- 1College of Resources and Environmental Engineering of Guizhou University, Guiyang, China
- 2College of Eco-Environmental Engineering of Guizhou Minzu University, Guiyang, China
- 3Key Laboratory of Metallogeny and Mineral Assessment, Ministry of Natural Resources of the PRC, Institute of Mineral Resources, Chinese Academy of Geological Sciences, Beijing, China
- 4National Research Center for Geoanalysis, Beijing, China
Platinum group elements (PGEs) occur mainly in basic–ultrabasic igneous rocks and are concentrated by the differentiation and crystallization of magma. Thin polymetallic layers including Ni, Mo, V, PGEs, and rare-earth elements are widely developed in lower Cambrian black shales in southern China. The PGE contents in such layers may not be economically significant but are still unusually enriched. PGE enrichment mechanisms have not been widely studied, but here the PGE compositions of polymetallic layers in the Shuidong (Nayong) and Niuchang (Weng’an) sections of the SE Yangtze block in China were determined, and results compared with published data for the region. Results indicate that PGEs are enriched in the polymetallic layers, whereas the surrounding country rocks are barren. The ΣPGE contents in the Shuidong Ni–Mo deposits are generally lower than in the Niuchang V deposits. PGE distribution patterns in the polymetallic layers are similar to those in basic–ultrabasic rocks, indicating that such rocks played a role in the PGE enrichment. Oceanic hypoxia during the Sinian–Cambrian transition resulted in the production of large amounts of organic matter and H2S in the ocean. When high-salinity brine reached the ocean bottom, rapid changes in Eh–pH conditions caused enrichment of metals at the sediment–seawater interface, and this enrichment was later enhanced during diagenesis.
Introduction
Lower Cambrian black shales contain important ore-bearing strata worldwide, including large and super-large deposits of Ni–Mo–V–PGE, rare-earth elements (REEs), barite, P, and stone coal (Fan et al., 1984, Fan et al., 1987; Mao et al., 2002; Xu et al., 2012; Paava et al., 2019). In China, such shales are distributed mainly on the southeastern margin of the Yangtze Block, in Yunnan, Guizhou, Sichuan, Shanxi, Hubei, Hunan, Guangdong, Guangxi, Zhejiang, and Jiangxi provinces (Figure 1A). Large-scale deposits are distributed mainly in Guizhou and Hunan provinces (Figure 1B). Nickel, REE, and platinum group elements (PGEs) have recently been listed as strategic key minerals in the “National Mineral Resources Planning (2016–2020) of China” and the “Draft List of Crisis Minerals” issued by the United States Department of the Interior (Jiang et al., 2019). Study of the formation mechanisms and metallogenic characteristics of polymetallic mineralization in lower Cambrian black shales is thus important to meet the strategic needs of various countries.
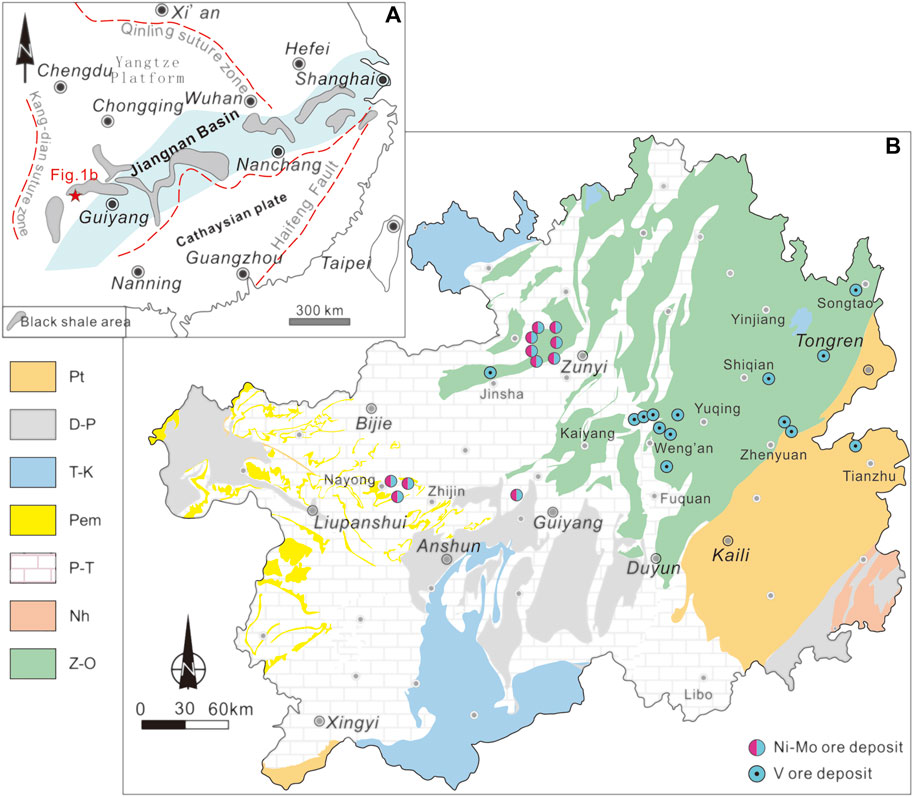
FIGURE 1. (A) Simplified paleogeographic map of the Yangtze Platform during the Cambrian (after Mao et al., 2002). (B) Simplified distribution of black shale polymetallic deposits in Gui Zhou (after Dai et al., 2014).
Several formation mechanisms have been proposed for lower Cambrian black-shale polymetallic deposits, including biogenesis (Jewell, 2000; Lu et al., 2004), a Ba-rich cold-spring origin (Paytan et al., 2002; Griffith and Paytan, 2012; Zhou et al., 2016), and hydrothermal origins (Wang and Li, 1991; Wu et al., 1999; Clark et al., 2004; Yang et al., 2007; Wu et al., 2009; Gao et al., 2018). The sources of Mo and Ni are possibly seawater (Mao et al., 2002; Lehmann et al., 2007; Xu et al., 2013) submarine hot water (Coveney, 2003; Jiang et al., 2006, Jiang et al., 2007; Han et al., 2017), and mixed sources of seawater and terrestrial hot water (Pašava et al., 2008). Vanadium sources include terrestrial environments, marine biogenesis, and deep-sea hot water (Fan et al., 1984; Fan et al., 1987). PGE deposits generally occur in basic–ultrabasic igneous rocks and are formed by the differentiation and crystallization of magma (Barnes and Lightfoot, 2005; Qi et al., 2008; Duan et al., 2016). However, this proposal is difficult to reconcile with the above mechanisms of PGE enrichment in black shale, with the PGEs likely being derived from multiple sources (Jiang et al., 2003). Ni–Mo sulfide ores in black shale of the Niutitang Formation, South China, have a submarine hydrothermal origin, and the extreme metal enrichment is likely to have occurred in an anoxic environment with abundant organic matter in an ocean basin (Jiang et al., 2007). PGE enrichment in black shale is similar to that in marine oil shale, with contributions by terrigenous and seawater sources (Han et al., 2015). Thus, the enrichment mechanism of PGE in black shale remains unclear and it can promote the understanding of the enrichment mechanism of polymetallic layers, and then guide the regional prospecting. This study aimed to improve our understanding of the genetic types of PGE deposit and to constrain the genesis of black-shale polymetallic deposits.
Geological Setting
Lower Cambrian black shales are commonly enriched in unusual combinations of elements including Mo, Ni, Se, Re, Os, REE, and PGE (Fan et al., 1984; Steiner et al., 2001; Mao et al., 2002; Jiang et al., 2007; Xu et al., 2013; Shi et al., 2020). Such enrichment occurs sporadically along a 1600 km NE-striking facies belt in South China (Figure 1A). However, economically mineable ores are exposed only in Guizhou and Hunan provinces, including the Zunyi, Nayong, and Zhenyuan districts of Guizhou Province, and the Dayong and Zhangjiajie districts of Hunan Province. In Guizhou during the early Cambrian, deep-sea sedimentary rocks were enriched mainly in Mo, V, and barite, while the shallow-sea carbonate platform was enriched mainly in Ni, Mo, V, and P (Figure 1B), forming one of the few economically viable Ni–Mo and V black-shale polymetallic deposits (Fan et al., 1984; Fan et al., 1987). Globally, it is rare for such Ni–Mo and V deposits to be economic (Coveney, 2003; Orberger et al., 2003).
The host sequence is a diachronous, transgressive black shale sequence of the Niutitang Formation. A conformable Ni–Mo polymetallic sulfide horizon occurs in the lowermost few centimeters of this succession. The linear geographic trend of Ni–Mo polymetallic sulfide ores in South China suggests the possibility of structural control by a major deep fault zone in the Neoproterozoic back-arc-basin/platform transition zone (Steiner et al., 2001). Alternatively, as the trend is parallel to the reconstructed Cambrian shoreline, it is also possible that water depth, distance from shore, sunlight availability, or other features of the paleo-environment controlled ore deposition (Xu et al., 2012; Pagès et al., 2018; Sarwar et al., 2019). The Niutitang Formation lies unconformably on dolomite of the Neoproterozoic Dengying Formation, which in turn is underlain by black shale, chert, phosphorite, and dolomite of the Doushantuo Formation (Fan et al., 1992). The Ni–Mo and V deposits have distinct spatial distributions, with the former being distributed mainly in the Bozhou and Huichuan districts of Zunyi City, and west of Meitan-Weng'an, Nayong, and Zhijin in Bijie City (Figure 1B). The combined Mo and Ni reserves of the known deposits in Guizhou are 897 kilotonne (kt) and 621 kt, respectively. These deposits include three large, three medium, and nine small ore deposits, and four ore-rich regions. Vanadium deposits are distributed mainly in the Tongren–Songtao–Wanshan, Shibing–Zhenyuan–Tianzhu, and Weng'an–Yuqing–Fuquan areas (Figure 1B). One large-scale, 15 medium-sized, and 43 small-scale V deposits have been found in Guizhou. Ni–Mo deposits, distributed mainly in the Yangtze area, occur in black carbonaceous mudstone at the base of the Niutitang Formation. Vanadium ore is distributed mainly in the transition zone of slope to basin and occurs in black carbonaceous mudstone, the lower part of the Niutitang Formation, and in siliceous rock of the Laobao Formation.
Section and Samples
The early Cambrian black-shale Ni–Mo and V polymetallic deposits have clearly defined distributions in Guizhou, with the Nayong–Zunyi area containing predominantly Ni–Mo deposits and the Weng’an–Tongren area V deposits. The XJ4 exploration well profile in Shuidong, and the Moshi profile in Niuchang, were chosen for study. The polymetallic layer and black shale were analyzed for PGE composition in the two study areas, with results being compared with published data for Ni–Mo–V–PGE polymetallic layers in black shales in South China. Sampling locations are shown in Figure 2.
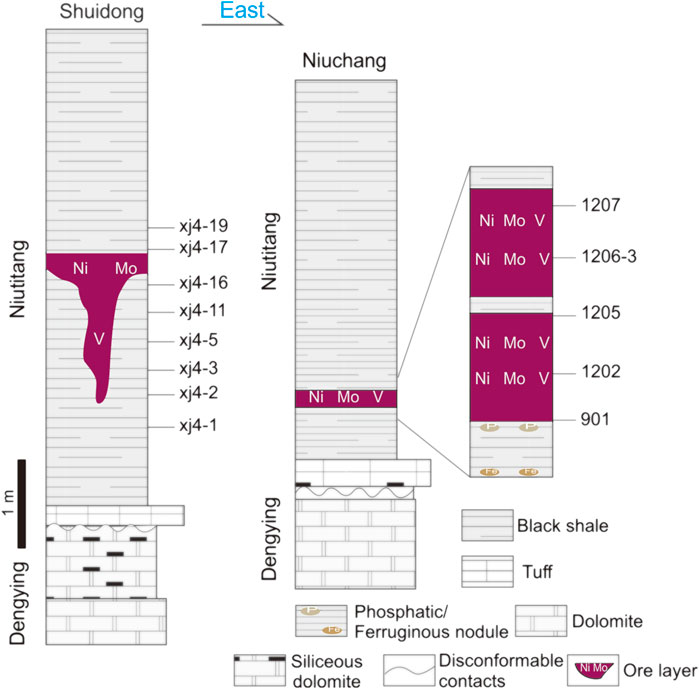
FIGURE 2. Lithological column and sampling locations in the studied sections at Shuidong and Niuchang, Guizhou Province.
Shuidong Section XJ4, Nayong
Lower Cambrian black shale in the Nayong area occurs in the western area of Guizhou Province and the southwestern area of the Yangtze Platform. The Shuidong Ni–Mo–polymetallic deposit lies ∼20 km southeast of Nayong County. Strata exposed in the mining area include the upper Sinian Dengying Formation; lower Cambrian Niutitang Formation; Mingxinsi Formation; upper Carboniferous Dapu, Huanglong, and Maping formations; Permian Liangshan, Qixia, Maokou Formation; and clasolite of Quaternary. The ore-bearing rock is within the first member of the lower Cambrian Niutitang Formation (0.5–18.0 m thick), and is in unconformable contact with the underlying Sinian Dengying Formation. The ore-bearing rock includes siliceous rock, banded dolomitic phosphorite, siliceous phosphorous rock, carbonaceous argillaceous siltstone, siliceous sandstone, carbonaceous mudstone, pyrite-bearing carbonaceous siltstone, and a Ni–Mo deposit (Figure 2A). The Ni–Mo deposit, which occurs on top of the ore-bearing rock group, is a dark-gray to gray-black shale layers. It has a scaly structure post-weathering. The deposit base is comprised siliceous siltstone and carbonaceous mudstone (0.05–0.10 m thick). The ore containing 0.50–7.40 wt% Mo (average 4.32 wt%) and 0.33–5.78 wt% Ni (average 2.37 wt%). The Mo and Ni contents tend to be negatively correlated.
Niuchang Section, Weng’an
The main Sinian–Cambrian strata in the Weng’an area include phosphorite of the Doushantuo Formation, dolomite of the Dengying Formation, black shale of the Niutitang Formation, and mudstone and sandstone of the Mingxinsi Formation. The Niuchang section shows that the stratum could divided seven sub-layers accordding to their lithology and mineralization from top to bottom, namely during the Sinian-Cambrian transition period (Figure 2B).
Gray massive dolomite (∼1.2 m thick) of the Dengying Formation, locally containing dissolution pores.
Fe–Mn oxide claystone (0.2 m thick), has a characteristics of typical paleo-weathering crust. It is discontinuous lens shape generally and distributed on concave and convex surfaces of the underlying dolomite of Sinian.
A Gy P-bearing dolomite layer, with a relatively high P content (0.2 m thick). It is the same age with the phosphorite in Zhijin of Guizhou and Kunyang of western Yunnan.
Carbonaceous silty hydromica claystone intercalated with lenticular phosphorite, total thickness 0.25 m.
Carbonaceous shale deposits containing Ni, Mo, and V. The metal sulfides are worm-like, bamboo-leaf-like and colloidal-like, distributed in a matrix of carbonaceous mica and silty sand. The ore bed is layered, lamella-like and lenticular. The boundary between the mineralized layer and the carbonaceous shale is clearly defined. The ore bed is generally 0.30 m thick.
A carbonaceous shale layer (0.1 m) containing V. The lamination is well developed and the bedding surface is smooth with a relatively high degree of cementation.
Carbonaceous hydromica claystone and silty claystone, total thickness >2 m.
Analytical Methods
PGE analyses were undertaken at the National Research Center for Geoanalysis (NRCG), Beijing, China. A digestion technique involving 100 ml Teflon beakers and stainless-steel pressure bomb was used (Qi et al., 2011). Powdered sample (2–3 g) was dissolved in HF + HNO3 in a 120 ml Teflon beaker to remove silicates and sulfides. The dried residue, with an appropriate amount of isotopic spike solution containing 101Ru, 193Ir, 105Pd, and 194Pt was digested with 5 ml HF + 15 ml HNO3 in a sealed beaker in the pressure bomb at 190°C for 48 h, and the resulting solution evaporated to dryness. HCl (5 ml) was added to remove residual HF and HNO3 during evaporation to dryness. The residue was dissolved in 40 ml 2 mol L−1 HCl and centrifuged. PGEs were preconcentrated from the supernate by coprecipitation with Te. The main interfering elements (Cu, Ni, Zr, and Hf) were removed by ion-exchange chromatography with Dowex 50 W X8 cation exchange resin and a P507 Levextrel resin. The eluate was analyzed by inductively coupled plasma–mass spectrometry (ELAN DRC-e), with detection limits ranging from 0.004 ppb for Ir to 0.014 ppb for Pt.
Results
PGE analysis results are listed in Table 1. The total PGE (ΣPGE) content of the polymetallic layer in the Weng’an area is in the range 538.87–989.59 ppb (average 773.18 ppb) with Os = 50.41–79.99 ppb; Ir = 1.82–3.09 ppb; Ru = 5.39–13.58 ppb; Rh = 1.56–6.19 ppb; Pt = 216.87–381 ppb; and Pd = 263–380.82 ppb. The ΣPGE of the polymetallic layer in the Nayong area is in the range 373.00–793.44 ppb (average 604.96 ppb) with Os = 21.07–51.87 ppb; Ir = 1.52–4.03 ppb; Ru = 1.93–6.66 ppb; Rh = 0.03–4.32 ppb; Pt = 142.67–370.28 ppb; and Pd = 205.76–356.95 ppb. The Rh content of sample Nayong xj4-11 is very low, possibly because Rh has no corresponding isotopic calibration and the analytical result is inaccurate.
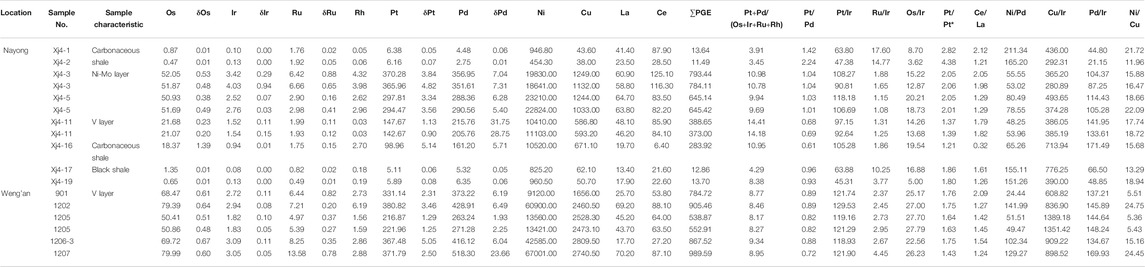
TABLE 1. PGE contents (ppb) of black shales in the lower Cambrian Niutitang Formation at Shuidong and Niuchang, Guizhou Province.
Discussion
Regional Distribution of PGE
Analysis of the Shuidong (Nayong) section indicates that PGEs are enriched only in the polymetallic layer, with low contents in country rocks and the overlying and underlying black shale (Figure 3). The Weng’an area contains predominantly V mineralization, with the PGE content of the polymetallic layer generally being higher than in the Nayong area. This difference implies that the PGE content varies between sedimentary environments. Previous studies have shown that the PGE contents of polymetallic layers in Zhongnan Village, Zunyi, and in Guizhou and Dayong, Hunan, are in the range 551–1215 ppb (Li et al., 2003), and that the Huangjiawan polymetallic layer in the Songlin, Zunyi (Guizhou) contains 773–1187 ppb (Mao et al., 2001). The PGE content of the V-bearing black-shale layer in western Hunan is 123 ppb (Wu et al., 2001), much lower than in regional polymetallic layers.
Carbonaceous black shale in the Nayong area has ΣPGE contents of 11.5–13.7 ppb with Os = 0.47–1.35 ppb; Ir = 0.08–0.13 ppb; Ru = 0.49–1.92 ppb; Rh = 0.05–0.19 ppb; Pt = 5.1–6.4 ppb; and Pd = 2.8–6.4 ppb. Its ΣPGE content is similar to those of deep-water black shale in western Hunan (Wu et al., 2001) and Cambrian black shale in the base of the Tarim Basin (Yu et al., 2003) (Figure 4). This indicates that under anoxic conditions, the adsorption capacity of organic-rich shale is similar for all PGEs and that the formation environments of these rocks were similar.
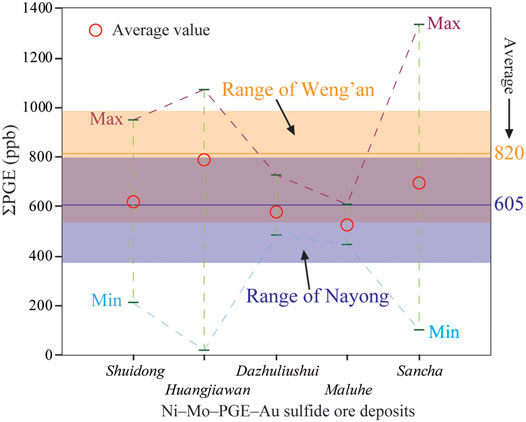
FIGURE 4. Comparison of ΣPGE values of the lower Cambrian black-shale polymetallic layer in Hunan–Guizhou (after Li and Gao, 2000; Mao et al., 2002; Luo et al., 2003; Lehmann et al., 2007; Han et al., 2015).
Nayong and Weng’an black shale samples exhibit PPGE (Pt + Pd) enrichment and relative IPGE (Os + Ir + Ru + Rh) depletion. The PPGE/IPGE ratio is generally >1 (3.8–14.4), with ratios for most samples being around 9 (barring sample XJ4-11) in the polymetallic layer, much higher than that of original mantle (0.88) and upper crust (1.3) but lower than the crustal ratio (15); and also higher than the value for modern South China Sea sediments (3.45; Zhu et al., 2010) and oceanic Co-rich crusts (1.62–7.32; Yao et al., 2002; Sun et al., 2006). Sample Pt/Pd ratios are generally in the range 0.7–2.2 and <1 in the polymetallic layer (0.6–1.0), much lower than in Co-rich oceanic crust (53–439; Yao et al., 2002; Sun et al., 2006), lower than normal seawater (4.5; Hodge et al., 1985), and similar to sulfides in basic–ultrabasic rock (Pašava et al., 2003; Pašava et al., 2004). The Os/Ir ratio in the polymetallic layer is in the range 12.9–26.6, much higher than in primitive mantle (∼1.1) and chondrite (∼1.0; McDonough and Sun, 1995), but similar to organic-rich shale such as oil shale in Tibet (12.2–15.8; Fu et al., 2010). There is strong positive correlation between Pt and Pd contents (Figure 5A). The Pt content is also positively correlated with Os, Ir, and Ru contents (Figures 5B–D). There is a lack of correlation between Ru–Rh and Os–Ir, among other elements (Figures 5E,F). The Ni/Cu, Ce/La, Ni/Pd, and Cu/Ir ratios are respectively 5.4–21.7, 0.3–2.1, 50–211, and 289–1384, and the Cu/Ir ratio of the polymetallic layer is much higher than that of black shale. The Ni/Pd ratio is generally lower than that of black shale (Table 1).
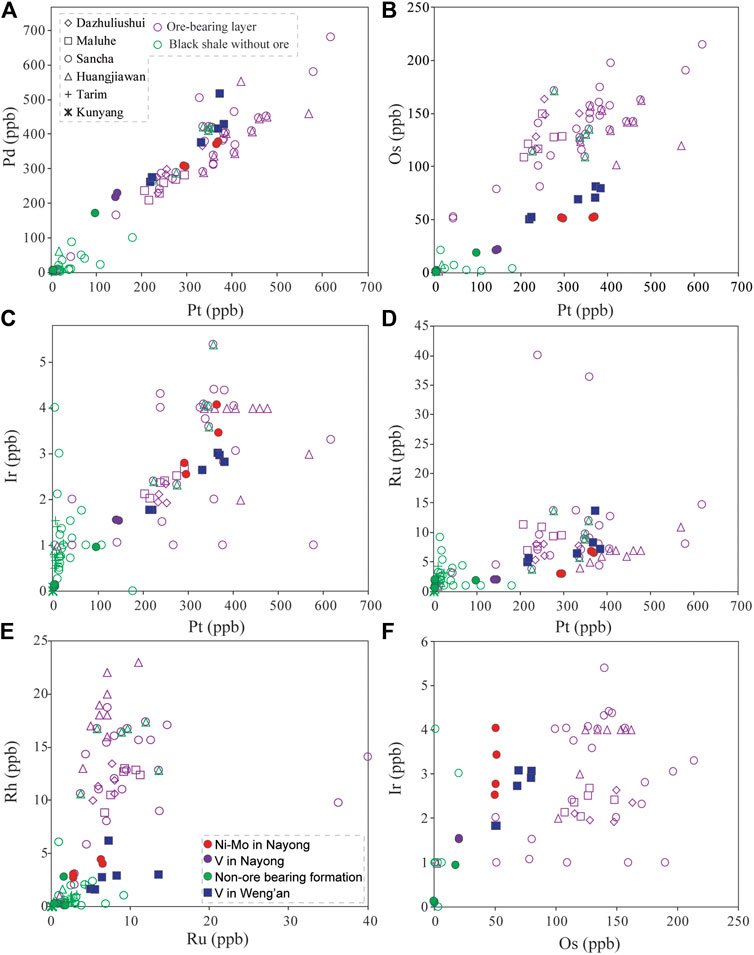
FIGURE 5. (A) Pd–Pt; (B) Os–Pt; (C) Ir–Pt; (D) Ru–Pt; (E) Rh–Ru; and (F) Ir–Os diagrams for the Cambrian ore-bearing layer and black shale.
PGE Distribution Model
PGEs are not strongly affected by low-temperature alteration and diagenesis; consequently, their distribution pattern in rocks is indicative of the environment in which the host rock was formed and of their source (Mao et al., 2001; Lehmann et al., 2003; Li et al., 2003; Pašava et al., 2003; Pašava et al., 2004). A PGE distribution model based on Ni–Mo–V–PGE contents of the polymetallic layer was developed for the black shales of Nayong and Weng’an. The elemental distribution curve is similar to that of the polymetallic layer in mining areas of Zunyi and west Hunan (Figure 6; Li and Gao, 2000; Wu et al., 2001; Mao et al., 2002; Yu et al., 2003; Lehmann et al., 2007; Xu et al., 2013; Han et al., 2015; Xing et al., 2015). The four regions of Nayong, Zunyi, Weng’an, and Zhangjiajie lie on the same metallogenic belt (Figures 1A, 6B) and have the same material source and metallogenic mechanism.
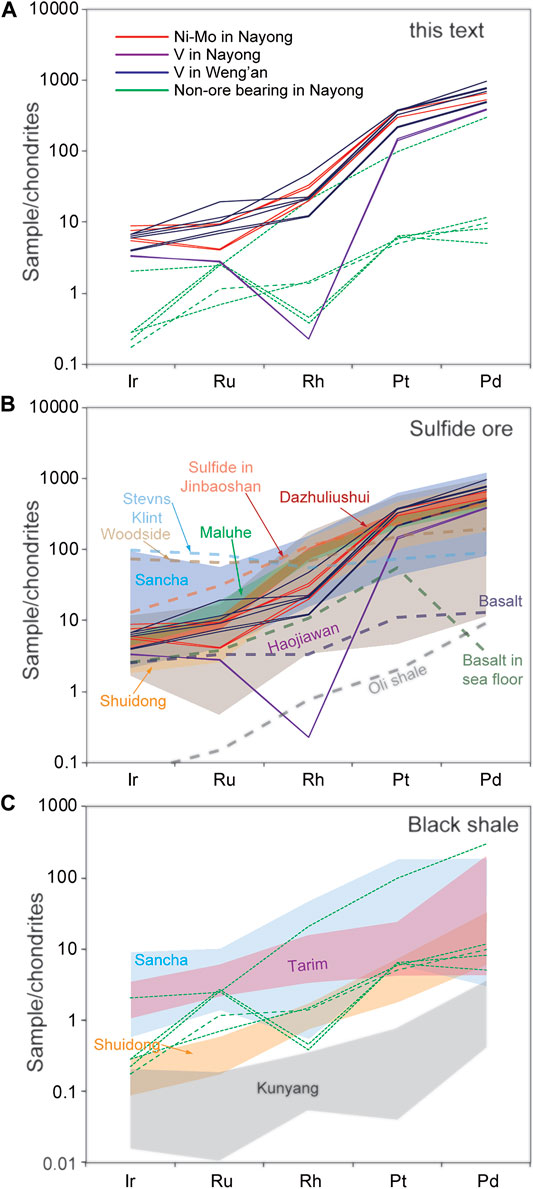
FIGURE 6. PGE/chondrite distribution pattern of the Cambrian ore-bearing layer and black shale. Chondrite data are from McDonough and Sun (1995); Jinbaoshan basic-rock sulfide-enclave data are from Wang et al. (2010); ocean-floor basalt data are from Sun et al. (2010); K–T boundary layer clay data are from Evans et al. (1993); oil shale data are from Fu et al. (2010); other data are from Wu et al. (2001), Li and Gao (2000), Mao et al. (2001), Wu et al. (2001), Xu et al. (2013), Xing et al. (2015), and Han et al. (2015).
The source of mineralization elements in the black-shale polymetallic layer at the base of the Niutitang Formation is considered in terms of our analytical data. Although the PGE content of seafloor Fe–Mn crusts is similar to that of the Cambrian black-shale polymetallic layer (Sun et al., 2006) and the PGE distribution model is consistent with normal oceanic sediments. PGE distributions are similar in basic–ultrabasic rocks, continental basalt, organic-rich black shale, and oil shale (Figure 6B), implying that either 1) PGEs in black shale are derived from basic–ultrabasic rocks in underlying strata (Li and Gao, 2000; Jiang et al., 2003; Jiang et al., 2007) or 2) the underlying basic–ultrabasic rocks are weathered and their contents transported to the ocean after being exposed on the surface, with extreme enrichment occurring due to changes in the Eh/pH balance of seawater (Lehmann et al., 2003; Lehmann et al., 2007).
Oil shale in Tibet and Cambrian black shale in the Tarim Basin have PGE distribution curves similar to that of the polymetallic layer in the Hunan–Guizhou area (Yu et al., 2003; Fu et al., 2010), further implying that the PGE distribution in organic-rich shale is controlled by the occurrence of PGE rocks. The PGE contents of different phases in black shales of South China indicate that PGEs are associated mainly with organic matter and sulfide phases (Wang et al., 2004). Other studies have found that Au (1900 μg g−1), Pt (600 μg g−1), and Pd (1900 μg g−1) are highly enriched in the organic-rich clay rock of black shale. In minerals containing arsenide, uranium, and thorium, Pt and U contents are positively correlated, while Pd is enriched in As–Au–Ag-containing aggregates (Kucha, 1982; Kucha and Przybylowicz, 1999). Adsorption by organic matter and sulfides in organic-rich shales thus affects the distribution of PGEs in black shale, meaning that not appropriate for clarifying the source of PGEs, simple according to the PGE distribution curves.
PGE Ratios in Polymetallic Layers
PGE ratios and their relationship with ore-forming elements are considered an effective geochemical method for tracing mineral sources (Li and Gao, 2000; Mao et al., 2001; Lehmann et al., 2003; Lehmann et al., 2007; Pašava et al., 2003; Pašava et al., 2004). The polymetallic layers in lower Cambrian black shales in South China contain elevated Ir contents, exceeding 10 ppb (Fan et al., 1984), similar to the Ir content of clays at the Cretaceous–Tertiary (K–T) boundary where the Os/Ir ratio of clay minerals is generally <1 (Evans et al., 1993) and different to chondrite values (McDonough and Sun, 1995). However, for the Cambrian polymetallic layer in South China the Os/Ir ratio is in the range 12–26 (Li et al., 2000; Mao et al., 2001; Jiang et al., 2007), much higher than that of the clay layer at the K–T boundary. Furthermore, the PGE distribution curve of the K–T clay layer is completely different to that of the polymetallic layer. The chondrite standard curve is near level, similar to that of the element distribution (Figure 6B).
Pt/Pd and Au/Pd ratios in the polymetallic layer of Cambrian black shale in South China are near unity (Lehmann et al., 2003; Lehmann et al., 2007), similar to the ratios in seawater (Nozaki, 1997). Cambrian seawater had high metal contents. The Mo isotopic studies of the PGE polymetallic layer indicating that Sinian–Cambrian-boundary seawater contained large amount of H2S (Lehmann et al., 2003; Lehmann et al., 2007). This is the mineralization model for sedimentation from normal seawater. However, Pt/Pd ratios in many PGE deposits are near unity, as in the Jinbaoshan basic–ultrabasic deposit, the Yangliuping deposit, and Archean komatiite sulfides (Brugmann et al., 1989; Tao et al., 2007; Liang et al., 2019).
The PGE enrichment factor in the black-shale polymetallic layer can reach 107–109, differing from the adjacent non-metallic layers by one to two orders of magnitude. If seawater were rich in metal elements together with excessive H2S, the PGE content of the black shale would be high. However, the PGE content above and below the polymetallic layer is not significantly different to that of typical sediments (Zhu et al., 2010). An example of anomalously high levels of PGE in sediments is cobalt-rich Fe–Mn oceanic crusts, reaching hundreds of ppb (Yao et al., 2002; Sun et al., 2006). The enrichment of many ore-forming elements in oceanic Fe–Mn crusts is controlled mainly by the deposition rate of the crust (Puteanus and Halbach, 1988). Therefore, a low deposition rate of black shale would favor the formation of polymetallic deposits (Lehmann et al., 2003; Lehmann et al., 2007). However, some studies have indicated that the deposition rate cannot be the dominant factor in Fe–Mn crust enrichment in mineral elements (Basolo and Pearson, 1973). The Eh–pH values in the formation environment of Fe–Mn crusts may promote oxidation/reduction reactions between free PGE ions in seawater and Mn2+ on the contact surface of the Fe–Mn crust. Free PGE ions would also form hydroxides and be adsorbed by the Fe–Mn crust under appropriate Eh–pH conditions. The PGE enrichment of Fe–Mn crusts is thus not controlled by deposition rate; rather, it is due to the geochemical conditions of seawater and PGE properties (Sun et al., 2006). Black shale is formed at relatively low Eh–pH values (Basolo and Pearson, 1973; Gao et al., 2018); i.e., under conditions suitable for PGE adsorption by Fe–Mn crusts. However, excessive PGE enrichment is rare in black shale. Globally, PGE-rich black-shale deposits occur mainly in the Dry Valley in Russia (Distler et al., 2004), the Yukon in Canada (Pašava et al., 2003), and Palentin in the Czech Republic (Pašava et al., 2003). These deposits were controlled by organic matter and sulfides, with their metallogenic setting being rift-type basins and with their ore-forming matters are mainly derived from hydrothermal fluid. Therefore, the model of PGE enrichment and mineralization in polymetallic layers formed by normal seawater deposition should be further discussed.
A comparison of the PGE distribution in black shales with that in modern submarine hydrothermal sulfides indicates that the polymetallic layers in black shales of South China were formed mainly by hot brine (Li et al., 2000; Jiang et al., 2003; Pašava et al., 2003; Pašava et al., 2004; Jiang et al., 2007). The distribution curve of PGEs in the polymetallic layers is similar to that in basic and ultrabasic rocks. Zinc-rich black shale in the Czech Republic and Ni-rich black shale in Canada have Pt/Pd ratios similar to those in the South China polymetallic layer (Figure 7; Pašava et al., 2003), while the ratios in Co-rich crusts formed by seawater sedimentation are completely different, tut similar with the Zn-rich black shale in the Czech Republic which was influenced by hydrothermal processes (Kucha, 1982). So the minerology of black shale in South China may also have been controlled by hydrothermal processes. Cu/Ir, Ni/Pd, Ni/Cu, and Pd/Ir ratios can be used to trace the material sources of ore-forming fluids (Pašava et al., 2003). The polymetallic layer in the Nayong and Weng’an areas plots in the ophiolite and PGE-vein fields in the (Cu/Ir)–(Ni/Pd) and (Ni/Cu)–(Pd/Ir) diagrams (Figure 8), implying that the main source of PGE elements in the Nayong–Weng’an polymetallic layers was related to ultrabasic rocks. The tectonic background of the region suggests that the Nayong–Zhijin–Zunyi–Weng’an–Dayong metallogenic belt was in an extensional setting in the Hunan–Guizhou area during the Cambrian (Wu et al., 1999; Li et al., 2003), with the many extensional fractures that developed providing sites for hydrothermal activity. Sulfides in the largest modern-day submarine hydrothermal area (the Trans-Atlantic Geotraverse, Mid-Atlantic Ridge) have a Pd content of up to 1 μg g−1, while those in submarine black chimneys can reach 136 ppb (Wang et al., 2004). Submarine hydrothermal solutions could thus provide large amounts of PGEs. A quartz vein was found cutting the phosphorous rock layer under the black shale polymetallic layer in the Huangjiawan deposit, Guizhou, and the Daping deposit, Hunan. Fluid inclusions in this ore-bearing vein have a fluid salinity of up to 30 wt% and a trapping temperature of 70–140°C (Wang et al., 2004), indicating that the formation of the black shale was directly related to hydrothermal fluids. Many “siliceous chimneys” have been discovered in the Ganziping section at Dayong but not at Nayong. The siliceous layer is only 50–70 cm below the black-shale polymetallic layer. Banded barite is common in the siliceous rock, confirming the existence of ancient hydrothermal fluids. Fluid inclusions in siliceous rocks have also been found to contain high-salinity brine with a salinity of >35% and sealing temperatures of 110–135°C (Chen et al., 2009).
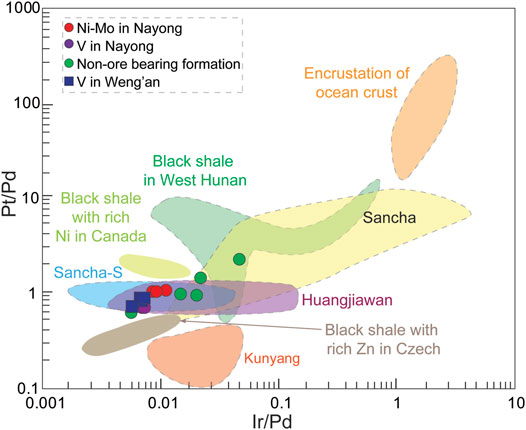
FIGURE 7. (Pt/Pd)–(Ir/Pd) diagram for different sediments (data sources as for Figure 6).
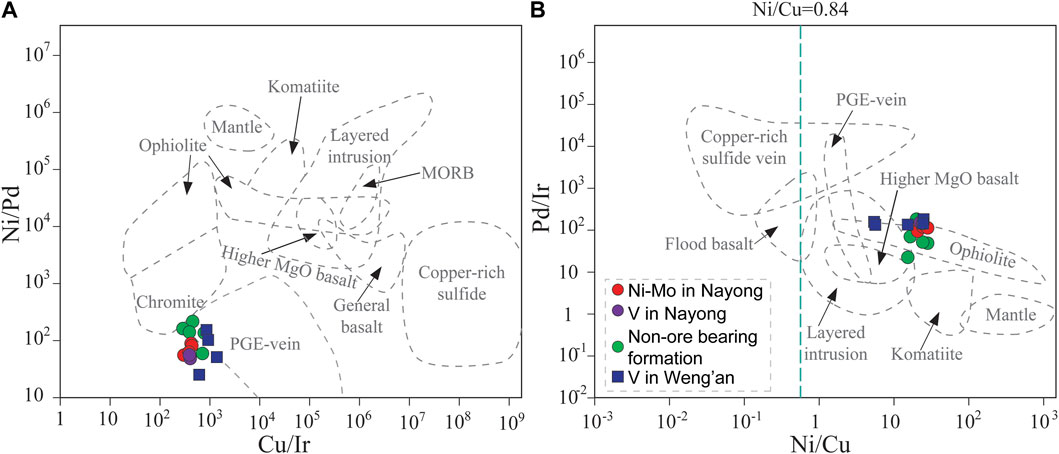
FIGURE 8. (Cu/Ir)–(Ni/Pd) and (Ni/Cu)–(Pd/Ir) diagrams for black shale and the polymetallic layer at the base of the Cambrian profile in Nayong–Weng’an (after Pašava et al., 2003).
During the Sinian–Cambrian period, seawater suddenly changed from being oxygen-rich to oxygen-deficient, with large amounts of organic matter accumulating in the oceans and sulfate rock being reduced to sulfide, providing conditions conducive to PGE enrichment (Wu et al., 1999; Mao et al., 2002; Jiang et al., 2007; Han et al., 2015). Volcanic tuff occurs near the bottom of the Cambrian profile in the Kunyang and Songlin areas, under the Ni–Mo layer and occuring as a lens shape on the southeastern edge of the Yangtze Platform (Luo et al., 2003). The tuff contains large amounts of sulfide and organic matter, with PGE contents of up to hundreds of ppb, including Pt and Pd at up to 434 and 142 ppb, respectively. The distribution of PGEs relative to chondrites is similar to that of PGEs in the black-shale polymetallic layer (Pašava et al., 2010). At the bottom of the black shale of Niutitang Formation, a thin layer of tuff is usually developed, indicating the presence of volcanism. In other words, the PGE and polymetallic sources may be contributed by volcanic rocks. There is no direct evidence for such a direct PGE source but it does appear that the PGEs were derived mainly from basic or ultrabasic rocks rather than directly from seawater.
Enrichment of PGES in Black Shale
The mineralization of PGEs in black shale in South China is controlled mainly by their source area and seawater Eh–pH conditions. PGEs are generally considered inert, with their separation and enrichment being associated mainly with high-temperature and high-pressure magmatic activity. Previous simulations of the effects of low-temperature hydrothermal activity on PGEs indicate Pd and Pt exist as PdCl2– 4 and PtCl3– in solution, respectively, at concentrations of up to >1 ppb (Mountain and wood, 1988; Gammons et al., 1992), and demonstrate the mobile nature of Pt and Pd in high-salinity hydrothermal fluid. Furthermore, a simulation of the solubility of PdS and PtS in H2S + NaHS solution indicates that the amounts dissolved exceed the number of chloro complexes (Gammons and Bloom, 1993). This may explain why the Pd sulfide content at the mouth of seafloor black chimneys can be as high as 1 ppb (Crocket, 1990), illustrating the ease with which Pt and Pd enter solution as complexes during low-temperature hydrothermal processes (Mountain, 1989; Gammons et al., 1992; Gammons and Bloom, 1993; Chen et al., 2009). We conclude that hydrothermal fluids participated during the early stages of the formation of the black-shale polymetallic layer in South China, leading to PGE enrichment.
PGEs are transported mainly in the form of chlorides (Figure 9), and when hydrothermal fluid crosses the seawater–sediment interface, Pt2+ and Pd4+ are released into the seawater. In seawater, Pt2+ is most stable as
Where
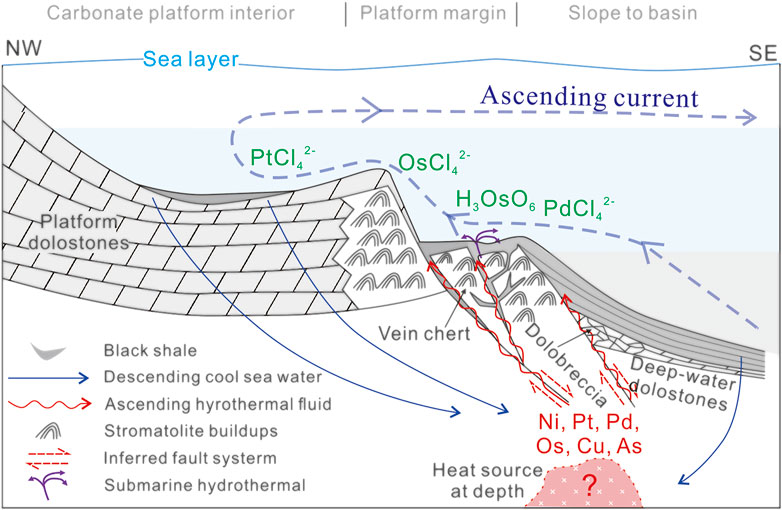
FIGURE 9. PGE metallogenic model for the Cambrian polymetallic layer in South China (after Wang et al., 2012; Li et al., 2020).
Pt and Pd do not enter sediments as chlorides for the waters of South China, but are adsorbed on sediment as sulfide, and arsenide forms with the MeCly x (Me = Pd–Pt–Os) + S2–/As2– = MeS/(As + Cl); MeCly x(Me = Pd, Pt, Os)—ne = Me + Cl−chemical reaction on the surface of sediment. Kucha (1982) found that PGEs in Zn-rich black shale are distributed mainly in arsenide and sulfide. Pt–Cl and Pd–Cl bond energies in K2Me(Pt, Pd)Cl4 are 372.6 and 347.5 kJ mol−1, respectively (Basolo and Pearson, 1967). In seawater, Pd ions are reduced before entering sediments, with Pd/Pt ratios in sediments thus being >1 in reducing environments.
The PGE distribution in black shale shows a typical Pt–Pd enrichment pattern, similar to that in many basalts (Rehkämper et al., 1999), with Pt/Ru ≈ 10 and Pt/Pd ≈ 1. Osmium is highly enriched (up to 100 ppb) due to its solubility in seawater where it exists mainly as
In summary, PGE-rich ore in the Ni–Mo polymetallic layer of black shale in South China was formed during the development of rifts, with fractures forming in basement rocks and frequent water–rock exchange reactions. Coincidentally, the geothermal gradient in the South China metallogenic belt increased rapidly, enhancing the thermal convection velocity and causing the overflow of high-salinity brine rich in metals (Li et al., 2020). Oceanic hypoxia events occurred during the Sinian–Cambrian, producing large amounts of organic matter and H2S in the oceans (Lehmann et al., 2003; Lehmann et al., 2007; Wang et al., 2012). When high-salinity brine reached the ocean floor as part of oceanic circulation, the rapid change in Eh–pH conditions caused many metal elements to become enriched at the sedimentary interface, ultimately enriching the ore. Metal ions were also transported in ocean circulation, resulting in distal precipitation and mineralization (Figure 9).
Conclusion
PGEs are enriched only in the polymetallic layer of the black shale examined here. The PGE contents in the vanadium-mining area of Niuchang are higher than in the Ni–Mo mining area of Shuidong. The accumulation of PGEs in the lower Cambrian black-shale polymetallic layer was controlled mainly by hydrothermal processes, which provided large amounts of ore-forming elements to the South China anoxic ocean basin. With ocean circulation and continuing sea-level rise, the productivity of bio-organisms increased, enriching the ocean floor in organic matter. The degradation of this organic matter produced H2S, changing seawater Eh–pH conditions and promoting PGE enrichment. Black-shale PGE-rich ore in South China is thus a syn-sedimentary ore formed under the action of hydrothermal fluids.
Data Availability Statement
The original contributions presented in the study are included in the article/'Supplementary Material, further inquiries can be directed to the corresponding author.
Author Contributions
YF led the conception and design of the paper. ZY and PX contributed the field work, summarize and analyze the distribution regularity of PGE in the Early Cambrian black shale in the region. CL contributed the experiments to test PGE and geochemical analysis.
Funding
This work was jointly supported by the National Natural Science Foundation of China (Grand No. 41763006, 42063009), Mineral Geology of China Project, China Geological Survey (Grand Nos. (DD20160346, DD20190379) and Guizhou Science and Technology Fund ((2020)1Y166).
Conflict of Interest
The authors declare that the research was conducted in the absence of any commercial or financial relationships that could be construed as a potential conflict of interest.
Acknowledgments
We thank to Associate Editor Prof. Xiaohua Deng and two reviewers for their constructive suggestions.
References
Barnes, S. J., and Lightfoot, P. C. (2005). Formation of magmatic nickel-sulfide ore deposits and processes affecting their copper and platinum-group element contents. Econ. Geology. 100, 179–213. doi:10.5382/AV100.08
Basolo, F., and Pearson, R. G. (1967). Mechanisms of inorganic reactions. New York, NY: Wiley. Chap. 3.
Basolo, F., and Pearson, R. G. (1973). Inorganic reactions. Nat. Phys. Sci. 241, 88. doi:10.1038/physci241088a0
Brugmann, G. E., Naldrett, A. J., and Macdonald, A. J. (1989). Magma mixing and constitution zone refining in the lac des iles complex, ontario; genesis of platinum-group element mineralization. Econ. Geology. 84 (6), 1557–1573. doi:10.2113/gsecongeo.84.6.1557
Chen, D. Z., Wang, J. G., Qing, H. R., Yan, D. T., and Li, R. W. (2009). Hydrothermal venting activities in the early Cambrian, South China: petrological, geochronological and stable isotopic constraints. Chem. Geology. 258 (3–4), 168–181. doi:10.1016/j.chemgeo.2008.10.016
Clark, S. H. B., Poole, F. G., and Wang, Z. (2004). Comparison of some sediment-hosted, stratiform barite deposits in China, the United States, and India. Ore Geol. Rev. 24 (1-2), 85–101. doi:10.1016/j.oregeorev.2003.08.009
Coveney, R. M. (2003). Re-Os dating of polymetallic ni-mo-pge-au mineralization in lower cambrian black shales of south china and its geological significance-A discussion. Econ. Geology. 98 (3), 661–662. doi:10.2113/98.3.661
Crocket, J. H (1990). Noble metals in seafloor hydrothermal mineralization from the Juan de Fuca and Mid-Atlantic Ridges: a fractionation of gold from platinum metals in hydrothermal fluids. Canadian Mineralogist 28, 636–648.
Dai, C. G., Chen, J. S., and Wang, X. H. (2014). A series of geological maps of Guizhou Province and a comprehensive study of the new generation of regional geological records of Guizhou Province. Management Res. Scientific Technol. Achievements 5, 50–55.[in Chinese]. doi:10.3772/j.issn.1673-6516.2014.05.017
Distler, V. V., Yudovskaya, M. A., Mitrofanov, G. L., Prokof'ev, V. Y., and Lishnevskii, E. N. (2004). Geology, composition, and genesis of the Sukhoi Log noble metals deposit, Russia. Ore Geology. Rev. 24, 7–44. doi:10.1016/j.oregeorev.2003.08.007
Duan, J., Li, C., Qian, Z., Jiao, J., Ripley, E. M., and Feng, Y. (2016). Multiple S isotopes, zircon Hf isotopes, whole-rock Sr-Nd isotopes, and spatial variations of PGE tenors in the Jinchuan Ni-Cu-PGE deposit, NW China. Miner Deposita 51 (4), 557–574. doi:10.1007/s00126-015-0626-8
Evans, N. J., Gregoire, D. C., Goodfellow, W. D., I. McInnes, B., Miles, N., and Veizer, J. (1993). Ru/Ir ratios at the Cretaceous-Tertiary boundary: implications for PGE source and fractionation within the ejecta cloud. Geochimica Et Cosmochimica Acta 57 (13), 3149–3158. doi:10.1016/0016-7037(93)90299-c
Fan, D. L., Yang, R. Y., and Huang, Z. X. (1984). “The Lower Cambrian black shale series and iridium anomaly in south China,” in Developments in geoscience, 27th international geological congress (Moscow: Beijing Science Press), 215–224.
Fan, D. L., Ye, J., and Liu, T. B. (1992). Black shale series-hosted silver-vanadium deposits of the upper Sinian Doushantuo formation, western Hubei province, China. Exploration Mining Geology. 1 (1), 29–38.
Fan, D. L., Ye, J., Yang, R. Y., and Huang, Z. X. (1987). The geological events and ore mineralization nearby the Precambrian-Cambrian boundary in Yangtze platform. Acta Sedimentol. Sinica 5 (3), 81–96. [in Chinese with English abstract].
Fu, X., Wang, J., Zeng, Y., Tan, F., and He, J. (2010). Concentrations and modes of occurrence of platinum group elements in the Shengli river oil shale, northern Tibet, China. Fuel 89 (12), 3623–3629. doi:10.1016/j.fuel.2010.07.043
Gammons, C. H., and Bloom, M. S. (1993). Experimental investigation of the hydrothermal geochemistry of platinum and palladium: II. The solubility of PtS and PdS in aqueous sulfide solutions to 300°C. Geochimica et Cosmochimica Acta 57, 2451–2467. doi:10.1016/0016-7037(93)90409-p
Gammons, C. H., Bloom, M. S., and Yu, Y. (1992). Experimental investigation of the hydrothermal geochemistry of platinum and palladium: I. Solubility of platinum and palladium sulfide minerals in NaCl/H2SO4 solutions at 300°C. Geochimica Et Cosmochimica Acta 56 (11), 3881–3894. doi:10.1016/0016-7037(92)90003-2
Gao, P., He, Z., Li, S., Lash, G. G., Li, B., Huang, B., et al. (2018). Volcanic and hydrothermal activities recorded in phosphate nodules from the Lower Cambrian Niutitang Formation black shales in South China. Palaeogeogr. Palaeoclimatol. Palaeoecol. 505 (15), 381–397. doi:10.1016/j.palaeo.2018.06.019
Griffith, E. M., and Paytan, A. (2012). Barite in the ocean - occurrence, geochemistry and palaeoceanographic applications. Sedimentology 59 (6), 1817–1835. doi:10.1111/j.1365-3091.2012.01327.x
Halbach, P., Kriete, C., Prause, B., and Puteanus, D. (1989). Mechanisms to explain the platinum concentration in ferromanganese seamount crusts. Chem. Geology. 76 (1-2), 95–106. doi:10.1016/0009-2541(89)90130-7
Han, T., Fan, H., Zhu, X., Wen, H., Zhao, C., and Xiao, F. (2017). Submarine hydrothermal contribution for the extreme element accumulation during the early Cambrian, South China. Ore Geology. Rev. 86, 297–308. doi:10.1016/j.oregeorev.2017.02.030
Han, T., Zhu, X., Li, K., Jiang, L., Zhao, C., and Wang, Z. (2015). Metal sources for the polymetallic Ni-Mo-PGE mineralization in the black shales of the Lower Cambrian Niutitang formation, South China. Ore Geology. Rev. 67, 158–169. doi:10.1016/j.oregeorev.2014.11.020
Hodge, V. F., Stallard, M., Koide, M., and Goldberg, E. D. (1985). Platinum and the platinum anomaly in the marine environment. Earth Planet. Sci. Lett. 72 (2-3), 158–162. doi:10.1016/0012-821x(85)90002-0
Jewell, P. W. (2000). Bedded barite in the geologic record. Mar. Authigenesis: Glob. Microb. 66, 147–161. doi:10.2110/pec.00.66.0147
Jiang, S.-Y., Chen, Y.-Q., Ling, H.-F., Yang, J.-H., Feng, H.-Z., and Ni, P. (2006). Trace- and rare-earth element geochemistry and Pb-Pb dating of black shales and intercalated Ni-Mo-PGE-Au sulfide ores in Lower Cambrian strata, Yangtze Platform, South China. Miner Deposita 41, 453–467. doi:10.1007/s00126-006-0066-6
Jiang, S.-Y., Yang, J.-H., Ling, H.-F., Chen, Y.-Q., Feng, H.-Z., Zhao, K.-D., et al. (2007). Extreme enrichment of polymetallic Ni-Mo-PGE-Au in Lower Cambrian black shales of South China: an Os isotope and PGE geochemical investigation. Palaeogeogr. Palaeoclimatol. Palaeoecol. 254, 217–228. doi:10.1016/j.palaeo.2007.03.024
Jiang, S., Yang, J., Ling, H., Feng, H., Chen, Y., and Chen, J. (2003). Re-Os isotopes and PGE geochemistry of black shales and intercalated Ni-Mo polymetallic sulfide bed from the Lower Cambrian Niutitang Formation, South China. Prog. Nat. Sci. 13, 788–794. [in Chinese with English abstract]. doi:10.1080/10020070312331344440
Jiang, S. Y., Wen, H J., Xu, C., Wang, Y., Su, H. M., and Sun, W. D. (2019). Earth sphere cycling and enrichment mechanism of critical metals: major scientific issues for future research. Bull. Natl. Nat. Sci. Found. China 33 (2), 10–16. (in Chinese with English abstract). doi:10.16262/j.cnki.1000-8217.2019.02.003
Koide, M., Goldberg, E. D., Niemeyer, S., Gerlach, D., Hodge, V., Bertine, , et al. (1991). Osmium in marine sediments. Geochimica et Cosmochimica Acta 55, 1641–1648. doi:10.1016/0016-7037(91)90135-R
Kucha, H. (1982). Platinum-group metals in the Zechstein copper deposits, Poland. Econ. Geology. 77 (6), 1587–1591. doi:10.2113/gsecongeo.77.6.1578
Kucha, H., and Przylowicz, W. (1999). Noble metals in organic matter and clay-organic matrices, kupferschiefer, Poland. Econ. Geology. 94 (7), 1137–1162. doi:10.2113/gsecongeo.94.7.1137
Lehmann, B., Mao, J. W., Li, S. R., Zhang, G. D., and Zeng, M. G. (2003). aRe-Os dating of polymetallic Ni-Mo-PGE-Au mineralization in lower cambrian black shales of south china and its geological significance--A reply. Econ. Geology. 98, 663–665. doi:10.2113/98.3.663
Lehmann, B., Nägler, T. F., Holland, H. D., Wille, M., Mao, J., Pan, J., et al. (2007). Highly metalliferous carbonaceous shale and Early Cambrian seawater. Geol 35, 403–406. doi:10.1130/g23543a.1
Li, C., Zhang, Z., Jin, C., Cheng, M., Wang, H., Huang, J., et al. (2020). Spatiotemporal evolution and causes of marine euxinia in the early Cambrian Nanhua Basin (South China). Palaeogeogr. Palaeoclimatol. Palaeoecol. 546, 109676. doi:10.1016/j.palaeo.2020.109676
Li, S., and Gao, Z. (2000). Source tracing of noble metal elements in Lower Cambrian black rock series of Guizhou-Hunan Provinces, China. Sci. China Ser. D-earth Sci. 43, 625–632. doi:10.1007/bf02879506
Li, S., Xiao, Q. Y., Shen, J. F., Sun, L., Liu, B., Yan, B. K., et al. (2003). Rhenium-osmium isotope constraints on the age and source of the platinum mineralization in the lower Cambrian black rock series of Hunan-Guizhou provinces, China. Sci. China Ser. D 46 (9), 919–927. doi:10.1360/01yd0277
Liang, Q.-L., Song, X.-Y., Wirth, R., Chen, L.-M., and Dai, Z.-H. (2019). Implications of nano- and micrometer-size platinum-group element minerals in base metal sulfides of the Yangliuping Ni-Cu-PGE sulfide deposit, SW China. Chem. Geology. 517, 7–21. doi:10.1016/j.chemgeo.2019.04.015
Lu, Z. C., Liu, C. Q., Liu, J. J., and Wu, F. C. (2004). Geochemical studies on the lower Cambrian witherite-bearing cherts in the northern daba mountains. Acta Geol. Sin. 78 (3), 390–406. [in Chinese with English abstract]. doi:10.3321/j.issn:0001-5717.2004.03.013
Luo, T. Y., Zhang, H., and Li, X. B. (2003). Mineralization characteristics of the multi-element-rich strata in the Niutitang formation black shale series, Zunyi, Guizhou, China. Acta Mineralogica Sin. 23 (4), 296–302. [in Chinese with English abstract]. doi:10.3321/j.issn:1000-4734.2003.04.002
Mao, J. W., Zhang, G. D., Du, A. D., Wang, T. Y., and Zeng, M. G. (2001). Geology, geochemistry, and Re-Os isotopic dating of the Huangjiawan Ni-Mo-PGE deposit, Zunyi, Guizhou province-with a discussion of the polymetallic mineralization of basal cambrian black shales in South China. Acta Geol. Sin. 35 (3), 454–472. [in Chinese with English abstract]. doi:10.3321/j.issn:0001-5717.2001.02.013
Mao, J., Lehmann, B., Du, A., Zhang, G., Ma, D., Wang, Y., et al. (2002). Re-Os dating of polymetallic Ni-Mo-PGE-Au mineralization in lower cambrian black shales of South China and its geologic significance. Econ. Geol. 97, 1051–1061. doi:10.2113/gsecongeo.97.5.1051
Mcdonough, W. F., and Sun, S. S. (1995). The composition of the earth. Chem. Geol. 120 (3-4), 223–253. doi:10.1016/0009-2541(94)00140-4
Mountain, B. W., and Wood, S. A. (1988). Chemical controls on the solubility, transport and deposition of platinum and palladium in hydrothermal solutions; a thermodynamic approach. Econ. Geol. 83 (3), 492–510. doi:10.2113/gsecongeo.83.3.492
Mountain, R. D. (1989). Molecular dynamics investigation of expanded water at elevated temperatures. J. Chem. Phys. 90 (3), 1866–1870. doi:10.1063/1.456028
Nozaki, Y. (1997). Updated (1996 version) table of the elements in seawater and its remarks. Bull. Soc. Sea Water Sci. Jpn. 51, 302–308. doi:10.11457/swsj1965.51.302
Orberger, B., Pasava, J., Gallien, J. P., Daudin, L., and Pinti, D. L. (2003). Biogenic and abiogenic hydrothermal sulfides: controls of rare metal distribution in black shales (Yukon territories, Canada). J. Geochem. Explor. 78 (1–2), 559–563. doi:10.1016/s0375-6742(03)00141-9
Paava, J., Chrastn, V., Loukola, R. K., and Ebek, O. (2019). Nickel isotopic variation in black shales from bohemia, China, Canada, and Finland: a reconnaissance study. Mineralium Deposita 54 (5), 719–742. doi:10.1007/s00126-018-0839-8
Pagès, A., Barnes, S., Schmid, S., Coveney, R. M., Schwark, L., Liu, W., et al. (2018). Geochemical investigation of the lower Cambrian mineralised black shales of South China and the late Devonian Nick deposit, Canada. Ore Geology. Rev. 94, 396–413. doi:10.1016/j.oregeorev.2018.02.004
Pašava, J., Barnes, S. J., and Vymazalova, A. (2003). The use of mantle normalization and metal ratios in the identification of the sources of platinum-group elements in various metal-rich black shales. Miner. Deposita 38, 775–783. doi:10.1007/s00126-003-0366-z
Pašava, J., Kříbek, B., Vymazalová, A., Sýkorová, I., Žák, K., and Orberger, B. (2008). Multiple sources of metals of mineralization in Lower Cambrian black shales of South China: evidence from geochemical and petrographic study. Resour. Geol. 58, 25–42. doi:10.1111/j.1751-3928.2007.00042.x
Pašava, J., Vymazalova, A., Petersen, S., and Herzig, P. (2004). PGE distribution in massive sulfides from the PACMANUS hydrothermal field, eastern Manus basin, Papua New Guinea: implications for PGE enrichment in some ancient volcanogenic massive sulfide deposits. Miner. Deposita 39, 784–792. doi:10.1007/s00126-004-0442-z
Pašava, J., ymazalová, A. V., Košler, J., Koneev, R. I., Jukov, A. V., and Khalmatov, R. A. (2010). Platinum-group elements in ores from the kalmakyr porphyry Cu-Au-Mo deposit, Uzbekistan: bulk geochemical and laser ablation ICP-MS data. Miner. Deposita 45 (5), 411–418. doi:10.1007/s00126-010-0286-7
Paytan, A., Mearon, S., Cobb, K., and Kastner, M. (2002). Origin of marine barite deposits: Sr and S isotope characterization. Geology 30 (8), 747–750. doi:10.1130/0091-7613(2002)030<0747:oombds>2.0.co;2
Puteanus, D., and Halbach, P. (1988). Correlation of co concentration and growth rate-a method for age determination of ferromanganese crusts. Chem. Geol. 69 (1-2), 73–85. doi:10.1016/0009-2541(88)90159-3
Qi, L., Gao, J., Huang, X., Hu, J., Zhou, M.-F., and Zhong, H. (2011). An improved digestion technique for determination of platinum group elements in geological samples. J. Anal. Spectrom. 26 (9), 1900–1904. doi:10.1039/c1ja10114e
Qi, L., Wang, C. Y., and Zhou, M.-F. (2008). Controls on the PGE distribution of permian emeishan alkaline and peralkaline volcanic rocks in longzhoushan, sichuan province, SW China. Lithos 106, 222–236. doi:10.1016/j.lithos.2008.07.012
Ravizza, G., Blusztajn, J., and Prichard, H. M. (2001). Re-Os systematics and platinum-group element distribution in metalliferous sediments from the Troodos ophiolite. Earth Planet. Sci. Lett. 188 (3-4), 369–381. doi:10.1016/s0012-821x(01)00337-5
Rehkämper, M., Halliday, A. N., Fitton, J. G., Lee, D.-C., Wieneke, M., and Arndt, N. T. (1999). Ir, Ru, Pt, and Pd in basalts and komatiites: new constraints for the geochemical behavior of the platinum-group elements in the mantle. Geochi. et Cosmochim. Acta 63 (22), 3915–3934. doi:10.1016/s0016-7037(99)00219-7
Sarwar, A. R., Liu, C., Gong, H., Dun, C., and Chamssidini, L. G. (2019). Paleo-sedimentary environment in relation to enrichment of organic matter of early cambrian black rocks of niutitang formation from Xiangxi area China. Mar. Pet. Geology. 112, 104057. doi:10.1016/j.marpetgeo.2019.104057
Sharma, M., Papanastassiou, D. A., and Wasserburg, J. G. (1997). The concentration and isotopic composition of Os in the oceans. Geochimica et Cosmochimica Acta 61 (16), 3287–3299. doi:10.1016/S0016-7037(97)00210-X
Shi, C. H., Cao, J., Han, S. C., Hu, K., Bian, L. Z., and Yao, S. P. (2020). A review of polymetallic mineralization in Lower Cambrian black shales in South China: combined effects of seawater, hydrothermal fluids, and biological activity. Palaeogeogr. Palaeoclimatol. Palaeoecol. 561, 110073. doi:10.1016/j.marpetgeo.2019.104057
Steiner, M., Wallis, E., Erdtmann, B. D., Zhao, Y., and Yang, R. (2001). Submarine-hydrothermal exhalative ore layers in black shales from South China and associated fossils-insights into a lower Cambrian facies and bio-evolution. Palaeogeogr. Palaeoclimatol. Palaeoecol. 169 (3-4), 165–191. doi:10.1016/s0031-0182(01)00208-5
Sun, T., Qian, Z. Z., Tang, Z. L., Jiang, C. Y., He, K., Sun, , et al. (2010). Zircon U-Pb chronology, platinum group element geochemistry characteristics of Hulu Cu-Ni deposit, east Xinjiang, and its geological significance. Acta Petrologica Sinica 26 (11), 3339–3349. (in Chinese with English abstract). doi:10.1130/G30929C.1
Sun, X. M., Xue, T., He, G. W., Ye, X. R., Zhang, M., Lu, H. F., et al. (2006). Noble gases isotopic compositions and sources of cobalt-rich crusts from west Pacific Ocean seamounts. Acta Petrologica Sin. 22 (9), 2331–2340. [in Chinese with English abstract]. doi:10.3969/j.issn.1000-0569.2006.09.008
Tao, Y., Li, C. S., Hu, R. Z., Ripley, E. M., Du, A. D., and Zhong, H. (2007). Petrogenesis of the Pt -Pd mineralized Jinbaoshan ultramafic intrusion in the Permian Emeishan large igneousprovince, SW China. Contrib. Mineral. Petrol. 153 (3), 321–337. doi:10.1007/s00410-006-0149-5
Wang, C. Y., Zhou, M. F., and Qi, L. (2010). Origin of extremely PGE-rich mafic magma system: an example from the Jinbaoshan ultramafic sill, Emeishan large igneous province, SW China. Lithos 119 (1-2), 147–161. doi:10.1016/j.lithos.2010.07.022
Wang, J., Chen, D., Wang, D., Yan, D., Zhou, X., and Wang, Q. (2012). Petrology and geochemistry of chert on the marginal zone of Yangtze Platform, western Hunan, South China, during the Ediacaran-Cambrian transition. Sedimentology 59, 809–829. doi:10.1111/j.1365-3091.2011.01280.x
Wang, M., Sun, X. M., and Yang, M. M. (2004). Geochemistry of ore-forming fluid and its metallogenetic significances of PGE-polymetallic deposits in Lower Cambrian black rock series, Southern China. Acta Scientiarum Naturalium Universitatis Sunyatseni 43 (5), 98–102. [in Chinese with English abstract]. doi:10.3321/j.issn:0529-6579.2004.05.028
Wang, Z. C., and Li, G. Z. (1991). Barite and witherite deposits in lower Cambrian shales of south China: stratigraphic distribution and geochemical characterization. Econ. Geol. 86 (2), 354–363. doi:10.2113/gsecongeo.86.2.354
Wu, C. D., Chen, Q. Y., and Lei, J. J. (1999). The genesis factors and organic petrology of black shale series from the upper Sinian to the lower Cambrian, southwest of China. Acta Petrologica Sin. 15 (3), 453–462. [in Chinese with English abstract]. doi:10.3969/j.issn.1000-0569.1999.03.014
Wu, C. D., Shen, Y. P., and Hou, Q. L. (2001). Geochemical characteristics and enrichment factors of platinum metals in black shales, West Hunan, China. Prog. Nat. Sci. 11 (5), 507–513. [in Chinses]. doi:10.3321/j.issn:1002-008X.2001.05.010
Xing, L., Zhou, M., Qi, L., and Huang, Z. (2015). Discussion on the PGE anomalies and source materials of K-bentonite (bed 5) in the lower cambrian meishucun section, yunnan. Chin. J. Geochem. 34 (3), 346–361. doi:10.1007/s11631-015-0057-3
Xu, L. G., Lehmann, B., and Mao, J. W. (2012). Seawater contribution to polymetallic Ni-Mo-PGE-Au mineralization in Early Cambrian black shales of South China: evidence from Mo isotope, PGE, trace element, and REE geochemistry. Ore Geol. Rev. 52, 66–84. doi:10.1016/j.oregeorev.2012.06.003
Xu, L., Lehmann, B., and Mao, J. (2013). Seawater contribution to polymetallic Ni-Mo-PGE-Au mineralization in early Cambrian black shales of South China: evidence from Mo isotope, PGE, trace element, and REE geochemistry. Ore Geol. Rev. 52, 66–84. doi:10.1016/j.oregeorev.2012.06.003
Yang, R. D., Wei, H. R., Bao, M., and Wang, W. (2007). Submarine hydrothermal venting—flowing sedimentary characters of the Cambrian Shanggongtang and Dahebian barite deposits, Tianzhu county, Guizhou province. Geol. Rev. 53 (5), 675–680. [in Chinese with English abstract]. doi:10.3321/j.issn:0371-5736.2007.05.012
Yao, D., Zhang, L. J., Wiltshire, J. C., Chu, F. Y., Du, A. D., Liu, X. Z., et al. (2002). PGE and Re-Os isotope compositions and their significances of Co-rich ferromanganese crusts. Mar. Geol. Quat. Geol. 22 (3), 53–58. [in Chinese with English abstract]. doi:10.16562/j.cnki.0256-1492.2002.03.008
Yu, B., Chen, J. Q., Li, X. W., and Lin, C. S. (2003). Geochemistry of black shale at the bottom of the Lower Cambrian in Tarim Basin and its significance for lithosphere evolution. Sci. China Ser. D 46 (5), 498–507. doi:10.1360/03yd9043
Zhou, X. Q., Yu, H., Huang, T. Y., Zhang, L. Y., Zhang, G. J., Fu, Y., et al. (2016). Genetic classification of sedimentary barites and discussion on the origin of the lower Cambrian barite-rich deposits in the Yangtze block, south China. Acta Sedimentol. Sin. 34 (6), 1044–1056. [in Chinese with English abstract]. doi:10.14027/j.cnki.cjxb.2016.06.004
Keywords: enrichment, black shale, platinum group element, lower cambrian, south China
Citation: Fu Y, Yang Z, Li C and Xia P (2021) Enrichment of Platinum Group Elements in Lower Cambrian Polymetallic Black Shale, SE Yangtze Block, China. Front. Earth Sci. 9:651948. doi: 10.3389/feart.2021.651948
Received: 11 January 2021; Accepted: 11 February 2021;
Published: 18 March 2021.
Edited by:
Xiaohua Deng, Beijing Institute of Geology for Mineral Resources, ChinaReviewed by:
Yiguan Lu, Tianjin Center, China Geological Survey, ChinaYayun Liang, University of Science and Technology Beijing, China
Copyright © 2021 Fu, Yang, Li and Xia. This is an open-access article distributed under the terms of the Creative Commons Attribution License (CC BY). The use, distribution or reproduction in other forums is permitted, provided the original author(s) and the copyright owner(s) are credited and that the original publication in this journal is cited, in accordance with accepted academic practice. No use, distribution or reproduction is permitted which does not comply with these terms.
*Correspondence: Yong Fu, byez1225@126.com; Zhen Yang, cugbyzh@163.com