- 1School of Earth Sciences, Northeast Petroleum University, Daqing, China
- 2Key Laboratory of Oil and Gas Reservoir Formation Mechanism and Resource Evaluation of Heilongjiang Province, Northeast Petroleum University, Daqing, China
- 3Department of Petroleum Engineering, Northeast Petroleum University, Daqing, China
- 4Key Laboratory of Enhanced Oil Recovery (Northeast Petroleum University), Ministry of Education, Daqing, China
Carbon dioxide (CO2) injection has become an important technology to enhance oil recovery in ultra-low permeability reservoirs. Compared with other CO2 flooding technologies, CO2 miscible flooding has a better development effect, and the minimum miscible pressure (MMP) is a key parameter to realize miscible flooding. Therefore, it is very important to accurately predict the MMP. The prediction methods of MMP generally include laboratory experiment method and theoretical calculation method. In this study, a long-slim-tube displacement experiment method was used to determine the MMP in the study area, and the experimental temperature and pressure were consistent with those under reservoir conditions. The research results show that the recovery ratio increased gradually with the increase of experimental pressure, but the increase amplitude gradually decreased. According to the relation curve between crude oil recovery ratio and experimental displacement pressure, when the experimental pressure was larger than 29.6 MPa, the recovery ratio did not increase significantly with the increase of displacement pressure, which indicates that the interfacial tension between crude oil and CO2 disappeared under this pressure and they reached a miscible state. It is speculated that the MMP between crude oil and CO2 system in the study area predicted by the long-slim-tube displacement experiment method was 29.6 MPa. The results of this study help to realize miscible flooding in ultra-low permeability reservoirs and thus enhance oil recovery.
Introduction
Enhanced oil recovery (EOR) by carbon dioxide (CO2) injection has been widely applied by the U.S., Russia, Canada, China, and other countries (Mogensen, 2016; Azizkhani and Gandomkar, 2020). CO2 injection applies to most ultra-low permeability oilfields. Compared to other gas injection technologies, CO2 injection has the merits of significantly enhanced oil recovery and low cost, so it is widely used (Jalilov et al., 2017; Kolster et al., 2017; Oschatz and Antonietti, 2018; Zhou et al., 2018; Chen et al., 2020). At the same time, some CO2 can be stored underground by CO2 flooding to reduce greenhouse gas emissions (Qin et al., 2015; Ahmadi et al., 2017; Birdja et al., 2019; Zhang et al., 2020a). In this regard, CO2 flooding has a broad application prospect in the context of energy shortage, energy conservation, and emission reduction (Guo et al., 2017; Berneti and Varaki, 2018; Mutailipu et al., 2019; Gong et al., 2020). Laboratory and field tests have shown that CO2 miscible flooding is superior to immiscible flooding in enhancing oil recovery for light oil and middle viscosity crude oil (Wu et al., 2015; Shao et al., 2020; Wu et al., 2020), and the minimum miscible pressure (MMP) is a key parameter to realize miscible flooding. Therefore, it is very important to accurately predict the MMP.
The determination methods of MMP generally include laboratory experiment method and theoretical calculation method. Laboratory experiment methods cover long-slim-tube displacement experiment method, interfacial tension method, rising bubble apparatus (RBA) method, and vapor density method. Theoretical calculation methods are mainly empirical formula method, equation of state method, and simulation calculation method (Zhang et al., 2008; Yang et al., 2019; Zhang et al., 2020b; Kaufmann and Connelly, 2020).
Long-Slim-Tube Displacement Experiment Method
This method is an effective one-dimensional flow displacement experimental model. When the pore size of the porous medium is very small, viscous fingering will be offset by lateral diffusion. The repeated accurate results can be given by the slim-tube experiment method. Ultimate recovery factors obtained from the displacement experiments are plotted into a curve, and the turning point in the curve is determined as the pressure at which dynamic miscibility appears for the first time (Han et al., 1989; Zuo et al., 1993; Yi, 2000; Li et al., 2002; Hao et al., 2005).
Interfacial Tension Method
From 1965 to 1989, Benham, Stalkup, Holm, Lake, and other scholars believed according to their research results that when the fluids mix at any ratio and reach miscibility, interface between fluids will disappear, that is, the interfacial tension becomes zero. When the interfacial tension under different gas components and different pressures is determined by an interfacial tension tester, and the interfacial tension is extrapolated as zero, the corresponding pressure is the minimum miscible pressure (MMP) (Zhang et al., 2006; Peng et al., 2007; Gunde et al., 2010; Ghorbani et al., 2014; Li et al., 2016).
RBA Method
Determining the MMP with this method was proposed by Christiansen and Kim in 1986. This method has the characteristics of short determination period and relatively low instrument requirements, allowing direct observation of the miscible process; the MMP is determined based on the shape and movement distance of the bubble, rather than the pressure associated with recovery ratio (Elsharkawy et al., 1996; Dong et al., 2001).
Vapor Density Method
This is a dynamic test method proposed by Harmon and Grigg in 1988 (Richard and Reid, 1988). It has the characteristics of short time and low cost. It directly determines the relationship between the density and pressure of injected gas-rich phase and confirms the MMP of miscible gas and crude oil using the dissolution characteristics of gas and oil.
Empirical Formula Method
It is a simple and fast theoretical method for calculation and often used to predict the MMP. Scholars have proposed many empirical formulas according to different applicable conditions (Yuan et al., 2005; Ye, 2009; Li et al., 2012), including MMP (pmm) correlation (Ye, 2009), National Petroleum Council (NPC) method (Ye, 2009), Holm and Josendal correlation (Li et al., 2012), Mungan correlation (Yuan et al., 2005), Glaso empirical formula (Glaso, 1985), Johnson and Pollin (JP) empirical formula (Johnson and Pollin, 1981), the Petroleum Recovery Institute empirical formula (Johnson and Pollin, 1981), Cronquist empirical formula (Cronquist, 1978), Yellig–Metcalfe correlation (Yellig and Metcalfe, 1980), empirical formula by ORR and SILVA (1987), empirical formula by Alston et al. (1985), and so on. The empirical formula method is simple and fast in calculation, but because the parameters examined are different and have their respective applicable scope, there are differences in prediction accuracy. In the practical application, the appropriate formula should be selected according to the actual situation of the reservoir.
Equation of State Method
This is a numerical method based on the system phase equilibrium theory and the equation of state. By studying the relationship between phase behavior and miscible function of the CO2–crude oil system, we can get the bubble point value of the system and then obtain the MMP value of the system (Guo et al., 1999; Sun et al., 2006; Ye et al., 2012; Fazlali et al., 2013). The equations of state for calculating the MMP include Peng–Robinson equation of state (PR-EOS) (Guo et al., 1999; Ye et al., 2012), Nasrifar–Moshfeghian equation of state (NM-EOS) (Fazlali et al., 2013) and modified statistical associating fluid theory equation of state (mSSAFT-EOS) (Sun et al., 2006). The MMP can be calculated quickly and accurately with the equation of state method, but there is no clear judgment criterion for the miscible function, so it needs to be considered comprehensively according to the actual reservoir conditions and fluid characteristics in calculation.
Simulation Calculation Method
The method includes phase behavior simulation calculation method and artificial intelligence algorithm (Chen, 2016). In the phase behavior simulation calculation method, the effect of CO2 gas injected into the reservoir on crude oil properties is investigated by the phase behavior simulation technology, then the parameters of equation of state are adjusted by fitting PVT experimental data to establish a phase state model that conforms to the real fluid, and finally the MMP of oil and gas system is calculated by simulating the multistage contact experiment process. Artificial intelligence algorithm is a new MMP predicting method in recent years, which mainly includes artificial neural network (ANN) method, genetic algorithm (GA), particle swarm optimization (PSO), ant colony algorithm (ACA), simulated annealing algorithm (SAA), least-squares support vector machine (LSSVM) (Shokrollahi et al., 2013; Alomair and Iqbal, 2014; Rostami et al., 2018), and gene expression programming (GEP) (Tatar et al., 2013; Kamari et al., 2015; Tian et al., 2020). Compared with other numerical methods, the artificial intelligence method has the unique ability to identify the implicit linear or nonlinear relationship between input variables and target output values and a large number of parallel operations; it has a high prediction accuracy and is capable of processing large amounts of data in parallel. However, as the prediction process is realized by multiple conversion calculations and programming of input parameters, the internal correlation between each input parameter and MMP cannot be intuitively observed.
Among all the prediction methods, the long-slim-tube displacement experiment method has been widely used and recognized by researchers and scholars (Han et al., 1989; Zuo et al., 1993; Yi, 2000; Li et al., 2002; Hao et al., 2005; Zhang et al., 2008; Ekundayo and Ghedan, 2013; Zhang and Gu, 2015; Yang et al., 2019). The long-slim-tube displacement experiment can be operated repeatedly. Compared with other methods, this method is more aligned with the characteristics of oil and gas displacement process in the porous medium of crude oil reservoir. Moreover, it can greatly eliminate the influence of unfavorable factors such as heterogeneity, mobility ratio, viscous fingering, and gravity separation of lithology. Therefore, the long-slim-tube displacement experiment method was chosen to determine the MMP in the study area.
Determination of Minimum Miscible Pressure
Experimental Material
The crude oil used in the experiment was the simulated crude oil prepared with the ground crude oil and natural gas in the study area according to formation conditions and fluid characteristics. The experiment temperature was 108.5°C. When the temperature and pressure of the formation were 108.5°C and 23.8 MPa, the viscosity of crude oil was 1.88 mPa s. The molecular weight of C7+ in crude oil composition was 347.29 g/mol, and the density of C7+ was 0.8971 g/cm3. The composition data of crude oil components are shown in Table 1. The purity of CO2 gas was 99.9%, and its properties were exactly the same as the gas injected in the study area.
Preparation of the Formation Oil Sample
The experimental formation oil was prepared with ground oil and natural gas. Preparation process: pour a certain amount of ground oil into the high pressure physical instrument PVT barrel, seal it and heat it up to the formation temperature, then add the gas into the PVT barrel at the saturation pressure, stir and increase pressure to the formation pressure, and measure the saturation pressure of crude oil and the dissolved gas–oil ratio. If the saturation pressure and the actual formation oil saturation pressure are not equal, we should adjust the amount of dissolved gas in the PVT barrel until the saturation pressure and gas–oil ratio measured are equal to that of the formation oil (Li, 2006; Lewis, 2008; Abdalla et al., 2014; Adekunle, 2014).
Experimental Apparatus
The experimental apparatus mainly included (Yang et al., 2015; Han et al., 2016; Fathinasab et al., 2018) the piston container of simulated crude oil, the piston container of CO2, the piston container of formation water, slim tube filled with quartz sand particles, back pressure control valve, gas flowmeter, liquid flowmeter, etc. The sketch of experimental apparatus and flow chart are shown in Figure 1.
The injection pump was ISCO full automatic pump, with working pressure between 0 and 70 MPa and accuracy of 0.01 ml. The working pressure range of back pressure control valve was −70 MPa. The long slim tube was a one-dimensional artificial porous medium spiral stainless steel coil tightly filled with approximately 200 meshes of pure quartz sand. The accuracy of gas flowmeter was 1 ml. The highest temperature of thermostat was 200°C. The experimental parameters are shown in Table 2.
Experimental Procedure
The experiment was carried out in accordance with the petroleum and natural gas industry standard of the People’s Republic of China (Measurement Method For Minimum Miscibility Pressure By Slim Tube Test, 2016) (SY/T 6573-2016).
(1) Experiment preparation
Temperature setting: turn on the power switch of the thermostat to heat up. When the temperature is close to the formation temperature, start the constant temperature controller. After 3 h, the temperature can be completely maintained at the predetermined value.
Tube cleaning: saturate the slim tube model adequately with petroleum ether, and use high-pressure air to dry the petroleum ether.
CO2 introduction: open the pump and push the piston back to the top of the piston container to vent the gas in the container; inject the displacing gas CO2 into the piston container and close the valve.
Vacuumize the long slim tube, then fill the sand filling slim tube with formation water, and calculate the porosity.
Clean the formation water in the sand filling slim tube with toluene, and dry it in a thermostat to evaporate the toluene.
Fill the sand filling slim tube with simulated oil, inject the simulated oil into the slim tube model with the ISCO pump, and stop injection when 1.5 PV simulated oil was injected. Then, the injection and output of simulated oil were calculated, and the saturated oil injected into the tube was determined according to the volume difference, which is the end of the preparation process. Get ready for a displacement experiment.
2) Start the ISCO constant-pressure and constant-speed pump and increase the pressure of gas in the piston container to be 1∼3 MPa lower than the displacement pressure.
3) Raise the back pressure to the predetermined displacement pressure with a manual pump.
4) Open the pump at constant speed to inject CO2 for displacement, open the slim tube outlet valve, and adjust the gas pressure to make it equal to or slightly higher than the displacement pressure.
5) In the process of displacement, determine oil production, gas volume, and pump reading regularly as required and check oil sample saturation. When the cumulative volume injected into the pump is greater than 1.2 PV, stop the displacement and calculate the recovery ratio at this pressure.
6) At the end of the displacement process, start the cleaning process, inject the petroleum ether directly injected into the slim tube model, and keep the appropriate back pressure. Control the flow rate of petroleum ether in the slim tube properly, so as not to affect the cleaning effect. Close the outlet for 1–2 h, allow petroleum ether to fully contact with residual oil under high pressure dissolve residual oil in petroleum ether, and release it from the outlet. Repeat several times and discharge the mixture of petroleum ether and residual oil. When the components of the mixture and pure petroleum ether were basically the same, the residual oil in the model was determined to have been completely removed and fully saturated by petroleum ether. Then, high-pressure air was injected from the inlet to blow the remaining petroleum ether in the slim tube into the oil–gas separator, and dry the tube for next experiment.
7) Follow the abovementioned steps for displacement at the next pressure point.
In general, the MMP of a gas can be analyzed by measuring the recovery ratio at more than five pressure points. The experimental back pressures in experiment were 20, 25, 30, 35, 40, and 45 MPa. Then, the MMP was calculated according to the relationship between the recovery ratio and the experimental displacement pressure.
Experimental Result
Through the above experimental process, the data and relation curve of recovery ratio and CO2 injection amount at different experimental pressures are shown in Table 3 and Figure 2.
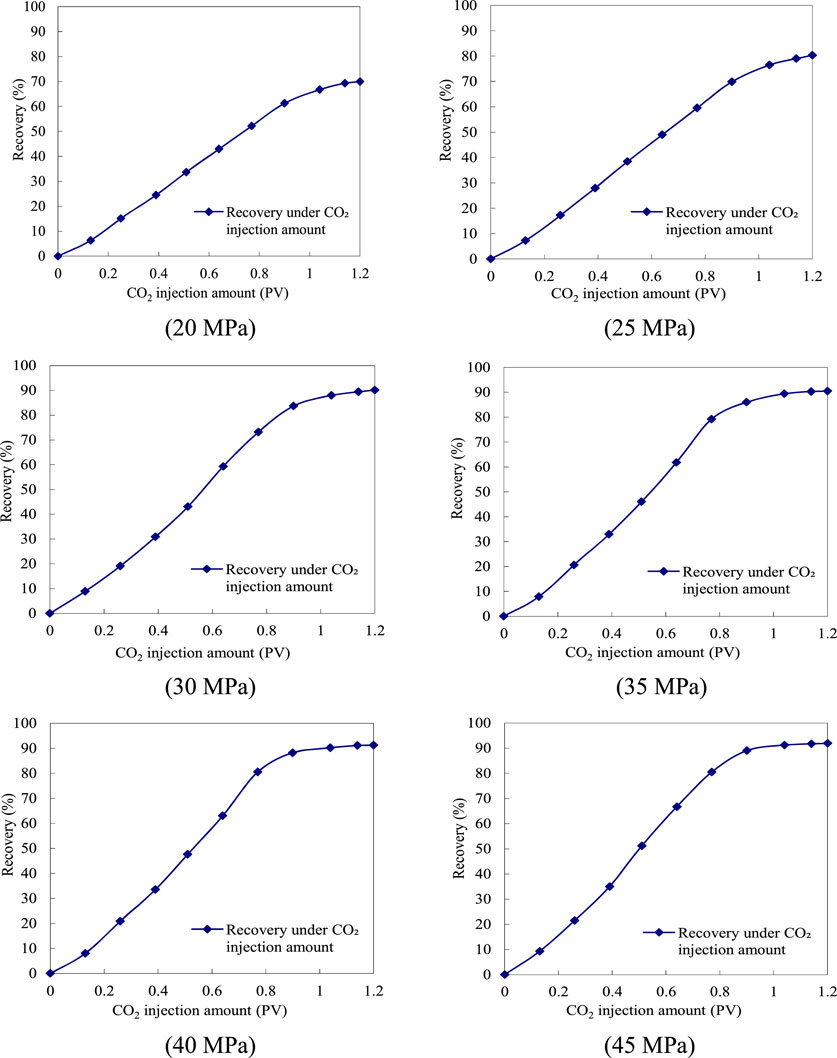
FIGURE 2. Relationship diagram between recovery ratio and CO2 injection amount under different pressures.
As seen from Table 3 and Figure 2, the recovery ratio increased with the increase of CO2 injection amount at different experimental pressures. When the experimental pressure was 20 and 25 MPa, the recovery ratio increased slightly after the CO2 injection amount was greater than 1.0 PV. When the experimental pressure was 30, 35, 35, and 40 MPa, the recovery ratio increased insignificantly after the CO2 injection amount was greater than 0.9 PV.
According to the experimental results, the recovery ratio by CO2 flooding under different experimental pressures can be obtained (Table 4).
The generally accepted criterion for miscible flooding in slim tube displacement experiment is that the recovery ratio is greater than 90% when the injection volume is 1.2 times the pore volume, and the displacement efficiency does not increase significantly with the increase of displacement pressure (Choubineh et al., 2016; KarimanMoghaddam and SaeediDehaghani, 2017; Ma et al., 2018; Ghorbani et al., 2019; Li et al., 2019; Yu et al., 2020). In Table 4, when the injected CO2 volume was 1.2 PV, the recovery ratio increased with increasing experimental pressure. When the experimental pressure was 20 and 25 MPa, the corresponding recovery ratio was lower than 90%, indicating that the CO2 flooding under these pressures was immiscible; when the experimental pressure was above 30 MPa, the corresponding recovery ratio was above 90%, indicating that the miscible flooding can be formed by CO2 flooding under the pressure of more than 30 MPa. According to the experimental results in Table 4, the relation curve between crude oil recovery ratio and experimental pressure when CO2 of 1.2 PV was injected was plotted (Figure 3). It can be seen from Figure 3 that the immiscible flooding stage and miscible flooding stage intersected at 29.6 MPa. When the experimental pressure was higher than 29.6 MPa, the recovery ratio did not increase significantly, which indicates that the interfacial tension between crude oil and CO2 disappeared under this pressure and they reached a miscible state. Therefore, the MMP between crude oil and CO2 system in the study area predicted by the long slim tube displacement experiment method was 29.6 MPa.
Conclusion
The crude oil used in the experiment was the simulated crude oil prepared with the ground crude oil and natural gas in the study area according to the formation conditions and fluid characteristics. The experiment was carried out in accordance with the petroleum and natural gas industry standard of the People’s Republic of China “Measurement Method for Minimum Miscible Pressure by Slim Tube Test” (SY/T 6573-2016). The experimental results show the recovery ratio increased with the increase of CO2 injection amount at different experimental pressures. When the experimental pressure was 20 and 25 MPa, the recovery ratio increased slightly after the CO2 injection amount was greater than 1.0 PV. When the experimental pressure was 30, 35, 35, and 40 MPa, the recovery ratio increased insignificantly after the CO2 injection amount was greater than 0.9 PV. When the experimental pressure was 20 and 25 MPa, the corresponding recovery ratio was lower than 90%, indicating that the CO2 flooding under these pressures was immiscible; when the experimental pressure was above 30 MPa, the corresponding recovery ratio was above 90%, indicating that the miscible flooding can be formed by CO2 flooding under the pressure of more than 30 MPa. The relation curve between recovery ratio and experimental pressure was plotted, and it shows that the oil recovery at CO2 injection of 1.2 PV increased with the increase of experimental pressure, and the immiscible flooding stage and miscible flooding stage intersected at 29.6 MPa. When the experimental pressure was higher than 29.6 MPa, the oil recovery did not increase significantly. Therefore, the MMP between crude oil and CO2 system in the study area determined by the long-slim-tube displacement experiment was 29.6 MPa. The results show that miscible flooding can be formed in the study area when the reservoir pressure is greater than 29.6 MPa; otherwise, it is impossible to achieve miscible flooding. The research results are applicable to oilfields with similar crude oil properties and CO2 properties.
Data Availability Statement
The original contributions presented in the study are included in the article/Supplementary Material; further inquiries can be directed to the corresponding author.
Author Contributions
GF and YZ contributed to conception and design of the study. XZ and YL organized the database. YZ, XZ, YL, and HC carried out the long-slim-tube displacement experiment. XZ, YL, and HC performed the statistical analysis. GF and YZ wrote the first draft of the manuscript. GF, YZ, XZ, YL, and HC wrote sections of the manuscript. All authors contributed to manuscript revision and read and approved the submitted version.
Funding
This work was financially supported by the National Natural Science Foundation of China (42002154 and 51834005), the Natural Science Foundation of Heilongjiang Province (E2016008 and QC2016055), the PetroChina Innovation Foundation (2020D-5007-0213), the Youths Science Foundation of Northeast Petroleum University (2019QNL-15), and the Open Fund Project of Heilongjiang Key Laboratory of Oil and Gas Reservoir Formation Mechanism and Resource Evaluation (KL20190101).
Conflict of Interest
The authors declare that the research was conducted in the absence of any commercial or financial relationships that could be construed as a potential conflict of interest.
Publisher’s Note
All claims expressed in this article are solely those of the authors and do not necessarily represent those of their affiliated organizations, or those of the publisher, the editors, and the reviewers. Any product that may be evaluated in this article, or claim that may be made by its manufacturer, is not guaranteed or endorsed by the publisher.
Acknowledgments
The authors would like to thank the editor and the reviewers of this paper for their valuable comments that helped improve the quality of the paper.
References
Abdalla, A. M. A., By, Zulkefli., and Abdurahman, H. N. (2014). Enhanced Oil Recovery Techniques Miscible and Immiscible Flooding. J. Appl. Sci. 14 (10), 1016–1022.
Adekunle, O. (2014). Master Thesis of the Colorado School of Mines. Experimental Approach to Investigate Minimum Miscibility Pressures in the Bakken
Ahmadi, M. A., Zendehboudi, S., and James, L. A. (2017). A Reliable Strategy to Calculate Minimum Miscibility Pressure of CO2-oil System in Miscible Gas Flooding Processes. Fuel 208, 117–126. doi:10.1016/j.fuel.2017.06.135
Alomair, O., and Iqbal, M. (2014). SPE Saudi Arabia Section Technical Symposium and Exhibition. Saudi Arabia: Al-Khobar. CO2 Minimum Miscible Pressure MMP Estimation Using Multiple Linear Regression MLR Technique. doi:10.2118/172184-MS
Alston, R. B., Kokolis, G. P., and James, C. F. (1985). CO2 Minimum Miscibility Pressure: A Correlation for Impure CO2 Streams and Live Oil Systems. Soc. Pet. Eng. J. 25 (02), 268–274. doi:10.2118/11959-pa
Azizkhani, A., and Gandomkar, A. (2020). A Novel Method for Application of Nanoparticles as Direct Asphaltene Inhibitors during Miscible CO2 Injection. J. Pet. Sci. Eng. 185, 106661. doi:10.1016/j.petrol.2019.106661
Berneti, S. M., and Varaki, M. A. (2018). Development of ε-insensitive Smooth Support Vector Regression for Predicting Minimum Miscibility Pressure in CO2 Flooding. Songklanakarin J. Sci. Technol. 40 (1), 53–59.
Birdja, Y. Y., Pérez-Gallent, E., Figueiredo, M. C., Göttle, A. J., Calle-Vallejo, F., and Koper, M. T. M. (2019). Advances and Challenges in Understanding the Electrocatalytic Conversion of Carbon Dioxide to Fuels. Nat. Energ. 4, 732–745. doi:10.1038/s41560-019-0450-y
Chen, G., Gao, H., Fu, K., Zhang, H., Liang, Z., and Tontiwachwuthikul, P. (2020). An Improved Correlation to Determine Minimum Miscibility Pressure of CO2-oil System. Green. Energ. Environ. 5, 97–104. doi:10.1016/j.gee.2018.12.003
Chen, G. Y. (2016). Simulation Prediction and Experimental Determination of CO2-oil Minimum Miscibility Pressure. Changsha, China: Doctoral dissertation of Hunan University.
Choubineh, A., Mousavi, S. R., Vafaee Ayouri, M., Ahmadinia, M., Choubineh, D., and Baghban, A. (2016). Estimation of the CO2-oil Minimum Miscibility Pressure for Enhanced Oil Recovery. Pet. Sci. Tech. 34 (22), 1847–1854. doi:10.1080/10916466.2016.1238936
Cronquist, C. (1978). Carbon Dioxide Dynamic Miscibility with Light Reservoir Oils. Proc. Tulsa: Fourth Annual US DOE Symposium, 28–30.
Dong, M., Huang, S., Dyer, S. B., and Mourits, F. M. (2001). A Comparison of CO2 Minimum Miscibility Pressure Determinations for Weyburn Crude Oil. J. Pet. Sci. Eng. 31 (1), 13–22. doi:10.1016/s0920-4105(01)00135-8
Ekundayo, J. M., and Ghedan, S. G. (2013). Minimum Miscibility Pressure Measurement with Slim Tube Apparatus - How Unique Is the Value. SPE 165966. doi:10.2118/165966-ms
Elsharkawy, A. M., Poettmann, F. H., and Christiansen, R. L. (1996). Measuring CO2 Minimum Miscibility Pressures: Slim-Tube or Rising-Bubble Method? Energy Fuels 10 (2), 443–449. doi:10.1021/ef940212f
Fathinasab, M., Ayatollahi, S., Taghikhani, V., and Shokouh, S. P. (2018). Minimum Miscibility Pressure and Interfacial Tension Measurements for N2 and CO2 Gases in Contact with W/O Emulsions for Different Temperatures and Pressures. Fuel 225, 623–631. doi:10.1016/j.fuel.2018.03.134
Fazlali, A., Nikookar, M., Agha-Aminiha, A., and Mohammadi, A. H. (2013). Prediction of Minimum Miscibility Pressure in Oil Reservoirs Using a Modified SAFT Equation of State. Fuel 108, 675–681. doi:10.1016/j.fuel.2012.12.091
Ghorbani, M., Gandomkar, A., and Montazeri, G. (2019). Describing a Strategy to Estimate the CO2-heavy Oil Minimum Miscibility Pressure Based on the Experimental Methods. Energy Sourc. A: Recovery, Utilization, Environ. Effects 41 (17), 2083–2093. doi:10.1080/15567036.2018.1549170
Ghorbani, M., Momeni, A., Safavi, S., and Gandomkar, A. (2014). Modified Vanishing Interfacial Tension (VIT) Test for CO2-oil Minimum Miscibility Pressure (MMP) Measurement. J. Nat. Gas Sci. Eng. 20, 92–98. doi:10.1016/j.jngse.2014.06.006
Glaso, O. (1985). Generalized Minimum Miscibility Pressure Correlation (Includes Associated Papers 15845 and 16287 ). Soc. Pet. Eng. J. 25 (06), 927–934. doi:10.2118/12893-pa
Gong, H., Qin, X., Shang, S., Zhu, C., Xu, L., San, Q., et al. (2020). Enhanced Shale Oil Recovery by the Huff and Puff Method Using CO2 and Cosolvent Mixed Fluids. Energy Fuels 34, 1438–1446. doi:10.1021/acs.energyfuels.9b03423
Gunde, A. C., Bera, B., and Mitra, S. K. (2010). Investigation of Water and CO2 (Carbon Dioxide) Flooding Using Micro-CT (Micro-computed Tomography) Images of Berea sandstone Core Using Finite Element Simulations. Energy 35 (12), 5209–5216. doi:10.1016/j.energy.2010.07.045
Guo, P., Hu, Y., Qin, J., Li, S., Jiao, S., Chen, F., et al. (2017). Use of Oil-Soluble Surfactant to Reduce Minimum Miscibility Pressure. Pet. Sci. Tech. 35 (4), 345–350. doi:10.1080/10916466.2016.1259630
Guo, X. Q., Rong, S. X., Yang, J. T., and Guo, T. M. (1999). The Viscosity Model Based on PR Equation of State. Acta Petrolei Sinica 20 (3), 56–61.
Han, H. S., Li, S., and Chen, X. L. (2016). Main Control Factors of Carbon Dioxide on Swelling Effect of Crude Hydrocarbon Components. Acta Petrolei Sinica 37 (3), 392–398.
Han, P. H., Jiang, Y. L., and Zhang, J. C. (1989). Prediction of CO2 Minimum Miscibility Pressure for Daqing Petroleum. Oilfield Chem. 6 (4), 309–316.
Hao, Y. M., Chen, Y. M., and Yu, H. L. (2005). Determination and Prediction of Minimum Miscibility Pressure in CO2 Flooding. Pet. Geology. Recovery Efficiency 12 (6), 64–66.
Jalilov, A. S., Li, Y., Kittrell, C., and Tour, J. M. (2017). Increased CO2 Selectivity of Asphalt-Derived Porous Carbon through Introduction of Water into Pore Space. Nat. Energ. 2, 932–938. doi:10.1038/s41560-017-0030-y
Johnson, J. P., and Pollin, J. S. (1981). Measurement and Correlation of CO2 Miscibility Pressures. SPE/DOE 9790, 269–274.
Kamari, A., Arabloo, M., Shokrollahi, A., Gharagheizi, F., and Mohammadi, A. H. (2015). Rapid Method to Estimate the Minimum Miscibility Pressure (MMP) in Live Reservoir Oil Systems during CO 2 Flooding. Fuel 153, 310–319. doi:10.1016/j.fuel.2015.02.087
Kariman Moghaddam, A., and Saeedi Dehaghani, A. H. (2017). Modeling of Asphaltene Precipitation in Calculation of Minimum Miscibility Pressure. Ind. Eng. Chem. Res. 56, 7375–7383. doi:10.1021/acs.iecr.7b00613
Kaufmann, R. K., and Connelly, C. (2020). Oil price Regimes and Their Role in price Diversions from Market Fundamentals. Nat. Energ. 5, 141–149. doi:10.1038/s41560-020-0549-1
Kolster, C., Masnadi, M. S., Krevor, S., Mac Dowell, N., and Brandt, A. R. (2017). CO2 Enhanced Oil Recovery: a Catalyst for Gigatonne-Scale Carbon Capture and Storage Deployment? Energy Environ. Sci. 10, 2594–2608. doi:10.1039/c7ee02102j
Lewis, E. (2008). Sweep Efficiency in Miscible Enhanced Oil Recovery Processes. Houston, TX: Doctoral Degree Dissertation of University of Houston.
Li, B. F., Ye, J. Q., Li, Z. M., Ji, Y. M., and Liu, W. (2016). Phase Interaction of CO2-oil-water System and its Effect on Interfacial Tension at High Temperature and High Pressure. Acta Petrolei Sinica 37 (10), 1265–1272.
Li, D., Li, X., Zhang, Y., Sun, L., and Yuan, S. (2019). Four Methods to Estimate Minimum Miscibility Pressure of CO 2 ‐Oil Based on Machine Learning. Chin. J. Chem. 37, 1271–1278. doi:10.1002/cjoc.201900337
Li, H., Qin, J., and Yang, D. (2012). An Improved CO2-Oil Minimum Miscibility Pressure Correlation for Live and Dead Crude Oils. Ind. Eng. Chem. Res. 51 (8), 3516–3523. doi:10.1021/ie202339g
Li, M. T. (2006). Laboratory Researches on Gas Injection and Porous Flow Mechanics for Low Permeability Reservoirs. Beijing, China: Doctoral Degree Dissertation of Chinese Academy of Science.
Li, X. L., Guo, P., Li, H. C., and Li, X. S. (2002). Determination of Minimum Miscibility Pressure between Formation Crude and Carbon Dioxide in Fan 124 Block of Daluhu Oilfield. Pet. Geology. Recovery Efficiency 9 (6), 62–63.
Ma, M., Liu, K., Shen, J., Kas, R., and Smith, W. A. (2018). In Situ Fabrication and Reactivation of Highly Selective and Stable Ag Catalysts for Electrochemical CO2 Conversion. ACS Energ. Lett. 3, 1301–1306. doi:10.1021/acsenergylett.8b00472
Measurement Method For Minimum Miscibility Pressure By Slim Tube Test, (2016). Petroleum and Natural Gas Industry Standard of the People's Republic of China, 6573–2016.
Mogensen, K. (2016). A Novel Protocol for Estimation of Minimum Miscibility Pressure from Slimtube Experiments. J. Pet. Sci. Eng. 146, 545–551. doi:10.1016/j.petrol.2016.07.013
Mutailipu, M., Jiang, L., Liu, X., Liu, Y., and Zhao, J. (2019). CO2 and Alkane Minimum Miscible Pressure Estimation by the Extrapolation of Interfacial Tension. Fluid Phase Equilibria 494, 103–114. doi:10.1016/j.fluid.2019.05.002
Orr, F. M., and Silva, M. K. (1987). Effect of Oil Composition on Minimum Miscibility Pressure-Part 2: Correlation. SPE 2, 479–491. doi:10.2118/14150-pa
Oschatz, M., and Antonietti, M. (2018). A Search for Selectivity to Enable CO2 Capture with Porous Adsorbents. Energ. Environ. Sci. 11, 57–70. doi:10.1039/c7ee02110k
Peng, B. Z., Luo, H., Chen, G. J., and Zhang, C. Y. (2007). Determination of the Minimum Miscibility Pressure of CO2 and Crude Oil System by Vanishing Interfacial Tension Method. Acta Petrolei Sinica 28 (3), 93–95.
Qin, J. S., Han, H. S., and Liu, X. L. (2015). Application and Enlightenment of Carbon Dioxide Flooding in the United States of America. Petrol. Explor. Dev. 42 (2), 209–216. doi:10.1016/s1876-3804(15)30010-0
Richard, A. H., and Reid, B. G. (1988). Vapor-density Measurement for Estimating Minimum Miscibility Pressure. SPE 15403.
Rostami, A., Arabloo, M., Lee, M., and Bahadori, A. (2018). Applying SVM Framework for Modeling of CO2 Solubility in Oil during CO2 Flooding. Fuel 214, 73–87. doi:10.1016/j.fuel.2017.10.121
Shao, Y., Luo, C., Deng, B.-w., Yin, B., and Yang, M.-b. (2020). Flexible Porous Silicone Rubber-Nanofiber Nanocomposites Generated by Supercritical Carbon Dioxide Foaming for Harvesting Mechanical Energy. Nano Energy 67, 104290. doi:10.1016/j.nanoen.2019.104290
Shokrollahi, A., Arabloo, M., Gharagheizi, F., and Mohammadi, A. H. (2013). Intelligent Model for Prediction of CO2 - Reservoir Oil Minimum Miscibility Pressure. Fuel 112, 375–384. doi:10.1016/j.fuel.2013.04.036
Silva, M. K., and Orr, F. M. (1987). Effect of Oil Composition on Minimum Miscibility Pressure-Part 1: Solubility of Hydrocarbons in Dense CO2. SPE 2, 468–478. doi:10.2118/14149-pa
Sun, Y. H., Lv, G. Z., Wang, Y. F., and Dong, A. Q. (2006). A Method of State Equation for Determining Minimum Miscibility Pressure of CO2. Pet. Geology. Recovery Efficiency 13 (1), 82–84.
Tatar, A., Shokrollahi, A., Mesbah, M., Rashid, S., Arabloo, M., and Bahadori, A. (2013). Implementing Radial Basis Function Networks for Modeling CO2-reservoir Oil Minimum Miscibility Pressure. J. Nat. Gas Sci. Eng. 15, 82–92. doi:10.1016/j.jngse.2013.09.008
Tian, Y., Ju, B., Yang, Y., Wang, H., Dong, Y., Liu, N., et al. (2020). Estimation of Minimum Miscibility Pressure during CO2 Flooding in Hydrocarbon Reservoirs Using an Optimized Neural Network. Energy Exploration & Exploitation 38 (6), 2485–2506. doi:10.1177/0144598720930110
Wu, S., He, J., and Li, Z. Z. (2015). Studies on the Chemical Agent System for Reducing in the Minimal Miscibility Pressure of CO2 Flooding. China Sciencepaper 10 (18), 2161–2164.
Wu, S., Li, Z., and Sarma, H. K. (2020). Influence of Confinement Effect on Recovery Mechanisms of CO2-enhanced Tight-Oil Recovery Process Considering Critical Properties Shift, Capillarity and Adsorption. Fuel 262, 116569. doi:10.1016/j.fuel.2019.116569
Yang, Z. M., Liu, X. W., and Zhang, Z. H. (2015). Physical Simulation of Staged-Fracturing Horizontal wells Using CO2 Huff and Puff in Tight Oil Reservoirs. Acta Petrolei Sinica 36 (6), 724–729.
Yang, Z., Wu, W., Dong, Z., Lin, M., Zhang, S., and Zhang, J. (2019). Reducing the Minimum Miscibility Pressure of CO2 and Crude Oil Using Alcohols. Colloids Surf. A: Physicochemical Eng. Aspects 568, 105–112. doi:10.1016/j.colsurfa.2019.02.004
Ye, A. P. (2009). Experience Formula of Determining Minimum Miscibility Pressure in CO2 Flooding. Fault-block Oil & Gas Field 16 (5), 75–76.
Ye, A. P., Guo, P., Wang, S. P., Cheng, Z. Z., and Jian, R. (2012). Determination of Minimum Miscibility Pressure for CO2 Flooding by Using PR Equation of State. Lithologic Reservoirs 24 (6), 125–128.
Yellig, W. F., and Metcalfe, R. S. (1980). Determination and Prediction of CO2 Minimum Miscibility Pressures (Includes Associated Paper 8876). J. Pet. Tech. 32 (1), 160–168. doi:10.2118/7477-pa
Yi, R. (2000). A New Method for Determining the Minimum Miscibility Pressure. Oil Drilling Prod. Tech. 22 (4), 72.
Yu, H., Lu, X., Fu, W., Wang, Y., Xu, H., Xie, Q., et al. (2020). Determination of Minimum Near Miscible Pressure Region during CO2 and Associated Gas Injection for Tight Oil Reservoir in Ordos Basin, China. Fuel 263, 116737. doi:10.1016/j.fuel.2019.116737
Yuan, H., Johns, R. T., Egwuenu, A. M., and Dindoruk, B. (2005). Improved MMP Correlation for CO2 Floods Using Analytical Theory. SPE Reservoir Eval. Eng. 8 (05), 418–425. doi:10.2118/89359-pa
Zhang, A., Fan, Z., and Zhao, L. (2020). An Investigation on Phase Behaviors and Displacement Mechanisms of Gas Injection in Gas Condensate Reservoir. Fuel 268, 117373. doi:10.1016/j.fuel.2020.117373
Zhang, C. Y., Wang, W. Q., Chen, G. J., and Ma, C. F. (2006). Interfacial Tension experiment of Oil and Water, Oil and Gas for CO2 Injected Reservoir Fluid System. J. China Univ. Pet. 30 (5), 109–112.
Zhang, G. D., Li, Z. Y., Liu, J. Y., Zhang, X., Xu, J. J., and Gao, P. (2008). Study on Determine Methods of Minimum Miscibility Pressure of Hydrocarbon Injection Miscible Flooding. Drilling Prod. Tech. 31 (3), 99–102.
Zhang, J., Zhang, H. X., Ma, L. Y., Liu, Y., and Zhang, L. (2020). Performance Evaluation and Mechanism with Different CO2 Flooding Modes in Tight Oil Reservoir with Fractures. J. Pet. Sci. Eng. 188, 106950. doi:10.1016/j.petrol.2020.106950
Zhang, K., and Gu, Y. (2015). Two Different Technical Criteria for Determining the Minimum Miscibility Pressures (MMPs) from the Slim-Tube and Coreflood Tests. Fuel 161, 146–156. doi:10.1016/j.fuel.2015.08.039
Zhou, N., Khanna, N., Feng, W., Ke, J., and Levine, M. (2018). Scenarios of Energy Efficiency and CO2 Emissions Reduction Potential in the Buildings Sector in China to Year 2050. Nat. Energ. 3, 978–984. doi:10.1038/s41560-018-0253-6
Keywords: supercritical carbon dioxide, carbon dioxide flooding, long-slim-tube displacement experiment, minimum miscible pressure, enhanced oil recovery
Citation: Fan G, Zhao Y, Zhang X, Li Y and Chen H (2021) Research on Minimum Miscible Pressure Between Crude Oil and Supercritical Carbon Dioxide System in Ultra-Low Permeability Reservoir by the Long-Slim-Tube Experiment Method. Front. Earth Sci. 9:694729. doi: 10.3389/feart.2021.694729
Received: 13 April 2021; Accepted: 08 September 2021;
Published: 07 October 2021.
Edited by:
Shengnan Chen, University of Calgary, CanadaReviewed by:
Zhezi Zhang, University of Western Australia, AustraliaLiguo Zhong, China University of Petroleum, China
Yousef Kazemzadeh, University of Tehran, Iran
Milad Arabloo, Sharif University of Technology, Iran
Copyright © 2021 Fan, Zhao, Zhang, Li and Chen. This is an open-access article distributed under the terms of the Creative Commons Attribution License (CC BY). The use, distribution or reproduction in other forums is permitted, provided the original author(s) and the copyright owner(s) are credited and that the original publication in this journal is cited, in accordance with accepted academic practice. No use, distribution or reproduction is permitted which does not comply with these terms.
*Correspondence: Yuejun Zhao, ODk2NDU0NzJAcXEuY29t