai-Xizang) Plateau Lakes. J. Paleolimnol. 30, 1–19. doi:10.1023/A:1024703012475
Zou, Y., Wang, L., Xu, H., Yan, Y., Zhang, J., Liu, Y., et al. (2020). Do changes in Water Depth and Water Level Influence the Diatom Diversity of Yunlong Lake, in Yunnan Province, Southwest China?. J. Paleolimnol. 64, 273–291. doi:10.1007/s10933-020-00137-x
Keywords: diatoms, transfer function, quantitative reconstruction, climate change, Yunlong Lake
Citation: Zou Y, Wang L, He H, Liu G, Zhang J, Yan Y, Gu Z and Zheng H (2021) Application of a Diatom Transfer Function to Quantitative Paleoclimatic Reconstruction — A Case Study of Yunlong Lake, Southwest China. Front. Earth Sci. 9:700194. doi: 10.3389/feart.2021.700194
Received: 25 April 2021; Accepted: 10 June 2021;
Published: 23 June 2021.
Edited by:
Xiangjun Liu, Northwest Normal University, ChinaReviewed by:
Xuhui Dong, Guangzhou University, ChinaRong Wang, Nanjing Institute of Geography and Limnology (CAS), China
Copyright © 2021 Zou, Wang, He, Liu, Zhang, Yan, Gu and Zheng. This is an open-access article distributed under the terms of the Creative Commons Attribution License (CC BY). The use, distribution or reproduction in other forums is permitted, provided the original author(s) and the copyright owner(s) are credited and that the original publication in this journal is cited, in accordance with accepted academic practice. No use, distribution or reproduction is permitted which does not comply with these terms.
*Correspondence: Yafei Zou, zouyafei123@ynu.edu.cn