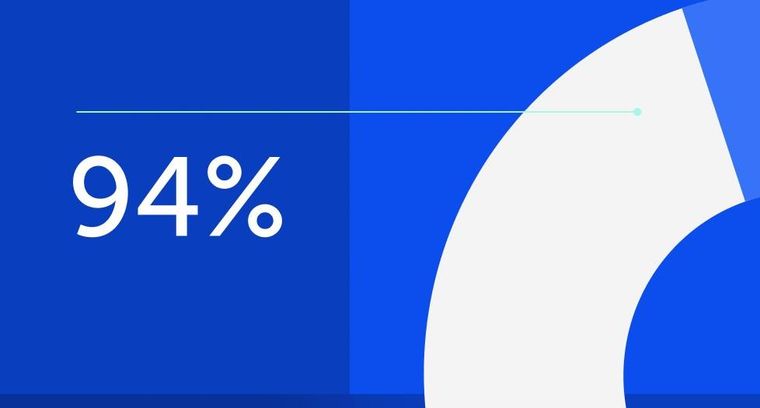
94% of researchers rate our articles as excellent or good
Learn more about the work of our research integrity team to safeguard the quality of each article we publish.
Find out more
BRIEF RESEARCH REPORT article
Front. Earth Sci., 23 August 2021
Sec. Quaternary Science, Geomorphology and Paleoenvironment
Volume 9 - 2021 | https://doi.org/10.3389/feart.2021.680180
This article is part of the Research TopicLate Quaternary Indo-Pacific Climate: Monsoons and Warm CurrentsView all 11 articles
Coastal regions of the northern South China Sea (SCS) strongly interact with the Asian monsoon circulation (AMC). Thus, variations of sea surface temperature (SST) here are newly suggested to document AMC changes in an effective manner, but additional physical parameters of oceanic conditions, probably also in relation to the AMC system, remain poorly understood. In this study, we analyzed glycerol dialkyl glycerol tetraethers (GDGTs) from a well-dated sediment core YJ, retrieved at the northern SCS coast, to further scrutinize the intrinsic response of water column to winter AMC strength. It shows that within the time frame of past ∼1,000 years, the tetraether index of lipids with 86 carbon atoms (
The Asian monsoon circulation (AMC), as triggered by large-scale thermal contrast between ocean and land, characterizes a seasonal reversal of prevailing wind directions. In the summertime, it carries an enormous amount of moisture from the Indian and Pacific Oceans toward southern and northeastern Asia, and, consequently, exerts a considerable influence over the water cycle and the terrestrial ecosystem (Wang et al., 2017; Zhang et al., 2017). In this regard, much attention has been drawn until now to explore summer AMC variability and the physical mechanism(s) from seasonal to orbital timescales (e.g., Hu et al., 2008; An et al., 2011; Liu et al., 2015; Xie et al., 2015; Cheng et al., 2016). In contrast, the winter component of the AMC itself often diverges cold-dry air from the Asian countries such as Siberia-Mongolia toward oceans, thus with little potential to deliver water vapor directly. Despite such fact, winter AMC is still of importance in transporting eolian dust and/or aerosol, and therefore in regulating the regional (and global) climate system (Maher et al., 2010; Kok et al., 2018). Combined with its impact upon the summer AMC precipitation subsequently (Bollasina et al., 2011; Li et al., 2016; Cai et al., 2019), a complete understanding of winter AMC variations at present and, if possible, before the instrumental era (after ∼1850 AD) (e.g., Wen et al., 2016; Kang et al., 2020) would provide constructive insight into their intrinsic link against both anthropogenic and natural backgrounds. Abundant analyses based on the grain size and geochemical proxies from Chinese loess sequences at available sparse sites (Stevens et al., 2007; Li and Morrill 2015), on the one hand, have indeed advanced our knowledge about this topic, but on the other hand, these paleorecords, distributed across continental interiors, rather face difficulty to draw a clear picture of winter AMC behavior, for example, its far-field effect on terrestrial ecosystem especially. For example, at Huguangyan Maar Lake, winter AMC intensity, as inferred from diatom assemblages (Wang et al., 2012) and magnetic susceptibility (Yancheva et al., 2007), respectively, presents controversial temporal features during the Holocene (since ∼11,700 years ago before present, “yr BP” hereafter).
Next to Huguangyan Maar Lake, the South China Sea (SCS) is also strongly involved into the AMC coupling process (e.g., Xie et al., 1998; Lau and Nath 2009; Wang et al., 2009; Liu and Zhu 2016) and hence well suited to fingerprint its variability. In fact, along the SCS northern coasts, sea surface temperature (SST) apparently exhibits shore-parallel gradient and intensive vertical mixing in winter, while horizontal homogenization and vertical stratification in summer (Figures 1A,B; Wang, 2007; Jing et al., 2009). Such seasonality of SST variations and their difference, for example, at both horizontal and vertical scales, are readily capable of revealing winter AMC signals across different timescales (e.g., Tian et al., 2010; Huang et al., 2011; Steinke et al., 2011; Kong, 2014a, Kong et al., 2014b). Particularly, our recent study (Zhang et al., 2019), based on a well-dated sediment core YJ, ∼200 km far away from the Pearl River delta (Figure 1), has shown extraordinary decrease (by up to ∼4°C) of alkenone SSTs and remarkable increase (by two to four orders of magnitude) of wind-borne terrigenous hopane contents during the Little Ice Age (LIA, ∼150–550 years BP), consequently demonstrating an overall intensification of winter AMC, relative to the Medieval Climate Anomaly (MCA, ∼700–1,100 years BP) and other intervals in the context of Holocene. This explanation, albeit well corroborated by a growing number of terrestrial paleorecords (e.g., Yancheva et al., 2007; Kang et al., 2020), still deserves independent evidence of oceanic conditions which, as inherently linked to SST change, would offer excellent opportunity to further illustrate the fundamental role of winter AMC variations in affecting coastal waters. To this end, the time window of last millennium covering both the LIA and MCA, two well-identified climate anomaly intervals during the late Holocene (Mann et al., 2008), is specifically focused here for a tentative attempt to examine how the northern SCS coastal conditions, for example, in terms of both salinity and thermal properties, would have responded to winter AMC change at multi-centennial timescales.
FIGURE 1. Regional setting and the site of core YJ, existing paleorecords in the northern South China Sea (black dots) and at Huguang Maar Lake (orange star) as mentioned in the main text, are plotted against long-term (1985–2006 AD) averaged January (A) and July (B) sea surface temperature (SST, color scale) from the AVHRR dataset (Casey, 2013). Chronology (C) and lithology (D) of core YJ are cited from Huang et al. (2018) and Zhang et al. (2019). Note that the core-top (C) is calculated based on 210Pb/137Cs dates, to be 2013 AD when our core YJ was retrieved.
Taking the advantage of sediment core YJ, including i) high-quality control of the chronological framework (Figures 1C,D) and ii) limited influence of the Pearl River freshwater discharge (Figure 2), we hence directly analyzed glycerol dialkyl glycerol tetraether (GDGT) lipid biomarkers on its uppermost ∼65 cm section. Together with the existing measurements of the alkenone unsaturation index (
FIGURE 2. Comparison between temperature estimates at the topmost sample based on
Geographically, sediment core YJ (112°8.08′ E, 21°31.44′ N) is raised at a water depth of ∼21 m from the inner continental shelf offshore Yangjiang city with a distance of ∼200 km to the southwest of the Pearl River estuary. This site, according to modern observations (e.g., Dunn and Ridgway 2002; Casey, 2013), characterizes prominent SST variations between ∼28.3°C in summer (June-July-August, JJA) and ∼20.9°C in winter (December–January–February, DJF), but small changes in sea surface salinity (i.e., ∼32.4 psu in JJA and ∼33.4 psu in DJF; Figure 2) due to limited influence of the Pearl River discharge. Most importantly, it is located at the coastal sector outside ∼1°C cooling effect of summer upwelling (e.g., to the east of the Pearl River delta and northeast of the Hainan Island, Figure 1B), while surface cooling here is largely determined by vertical mixing of the onsite water column in winter (Figure 1A). This site is hence well suited to examine the response of northern SCS coastal conditions to winter AMC changes, for example, by using the
The age model of this core, as already published before by Huang et al. (2018) and Zhang et al. (2019), was achieved by combining both lead (210Pb)/cesium (137Cs) and radiocarbon (14C) methods. To summarize, measurements of 13 210Pb/137Cs radionuclide activity and 18 14C dates (at Beta Analytic Inc., United States) were implemented on samples of bulk sediments above 13 cm and complete shells below this depth, respectively. These age control points were then operated within R script BACON software (version 2.2, Blaauw and Christen 2011) and the Marine 13 calibration curve (Reimer et al., 2013), using default parameters and a 252-year correction of regional reservoir age (Southon et al., 2002; Yu et al., 2010), to compute the mean age and 2σ uncertainty at 1 cm resolution. Such a chronological framework hints a possible hiatus of sedimentary deposit at the depth between ∼65 and 85 cm (Figure 1C; see details in Zhang et al., 2019). Hence, we mainly focus on the topmost 65 cm of the core YJ, roughly spanning the past ∼1,000 years, to analyze GDGT biomarkers for detecting the AMC signal across the LIA and MCA.
Core YJ was sampled continuously with a step of 1 cm down its uppermost 65 cm, which, based on our chronology as stated in Core Site and Chronology section, guaranteed a temporal resolution of ∼10–15 years per sample for the past ∼1,000 years. Afterward, bulk sediment samples (∼5 g) were freeze-dried, then grounded, and soaked to extract total lipids by solvent dichloromethane (DCM): methanol (MeOH) (9:1; v/v) in 60 ml vials, under an ultrasonic wave in the 40°C water bath for three cycles (∼15 min each). The extract was subsequently hydrolyzed with 6% KOH in MeOH to remove alkenoates and separated into three fractions via silica gel column chromatography with successive eluents of n-hexane, DCM, and MeOH, respectively. Finally, GDGTs were isolated in MeOH fraction, alkenones in DCM fraction, and n-alkanes in hexane fraction.
Analyses of MeOH fraction were conducted on high-performance liquid chromatography atmospheric pressure chemical ionization (HPLC-APCI)-mass spectrometry (e.g., Liu et al., 2013). An aliquot of the fraction was directly dried under N2, and then redissolved in hexane: isopropanol (99:1; v/v) and filtered after mixing with a known amount of C46 internal standard (Huguet et al., 2006). Selected ion monitoring (SIM), which targets specific mass numbers for GDGT components (membrane lipids biosynthesized as multiple homolog series of isoprenoid or methyl-branched isomers, termed isoprenoid-GDGTs, and branched-GDGTs, respectively, see detailed description in Schouten et al., 2013), was used to enhance the detection sensitivity. Quantification was carried out by integrating the peak area of [M + H]+ ions in the extracted ion chromatogram and comparing with the C46 internal standard. We then calculated the ACE, BIT, and
Throughout the past millennium, ACE values appear to be relatively high during the LIA, especially at its onset (centered around ∼500 years BP), as compared to the MCA (Figure 3A). In contrast, the BIT index generally experiences a gradual declining trend from ∼0.3 during the MCA (and the earlier epochs, marked by a possible hiatus in sediment accumulation and hence not shown here) toward ∼0.15 in the recent years (Figure 3B). Unlike these two modes,
FIGURE 3. GDGT proxies of sediment core YJ during the last millennium, for example, (A) ratio of archaeol to caldarchaeol (ACE) (higher values downward), (B) the branched and isoprenoid tetraether (BIT), (C)
FIGURE 4. Organic geochemical proxies of core YJ over the last millennium, including (A)
Recent studies have shown that the possible source of brGDGTs, for example, terrigenous originated (e.g., soil) or in situ synthesized (mainly at subsurface waters), is critical to determine the BIT index and thus its proper explanation (Weijers et al., 2014; Xiao et al., 2016; Wang et al., 2021). For example, more subsurface production of brGDGTs in the Qiongzhou Strait is suggested to be responsible for higher BIT values (∼0.4–0.6), which, as a result, reflect enhanced stratification of the onsite water column and thus change in summer AMC strength (Wang et al., 2021). At our study site YJ, BIT values, primarily subjected to crenarchaeol (one major component of isoGDGTs) rather than brGDGT variations (Supplementary Figure 1), also imply water column stratification. A set of field surveys, based on collection of both the sediment trap and core-top samples, show that, at the transition zones between the Pearl River estuary and the SCS northern coast, the bloom of autotrophic ammonia-oxidizing Thaumarchaeota, main producers of isoGDGTs with limited brGDGTs, tends to preferably occur under the hydrological conditions in the coldest months, like low light levels (e.g., Zhang et al., 2013; Wang et al., 2015; Jia et al., 2017) and less stratified water. Meanwhile, at normal marine settings, including those on the continental shelf, light and redox conditions can also yield redistribution of Euryarchaeota/Archaea community, leading to stratification of archaeal membrane lipids (with relatively high archaeol in subsurface waters, Turich et al., 2007; Weijers et al., 2014; Xiao et al., 2016; Zhu et al., 2016). In this sense, the coeval variations of isoGDGTs and archaeol abundance in our particular case may cause opposite temporal patterns of BIT and ACE indices (Supplementary Figures 1, 2). This fact, in contrary to a recent study presented by Wang et al. (2021) who have applied the concomitant increase in these two proxies to represent enhanced stratification of the northern SCS coastal water, thereby calls for other interpretation(s) to reconcile competing patterns of our BIT and ACE proxies (Figures 3A,B). Considering the small variations of BIT values and brGDGTs (Supplementary Figure 1), we thus interpret relatively low BIT ratios during the LIA as increased production of the ubiquitous Thaumarchaeota, relative to other Euryarchaeota/Archaea. Besides, it is also worth stressing that despite similar features of changes in crenarchaeol and caldarchaeol (GDGT-0) (Supplementary Figures 1, 2), two most abundant components of isoGDGTs, the observed ACE values here may still primarily respond to Euryarchaeota/Archaea community changes, therefore no longer being an indicator of water column stratification (e.g., Wang et al., 2021).
Based on the results of previous studies (Turich and Freeman, 2011; He et al., 2020), the ACE index might represent salinity if it mainly responds to Euryarchaeota/Archaea community changes. This prerequisite indeed exists in our case, because one could apparently see a major control of Euryarchaeota/Archaea on the ACE record (Supplementary Figure 2). Due to the different characteristics of BIT and ACE records that strongly exclude the latter as a tracer of stratification (Wang et al., 2021), we instead assume ACE to manifest salinity. As such, multi-centennial–scale variations in our ACE record, as depicted in Figure 4G, suggest increased (decreased) salinity of the onsite water column across the LIA (MCA) (Turich and Freeman, 2011). Together with the inference of the available
The physical mechanism for our inference is further substantiated by the BIT index and
In our case, downcore
Since
We used a sediment core YJ, collected from the northern SCS coast, to analyze GDGT lipid biomarkers during the past millennium. These proxies, together with published alkenone (
The original contributions presented in the study are included in the article/Supplementary Material; further inquiries can be directed to the corresponding author.
Conceptualization: ZL; investigation: KZ, CH, DK, YH, HW, and ZX; formal analysis: YZ and ZL; resources: WL, GW, and ZL; funding acquisition: WL and ZL; writing: YZ and ZL led the writing with intellectual contributions from all coauthors.
This work was supported by the National Key Research and Development Program of China (2016YFA0601204) and Hong Kong RGC Grant 17325516.
The authors declare that the research was conducted in the absence of any commercial or financial relationships that could be construed as a potential conflict of interest.
The reviewer (YH) declared a shared affiliation with several of the authors, (HW, WL, ZX), to the handling editor at time of review.
All claims expressed in this article are solely those of the authors and do not necessarily represent those of their affiliated organizations, or those of the publisher, the editors and the reviewers. Any product that may be evaluated in this article, or claim that may be made by its manufacturer, is not guaranteed or endorsed by the publisher.
We sincerely thank guest editors for inviting contribution to this special issue and anonymous referees for providing insightful comments to improve our manuscript.
The Supplementary Material for this article can be found online at: https://www.frontiersin.org/articles/10.3389/feart.2021.680180/full#supplementary-material
An, Z., Clemens, S. C., Shen, J., Qiang, X., Jin, Z., Sun, Y., et al. (2011). Glacial-interglacial Indian Summer Monsoon Dynamics. Science 333, 719–723. doi:10.1126/science.1203752
Blaauw, M., and Christen, J. A. (2011). Flexible Paleoclimate Age-Depth Models Using an Autoregressive Gamma Process. Bayesian Anal. 6, 457–474. doi:10.1214/ba/1339616472
Bollasina, M. A., Ming, Y., and Ramaswamy, V. (2011). Anthropogenic Aerosols and the Weakening of the South Asian Summer Monsoon. Science 334, 502–505. doi:10.1126/science.1204994
Cai, W., Wu, L., Lengaigne, M., Li, T., McGregor, S., Kug, J.-S., et al. (2019). Pantropical Climate Interactions. Science 363, eaav4236. doi:10.1126/science.aav4236
Casey, K. (2013). US DOC/NOAA/NESDIS > National Oceanographic Data Center, AVHRR Pathfinder Version 5.0 Global 4km Sea Surface Temperature (SST) Cloud-Screened Monthly Climatologies for 1985-2006 (NODC Accession 0110657). NOAA: National Oceanographic Data Center.
Cheng, H., Edwards, R. L., Sinha, A., Spötl, C., Yi, L., Chen, S., et al. (2016). The Asian Monsoon over the Past 640,000 Years and Ice Age Terminations. Nature 534 (7609), 640–646. doi:10.1038/nature18591
Dunn, J. R., and Ridgway, K. R. (2002). Mapping Ocean Properties in Regions of Complex Topography. Deep Sea Res. Oceanographic Res. Pap. 49 (3), 591–604. doi:10.1016/s0967-0637(01)00069-3
Dykoski, C., Edwards, R., Cheng, H., Yuan, D., Cai, Y., Zhang, M., et al. (2005). A High-Resolution, Absolute-Dated Holocene and Deglacial Asian Monsoon Record from Dongge Cave, China. Earth Planet. Sci. Lett. 233, 71–86. doi:10.1016/j.epsl.2005.01.036
He, Y., Wang, H., Meng, B., Liu, H., Zhou, A., Song, M., et al. (2020). Appraisal of Alkenone- and Archaeal Ether-Based Salinity Indicators in Mid-latitude Asian Lakes. Earth Planet. Sci. Lett. 538, 116236. doi:10.1016/j.epsl.2020.116236
Hopmans, E. C., Weijers, J. W. H., Schefuss, E., Herfort, L., Sinninghe Damsté, J. S., and Schouten, S. (2004). A Novel Proxy for Terrestrial Organic Matter in Sediments Based on Branched and Isoprenoid Tetraether Lipids. Earth Planet. Sci. Lett. 224, 107–116. doi:10.1016/j.epsl.2004.05.012
Hu, C., Henderson, G. M., Huang, J., Xie, S., Sun, Y., and Johnson, K. R. (2008). Quantification of Holocene Asian Monsoon Rainfall from Spatially Separated Cave Records. Earth Planet. Sci. Lett. 266, 221–232. doi:10.1016/j.epsl.2007.10.015
Huang, C., Zeng, T., Ye, F., Xie, L., Wang, Z., Wei, G., et al. (2018). Natural and Anthropogenic Impacts on Environmental Changes over the Past 7500 Years Based on the Multi-Proxy Study of Shelf Sediments in the Northern South China Sea. Quat. Sci. Rev. 197, 35–48. doi:10.1016/j.quascirev.2018.08.005
Huang, E., Tian, J., and Steinke, S. (2011). Millennial-scale Dynamics of the winter Cold Tongue in the Southern South China Sea over the Past 26 Ka and the East Asian winter Monsoon. Quat. Res. 75, 196–204. doi:10.1016/j.yqres.2010.08.014
Huguet, C., Hopmans, E. C., Febo-Ayala, W., Thompson, D. H., Sinninghe Damsté, J. S., and Schouten, S. (2006). An Improved Method to Determine the Absolute Abundance of Glycerol Dibiphytanyl Glycerol Tetraether Lipids. Org. Geochem. 37, 1036–1041. doi:10.1016/j.orggeochem.2006.05.008
Jia, G., Wang, X., Guo, W., and Dong, L. (2017). Seasonal Distribution of Archaeal Lipids in Surface Water and its Constraint on Their Sources and the TEX86 Temperature Proxy in Sediments of the South China Sea. J. Geophys. Res. Biogeosci. 122, 592–606. doi:10.1002/2016jg003732
Jing, Z.-y., Qi, Y.-q., Hua, Z.-l., and Zhang, H. (2009). Numerical Study on the Summer Upwelling System in the Northern continental Shelf of the South China Sea. Continental Shelf Res. 29, 467–478. doi:10.1016/j.csr.2008.11.008
Kang, S., Du, J., Wang, N., Dong, J., Wang, D., Wang, X., et al. (2020). Early Holocene Weakening and Mid- to Late Holocene Strengthening of the East Asian winter Monsoon. Geology 48 (11), 1043–1047. doi:10.1130/g47621.1
Kim, J.-H., van der Meer, J., Schouten, S., Helmke, P., Willmott, V., Sangiorgi, F., et al. (2010). New Indices and Calibrations Derived from the Distribution of Crenarchaeal Isoprenoid Tetraether Lipids: Implications for Past Sea Surface Temperature Reconstructions. Geochimica et Cosmochimica Acta 74, 4639–4654. doi:10.1016/j.gca.2010.05.027
Kok, J. F., Ward, D. S., Mahowald, N. M., and Evan, A. T. (2018). Global and Regional Importance of the Direct Dust-Climate Feedback. Nat. Commun. 9, 241. doi:10.1038/s41467-017-02620-y
Kong, D. (2014a). Climatic Changes in the Northern South China Sea since the Last Glacial Maximum. PhD Thesis. Hong Kong: University of Hong Kong. doi:10.5353/th_b5312314
Kong, D., Wei, G., Chen, M.-T., Peng, S., and Liu, Z. (2017). Northern South China Sea SST Changes over the Last Two Millennia and Possible Linkage with Solar Irradiance. Quat. Int. 459, 29–34. doi:10.1016/j.quaint.2017.10.001
Kong, D., Zong, Y., Jia, G., Wei, G., Chen, M.-T., and Liu, Z. (2014b). The Development of Late Holocene Coastal Cooling in the Northern South China Sea. Quat. Int. 349, 300–307. doi:10.1016/j.quaint.2013.08.055
Lau, N.-C., and Nath, M. J. (2009). A Model Investigation of the Role of Air-Sea Interaction in the Climatological Evolution and ENSO-Related Variability of the Summer Monsoon over the South China Sea and Western North Pacific. J. Clim. 22, 4771–4792. doi:10.1175/2009jcli2758.1
Lee, W.-M., Poon, K.-C., Kong, D., Sewell, R. J., Zong, Y., Zhang, Y., et al. (2019). Summer Monsoon-Induced Upwelling Dominated Coastal Sea Surface Temperature Variations in the Northern South China Sea over the Last Two Millennia. The Holocene 29 (4), 691–698. doi:10.1177/0959683618824715
Li, Y., and Morrill, C. (2015). A Holocene East Asian winter Monsoon Record at the Southern Edge of the Gobi Desert and its Comparison with a Transient Simulation. Clim. Dyn. 45, 1219–1234. doi:10.1007/s00382-014-2372-5
Li, Z., Lau, W. K. M., Ramanathan, V., Wu, G., Ding, Y., Manoj, M. G., et al. (2016). Aerosol and Monsoon Climate Interactions over Asia. Rev. Geophys. 54, 866–929. doi:10.1002/2015rg000500
Liu, B., and Zhu, C. (2016). A Possible Precursor of the South China Sea Summer Monsoon Onset: Effect of the South Asian High. Geophys. Res. Lett. 43, 11072–11079. doi:10.1002/2016gl071083
Liu, J., Chen, J., Zhang, X., Li, Y., Rao, Z., and Chen, F. (2015). Holocene East Asian Summer Monsoon Records in Northern China and Their Inconsistency with Chinese Stalagmite δ18O Records. Earth-Science Rev. 148, 194–208. doi:10.1016/j.earscirev.2015.06.004
Liu, W., Wang, H., Zhang, C. L., Liu, Z., and He, Y. (2013). Distribution of Glycerol Dialkyl Glycerol Tetraether Lipids along an Altitudinal Transect on Mt. Xiangpi, NE Qinghai-Tibetan Plateau, China. Org. Geochem. 57, 76–83. doi:10.1016/j.orggeochem.2013.01.011
Maher, B. A., Prospero, J. M., Mackie, D., Gaiero, D., Hesse, P. P., and Balkanski, Y. (2010). Global Connections between Aeolian Dust, Climate and Ocean Biogeochemistry at the Present Day and at the Last Glacial Maximum. Earth-Science Rev. 99, 61–97. doi:10.1016/j.earscirev.2009.12.001
Mann, M. E., Zhang, Z., Hughes, M. K., Bradley, R. S., Miller, S. K., Rutherford, S., et al. (2008). Proxy-based Reconstructions of Hemispheric and Global Surface Temperature Variations over the Past Two Millennia. Proc. Natl. Acad. Sci. 105 (36), 13252–13257. doi:10.1073/pnas.0805721105
Prahl, F. G., Muehlhausen, L. A., and Zahnle, D. L. (1988). Further Evaluation of Long-Chain Alkenones as Indicators of Paleoceanographic Conditions. Geochimica et Cosmochimica Acta 52, 2303–2310. doi:10.1016/0016-7037(88)90132-9
Reimer, P. J., Bard, E., Bayliss, A., Beck, J. W., Blackwell, P. G., Ramsey, C. B., et al. (2013). IntCal13 and Marine13 Radiocarbon Age Calibration Curves 0-50,000 Years Cal BP. Radiocarbon 55, 1869–1887. doi:10.2458/azu_js_rc.55.16947
Schouten, S., Hopmans, E. C., Schefuss, E., and Sinninghe Damsté, J. S. (2002). Distributional Variations in marine Crenarchaeotal Membrane Lipids: a New Tool for Reconstructing Ancient Sea Water Temperatures? Earth Planet. Sci. Lett. 204, 265–274. doi:10.1016/s0012-821x(02)00979-2
Schouten, S., Hopmans, E. C., and Sinninghe Damsté, J. S. (2013). The Organic Geochemistry of Glycerol Dialkyl Glycerol Tetraether Lipids: a Review. Org. Geochem. 54, 19–61. doi:10.1016/j.orggeochem.2012.09.006
Southon, J., Kashgarian, M., Fontugne, M., Metivier, B., and W-S Yim, W. (2002). Marine Reservoir Corrections for the Indian Ocean and Southeast Asia. Radiocarbon 44, 167–180. doi:10.1017/s0033822200064778
Steinke, S., Glatz, C., Mohtadi, M., Groeneveld, J., Li, Q., and Jian, Z. (2011). Past Dynamics of the East Asian Monsoon: No Inverse Behaviour between the Summer and winter Monsoon during the Holocene. Glob. Planet. Change 78, 170–177. doi:10.1016/j.gloplacha.2011.06.006
Stevens, T., Thomas, D., Armitage, S., Lunn, H., and Lu, H. (2007). Reinterpreting Climate Proxy Records from Late Quaternary Chinese Loess: A Detailed OSL Investigation. Earth-Science Rev. 80, 111–136. doi:10.1016/j.earscirev.2006.09.001
Tian, J., Huang, E., and Pak, D. K. (2010). East Asian winter Monsoon Variability over the Last Glacial Cycle: Insights from a Latitudinal Sea-Surface Temperature Gradient across the South China Sea. Palaeogeogr. Palaeoclimatol. Palaeoecol. 292, 319–324. doi:10.1016/j.palaeo.2010.04.005
Turich, C., and Freeman, K. (2011). Archaeal Lipids Record Paleosalinity in Hypersaline Systems. Org. Geochem. 42, 1147–1157.
Turich, C., Freeman, K., Freeman, K. H., Bruns, M. A., Conte, M., Jones, A. D., et al. (2007). Lipids of marine Archaea: Patterns and Provenance in the Water-Column and Sediments. Geochimica et Cosmochimica Acta 71, 3272–3291. doi:10.1016/j.gca.2007.04.013
Wang, B., Huang, F., Wu, Z., Yang, J., Fu, X., and Kikuchi, K. (2009). Multi-scale Climate Variability of the South China Sea Monsoon: A Review. Dyn. Atmospheres Oceans 47, 15–37. doi:10.1016/j.dynatmoce.2008.09.004
Wang, H., Liu, W., Zhang, C. L., Jiang, H., Dong, H., Lu, H., et al. (2013). Assessing the Ratio of Archaeol to Caldarchaeol as a Salinity Proxy in highland Lakes on the Northeastern Qinghai-Tibetan Plateau. Org. Geochem. 54, 69–77. doi:10.1016/j.orggeochem.2012.09.011
Wang, J.-X., Wei, Y., Wang, P., Hong, Y., and Zhang, C. L. (2015). Unusually Low TEX86 Values in the Transitional Zone between Pearl River Estuary and Coastal South China Sea: Impact of Changing Archaeal Community Composition. Chem. Geology. 402, 18–29. doi:10.1016/j.chemgeo.2015.03.002
Wang, L., Li, J., Lu, H., Gu, Z., Rioual, P., Hao, Q., et al. (2012). The East Asian winter Monsoon over the Last 15,000 Years: its Links to High-Latitudes and Tropical Climate Systems and Complex Correlation to the Summer Monsoon. Quat. Sci. Rev. 32, 131–142. doi:10.1016/j.quascirev.2011.11.003
Wang, M., Wang, H., Zhu, Z., Yang, X., Zhang, K., Zhang, Y., et al. (2021). Late Miocene-Pliocene Asian Summer Monsoon Variability Linked to Both Tropical Pacific Temperature and Walker Circulation. Earth Planet. Sci. Lett. 561 (116823), 116823. doi:10.1016/j.epsl.2021.116823
Wang, P. X., Wang, B., Cheng, H., Fasullo, J., Guo, Z., Kiefer, T., et al. (2017). The Global Monsoon across Time Scales: Mechanisms and Outstanding Issues. Earth-Science Rev. 174, 84–121. doi:10.1016/j.earscirev.2017.07.006
Wang, Y., Cheng, H., Edwards, R., He, Y., Kong, X., An, Z., et al. (2005). The Holocene Asian Monsoon: Links to Solar Changes and North Atlantic Climate. Science 308, 854–857. doi:10.1126/science.1106296
Wei, B., Jia, G., Hefter, J., Kang, M., Park, E., Wang, S., et al. (2020). Comparison of the U37K′, LDI, TEX86H, and RI-OH Temperature Proxies in Sediments from the Northern Shelf of the South China Sea. Biogeosciences 17, 4489–4508. doi:10.5194/bg-17-4489-2020
Weijers, J. W. H., Schefuß, E., Kim, J.-H., Sinninghe Damsté, J. S., and Schouten, S. (2014). Constraints on the Sources of Branched Tetraether Membrane Lipids in Distal marine Sediments. Org. Geochem. 72, 14–22. doi:10.1016/j.orggeochem.2014.04.011
Wen, X., Liu, Z., Wang, S., Cheng, J., and Zhu, J. (2016). Correlation and Anti-correlation of the East Asian Summer and winter Monsoons during the Last 21,000 Years. Nat. Commun. 7. doi:10.1038/ncomms11999
W. Wang (2007). Study on the Coastal Geomorphological Sedimentation of the South China Sea (Guangzhou, China: Guangdong Economy Publishing House), 344 in Chinese.
Xiao, W., Wang, Y., Zhou, S., Hu, L., Yang, H., and Xu, Y. (2016). Ubiquitous Production of Branched Glycerol Dialkyl Glycerol Tetraethers (brGDGTs) in Global marine Environments: a New Source Indicator for brGDGTs. Biogeosciences 13, 5883–5894. doi:10.5194/bg-13-5883-2016
Xie, A., Chung, Y., Liu, X., and Ye, Q. (1998). The Interannual Variations of Summer Monsoon Onset Over the South China Sea. Theor. Appl. Climatol. 59, 201–213.
Xie, S.-P., Deser, C., Vecchi, G. A., Collins, M., Delworth, T. L., Hall, A., et al. (2015). Towards Predictive Understanding of Regional Climate Change. Nat. Clim Change 5, 921–930. doi:10.1038/nclimate2689
Xing, L., Sachs, J. P., Gao, W., Tao, S., Zhao, X., Li, L., et al. (2015). TEX 86 Paleothermometer as an Indication of Bottom Water Temperature in the Yellow Sea. Org. Geochem. 86, 19–31. doi:10.1016/j.orggeochem.2015.05.007
Yamamoto, M., Kishizaki, M., Oba, T., and Kawahata, H. (2013). Intense winter Cooling of the Surface Water in the Northern Okinawa Trough during the Last Glacial Period. J. Asian Earth Sci. 69, 86–92. doi:10.1016/j.jseaes.2012.06.011
Yancheva, G., Nowaczyk, N. R., Mingram, J., Dulski, P., Schettler, G., Negendank, J. F. W., et al. (2007). Influence of the Intertropical Convergence Zone on the East Asian Monsoon. Nature 445, 74–77. doi:10.1038/nature05431
Yu, K., Hua, Q., Zhao, J.-x., Hodge, E., Fink, D., and Barbetti, M. (2010). Holocene marine14C Reservoir Age Variability: Evidence from230Th-Dated Corals in the South China Sea. Paleoceanography 25. doi:10.1029/2009PA001831
Zhang, C., Tang, Q., Chen, D., Li, L., Liu, X., and Cui, H. (2017). Tracing Changes in Atmospheric Moisture Supply to the Drying Southwest China. Atmos. Chem. Phys. 17, 10383–10393. doi:10.5194/acp-17-10383-2017
Zhang, J., Bai, Y., Xu, S., Lei, F., and Jia, G. (2013). Alkenone and Tetraether Lipids Reflect Different Seasonal Seawater Temperatures in the Coastal Northern South China Sea. Org. Geochem. 58, 115–120. doi:10.1016/j.orggeochem.2013.02.012
Zhang, P., Cheng, H., Edwards, R. L., Chen, F., Wang, Y., Yang, X., et al. (2008). A Test of Climate, Sun, and Culture Relationships from an 1810-year Chinese Cave Record. Science 322, 940–942. doi:10.1126/science.1163965
Zhang, Y., Zhu, K., Huang, C., Kong, D., He, Y., Wang, H., et al. (2019). Asian winter Monsoon Imprint on Holocene SST Changes at the Northern Coast of the South China Sea. Geophys. Res. Lett. 46, 13363–13370. doi:10.1029/2019gl085617
Zhu, C., Wakeham, S. G., Elling, F. J., Basse, A., Mollenhauer, G., Versteegh, G. J. M., et al. (2016). Stratification of Archaeal Membrane Lipids in the Ocean and Implications for Adaptation and Chemotaxonomy of Planktonic Archaea. Environ. Microbiol. 18, 4324–4336. doi:10.1111/1462-2920.13289
Keywords: South China Sea, coastal conditions, GDGTs, last millennium, Asian winter monsoon
Citation: Zhang Y, Zhu K, Huang C, Kong D, He Y, Wang H, Liu W, Xie Z, Wei G and Liu Z (2021) Asian Winter Monsoon Imprint on the Water Column Structure at the Northern South China Sea Coast. Front. Earth Sci. 9:680180. doi: 10.3389/feart.2021.680180
Received: 13 March 2021; Accepted: 12 July 2021;
Published: 23 August 2021.
Edited by:
Shengfa Liu, Ministry of Natural Resources, ChinaReviewed by:
Kefu Yu, Guangxi University, ChinaCopyright © 2021 Zhang, Zhu, Huang, Kong, He, Wang, Liu, Xie, Wei and Liu. This is an open-access article distributed under the terms of the Creative Commons Attribution License (CC BY). The use, distribution or reproduction in other forums is permitted, provided the original author(s) and the copyright owner(s) are credited and that the original publication in this journal is cited, in accordance with accepted academic practice. No use, distribution or reproduction is permitted which does not comply with these terms.
*Correspondence: Yancheng Zhang, emhhbmd5Y2g5OUBtYWlsLnN5c3UuZWR1LmNu
Disclaimer: All claims expressed in this article are solely those of the authors and do not necessarily represent those of their affiliated organizations, or those of the publisher, the editors and the reviewers. Any product that may be evaluated in this article or claim that may be made by its manufacturer is not guaranteed or endorsed by the publisher.
Research integrity at Frontiers
Learn more about the work of our research integrity team to safeguard the quality of each article we publish.