- 1College of Resource and Environment, Yangtze University, Wuhan, China
- 2Oil and Gas Survey, China, Geological Survey, Beijing, China
- 3PetroChina Research Institute of Petroleum Exploration and Development, Beijing, China
- 4Institute of Geology and Geophysics, Chinese Academy of Sciences, Beijing, China
The chemical and isotopic compositions of the natural gas and the co-produced flowback water from the XJC 1 well in Junggar Basin, China, were analyzed to determine the origin of gases in the Permian Lucaogou Formation (P2l) and the Triassic Karamay Formation (T2k) in the Bogda Mountain periphery area of the Southern Junggar Basin. The value of carbon isotope composition of the P2l lacustrine shale gas in the Junggar Basin was between the shale gas in Chang 7 Formation of Triassic (T1y7) in the Ordos Basin and that in the Xu 5 Formation of Triassic (T3x5) in the Sichuan Basin. The difference in gas carbon isotope is primarily because the parent materials were different. A comparison between compositions in the flowback water reveals that the P2l water is of NaHCO3 type while the T2k water is of NaCl type, and the salinity of the latter is higher than the former, indicating a connection between P2l source rock and the T2k reservoir. In combination with the structural setting in the study area, the gas filling mode was proposed as follows: the gas generated from the lacustrine source rocks of the Permian Lucaogou Formation is stored in nearby lithological reservoirs from the Permian. Petroleum was also transported along the faults to the shallow layer of the Karamay Formation over long distances before it entered the Triassic reservoir.
Introduction
Shale gas is a growing part of global unconventional energy, and the development of hydraulic fracturing technologies has paved the way for increasing gas production. China possesses the largest shale gas reserves worldwide, with a geological resource volume of 80.45 trillion m3. In 2019, China’s annual shale gas production reached 15.4 billion cubic meters, the second-highest in the world. However, many environmental concerns are associated with hydraulic fracturing for shale gas. Two major geochemical fields related to shale gas are currently focused on: 1) the composition and isotopes of shale gases and the related issues such as the gas origin and generating mechanism (Jenden et al., 1993; Hill et al., 2007; Martini et al., 2008; Burruss and Laughrey, 2010; Strąpoć et al., 2010; Hunt et al., 2012; Zumberge et al., 2012; Hao and Zou, 2013; Tilley and Muehlenbachs, 2013; Dai et al., 2014; Feng et al., 2016); and 2) the geochemistry of water coproduced with the shale gas and related environmental issues (Chapman et al., 2012; Warner et al., 2013, 2014; Vengosh et al., 2014; Vengosh et al., 2017; Lauer et al., 2016; Zheng et al., 2017; Ni et al., 2018; Zou et al., 2018; Liu et al., 2020).
Most global gas resources are located in marine shale. As a result, marine shale gas geochemistry has been extensively studied in the United States, Canada (Jenden et al., 1993; Hill et al., 2007; Martini et al., 2008; Burruss and Laughrey, 2010; Strąpoć et al., 2010; Hunt et al., 2012; Zumberge et al., 2012; Tilley and Muehlenbachs, 2013), and the Sichuan Basin of China (Hao and Zou, 2013; Dai et al., 2014; Feng et al., 2016). Moreover, the majority of studies on water geochemistry and the environmental issues related to shale gas exploration are about marine shale (Chapman et al., 2012; Vengosh et al., 2014, 2017; Warner et al., 2014; Ni et al., 2018; Zou et al., 2018; Liu et al., 2020). Few studies have been conducted on the geochemical characteristics of terrestrial shale gas (Dai et al., 2016) and the impact of hydraulic fracturing in terrestrial shale (Zheng et al., 2017).
From the Precambrian to the Neogene, abundant organic-rich shale strata developed in China. The shale formed in three major sedimentary environments, namely marine facies, marine-terrestrial transitional-lacustrine coal facies, and lacustrine facies. The recoverable shale gas generated from continental shale (marine-terrestrial transitional shale and lacustrine shale) reached 4.03 trillion m3, accounting for 31% of the recoverable shale gas resources in China. The gas geochemistry of the continental shale differs from that of marine shale, and the water chemistry and water-rock reaction process of the two kinds of shale are also quite different. The origin of shale gas and wastewater treatment plans both require further studies.
In 2019, the completion of Well XJC 1 in the Bogda Piedmont of Junggar Basin marked a significant breakthrough (Figure 1), with the daily gas production of 1.8 × 104 m3 and daily oil production of 0.41 t in the Permian Lucaogou Formation. Later, in the same well, gas was detected in the overlying Triassic Karamay Formation. This is the first time that the industrial gas flow of Lucaogou Formation and the Karamay Formation on the southern margin of the Junggar Basin have been obtained, and the result highlights the shale gas exploration potential in the Bogda piedmont of the Junggar Basin. The Lucaogou shale is of lacustrine facies and the continental shale is widely distributed in Western China.
In this work, the composition and isotope of gas in the Lucaogou Formation of Permian (P2l) was analyzed for well XJC 1. The source rock of the Lucaogou Formation in the periphery of Bogda Mountain was evaluated to establish future areas for shale gas exploration in the study area. The geochemical data of marine shale gas in the Longmaxi Formation and swamp shale gas in the Xu 5 Formation (both situated in the Sichuan Basin), and lake shale gas in the Chang 7 Formation (situated in the Ordos Basin) were obtained from the literature (Dai et al., 2016) to compare with the Lucaogou shale gas. The gas in the Karamay Formation of Triassic (T2k) could not be obtained; thus, the flowback water from the T2k reservoir and P2l shale was compared, in combination with the geological setting, to study the T2k and P2l gas origin and the gas filling mode. Additionally, the flowback fluid of unconventional shale reservoirs in the Lucaogou Formation and that of conventional reservoirs in the Karamay Formation were compared to analyze the unconventional flowback water and establish water environmental protection measures that should be implemented during shale gas exploration and development in the area.
Geological Characteristics of the Study Area
Regional Setting
The Bogda Mountain periphery includes two secondary structural units, the Fukang Fault Zone on the northern margin and the Chaiwopu Sag on the southern margin, located in the eastern part of the southern Junggar thrust belt in the Junggar Basin (Figure 1). The Fukang Fault Zone and the Chaiwopu Sag are divided by the uplift of the Bogda Mountains, structural evolution, and hydrocarbon generation. The accumulation area is structurally too complex for oil and gas exploration in northern Xinjiang. Well XJC 1 is located in the Fukang Fault Zone. This area has complex structural deformations, highly developed faults, and diverse trap types. Insufficient research on the source rocks of the Permian Lucaogou Formation limits the evaluation of the oil and gas resource potential for this formation.
Structural Characteristics
The tectonic evolution of the Bogda Mountain periphery is divided into five stages: 1) Early Permian-Middle Permian when the basin was in the extensional rift stage. The whole area experienced the tectonic setting of crustal extension, and the basin was typically a rift or fault basin. 2) Late Permian-Triassic, the compression and flexure stage. In the Late Permian period, the basin entered the intra-continental evolution stage, the entire Triassic sedimentary depression continued to expand, and the continental deposits developed further in the southern Junggar. During this stage, the sedimentary depression was primarily braided river delta facies and semi-deep lake facies. 3) The Jurassic extension stage, when the early Yanshan movement caused substantial uplift and denudation of a wide range of the Jurassic deposits. These coal-measure strata evolved into the current scattered deposits in the Bogda Mountains and Tianshan Mountains. 4) The foreland stage of the Cretaceous depression. During the Cretaceous sedimentation period, the southern Junggar Basin was generally uplifted in a state of denudation. The basin was in the early Cretaceous compression depression with depositing filling after the late Jurassic denudation. The Late Cretaceous deposit was generally absent in the southern Junggar thrust belt. 5) During the Cenozoic compression foreland stage, the tectonic load generated by the thrust of the orogenic belt into the basin caused the crust of the orogenic belt to sink rapidly, forming the current structure of the basin (Figure 2A).
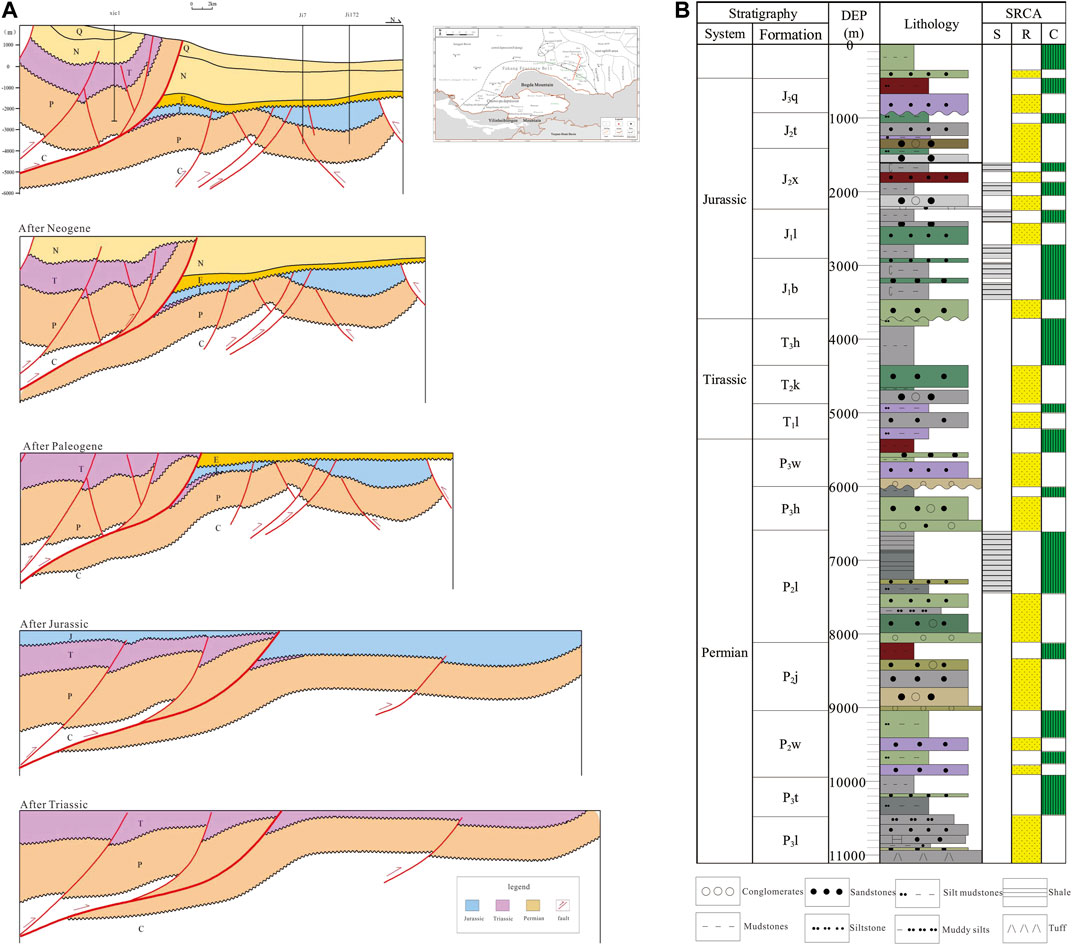
FIGURE 2. (A) The tectonic evolution of the periphery of the Bogda Mountain; (B) Chronostratigraphy of southern Junggar Basin.
Stratigraphic Characteristics
From bottom to top, the lower Permian includes the Shirenzigou Formation (P1l) and the Tashikula Formation (P1t), the Middle Permian includes the Wulabo (P2w), the Jingjingzigou (P2j), and the Lucaogou (P2l) Formation. The upper Permian is composed of the Hongyanchi (P3h) and Wutonggou (P3w) Formation. This study focused on the Permian Lucaogou Formation, which is composed of upward blackening mudstone interbedded with gray argillaceous sandstone in the lower section. The upper section of the Lucaogou Formation is dominated by fine-grained sediments, primarily dark gray sandy mudstone, mudstone, gray-black mudstone, and silty mudstone (Figure 2B). The Triassic is divided into the Lower Triassic Jiucaiyuan (T1l), the middle Triassic Karamay Formation (T2k), and Upper Triassic Huangshanjie Formation (T3h) from bottom to top. The lithology of the Karamay Formation is mainly gray, gray-green, yellow sandstone, compound sandstone, lithic sandstone, mixed with mudstone and conglomerate (Figure 2B).
Sample Collection
Three shale gas samples were collected from well XJC 1 in the Lucaogou Formation. The natural gas composition and carbon isotope characteristics were analyzed at the Xinjiang Oilfield Research Institute. Moreover, geochemical data of marine shale gas in the Longmaxi Formation and swamp shale gas in the Xu 5 Formation (both situated in the Sichuan Basin), and lake shale gas in the Chang 7 Formation (situated in the Ordos Basin) were obtained from the literature for comparison. Flowback water was collected from the well XJC 1 in the Karamay Formation from the first day after hydraulic fracturing. A total of 34 samples were collected during a 66–days period after the hydraulic fracturing of well XJC 1. The fracturing fluid for the Karamay Formation was also collected. The flowback fluid samples from the Lucaogou Formation in well XJC 1 were collected from day 14 to day 20 after fracturing, and a total of five samples were collected. Major element, trace element, and cation analyses of the water samples was conducted at the Beijing Institute of Geology of Nuclear Industry.
Evaluation of Lucaogou Shale
Geological Characteristics of Shale in the Lucaogou Formation
According to the comparison results of the drilling strata in the XJC 1, ZY 4, BC 1, MC 2, and CS 1 wells, the Permian source rock of the Lucaogou Formation is a set of deep lake-semi-deep lake deposits on the periphery of the Bogda Mountain (Fukang Fault Zone and Chaiwopu Sag, Figure 1). The deposition center is situated close to Bogda Mountain. The lithology is mainly organic-rich black-gray-black shale and mudstone, with silty mudstone and dolomite mudstone, representing a thick set of high-quality lacustrine argillaceous source rocks.
Distribution of Lucaogou Shale
The source rocks of the Lucaogou Formation are distributed throughout the region in the Fukang Fault Zone at the northern foot of the Bogda Mountain, with a thickness of approximately 260–50 m (Figure 1). The thicknesses of the Lucaogou Formation’s source rocks determined from the XJC 1, BC 1, and ZY 4 wells were 410 m, 330 m, and 270 m, respectively (Figure 1). In the Chaiwopu Sag at the southern foot of the Bogda Mountain, five wells, including Xiao1, Da1, and CS 1, revealed varying thicknesses of the Lucaogou Formation (Figure 1). Using well Xiao 1 as a representative, the source rock thickness of the Lucaogou Formation revealed is 284 m. From a horizontal perspective, the thickness of the Lucaogou Formation’s source rock in the Dahuangshan Piedmont is approximately 376 m and shows a trend of gradually increasing thickness from west to east.
Shale Geochemical Characteristics
The source rock of the Lucaogou Formation is rich in organic matter. Four wells were drilled in the source rock in the Fukang Fault Zone at the northern foot of the Bogda Mountain, namely XJC 1, BC 1, ZY 2, and ZY 4. The total organic carbon (TOC) contour map was drawn based on the TOC data of these wells (Figure 3). The average TOC content of the 161 source rock samples from well XJC 1 was 1.03%, and the highest TOC value was 5.45%. (Figure 4A). The average content of chloroform bitumen "A" of the 66 samples was 0.05%, and the highest was 0.16% (Figure 4B).
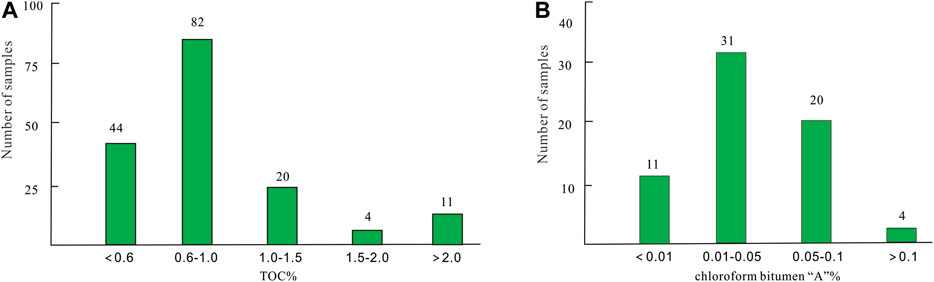
FIGURE 4. (A) TOC content frequency distribution and (B) chloroform bitumen “A”(%) content frequency distribution of source rock of Lucaogou Formation in Well XJC one.
Moreover, microscopic kerogen analysis of the Lucaogou Formation indicated that algae and amorphous bodies account for 60–83% of the kerogen composition, with trace concentrations of vitrinite and inertite. Most of the source rocks belong to type I-II1 kerogen, and their primary parent material was sourced from lower aquatic organisms dominated by algae (Figure 5).
The organic matter maturity of the shale in the Lucaogou Formation around the Bogda Mountain varies. The Lucaogou Formation in Quanzijie, where well XJC 1 is located, is deeply buried and has a high degree of thermal maturity. Light oil and wet gas are mainly produced in this region. The outcrop areas of the Dahuangshan and Fukang faults are shallow-buried and the thermal maturity degree is low. Moreover, significant quantities of oil shale occur in this region. The Chaiwopu and Jimsar sags display a moderate degree of thermal maturity and generate significant oil quantities (Figure 6).
The source rock maturity of the Lucaogou Formation encountered in well XJC 1 was generally high. The vitrinite reflectance (Ro) of 13 samples collected from this well ranged from 1.31 to 1.83, with an average of 1.71. Moreover, 12 of the samples exhibited Ro greater than 1.5, indicating that these rocks have entered a high-maturity stage (Figures 6, 7). Therefore, the production of gas instead of oil from well XJC 1 was attributed to the high maturity of the source rocks.
Origin and Filling Mode of the Gases
In this study, we used analysis data from lacustrine Lucaogou Formation shale gas in the Junggar Basin and data from several marine and terrestrial shale gas samples obtained from the literature (Dai et al., 2016; Feng et al., 2016), namely the continental Chang 7 shale gas in the southeastern Ordos Basin, marine Wufeng-Longmaxi shale gas in the southern Sichuan Basin, and swamp Xu 5 shale gas in the Xinchang gas field, to compare the alkane gas components and carbon isotopes in the lacustrine shale gas of the Lucaogou Formation with other marine and terrestrial shale gas samples, and to discuss the origin of the Lucaogou shale gas. Furthermore, we compared the flowback water of the Karamay Formation and the Lucaogou Formation to ascertain the origin and migration pathway of the gases. In combination with the geological setting, the filling mode of Permian and Triassic gas in the periphery of Bogda Mountain of the Junggar Basin was then proposed.
Origin of the Lucaogou Gas
Alkane Gas Composition
The methane, ethane, propane, and butane contents in the shale gas of well XJC 1 ranged from 72.32 to 77.12%, 7.92–8.92%, 2.01–2.21%, and 1.90–2.03%, with average contents of 74.14%, 8.49%, 2.13%, and 1.96%, respectively. Therefore, shale gas from the Lucaogou Formation is characterized by a high content of heavy hydrocarbon gas and is classified as wet gas, similar to that of the mature Ordos Chang 7 shale and the Barnett shale, one of the largest onshore natural gas fields in the United States (Figure 8).
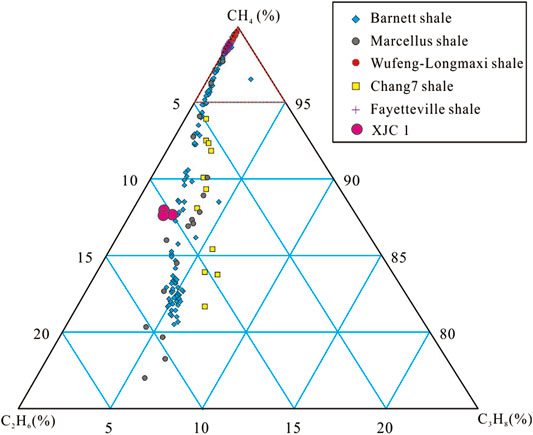
FIGURE 8. Triangular diagram of CH4-C2H6-C3H8 components of major shale gas in China and the United States (modified after Dai et al., 2016).
The sequence of heavy hydrocarbon gas (C2-5) content in shales from the study area is as follows: Lucaogou shale in the Junggar Basin > gas in the Lower Triassic Chang 7 (T1y7) shale of the Ordos Basin > gas in the Upper Triassic Xu 5 (T3x5) Shale of the Sichuan Basin. Moreover, the maturity of the Lucaogou Formation shale exceeded that of the other two sets of continental shale, indicating that the Lucaogou shale gas should be drier. Therefore, the variation in the alkane gas composition of these shale gases is predominately affected by the parent source rock and not the maturity of the shale rock. Although the source rocks for the Lucaogou and Chang 7 gases are both sapropel shale rocks, the Lucaogou shale contains more saprolite components that are prone to generate heavy hydrocarbons. Conversely, the Chang 7 shale gas, which is derived from humic parent material, is wetter than the Xu 5 shale gas, which is sourced from a sapropel parent material.
As can be seen from Figure 8, the marine Wufeng-Longmaxi shale gas has a high methane content, with an average of 98.00%, and a low heavy hydrocarbon gas content, while the continental Lucaogou, Chang 7, and Xu 5 shale gas all have low methane contents and high heavy hydrocarbon gas contents. These variations primarily arise from differences in the source rock maturity.
Carbon Isotopic Composition of Alkane Gas
The δ13C1 and δ13C2 compositions of the Lucaogou Formation gas ranged from −41.0‰ to −41.9‰ and 30.7‰ to −31.3‰, with averages of −41.5‰ and −31.1‰, respectively. The δ13C3 content ranged from −28.1‰ to −29.0‰, with an average of −28.6‰, and a δ13C4 range of −29.4‰ to −29.5‰ was recorded, with an average of −29.4‰.
Compared to the other continental shale gas samples, the carbon isotope values of shale gas in the Lucaogou Formation was higher than those of the Chang 7 shale gas, with δ13C1, δ13C2, δ13C3, and δ13C4 averages of −48.7‰, −36.4‰, −31.3‰, and −31.6‰, respectively. Moreover, the carbon isotope values of shale gas in the Lucaogou Formation were lower than those of the Xu 5 (T3x5) shale gas, which recorded δ13C1, δ13C2, δ13C3, and δ13C4 averages of −36.2‰, −25.1‰, −22.9‰, and −22.4‰. A maturity comparison of the three sets of shale revealed that the thermal maturity of the Lucaogou Formation shale exceeded that of the Chang 7 and Xu 5 shales. The higher the maturity, the heavier the carbon isotope composition of the alkane gas. The Luchaogou and Chang 7 shales have a similar parent material type. Therefore, the heavier alkane gas isotopes of the Lucaogou Formation gases are predominately caused by source rock maturity. The Xu 5 shale gas is derived from coal source rocks dominated by swamp facies in the Xujiahe Formation (Dai et al., 2014). The carbon isotopes of methane and the homologs produced by humic source rocks are heavier than those produced by saprophytic source rocks of similar maturity (Stahl and Carey, 1975; Dai and Qi, 1989). Zheng and Chen (2000) pointed out that the δ13C1 value of methane formed by marine sapropelic source rock is approximately 14‰ lower than that of terrestrial humic source rocks at the same maturity. Therefore, differences between the carbon isotope composition of alkane gas from the Lucaogou and Xu 5 shales can be attributed to different source rocks.
Figure 9 indicates that the continental shale gas from the Lucaogou, Chang 7, and Xu 5 formations displayed a predominately positive carbon isotope series (δ13C1<δ13C2<δ13C3<δ13C4). Conversely, the Wufeng-Longmaxi Formation shale gas samples recorded a negative carbon isotope series (δ13C1>δ13C2>δ13C3>δ13C4). The variations in the carbon isotope composition between the continental shale formations and the marine shale formation were attributed to varying degrees of shale maturity. The continental shale gases are in the mature to the high-mature stage, while the Wufeng-Longmaxi Formation marine shale is in the over-mature stage when carbon isotope reversal occurs.
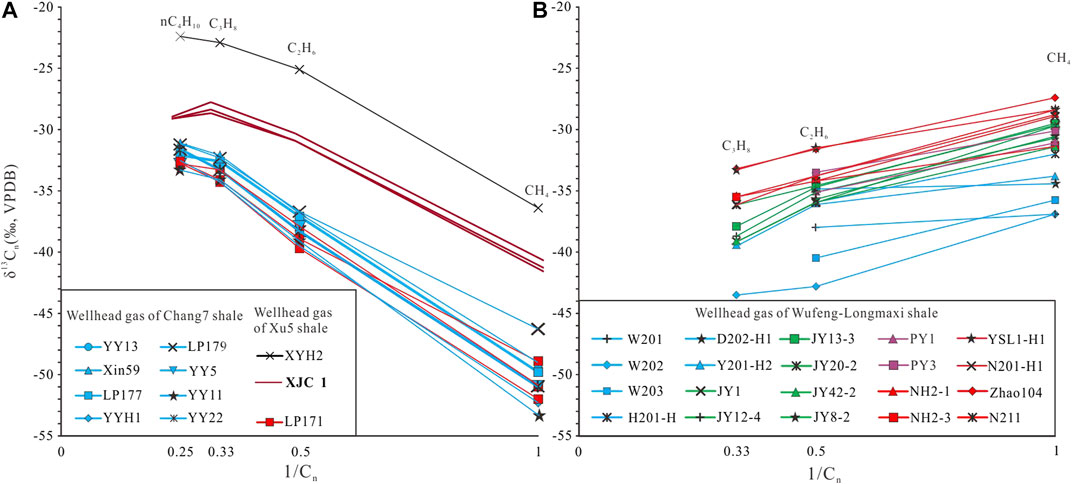
FIGURE 9. Comparison of carbon isotope series types of alkanes in Chang 7, Xu 5 (A) and Wufeng-Longmaxi Formation (B) (modified after Dai et al., 2016).
Overall, the geochemical analysis of well XJC 1 indicated that the gas was generated from the mature lacustrine source rock. The anomalous gas production of this well compared to other wells in the vicinity could be attributed to the high maturity of the source rocks. Therefore, future shale gas exploration of the Lucaogou Formation should focus on superimposed areas with high TOC and maturity.
Composition of Reservoir Waters From the XJC1 Well
Both the unconventional shale reservoirs in the Lucaogou Formation and the conventional reservoirs in the Karamay Formation have undergone hydraulic fracturing, and both reservoirs have produced flowback fluid. As the T2k gas was not collected in this study, the gas origin could not be determined directly. We, therefore, used the chemistry of water coproduced with the gas to examine the gas origin. We conducted a comparison of the geochemical characteristics of the flowback fluid for these two reservoirs to analyze the gas migration pathway, the reaction process of the fracturing fluid and formation water, water-rock reactions, and the sources of various elements in the flowback fluid of the different rocks.
Flowback Fluid of Karamay Formation Sandstone
Fracturing fluid samples used in the hydraulic fracturing of the Karamay Formation and flowback fluid from day 1–66 after hydraulic fracturing were collected and analyzed for comparison. A comparison of the results from the flowback fluid, the fracturing fluid, and the evolution trend of the flowback fluid over the production time, revealed several different sources of elements in the flowback fluid. Figure 10 indicates that the evolution trend of different elements in the flowback fluid can be divided into three types:
1) Type 1: This group comprised Li, B, F, and Br. The concentration of type 1 elements in the fracturing fluid was very high. In the flowback fluid, the type 1 element composition decreased rapidly on the first day after fracturing. However, on the 12th day (or possibly earlier–no samples were collected between days 1–12) the type 1 element concentration in the flowback fluid increased to the same level as the fracturing fluid and remained at a higher level during the later flowback process. In the flowback process, at the beginning of fracturing, the water injected into the formation entered the fracture. On the first day after hydraulic fracturing, most of the flowback fluid was in the target formation. Therefore, the compositions of these elements in the formation water were low. However, after approximately 12 days after fracturing, the proportion of fracturing fluid in the flowback fluid increased until the flowback fluid consisted predominately of fracturing fluid. Therefore, the concentration of type 1 elements in the flowback fluid increased and continued to maintain a level equivalent to that in the fracturing fluid.
2) Type 2: This group comprised Ca, Cl, Mg, K, Na, HCO3, and SO4. On the first day after fracturing, the concentration of type 2 elements in the flowback fluid increased rapidly and was significantly higher than in the fracturing fluid. On the 12th day after fracturing, the concentrations of these elements in the flowback fluid gradually decreased to a level equivalent to that of the fracturing fluid. The source of these elements was similar to the type 1 elements, except that the concentrations of these elements were high in the formation water and low in the fracturing fluid. At the start of fracturing, the flowback fluid was dominated by formation water; hence, the contents of these elements in the flowback fluid were higher than in the fracturing fluid. However, in the days following fracturing, the proportion of formation water in the flowback fluid progressively decreased, and the concentration of type 2 elements in the flowback fluid decreased to a level similar to that of the fracturing fluid.
3) Type 3: The third element group contained Mn, Ba, and Sr The concentration of type 3 elements in the fracturing fluid was low. However, during the flowback process, the elemental concentration remained high regardless of the number of days passed since hydraulic fracturing, and the concentration of type 2 elements in the flowback fluid significantly exceeded that of the fracturing fluid. Since type 2 elements could not be derived from the fracturing fluid or the formation water as they maintained a high concentration in the flowback fluid, it was inferred that these elements originated from reservoir rocks. The freshening process caused these elements to be released from the rock, whereby they entered the flowback fluid and returned to the surface.
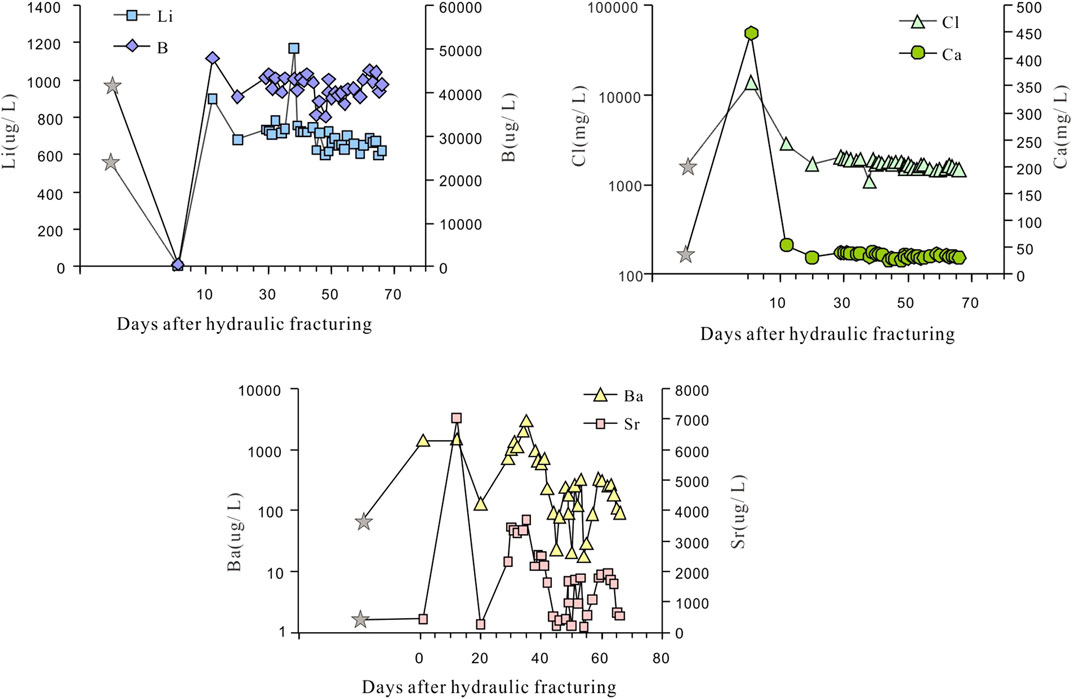
FIGURE 10. Evolution trend of flowback geochemistry in conventional Karamay Formation (the stars in the diagrams indicate the corresponding characteristics of the fracturing fluid).
In summary, in conventional flowback fluid, type 1 elements (Li, B, F, and Br) were derived from the fracturing fluid, type 2 elements (Ca, Cl, Mg, K, Na, HCO3, and SO4) originated from the formation water, and type 3 elements (Mn, Ba, and Sr) were leached from source rocks. As a result, the contents of Ca, Cl, Mg, K, Na, HCO3, and SO4 in the flowback water could represent the characteristics of the Formation water in Karamay Sandstone.
Comparison of Lucaogou and Karamay Formation Flowback Water
The sodium chloride coefficient (γNa/γCl) indicates the degree of concentration metamorphism of formation water. The smaller the value of γNa/γCl, the more concentrated the better sealing of the formation water is. From Table 1, the sodium chloride coefficient of T2k water (γNa/γCl = 1.3–1.7) is smaller than the P2l water (γNa/γCl = 2.3–2.7), indicating that the T2k water was preserved in a more closed system than the P2l water. The water samples from the T2k reservoir have a chloride content of 1,092–2,855 mg/L (mean 1707 mg/L), higher than those from the P2l reservoirs (Cl = 636–663 mg/L, mean 647 mg/L), also indicating that the T2k water preserved in a relatively closed system and experienced less mixing from meteoric water. In contrast, the P2l water was preserved in a relatively open system.
The water samples from the T2k reservoir are a typical NaCl type. The water samples from the P2l reservoir are dominated by a NaHCO3 type. The CaCl2 type water reflects deep cycling and closed water environment, while the NaHCO3 type water represents a weak runoff water environment, with a certain hydraulic connection with other formations or surface water (Xu et al., 2000; Li et al., 2005; Tang et al., 2018). It has been proven (Wang and Zhang, 1998) that the NaHCO3 type water with high salinity is related to the latent deep fault in the deep layer. The deep fault can contribute high concentrations of CO2 to the deep layer, which then enter the formation water, causing the HCO3− + CO23- concentration in the formation water to be greater than the Cl− + SO42−concentration. While low salinity NaHCO3 type water is caused by leaching and infiltration of surface water, the NaHCO3 type water of the P2l reservoir indicates the existence of a deep fault connecting the Lucaogou formation and the surface. NaCl type water is produced by mixing CaCl2 type water with high salinity NaHCO3 type water (Chen et al., 2000). The NaCl type water in the T2k reservoir indicates the existence of fault between the T2k reservoir and P2l, which can be a good channel for the migration of oil, gas, and water. The deep P2l NaHCO3 type water rises along the fault and mixes with the upper CaCl2 type water to form medium and high salinity NaCl type water in the T2k formation.
Filling Mode
In the study area, the Triassic strata lack a sufficient source rock. The potential source rock in the Karamay Formation of the Triassic shows low TOC. The vitrinite reflectance (Ro) of the 6 samples that are collected from the T2k reservoir of XJC 1 range from 1.01% to 1.21%, with an average of 1.09%. The Tmax values range from 453 to 458°C, indicating that the T2k source rock has just entered the mature stage and cannot yet generate gas. Thus, the Lucaogou shale is more likely to be the source of the T2k gas.
From the structural characteristics of the study area (Figure 2A) and the water composition, we can conclude that there is a fault between the Permian Lucaogou shale source rock and the Triassic Karamay reservoir. As a result, the gas filling mode is proposed as follows, below.
The continuously active faults of the Fukang fault zone and the multi-stage unconformity surface and the internal permeability sand bodies of the reservoir, provide channels for the lateral migration of oil and gas. The fault and the unconformity constitute the oil and gas transport system in the Bogda Piedmont. Thus the Permian Lucaogou Formation continental source rock kitchen and Triassic reservoir were connected (Figure 11). The oil and gas were generated from the lacustrine source rocks of the Permian Lucaogou Formation reserved in nearby lithological oil and gas reservoirs in the Permian. The petroleum was transported along the faults to the shallow layer over long distances to enter the Triassic reservoir. Within the Triassic formation, the petroleum migrated laterally through the weathering crust of the unconformity and the internal permeability sandstone, and it migrated horizontally upward along the vertical fault to the target area to form oil and gas reservoirs. The deep Permian Lucaogou Formation primarily developed lithologic oil and gas reservoirs with integrated source and reservoir and large area distribution, while the shallow Triassic Karamay Formatio developed structural-type gas reservoirs with fault transport, structural control, and late accumulation.
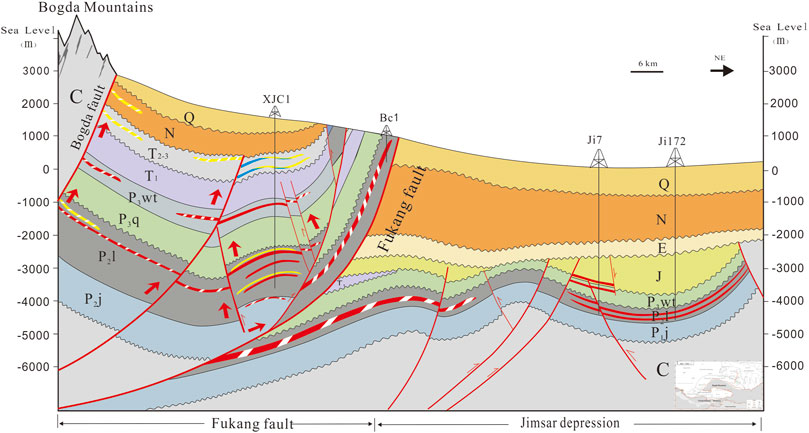
FIGURE 11. Petroleum accumulation and filling model through well XJC 1 of Fukang fault zone (the cross-well profile line is indicated as the red line in Figure 1).
Environmental Issues Associated With Shale Gas Development in the Junggar Basin
The overall aim of characterizing the geochemical flowback water was to contribute to the environmental protection of the water resources associated with the formations undergoing shale oil and gas exploration and development.
Geochemical Fingerprint of Conventional Vs. Unconventional Oil and Gas Wastewater
One of the issues associated with the environmental effects of shale gas development is the impact of unconventional shale gas exploration and contamination from the operation of conventional oil and gas. Thus, it is important to find the distinct characteristics of the waste water from the unconventional shale reservoir.
The shale strata of the Lucaogou Formation measured a higher Li concentration than that of the conventional Karamay Formation (Figure 12). Previous studies on Marcellus shale and Longmaxi shale in the Sichuan Basin have indicated that Li and B in the flowback fluid are predominately derived from clay minerals in shale (Warner et al., 2014; Ni et al., 2018). Because Li and B cannot be sourced from sandstone, the main rock-type in both formations, the concentrations of these elements in the flowback fluid was low. Moreover, a comparison of the conventional and unconventional Li content in the Junggar Basin was consistent with previous research results, indicating that Li in the shale flowback fluid is predominately sourced from the shale layer. However, the B concentration differed from those obtained in previous studies and was attributed to the marine character of the Sichuan and Marcellus shale compared to the continentally derived Junggar shale. The B element is present in high concentrations in marine facies and low concentrations in continental shale, further indicated by the Qaidam Basin in northwest China (Zheng et al., 2017). Therefore, the B content in the flowback fluid of the conventional Karamay Formation was derived from the fracturing fluid, and the significantly high B concentration in the fracturing fluid resulted in a high B concentration in the flowback fluid. Furthermore, the Sr content in the conventional flowback was relatively higher than in the unconventional reservoir. The different properties of the flowback water derived from these two formations result in the unique contrasting characteristics observed in the conventional and unconventional flowback in the Junggar Basin. Similar to B, the Cl content in the conventional formations was significantly higher than in the unconventional flowback fluid, and was attributed to the high Cl content in the conventional fracturing fluid. The contents of Mn, Ba, and Sr in the unconventional flowback fluid were higher than those in the conventional flowback fluid. These elements are mainly derived from rock formations, indicating that the concentrations of these elements in the continental shale layer are higher, or that these elements are more mobile and readily enter the flowback fluid. The above discussion implies that the Li, B, Sr contents could be used as potential indicators for identifying possible migration of wastewater from Lucaogou shale.
Pollutant Concentration in the Flowback Fluid
One of the key environmental issues related to shale gas development is the quality of flowback water coproduced with the shale gas. The ability to reuse and manage the waste water is dependent on the water quantity and quality. Several water quality components in the flowback fluid sampled from the Junggar Basin exceed the three major water quality standards in China: the drinking water quality standard (WQS) (GB 5749–2006), Environmental Quality Standards for Surface Water (EQS) (GB3838–2002), and China’s Integrated Wastewater Discharge Standard (IWDS) (GB 8978–1996). Components exceeding these standards include Fe, Ba, Mn, B, Cl, and F (Table 2).
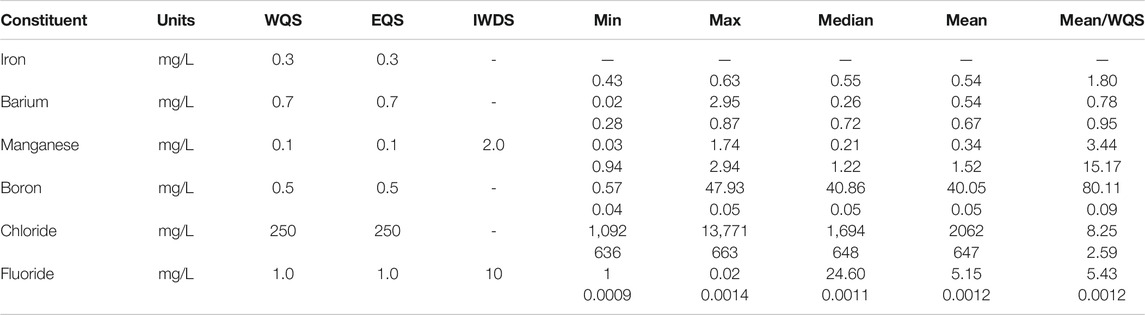
TABLE 2. Concentrations of inorganic chemicals in flowback water of the Junggar Basin. Highlighted rows indicate Mean/WQS ratio >1. WQS is the Water Quality Standards for drinking water from China’s sanitary (GB 5749–2006), EQS is the Environmental Quality Standards for Surface Water (GB3838–2002), and IWDS is the Integrated Wastewater discharge standard of China (GB 8978–1996). The cells with dark background represent conventional T2k flowback, and the corresponding cells represent unconventional P2l flowback water.
The ratios of the average value of B, Cl, and F in the conventional flowback fluid to the drinking water quality standards (mean/WQS) reached 80.11, 8.25, and 5.43, respectively, and were considerably higher than those of the unconventional flowback fluid. This was mainly due to the high concentrations of these elements in the unconventional fracturing fluid. Hence, water remediation strategies should focus on the treatment of these elements in the wastewater of conventional wells in the Karamay Formation. In the unconventional Lucaogou Formation, the ratio of Fe and Mn in the flowback fluid to the drinking water quality standards (mean/WQS) peaked at 1.80 and 15.17. These ratios were significantly higher than those recorded in the conventional flowback fluid. The Ba content in the two flowback fluids, however, only slightly exceeded the WQS, and thus its effect on the wastewater was considered negligible.
Compared with the drinking water standard (WQS), the concentrations of B in the conventional flowback fluid and the Mn concentration in the unconventional flowback fluid were significantly high (Mean/WQS values are the highest, Table 1), and effective removal strategies are required in the wastewater treatment process.
Conclusion
This study aimed to determine the gas origin and filling mode of P2l and T2k gases in the Junggar Basin and to propose suggestions for water treatment. Based on our results, the following conclusions were made:
1) The average contents of CH4, C2H6, C3H8 of the P2l lacustrine shale gas are 74.14%, 8.49%, 2.13%, and 1.96%, respectively. Compared with the gas in the Lower Triassic Chang 7 (T1y7) continental shale of the Ordos Basin and gas in the Upper Triassic Xu 5 (T3x5) continental shale of the Sichuan Basin, the Lucaogou shale gas contains the highest content of heavy hydrocarbon despite its higher maturity. The average values of δ13C1, δ13C2, δ13C3 of P2l lacustrine shale gas are −41.5‰, −31.1‰, and −28.6‰, respectively, between the T1y7 lacustrine shale gas in the Yanchang gas field and the T3x5 shale gas in the Xinchang gas field. The alkane composition and isotope of P2l gas indicate that the gas was generated from continental shale containing more saprolite components that are prone to generate heavy hydrocarbons, which is in accordance with the Lucaogou shale.
2) From the evolution trend of flowback chemistry in the T2k formation, it can be concluded that the Ca, Cl, Mg, K, Na, HCO3, and SO4 in the flowback water are mainly from the formation water and that the content of these chemicals could represent the characteristics of the Formation water. The comparison between compositions in the flowback water reveals that the P2l water is of NaHCO3 type while the T2k water is of NaCl type, and the salinity of the latter is higher than the former, indicating a connection between P2l source rock and the T2k reservoir. In combination with the structural setting in the study are, the gas filling mode was proposed as follows: the gas generated from the lacustrine source rocks of the Permian Lucaogou Formation reserved in nearby lithological reservoirs in the Permian. The petroleum was transported along the faults to the shallow layer over long distances to enter the Triassic reservoir.
3) The high contents of B, Cl, and F in the flowback fluid of the Karamay Formation were attributed to the high concentration of these elements in the fracturing fluid. Therefore, water remediation strategies should focus on the removal of these elements in the flowback fluid. Moreover, elevated concentrations of Mn and Fe recorded in the Lucaogou Formation should be the focus of research on the sewage treatment process of shale flowback fluid.
The findings of this research could indicate potential targets for shale gas exploration. Moreover, the suggestions for wastewater treatment based on water quality analysis can help mitigate, to some extent, the environmental issues faced during shale gas development.
Data Availability Statement
The original contributions presented in the study are included in the article/Supplementary Material, further inquiries can be directed to the corresponding author.
Author Contributions
LZ: data curation, formal analysis. DL: writing, review, and editing the manuscript. YG: writing, review and editing the manuscript. MZ: writing, review and editing the manuscript.
Funding
This study was supported by the National Natural Science Foundation for Young Scientists of China (Grant No. 41702161), the International Partnership Program of the Chinese Academy of Sciences (Grant No. 132A11KYSB20180012), the China Geological Survey Program (Grant/Award Numbers DD20160,170, DD20160,203, and DD20190,090), and the China Geological Survey Bureau special project “basic geological survey of oil and gas in Junggar Basin and its surrounding areas” (Grant No. 121201021000150007).
Conflict of Interest
DL was employed by the company PetroChina.
The remaining authors declare that the research was conducted in the absence of any commercial or financial relationships that could be construed as a potential conflict of interest.
Acknowledgments
The authors would like to thank the College of Resources and Environment at Yangtze University, the PetroChina Xinjiang Oilfield Company Research Institute of Petroleum Exploration and Development for experimental analysis, and the Oil and Gas Survey of CGS for providing project funding support.
References
Burruss, R. C., and Laughrey, C. D. (2010). Carbon and hydrogen isotopic reversals in deep basin gas: evidence for limits to the stability of hydrocarbons. Org. Geochem. 41, 1285–1296. doi:10.1016/j.orggeochem.2010.09.008
Chapman, E. C., Capo, R. C., Stewart, B. W., Kirby, C. S., Hammack, R. W., Schroeder, K. T., et al. (2012). Geochemical and strontium isotope characterization of produced waters from Marcellus shale natural gas extraction. Environ. Sci. Technol. 46 (6), 3545–3553. doi:10.1021/es204005g
Chen, J. P., Cha, M., and Zhou, Y. Q. (2000). Chemical characteristics of formation water in relation with oil/gas in the northwestern parts of Junggar Basin. Geology-Geochemistry 28 (3), 54–58. doi:10.3969/j.issn.1672-9250.2000.03.006
Dai, J., Gong, D., Ni, Y., Huang, S., and Wu, W. (2014). Stable carbon isotopes of coal-derived gases sourced from the Mesozoic coal measures in China. Org. Geochem. 74, 123–142. doi:10.1016/j.orggeochem.2014.04.002
Dai, J., and Qi, H. (1989). The relationship between δ13C and Ro in coal- genetic gas. Chin. Sci. Bull. 34 (9), 690–692. doi:10.1360/csb1989-34-9-690
Dai, J. X., Zou, C. N., Dong, D. Z., Ni, Y. Y., Wu, W., Gong, D. Y., et al. (2016). Geochemical characteristics of marine and terrestrial shale gas in China. Mar. Pet. Geol. 76, 44–463. doi:10.1016/j.marpetgeo.2016.04.027
Feng, Z., Liu, D., Huang, S., Wu, W., Dong, D., Peng, W., et al. (2016). Carbon isotopic composition of shale gas in the silurian Longmaxi Formation of the changning area, Sichuan Basin. Pet. Exploration Dev. 43, 769–777. doi:10.1016/s1876-3804(16)30092-1
Hao, F., and Zou, H. (2013). Cause of shale gas geochemical anomalies and mechanisms for gas enrichment and depletion in high-maturity shales. Mar. Pet. Geology. 44, 1–12. doi:10.1016/j.marpetgeo.2013.03.005
Hill, R. J., Jarvie, D. M., Zumberge, J., Henry, M., and Pollastro, R. M. (2007). Oil and gas geochemistry and petroleum systems of the Fort Worth Basin. Bulletin 91, 445–473. doi:10.1306/11030606014
Hunt, A. G., Darrah, T. H., and Poreda, R. J. (2012). Determining the source and genetic fingerprint of natural gases using noble gas geochemistry: a northern Appalachian Basin case study. Bulletin 96 (10), 1785–1811. doi:10.1306/03161211093
Jenden, P. D., Draza, D. J., and Kaplan, I. R. (1993). Mixing of thermogenic natural gas in northern Appalachian basin. AAPG Bull. 77 (6), 980–998. doi:10.1306/BDFF8DBC-1718-11D7-8645000102C1865D
Lauer, N. E., Harkness, J. S., and Vengosh, A. (2016). Brine spills associated with unconventional oil development in north Dakota. Environ. Sci. Technol. 50 (10), 5389–5397.doi:10.1021/acs.est.5b06349
Li, X. Q., Hou, D. J., Hu, G. Y., and Li, J. (2005). the Formation fluid characteristics and gas accumulation of central gas fields in the Ordos Basin. Beijing: Geological Publishing House. (in Chinese).
Liu, Dan., Jian, Li., Zou, Caineng., et al. (2020). Recycling flowback water for hydraulic fracturing in China: implications for gas production and water footprint of flowback water. Fuel 272 (15). doi:10.1016/j.fuel.2020.117621
Martini, A. M., Walter, L. M., and McIntosh, J. C. (2008). Identification of microbial and thermogenic gas components from Upper Devonian black shale cores, Illinois and Michigan basins. Bulletin 92 (3), 327–339. doi:10.1306/10180706037
Ni, Y., Zou, C., Cui, H., Li, J., Lauer, N., Harkness, J., et al. (2018). Origin of flowback and produced waters from Sichuan Basin, China. Environ. Sci. Technol. 52, 14519–14527. doi:10.1021/acs.est.8b04345
Stahl, W. J., and Carey, B. D. (1975). Source-rock identification by isotope analyses of natural gases from fields in the Val Verde and Delaware basins, west Texas. Chem. Geology. 16, 257–267. doi:10.1016/0009-2541(75)90065-0
Strąpoć, D., Mastalerz, M., Schimmelmann, A., Drobniak, A., and Hasenmueller, N. R. (2010). Geochemical constraints on the origin and volume of gas in the new albany shale (Devonian–Mississippian), eastern Illinois basin. AAPG Bull. 94 (11), 1713–1740. doi:10.1306/06301009197
Tang, S., Tang, D., Li, S., Geng, Y., Xu, H., Tao, S., et al. (2018). Geochemical characteristics and origin of natural gas and gas -filling mode of the Paleozoic in the Yanchuannan gas field, Ordos Basin, China. J. Nat. Gas Sci. Eng. 49, 286–297. doi:10.1016/j.jngse.2017.11.013
Tilley, B., and Muehlenbachs, K. (2013). Isotope reversals and universal stages and trends of gas maturation in sealed, self-contained petroleum systems. Chem. Geology. 339, 194–204. doi:10.1016/j.chemgeo.2012.08.002
Vengosh, A., Jackson, R. B., Warner, N., Darrah, T. H., and Kondash, A. (2014). A critical review of the risks to water resources from unconventional shale gas development and hydraulic fracturing in the United States. Environ. Sci. Technol. 48 (15), 8334–8348.doi:10.1021/es405118y
Vengosh, A., Mitch, W. A., and McKenzie, L. M. (2017). Environmental and human impacts of unconventional energy development. Environ. Sci. Technol. 51 (18), 10271–10273. doi:10.1021/acs.est.7b04336
Wang, Z. H., and Zhang, S. J. (1998). The Discovery and characteristics of high salinity sodium bicarbonate type water in Karamay Oilfield, China. Pet. Geology. Exp. 20 (1), 39–43. doi:10.11781/sysydz199801039
Warner, N. R., Christie, C. A., Jackson, R. B., and Vengosh, A. (2013). Impacts of shale gas wastewater disposal on water quality in western Pennsylvania. Environ. Sci. Technol. 47 (20), 11849–11857.doi:10.1021/es402165b
Warner, N. R., Darrah, T. H., Jackson, R. B., Millot, R., Kloppmann, W., and Vengosh, A. (2014). New tracers identify hydraulic fracturing fluids and accidental releases from oil and gas operations. Environ. Sci. Technol. 48 (21), 12552–12560. doi:10.1021/es5032135
Xu, G. S., Song, H. R., and Zhou, W. (2000). Hydrochemical conditions and natural gas accumulation in the middle part gasfield of the Ordos Basin. Exp. Pet. Geol. 22, 330–335. doi:10.11781/sysydz200004319
Zheng, Y., and Chen, J. (2000). Stable isotope geochemistry. Beijing: Science Press, 196–200. (In Chinese).
Zheng, Z., Zhang, H., Chen, Z., Li, X., Zhu, P., and Cui, X. (2017). Hydrogeochemical and isotopic indicators of hydraulic fracturing flowback fluids in shallow groundwater and stream water, derived from dameigou shale gas extraction in the northern Qaidam Basin. Environ. Sci. Technol. 51 (11), 5889–5898. doi:10.1021/acs.est.6b04269
Zou, C., Ni, Y., Li, J., Kondash, A., Coyte, R., Lauer, N., et al. (2018). The water footprint of hydraulic fracturing in Sichuan Basin, China. Sci. Total Environ. 630, 349–356. doi:10.1016/j.scitotenv.2018.02.219
Keywords: shale gas, potential, maturity, parent material, exploration and development
Citation: Zhang L, Liu D, Gao Y and Zhang M (2021) Geochemical Characteristics of Gas and Flowback Water in Lake Facies Shale: A Case Study From the Junggar Basin, China. Front. Earth Sci. 9:635893. doi: 10.3389/feart.2021.635893
Received: 30 November 2020; Accepted: 15 February 2021;
Published: 26 March 2021.
Edited by:
Guodong Zheng, Chinese Academy of Sciences (CAS), ChinaReviewed by:
Wenhui Liu, Northwest University, ChinaQuanyou Liu, SINOPEC Petroleum Exploration and Production Research Institute, China
Copyright © 2021 Zhang, Liu, Gao and Zhang. This is an open-access article distributed under the terms of the Creative Commons Attribution License (CC BY). The use, distribution or reproduction in other forums is permitted, provided the original author(s) and the copyright owner(s) are credited and that the original publication in this journal is cited, in accordance with accepted academic practice. No use, distribution or reproduction is permitted which does not comply with these terms.
*Correspondence: Dan Liu, liudan86@petrochina.com.cn