- 1CONICET-Laboratorio de Anatomía Comparada y Evolución de Los Vertebrados, Museo Argentino de Ciencias Naturales “Bernardino Rivadavia”, Buenos Aires, Argentina
- 2Fundación de Historia Natural “Félix de Azara”, Universidad Maimónides, Buenos Aires, Argentina
In 1976 John Ostrom published an enlightening paper about the anatomical transformations in the shoulder girdle and forelimb elements along the origin of birds. Most of his ideas were based on comparing Archaeopteryx lithographica with the extant New World vulture Cathartes aura. Ostrom offered innovative ideas about range of movements and function of wing elements in the basal bird Archaeopteryx. Further, he explored anatomical transformations that may have occurred at early stages of the evolution of flight and established several hypothetical steps toward the acquisition of flapping flight in modern birds. Since then, however, our understanding of paravian diversity and anatomy has increased dramatically. Based on novel information derived from recent experiments, and currently available anatomical evidence of basal paravians, the present paper aims to review some important topics on pectoral girdle anatomy related to flight origins. Further, a brief analysis of pectoral girdle osteology and myology of the extant paleognath Rhea americana is also included with the aim to test whether Ostrom’s ideas still remain valid under this new context, based on available phylogenetic and anatomical frameworks.
Introduction
The origin and early evolution of birds and flight are one of the most debated topics on evolutionary biology. By the 1970 and 1980 decades the work of several authors, but most notably the contributions by John Ostrom (1928–2005), were essential to the building of the modern theory of bird origins (Witmer, 2002). In 1976, Ostrom published a detailed comparison between the early bird Archaeopteryx lithographica and the extant New World vulture Cathartes aura, entitled “Some hypothetical anatomical stages in the evolution of avian flight”. In this contribution he speculated about the anatomical transformations and evolutionary steps in the shoulder girdle and forelimb during avian evolution, taking Archaeopteryx as the “starting point” of this sequence of evolutionary changes. Ostrom offered ideas about range of movements and function of limb elements in Archaeopteryx. He gave special importance to the modifications of the dinosaurian “biceps tubercle” (homologous with the modern avian acrocoracoidal process) and its direct relation with the course of one of the main wing elevators, the m. supracoracoideus. Ostrom clearly demonstrated the absence in Archaeopteryx of such anatomical and functional adaptations, considering this taxon as representative of a “pre-flying stage”. Within this interpretive framework Ostrom also recognized a series of “hypothetical stages” in the transformation of the pectoral girdle between the ancestral stage, as represented by Archaeopteryx, and the highly modified girdle of living flying birds.
More than four decades have passed since Ostrom’s seminal contribution, and a lot of novel anatomical, biomechanical, and phylogenetic information on extinct and extant theropods has become available. Further, in the last decades the number of bird-like theropods and basal birds has dramatically increased (Witmer, 1991, Witmer, 2002; Chiappe and Vargas, 2003; Xu et al., 2014; Brusatte et al., 2015; Cau et al., 2017).
The aim of the present paper is to emphasize the anatomical similarities of some key features of the pectoral girdle in basal paravians, basal avialans and extant flightless paleognaths. Specifically, comparisons are detailed with the Greater Rhea (Rhea americana), and we provide a brief description of its musculature with the goal of comparing it with the inferred musculature of basal paravians. The shoulder girdle of large secondarily flightless paleognaths like R. americana may have partially converged on the condition of primarily flightless theropods, and indeed our comparison finds features, like the locations of muscle attachment sites and gross morphology of the bones, that can easily be interpreted in the light of the Greater Rhea. With all this information at hand we briefly re-analyze here the “hypothetical stages” in the acquisition of bird flight as originally proposed by Ostrom.
Material and Methods
Phylogenetic Framework
We follow the phylogenetic framework proposed by Agnolín and Novas (2013); (see also Agnolín and Novas, 2011; Agnolín et al., 2019; Motta et al., 2020) in which Deinonychosauria is interpreted as a non-monophyletic group, and Dromaeosauridae is formed by Velociraptor, Dromaeosaurus, Deinonychus, and taxa more closely related to them than to Microraptor, Unenlagia, Rahonavis or Archaeopteryx. Microraptoria and Unenlagiidae are interpreted to form successively closer relatives of the clade Avialae (Figure 1). The clade Scansoriopterygidae is excluded from Paraves following Agnolín and Novas (2013); (see also O’Connor and Sullivan, 2014). The paravian clade including Dromaeosauridae and Avialae, but not Troodontidae, is termed Eumaniraptora (see discussion in Padian et al., 1999). Our interpretative scheme about the phylogenetic relationships among basal paravians departs from others (e.g., Norell and Clarke, 2001; Makovicky et al., 2005; Xu and Kim, 2011; Turner et al., 2012; Gianechini et al., 2017; Lefèvre et al., 2017; Pei et al., 2017; Hartman et al., 2019; Cau, 2020; Pei et al., 2020) in that we propose that Troodontidae, Dromaeosauridae, Microraptoria, Unenlagiidae and Anchiornithidae form successively closer relatives of Avialae. We choose to follow this scheme, because a detailed overview of other phylogenetic analyses (e.g., TWIG) resulted in poorly resolved phylogenetic relationships among derived paravians (Agnolín et al., 2019).
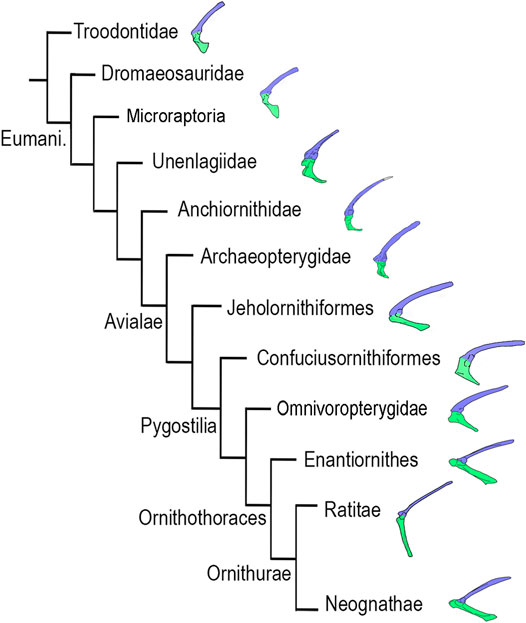
FIGURE 1. Phylogenetic framework following Agnolín and Novas (2013); see also Agnolín and Novas, 2011; Agnolín et al., 2019; Motta et al., 2020) indicating the left scapulocoracoid in lateral view. Abbreviations. Euman, Eumaniraptora. Blue, scapula; green, coracoid. Not to scale.
In the present contribution we do not include the basal bird clade Jinguofortidae, as represented by Jinguofortis and Chongmingia (Wang et al., 2016, Wang et al., 2018), because the reconstructions and interpretations on the pectoral girdle in both genera are problematic. This is due to the incomplete and bidimensional preservation of the specimens.
We also do not include detailed analysis of the scapulocoracoid morphology of Jeholornis. Since its discovery, the coracoid of Jeholornis has been usually described as robust and strut-like (Zhou and Zhang, 2003a), but the deformation of the available specimens makes recognition of several anatomical features very difficult. Due to the differential preservation of each available coracoid (Wang et al., 2020), the recognition of anatomical details is not certain.
The pectoral girdle of flightless paleognaths was considered the best living analog to compare with the anatomy of extinct paravians (Feduccia, 1986; Novas et al., 2020). We will pay special attention to those morphological traits of flightless paleognaths that look closer to early diverging paravians than to flying avians. We are aware, however, that these birds are secondarily flightless, being descendants of flying avian ancestors (Yonezawa et al., 2017; Sackton et al., 2019), and that flightless paleognaths are sharply distinguished from the remaining avians (as well as from extinct early diverging paravians) in several anatomical traits. It is clear to us that, regardless of which pennaraptoran phylogenetic tree is followed, the osteological similarities between Rhea and basal paravians (e.g., Buitreraptor, Archaeopteryx) are analogous, and not strict homologies. In any case, the Rhea shoulder girdle (see Novas et al., 2020) joins models previously studied by several authors (Dial et al., 1991; Baier et al., 2007), and we believe that we cannot ignore the information yielded by the anatomy of this bird and its possible implications on the origin of flight.
Nomenclature
The anatomical nomenclature follows Baumel et al. (1993) and the abbreviations used in the text are as follows: m (musculus), mm (musculi) and p (pars). Because the “coracoid tuberosity” or “biceps tubercle” in theropods is considered by most authors as homologous with the modern avian “acrocoracoid process” (Ostrom, 1976), we only employ the latter term throughout the text.
We follow Jasinoski et al. (2006) in that the m. coracobrachialis brevis is a homologue to the m. coracobrachialis cranialis.
We use the form Unenlagiidae (Agnolín and Novas, 2011, 2013; Motta et al., 2020) instead of the form Unenlagiinae that has been employed by several authors (Makovicky et al., 2005; Turner et al., 2007, Turner et al., 2012; Brusatte et al., 2015; Cau et al., 2017; Rauhut et al., 2018; Pei et al., 2020). The name Dromaeosauridae is instead restricted to the paravian Laurasian clade including Dromaeosaurus but not Passer and Troodon (see Agnolín et al., 2019). The Late Cretaceous Malagasy taxon Rahonavis ostromi (Forster et al., 1998) is here considered outside of Dromaeosauridae, following recent interpretations (e.g., O’Connor et al., 2011; Agnolín and Novas, 2013; Godefroit et al., 2013; Lefèvre et al., 2017; Novas et al., 2018; Motta et al., 2020).
Scapulocoracoid Position
The discussion on the position of the pectoral girdle in extinct theropods has not been continued since Senter (2006). Despite the lack of agreement, and the sake of clarity and for comparative purposes, we opt to place paravian scapulocoracoids with the scapular blade oriented in an almost horizontal position, with a slight ventral inclination of its anterior extremity, in a way similar to what is seen in living flying birds.
Data Collection
The specimens of Rhea americana were not stored for more than six months, and only those muscles and body parts that were in good condition were considered for the study. Before the dissection, each specimen was defrosted in the fridge (4–5°C). We dissected the wing muscles of both sides. The wing muscle data was available in two specimens (MACN-Or 9428, 9583). Following a classical anatomical approach of describing wing musculature, we removed each muscle and documented the origin and insertion sites of principal muscles of the shoulder girdle that originated on the acrocoracoid process, as well as their general features and appearance. We manipulated the muscles in order to observe the movement ratios in the wing.
Institutional Abbreviations
IVPP, Institute of Vertebrate Paleontology and Paleoanthropology, Beijing, China; MACN-Or, Museo Argentino de Ciencias Naturales Bernardino Rivadavia, sección Ornitología, Buenos Aires, Argentina; MPCA, Museo Provincial Carlos Ameghino, Cipolletti, Río Negro, Argentina; MPCN-PV, Museo Provincial de Ciencias Naturales, General Roca, Río Negro, Argentina.
Anatomical Abbreviations
Acr pr, acromion process; acroc pr, acrocoracoid process; acroc-acr lig, acrocoraco-acromial ligament; BB, m. biceps brachii; CBB, m. coracobrachialis brevis; CBCr, m. coracobrachialis cranialis; corac f, coracoid foramen; fur, furcula; gl, glenoid; h, humerus; P, m. pectoralis; post cor pr, posterior coracoid process; pro pr, procoracoid process; SC, m. supracoracoideus; sc canal, supracoracoid canal; trioss canal, triosseal canal.
Results
Elsewhere (Novas et al., 2020) we preliminarily analyzed the pectoral girdle and forearm of extant non-tinamid paleognaths, emphasizing some similarities with early diverging paravians (i.e., Archaeopteryx, Buitreraptor). Main similarities concern the subvertical orientation of the glenoid cavity and poor development of acrocoracoid process, which may reflect similar postural activities of the forelimbs not only in paravians, but also in non-avian pennaraptorans. We present here a summary description of the main muscle masses of the shoulder girdle of the Greater Rhea (Rhea americana), specifically those muscles that originate on the coracoid and acrocoracoid (i.e., mm. pectoralis, supracoracoideus, biceps brachii, and coracobrachialis cranialis), also constituting the most important muscles for the movement of the wing.
Myology of the Shoulder Girdle of the Greater Rhea (Rhea americana)
M. pectoralis (P, Figure 2). In Rhea this muscle has only one belly as it also occurs in some specimens of Struthio and most neognaths (Jasinoski et al., 2006). This muscle has a fleshy origin on the anterolateral surface of coracoid. In accordance with Beddard (1898) and Lowe (1928), the origin of the m. pectoralis in Rhea does not reach the sternum or the ribs, a condition contrasting with the notable expansion of the m. pectoralis in tinamous and neognaths (Baumel et al., 1993; Jasinoski et al., 2006; Suzuki et al., 2014).
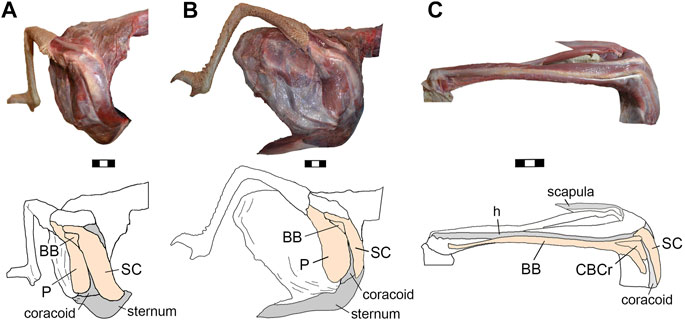
FIGURE 2. Rhea americana, photographs and schematic drawings of selected muscles of right shoulder girdle in anterolateral (A) and lateral (B) views, and proximal humerus in lateral (C) view. Abbreviations. h, humerus; BB, m. biceps brachii; CBCr, m. coracobrachialis cranialis; P, m. pectoralis; SC, m. supracoracoideus. The bones were shaded in gray and the muscles in orange. Scale bars 3 cm.
The function of the m. pectoralis in Rhea is to protract and abduct the arm, when the wing is unfolded. This function is similar to that in Struthio (Jasinoski et al., 2006), but it is different from neognaths in which the m. pectoralis is the main adductor and depressor of the arm (Raikow, 1985; Dial, 1992; Baumel et al., 1993). Ostrom (1976) indicated that the m. pectoralis in “Carinatae” birds is the main adductor/depressor of the humerus during flapping flight, and the same function was inferred for Archaeopteryx. Based on information afforded by Rhea, we believe that m. pectoralis in Archaeopteryx also played a role of protractor and adductor of the arm.
M. supracoracoideus (SC, Figure 2). In Rhea this muscle is fleshy and fan-shaped, originating on the medial portion of the anterior surface of the coracoid, on the processus procoracoideus, on the coracoid membrane [homologous with the sternocoracoclavicular membrane of neognaths, according to Baumel et al. (1993) and Livezey and Zusi (2007)], and on the small cranial area of the sternum (close to the sternal-coracoidal articulation).
In specimen MACN-Or 9428 of Rhea americana the origin area of the m. supracoracoideus is smaller than in other individuals and lacks contact with the sternum, suggesting that this muscle has considerable intraspecific variation. In other paleognaths, like Struthio and Dromaius, the area of origin of the m. supracoracoideus only includes the coracoid (Jasinoski et al., 2006; Maxwell and Larsson, 2007), while in extant flying birds the origin is variable but large, usually including the ventral surface of the sternum, the lateral and basal part of the sternal carina, the lateral margin of the furcula, the sternocoracoclavicular membrane, the medial portion of the coracoid and the processus procoracoideus (Baumel et al., 1993; Jasinoski et al., 2006; Suzuki et al., 2014; Lo Coco et al., 2020).
In Rhea, the m. supracoracoideus runs through the supracoracoideus canal and inserts as a tendon on the dorsal and proximal end of the humerus. In Struthio, the insertion of the m. supracoracoideus instead is more anteriorly located on the humerus (Jasinoski et al., 2006) while in neognaths the tendon of the m. supracoracoideus inserts into the proximal end of the crista deltopectoralis (Baumel et al., 1993).
The m. supracoracoideus of Rhea protracts the humerus and barely elevates it (Novas et al., 2020). In Struthio, this muscle protracts the humerus (Jasinoski et al., 2006), while in neognaths and tinamids this muscle is the main elevator of this bone (Raikow, 1985; Dial, 1992; Suzuki et al., 2014). The rotation of the humerus, as occurs in neognathan birds (Dial et al., 1991), has not been observed in Rhea. Ostrom (1976) originally inferred for Archaeopteryx that the m. supracoracoideus produced abduction, a conclusion that is in agreement with the protractor role of this muscle in extant non-tinamid paleognaths.
M. biceps brachii (BB, Figure 2). In Rhea, this is a fusiform muscle that has two well-differentiated bellies from its origin (Lowe, 1928). One belly is tendinous and originates on the processus acrocoracoideus of the coracoid, while the other belly is fleshy and originates on the lateral edge of the coracoid (Lowe, 1928). In most neognaths the m. biceps brachii also has two bellies with double origins, although in Rhea the double origin is present on the coracoid but not on the proximal end of the humerus, like other extant non-tinamid paleognaths (George and Berger, 1966; McGowan, 1982; Jasinoski et al., 2006).
In Rhea, the two bellies of the m. biceps brachii become fused close to the proximal humerus, and have a strong contact with the skin and the crista deltopectoralis. The insertion is double and tendinous into the proximal end of the radius and the ulna, as in most of the neognaths and tinamids (McGowan, 1982; Baumel et al., 1993; Picasso and Mosto, 2018; Lo Coco et al., 2020) but different than Struthio (Jasinoski et al., 2006) and Dromaius (Maxwell and Larsson, 2007).
The function of the m. biceps brachii in Rhea is to flex the forearm as in Struthio and neognaths (Baumel et al., 1993; Jasinoski et al., 2006). Nevertheless, in Rhea, this muscle also abducts and protracts the arm, similar to Struthio in which the m. biceps brachii protracts the arm to a small degree (Jasinoski et al., 2006). Ostrom (1976) hypothesized for Archaeopteryx that the m. biceps brachii was a primary flexor of the forearm, but based on extant non-tinamid paleognaths we also hypothesize that it acted to abduct and protract the arm.
M. coracobrachialis. In Rhea, only a single belly (i.e., p. cranialis, CBCr, Figure 2) of m. coracobrachialis is present. This is in congruence with observations in other flightless paleognaths (McGowan, 1982; Jasinoski et al., 2006), but contrasting with neognaths and tinamids in which both p. cranialis and p. caudalis are present (Hudson et al., 1972; Baumel et al., 1993; Jasinoski et al., 2006; Suzuki et al., 2014). The m. coracobrachialis cranialis is a fusiform muscle that originates from the subglenoid fossa (lateral subtriangular area of the omal end of the scapulacoracoid, ventral to the glenoid cavity) and associated tendinous tissue. The insertion is located in a wide area between the crista deltopectoralis and the crista bicipitalis of the humerus, similar to the condition present in Struthio (Jasinoski et al., 2006). This contrasts with the smaller insertion area observed in neognaths (Baumel et al., 1993; Picasso and Mosto, 2018; Lo Coco et al., 2020).
The function of the m. coracobrachialis cranialis in Rhea is to protract and adduct the humerus. In Struthio and in neognaths the m. coracobrachialis cranialis protracts the humerus (Jasinoski et al., 2006). Ostrom (1976) interpreted the m. coracobrachialis p. cranialis in Archaeopteryx as a primary extensor of the humerus, a role that is in agreement with the protractor role documented in extant non-tinamid paleognaths.
The m. deltoideus minor was not observed during the dissection of Rhea, in congruence with other non-tinamid paleognaths (Beddard, 1898; Lowe, 1928; McGowan, 1982; Jasinoski et al., 2006). Because this muscle is also absent in crocodiles (Jasinoski et al., 2006) but present in tinamids (Suzuki et al., 2014) and neognaths (Baumel et al., 1993; Picasso and Mosto, 2018; Lo Coco et al., 2020), we assume that it was probably not present in basal paravians and basal birds, and thus, it is not included in present analysis.
Discussion
Ostrom paid attention to the strong anatomical differences between Archaeopteryx and the skillful flyers among extant birds. Moreover, no recognized taxon was known in the 1970s to be evolutionarily intermediate between Archaeopteryx and birds of modern aspect (e.g., Ichthyornis). In the last 40 years, but especially the last 20, the diversity of birds phylogenetically intermediate between Archaeopteryx and living birds has considerably expanded, with recognition of many different and speciose new clades (e.g., Jinguofortidae, Jeholornithiformes, Confuciusornithidae, Omnivoropterygidae, Enantiornithes). Also important is the discovery of different non-avian paravian taxa which closely resemble the pectoral morphology of Archaeopteryx (i.e., Buitreraptor, Sinornithosaurus, Microraptor), thus indicating that any functional conclusion arrived for Archaeopteryx may be also extrapolated for the remaining basal eumaniraptorans. A third aspect is that the information on postural activities of the wings in flightless paleognath birds has to be taken into account now to discuss the anatomical transformations that may have occurred at early stages of the evolution of flight.
Current knowledge of Mesozoic bird anatomy invites a review of Ostrom’s ideas on the evolutionary changes that have occurred in the pectoral girdle from the time of Archaeopteryx to extant birds.
Origin of the M. Supracoracoideus Pulley
The m. supracoracoideus is one of the most important elevator muscles of the wing in extant flying birds (Dial et al., 1991; Mayr, 2017). This muscle arises from the dorsal part of the sternal carina and adjacent body of the sternum. The tendon of the m. supracoracoideus passes dorsally through the triosseal canal and inserts on the proximal portion of the dorsomedial surface of humerus (Lowe, 1928). This deflection of the m. supracoracoideus in extant flying birds is due to the well-developed acrocoracoid process, which projects antero-dorso-medially and is located above the level of the glenoid cavity.
Ostrom (1976) proposed that in Archaeopteryx the m. supracoracoideus must have depressed and adducted laterally the wing because the biceps tubercle (= acrocoracoid process) was much less developed and located well below the level of the glenoid cavity. His main conclusion was that in Archaeopteryx the m. supracoracoideus acted as a wing depressor, different from its elevator role in living flying birds. Based on comparisons between Archaeopteryx and Cathartes, Ostrom masterfully hypothesized that the evolutionary elongation of the acrocoracoid process progressively changed the path of the tendon of the m. supracoracoideus, modifying its role from a depressor to an elevator of the wing.
We concur with Ostrom in the main conclusions, but some considerations have to be made regarding with the origin of this pulley for the m. supracoracoideus, as well as the evolutionary trends of the coracoid in general and the acrocoracoid process in particular, in birds that are closer to the crown than Archaeopteryx.
First, the shape, orientation and relative size of the acrocoracoid process in Archaeopteryx do not represent an avialan autapomorphy, but a similarly constructed acrocoracoid process is also present in other paravians such as Buitreraptor, Saurornitholestes and Microraptor. In other words, Archaeopteryx does not represent an evolutionary “starting point” for this condition, because a closely similar coracoid evolved prior to avian origins. The kind of acrocoracoid process present in Archaeopteryx and closely related taxa (Buitreraptor, Saurornitholestes and Microraptor), albeit small with respect to more derived birds, is notably larger when compared with more basal theropods (e.g., ornithomimosaurs, tyrannosauroids, therizinosauroids; Agnolín and Novas, 2013). That means that at the base of Paraves the m. supracoracoideus anteriorly protracted the humerus, which was somewhat different from the action performed in early diverging archosaurs (i.e., crocodiles) and retained in basal coelurosaurs, in which the humerus is anteroventrally protracted. In other words, the common ancestor of Paraves (or possibly, Pennaraptora), evolved a large m. supracoracoideus with an anteriorly protractive function. This indicates that basal paravians (or more inclusively, pennaraptorans) attained a cranially increased range of movements of their forelimbs compared with non-pennaraptoran theropods. Despite this increase in protracting capabilities (presumably allowing reaching items in front of the animal), we are unable to identify any function of the m. supracoracoideus for flying activities.
Manipulation of m. supracoracoideus in Rhea (Lowe, 1928; Novas et al., 2020) shows that its contraction protracts the humerus, thus supporting Ostrom’s interpretation for Archaeopteryx and early diverging avialans. Ostrom considered Archaeopteryx as representing a pre-flight stage in bird evolution, and the inferred action of the m. supracoracoideus is in accordance with his view.
The interesting point here is that three successive clades of early avialans with well-developed wings, Jeholornithiformes, Confuciusornithidae and Omnivoropterygidae, show a coracoid shape that does not fit with the sequence of progressive transformations expected in Ostrom’s hypothetical stages: on the contrary, the coracoids of omnivoropterygids are subquadrangular and notably wide, and those of jeholornithiforms are proportionally much longer than confuciusornithids and omnivoropterygids. Curiously, jeholornithiforms show a coracoidal shape that superficially resembles the strut-like condition of the phylogenetically distant ornithothoracine birds. This indicates that coracoidal evolution and shape at the base of avian tree was more diverse than thought.
Also interesting is the fact that in Jeholornithiformes, Confuciusornithidae and Omnivoropterygidae (Zhou and Zhang, 2003a, Zhou and Zhang, 2003b; Chiappe and Meng, 2016) the acrocoracoid process, albeit more prominent than in Archaeopteryx and other basal paravians, still retained a lower position with respect to the glenoid cavity, indicating that the path of the m. supracoracoideus was not modified substantially with respect to more basal paravians, but still acted as a humeral protractor. In birds more derived than Archaeopteryx, Confuciusornis and Sapeornis, the coracoid instead became strut-like and the acrocoracoidal process became even more elongate and related with a very deep supracoracoid canal (Mayr, 2017).
Another aspect to be considered concerns the formation of the triosseal foramen and its function. In Buitreraptor (MPCA-245; MPCN-PV-598) and Archaeopteryx the cranial surface of the coracoid, between the acrocoracoid process and the medial coracoidal margin, exhibits a deep transverse concavity (Novas et al., 2018). This dorsoventral groove is suggestive of a deep canalization of the belly of the m. supracoracoideus. The cranial concavity on the coracoid of Buitreraptor is in concert with similar modifications on the proximal end of the scapula, from which the acromial process also projects cranially, thus defining a trough on the lateral surface of the proximal scapula (Novas et al., 2018). This trough on the cranial surface of the scapulocoracoid of Buitreraptor may represent an initial stage of the formation of the supracoracoidal canal of more derived birds. This suggests a change in anatomy and function of the m. supracoracoideus at the base of Eumaniraptora (in contrast, in more basal coelurosaurs the outer surface of the scapulocoracoid is flattened or slightly convex, and such a canal is absent).
In Rhea the furcula is lacking and consequently a triosseal canal is absent. However, a supracoracoid canal does exist, being delimited by the acromion process, the acrocoracoid process, and a strong acrocoraco-acromial ligament which connects the acrocoracoidal and acromial processes. The acrocoraco-acromial ligament forms a bridge under which the m. supracoracoideus slides. This ligament is also present in modern flying birds, but it is cranially covered by the omal end of the furcula. Ultimately, the foramen through which the tendon of the m. supracoracoideus slides is basically the same (and homologous) in both flying and flightless living birds, with the only distinction of the presence or absence of a bony enclosure by the furcula. We suspect that an acrocoraco-acromial ligament was already present in basal Eumaniraptora, in congruence with the presence of a deep supracoracoidal groove on the cranial surface of the coracoid and the proximal end of the scapula.
Rhea shows a path of the m. supracoracoideus tendon that is not present in flying birds. In dorsal view, the coracoid of Rhea exhibits a “coracoidal plate” between the glenoid and the acromion process (Figure 3E, portion of the coracoid in red color). This “coracoidal plate” delimits the path of the supracoracoid tendon. In this way, the m. supracoracoideus originates at the anterior surface of the coracoid and passes through the supracoracoid canal (homologous to the “triosseal canal”), and finally inserts dorsally into the proximal humerus. This results in movements of the wing that are different from flying birds, because when the m. supracoracoideus is contracted, it produces an anterior shift of the wing, contrasting with the dorsal movement of the wing in flying birds where the triosseal canal is well developed. In extant volant birds the triosseal canal opens ventrally and medially to the glenoid (Figure 3F); due to the absence of the “coracoidal plate” the path of the m. supracoracoideus runs dorsally (Ostrom, 1976), and not posteriorly as occurs in Rhea.
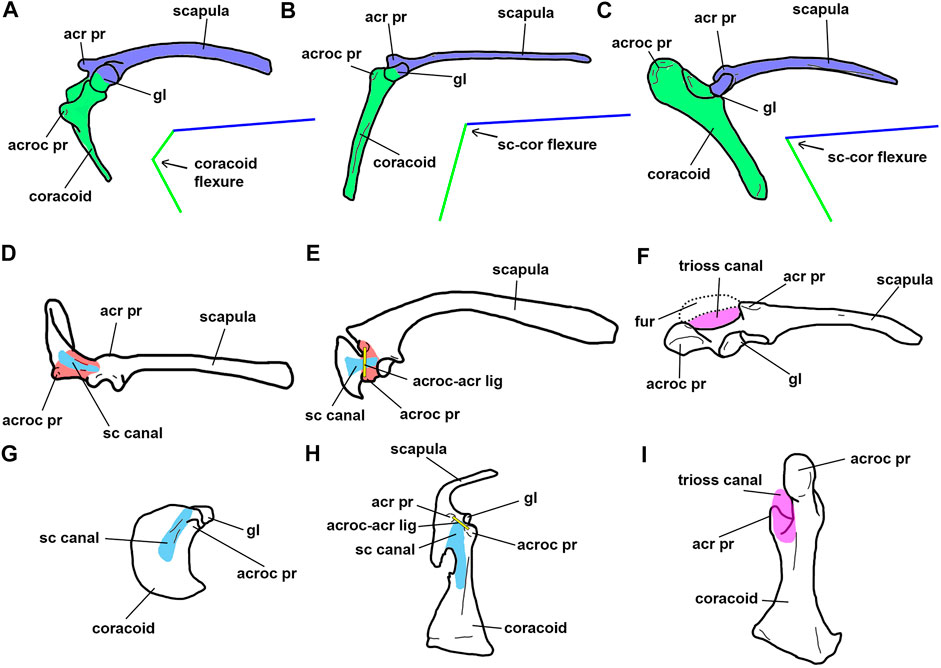
FIGURE 3. Selected paravian scapulocoracoid in lateral (A–C), dorsal (D–F) and anterior (G–I) views. (A, D, G): Archaeopteryx lithographica; (B, E, H), Rhea americana; (C, F, I), Vultur gryphus. Abbreviations. acr pr, acromion process; acroc pr, acrocoracoid process; acroc-acr lig, acrocoraco-acromial ligament; fur, furcula; gl, glenoid; sc canal, supracoracoid canal; trioss canal, triosseal canal. Blue, scapula; red, coracoidal plate; green, coracoid; light blue, supracoracoid canal; yellow, acrocoraco-acromial ligament; violet, triosseal canal. Not to scale.
Previous observations lead to the following considerations: the triosseal canal, in the way it is defined by the bony encounter of furcula, scapular acromium and coracoidal acrocoracoid, was absent in Buitreraptor and Archaeopteryx. Nevertheless, the trough present on the cranial surface of the coracoid and the proximal end of the scapula suggests that: 1) an acrocoraco-acromial ligament connecting the acrocoracoidal and acromial processes was probably present in basal Eumaniraptora; and 2) the m. supracoracoideus changed its course running through the supracoracoid canal.
As a corollary of these observations, we must conclude that the change in course of the m. supracoracoideus in early paravian evolution did not require the presence of a proper triosseal foramen. It is feasible that as the supracoracoidal sulcus became deeper and its orientation changed from anterodorsal (basal paravians and basal birds) to medial (extant flying birds), the function of the m. supracoracoideus changed from protractor to protractor-elevator of the humerus.
In Enantiornithes a true triosseal canal and foramen are not complete. As indicated by reconstructions of the pectoral girdle (Martin, 1995; Mayr, 2017), in Enantiornithes the omal extremity of the furcula articulated with the tip of the acromion of the scapula but the coracoid lacked a bony contact with the furcula. This lack of contact was due to the straight and cranially projected acrocoracoid, different from the hook-like, anteromedially curved acrocoracoid process of extant flying birds. This hook-like process creates a bony contact with the furcula, thus participating in the cranial enclosure of the triosseal canal. It is unknown whether non-carinate birds with a strut-like coracoid possessed a cartilaginous medial prolongation of the simple acrocoracoid process for contacting the furcula.
The basal euornithines Apsaravis and Patagopteryx (Chiappe, 2002; Clarke and Norell, 2002) also show a similar groove on the proximal end of the scapula, suggesting a similar path of the supracoracoid pulley as that present in enantiornithine birds. In this way, Enantiornithes and probably the basalmost euornithines had flight mechanics that were different from extant birds (Mayr, 2017).
Classically, the triosseal canal was interpreted as a pulley that deflected the tendon of the m. supracoracoideus, such that this muscle became the primary humeral elevator/supinator in the upstroke. However, the path of the supracoracoideus tendon is already deflected in basal paravians by virtue of the presence of a supracoracoidal canal. Despite the fact that the triosseal canal of modern birds is the result of the cranial closure of this sulcus, the strong deepening of the canal is the main responsible factor for the change in orientation of the supracoracoideus tendon in neognaths (Figures 3E–H). Mechanically, the supracoracoidal sulcus acted almost in the same way with or without the cranial closure by the furcula.
It is possible that the change in the orientation of the m. supracoracoideus is mostly the result of the deepening and orientation of the supracoracoid canal, in a way that the muscle tendon changed from tangential to perpendicular to the margin of the glenoid cavity. In fact, it seems that the change in pull direction of this muscle is related with the inclination of the greater axis of the glenoid: in forms with a glenoid cavity that is aligned with the main axis of the scapula, the pull direction of the m. supracoracoideus is tangential to the glenoid; in contrast, in those birds in which the glenoid is subhorizontal, the pull of the m. supracoracoideus is perpendicular to the long axis of the glenoid. This change in orientation of the glenoid cavity apparently occurred at the Ornithothoraces node, and resulted in the main difference in humeral movements from mainly protraction in non-ornithothoracine birds to mainly elevation in ornithothoracines (Agnolín et al., 2019; Novas et al., 2020).
In Ornithothoraces the dorsal elongation of the acrocoracoid increased the origin of the mm. biceps brachii, coracobrachialis cranialis and, probably, deltoideus minor. In addition, the acrocoracoid process also offered attachment for the ligamentum acrocoracohumerale, which connects the coracoid with the transverse sulcus of the proximal end of the humerus and replaced the plesiomorphic muscle-based system which prevented humeral ventral dislocation (Baier et al., 2007).
In sum, it is here hypothesized that the action of the m. supracoracoideus was at first modified with the definition of the supracoracoidal canal (in early paravians), and that the capacity for rapid rotation (elevation and supination) of the humerus was acquired later in avian history (probably at the base of Ornithothoraces) with the rotation of the glenoid cavity involving the increase in size of the m. supracoracoideus (as expressed by the formation of a keeled sternum; Mayr, 2017).
Changes in Glenoid Orientation
The morphology and orientation of the glenoid cavity apparently has utmost importance in the range of humeral (and then, wing) movements (Jenkins, 1993; Baier et al., 2007; Agnolín et al., 2019). Nevertheless, the effects of the inclination of the greater glenoid axis on wing posture remain poorly explored.
Basal paravians like Saurornitholestes, Buitreraptor, Anchiornis, and Archaeopteryx share a similar orientation of the scapular glenoid. One of the main distinctions from flying euornithines is the orientation of the glenoid cavity and the inclination of its major axis, being laterally faced and with a horizontal major axis in flying forms, and laterally facing and with a subvertical major axis in basal paravians. The glenoid cavity in Rhea, as in other flightless paleognaths, faces laterally, and its main axis is subvertically oriented (MACN-Or 9428, 9583). In Rhea the subvertical orientation of the major axis of the glenoid almost restricts humeral abduction-adduction movements and allows movements in an anteroposterior direction to be more prevalent (Agnolín et al., 2019; Novas et al., 2020). In flightless paleognaths the position of the pectoral girdle “frame” is closer to that of a basal archosaur, rather than a flying euornithine. Morphological similarities among living non-tinamid paleognaths and basal paravians suggest resemblance in forelimb posture and range of movements (Figure 4).
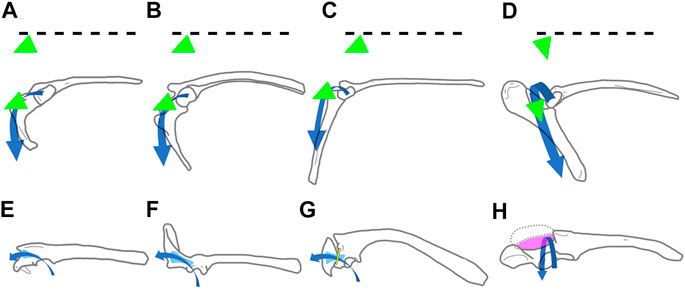
FIGURE 4. Right scapulocoracoid of selected paravians in lateral (A–D), and dorsal (E–H) views. (A, E), Buitreraptor gonzalezorum; (B, F), Archaeopteryx lithographica; (C, G), Rhea americana; (D, H), Vultur gryphus. The broken line indicates the main antero-posterior axis of the scapulacoracoid. Blue, path of the SC; green, first and main action vector of the SC; light blue, supracoracoid canal; yellow, acrocoraco-acromial ligament; violet, triosseal canal. Not to scale.
In most theropods the scapular and coracoidal glenoid lips are prominent (Bakker et al., 1992). In basal theropods as Syntarsus and Allosaurus (Madsen, 1976; Raath, 1978) the scapular lip occupies a much more laterally salient position than the coracoidal lip, whereas in paravians as Bambiraptor and Deinonychus (Burnham et al., 2000; Ostrom, 1969, 1974) both lips are equally projected outwards. In contrast, in unenlagiids (e.g., Buitreraptor) and neognath birds (flying or flightless) the coracoidal lip laterally surpasses the level of the scapular one. Additionally, in basal birds (e.g., Archaeopteryx, Confuciusornis) the glenoid facet retained the lateral orientation seen in more basal paravians (e.g., Buitreraptor), and both sections (scapular and coracoidal) are subequal in size and aligned on the same dorsoventral plane. This lack of a “twisted” glenoid (that is, without a large coracoidal surface that faces dorsally) suggests that basal birds had not yet attained the same amplitude of forelimb movements, nor the humeral trajectory over the glenoid, as that present in living flying birds (see Novas et al., 2020).
In Rhea the acrocoracoid process is poorly developed and separated from the glenoid cavity. A similar condition is present in most basal paravians (e.g., Bambiraptor, Buitreraptor, Archaeopteryx) and basal birds (Sapeornis, Confuciusornis), in which the acrocoracoid process is differentiated and well separated from the glenoid cavity. On the contrary, in extant flying birds the acrocoracoid process is strongly expanded and is adjacent to the glenoid cavity.
The rotation of the glenoid (from subvertical to horizontal) constitutes the main responsible factor for the range of humeral movements, thus accompanying the direction of the pull of the m. supracoracoideus. The dorsal elongation of the acrocoracoid was accompanied by the change in the orientation of the glenoid cavity, of which the cranial (coracoidal) half surpassed the size of the caudal (scapular) half, adopting a horizontal position, and came to be dorsally and slightly posteriorly oriented.
We hypothesize that the strong development of the acrocoracoid process may not be directly correlated with the change in the orientation of the m. supracoracoideus, but instead the full rotation of the glenoid cavity had a main role in the change of direction of the m. supracoracoideus.
Scapulocoracoid Flexure
In living volant birds the scapula and the coracoid articulate with each other, forming an acute angle (Figure 3C). This scapulocoracoid flexure is variable within a single taxon during ontogeny, and the angle between the main shafts of coracoid and scapula decreases during growth (Heers and Dial, 2012). Contrary, a higher angle (obtuse) between scapula and coracoid is observed in extant and extinct flightless birds, including non-tinamid paleognaths (Figure 3B; Olson, 1973; Livezey, 1989; Agnolín et al., 2019).
In basal paravians (e.g., Deinonychus, Buitreraptor, Archaeopteryx) the scapulocoracoid is remarkably “L”-shaped when viewed from the side (Paul, 2002). However, in the case of Buitreraptor and Archaeopteryx this flexure is almost restricted to the coracoid (Figure 3A). In these cases, it is observed that at the level of the acrocoracoid process the coracoid has a strong posterior tilting resulting in an angle of more than 90°. A similar condition is present in basal birds, such as Sapeornis and Confuciusornis, although this angle is even more acute (Zhou and Zhang, 2003b; Wang et al., 2018). The condition of Jeholornis is difficult to discern, because the studied specimens of this avialan (IVPP V13274; IVPP V13553) preserve this bone in dorsal or ventral views, thus presence or absence of such a flexure is difficult to ascertain. In contrast to basal paravians, and more derived birds including Euornithes (e.g., Rhea, Vultur) and Enantiornithes (Walker and Dyke, 2009), the coracoid tends to be strut-like and lacks any sign of flexure (Figures 3B,C).
It is worth mention that the existence of an angled scapulocoracoid in basal paravians does not mean that the m. supracoracoideus acted exactly in the same way as in Euornithes. In basal paravians such as Buitreraptor, Archaeopteryx, and Rhea, the supracoracoidal canal does not reach the medial margin of the glenoid cavity because is not deep enough and the “coracoidal plate” is still relatively large (Figures 3D,E). In this case the supracoracoideus tendon runs anteriorly on the “coracoidal plate” before inserting into the humerus, and therefore its main action is to protract the humerus. In contrast, in extant flying birds and probably Enantiornithes, the supracoracoideus tendon attaches to the humerus from the medial aspect of the glenoid due to the development of the triosseal canal (Figures 3F,I). This part of the tendon of the m. supracoracoideus creates a pulley effect and elevates the humerus.
In whichever way the scapulocoracoid flexure is acquired (in the case of basal paravians due to coracoid tilting, and in the case of Enantiornithes and flying Euornithes by the increased angle between the scapular and coracoid main axes; Figure 3C), this results in an increase of the lever arm of the m. supracoracoideus. In this way, such an increase is not only related to the development of the acrocoracoidal process, but also the result of the scapulocoracoidal angle.
Variability of Coracoid Morphology Among Basal Paravians
It is important to remark that both coracoid shape and the development of the acrocoracoid process are not uniform among basal paravians. The kind present in Archaeopteryx is closely similar to that of Microraptor, Sinornithosaurus, and Buitreraptor, but in Bambiraptor (Burnham et al., 2000) the coracoid is notably low and transversely wide, the acrocoracoid process is proportionally smaller than in the above mentioned taxa, and the cranial surface of the coracoid is convex, thus lacking a supracoracoid sulcus as in Buitreraptor, for example. This condition described for Bambiraptor is also found in all known troodontids, and possibly also occurs in the basal paravian Anchiornis (Figures 5, 6).
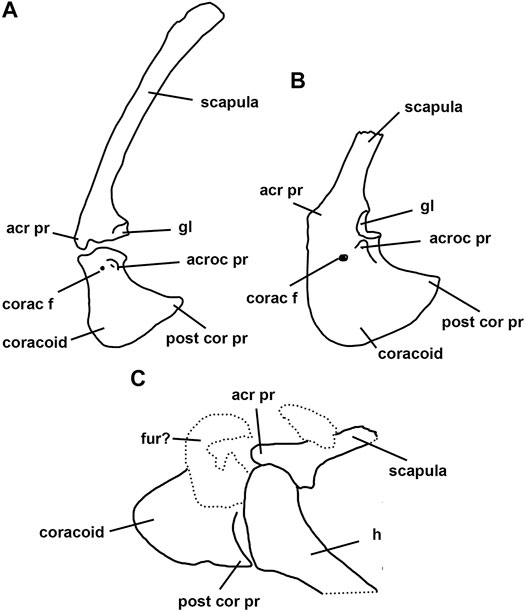
FIGURE 5. Right scapulocoracoid of selected troodontids in anterodorsal view. (A), Gobivenator mongoliensis; (B), Sinornithoides youngi; (C), Jianianhualong tengi [(A) modified from Tsuihiji et al., 2014; (B) modified from Currie and Dong, 2001; (C) modified from Xu et al., 2017). Abbreviations. acr pr, acromion process; acroc pr, acrocoracoid process; corac f, coracoid foramen; fur? furcula? gl, glenoid; h, humerus; post cor pr, posterior coracoid process. Not to scale.
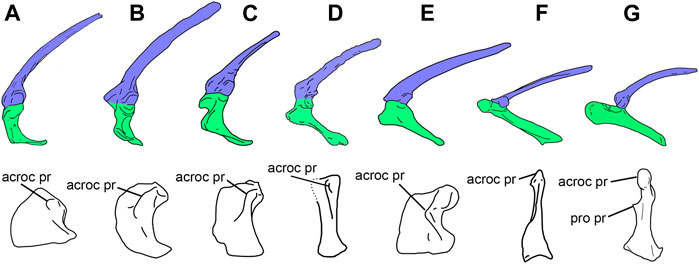
FIGURE 6. Selected scapulocoracoids and coracoids from the left side in lateral (top) and anterolateral (bottom) views, showing variation in shape among basal paravians. (A), Anchiornis huxleyi (Pei et al., 2017); (B), Archaeopteryx lithographica (London specimen); (C), Buitreraptor gonzalezorum; (D), Confuciusornis sanctus (Chiappe and Meng, 2016; the anterolateral view is distorted in the original specimen, and thus, its reconstruction is uncertain); (E), Sapeornis chaoyangensis; (F), Enantiornithine bird (modified from Mayr, 2017); (G), Vultur gryphus. Scapula shaded in blue, coracoid shaded in green. Abbreviations. acroc pr, acrocoracoid process; pro pr, procoracoid process. Not to scale.
Remarkably, the troodontid scapulocoracoid lacks most derived features observed in other paravians. The scapulocoracoid of troodontids is completely preserved in a number of taxa, including Gobivenator, Sinornithoides, Mei, and Jianianhualong (Russell and Dong, 1993; Currie and Dong, 2001; Xu et al., 2004; 2017; Tsuihiji et al., 2014; Figure 5).
The coracoid of Gobivenator lacks the coracoid flexure described above as typical for basal paravians (e.g., Buitreraptor, Bambiraptor, Archaeopteryx). In this way, the coracoid in troodontids is nearly straight or slightly convex in lateral view. Further, the outer surface of the coracoid lacks any sign of the supracoracoid sulcus present in other paravians.
In sum, basal dromaeosaurids (e.g., Bambiraptor) and troodontids lack some derived features that characterize Eumaniraptora, indicating that not all basal pennaraptorans had already evolved forelimb movements that were prerequisites for the acquisition of flight.
Muscle Changes
The m. supracoracoideus expanded its origin surface with the dorsoventral elongation of the coracoid, the development of osseous sternal plates and the later emergence of the sternal keel (Mayr, 2017), and by modifying the passage of the supracoracoidal tendon with the change of the depth and orientation of the supracoracoidal canal. Therefore, m. supracoracoideus changed its function by the evolutionary modifications that happened in the groups of basal paravians and basal birds.
The m. pectoralis also increased its origin area with the expansion of the coracoid and the appearance of osseous sternal plates and the sternal keel. The m. pectoralis in basal coelurosaurs and basal birds has been interpreted as a humerus depressor (Ostrom, 1976; Nicholls and Russell, 1985; Jasinoski et al., 2006). Its function was also enhanced by coracoid flexion and/or the flexion between coracoid and scapula.
While the origin of the m. biceps brachii has been thought to lie on the top of the acrocoracoid process in basal coelurosaurs (Nicholls and Russell, 1985; Jasinoski et al., 2006) and basal birds (Ostrom, 1976), the origin of the m. coracobrachialis cranialis (= brevis) was wider on the area of the subglenoid fossa (Nicholls and Russell, 1985). It is interesting to note that although the subglenoid fossa varied in shape and area among basal paravians and basal birds, in these groups the m. coracobrachialis cranialis always occupied a caudoventral position to the origin of the m. biceps brachii. This condition is shared with Rhea but contrasts with the derived attachment seen in flying Euornithes, in which both, mm. biceps brachii and coracobrachialis cranialis, originate on the acrocoracoid process, the former being dorsal to the latter (Figure 7). The presence of a well-developed subglenoid fossa in the coracoid of Confuciusornis (Figure 6) indicates that the plesiomorphic location of the m. coracobrachialis cranialis was retained by early pygostylians and contrasts with the derived attachment of the mm. biceps brachii and coracobrachialis cranialis present in tinamids and Neognathae (Figure 7).
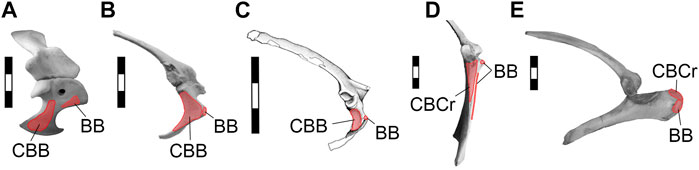
FIGURE 7. Selected scapulocoracoids from the right side in lateral views, showing the mm. biceps brachii and coracobrachialis origins. (A), Caiman latirostris; (B), Archaeopteryx lithographica (London specimen); (C), Buitreraptor gonzalezorum; (D), Rhea americana; (E), Vultur gryphus. Abbreviations. BB, m. biceps brachii; CBB, m. coracobrachialis brevis; CBCr, m. coracobrachialis cranialis. Light red, muscle origins. Scale bars 3 cm.
Although we do not know with certainty the origin area of the m. biceps brachii, it was probably located at the tip of the acrocoracoid process in Eumaniraptora. In contrast, the m. coracobrachialis cranialis occupied the entire subglenoid fossa in non-avialan eumaniraptorans. Thus, we hypothesize that this muscle had a larger area of origin than the one for the m. biceps brachii and, therefore, it could have presented greater volume and strength for humerus movements. This interpretation would indicate that m. coracobrachialis cranialis had a relevant role in protractor and depressor movements of the humerus and the wing in basal eumaniraptorans.
Implications for Ostrom’s (1976) Hypothetical Stages
The main modifications during the transition among basal theropods and paravians include:
1) Transverse rotation of the whole scapulocoracoid toward an anterior position on the chest,
2) Reduction of the area of origin of the deltoid muscle (mirroring the reduction of the acromion),
3) Great development of the m. supracoracoideus (probably following the backward expansion of the coracoidal shaft),
4) Change in the course of the tendon of the m. supracoracoideus that became encased in a supracoracoidal canal,
5) Flexure of the scapulocoracoid, increasing the lever arm of the m. supracoracoideus.
It is interesting to note that all these modifications occurred prior to the acquisition of the novel glenoid cavity of flying euornithines, which is horizontally oriented and located laterally with respect to the reduced scapular lip.
We have to ask here: what the functional implications of the above enumerated modifications on the movement and posture of the forelimb in basal paravians were? We ignore the response, but all suggest that this set of evolutionary novelties were not related to flying cappabilities.
Based on anatomical traits of the pectoral girdle, as well as field observations of non-tinamid paleognath behavior, the wings of non-tinamid paleognaths move predominantly in an anteroposterior direction, lacking the complex and largely dorsoventral wing excursion seen in living neognaths (see Novas et al., 2020). Because of the strong similarities in pectoral girdle structure between flightless paleognaths and basal avialans and paravians such as Archaeopteryx and Buitreraptor, it can be inferred that the main forelimb movements were from anterodorsal to posteroventral in the latter group. The primitive-looking pectoral girdle of Archaeopteryx is in agreement with other lines of evidence, including feather morphology and bone geometry (Feo et al., 2015; Mayr, 2017; Voeten et al., 2018), that suggest that flight capability was poor or absent (as originally advocated by Ostrom, 1976).
As indicated in a previous contribution (Novas et al., 2020), most discussions about flight origin assume that basal birds moved the wings like modern avialans. However, we think that as important as the wing range of motion is the orientation of the wing beat. As suggested by comparisons between Rhea and early paravians, the basal bird Archaeopteryx had a wing posture different from neognaths, with an arc of movement from anterodorsal to posteroventral, and a main wing surface that was posteroventrally oriented in maximum abduction (Voeten et al., 2018; Novas et al., 2020). This reconstruction of the wing posture suggests that this obliquely oriented wing surface may not have generated enough lift to allow these early birds to be airborne. This is not congruent with previous proposals on which early birds are inferred to be gliders with the wing surface subparallel to the ground (Beebe, 1915; Geist and Feduccia, 2000; Long et al., 2003; Xu et al., 2004; Zhou, 2004; Xu and Zhang, 2005; Longrich, 2006; Chatterjee and Templin, 2007; Hu et al., 2009; Alexander et al., 2010; Hone et al., 2010; Ruben, 2010).
The evolutionary stage represented by the basal pygostylians Confuciusornis and Sapeornis is functionally intriguing. Confuciusornis and Sapeornis exhibit enlarged wings of modern aspect, but their pectoral girdles retained the plesiomorphic morphology of more basal paravians, including the subvertical orientation of the major axis of the glenoid cavity and the lack of a “twisted” morphology, and the anteroventral position of the acrocoracoid process. If the basalmost pygostylians Confuciusornis and Sapeornis were capable of flying, as their wings suggest, then they lacked the cycle of wing movements around the glenoid of extant flying birds. Reconstruction of the wing beat in these basal birds, with an archaic construction of their shoulder girdles, represents an exciting challenge that needs to be seriously discussed in the years to come.
Conclusion
Inspired by Ostrom (1976) research, we evaluated some key-features of pectoral girdle morphology that may have importance in the rise of bird flight.
Important selective forces occurred at the level of Pennaraptora, with modifications of the thorax as a whole and the shoulder girdle in particular, positioning the coracoids over the cranial margin of the sternum (Paul, 2002). The laterally oriented glenoid cavity indicates that pennaraptorans differ from more basal coelurosaurs in having evolved a cranial shift of humeral movements, in agreement with the increase in size of the acrocoracoid process (which mirrors the increase in size and cranial pulling of the mm. coracobrachialis cranialis and biceps brachii). Explaining the reasons for such modifications is difficult, and we feel unable to envisage relationships with postural activities of the forelimbs other than increased capabilities for hunting, fighting, grasping, etc., but not for flying, although they were antecedents of the avian flight stroke.
Among paravians, the plesiomorphic pectoral girdle morphology is retained by troodontids like Gobivenator which lack a scapulocoracoid flexure and supracoracoid groove. More birdlike pectoral girdle features are shared by other basal paravians (i.e., Eumaniraptora) including basal birds such as Archaeopteryx, Confuciusornis and Sapeornis, which exhibit a derived coracoidal flexure and a well-defined supracoracoid sulcus, but retain an acrocoracoid that slightly surpasses the level of the glenoid cranially, and still lack an osseous bridge enclosing the triosseal canal. This combination of features is absent in non-paravian theropods, including troodontids, suggesting that an important change in pectoral girdle anatomy occurred at the base of Eumaniraptora. Possibly, the supracoracoid tendon in eumaniraptorans was housed in a deep supracoracoid sulcus that was roofed by an acrocoraco-acromial ligament, similarly to extant non-tinamid paleognaths.
We found that the m. supracoracoideus was much more developed in paravians more derived than troodontids, and that the existence of the tendon of m. supracoracoideus was probably diagnostic of eumaniraptorans. It is possible that the change in orientation of this muscle, the most important elevator muscle of the wing in extant birds, occurred not only through the extension of the acrocoracoid process, as Ostrom originally proposed, but by the channeling of the supracoracoidal sulcus and later by the rotation of the glenoid cavity. The increase of the lever arm of the m. supracoracoideus may have been increased in tandem with the coracoidal flexure, which is strong in paravians as Buitreraptor and Archaeopteryx, and even more pronounced in basal birds like Sapeornis and Confuciusornis (Agnolín et al., 2019).
The shape of the pectoral girdle and orientation of the glenoid of basal eumaniraptorans (including dromaeosaurids, unenlagiids, Archaeopteryx) is difficult to interpret both morphologically and functionally. As indicated previously, these early paravians probably had a wing posture similar to that of flightless paleognaths, with an anterodorsal-to-posteroventral arc of movement, and a wing surface that was posteroventrally oriented in maximum abduction.
The triosseal canal, as defined on the basis of bony contacts, was acquired in euornithine birds, but a foramen for the passage of the m. supracoracoideus was probably operative earlier (probably at the base of Pennaraptora, as shown by oviraptorosaurs with a cranially turned, pencil-like acromial process of the scapula) and bounded by bone (the acrocoracoid process), ligaments (the acrocoraco-acromial ligament bridging above the m. supracoracoideus), and eventually cartilage (the procoracoid process of the coracoid and the proximal end of the furcula).
In ornithothoracine birds the coracoid became even more elongate and lost its internal flexure. However, in enantiornithes and basal euornithines a groove on the proximal end of the scapula probably served for the path for the tendon of the m. supracoracoideus. This condition is usually correlated with the absence of a true triosseal canal and foramen. The latter was probably acquired in more derived euornithines as clearly observed in basal forms such as Yanornis and Ichthyornis (Clarke, 2004).
Data Availability Statement
The original contributions presented in the study are included in the article/Supplementary Material, further inquiries can be directed to the corresponding authors.
Author Contributions
All authors listed have made a substantial, direct, and intellectual contribution to the work and approved it for publication.
Conflict of Interest
The authors declare that the research was conducted in the absence of any commercial or financial relationships that could be construed as a potential conflict of interest.
Acknowledgments
X. Xu, C. Muñoz, P. Chafrat, D. Lijtmaer, P. Tubaro and S. Bogan for allowing access to osteological collections under their care. We also acknowledge R. Stoll, G. Stoll, M. Isasi, M. Cerroni, A.M. Aranciaga-Rolando and J.A. García Marsà for their help during experimentation with Rhea americana as well as for technical support. We thank David Marjanović and two anonymous reviewers for their comments that greatly improved the quality of this paper. We also thank Jingmai O’Connor and Ursula Rabar for their help and patience. We further thank J. O’Connor for inviting us to participate in this special issue. This contribution is part of the PICT 2018-01390 grant to FLA.
References
Agnolín, F. L., Motta, M. J., Brissón Egli, F., Lo Coco, G., and Novas, F. E. (2019). Paravian Phylogeny and the Dinosaur-Bird Transition: an Overview. Front. Earth Sci. 6, 252. doi:10.3389/feart.2018.00252
Agnolín, F. L., and Novas, F. E. (2013). Avian Ancestors: A Review of the Phylogenetic Relationships of the Theropods Unenlagiidae, Microraptoria, Anchiornis and Scansoriopterygidae. Dordrecht: Springer Science & Business Media), 1–96.
Agnolín, F. L., and Novas, F. E. (2011). Unenlagiid Theropods: Are They Members of the Dromaeosauridae (Theropoda, Maniraptora)? Acad. Bras. Ciênc. 83 (1), 117–162. doi:10.1590/s0001-37652011000100008
Alexander, D. E., Gong, E., Martin, L. D., Burnham, D. A., and Falk, A. R. (2010). Model Tests of Gliding with Different Hindwing Configurations in the Four-Winged Dromaeosaurid Microraptor gui. Proc. Natl. Acad. Sci. 107 (7), 2972–2976. doi:10.1073/pnas.0911852107
Baier, D. B., Gatesy, S. M., and Jenkins, F. A. (2007). A Critical Ligamentous Mechanism in the Evolution of Avian Flight. Nature 445 (7125), 307–310. doi:10.1038/nature05435
Bakker, R. T., Siegwarth, J., Kralis, D., and Filla, J. (1992). Edmarka rex, a New, Gigantic Theropod Dinosaur from the Middle Morrison Formation, Late Jurassic of the Como Bluff Outcrop Region. Hunteria 2 (9), 1–24.
Baumel, J. J., King, A. S., Lucas, A. M., Breazile, J. E., Evans, H. E., and Vanden Berge, J. C. (1993). Handbook of Avian Anatomy: Nomina Anatomica Avium. U.S.A.: Publ. of the Nuttall Ornithol. Club.
Brusatte, S. L., O’Connor, J. K., and Jarvis, E. D. (2015). The Origin and Diversification of Birds. Curr. Biol. 25 (19), R888–R898. doi:10.1016/j.cub.2015.08.003
Burnham, D. A., Derstler, K. L., Currie, P. J., Bakker, R. T., Zhou, Z., and Ostrom, J. H. (2000). Remarkable New Birdlike Dinosaur (Theropoda: Maniraptora) from the Upper Cretaceous of Montana. Univ. Kans. Paleontol. Contrib. Pap. 13, 1–13. doi:10.17161/pcns.1808.3761
Cau, A., Beyrand, V., Voeten, D. F. A. E., Fernandez, V., Tafforeau, P., Stein, K., et al. (2017). Synchrotron Scanning Reveals Amphibious Ecomorphology in a New Clade of Bird-like Dinosaurs. Nature 552 (7685), 395–399. doi:10.1038/nature24679
Cau, A. (2020). The Body Plan of Halszkaraptor escuilliei (Dinosauria, Theropoda) Is Not a Transitional Form along the Evolution of Dromaeosaurid Hypercarnivory. PeerJ 8, e8672. doi:10.7717/peerj.8672
Chatterjee, S., and Templin, R. J. (2007). Biplane Wing Planform and Flight Performance of the Feathered Dinosaur Microraptor gui. Proc. Natl. Acad. Sci. 104 (5), 1576–1580. doi:10.1073/pnas.0609975104
Chiappe, L. M., and Meng, Q. (2016). Birds of Stone: Chinese Avian Fossils from the Age of Dinosaurs. Baltimore: JHU Press.
Chiappe, L. M. (2002). “Osteology of the Flightless Patagopteryx deferrariisi from the Late Cretaceous of Patagonia (Argentina),” in Mesozoic Birds: Above the Heads of Dinosaurs. Editors L. M. Chiappe, and L. M. Witmer (California: University of California Press), 281–316.
Chiappe, L. M., and Vargas, A. (2003). Emplumando dinosaurios: la transición evolutiva de terópodos a aves. Hornero 18 (1), 1–11.
Clarke, J. A. (2004). Morphology, Phylogenetic Taxonomy, and Systematics of Ichthyornis and Apatornis (Avialae: Ornithurae). Bull. Am. Mus. Nat. Hist. 286 (286), 1–179. doi:10.1206/0003-0090(2004)286<0001:mptaso>2.0.co;2
Clarke, J. A., and Norell, M. A. (2002). The Morphology and Phylogenetic Position of Apsaravis ukhaana from the Late Cretaceous of Mongolia. Am. Mus. Novitates 3387 (3387), 1–46. doi:10.1206/0003-0082(2002)387<0001:tmappo>2.0.co;2
Currie, P. J., and Zhiming, D. (2001). New Information on Cretaceous Troodontids (Dinosauria, Theropoda) from the People's Republic of China. Can. J. Earth Sci. 38 (12), 1753–1766. doi:10.1139/e01-065
Dial, K. P. (1992). Avian Forelimb Muscles and Nonsteady Flight: Can Birds Fly without Using the Muscles in Their Wings? The Auk 109 (4), 874–885. doi:10.2307/4088162
Dial, K. P., Goslow, G. E., and Jenkins, F. A. (1991). The Functional Anatomy of the Shoulder in the European Starling (Sturnus vulgaris). J. Morphol. 207 (3), 327–344. doi:10.1002/jmor.1052070309
Feduccia, A. (1986). The Scapulocoracoid of Flightless Birds: a Primitive Avian Character Similar to that of Theropods. Ibis 128, 128–132. doi:10.1111/j.1474-919X.1986.tb02099.x
Feo, T. J., Field, D. J., and Prum, R. O. (2015). Barb Geometry of Asymmetrical Feathers Reveals a Transitional Morphology in the Evolution of Avian Flight. Proc. R. Soc. B. 282 (1803), 20142864. doi:10.1098/rspb.2014.2864
Forster, C. A., Sampson, S. D., Chiappe, L. M., and Krause, D. W. (1998). The Theropod Ancestry of Birds: New Evidence from the Late Cretaceous of Madagascar. Sci 279, 1915–1919. doi:10.1126/science.279.5358.1915
Geist, N. R., and Feduccia, A. (2000). Gravity-defying Behaviors: Identifying Models for Protoaves. Am. Zool. 40 (4), 664–675. doi:10.1093/icb/40.4.664
Gianechini, F. A., Makovicky, P. J., and Apesteguía, S. (2017). The Cranial Osteology of Buitreraptor gonzalezorum Makovicky, Apesteguía, and Agnolín, 2005 (Theropoda, Dromaeosauridae), from the Late Cretaceous of Patagonia, Argentina. J. Vertebr. Paleontol. 37 (1), e1255639. doi:10.1080/02724634.2017.1255639
Godefroit, P., Cau, A., Dong-Yu, H., Escuillié, F., Wenhao, W., and Dyke, G. (2013). A Jurassic Avialan Dinosaur from China Resolves the Early Phylogenetic History of Birds. Nature 498 (7454), 359–362. doi:10.1038/nature12168
Hartman, S., Mortimer, M., Wahl, W. R., Lomax, D. R., Lippincott, J., and Lovelace, D. M. (2019). A New Paravian Dinosaur from the Late Jurassic of North America Supports a Late Acquisition of Avian Flight. PeerJ 7, e7247. doi:10.7717/peerj.7247
Heers, A. M., and Dial, K. P. (2012). From Extant to Extinct: Locomotor Ontogeny and the Evolution of Avian Flight. Trends Ecol. Evol. 27, 296–305. doi:10.1016/j.tree.2011.12.003
Hone, D. W. E., Tischlinger, H., Xu, X., and Zhang, F. (2010). The Extent of the Preserved Feathers on the Four-Winged Dinosaur Microraptor gui under Ultraviolet Light. PLoS ONE 5 (2), e9223. doi:10.1371/journal.pone.0009223
Hu, D., Hou, L., Zhang, L., and Xu, X. (2009). A Pre-archaeopteryx Troodontid Theropod from China with Long Feathers on the Metatarsus. Nature 461 (7264), 640–643. doi:10.1038/nature08322
Hudson, G. E., Schreiweis, D. O., Wang, S. Y., and Lancaster, D. A. (1972). A Numerical Study of the Wing and Leg Muscles of Tinamous (Tinamidae). Northwest. Sci. 46, 207–255.
Jasinoski, S. C., Russell, A. P., and Currie, P. J. (2006). An Integrative Phylogenetic and Extrapolatory Approach to the Reconstruction of Dromaeosaur (Theropoda: Eumaniraptora) Shoulder Musculature. Zool. J. Linn. Soc. 146 (3), 301–344. doi:10.1111/j.1096-3642.2006.00200.x
Jenkins, F. A. (1993). The Evolution of the Avian Shoulder Joint. Am. J. Sci. 293, 253–267. doi:10.2475/ajs.293.a.253
Lefèvre, U., Cau, A., Cincotta, A., Hu, D., Chinsamy, A., Escuillié, F., et al. (2017). A New Jurassic Theropod from China Documents a Transitional Step in the Macrostructure of Feathers. Sci. Nat. 104 (9-10), 74. doi:10.1007/s00114-017-1496-y
Livezey, B. C. (1989). Phylogenetic Relationships and Incipient Flightlessness of the Extinct Auckland Islands Merganser. Wilson Bull. 101 (3), 410–435.
Livezey, B. C., and Zusi, R. L. (2007). Higher-order Phylogeny of Modern Birds (Theropoda, Aves: Neornithes) Based on Comparative Anatomy. II. Analysis and Discussion. Zool. J. Linn. Soc. 149 (1), 1–95. doi:10.1111/j.1096-3642.2006.00293.x
Lo Coco, G. E., Motta, M. J., Mosto, M. C., and Picasso, M. B. (2020). Wing and Tail Myology of Tyto furcata (Aves, Tytonidae). J. Morphol. 281 (4-5), 450–464. doi:10.1002/jmor.21111
Long, C. A., Zhang, G. P., George, T. F., and Long, C. F. (2003). Physical Theory, Origin of Flight, and a Synthesis Proposed for Birds. J. Theor. Biol. 224, 9–26. doi:10.1016/s0022-5193(03)00116-4
Longrich, N. (2006). Structure and Function of Hindlimb Feathers in Archaeopteryx lithographica. Paleobiology 32 (3), 417–431. doi:10.1666/04014.1
Lowe, P. R. (1928). Studies and Observations Bearing on the Phylogeny of the Ostrich and its Allies. Proc. Zool. Soc. Lond. 98, 185–247. doi:10.1111/j.1469-7998.1928.tb07148.x
Madsen, J. H. (1976). Allosaurus fragilis: a Revised Osteology. Utah Geol. Mineral. Surv. Bull. 109, 1–163.
Makovicky, P. J., Apesteguía, S., and Agnolín, F. L. (2005). The Earliest Dromaeosaurid Theropod from South America. Nature 437 (7061), 1007–1011. doi:10.1038/nature03996
Martin, L. D. (1995). The Enantiornithes: Terrestrial Birds of the Cretaceous. Cour. Forsch. Senckenberg 181, 23–36.
Maxwell, E. E., and Larsson, H. C. E. (2007). Osteology and Myology of the Wing of the Emu (Dromaius novaehollandiae), and its Bearing on the Evolution of Vestigial Structures. J. Morphol. 268 (5), 423–441. doi:10.1002/jmor.10527
Mayr, G. (2017). Pectoral Girdle Morphology of Mesozoic Birds and the Evolution of the Avian Supracoracoideus Muscle. J. Ornithol. 158 (3), 859–867. doi:10.1007/s10336-017-1451-x
McGowan, C. (1982). The Wing Musculature of the Brown kiwi Apteryx australis mantelli and its Bearing on Ratite Affinities. J. Zool. 197 (2), 173–219. doi:10.1111/jzo.1982.197.2.173
Motta, M. J., Agnolín, F. L., Brissón Egli, F., and Novas, F. E. (2020). New Theropod Dinosaur from the Upper Cretaceous of Patagonia Sheds Light on the Paravian Radiation in Gondwana. Sci. Nat. 107 (3), 1–8. doi:10.1007/s00114-020-01682-1
Nicholls, E. L., and Russell, A. P. (1985). Structure and Function of the Pectoral Girdle and Forelimb of Struthiomimus altus (Theropoda: Ornithomimidae). Palaeontol 28, 643–677.
Norell, M. A., and Clarke, J. A. (2001). Fossil that Fills a Critical Gap in Avian Evolution. Nature 409 (6817), 181–184. doi:10.1038/35051563
Novas, F. E., Agnolín, F., Brissón Egli, F., and Lo Coco, G. E. (2020). Pectoral Girdle Morphology in Early-Diverging Paravians and Living Ratites: Implications for the Origin of Flight. Bull. Am. Mus. Nat. Hist. 440 (1), 345–353. doi:10.5531/sd.sp.44
Novas, F. E., Brissón Egli, F., Agnolín, F. L., Gianechini, F. A., and Cerda, I. (2018). Postcranial Osteology of a New Specimen of Buitreraptor gonzalezorum (Theropoda, Unenlagiidae). Cretaceous Res. 83, 127–167. doi:10.1016/j.cretres.2017.06.003
O’Connor, J. K., Chiappe, L. M., and Bell, A. (2011). “Pre-modern Birds: Avian Divergences in the Mesozoic,” in Living Dinosaurs: The Evolutionary History of Modern Birds. Editors G. D. Dyke, and G. Kaiser (Hoboken, NJ: J. Wiley & Sons), 39–114. doi:10.1002/9781119990475.ch3
O’Connor, J. K., and Sullivan, C. (2014). Reinterpretation of the Early Cretaceous Maniraptoran (Dinosauria: Theropoda) Zhongornis haoae as a Scansoriopterygid-like Non-avian, and Morphological Resemblances between Scansoriopterygids and Basal Oviraptorosaurs. Vert. Pala. 52 (1), 3–30.
Olson, S. L. (1973). Evolution of the Rails of the South Atlantic Islands (Aves: Rallidae). Smithson. Contrib. Zool. 152, 1–53. doi:10.5479/si.00810282.152
Ostrom, J. H. (1969). Osteology of Deinonychus antirrhopus, an Unusual Theropod from the Lower Cretaceous of Montana. Peabody Mus. Bull. 30, 1–165. doi:10.2307/j.ctvqc6gzx
Ostrom, J. H. (1974). The Pectoral Girdle and Forelimb Function of Deinonychus (Reptilia: Saurischia): a Correction. Postilla 165, 1–11.
Ostrom, V. (1976). The American Experiment in Constitutional Choice. Public Choice 27, 1–12. doi:10.1007/bf01718942
Padian, K., Hutchinson, J. R., and Holtz, T. R. (1999). Phylogenetic Definitions and Nomenclature of the Major Taxonomic Categories of the Carnivorous Dinosauria (Theropoda). J. Vertebr. Paleontol. 19 (1), 69–80. doi:10.1080/02724634.1999.10011123
Paul, G. S. (2002). Dinosaurs of the Air: The Evolution and Loss of Flight in Dinosaurs and Birds. Baltimore: JHU Press.
Pei, R., Li, Q., Meng, Q., Norell, M. A., and Gao, K.-Q. (2017). New Specimens of Anchiornis huxleyi (Theropoda: Paraves) from the Late Jurassic of Northeastern China. Bull. Am. Mus. Nat. Hist. 411, 1–67. doi:10.1206/0003-0090-411.1.1
Pei, R., Pittman, M., Goloboff, P. A., Dececchi, T. A., Habib, M. B., Kaye, T. G., et al. (2020). Potential for Powered Flight Neared by Most Close Avialan Relatives, but Few Crossed its Thresholds. Curr. Biol. 30 (20), 4033–4046. doi:10.1016/j.cub.2020.06.105
Picasso, M. B., and Mosto, M. C. (2018). Wing Myology of Caracaras (Aves, Falconiformes): Muscular Features Associated with Flight Behavior. Vertebr. Zool. 68 (2), 177–190.
Raath, M. A. (1978). The Anatomy of the Triassic Theropod Syntarsus rhodesiensis (Saurischia: Podokesauridae) and a Consideration of its Biology. PhD Thesis. Grahamstown (Makhanda): Rhodes University, Faculty of Science, Zoology and Entomology.
Raikow, R. J. (1985). “Locomotor System,” in Form and Function in Birds. Editors A. King, and J. McLelland (London: Academic Press), 57–147.
Rauhut, O. W., Foth, C., and Tischlinger, H. (2018). The Oldest Archaeopteryx (Theropoda: Avialiae): a New Specimen from the Kimmeridgian/Tithonian Boundary of Schamhaupten, Bavaria. PeerJ 6, e4191. doi:10.7717/peerj.4191
Ruben, J. (2010). Paleobiology and the Origins of Avian Flight. Proc. Natl. Acad. Sci. 107 (7), 2733–2734. doi:10.1073/pnas.0915099107
Russell, D. A., and Dong, Z.-M. (1993). A Nearly Complete Skeleton of a New Troodontid Dinosaur from the Early Cretaceous of the Ordos Basin, Inner Mongolia, People's Republic of China. Can. J. Earth Sci. 30 (10), 2163–2173. doi:10.1139/e93-187
Sackton, T. B., Grayson, P., Cloutier, A., Hu, Z., Liu, J. S., Wheeler, N. E., et al. (2019). Convergent Regulatory Evolution and Loss of Flight in Paleognathous Birds. Science 364 (6435), 74–78. doi:10.1126/science.aat7244
Senter, P. (2006). Scapular Orientation in Theropods and Basal Birds, and the Origin of Flapping Flight. Acta Palaeontol. Pol. 51 (2). 305-313.
Suzuki, D., Chiba, K., VanBuren, C. S., and Ohashi, T. (2014). The Appendicular Anatomy of the Elegant Crested Tinamou (Eudromia elegans). Bull. Kitaky. Mus. Nat. Hist. Hum. Histo., Seri. A. 12, 1–48.
Tsuihiji, T., Barsbold, R., Watabe, M., Tsogtbaatar, K., Chinzorig, T., Fujiyama, Y., et al. (2014). An Exquisitely Preserved Troodontid Theropod with New Information on the Palatal Structure from the Upper Cretaceous of Mongolia. Naturwissenschaften 101 (2), 131–142. doi:10.1007/s00114-014-1143-9
Turner, A. H., Hwang, S. H., and Norell, M. A. (2007). A Small Derived Theropod from Öösh, Early Cretaceous, Baykhangor, Mongolia. Am. Mus. Novit. 2007 (3557), 1–27. doi:10.1206/0003-0082(2007)3557[1:asdtfs]2.0.co;2
Turner, A. H., Makovicky, P. J., and Norell, M. A. (2012). A Review of Dromaeosaurid Systematics and Paravian Phylogeny. Bull. Am. Mus. Nat. Hist. 371 (371), 1–206. doi:10.1206/748.1
Voeten, D. F., Cubo, J., Margerie, E., Röper, M., Beyrand, V., Bureš, S., et al. (2018). Wing Bone Geometry Reveals Active Flight in Archaeopteryx. Nat. Commun. 9 (1), 1–9. doi:10.1038/s41467-018-03296-8
Walker, C. A., and Dyke, G. J. (2009). Euenantiornithine Birds from the Late Cretaceous of El Brete (Argentina). Irish J. Earth Sci. 27, 15–62. doi:10.3318/ijes.2010.27.15
Wang, M., Stidham, T. A., and Zhou, Z. (2018). A New Clade of Basal Early Cretaceous Pygostylian Birds and Developmental Plasticity of the Avian Shoulder Girdle. Proc. Natl. Acad. Sci. USA 115 (42), 10708–10713. doi:10.1073/pnas.1812176115
Wang, M., Wang, X., Wang, Y., and Zhou, Z. (2016). A New Basal Bird from China with Implications for Morphological Diversity in Early Birds. Sci. Rep. 6, 19700. doi:10.1038/srep19700
Wang, X., Huang, J., Kundrát, M., Cau, A., Liu, X., Wang, Y., et al. (2020). A New Jeholornithiform Exhibits the Earliest Appearance of the Fused Sternum and Pelvis in the Evolution of Avialan Dinosaurs. J. Asian Earth Sci. 199, 104401. doi:10.1016/j.jseaes.2020.104401
Witmer, L. M. (2002). “The Debate on Avian Ancestry: Phylogeny, Function, and Fossils,” in Mesozoic Birds: Above the Heads of Dinosaurs. Editors L. M. Chiappe, and L. M. Witmer (Berkeley: University of California Press), 3–30.
Witmer, L. M. (1991). “Perspectives on Avian Origins,” in Origins of the Higher Groups of Tetrapods: Controversy and Consensus. Editors H. P. Schultze, and L. Trueb (Ithaca: Cornell University Press), 427–466.
Xu, X., Currie, P., Pittman, M., Xing, L., Meng, Q., Lü, J., et al. (2017). Mosaic Evolution in an Asymmetrically Feathered Troodontid Dinosaur with Transitional Features. Nat. Commun. 8 (1), 1–12. doi:10.1038/ncomms14972
Xu, X., and Kim, S. K. (2011). The Early Bird Catches the Worm: New Technologies for the Caenorhabditis elegans Toolkit. Nat. Rev. Genet. 12 (11), 793–801. doi:10.1038/nrg3050
Xu, X., Norell, M. A., Kuang, X., Wang, X., Zhao, Q., and Jia, C. (2004). Basal Tyrannosauroids from China and Evidence for Protofeathers in Tyrannosauroids. Nature 431, 680–684. doi:10.1038/nature02855
Xu, X., and Zhang, F. (2005). A New Maniraptoran Dinosaur from China with Long Feathers on the Metatarsus. Naturwissenschaften 92, 173–177. doi:10.1007/s00114-004-0604-y
Xu, X., Zhou, Z., Dudley, R., Mackem, S., Chuong, C. M., Erickson, G. M., et al. (2014). An Integrative Approach to Understanding Bird Origins. Sci 346 (6215), 1341. doi:10.1126/science.1253293
Yonezawa, T., Segawa, T., Mori, H., Campos, P. F., Hongoh, Y., Endo, H., et al. (2017). Phylogenomics and Morphology of Extinct Paleognaths Reveal the Origin and Evolution of the Ratites. Curr. Biol. 27 (1), 68–77. doi:10.1016/j.cub.2016.10.029
Zhou, Z. (2004). The Origin and Early Evolution of Birds: Discoveries, Disputes, and Perspectives from Fossil Evidence. Naturwissenschaften 91 (10), 455–471. doi:10.1007/s00114-004-0570-4
Zhou, Z., and Zhang, F. (2003b). Anatomy of the Primitive Bird Sapeornis chaoyangensis from the Early Cretaceous of Liaoning, China. Can. J. Earth Sci. 40 (5), 731–747. doi:10.1139/e03-011
Keywords: Archaeopteryx, hypothetical stages, flight origins, pectoral girdle, Ostrom
Citation: Novas FE, Motta MJ, Agnolín FL, Rozadilla S, Lo Coco GE and Brissón Egli F (2021) Comments on the Morphology of Basal Paravian Shoulder Girdle: New Data Based on Unenlagiid Theropods and Paleognath Birds. Front. Earth Sci. 9:662167. doi: 10.3389/feart.2021.662167
Received: 31 January 2021; Accepted: 04 May 2021;
Published: 25 May 2021.
Edited by:
Jingmai Kathleen O’Connor, Field Museum of Natural History, United StatesReviewed by:
Alexander Wilhelm Armin Kellner, Federal University of Rio De Janeiro, BrazilDavid Marjanović, Museum of Natural History Berlin (MfN), Germany
Thomas Dececchi, Mount Marty College, United States
Copyright © 2021 Novas, Motta, Agnolín, Rozadilla, Lo Coco and Brissón Egli. This is an open-access article distributed under the terms of the Creative Commons Attribution License (CC BY). The use, distribution or reproduction in other forums is permitted, provided the original author(s) and the copyright owner(s) are credited and that the original publication in this journal is cited, in accordance with accepted academic practice. No use, distribution or reproduction is permitted which does not comply with these terms.
*Correspondence: Fernando E. Novas, ZmVybm92YXNAeWFob28uY29tLmFy; Federico L. Agnolin, ZmVkZWFnbm9saW5AeWFob28uY29tLmFy