- 1Faculty of Environmental Science and Engineering, Babes-Bolyai University, Cluj-Napoca, Romania
- 2Department of Physics and Geology, University of Perugia, Perugia, Italy
- 3Department of Geology, Babeş-Bolyai University, Cluj-Napoca, Romania
- 4Romanian Association of Hydrogeologists, Bucuresti, Romania
The Apuseni Mountains are located between the large geothermal area of the Pannonian Basin and the low thermal flux Transylvanian Basin. Thermal and mineral waters have been sampled from 42 points along a NW-SE transect. The general chemistry and the water isotope (deuterium and oxygen-18) composition were analyzed. Most of the thermal aquifers are located in carbonate reservoirs. The waters mainly belong to the Ca-HCO3 hydrochemical type, excepting the western side, towards the Pannonian Basin, where the Na-HCO3 type may occur. The isotope composition indicates aquifer recharge from precipitation. The geochemical characteristics and the structural position of the study area suggest two distinct geothermal contexts. The Southern Apuseni area geothermal features are likely connected to the Neogene—Early Quaternary magmatic activity from the Mureş Valley and Zarand Depression. The geothermal manifestations in the north-western part of the study area, at the border between the Northern Apuseni and the Pannonian Depression, share features of the latter one.
Introduction
The mineral and thermal waters from the Apuseni Mountains have been less studied by respect to other areas in Romania, although their resources are not negligible. Some of the thermal sources, as Geoagiu (Germisara) and Călan (Ad Aquas), are known since the Roman times (Fodorean, 2012). However, the geochemical information able to deepen our understanding on the geothermal potential and the genesis of the thermal and mineral waters in the Apuseni area are scarce.
The Apuseni Mountains are bordered towards the west by the Romanian part of the Pannonian Basin, an extensive unit well known for its rich geothermal resources (e.g.,: Horvath et al., 2006; Szocs et al., 2018; Rman et al., 2019). On the opposite side, the Transylvanian Basin is recognized as having low heat flow compared to the Pannonian Basin, but comparable values to other European areas (Demetrescu and Andreescu, 1994; Tiliţă et al., 2018).
Information about the mineral and thermal waters from the Apuseni Mts. is available in general works regarding the Romanian mineral waters (e.g.,: Institutul de Balneologie şi Fizioterapie (IBF), 1970; Pricajan, 1972; Pricăjan and Airinei, 1981). Groundwater with temperatures higher than 20°C is generally considered as thermal water. A series of relevant contributions to the hydrogeological description of the Apuseni Mts., especially of the karst areas, were authored by Orăşeanu, here including also mineral and thermal waters (e.g., Orăşeanu, 1987; Orăşeanu and Mather, 2000), and synthesized by Orăşeanu (2016, 2020). The thermal waters are used or have been used for bathing purposes in resorts as Băile Felix, Geoagiu, Moneasa, Vaţa, Călan. Carbonated waters are bottled in Boholt, Băcâia, and Chimindia.
The present contribution aims to extend the current knowledge on the occurrence and characteristics of the mineral and thermal waters in the Apuseni Mts. area, by adding a set of hydrogeochemical data that includes chemical and isotopic analyses. These results will help in better constraining the thermal features of the study region. Water samples from 42 locations along a NW-SE transect were collected. Groundwater sources with temperatures between 10 and 20°C, although not corresponding to the definition of thermal water, were included in this study as indicators of the geothermal influence. The analytical results are interpreted by geochemical methods, and an assessment of the origin and geothermal context of the investigated waters is presented, correlated with the geological setting of the study area.
Geological Setting
The Apuseni Mountains represent a segment of the Carpathian orogen, with an inner position relative to the main ridge. They are located between two connected Neogene sedimentary basins, the Pannonian Basin to the west, and the Transylvanian Basin to the east. Although the Apuseni Mts. have the appearance of an island, their structure continues beneath the sediments of the two basins.
The basement of the Pannonian Basin consists of two entities with different origin and evolution, the ALCAPA and Tisza/Tisia mega-units, separated by the Mid Hungarian fault zone (Schmid et al., 2020). The ALCAPA block, originated from the Adria realm, comprises the Austroalpine unit of the Alps and internal units of the Western Carpathians. A significant part of it, representing the basement of the north-western part of the Pannonian Basin, is covered by Neogene sediments. Tisza mega-unit is a Europe-derived block, which forms the basement of the south-eastern side of the Pannonian Basin, being visible in the Mecsek Mts. in Hungary, or the Slavonian Hills in Croatia. The largest outcropping area of the Tisia block corresponds to the Northern Apuseni Mts. (Inner Dacides, after Săndulescu, 1984), where the upper two units of this block, the Bihor and Codru nappe systems are exposed. These units generally consist of an Early Proterozoic—Variscan basement, basement-cover and pre-Alpine cover nappes (Seghedi, 2004).
The Bihor unit consists of medium-grade crystalline rocks, with granitic intrusions, covered by Permian—Mesozoic detrital and carbonate sedimentary formations (Săndulescu, 1984). The lithologic composition of the Codru unit is dominated by Permian—Mesozoic sedimentary formations, with Permian volcanics and few low- or medium-grade metamorphic elements underlying some of the nappes.
A north-vergent thrusting shear zone, corresponding to the Biharia unit, separates the North and South Apuseni Mts. (Seghedi, 2004). It mainly consists of medium-grade metamorphic rocks. The Biharia nappe system belongs to the Dacia mega-unit, as an equivalent of the Supragetic nappe system from the Southern Carpathians (Schmid et al., 2008, 2020), and overthrusts the Bihor and Codru units.
The South Apuseni segment is the remnant of a branch of Neotethys, the Eastern Vardar mobile area. It mainly consists of ophiolitic units, placed over the margin of the Dacia block during the Upper Jurassic (Schmid et al., 2008). They are the result of an intra-oceanic back-arc basin that persisted a very short period, between the Middle Jurassic and Late Kimmeridgian to Tithonian. The closure of the basin is marked by the obduction of the ophiolitic units on the Biharia nappe system (Schmid et al., 2020). The ophiolitc complex is represented by Middle Jurassic tholeites and Late Jurassic to Early Cretaceous calc-alkaline magmatic rocks (Mutihac, 1990). An intensive Late Jurassic to Late Cretaceous sedimentation has accompanied and followed the ophiolitic magmatism. Two types of sediments dominate: the reef limestone (Malm to Lower Cretaceous), and flysch-type detrital units.
The Study Area
Two distinct areas have been considered within the study region, which show different geological, hydrogeological, and structural features. The southern area corresponds to the depression between the Apuseni Mts. and the Southern Carpathians, filled with Neogene-Quaternary deposits along the Mureş and Strei Rivers, and to the southern part of the Apuseni Mountains. The northern area corresponds to the Northern Apuseni structural units, including also the Neogene depressions on the western side of the mountain range (Figure 1).
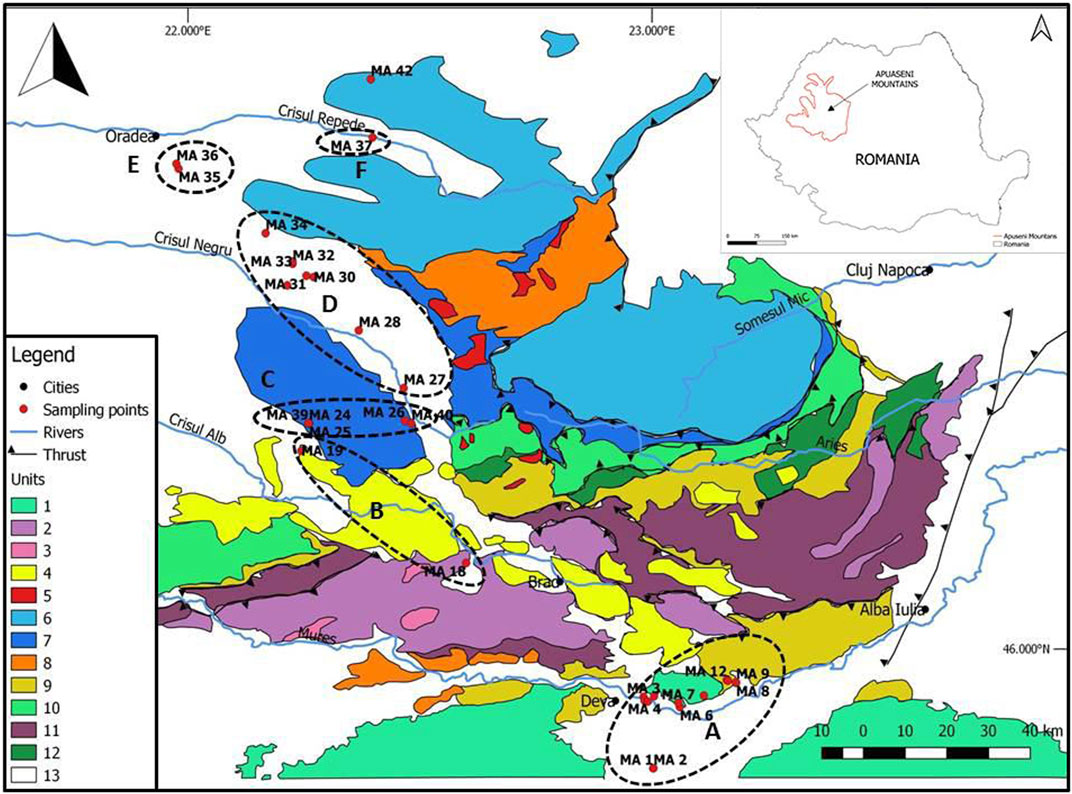
FIGURE 1. The general geological structure of the Apuseni Mts. and distribution of the investigated areas (map modified after Kounov and Schmid, 2013; Gallhofer et al., 2017). 1. Supragetic, getic nappes; 2. Ophiolites (mainly Jurassic); 3. Jurassic granitoids; 4. Neogene magmatic rocks; 5. Late Cretaceous intrusions; 6. Bihor nappe; 7. Codru nappe system; 8. Late Cretaceous volcanics; 9. Gosau-type sediments; 10. Highiş-Poiana, Biharia, Muncel-Lupşa nappes; 11. Early Cretaceous flysch; 12. Baia de Arieş nappe; 13. Paleogene to recent sedimentary units; A—Mureş area; B—Zarand basin; C—Moneasa-Vaşcău; D—Beiuş Depression; E—Felix area; F—Vad-Borod Depression.
Within the southern area (A), two distinct subareas have been investigated. Rapolt subarea corresponds to the crystalline isolated massif of Rapolt and its surroundings, extending southward along Strei River. Its southern part is represented by a flat area filled with Neogene-Quaternary sediments. The northern part includes the Rapolt crystalline body, the Măgura Uroiului shoshonitic body (Seghedi et al., 2011), and the surrounding sedimentary areas that host geothermal sources. The following sources have been investigated within this subarea: Călan, Chimindia, Banpotoc, Rapolţel, Feredee, Rovina, Nătău, Geoagiu.
Zarand subarea (B) corresponds to a NW-SE oriented depression along Crişul Alb River, within the Southern Apuseni Mts, narrower in the SE (Brad area), and extending gradually to the Pannonian Basin. This intramountainous area is filled with Neogene sediments penetrated by Neogene magmatic bodies (Pécskay et al., 1995; Roşu et al., 1997; Roşu et al., 2004; Seghedi and Downes, 2011). The investigated sources in Zarand subarea are Vaţa and Dezna.
Within the Northern Apuseni, four subareas have been separated. Moneasa (C), although geographically located very close to the northern edge of Zarand depression, shows features that confirm its belonging to the Northern Apuseni. Beiuş subarea (D) is a NW-SE oriented depression, filled with Neogene sediments, and extending along Crişul Negru River, widely opening to the Pannonian Depression. The following localities have been investigated: Ştei, Beiuş, Răbăgani, Coşdeni, Albeşti, Rotăreşti and Ceica. A similar but smaller depression occurs along Crişul Repede River, where Aleşd well was sampled (F). Baile Felix is located on a westward extension of the Mesozoic carbonate units to the Pannonian Depression (E). The northernmost investigated source is Pădurea Neagră (MA 42), laying on the northern edge of the Apuseni metamorphic units (Plopis Mts.).
Material and Methods
Three field campaigns for site investigation and sample collection were conducted—the southern area in October 2018, the northern area in February 2019, and samples 38 to 42 in November 2019 (Supplementary Table S1—Supplementary material). A total of 42 sampling points has been selected, 20 of them being located in the southern area, and 22 in the northern one (Figure 1). The coordinates and elevation of the sampling points are inserted in Supplementary Table S1. A GoogleEarth map showing the position of the sampling points is available as Supplementary Figure S1 in Supplementary material. The sampling sites were selected based on the water temperature at the outlet, considering that temperatures higher than 10°C may reveal a potential geothermal influence. The background information was mainly extracted from the works of Orăşeanu (2016, 2020) and Pricăjan (1972). The investigated physico-chemical parameters (temperature—T°C, pH, redox potential—Eh, and electrical conductivity—EC) were measured in situ, using a portable multiparameter instrument (WTW Multi 350i), which was previously calibrated using standard solutions. For the determination of alkalinity on site as HCO3, the titrimetric method with methylorange was applied, using a microdosimeter. The flow rate (Supplementary Table S1) was determined where possible as an average of more measurements, by measuring the filling time in calibrated vessels (Nicula et al., 2019).
Water samples for ion analysis have been collected in 250-ml pre-cleaned polyethylene bottles. The samples were filtered in the field by using a syringe and 0.45 µm Millipore™ filters, and then transported in cool boxes to the laboratory, where they have been stored at 4°C in the refrigerator.
Chemical analyses were performed in the Laboratories of the Faculty of Environmental Science and Engineering, Babeş-Bolyai University. A Dionex 1500 IC ion chromatography system was used for the dissolved ion analysis (F−, Cl−, Br−, NO2−, NO3−, SO42−, PO43−, Li+, Na+, K+, NH4+, Mg2+, and Ca2+).
The quantifications were performed based on the external standard method, using the calibration curves plotted for six standard solutions prepared by serial dilutions of the stock solutions: Dionex™ Combined Seven Anion Standard II/057590 and Dionex™ Combined Six Cation Standard-II/046070. The method proved to have a good linearity (R2 > 0.999) and low detection limits. The limits of detection (LoD) were: 10.3 μg/L (Li+), 12.2 μg/L (Na+), 11.9 μg/L (NH4+), 12.7 μg/L (K+), 11.8 μg/L (Mg2+), 17.1 μg/L (Ca2+), 10.1 μg/L (F−), 21.2 μg/L (Cl−), 12.4 μg/L (Br−), 11.4 μg/L (NO2−), 12.0 μg/L (NO3−), 15.8 μg/L (PO43−), and 12.7 μg/L (SO42−).
In order to quantify the amount of dissolved H2S as HS− ions, 10 ml of water were collected in falcon tubes containing 2 ml of ammonia-cadmium solution. For preparing the ammonia-cadmium solution, 0.5 mol of Cd(CH3COO)2 was dissolved in 225 ml of 30%v/v NH3 solution in 1 L of ultrapure (Milli-Q quality) water. At the final stage, the samples are prepared based on the methodology developed by Montegrossi et al. (2006), and measured by ion-chromatography as sulfate ions.
Distinct samples were collected for measuring the metal content. The samples were filtered in the field, using 0.45 µm syringe filters, and acidified to pH = 2 with HNO3 65% (Merck). The analyses were performed by atomic absorption spectrometry (AAS), by using a ZEEnit 700 system (Analytik Jena) equipped with air—acetylene burner and a specific hollow-cathode lamp for each metal. The calibration curves were plotted for six standard solutions prepared by serial dilutions of an ICP multi-element standard solution (1,000 mg/L, standard IV/111355, Merck). The method proved to have good linearity (R2 > 0.999). The detection limits for flame atomization were 12 μg/L (Fe and Cd), 35.0 μg/L (Cr), 38 μg/L (Ni), 13 μg/L (Zn), 83 μg/L (Pb), and 36 μg/L (Cu).
Mineral saturation indices (SI) for carbonates [calcite and aragonite—CaCO3, dolomite—CaMg(CO3)2 and siderite (FeCO3)], sulfates [gypsum—CaSO4·2H2O, anhydrite CaSO4, K-jarosite—KFe3 3(OH)6(SO4)2 and melanterite—FeSO4·7H2O], halides (fluorite—CaF2, halite—NaCl and sylvite—KCl) and iron oxides [goethite—α-FeO(OH), hematite—α-Fe2O3] minerals were used to predict the tendency for precipitation or dissolution of those minerals. A saturation index of 0 indicates thermodynamic equilibrium, while SI > 0 indicates oversaturation, and SI < 0 indicates undersaturation. Mineral saturation indices of waters from the study area were calculated at the in situ measured spring temperatures and pH using the PHREEQC computer program, with the default PHREEQC database (version 3, USGS, Denver, CO, United States). The most representative samples for each subarea have been selected.
For measuring the stable isotopes of water, the samples have been collected in 4-ml glass vials, and analyzed at the Babeş-Bolyai University Stable Isotope Laboratory using a Picarro L2130i cavity ring-down spectroscopy system. Detail on the method, calibration and errors are provided elsewhere (Wassenaar et al., 2013; Dumitru et al., 2017). The isotopic data are expressed using the standard notation, as δ (‰) = (Rsample/Rstandard –1) × 1,000. Internal laboratory standards calibrated with international standards VSMOW2 and VSLAP2 were used for data calibration. The measurement spectra were analysed for spectral contamination (organic interferences) using the ChemCorrect software after each measurement. None of the samples showed spectral contamination that can affect the isotopic measurement.
Results and Discussion
Physicochemical Features
The physicochemical parameters measured in the field are listed in Supplementary Table S1. The pH of the waters ranges between 6.16 (MA 42—Pădurea Neagră) and 8.80 (MA 18—Vaţa Băi 2). The waters collected from the southern area generally show lower pH than the samples from the northern area, reflecting the presence of CO2 in free and dissolved forms, in most of the samples. The main exception to this general trend is Pădurea Neagră, which shows the lowest pH, although located in the northern area, due to the CO2 content.
Many of the investigated water sources show temperature at the outlet between 20 and 30°C (19 out of 42 sources). This is the case of the sources from the areas of Călan, Rapolt (Banpotoc, Rapolţel, Rovina, and Nătău), Geoagiu, Moneasa, Răbăgani, and La Feredee (subarea A). Higher temperatures, between 30 and 40°C, were measured in six sources, at Geoagiu (one source), Dezna, Vaţa, Felix, and Aleşd. Temperatures above 40°C were recorded in only two deep wells (subarea D), from Ştei (44.5°C) and Beiuş (79.8°C). Fifteen of the investigated sources show temperatures between 11 and 20°C, however they were taken into account, as compared to the background temperatures, a geothermal influence is visible. Low electrical conductivities, below 500 μS/cm, were recorded in Moneasa area and in the sources located close to the Pannonian Basin, as Felix, Dezna, and Beiuş Basin. The temperature and electrical conductivity of the water released by drilled wells is generally higher than in the case of springs. The springs are likely influenced by the shallow infiltration water.
Water Chemistry and Isotopic Composition
The concentration of the main cations (Na+, K+, Li+, NH4+, Ca2+, and Mg2+) and anions (Cl−, F−, NO3−, HCO3−, and SO42−) are listed in Supplementary Table S2—Supplementary material. As revealed by the Piper diagram (Figure 2), the HCO3 water-type is dominant for most of the sampled waters. The cation composition is more variable, generally in the range of the Ca-Mg type. The Ca (Mg)—HCO3 water type is also dominant in the surface water and cold aquifers in the area. This situation is expected, taking into account that most of the aquifers are confined in carbonate reservoirs. Particular features show the waters from the western part of the investigated area, with affinities with the Pannonian Basin (Ceica, Rotăreşti, Coşdeni, and Albeşti), with a very peculiar Na-HCO3 type. This particular water type is common in the Pannonian Basin and it is generally explained by cation exchange and dissolution of minerals such as mica and feldspars (Rowland et al., 2011 and references therein). Different hydrochemical features by respect to the general trend are noticed in the case of the Mg-SO4 water from Ştei well (MA 27), and Na-SO4-Cl water from Vaţa Băi (MA 18). The total ionic salinity (TIS) plot (Supplementary Figure S2 Supplementary material) indicates relatively low salinities for most of the samples, mainly determined by the HCO3−-Cl− ion pair. It is visible the exception in the case of SO42−-rich MA 27 water. The MA 34 Ceica and MA 41 Chimindia samples, with high HCO3+Cl contents (55.06 and 34.10 meq/L, respectively), and low SO4 content, fall outside the plot. The AAS analysis has revealed metal concentrations (Cu, Cr, Ni, Pb, Zn, and Cd) below the detection limit in all the samples. The only metal with high concentrations is iron, that is detectable in all samples, and shows higher contents in the CO2-rich, sparkling waters, also revealed by the Fe-hydroxide yellow deposits around the source. The concentrations of iron were between 0.013 and 4.97 mg/L.
Lithium (Li+) was found in only two samples: MA 34 (1.06 mg/L) and MA 35 (0.01 mg/L). The sodium (Na+) concentrations were between 1.1 and 794.23 mg/L (standard deviation σ = 155.68). Potassium (K+) has shown concentrations between 0.02 and 55.59 mg/L (σ = 14.98). Calcium (Ca2+) and magnesium (Mg2+) are generally abundant in the analyzed waters, with concentrations between 4.02 and 649 mg/L (σ = 132.55) for calcium, and 0.22–545.33 mg/L (σ = 84.34) for magnesium. Ammonium (NH4+) was not identified in the analyzed samples (LoD = 11.9 μg/L NH4+).
The chloride (Cl−) and sulphate (SO42−) anions were identified in all the analyzed water samples, with concentrations between 1.31 and 260.38 mg/L for chloride (σ = 50.74), and sulphate concentrations between 0.37 and 875.17 mg/L (σ = 144.50). The fluoride (F−) concentrations were between 0.02 and 2.78 mg/L. Nitrate (NO3−) concentration has shown values between 0.04 and 160 mg/L, while nitrite (NO2−) was found in a single sample (0.61 mg/L in MA 17). Bromide (Br−) and phosphate (PO43−) concentrations were below the detection limit in all the investigated samples. Alkalinity measured as HCO3− varied in the range of 115.9 mg/L to 2,754.15 mg/L (σ = 766.87).
Some of the investigated sources contain gases in free or dissolved form. Orăşeanu (2020) reports the occurrence of gases in thermal and non-thermal waters in different units of the Apuseni Mts: Beiuş Basin—Vaşcău, Moneasa, Rapolt area, Călan, Bihor Mts., Pădurea Craiului Mts. In most of the cases, the composition of the gas mixtures is dominated by nitrogen, and the N2-O2 ratio suggests their atmospheric origin. Most of the sampling locations in the southern area (Călan, La Feredee, Geoagiu, and Chimindia), and some sites from the western part of the study area (Felix, Ceica, Beius), as well as Pădurea Neagră in the north, contain various proportions of CO2. High concentrations of methane were found on the western side, as free gas at Ceica, or dissolved with much lower concentrations at Chimindia, Geoagiu, Albeşti, and Rotăreşti. The presence of hydrocarbons in water in this area was also reported by Rowland et al. (2011), Ionescu et al. (2017) and Baciu et al. (2018).
Most of the samples contain small amounts of dissolved H2S. The highest amount was found in the Southern Apuseni area, and in some cases in the western part of Beiuş Depression, with the highest concentration in Ştei borehole (109.07 mg/L).
The saturation indices (SI) generally show slight oversaturation in aragonite, calcite and dolomite (Supplementary Table S3—Supplementary material). In the areas of Călan, Banpotoc, Rapolţel, and Geoagiu (Group A), where CO2 emissions are also present, this is also indicated by the presence of thick travertine deposits. Same tendency can be observed at Vaţa, Dezna, and in Beiuş and Vad-Borod depressions, but without travertine deposition. Undersaturation by respect to the carbonates has been found in Stei and Pădurea Neagră sites. Most of the samples show oversaturation in iron minerals goethite and hematite. Undersaturation by respect to sulphate minerals is the common case in the study area. The highest SI for sulphate minerals was found in Ştei well.
The stable isotope values, as presented in Figure 3, confirm the meteoric origin for all the investigated sources. In most of the cases, the values plot along the LMWL drawn by Cozma et al. (2017) for Roşia Montană, while another part are located on the LMWL drawn by Forizs et al. (2004) based on the isotopic composition of samples from Focul Viu ice cave. The correlation line intersects all three water lines (GMWL, Focul Viu, and Roşia Montană), indicating the possibility that the samples fall along this theoretical “mixing” line. Based on this we infer that the samples intersecting the Focul Viu line could be of a deeper circulation, “older water”, while the ones intersecting Roşia Montană LMWL could have a shallower, more rapid circulation. However, further analyses are needed to demonstrate this. The range of elevations of the sampling points is relatively narrow, spanning over 226 m (from 139 to 365 m. a.s.l.), thus the effect of elevation on the isotopic composition is expected to be less significant.
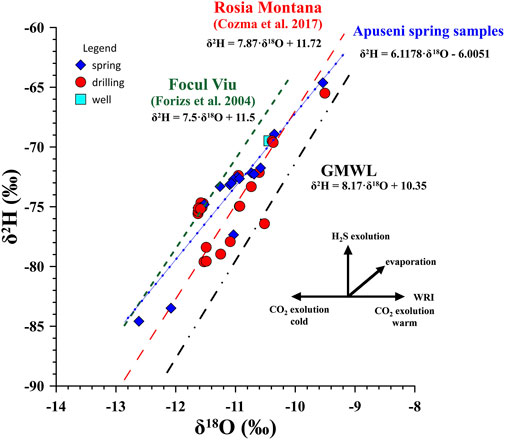
FIGURE 3. Water stable isotope composition of the sampled sources. Symbols as in Figure 2.
Based on GNIP precipitation data from IAEA (Nucleus server) for Romania, the average δ18O and δ2H is –8.91 and –63.08‰, respectively. These values are not significantly different from GNIR surface water data from IAEA (Nucleus server) for Romania (–9.90‰ and –70.60‰). The average of the δ18O and δ2H values in the case of the springs we investigated is –11.62‰ and –75.87‰, respectively. The limited number of samples and the lack of drilled wells water ages prevents us from further advancing in the interpretation of the isotopic data.
Within the same area, significant differences in the position of points along the LMWL may be observed, supporting the variability of the residence time for the concerned aquifers and the influence of other factors, as exsolution of CO2 from water exposed to atmospheric temperature and pressure, in the southern area (e.g., Geoagiu). Evaporation may also influence the results when the samples have been collected from open pools, as in the case of La Feredee and Călan, or when the water path through pipelines from the source to the sampling outlet is long, and contact with atmospheric air is possible.
The content and ratio of some indicative chemical compounds from geothermal waters may be indicative for the reservoir temperature. In the case of our particular study area, the Giggenbach ternary plot and several cation geothermometers have been used. In the Giggenbach ternary diagram (Figure 4), most of the points fall in the Immature waters field, also suggesting shallow circulation. The samples from Beiuş Depression plot in the partial equilibration field. They contain different amounts of hydrocarbons, as reported by Ionescu et al. (2017). The inferred equilibrium temperature based on the Giggenbach diagram is around 60°C for Beiuş Depression, excepting Ceica, which shows the highest amount of methane in solution but also as free gas, with an equilibrium temperature around 160°C.
Conclusion
In the Apuseni Mountains and the adjacent southern and western areas, the geothermal potential is revealed by several thermal water sources and localized emissions of CO2. The temperature of the investigated sources is low (hypothermal, between 20 and 30°C) in most of the cases, with the exception of the deep wells (2000 m deep), that may rise up to 80°C.
Chemical and isotopic analyses were performed on samples collected from 42 locations. The dominant hydrochemical type is Ca-HCO3. Only in the northern part of the study area (Beiuş Depression), at some points, the waters exhibit Na-HCO3 type. The isotopic measurements indicate recharging of the aquifers with meteoric water and a predominantly shallow circulation. The short time of water residence in the aquifer limits the processes of water-rock interaction. The geochemical features and the structural setting of the study area suggest two main heat sources for the thermal aquifers in the Apuseni Mts. The Southern Apuseni area hosts large volumes of Neogene igneous rocks, with the last episode of magmatic activity during the Early Pleistocene. Strong CO2 emissions occur in the area, possibly related to carbonate metamorphism. A more detailed geochemical investigation, currently in progress, will likely increase our understanding on the occurrence and nature of the heat sources in the investigated area.
The western part of the study area corresponds to the limit between the Apuseni Mts. and the Pannonian Depression, with its demonstrated high heat flux. The geochemical features of the investigated waters from this area suggest their affinity with the Pannonian geothermal region, expressed by the Na-HCO3 hydrochemical type, occurrence of dissolved hydrocarbons, and relatively high temperatures.
This study represents a preliminary approach of the hydrogeochemical and geothermal features of the Apuseni Mountains, an area that has the potential to yield further interesting results. Additional isotopic data are needed in order to better understand these thermal and mineral water systems. A geothermal model of the Apuseni Mts. region would greatly benefit from the geochemical data, based on compositional and isotopic analysis of fluids (water and gas), and accurate geothermometers.
Data Availability Statement
The original contributions presented in the study are included in the article/Supplementary Material, further inquiries can be directed to the corresponding author.
Author Contributions
A-MN, AI, and CB contributed to the conception and design of this study, writing the manuscript and field work; I-CP contributed to the sampling and fieldwork; CR contributed to the laboratory analyses; FLF contributed to the laboratory analyses and isotope data interpretation; and IO contributed to the fieldwork and literature data analysis. All authors contributed to manuscript revision, read and approved the submitted version.
Conflict of Interest
The authors declare that the research was conducted in the absence of any commercial or financial relationships that could be construed as a potential conflict of interest.
Publisher’s Note
All claims expressed in this article are solely those of the authors and do not necessarily represent those of their affiliated organizations, or those of the publisher, the editors and the reviewers. Any product that may be evaluated in this article, or claim that may be made by its manufacturer, is not guaranteed or endorsed by the publisher.
Acknowledgments
The authors acknowledge support from the Deep Energy and Reservoir and Fluxes communities of the Deep Carbon Observatory. We also thank the Editor of this special volume, and three reviewers, whose comments and suggestions greatly helped improve and clarify this manuscript.
Supplementary Material
The Supplementary Material for this article can be found online at: https://www.frontiersin.org/articles/10.3389/feart.2021.648179/full#supplementary-material
References
Baciu, C., Ionescu, A., and Etiope, G. (2018). Hydrocarbon Seeps in Romania: Gas Origin and Release to the Atmosphere. Mar. Pet. Geology. 89 (1), 130–143. doi:10.1016/j.marpetgeo.2017.06.015
Cozma, A., Baciu, C., Papp, D., Roşian, G., and Pop, C. (2017). Isotopic Composition of Precipitation in Western Transylvania (Romania) Reflected by Two Local Meteoric Water Lines. Carpathian J. Earth Environ. Sci. 12, 357–364.
Demetrescu, C., and Andreescu, M. (1994). On the Thermal Regime of Some Tectonic Units in a continental Collision Environment in Romania. Tectonophysics 230, 265–276. doi:10.1016/0040-1951(94)90140-6
Dumitru, O. A., Forray, F. L., Fornós, J. J., Ersek, V., and Onac, B. P. (2017). Water Isotopic Variability in Mallorca: A Path to Understanding Past Changes in Hydroclimate. Hydrol. Process. 31, 104–116. doi:10.1002/hyp.10978
Fodorean, F. (2012). “Spa” Vignettes in Tabula Peutingeriana. Travelling Ad Aquas: thermal Water Resources in Roman Dacia. Ephemeris Napocensis 22, 211–221.
Forizs, I., Kern, Z., Szanto, Z., Nagy, B., Palcsu, L., and Molnar, M. (2004). Environmental Isotopes Study on Perennial Ice in the Focul Viu Ice Cave, Bihor Mountains, Romania. Theor. Appl. Karstology 17, 61–69.
Gallhofer, D., von Quadt, A., Schmid, S. M., Guillong, M., Peytcheva, I., and Seghedi, I. (2017). Magmatic and Tectonic History of Jurassic Ophiolites and Associated Granitoids from the South Apuseni Mountains (Romania). Swiss J. Geosci. 110, 699–719. doi:10.1007/s00015-016-0231-6
Horváth, F., Bada, G., Szafián, P., Tari, G., Ádám, A., and Cloetingh, S. (2006). Formation and Deformation of the Pannonian Basin: Constraints from Observational Data. Geol. Soc. Lond. Mem. 32, 191–206. doi:10.1144/gsl.mem.2006.032.01.11
Institutul de Balneologie şi Fizioterapie (IBF) (1970). Apele Minerale Şi Namolurile Terapeutice Din Republica Populară Romînă (Mineral Waters and Therapeutic Muds from Romania), Vols. I–IV. Bucharest: Edit. Medic. in Romanian.
Ionescu, A., Baciu, C., Kis, B.-M., and Sauer, P. E. (2017). Evaluation of Dissolved Light Hydrocarbons in Different Geological Settings in Romania. Chem. Geology. 469, 230–245. doi:10.1016/j.chemgeo.2017.04.017
Kounov, A., and Schmid, S. M. (2013). Fission-track Constraints on the thermal and Tectonic Evolution of the Apuseni Mountains (Romania). Int. J. Earth Sci. 102, 07–233. doi:10.1007/s00531-012-0800-5
Montegrossi, G., Tassi, F., Vaselli, O., Bidini, E., and Minissale, A. (2006). A New, Rapid and Reliable Method for the Determination of Reduced sulphur (S2−) Species in Natural Water Discharges. Appl. Geochem. 21, 849–857. doi:10.1016/j.apgeochem.2006.02.007
Mutihac, V. (1990). Structura Geologică a Teritoriului României (The Geological Structure of Romania). Bucharest: Edit. Tehn., 418. in Romanian.
Nicula, A. M., Ionescu, A., Pop, I. C., Orăşeanu, I., and Baciu, C. (2019). Preliminary Considerations Regarding the Geothermal Potential in the Apuseni Mountains Area. Studia UBB Ambientum 64 (2), 45–56. doi:10.24193/subbambientum.2019.2.04
Orăşeanu, I. (2016). Hidrogeologia Carstului Din Munţii Apuseni (Karst Hydrogeology from Apuseni Mts.). Oradea: Edit. Belvedere, 300. in Romanian.
Orăşeanu, I. (2020). Hidrogeologia Carstului Din Munţii Apuseni (Karst Hydrogeology from Apuseni Mts.. 2nd ed. Oradea: Edit. Belvedere, 349. (in Romanian).
Orăşeanu, I. (1987). Hydrogeological Study of Moneasa Area (Codru Moma Mountains). Theor. Appl. Karstology 3, 175–199.
Orăşeanu, I., and Mather, J. (2000). Karst Hydrogeology and Origin of thermal Waters in the Codru Moma Mountains, Romania. Hydrogeology J. 8, 379–389. doi:10.1007/s100400000080
Pécskay, Z., Edelstein, O., Seghedi, I., Szakács, A., Kovács, M., Crihon, M., et al. (1995). K-Ar Datings of Neogene-Quaternary Calcalkaline Volcanic Rocks in Romania. Acta Vulcanologica 7 (2), 53–61.
Pricăjan, A. (1972). Apele minerale si termale din Romania (Mineral and thermal waters from Romania). Bucharest: Tehn., 206. in Romanian.
Pricăjan, A., and Airinei, S. (1981). Bogatia Hidrotermala Balneara Din Romania (The Hydrothermal Wealth of Romania). Bucharest: Stiintifica si Enciclopedica, 134. in Romanian.
Rman, N., Bălan, L.-L., Bobovečki, I., Gál, N., Jolović, B., Lapanje, A., et al. (2019). Geothermal Sources and Utilization Practice in Six Countries along the Southern Part of the Pannonian basin. Environ. Earth Sci. 79, 1. doi:10.1007/s12665-019-8746-6
Rowland, H. A. L., Omoregie, E. O., Millot, R., Jimenez, C., Mertens, J., Baciu, C., et al. (2011). Geochemistry and Arsenic Behaviour in Groundwater Resources of the Pannonian Basin (Hungary and Romania). Appl. Geochem. 26, 1–17. doi:10.1016/j.apgeochem.2010.10.006
Roşu, E., Pécskay, Z., Ştefan, A., Popescu, G., Panaiotu, C., and Panaiotu, C. E. (1997). The Evolution of the Neogene Volcanism in the Apuseni Mountains (Romania): Constraints from New K-Ar Data. Geologica Carpathica 48 (6), 353–359.
Roşu, E., Seghedi, I., Downes, H., Alderton, D. H. M., Szakács, A., Pécskay, Z., et al. (2004). Extension-related Miocene Calc-Alkaline Magmatism in the Apuseni Mountains, Romania: Origin of Magmas. Schweizerische Mineralogische und Petrographische Mitteilungen 84, 153–172.
Schmid, S. M., Bernoulli, D., Fügenschuh, B., Matenco, L., Schefer, S., Schuster, R., et al. (2008). The Alpine-Carpathian-Dinaridic Orogenic System: Correlation and Evolution of Tectonic Units. Swiss J. Geosci. 101, 139–183. doi:10.1007/s00015-008-1247-3
Schmid, S. M., Fügenschuh, B., Kounov, A., Maţenco, L., Nievergelt, P., Oberhänsli, R., et al. (2020). Tectonic Units of the Alpine Collision Zone between Eastern Alps and Western Turkey. Gondwana Res. 78, 308–374. doi:10.1016/j.gr.2019.07.005
Seghedi, I. (2004). “Geological Evolution of the Apuseni Mountains with Emphasis on the Neogene Magmatism – a Review,” in Au-Ag-telluride Deposits of the Golden Quadrilateral, Apuseni Mts., Romania, Guidebook of the International Field Workshop of IGCP Project 486, IAGOD Guidebook Series. Editors N.J. Cook, and C.L. Ciobanu, 11, 5–23.
Seghedi, I., and Downes, H. (2011). Geochemistry and Tectonic Development of Cenozoic Magmatism in the Carpathian-Pannonian Region. Gondwana Res. 20 (4), 655–672. doi:10.1016/j.gr.2011.06.009
Seghedi, I., Maţenco, L., Downes, H., Mason, P. R. D., Szakács, A., and Pécskay, Z. (2011). Tectonic Significance of Changes in post-subduction Pliocene-Quaternary Magmatism in the South East Part of the Carpathian-Pannonian Region. Tectonophysics 502, 146–157. doi:10.1016/j.tecto.2009.12.003
Szocs, T., Rman, N., Rotár-Szalkai, Á., Tóth, G., Lapanje, A., Černák, R., et al. (2018). The Upper Pannonian thermal Aquifer: Cross Border Cooperation as an Essential Step to Transboundary Groundwater Management. J. Hydrol. Reg. Stud. 20, 128–144. doi:10.1016/j.ejrh.2018.02.004
Săndulescu, M. (1984). Geotectonica Romaniei (Geotectonics of Romania). Bucuresti: Editura Academiei Romane, 336. in Romanian.
Tiliţă, M., Lenkey, L., Maţenco, L., Horvath, F., Suranyi, G., and Cloetingh, S. (2018). Heat Flow Modelling in the Transylvanian basin: Implications for the Evolution of the Intra Carpathian Area. Glob. Planet. Change 171, 148–166. doi:10.1016/j.gloplacha.2018.07.007
Keywords: geothermal, mineral water, hydrogeochemistry, water isotopes, geothermometer, Apuseni Mts, Romania
Citation: Nicula A-M, Ionescu A, Pop I-C, Roba C, Forray FL, Orăşeanu I and Baciu C (2021) Geochemical Features of the Thermal and Mineral Waters From the Apuseni Mountains (Romania). Front. Earth Sci. 9:648179. doi: 10.3389/feart.2021.648179
Received: 31 December 2020; Accepted: 05 August 2021;
Published: 17 August 2021.
Edited by:
Giovanni Martinelli, National Institute of Geophysics and Volcanology, ItalyReviewed by:
Giovanni Vespasiano, University of Calabria, ItalyTamara Marković, Croatian Geological Survey, Croatia
Nina Rman, Geological Survey Ljubljana, Slovenia
Copyright © 2021 Nicula, Ionescu, Pop, Roba, Forray, Orăşeanu and Baciu. This is an open-access article distributed under the terms of the Creative Commons Attribution License (CC BY). The use, distribution or reproduction in other forums is permitted, provided the original author(s) and the copyright owner(s) are credited and that the original publication in this journal is cited, in accordance with accepted academic practice. No use, distribution or reproduction is permitted which does not comply with these terms.
*Correspondence: Călin Baciu, calin.baciu@ubbcluj.ro