- 1Alfred Wegener Institute, Research Unit Potsdam, Polar Terrestrial Environmental Systems, Potsdam, Germany
- 2Institute of Geology and Mineralogy, University of Cologne, Cologne, Germany
- 3Institute of Geology and Petroleum Technologies, Kazan Federal University, Kazan, Russia
- 4Northeastern Interdisciplinary Scientific Research Institute, Far East Branch of the Russian Academy of Sciences, Magadan, Russia
Upper Pliocene sediments from a number of fluvial outcrops in central Chukotka, northeastern Russian Arctic, along the Enmyvaam, Mechekrynnetveem, and Chanuvenvaam Rivers, have been newly studied for pollen, non-pollen-palynomorphs and, for the first time for Pliocene sediments in Eurasia, charcoals. The sediments have survived the El’gygytgyn meteorite impact event at ∼3.58 Ma. The stratigraphy of the studied outcrops suggests that the lowermost sediments were accumulated shortly before the impact event, between ∼3.60 and 3.58 Ma. At that time, coniferous forests with spruces, pines, firs, birches, larches, and alders dominated in the area. Some relatively thermophilic broad-leaved taxa (Corylus, Carpinus, Ulmus, and Myrica) might also have grown in local forests. Summer temperatures were at least 10°C warmer than today. Charcoal concentrations and composition suggest the presence of high intensity fires. Periods of rather wet climate and soil conditions are marked by common shrubby and boggy habitats with ericaceous plants and Sphagnum, and are associated with less, and probably low-intensity surface fires with less charcoal. The impact event caused widespread fires reflected by up to 4 times higher charcoal concentrations in the sediments. The sediments found above the so-called “chaotic horizon” (sediments accumulated synchronously or very shortly after the impact event) contain late Pliocene pollen assemblages comparable to those in Lake El’gygytgyn, reflecting that pine-spruce forests with some firs, birches, larches, and alder dominated in the study area. Some thermophilic taxa might also still have grown in the area. However, the age control for the sediments above the so-called chaotic horizon is poor. The uppermost sediments from the studied sections can be attributed with certainty to the Late Pleistocene and Holocene according to their stratigraphic positions and pollen assemblages. The combined pollen and charcoal analysis allowed correlating hardly datable fluvial sediments and points to varying fire regimes in warmer-than-present climates, when forest extended further north compared to today.
Introduction
Pollen studies on the 318-m long lacustrine sediment record from Lake El’gygytgyn, central Chukotka (site 5011-1 of the International Continental Drilling Program, ICDP, Melles et al., 2011; Figure 1A), have demonstrated that the sedimentary infill of the El’gygytgyn meteorite impact crater is a highly sensitive and unique archive of vegetation and climate changes in the northeastern Russian Arctic since ca. 3.56 Ma (e.g., Melles et al., 2012; Brigham-Grette et al., 2013; Tarasov et al., 2013; Andreev et al., 2014, 2016). However, the environmental history of the region before the meteorite impact is only fragmentary known from studies on fluvial sediments in a number of river bank outcrops exposed along the Enmyvaam, Mechekrynnetveem, and Chanuvenvaam Rivers (e.g., Glushkova and Smirnov, 2007 and reverences therein; Figure 1B). At the base of these outcrops, above weathered basement rocks, stratified floodplain deposits with thicknesses of up to 3 m occur (Glushkova and Smirnov, 2007). These fluvial sediments consist of well-rounded small pebbles, gravel, and fine-grained sand with lenses and intercalations of lignified plant remains (the so-called lignified horizon). They are often overlain by the so-called “chaotic horizon,” which is an up to 6 m thick mixed layer of impactites, fragments of volcanic rocks, sands, and clays (Glushkova, 1993; Glushkova and Smirnov, 2007). The impact origin of the “chaotic horizon” is clearly indicated by the presence of spherules of impact melt (Smirnov et al., 2011). Thus, the bottom part of this horizon is obviously synchronous with the meteorite impact event that has formed the El’gygytgyn Lake depression 3.58 ± 0.04 Ma ago (Layer, 2000).
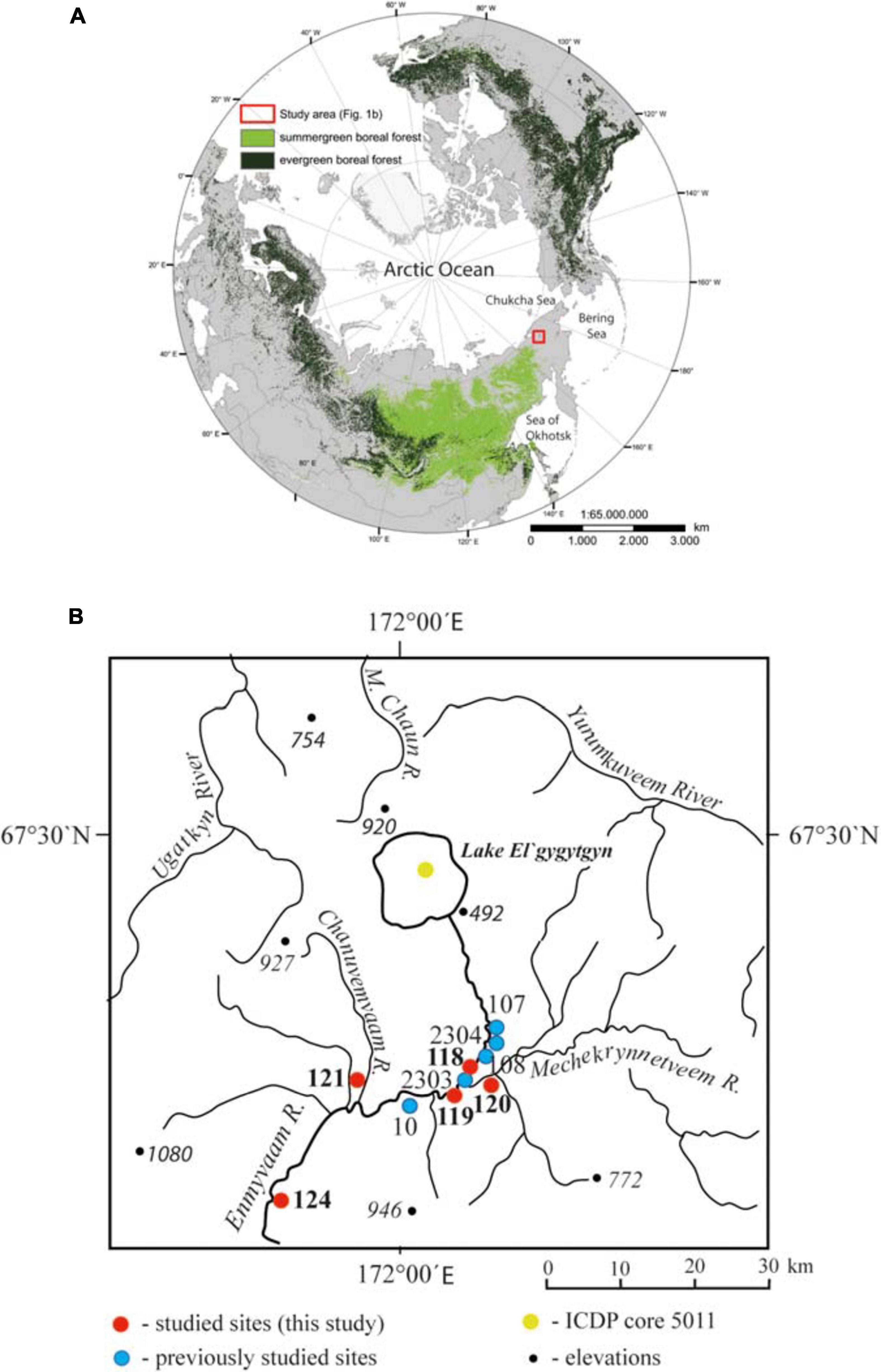
Figure 1. (A) Polar view on the high northern latitudes with the distribution of summergreen and evergreen boreal forests and the location of study area in northeastern Siberia. (B) Locations of fluvial sediment sections studied in the vicinity of Lake El’gygytgyn and of ICDP Site 5011-1 in the lake center.
Consequently, the sediments with the lignified plant remains underneath predate the meteorite impact and were presumably formed during early to middle Pliocene. According to preliminary studies (Glushkova, 1993; Belyi et al., 1994), the pollen assemblages in the lignified horizon are rather homogenous, with dominance of Pinus s/g Haploxylon, Picea, Betula, and Alnus and some Abies, Larix, and Tsuga. Single pollen grains of broad-leaved taxa, such as Corylus, Carpinus, Myrica, Juglans, and Lonicera were also found. Since these pollen data unfortunately are not available for quantitative paleoenvironmental reconstructions, the environmental history of the area before 3.58 Myr remains poorly known.
In this study, we investigated the pollen and sedimentary charcoal composition of samples collected in 1991 and 1993 in five fluvial outcrops, in order to reconstruct the environmental history of the El’gygytgyn area prior to the meteorite impact. These results shall help to clarify whether the impact has drastically and persistently changed the vegetation in the area or whether potential vegetation changes were rather driven by past climatic changes and/or other factors, such as wildfires. Although the obtained paleoenvironmental records are fragmented and have rather poor age control, these new records complement our understanding of the pre- and post-impact vegetation and climate changes from the region of Lake El’gygytgyn, northeastern Russia.
Geographical Setting
The El’gygytgyn impact crater is located in the central part of the Anadyr Upland within the Middle Cretaceous Okhotsk-Chukchi volcanic belt (Belyi and Belaya, 1998; Wennrich et al., 2016 and references therein). The small mountain range with altitudes between 700 and 800 m, and a maximum of 941 m above sea level (a.s.l.), subdivides the Arctic and the Pacific watersheds and is the main orographic barrier in the study area. Lake El’gygytgyn is situated at 492 m a.s.l. and is fed by 50 tributaries (Nolan and Brigham-Grette, 2007). Its only outflow, the 230-km long Enmyvaam River (Figure 1B), is a tributary of the Yurumkuveem River that drains via the Anadyr River into the northernmost Pacific Ocean. The Enmyvaam River is accompanied by several river terraces. The most prominent terrace occurs ∼25–30 m above the active river channel near the mouth of the Mechekrynnetveem River at about 420–430 m a.s.l. (Figure 1B). Additional river terraces gradually merge into the broad Mechekrynnetveem depression. The Chanuvenvaam River contributes to the Enmyvaam River from the north, ∼15 km downstream of the Mechekrynnetveem-Enmyvaam confluence.
The study area is situated in the continuous permafrost up to 300–400 m (Mottaghy et al., 2013) and a mean annual ground temperature of −10°C at 12.5 m depth and an active-layer thickness of up to 80 cm in sandy soils (Schwamborn et al., 2008). The regional climate is characterized by an average annual air temperature of c. −10°C, mean July temperatures of – 4–8°C, and mean January temperatures of −32 to −36°C. The precipitation consists of 70 mm summer rainfall (June-September) and ca. 110 mm water equivalent of snowfall (Nolan and Brigham-Grette, 2007). The region belongs to the subzone of southern shrub and typical tundra (Galanin et al., 1997). The modern treeline for larch (Larix cajanderi) is positioned roughly 80 km to the south and west.
The lowermost Enmyvaam terrace deposits at site 124 consist of 4–7 m of laminated sand with small pebbles, overlain by up to 1.5–2.5 m thick lignified peaty layer and the so-called chaotic horizon with clay, sand, fragments of volcanic rocks, and impactites of up to 5–6 m thickness. The upper 17–23 m of the studied outcrops are homogenous alluvial sediments (Belyi et al., 1994). A similar sediment stratigraphy was discovered at the Chanuvenvaam River study area (Figure 1B). Earlier pollen studies of sediments below the chaotic horizon (sites 10, 107, 108, 2304, and 2303 on Figure 1B; Belyi et al., 1994; Glushkova et al., 2005; Minyuk et al., 2006) suggested that forests with pine, spruce, birch, alder, and rare thermophilic trees were growing in the study area during the early Pliocene. Unfortunately, the revealed pollen records were neither properly published nor are the original pollen slides available anymore.
Materials and Methods
Fieldwork and Geographic Location of Studied Sections
During field campaigns in summers, 1991 and 1993, geological-geomorphological investigations were carried out about 20–40 km to the south of the El’gygytgyn Impact Crater, which included the sedimentological description and the sampling of five fluvial outcrops of the Enmyvaam, Mechekrynnetveem, and Chanuvenvaam Rivers (sections 118, 119, 120, 121, 124, Figure 1B). Sections 118 (67.250522°N, 172.268290°E, 433 m a.s.l.) and 119 (67.239552°N, 172.233839°E, 424 m a.s.l.) are situated on the left bank of Enmyvaam River, ∼6.5 km and 1.5 km above the Mechekrynnetveem-Enmyvaam river confluence, respectively. Sections 120 (67.243589°N, 172.306890°E, 439 m a.s.l.) and 121 (67.244438°N, 171.929236°E, 429 m a.s.l.) are situated on the right banks of the Mechekrynnetveem and Chanuvenvaam Rivers, ∼4.5 km and 3.5 km above their confluences with the Enmyvaam River. Further to the south, Section 124 (67.098098°N, 171.646010°E, 399 m a.sl.) is placed on the left bank of Enmyvaam River, ∼20 km downstream from the Enmyvaam-Chanuvenvaam confluence (Figure 1B).
The stratigraphic units of these sections were distinguished in the field based on bedding, grain size, and sediment colors. A total of 56 samples were collected for pollen analysis from pre- and post-impact deposits.
Paleoecological Analyses
Pollen preparation was mainly carried out in the pollen lab of Alfred Wegener Institute for Polar and Marine Research (Potsdam) by a standard HF technique (Berglund and Ralska-Jasiewiczowa, 1986). A tablet of Lycopodium marker spores was added to the samples to allow for the calculation of total pollen and spore concentrations following Stockmarr (1971). No Lycopodium marker spores were added to the samples from section 124, which were prepared in the pollen lab of the North-East Interdisciplinary Scientific Research Institute (Magadan). In all cases, water-free glycerol was used for sample storage and preparation of microscopic slides.
Pollen and spores were identified at magnifications of 400X, with the aid of published pollen keys and atlases (Kupriyanova and Alyoshina, 1972, 1978; Bobrov et al., 1983; Reille, 1992, 1995, 1998). Where possible, at least 250 pollen grains were counted in each sample. Some samples contain only single pollen grains and therefore were either not used for paleoenvironmental reconstructions or used with caution. In addition to pollen and spores, non-pollen-palynomorphs (NPP), such as fungi spores as well as remains of algae and invertebrate, were identified and counted, if possible. NPP are valuable indicators of past environments, especially of local microhabitats (e.g., van Geel, 2001 and references therein). The percentages of pollen taxa were calculated from the sum of terrestrial pollen taxa. The percentages of spores, NPP, and algae are based on the sums of pollen and spores, pollen and NPP, and pollen and algae, respectively.
To consider wildfires as factors influencing the vegetation composition, charcoal concentrations were calculated in 16 samples from sections 119, 121, and 124. For this purpose, a minimum of 300 microscopic charcoal particles were counted together with Lycopodium spores. Microscopic charcoal particles were categorized into six charcoal morphotypes by adopting the classification scheme of Enache and Cumming (2007), which was developed for charcoal >150 μm and supported by biomass burn experiments (Mustaphi and Pisaric, 2014; Pereboom et al., 2020). We expect that the breakage of charcoal during pollen preparation preserves the original patterns of charcoal particle appearance (shape and opaqueness), which is related to the type of burnt biomass, with high opaqueness of particles qualitatively suggesting higher fire intensities (Conedera et al., 2009). We might slightly overestimate the relative amounts of elongated, fragile relative to the massive, blocky particles that are less breakable. The percentages of the charcoal morphotypes are based on the total charcoal counts.
TGView software (Grimm, 2004) was used for the calculation of percentages and for drawing the diagrams. The diagrams were zoned by a qualitative inspection of significant changes in pollen assemblages, pollen concentrations, and the occurrence of particularly indicative pollen and NPP taxa. Sediments from horizons with coarse material often contain no palynomorphs. These samples are not presented on the pollen diagrams.
Results
Stratigraphic Characteristics
The analyzed fluvial terrace sections are marked by a heterogeneous sediment thickness and lithological composition, and have been classified in the field into stratigraphic units of individual composition (see detailed descriptions in Table 1). In the c. 12 m thick section 118, a total of 11 loam- to pebble-rich stratigraphic units have been identified above bedrock basalts. Section 119 comprises 20 m of sediments consisting of five stratigraphic units above the current channel bed. In section 120, two units rich in black clay in the lower 5.5 m are overlain by five units of sand- to pebble-rich sediments in the upper 3.5 m. In the c. 18 m thick section 121, 18 units could be distinguished above a basaltic tuff. Section 124 comprises 30 m thick sediments above the current Enmyvaam channel, which were distinguished into 11 units. The “chaotic horizons,” which consist of sediments with a chaotic structure that contain glass spherules of up to several mm in size, have been preserved in sections 119 and 121. The macro- and microscopic appearance of these glass spherules is very similar to those found in the El’gygytgyn Crater and ICDP core from Lake El’gygytgyn (Smirnov et al., 2011; Goderis et al., 2013; Wittmann et al., 2013). Based on their geochemical composition the glass spherules from the “chaotic horizons” of the studied sections 119 and 121 have been attributed to the meteorite impact that formed the Lake El’gygytgyn crater (Smirnov et al., 2011).
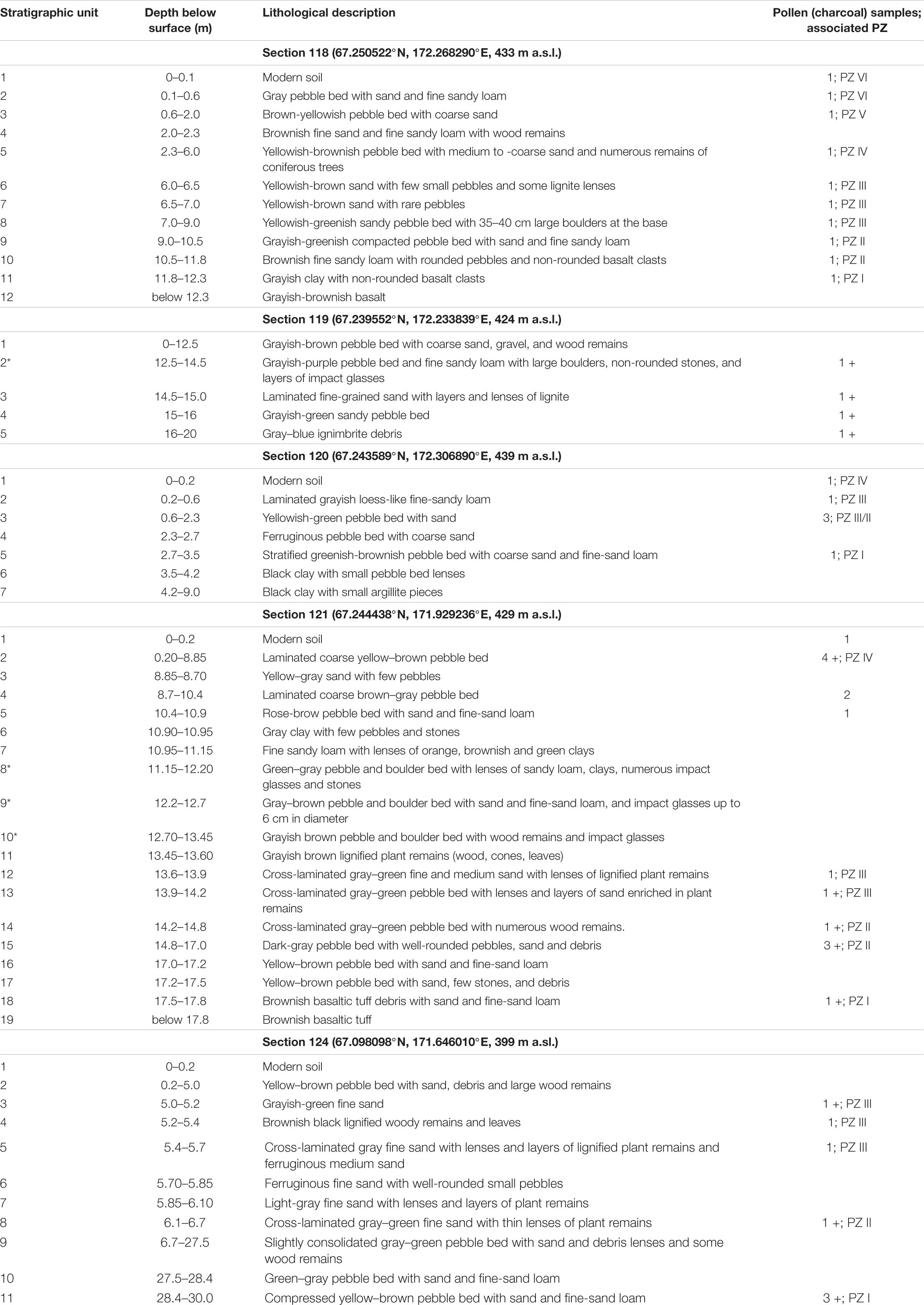
Table 1. Stratigraphy of fluvial terrace sections downstream of Lake El’gygytgyn (“chaotic horizons” marked with *) with their lithological descriptions, the number of samples investigated for pollen and non-pollen palynomorph taxa (and sedimentary charcoal indicated by ±) and their linkage with associated pollen zones (PZ).
Paleoecological Results
The paleoecological results obtained on the investigated sections, complemented by examples of charcoal morphotypes and woody macrofossils found in the records, are presented in Figures 2–8.
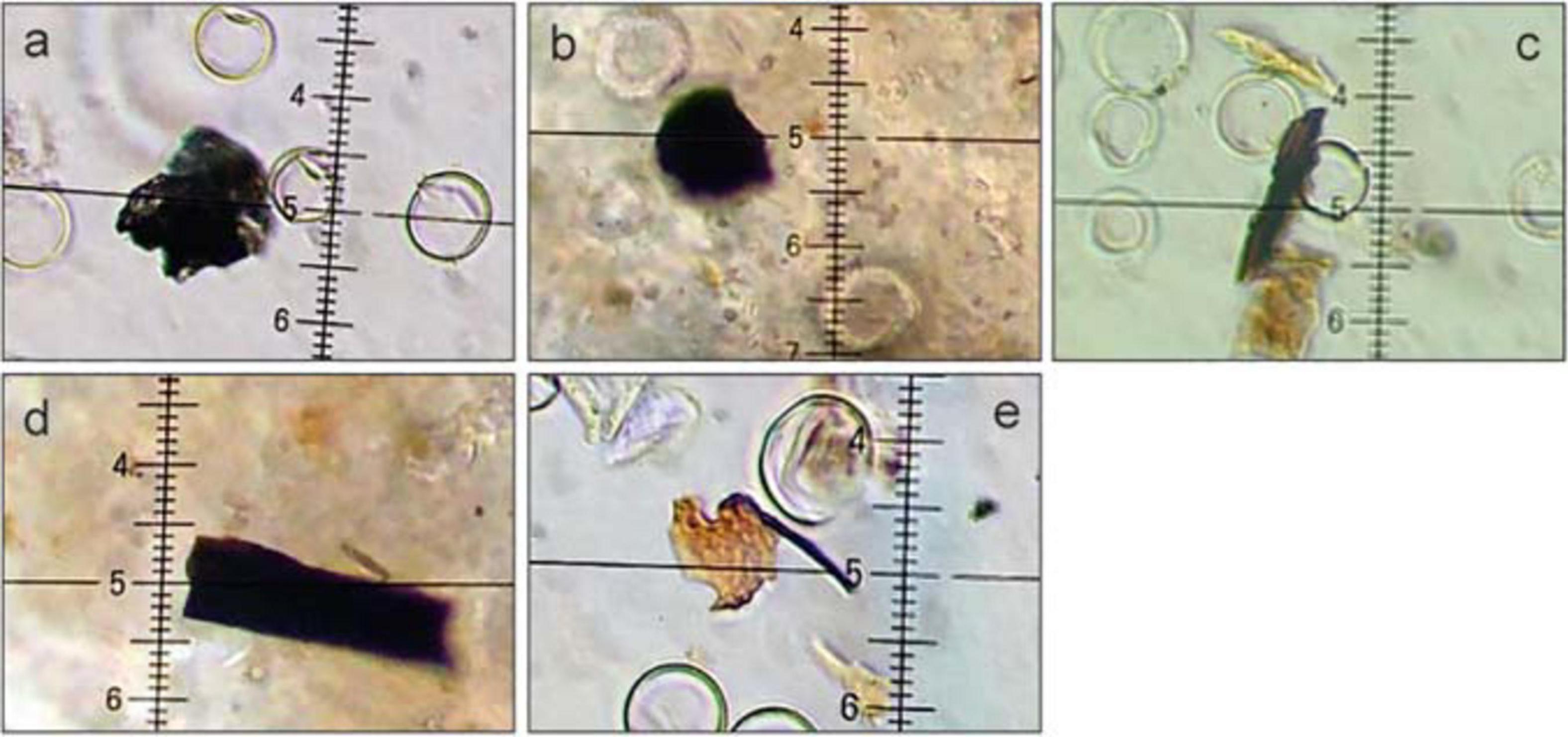
Figure 2. Microscopic charcoal morphotypes common in the pollen samples of sections 119, 121 and 124: (a) translucent, irregular type M; (b) opaque irregular type P; (c) angular, translucent type B; (d) angular, opaque type C and (e) elongated type F. The globose and transparent cysts in all photos were possibly produced by the so-called snow-algae species from genera of Chlamydomonas, Chloromonas, Haematococcus (Sphaerella). Photos by Tabea Tessendorf (AWI), March 2020.
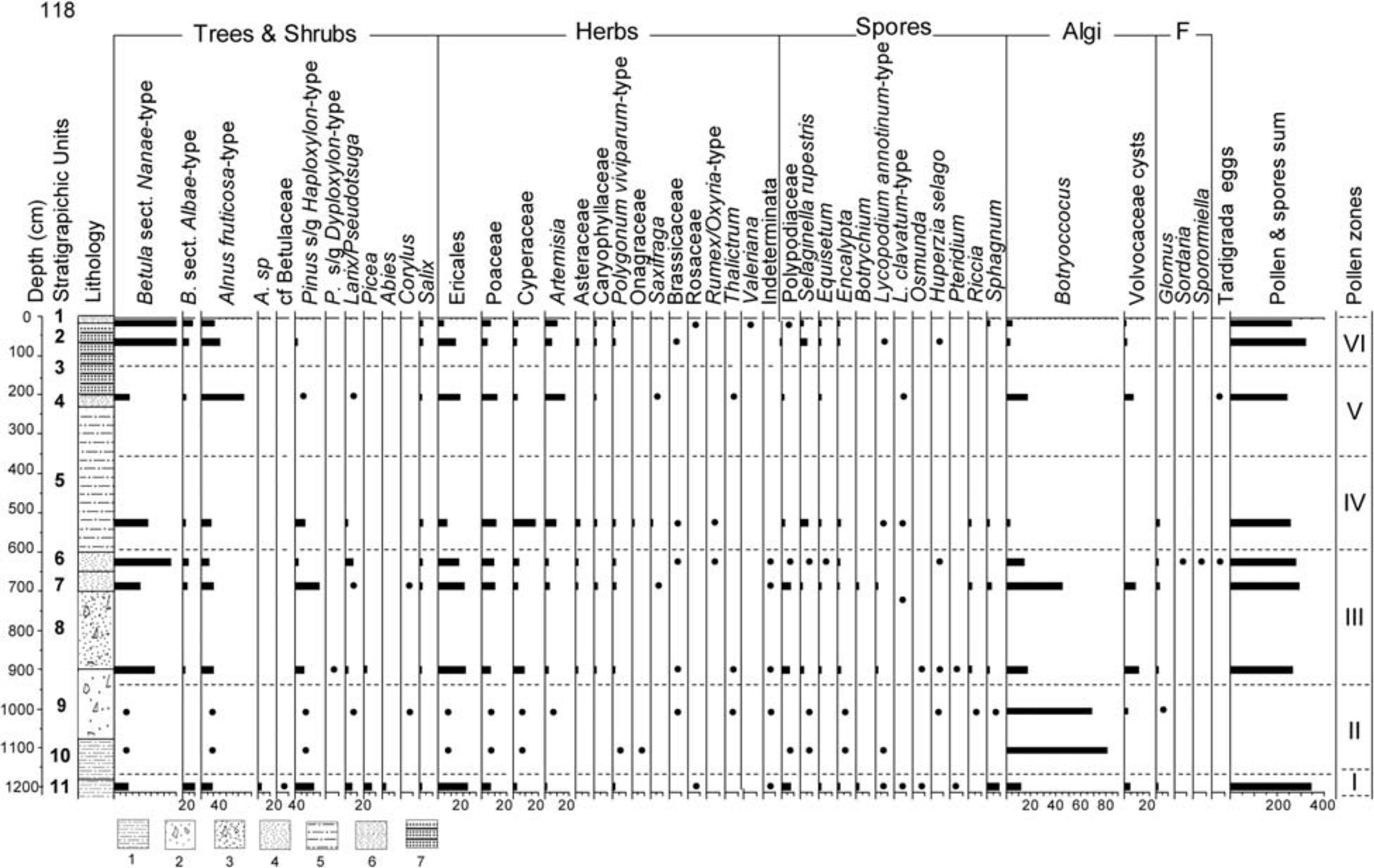
Figure 3. Percentage pollen, spore, and non-pollen-palynomorph diagram of site 118 (for location see Figure 1B). 1 – grayish clay (11.8–12.3 m) and brownish fine sandy loam (10.5–11.8 m); 2 – grayish-greenish compacted pebble bed with sand and fine sandy loam; 3 – yellowish-greenish sandy pebble bed with boulders at the base; 4 – yellowish-brown sand with few small pebbles and some lignite lenses between 6.0–6.5 m; 5 – yellowish-brownish pebble bed with medium to -coarse sand and numerous remains of coniferous trees; 6 – sand and fine sandy loam; 7 – brown-yellowish pebble bed with coarse sand.
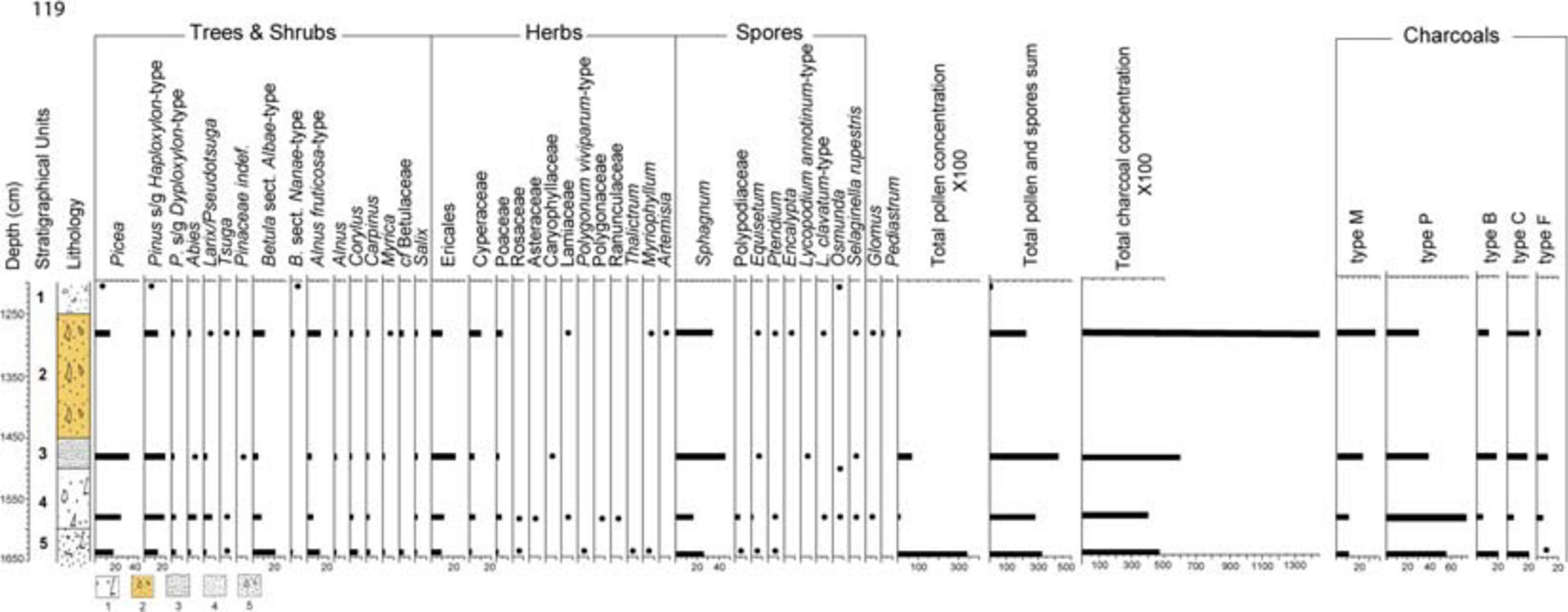
Figure 4. Percentage pollen, spore, non-pollen-palynomorph and charcoal diagram of site 119 (for location see Figure 1B). 1 – grayish-green sandy pebble bed; 2 – pebble bed and fine sandy loam with boulders, stones and layers of impact glasses (chaotic horizon); 3 – fine-grained sand with lignite layers and lenses; 4 – grayish-brown pebble bed with coarse sand; 5 – gray-blue ignimbrite debris.
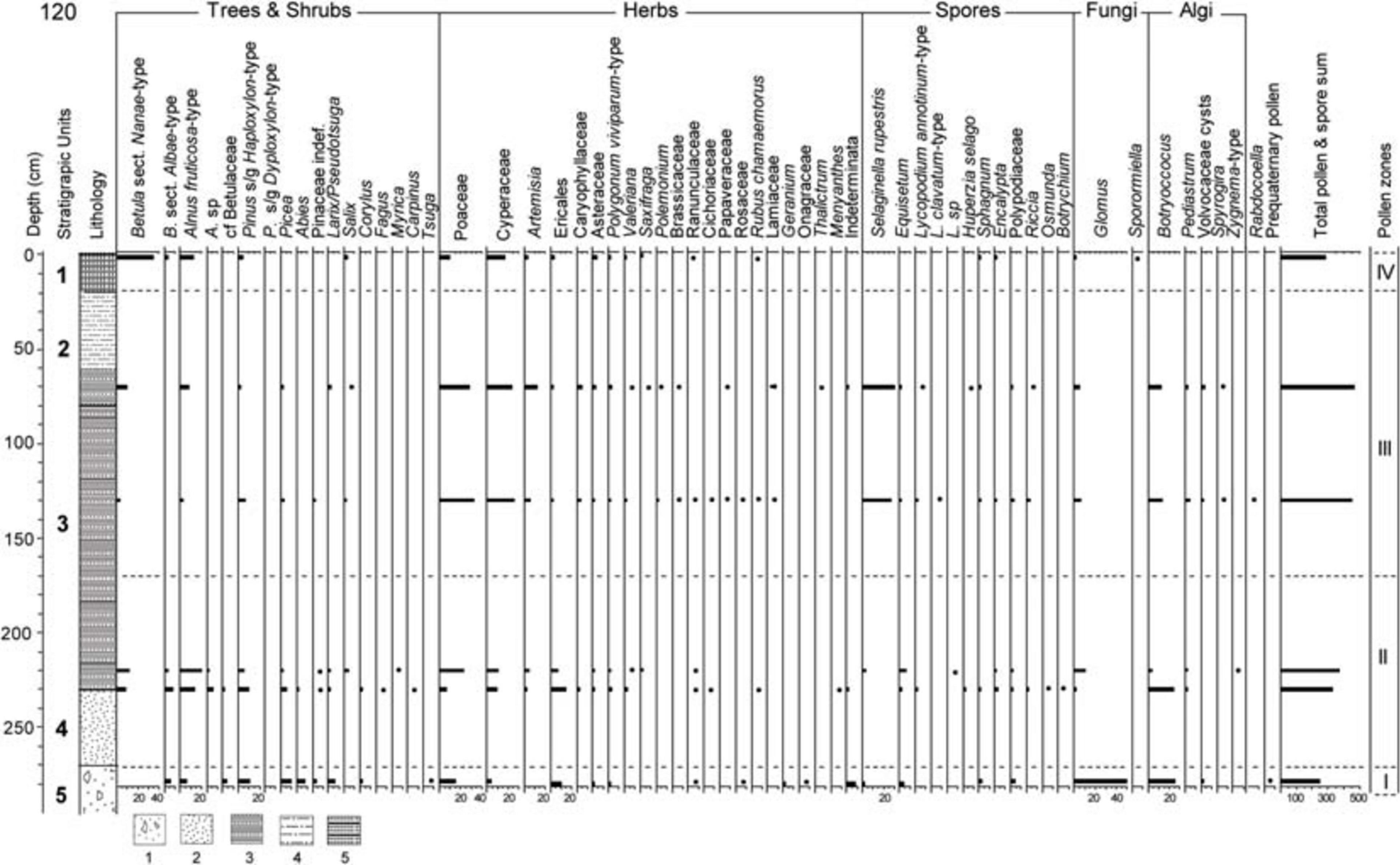
Figure 5. Percentage pollen, spore, and non-pollen-palynomorph diagram of site 120 (for location see Figure 1B). 1 – greenish-brownish pebble bed with coarse sand and fine-sand loam; 2 – ferruginous pebble bed with coarse sand; 3 – yellowish-green pebble bed with sand; 4 – laminated grayish loess-like fine-sandy loam; 5 – modern sandy soil.
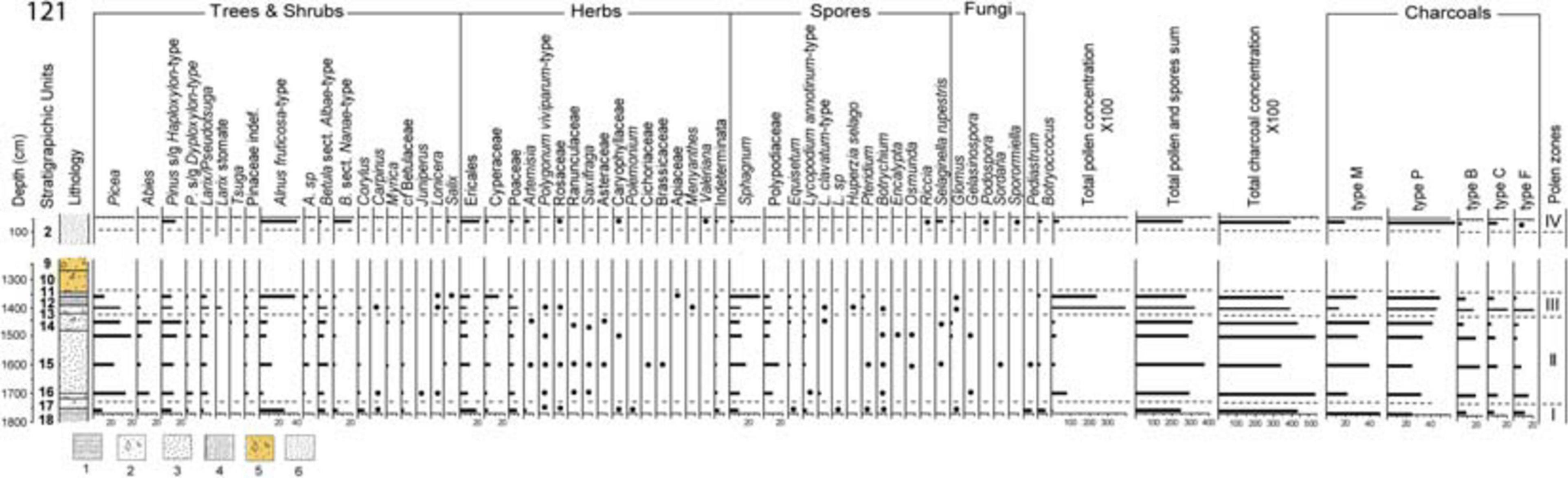
Figure 6. Percentage pollen, spore, and non-pollen-palynomorph and charcoal diagram of site 121 (for location see Figure 1B). 1 – brownish basaltic tuff debris with sand and fine-sand loam; 2 – yellow-brown pebble bed with sand; 3 – dark-gray pebble bed with well-rounded pebbles, sand and debris; 4 – grayish brown lignified plant remains (wood, cones, leaves); 5 – grayish brown pebble and boulder bed with impact glasses; 6 – laminated coarse yellow-brown pebble bed.
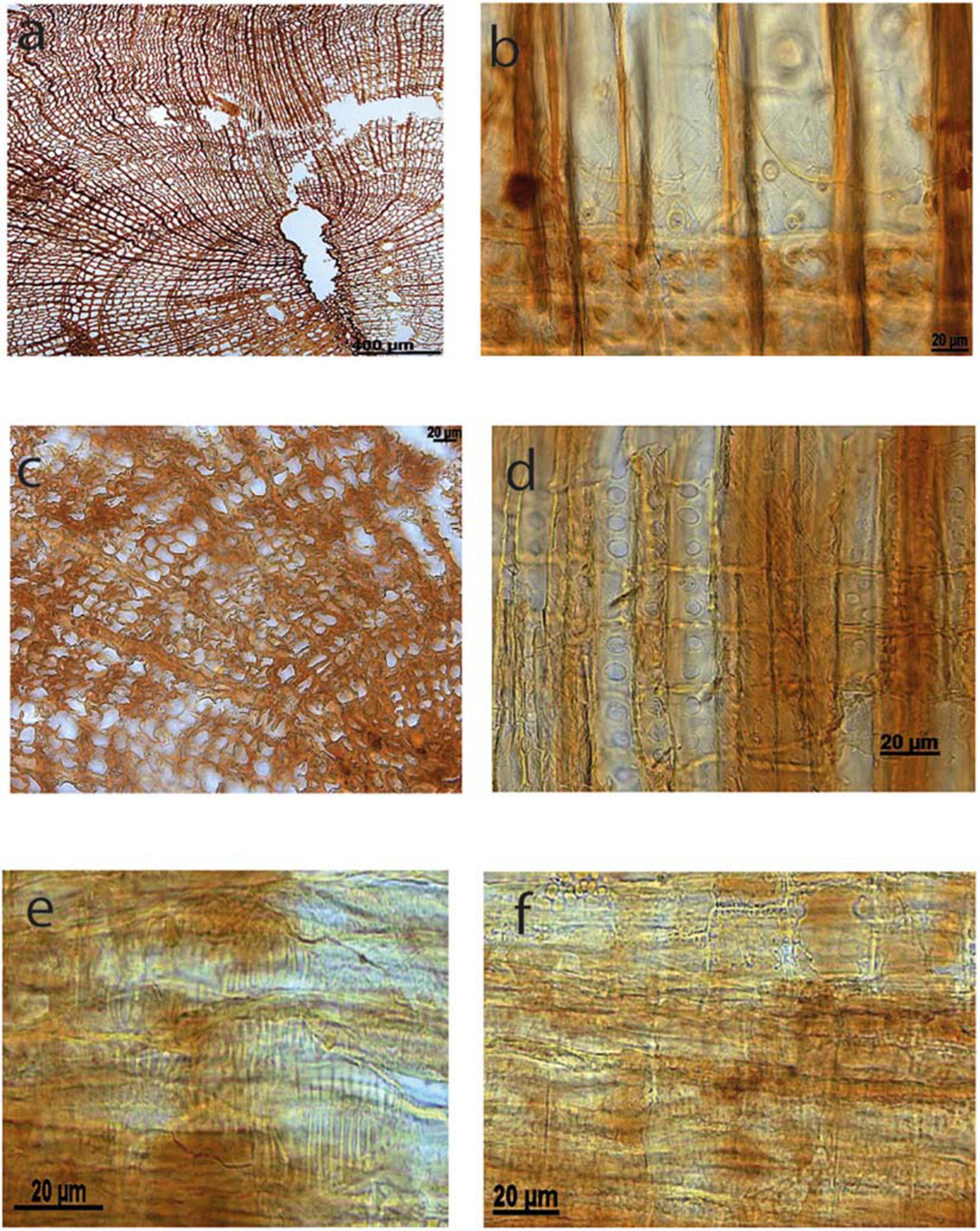
Figure 7. Microtome sections of woody macrofossils found in site 121 sediments: (a) a cross section cut of a Larix sp. root; (b) a radial cut of a Larix sp. root; (c) a cross section cut of an Abies sp. twig; (d) a radial cut of an Abies sp. twig; (e) – a radial cut of an Ericaceae twig; (f) – a cross section cut of an Ericaceae twig. Photos by V.R. Filin (Moscow State University) November 2019.
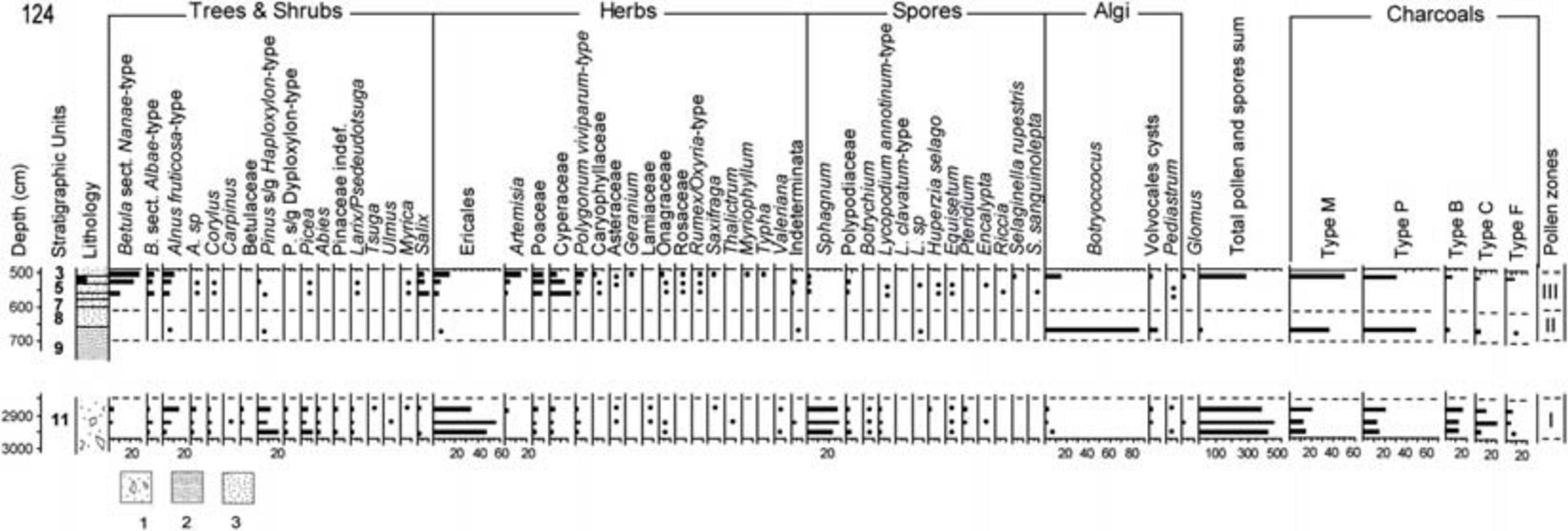
Figure 8. Percentage pollen, spore, and non-pollen-palynomorph and charcoal diagram of site 124 (for location see Figure 1B). 1 – green–gray pebble bed with sand and fine-sand loam; 2 – slightly consolidated gray–green pebble bed with sand and debris lenses and some wood remains; 3 – fine sand with lenses and layers of plant and woody remains, partly laminated.
Section 118
A total of 44 different pollen, spore, and NPP types have been found in the 10 samples studied for palynomorphs from section 118, which consists of 12 stratigraphic units (Table 1 and Figure 3). The revealed pollen assemblages can be subdivided into 6 pollen zones (PZ).
PZ I (∼12.0–11.5 m) is dominated by Ericales, Betula, Pinus s/g Haploxylon, and Alnus fruticosa pollen as well as Sphagnum spores. There are rather numerous coniferous Picea, Abies, Larix and Poaceae pollen, Polypodiaceae spores, and remains of green algae colonies (Botryococcus). PZ II (∼11.5–9.5 m) contains only very few pollen grains but numerous Botryococcus remains. PZ III (∼9.5–5.8 m) is dominated by pollen of Betula, A. fruticosa, Ericales, Poaceae, Pinus s/g Haploxylon, Cyperaceae and Polypodiaceae spores. Algae remains (Botryococcus colonies and cysts of Volvocales) are also very common. In PZ IV (∼5.8–3.5 m) the percentages of Cyperaceae and Artemisia pollen as well as Selaginella spores are significantly higher than in PZ III, while Botryococcus remains are very rare. PZ V (∼3.5–1.1 m) is characterized by a remarkable increase of A. fruticosa pollen and higher percentages of Botryococcus remains and cysts of Volvocales. PZ VI (∼1.1–0.0 m) is dominated by pollen of Betula sect. Nanae, A. fruticosa, Ericales, Poaceae, Artemisia, and Cyperaceae.
Section 119
In the 10 samples studied for palynomorphs and charcoals from section 119, which cover four stratigraphic units bracketing the “chaotic horizon” in unit 2, a total of 40 different pollen, spore, and NPP types have been found (Figure 4). For associated stratigraphic units see Table 1. The assemblages are dominated by pollen of Picea, Pinus s/g Haploxylon, Betula, and Ericales and Sphagnum spores. Single pollen grains of broad-leaved taxa, such as Corylus, Myrica, and Carpinus, also occur. The sample from the “chaotic horizon” differs from the other samples in particular by 2–4 times higher concentration of microscopic charcoals.
The charcoals in section 119 do not only differ in concentration but also in shape. The irregularly shaped and structured morphotype M (Figure 2a) shows highest concentration in the “chaotic horizon” of unit 2. The irregularly shaped but structureless morphotype P (Figure 2b) is most abundant in units 4 and 5. The angular, structured, partially charred morphotype B (Figure 2c) and the angular, structureless morphotype C (Figure 2d) occur in all units, but much reduced in unit 4. The elongated charcoal type F (Figure 2e) is of minor importance (Figure 4).
Section 120
A total of 6 samples were studied for palynomorphs from section 120, which consists of 7 stratigraphic units (Table 1). In these samples, 60 different pollen, spore, and NPP types have been found (Figure 5). The revealed pollen assemblages can be subdivided into 4 PZs.
PZ I (∼2.7–2.8 m) is dominated by Poaceae, Ericales, A. fruticosa, and coniferous (Pinus s/g Haploxylon, Picea, Abies, Larix) pollen. There are rather numerous spores of Glomus and remains of Botryococcus colonies. PZ II (∼2.7–1.7 m) is characterized by an increase of Betula and Alnus pollen percentages, while percentages of Glomus spores are significantly lower. PZ III (∼1.7–0.2 m) is dominated by pollen of Poaceae, Cyperaceae, and Artemisia. Spores of Selaginella rupestris become an important component in this zone and Botryococcus remains keep common. Pollen spectra in PZ IV (∼0.2–0.0 m) are dominated by Betula sect. Nanae and A. fruticosa. Percentages of Poaceae, Cyperaceae, and Artemisia pollen are significantly decreased, while Selaginella spores and Botryococcus remains completely disappear.
Section 121
In section 121, which consists of 19 stratigraphic units (Table 1), 22 samples were investigated for palynomorphs. However, only 8 samples contain pollen grains. They were also studied for charcoals (Figure 6). In these samples, a total of 56 different pollen, spore, and NPP types have been identified. The samples below 17.8 m (basaltic tuff debris, Table 1) do not contain pollen. The pollen assemblages revealed in samples above 17.8 m can be subdivided into 4 PZs.
PZ I (∼17.6–17.2 m) is dominated by pollen of A. fruticosa, Picea, Pinus s/g Haploxylon, Ericales, and Betula and Sphagnum spores. Pollen of broad-leaved taxa (Corylus, Carpinus) are also found. The zone differs from the other PZs by rather numerous algae remains (Botryococcus and Pediastrum). In PZ II (∼17.2–14.2 m) pollen of Picea, Pinus s/g Haploxylon, Abies, Betula, Alnus, Ericales, Poaceae, and Cyperaceae are dominating. Sphagnum and Polypodiaceae spores are very numerous. PZ III (∼14.2–13.5 m) is characterized by a drastic increase in pollen concentration. The percentages of Cyperaceae and A. fruticosa pollen and Sphagnum spores are significantly increased in the upper two samples. In addition, PZ III contains numerous woody remains, some of which have been identified at magnifications of 400× as Abies, Larix, and Ericaceae based on their cell structures (V. Filin personal comm., 2019; Figure 7). The only one sample from the upper sediments (∼9.5–0 m, PZ IV) contained pollen. The spectrum is dominated by pollen of A. fruticosa, Pinus s/g Haploxylon, Betula sect. Nanae, and Ericales. Percentages of Sphagnum spores are highly reduced, while Selaginella spores are increased.
The charcoal concentration in section 121 is comparable to that in the sediments underlying the “chaotic horizon” in section 119. It shows little variation in total charcoal concentration, but the charcoal morphotype composition varies across PZ (Figure 6, for charcoal morphotype images see Figure 2). In PZ I, morphotype M (Figure 2a) shows highest concentration. PZ II shows roughly equal contributions from morphotypes M and P (Figure 6) and differs from the other zones mainly by highest concentration of morphotype B (Figure 6, for example of morphotype B see Figure 2c). In PZ III, the charcoal concentration is slightly reduced, showing elevated percentages in morphotype P and maximum values in the record of morphotypes C and F (Figure 6). The charcoal composition of PZ IV is characterized by a maximum of morphotype P (Figure 2b), relatively high content of morphotype C (Figure 2d), and low values of all other morphotypes.
Section 124
In section 124, which consists of 11 stratigraphic units (Table 1), 5 samples were investigated for palynomorphs and charcoal (Figure 8). A total of 51 pollen, spore, and NPP types have been found and the revealed pollen assemblages were subdivided into 3 PZs.
PZ I (∼29.7–28.5 m) is dominated by Ericales, Pinus, Alnus, Picea, Abies, and Betula pollen and Sphagnum spores. Single pollen of broad-leaved taxa (Corylus, Carpinus, and Ulmus) also occur. PZ II (∼7–6 m) is very poor in pollen and spore grains, but there are numerous Botryococcus remains. PZ III (∼6.0–4.9 m) is dominated by pollen of Betula, A. fruticosa, Salix, Ericales, Poaceae, and Cyperaceae. The uppermost sample furthermore has a much higher concentration of pollen and spores then the other samples and an elevated percentages of Artemisia pollen and Botryococcus remains. Unfortunately, total pollen and charcoal concentrations cannot be determined as no Lycopodium spores were added in the samples prepared in the Magadan pollen laboratory.
PZ I shows clearly elevated percentages of charcoal morphotypes B and C (Figure 8, see examples for morphotypes in Figures 2c,d). In PZ II, morphotype M (Figure 2a) is enriched and morphotype P (Figure 2b) shows highest values in the entire record. In the uppermost sample of PZ III, in contrary, morphotype M reaches highest values in the record (Figure 8).
Discussion
Pre-impact Environments
According to Ar/Ar dating of impact glass, the El’gygytgyn Impact took place at 3.58 ± 0.04 Ma (Layer, 2000). This age is supported by a normal polarity of the oldest sediments in Lake El’gygytgyn that are thought to be formed after the impact event in Lake El’gygytgyn, suggesting deposition during the Gauss Chron, after 3.588 Ma (Nowaczyk et al., 2013).
The oldest pollen and spore assemblages in the region may occur in the black clays at the bottom section 120 (units 6 and 7, Table 1). According to Belaya (personal comm., 2005), samples from these layers, from which no material was left for new pollen analyses, contained numerous pollen grains of Taxodiaceae, such as Taxodiaceaepolentis, Taxodiumpolentis, Sequoipolentis, and spores, such as Laevigatosporites and Sphagumsporites. They were attributed to the late Turonian Stage (c. 90 Ma), based on similarities to pollen assemblages in dated sections in the Mechekrynnetveem River valley (sections 105 and 106 in Belyi and Belaya, 1998; Figure 1B). These black clays with Turonian palynomorphs are discontinuously overlain by Quaternary deposits (Figure 5), without a “chaotic horizon” containing impactite glass spherules in between, as evidenced by the dominance of typical Quaternary taxa in the respective sediments (see below).
We do not know the absolute age of the studied pre-“chaotic horizon” sediments accumulated in the sections 119 and 121. Paleomagnetic measurements of the lowermost sediments below the chaotic horizon in the closely situated sections 2303 and 2403 (Figure 1B) yield an inverse polarity most likely pointing to a formation during the Gilbert Chron, ca. 5.9–3.6 Ma (Minyuk et al., 2006). However, the majority of the paleomagnetic samples from the pre-chaotic horizon from these sections demonstrate a normal polarity implying a sedimentation after the Gilbert/Gauss polarity change at 3.588 Ma (Nowaczyk et al., 2013). Taking in consideration paleomagnetic measurements of the sediments from the sections 2303 and 2403 and the age of the meteorite impact, we assume that the pre-“chaotic horizon” sediments in the sections 119 and 121 were also accumulated shortly before and/or after the Gilbert/Gauss polarity change but before the El’gygytgyn impact event around 3.588 Ma.
Pliocene sediments overlain by the chaotic horizon and most likely accumulated shortly before the meteorite impact event were found only in sections 119 (Figure 4) and 121 (Figure 6 and Table 1). Based on the section stratigraphy and its pollen composition (rather high percentages of broadleaved taxa), we assume that the oldest pollen assemblage of all analyzed samples is present in PZ I of section 121 (Figure 6). Pollen composition of PZ I reflects a dominance of spruce and shrub alder in the local vegetation. Pines were also common, however, it is difficult to conclude, whether pollen of Pinus s/g Haploxylon-type was produced by tree pines (e.g., modern trees of P. sibirica or P. koraiensis that belong to this subgenus are growing in southern regions of Siberia and southern Far East) or by shrub stone pine (e.g., P. pumila broadly distributed today in the north-eastern and southern Siberia, Kamchatka, and northern Japan). Because of the high percentages of Picea and Corylus as well as presence of Abies, we assume that most of fossil Pinus pollen grains were presumably produced by tree pines. The relatively high percentages of A. fruticosa pollen suggests that shrub alders were also common in the regional forests and most likely stone pine could also grow in the area, especially at higher elevations.
Pollen grains of Larix/Pseudotsuga-type are also rather common in PZ I, suggesting that larch was common in the region. However, it is also possible that these pollen grains might at least partly be produced by Pseudotsuga (Douglas fir), which nowadays grows in western North America and in eastern Asia.
High charcoal percentages in PZ I of section 121 indicate that wildfires were frequent during the accumulation of PZ I sediments. The dominance of morphotype M charcoals with some contributions of elongated morphotypes point to burning of leaves, structured woody biomass and grasses present in forest undergrowth (Enache and Cumming, 2007; Pereboom et al., 2020).
A rather high percentages of Corylus pollen as well as the presence of pollen of other relatively thermophilic taxa in the sediments point to a relatively open forest and a rather warm climate during the PZ I interval. The summer temperatures were probably at least 10°C warmer than today as suggested by the presence of hazel in the local vegetation, thus further supporting the spread of fires. The high presence of ericaceous pollen and Sphagnum spores reflect that open wetlands with shrubby and boggy habitats were common in the area. Numerous colonies of Botryococcus and Pediastrum found in the spectrum of the loamy host sediments indicate a sedimentation in a small pond, in which these green algae could grow (Table 1).
Pollen assemblages of PZ II of section 121 (Figure 6) are similar to the lowermost three samples in section 119 (Figure 4) and reflect a dominance of spruce forests in the study area. Pines, firs, birches, larches, and alder, however, became more common compared to the time interval reflected by PZ I of section 121. Charcoal percentages reaching maximum values in two PZ II samples document increased wildfire activities around the study site. The permanent presence of pollen of broad-leaved (Corylus, Carpinus, and Ulmus) and single pollen grains of other relatively thermophilic taxa (Tsuga, Myrica, and Lonicera) indicate the growth of these plants might have also grown in the study area. The inferred climate conditions remained rather warm during this interval, although the broader distribution of shrubby ericaceous, fern- and Sphagnum-dominated habitats in the area point to wetter conditions.
Generally, pollen assemblages from PZ III of section 121, which directly underlies the chaotic horizon (Figure 6 and Table 1), reflect environmental conditions similar to the previous interval. However, the PZ III sediments are especially rich in plant remains compared to the underlain sediments. The identified macrofossil remains (Figure 7) confirm that firs, larches and ericaceous shrubs grew in the area. The increased pollen percentages of Alnus, Cyperaceae, and Sphagnum spores in the uppermost pollen assemblage point to significantly wetter conditions shortly before the impact event. This is also supported by a slightly lower charcoal concentrations, which were maximum in comparison of all analyzed samples. A slight shift from dominating more translucent morphotype M charcoals to opaque type P might reflect a slight change toward woodier burnt biomass or slightly higher intensities of fires.
Generally, sediments below the chaotic horizon were previously known from the study area: sites 10, 107, and 108 on Figure 1B (Belyi et al., 1994) sites 2304 and 2303 on Figure 1B (Glushkova et al., 2005; Minyuk et al., 2006). These sediments contain pollen of Picea, Pinus s/g Haploxylon, Larix, Abies, Betula, and Alnus with some rare pollen of relatively thermophilic taxa like Tsuga, Myrica, Carpinus, Corylus, Quercus, Juglans, Ulmus, Ilex, and Acer, Unfortunately, these pollen data were not properly published, and therefore are not available for the comparison with the newly studied records.
Sediments below the chaotic horizon collected for macrofossil and pollen studies during the expedition ‘El’gygytgyn Lake 2003’ (sites 2303 and 2403 on Figure 1B; Glushkova et al., 2005) contained numerous plant remains of Larix, Betula, Alnus, Pinus, Rubus, and Aracispermum, an extinct thermophilic shrub from the Myricaceae family (Minyuk et al., 2006). Numerous remains of Sphagnum and ericaceous plants (Ledum palustre, Andromeda, and Vaccinium) typical for Sphagnum-dominated habitats also occur in the studied sediments.
Upper Pliocene pollen records from adjacent areas of Chukotka and northeastern Siberia (e.g., Fradkina, 1983, 1988; Giterman, 1985; Volobueva et al., 1990; Grinenko et al., 1998; Fradkina et al., 2005a,b and references therein) also document the widespread distribution of forests with spruce, birch, alder, larch, and hemlock. Unfortunately, the poor age control does not allow a more precise comparison of the records. Early Pliocene pollen and macrofossil records from central and northern Yukon and Alaska exhibit that mixed boreal forests with pine, fir, larch, spruce, birch, alder, larch, and Douglas fir dominated the local vegetation there (e.g., Schweger et al., 2011 and references therein). In the Canadian Arctic (Ellesmere Island), an early Pliocene record from Strathcona Fjord shows that forest with larch, spruce, pine, birch and alder dominated nowadays treeless area (Fletcher et al., 2019).
In accordance with these Pliocene pollen data showing widespread forests north of the modern treeline, the Late Pliocene pollen record from Lake Baikal yielded widespread mixed coniferous (Pinus, Abies, Larix, and Tsuga) forests with some broadleaved taxa (Quercus, Tilia, Corylus, and Juglans) in southern Siberia (Demske et al., 2002). This points to significantly warmer environmental conditions than today also in the southern boreal forests around Lake Baikal.
Based on pollen and macrofossil records from several sites in Chukotka, Glushkova and Smirnov (2007) assumed that the Pliocene climate was much warmer than today, with mean January temperatures estimated to be −13 to −17°C (at least 15° higher than modern) and mean July temperatures 14–17°C (ca. 8–10° higher than modern). These estimates are similar to biomarker-based average mean summer temperature estimates of 15.4 ± 0.8°C in the Canadian Arctic around that time (Fletcher et al., 2019). This summer temperature estimate clearly exceed the proposed threshold that predicts a substantial increase in wildfire in the modern high latitudes (Young et al., 2017), which is confirmed by high concentrations of sedimentary charcoal in the pre-impact sediments.
Chaotic Horizon
Investigations of palynomorphs and charcoal in the “chaotic horizon” were carried out in the upper part of section 119 (Figure 4). Palynomorph compositions are similar to the pre-impact pollen assemblages, except for a lower pollen and a higher charcoal concentration. This confirms earlier palynological studies by Belaya (personal comm., 2005) on the “chaotic horizon” of sections 121, 10, and 108 (Figure 1B; Belyi et al., 1994). In contrast to the pre-impact sediments, the “chaotic horizon” of section 119 is distinctive because of its high charcoal concentrations (Figure 4).
We assume that the “chaotic horizon,” which has a thickness of ca. 2 m in section 119, was caused by the meteorite impact and accumulated soon after the event. The chaotic nature of the sediments and the similarity of the pollen assemblages within and below the horizon suggest that the sediments originates from pre-impact material that was disturbed by impact-induced earthquake activity or shock waves of near-surface sediments, which were formed shortly before the impact and redeposited by fluvial-alluvial or aerial transport. An allochthonous contribution to the “chaotic horizon” is evident by the distinctly increased admixture of charcoal (maximal charcoal concentrations in comparison of all analyzed samples), which originates from widespread burning of existing vegetation caused by the meteorite impact affecting a much larger amount of biomass compared to normal wildfires.
Post-impact Event Environments
It is difficult to estimate the age of the investigated sediments on top of the chaotic horizon, taking in consideration the possible hiatuses and lack of any absolute age control. From the comparison with the El’gygytgyn pollen record (Andreev et al., 2014), however, the pollen assemblages and in particular the common high percentages of Picea and Abies pollen in PZ I of section 124 (Figure 8), PZ I of section 120 (Figure 5), and PZ I of section 118 (Figure 3) enables us to constrain to a Late Pliocene age of these deposits.
Amongst the Late Pliocene sediments, PZ I in section 124 (Figure 8) is unique, because the sequence lacks a ‘chaotic horizon’, suggesting a post-impact age. Its pollen assemblages however, document that pine-spruce forests with some firs, birches, larches, and alder dominated the vegetation. The presence of pollen of thermophilic taxa (Corylus, Carpinus, Ulmus, Tsuga, and Myrica) reflect that these taxa might still have grown in the area, thus suggesting that the sediments were deposited during an interval, which was the warmest of all post-impact sequences, potentially equivalent to oldest Late Pliocene in the Lake El‘gygytgyn record (Andreev et al., 2014). The dominance of irregular, opaque morphotype P charcoals with minor contribution of the angular opaque type C suggests relatively high fire intensities typical for burning woody biomass in forest fires. Sphagnum-dominated habitats with numerous ericaceous plants were common, thus pointing to a relatively wet climate. Hence, the vegetation cover and climate conditions shortly after the impact event were rather similar to those before, though decreased amounts of broad-leaved pollen taxa suggest a slight cooling.
The pollen spectra from PZs I of sections 120 (Figure 5) and 118 (Figure 3) reflect that mainly pine forests with some spruce, birches, larches, firs and alder dominated the vegetation in the area. Despite an obvious sub-aquatic formation of the partly loamy sediments (Table 1) as also indicated by high percentages of Botryococcus colonies, reduced amounts of Sphagnum spores and Ericales pollen indicate a general reduction of boggy habitats in the study area. The climate conditions reconstructed for PZs I of sections 120 and 118 were much drier and colder compared to PZ I of section 124, indicating an asynchronous formation, presumably some time later.
Although the age control of PZ II in section 120 is also limited, the comparison with the continuous El’gygytgyn pollen record (Andreev et al., 2014) suggests that the pollen spectra were accumulated during some Late Pliocene interstadial. Larch forests with stone pines and some birches, spruces, and shrub alders dominated the vegetation in the area. Open shrubby habitats with dwarf birches and ericaceous low shrubs were also common.
Upper Pleistocene
Pollen assemblages of PZ III in section 118 (Figure 3) are dominated by Betula, Alnus and Pinus. In the El’gygytgyn pollen record (e.g., Lozhkin et al., 2007, 2017; Matrosova, 2009; Andreev et al., 2014; Zhao et al., 2018, 2019), similar pollen assemblages have only been reported from the Late Pleistocene. During PZ III of section 118, larch forests with stone pines, shrub alders, dwarf birches, and ericaceous low shrubs in underbrush dominated the vegetation cover. Climate conditions were relatively mild as can be inferred from pollen assemblages. High percentages of Botryococcus colonies and cysts of Volvocales (Figure 2) point to accumulation in an aquatic environment, probably in a floodplain pond. Similar contributions of larch pollen enables a correlation of PZ III in section 118, to environmental conditions during an interglacial in the well-dated Lake El’gygytgyn record, tentatively Marine Isotope Stage (MIS) 5e. The pollen assemblage of the overlaying PZ IV of section 118 (Figure 3), with increased Artemisia and S. rupestris percentages, reflects colder and drier environmental conditions, which might have occurred during Late Pleistocene (MIS 4 – MIS 2). Furthermore, the drastic decrease in algae remains points to a local shift toward sedimentation in a non-lacustrine environment.
Very similar pollen assemblages of PZ III in section 124 (Figure 8) and PZ IV in section 121 (Figure 6) indicate a synchronous deposition during MIS 5. Differing charcoal morphotype compositions, on the other hand, suggest that fire regimes were different during the deposition of the associated stratigraphic units of section 121 and 124. A dominance of irregular, translucent morphotype M charcoals and high contributions of elongated charcoals in PZ III of section 124 potentially points to lower fire intensities due to burning of leaves, branches and grasses during forest surface fires. In PZ IV of section 121, in contrast, opaque, irregular morphotype P and angular opaque type C point to higher-intensity fires, which have burned woodier biomass.
Late Glacial and Holocene
PZ III pollen assemblages of section 120 (Figure 5) exhibit low pollen percentages of shrubs and trees, while Artemisia and S. rupestris percentages are relatively high. Comparisons with regional pollen records (e.g., Lozhkin et al., 2007; Matrosova, 2009; Andreev et al., 2012) suggest that the PZ III sediments were formed during the Late Glacial.
The topmost PZ V pollen spectrum of section 118 (Figure 3) with relatively high percentages of shrubs and trees, most likely accumulated during the Holocene thermal optimum (early and middle Holocene). Shrub alder stands dominated the study area during this time, whereas, open dwarf birch and herb (Poaceae, Cyperaceae, Artemisia) plant communities were also common in the Lake El’gygytgyn area (Andreev et al., 2012 and references therein). Furthermore, relatively high percentages of Botryococcus colonies in the sediments point to an accumulation in an aquatic environment (e.g., floodplain pond as also suggested be the loamy host sediment, Table 1).
Finally, the pollen assemblages from the uppermost PZs of sections 120 (Figure 5) and 118 (Figure 3), representing the modern soils (Table 1), well reflect the modern regional vegetation cover with numerous dwarf birches and some shrub alder stands that prevailed during most of the Holocene in the valleys of the rivers around Lake El’gygytgyn (Andreev et al., 2012 and references therein).
Overall, the preservation of fluvial sediments downstream of Lake El’gygytgyn during the Late Pliocene and Late Pleistocene and the lack of sediments with typical early Pleistocene pollen assemblages might be related to an incomplete record of fluvial sediment sequences mapped in the field. However, it could also point to distinct changes in the long-term fluvial network evolution of the upper catchment of the Anadyr River basin due to varying rates of fluvial backward incision and sediment aggregation in response to global sea and base level changes – requiring a more comprehensive mapping of fluvial terrace sediments together with the usage of modern dating approaches.
Conclusion
Pollen assemblages in non-continuous terrestrial deposits have been used for a long time to characterize paleoenvironments and as relative stratigraphic markers for sediments that lack an absolute age control. Here, we also included microcharcoal composition as complementary stratigraphic markers, extending the approach using charcoal concentrations and size classes (Innes and Simmons, 2000) with charcoal morphotypes. Furthermore, charcoal morphotype composition allows to compare relative changes in fire intensities and type of biomass burnt, even from pollen-slide derived charcoals.
The recurrence of similar environmental conditions during multiple warm-to-cold glacial-interglacial cycles over the Pliocene and Pleistocene, as evidenced by pollen composition of the continuously deposited Lake El’gygytgyn sediments, hampers a precise temporal correlation of stratigraphic units. However, these first charcoal records from the northeastern Russian Arctic together with the new palynological records suggest that vegetation, fire and climate regimes were clearly different during the deposition of the five fluvial sediment sections south of Lake El’gygytgyn. Using the ‘chaotic’ impact horizon deposited that formed 3.58 ± 0.04 Ma as an absolute time marker, we reconstructed the pre-impact environments, thus extending the Lake El’gygytgyn record and offer unique insights on the impact of an meteorite impact on the regional vegetation:
(i) Prior to the impact event (between ca. 3.60 and 3.58 Ma), spruce forests with pines, firs, birches, larches, and alder dominated the study area. Some broad-leaved and relatively thermophilic taxa such as hazel might have also grown in the area. Wildfires were common as sufficient flammable biomass was available to burn and critical climate thresholds exceeded to allow fires to spread. Shrubby and boggy habitats with ericaceous plants and Sphagnum were very common in the area during rather wet intervals and reduced wildfires.
(ii) The impact event 3.58 ± 0.04 Ma ago was associated with strongly enhanced fires, which are reflected in up to 4 times elevated charcoal concentrations in the associated “chaotic horizon.” Yet, the event had no long-lasting impact on the regional vegetation, which obviously reestablished relatively shortly after the event without major compositional changes.
(iii) Pine-spruce forests with some firs, birches, larches, and alder dominated the Late Pliocene vegetation in the study after the impact event. Some thermophilic taxa might still grow in the area reflecting rather warm and wet climate, which is in accordance to the Lake El’gygytgyn pollen record.
(iv) Larch forests with shrub stone pines, shrub alders, dwarf birches, and ericaceous taxa in underbrush dominated in vegetation cover during a Late Pleistocene interglacial, attributed to MIS 5. Pollen spectra from the upper sediments reflect a treeless glacial landscape, while pollen spectra from the uppermost sediments and modern soils reflect dominance of Holocene shrubby vegetation.
The finding of sedimentary charcoal in all analyzed samples, even in rather coarse sediments of a dynamic fluvial environment, points to the important role of wildfires throughout the Late Pliocene when coniferous forests grew in the high-latitudes of northeastern Russia. The variability in charcoal morphotypes suggest varying fire regimes with time, which shows a high potential to quantify past fire regimes from periods that can serve as climatic analogs in a warmer future using the well-dated, continuous sediments of Lake El’gygytgyn.
Data Availability Statement
The raw data supporting the conclusions of this article will be made available by the authors, without undue reservation.
Author Contributions
OG and VS described the sediment sections and collected the samples. AA performed the pollen and NPPs analyses. ED performed the charcoal analyses. AA and ED prepared the first draft of the manuscript. All authors listed have made a substantial, direct and intellectual contribution to the work, and approved it for publication.
Funding
The work of AA was financed by Glacial Legacy grant from European Research Council Consolidator Grant 2018–2023 and partly supported by the Russian Government Program of Competitive Growth of Kazan Federal University. ED was funded by a project of the German Research Foundation within the ICDP priority program 1006 (DI 2544/1-1 and # 419058007).
Conflict of Interest
The authors declare that the research was conducted in the absence of any commercial or financial relationships that could be construed as a potential conflict of interest.
Acknowledgments
We warmly acknowledge the assistance of Tabea Tessendorf in the charcoal analysis. We also would like to acknowledge Angelica Feurdean and Julie Brigham-Grette for their valuable comments and suggestions for the earlier version of the manuscript.
References
Andreev, A. A., Morozova, E., Fedorov, G., Schirrmeister, L., Bobrov, A. A., Kienast, F., et al. (2012). Vegetation history of central Chukotka deduced from permafrost paleoenvironmental records of the El’gygytgyn Impact Crater. Clim. Past 8, 1287–1300. doi: 10.5194/cp-8-1287-2012
Andreev, A. A., Tarasov, P. E., Wennrich, V., and Melles, M. (2016). Millennial-scale vegetation changes in the north-eastern Russian Arctic during the Pliocene/Pleistocene transition (2.7-2.5 Myr BP) inferred from the Lake El’gygytgyn pollen record. Quat. Sci. Rev. 147, 245–258. doi: 10.1016/j.quascirev.2016.03.030
Andreev, A. A., Tarasov, P. E., Wennrich, V., Raschke, E., Herzschuh, U., Nowaczyk, N. R., et al. (2014). Late Pliocene and Early Pleistocene environments of the north-eastern Russian Arctic inferred from the Lake El’gygytgyn pollen record. Clim. Past 10, 1–23.
Belyi, V. F., and Belaya, B. V. (1998). The late stage of the Okhotsk-Chukchi volcanogenic belt development (the upper stream of the Enmyvaam River). Magadan: SVKNII, FEB RAS.
Belyi, V. F., Belaya, B. V., and Raikevich, M. I. (1994). Pliocene sediments of the upper stream of the Enmyvaam River and the age of impact genesis of the El’gygytgyn Lake basin., Magadan: SVKNII.
Berglund, B. E., and Ralska-Jasiewiczowa, M. (1986). “Pollen analysis and pollen diagrams,” in Handbook of Holocene palaeoecology and palaeohydrology, ed. B. E. Berglund (Chichester: Wiley), 455–484.
Bobrov, A. E., Kupriyanova, L. A., Litvintseva, M. V., and Tarasevich, V. F. (1983). Spores and pollen of gymnosperms from the flora of the European part of the USSR. Leningrad: Nauka.
Brigham-Grette, J., Melles, M., Minyuk, P., Andreev, A., Tarasov, P., DeConto, R., et al. (2013). Pliocene warmth, extreme polar amplification, and stepped Pleistocene cooling recorded in NE Russia. Science 340, 1421–1427. doi: 10.1126/science.1233137
Conedera, M., Tinner, W., Neff, C., Meurer, M., Dickens, A. F., and Krebs, P. (2009). Reconstructing past fire regimes: methods, applications, and relevance to fire management and conservation. Quat. Sci. Rev. 28, 555–576. doi: 10.1016/j.quascirev.2008.11.005
Demske, D., Mohr, B., and Oberhänsli, H. (2002). Late Pliocene vegetation and climate of the Lake Baikal region, southern East Siberia, reconstructed from palynological data. Palaeogeog. Palaeoclimatol. Palaeoecol. 184, 107–129. doi: 10.1016/s0031-0182(02)00251-1
Enache, M. D., and Cumming, B. F. (2007). Charcoal morphotypes in lake sediments from British Columbia (Canada): an assessment of their utility for the reconstruction of past fire and precipitation J. Paleolimnol. 38, 347–363. doi: 10.1007/s10933-006-9084-8
Fletcher, T. L., Warden, L., Sinninghe Damsté, J. S., Brown, K. J., Rybczynski, N., Gosse, J. C., et al. (2019). Evidence for fire in the Pliocene Arctic in response to amplified temperature. Clim. Past 15, 1063–1081. doi: 10.5194/cp-15-1063-2019
Fradkina, A. F. (1983). Neogene palynofloras of North-East Asia: Yakutia, Okhotsk Region, Chukotka, and Kamchatka). Moscow: Nauka.
Fradkina, A. F. (1988). “Palynology of Paleogene and Neogene of North-East Asia,” in Palinologiya v SSSR, ed. A. F. Khlonova (Novosibirsk: Nauka), 134–130.
Fradkina, A. F., Alekseev, M. N., Andreev, A. A., and Klimanov, V. A. (2005a). East Siberia, in: Cenozoic climatic and environmental changes in Russia. Geol. Soc. Amer. Spec. Pap. 382, 89–103.
Fradkina, A. F., Grinenko, O. V., Laukhin, S. A., Nechaev, V. P., Andreev, A. A., and Klimanov, V. A. (2005b). Northeastern Asia. in Cenozoic climatic and environmental changes in Russia. Geolog. Soc. Amer. Spec. Pap. 382, 105–120.
Galanin, A. V., Belikovich, A. V., Galanin, A. A., and Tregubov, O. D. (1997). Priroda i resursy Chukotki (Nature and sources of Chukotka). Magadan: IBPS FEB RAS.
Glushkova, O. (1993). Geomorphology and the history of the relief development of the Lake El’gygytgyn region. In Nature of the Lake El’gygytgyn basin (problems of study and protection). Ed V. F. Belyi (Magadan: SVKNII FEB RAS).
Glushkova, O. Yu, and Smirnov, V. N. (2007). Pliocene to Holocene geomorphic evolution and paleogeography of the Lake El’gygytgyn region, NE Russia. J. Paleolim. 37, 37–47.
Glushkova, O., Smirnov, V., and Minyuk, P. (2005). Enmyvaam River. Berichte zur Polar und Meeresforschung 509, 71–84.
Goderis, S., Wittmann, A., Zaiss, J., Elburg, M., Ravizza, G., Vanhaecke, F., et al. (2013). Testing the ureilite projectile hypothesis for the El’gygytgyn impact: determination of siderophile element abundances and Os isotope ratios in ICDP drill core samples and melt rocks. Meteorit. Planet. Sci. 48, 1296–1324. doi: 10.1111/maps.12047
Grinenko, O. V., Sergeenko, A. I., and Belolyubsky, I. N. (1998). Paleogene and Neogene of northeastern Russia. Part 1. Explanatory note to the regional stratigraphic scheme of Paleogene and Neogene sediments in North-East of Russia. Yakutsk: Yakutsk Scientific Center of SB RAS.
Innes, J. B., and Simmons, I. G. (2000). Mid-Holocene charcoal stratigraphy, fire history and palaeoecology at North Gill, North York Moors, UK. Palaeogeogr. Palaeoclimatol. Palaeoecol. 164, 151–165. doi: 10.1016/s0031-0182(00)00184-x
Kupriyanova, L. A., and Alyoshina, L. A. (1972). Pollen and spores of plants from the flora of European part of USSR, Vol. I., Leningrad: Academy of Sciences USSR, Komarov Botanical Institute.
Kupriyanova, L. A., and Alyoshina, L. A. (1978). Pollen and spores of plants from the flora of European part of USSR. Academy of Sciences USSR. Leningrad: Komarov Botanical Institute.
Layer, P. W. (2000). Argon–40/argon–39 age of the El’gygytgyn impact event, Chukotka, Russia. Meteorit. Planet. Sci. 35, 591–599. doi: 10.1111/j.1945-5100.2000.tb01439.x
Lozhkin, A. V., Anderson, P. M., Matrosova, T. V., and Minyuk, P. S. (2007). The pollen record from El’gygytgyn Lake: implications for vegetation and climate histories of northern Chukotka since the late middle Pleistocene. J. Paleolim. 37, 135–153. doi: 10.1007/s10933-006-9018-5
Lozhkin, A. V., Minyuk, P. S., Anderson, P. M., Nedorubova, E. Yu, and Korzun, Y. A. (2017). Variability in landscape and lake system responses to glacial and interglacial climates during the Middle Pleistocene based on palynological and geochemical data from Lake El’gygytgyn, eastern Arctic. Rev. Palaeobot. Palynol. 246, 1–13. doi: 10.1016/j.revpalbo.2017.06.004
Matrosova, T. V. (2009). Vegetation and climate changes in northern Chukotka during the last 350000 years inferred from palynological data from sediments of El’gygytgyn Lake. Vestnik 2, 23–30.
Melles, M., Brigham-Grette, J., Minyuk, P. S., Nowaczyk, N. R., Wennrich, V., DeConto, R. M., et al. (2012). 2.8 Million years of arctic climate change from Lake El’gygytgyn. NE Russia. Sci. 337, 315–320.
Melles, M., Brigham-Grette, J., Minyuk, P., Koeberl, C., Andreev, A., Cook, T., et al. (2011). The Lake El’gygytgyn Scientific Drilling Project - Conquering Arctic Challenges in Continental Drilling. Sci. Drill. 11, 29–40. doi: 10.5194/sd-11-29-2011
Minyuk, P. S., Glushkova, O. Y., Smirnov, V. N., Lozhkin, A. V., Matrosova, T. V., Plyshkevich, A. A., et al. (2006). Paleoclimatic data of El’gygytgyn Lake. Information report for 2004-2006. Magadan: NEISRI FEB RAS.
Mottaghy, D., Schwamborn, G., and Rath, V. (2013). Past climate changes and permafrost depth at the Lake El’gygytgyn site: implications from data and thermal modeling. Clim. Past 9, 119–133. doi: 10.5194/cp-9-119-2013
Mustaphi, C. J. C., and Pisaric, M. F. J. (2014). A classification for macroscopic charcoal morphologies found in Holocene lacustrine sediments. Progress Phys. Geogr. Earth Environ. 38, 734–754. doi: 10.1177/0309133314548886
Nolan, M., and Brigham-Grette, J. (2007). Basic hydrology, limnology, and meteorology of modern 645 El’gygytgyn Lake. Siberia. J. Paleolim. 37, 17–35. doi: 10.1007/s10933-006-9020-y
Nowaczyk, N. R., Haltia, E. M., Ulbricht, D., Wennrich, V., Sauerbrey, M. A., Rosén, P., et al. (2013). Chronology of Lake El’gygytgyn sediments – a combined magnetostratigraphic, palaeoclimatic and orbital tuning study based on multi-parameter analyses. Clim. Past 9, 2413–2432. doi: 10.5194/cp-9-2413-2013
Pereboom, E. M., Vachula, R. S., Huang, Y., and Russell, J. (2020). The morphology of experimentally produced charcoal distinguishes fuel types in the arctic tundra. Holocene 30, 1091–1096. doi: 10.1177/0959683620908629
Reille, M. (1992). Pollen et spores d’Europe et d’Afrique du nord. Marseille: Laboratoire de Botanique Historique et Palynologie.
Reille, M. (1995). Pollen et spores d’Europe et d’Afrique du nord, supplement 1. Marseille: Laboratoire de Botanique Historique et Palynologie.
Reille, M. (1998). Pollen et spores d’Europe et d’Afrique du nord, supplement 2. Marseille: Laboratoire de Botanique Historique et Palynologie.
Schwamborn, G., Fedorov, G., Schirrmeister, L., Meyer, H., and Hubberten, H.-W. (2008). Periglacial sediment variations controlled by late Quaternary climate and lake level change at El’gygytgyn Crater. Arctic Siberia. Boreas 37, 55–65. doi: 10.1111/j.1502-3885.2007.00011.x
Schweger, C., Froese, D., White, J. M., and Westgate, J. (2011). Pre-glacial and interglacial pollen records over the last 3 Ma from northwest Canada: Why do Holocene forests differ from those of previous interglaciations? Quat. Sci. Rev. 30, 2124–2133. doi: 10.1016/j.quascirev.2011.01.020
Smirnov, V. N., Savva, N. E., Glushkova, O., and Yu. (2011). New data on spherules from the El’gygytgyn Crater. Geochemistry Inter. 49, 314–318. doi: 10.1134/s0016702911030086
Stockmarr, J. (1971). Tablets with spores used in absolute pollen analysis. Pollen et Spores 13, 614–621.
Tarasov, P. E., Andreev, A. A., Anderson, P. M., Lozhkin, A. V., Haltia-Hovi, E., Nowaczyk, N. R., et al. (2013). A pollen-based biome reconstruction over 3.562 million years in Far East Russian Arctic - new insides into climate-vegetation relationship in regional scale. Clim. Past 9, 2759–2775. doi: 10.5194/cp-9-2759-2013
van Geel, B. (2001). “Non-pollen palynomorphs,” in Tracking environmental change using lake sediments. Volume 3: Terrestrial, algal and silicaceous indicators, eds J. P. Smol, H. J. B. Birks, W. M. Last, R. S. Bradley, and K. Alverson (Dordrecht: Kluwer), 99–119.
Volobueva, V. I., Belaya, B. V., Polovova, T. P., and Narkhinova, V. E. (1990). Marine and continental Neogene of North-East of USSR, Issue 1. Magadan: Miocene SVKNII.
Wennrich, V., Andreev, A. A., Tarasov, P. E., Fedorov, G., Zhao, W., Gebhardt, C. A., et al. (2016). 3.6 Myr climate and environmental variability in the terrestrial Arctic as inferred from the unique sediment record of Lake El’gygytgyn, Far East Russia – a review. Quart. Sci. Rev. 147, 221–244. doi: 10.1016/j.quascirev.2016.03.019
Wittmann, A., Goderis, S., Claeys, P., Vanhaecke, F., Deutsch, A., and Adolph, L. (2013). Petrology of impactites from El’gygytgyn Crater: breccias in ICDP-drill core 1C, glassy impact melt rocks and spherules. Meteorit. Planet. Sci. 48, 1199–1235. doi: 10.1111/maps.12019
Young, A. M., Higuera, P. E., Duffy, P. A., and Hu, F. S. (2017). Climatic thresholds shape northern high-latitude fire regimes and imply vulnerability to future climate change. Ecography 40, 606–617. doi: 10.1111/ecog.02205
Zhao, W., Andreev, A. A., Tarasov, P. E., Wennrich, V., and Melles, M. (2019). Vegetation and climate during the penultimate interglacial of the northeastern Russian Arctic: the Lake El’gygytgyn pollen record. Boreas 48, 505–515.
Zhao, W., Tarasov, P. E., Lozhkin, A. V., Anderson, P. M., Andreev, A. A., Korzun, J. A., et al. (2018). High-latitude vegetation and climate changes during the Mid-Pleistocene Transition inferred from a palynological record from Lake El’gygytgyn, NE Russian Arctic. Boreas 47, 137–149. doi: 10.1111/bor.12262
Keywords: fluvial sedimentary outcrops, pollen, charcoals, northeastern Russian Arctic, early Pliocene
Citation: Andreev A, Dietze E, Glushkova O, Smirnov V, Wennrich V and Melles M (2021) The Environment at Lake El’gygytgyn Area (Northeastern Russian Arctic) Prior to and After the Meteorite Impact at 3.58 Ma. Front. Earth Sci. 9:636983. doi: 10.3389/feart.2021.636983
Received: 02 December 2020; Accepted: 29 March 2021;
Published: 16 April 2021.
Edited by:
Daniel Nývlt, Masaryk University, CzechiaReviewed by:
Angelica Feurdean, Goethe University Frankfurt, GermanyJulie Brigham-Grette, University of Massachusetts Amherst, United States
Copyright © 2021 Andreev, Dietze, Glushkova, Smirnov, Wennrich and Melles. This is an open-access article distributed under the terms of the Creative Commons Attribution License (CC BY). The use, distribution or reproduction in other forums is permitted, provided the original author(s) and the copyright owner(s) are credited and that the original publication in this journal is cited, in accordance with accepted academic practice. No use, distribution or reproduction is permitted which does not comply with these terms.
*Correspondence: Andrei Andreev, aandreev@awi.de; Elisabeth Dietze, elisabeth.dietze@awi.de