- 1Department of Earth Sciences, Natural History Museum, London, United Kingdom
- 2Evolutionary Studies Institute, University of the Witwatersrand, Johannesburg, South Africa
The Beaufort Group of the main Karoo Basin of South Africa records two major extinction events of terrestrial vertebrates in the late Palaeozoic. The oldest of these has been dated to the late Capitanian and is characterized by the extinction of dinocephalian therapsids and bradysaurian pareiasaurs near the top of Tapinocephalus Assemblage Zone. Faunal turnover associated with the extinction of dinocephalians is evident in vertebrate faunas from elsewhere in Pangaea but it can be best studied in the Karoo Basin, where exposures of the upper Abrahamskraal and lower Teekloof formations allow continuous sampling across the whole extinction interval. Here we present field data for several sections spanning the Capitanian extinction interval in the southwestern Karoo and discuss recent work to establish its timing, severity, and causes. A large collections database informed by fieldwork demonstrates an increase in extinction rates associated with ecological instability that approach that of the end-Permian mass extinction, and shows significant turnover followed by a period of low diversity. Extinctions and recovery appear phased and show similarities to diversity patterns reported for the end-Permian mass extinction higher in the Beaufort sequence. In the Karoo, the late Capitanian mass extinction coincides with volcanism in the Emeishan Large Igneous Province and may have been partly driven by short-term aridification, but clear causal mechanisms and robust links to global environmental phenomena remain elusive.
Introduction
The Capitanian (late Guadalupian) mass extinction is a relatively recent addition to the list of major biodiversity crises of the Phanerozoic. During the establishment of the Big 5 marine mass extinction events, Raup and Sepkoski (1982) grouped extinctions from the Guadalupian with those of the late Permian and it was only later that Stanley and Yang (1994) recognised a separate, end-Guadalupian crisis. Subsequent, higher-resolution stratigraphic studies and continually improving chronological constraints have demonstrated that the marine extinctions associated with this event may have occurred at different points clustered in the later part of the Capitanian stage, rather than at the boundary between the Guadalupian and Lopingian series (Shen and Shi, 2009; Wignall et al., 2009; Bond et al., 2010, Bond et al, 2015). Most data come from marine deposits of southern China, where large losses are recorded among fusilinacean foraminiferans, calcareous algae, brachiopods, corals, and ammonoids (see Bond et al., 2010) but the extinction has also been recorded outside of the former Tethys in Norway and Canada (Bond et al., 2015; Bond et al., 2020).
The Capitanian mass extinction has received increasing attention in the last decade because of likely causal links to volcanism in the Emeishan Large Igneous Province (Wignall et al., 2009; McGhee et al., 2013; Bond and Wignall, 2014; Bond and Grasby, 2017; Bond et al., 2020). Estimates of its overall severity have varied, depending on the calculations and datasets used, but one of the most recent large-database estimates suggests it may have led to the extinction of 33–35% of marine genera, making it comparable in magnitude to the Cretaceous/Palaeogene mass extinction (Stanley, 2016). Even when its severity in terms of taxonomic losses has been calculated to be low (∼25%), the Capitanian mass extinction appears to have been characterised by ecological restructuring of reef systems that exceeded those of the end-Ordovician (Hirnantian) mass extinction (McGhee et al., 2013). Though a consensus has yet to be reached, there is sufficient evidence to suggest the Capitanian mass extinction may rank among the most notable extinction events of the Phanerozoic.
In the terrestrial realm, the Capitanian mass extinction manifests as a concentration of extinctions that drastically reduced tetrapod diversity by removing early branching species from most clades and entirely eliminating others, most notably the taxonomically diverse dinocephalian therapsids (Day et al., 2015a; Lucas, 2017). Non-marine sedimentary sequences of late Capitanian/early Lopingian age that have produced tetrapod fossils are known from basins across Pangaea (e.g., Olroyd and Sidor, 2017; Lucas, 2017) but only in South Africa is this transition clearly exposed in continuous sections. There, it corresponds to the transition between the Tapinocephalus Assemblage Zone (AZ) and the Tropidostoma-Gorgonops subzone of the Endothiodon AZ (incorporating the former Pristerognathus AZ; see Day and Smith, 2020), and is associated with an estimated 74–80% loss of tetrapod generic richness (Day et al., 2015a). The extinction interval is situated around the lithostratigraphic boundary between the Abrahamskraal and Teekloof formations, within the lower Beaufort Group (Figure 1). The Beaufort Group also records the terrestrial end-Permian mass extinction, in the uppermost Balfour Formation (e.g. Ward et al., 2005; Smith and Botha-Brink, 2014; Botha et al., 2020), which provides an opportunity to compare patterns of extinction between the two events.
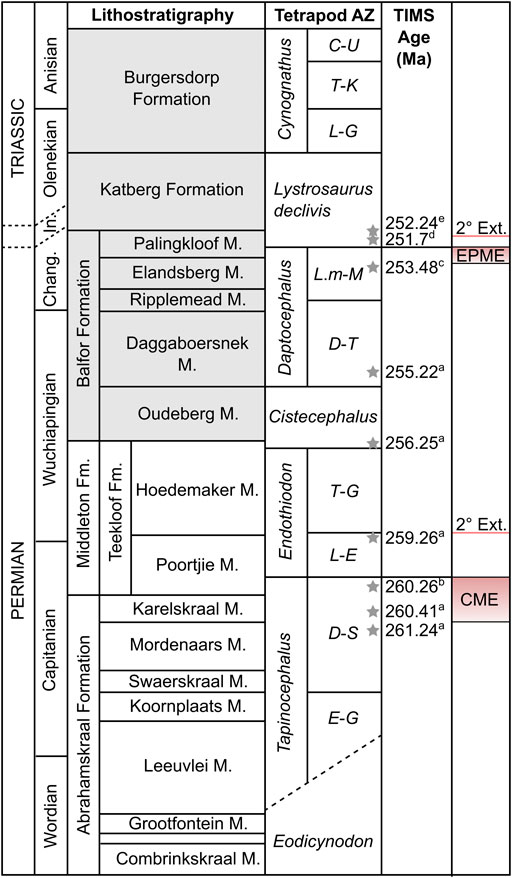
FIGURE 1. Stratigraphy of the Beaufort Group showing position of mass extinction intervals. Subzone abbreviations: C-U, Cricodon-Ufudocyclops; D-S, Diictodon-Styracocephalus; D-T, Dicynodon-Theriognathus; E-G, Eosimops-Glanosuchus; L-E, Lycosuchus-Eunotosaurus; L-G, Langbergia-Garjainia; L.m-M, Lystrosaurus maccaigi-Moschorhinus; T-G, Tropidostoma-Gorgonops; T-K, Trirachodon-Kannemeyeria. Other abbreviations: CME, Capitanian mass extinction; Changh, Changhsingian; Fm., Formation; In, Induan; M, member; EPME, end-Permian mass extinction. Lithostratigraphic units in grey found only in the Eastern Cape. Stratigraphy and biozonation modified after Smith et al. (2020) and position of end-Permian mass extinction after Botha et al. (2020). U-Pb ages after: a, Rubidge et al., 2013; b, Day et al., 2015a; c, Gastaldo et al., 2015; d, Botha et al., 2020; e, Gastaldo et al., 2020. Actual position of the Permo-Triassic boundary is disputed.
Retallack et al. (2006) suggested a link between a period of high extinction and turnover in terrestrial tetrapod faunas and the marine Guadalupian mass extinction, but it was only with improvements in biostratigraphic resolution and geochronological constraints on the mid-late Permian terrestrial sequence of the Main Karoo Basin in South Africa that such comparisons could be directly supported (Rubidge et al., 2013; Day et al., 2015a). Subsequent quantitative estimates of diversity change associated with the Capitanian mass extinction in the Karoo have relied mostly on species lists for assemblages (e.g., Lucas, 2009), but the recent generation of a database comprising occurrence information for museum collections (specimens) constrained to one or more 50 m stratigraphic bins in the Abrahamskraal and lower Teekloof formations has allowed much higher resolution assessments of diversity change and rates of extinction (Day et al., 2015a; Day et al., 2018a). The creation of this database was informed by fieldwork that resulted in metre-level biostratigraphic data for several sections in the southwestern Karoo, which document the transition between the Tapinocephalus and Endothiodon AZs. These sections provide more detailed glimpses of the sequence of events comprising the extinction event, and we present the three most informative sections here (Figures 2, 3).
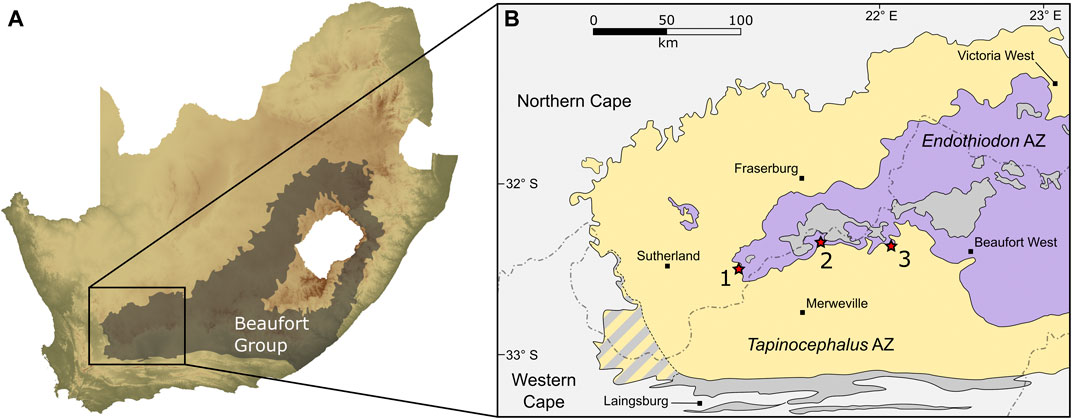
FIGURE 2. Map showing the study area within the Beaufort Group in the southwestern part of the Karoo Basin of South Africa (A), and the three localities along the contact of the Tapinocephalus and Endothiodon assemblage zones (B). 1, Puntkraal; 2, Muggefontein; 3, Vanvuurenskop.
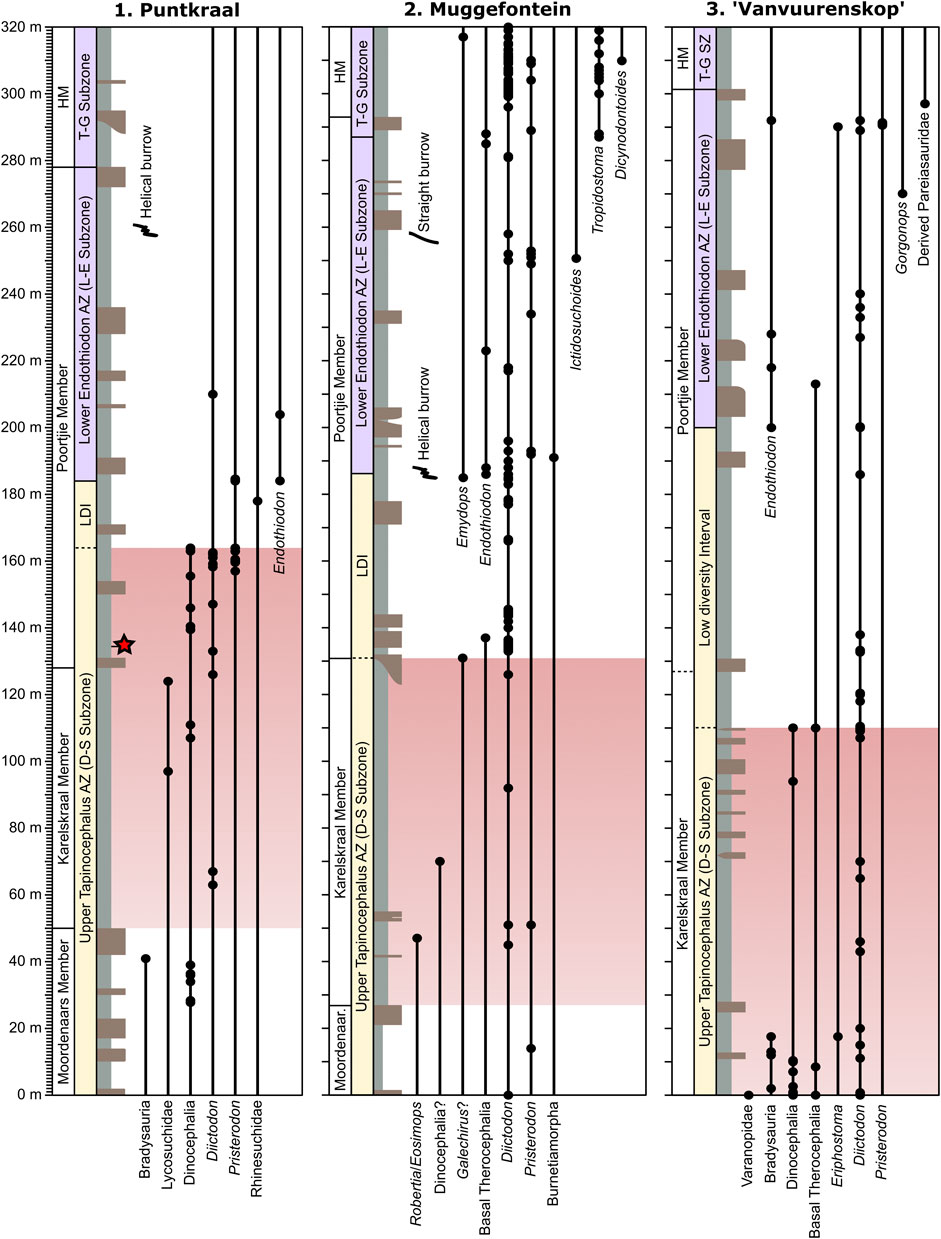
FIGURE 3. Stratigraphic sections showing fossil occurrences at the three localities: Puntkraal, Muggefontein, and Vanvuurenskop (see Figure 2 for map). Red star = date of 260.26 ± 0.081 Ma (Day et al., 2015a). LDI, Low diversity interval; L-E, Lycosuchus-Eunotosaurus; D-S, Diictodon-Styracocephalus; H, Hoedemaker Member; T-G, Tropidostoma-Gorgonops.
Sedimentological research has sought evidence for environmental change associated with the transition between the Abrahamskraal and Teekloof formations but beyond general trends toward greater aridity these have mostly invoked tectonic or autogenic controls on any change in depositional environment (e.g., Turner, 1985; Cole and Wipplinger, 2001; Wilson et al., 2014; though see; Retallack et al., 2006). Most recently, Rey et al. (2018) studied the carbon isotope geochemistry on fossilised vertebrate tissue and found evidence of a short-lived period of greater aridity in the upper Karelskraal Member and lowermost Poortjie Member but neither this nor rock geochemistry have indicated meaningful long-term shifts in climate across the extinction interval (see Paiva, 2016). Palaeoenvironmental studies have not yet taken full advantage of the continuity of the Karoo record.
This paper aims to summarise the current state of research on the biotic and non-biotic aspects of the Capitanian mass extinction in the Karoo Basin and to discuss these patterns with respect to the end-Permian mass extinction. We also discuss patterns from correlated tetrapod-bearing successions elsewhere in the world.
Materials and Methods
Fieldwork
Palaeontological and stratigraphic data from the Abrahamskraal and overlying Teekloof formations has been collected by our team from numerous localities over the past four decades. The stratigraphic sections presented here were measured by MOD during three field seasons between 2012 and 2014 using a Jacob’s staff and Abney level at localities that expose outcrops spanning the upper Abrahamskraal and lower Teekloof formations. Two of the three section localities (Muggefontein and Vanvuurenskop) are located along the Nuweveld escarpment in the Beaufort West district of the Western Cape Province, whereas the third is located further west, near Sutherland in the Karoo Hoogland district of the Northern Cape Province (Figure 2). The lithostratigraphic boundary between the Abrahamskraal and Teekloof formations (and Karelskraal and Poortjie members, respectively) was taken initially from the 1:250,000 metallogenic map of Sutherland (Geological Survey, 1997) and the geological map of Beaufort West (Geological Survey, 1979) but where they were not clear, contacts between members were determined in the field. Simultaneously, a team from the Evolutionary Studies Institute, University of the Witwatersrand, collected vertebrate fossils in the vicinity and recorded the coordinates of the finds using GPS handsets. Sandstone bodies were traced out laterally from the line of section, either in the field or subsequently on Google Earth, to enable correlation of fossil occurrences. All material collected is curated at the Evolutionary Studies Institute in Johannesburg.
Taxon Abundance
Taxon abundance information through the extinction interval was calculated from a dataset of fossil occurrences within the Abrahamskraal and lower Teekloof formations outlined by Day et al. (2018a), in which the occurrence of fossil specimens from a number of museum collections are constrained to one or more approximately 50 m stratigraphic bins (Supplementary Table S1). Across the extinction interval this was practically implemented by considering the upper 50 m of the Moordenaars Member as one bin, two bins within the Karelskraal Member (which varies in thickness between 70 m and 116 m; Day and Rubidge, 2014), three bins for the Poortjie Member (which varies in thickness between 150 m and about 180 m in the study area; Figure 2), and one for the lower third of the Hoedemaker Member.
Treatment of the data follows a similar methodology as Day et al. (2018a), whereby specimens that are not well constrained stratigraphically and those with uncertain identification have been excluded. The dataset used here differs from that of Day et al. (2018a) mainly in that data from the Victoria West area have been excluded due to concerns over the relationship between biostratigraphy and mapped lithostratigraphic units in that district (Day and Rubidge, 2019); fossil occurrences in this area are primarily of Diictodon and also fall outside the immediate area of the sections presented here. We are thus presenting a subset of the basin dataset that can be most reliably tied to the lithostratigraphic sequence along the escarpment, where continuous exposures are most readily found (Supplementary Table S1). Several specimen identifications and stratigraphic constraints have been updated in light of recent work.
Stratigraphic Sections
Puntkraal, Sutherland, Northern Cape
Puntkraal is situated at the western end of the Nuweveld mountains and has good outcrops of the upper Abrahamskraal and lower Teekloof formations. Exposures occur widely on the slopes between terraces, particularly the lower Poortjie Member. Large dinocephalian fossils were abundant in several horizons within the upper Moordenaars Member and within the lower Poortjie Member, where a titanosuchid, a tentative anteosaurid, and two tapinocephalid fossils were recovered (Figure 3; see Supplementary Table S2). The Poortjie dinocephalians all occur within the 30 m interval above a tuff horizon that has produced a CA-TIMS age of 260.26 Ma, which constrains them to the late Capitanian (Day et al., 2015a). The upper Poortjie Member produced few identifiable fossils but two specimens of Endothiodon indicate the presence of the Lycosuchus-Eunotosaurus Subzone of the Endothiodon AZ in the middle part of the member. Helical vertebrate burrows similar to those attributed to small dicynodonts from sites in the upper Poortjie and lower Hoedemaker members (Smith, 1987) are present in the upper part of the Poortjie Member at this locality.
The stratigraphic thickness between the last occurrence of dinocephalians and Endothiodon, which constrains the low diversity interval at the top of the Tapinocephalus AZ in the immediate aftermath of the Capitanian mass extinction (Day and Rubidge, 2020), is less at Puntkraal than any other locality (20 m; Figure 3). At this locality there is a noticeable abundance in the lower Poortjie Member of the small dicynodonts Diictodon and Pristerodon. Sampling of the upper Poortjie Member was low and those specimens that were recovered from above 210 m were not identifiable to a biostratigraphically meaningful level.
Muggefontein, Beaufort West, Western Cape
Muggefontein is situated south of Teekloof Pass and provides excellent and wide exposures of the Teekloof Formation, particularly the Poortjie Member. Outcrop of the Abrahamskraal Formation is more limited to the southern part of the farm, where its lateral extent is constrained by a large dolerite sill. Few fossils were found in the Abrahamskraal Formation and although large bones were evident in places, these were all too scrappy to be collected. A small therapsid skeleton identified as a basal anomodont similar to Galechirus (BP/1/7553) was discovered in the basal sandstone of the Poortjie Member, which established the presence of the Tapinocephalus AZ there.
In the Abrahamskraal Formation and lower Poortjie Member the majority of fossils recovered belong to small dicynodonts such as Diictodon and Pristerodon. The appearance of Endothiodon about 1/3 of the way up the Poortjie Member establishes the base of the Lycosuchus-Eunotosaurus Subzone of the Endothiodon AZ, which at this locality produced the snout of the baurioid therocephalian Ictidosuchoides (BP/1/7492; Figure 3). The base of the Endothiodon AZ also coincides with the first occurrence of a helical burrow, and a straight vertebrate burrow was found in the upper Poortjie Member (at about 257 m). The base of the Tropidostoma-Gorgonops Subzone of the Endothiodon AZ is clearly defined by the appearance of Tropidostoma close to the contact of the Poortjie and Hoedemaker members, above which Tropidostoma becomes abundant. The kingoriid dicynodont Dicynodontoides occurs 17 m above the base of Hoedemaker. Diictodon is extremely abundant in the Teekloof Formation.
‘Vanvuurenskop’, Beaufort West, Western Cape
This locality is spread across three farms whose boundaries meet at the summit of a small mountain called Vanvuurenskop, situated on a spur of the Nuweveld Escarpment. To the south, the upper Abrahamskraal Formation and lowermost Teekloof Formation crop out on the farms Wolwehoek and Brakleegte, with the upper Abrahamskraal Formation being well-exposed on the flats and on the lower slopes of the spur. To the north, surrounding Vanvuurenskop itself, are good outcrops of the upper Poortjie Member on the farm Losberg (part of cadastral farm Balaauwkrans 216). The section presented is a composite as the two farms are separated by a dolerite dyke, though the base of the lower Poortjie does occur on the Losberg side of the dyke (though did not produce fossils) so thicknesses were used to correlate the major sandstone bodies.
On Wolwehoek and Brakleegte, the upper Abrahamskraal Formation produced a diverse fauna of Tapinocephalus AZ forms. These occurred into the upper part of the Karelskraal Member, above a thick terrace-forming sandstone, where a small dinocephalian snout was found (BP/1/7255) along with a therocephalian cf. Lycosuchidae. Small dicynodonts increased in abundance at this horizon and were the only fossils found in the lower Poortjie Member. Endothiodon fossils were found in the middle part of the Poortjie Member up to the base of the Hoedemaker, which was not collected. A specimen of the gorgonopsian Eriphostoma was found close to the top of the Poortjie Member (Kammerer et al., 2015).
Discussion
Stratigraphy and Extinction Patterns
The existence of unusually high turnover of fossil tetrapod species between the Tapinocephalus AZ and succeeding Endothiodon AZ had become increasingly apparent by the early 20th Century (e.g., Watson, 1914) but the first quantitative work on the horizon later connected with the Capitanian extinction appeared only after the Geological Survey of South Africa mapped the Merweville area during the 1940s. The subsequent report by Rossouw and De Villiers (1953) revealed that the relative abundance of pareiasaur and dinocephalian fossils decreased in the uppermost part of the Tapinocephalus AZ whereas dicynodonts became more abundant. Boonstra (1969) went further and suggested that very few species were present in the uppermost part of the Tapinocephalus AZ and that pareiasaurs and dinocephalians were not present at all. In their stratigraphic review of the Beaufort Group, Keyser and Smith (1978) detached these uppermost strata and recognized the extinction of the dinocephalians as the upper boundary of their Dinocephalian AZ, which they correlated with a chert horizon about 120 m below the base of the Poortjie Member (Teekloof Formation).
Smith and Keyser (1995) considered the top of the Dinocephalian AZ, then renamed the Tapinocephalus AZ, to occur closer to the base of the Poortjie Member, and it was this horizon that was first linked with Capitanian mass extinction (Retallack et al., 2006). In this scenario, the extinction of the majority of Tapinocephalus AZ taxa was assumed to be mostly contemporaneous in a catastrophic event, although the exact ranges of the constituent taxa were poorly understood. The presence of dinocephalians in the basal strata of the Poortjie Member was first reported by Day et al. (2015b) and, together with the data from the Puntkraal locality presented here, suggests that three dinocephalian genera survived beyond this horizon (Anteosaurus, Criocephalosaurus, and Titanosuchus; Figure 4B). Dinocephalians are not observed at this level at Muggefontein or at Vanvuurenskop but at the latter locality the base of the Poortjie Member was not clearly defined and its lower strata difficult to access. While it remains a possibility that the base of the Poortjie Member is not isochronous, we suspect that the absence of dinocephalian fossils from the lower Poortjie at some localities is likely to be an artifact of poor sampling.
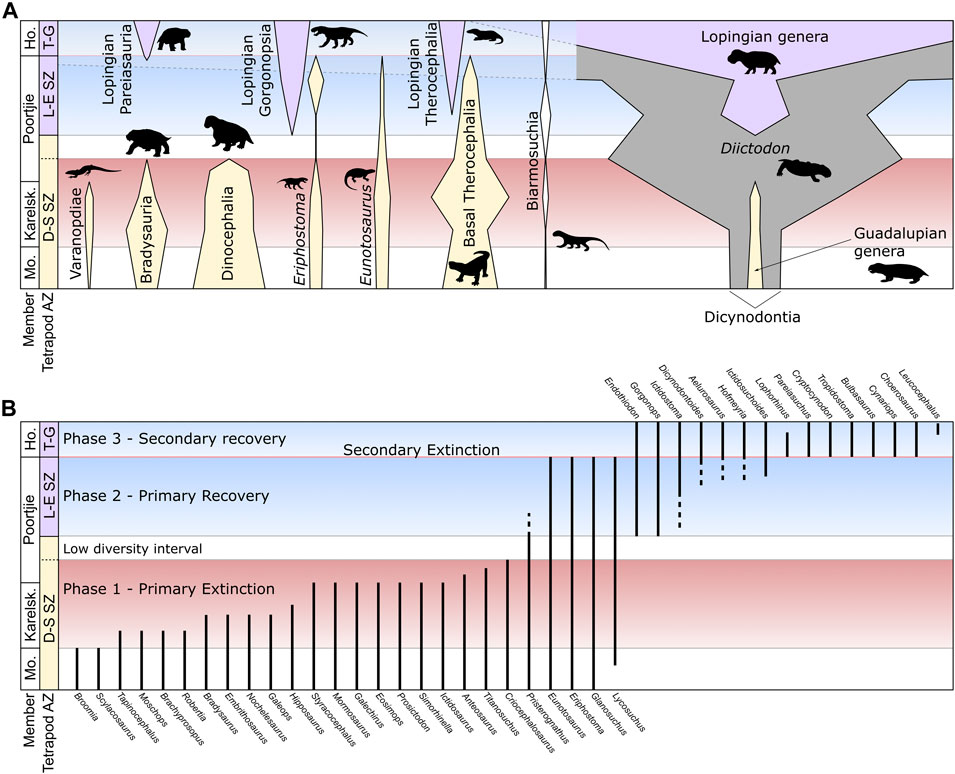
FIGURE 4. Illustrative section through the Abrahamskraal-Teekloof Formation boundary showing (A) the relative abundances of selected taxa, corresponding to numbers of specimens (see Appendix 2), and (B) a summary of the stratigraphic ranges for individual genera. Genera shown in (B) taken from Day and Rubidge (2020) and Day and Smith (2020) but excludes range-through taxa (Diictodon feliceps, Pristerodon mackayi, Emydops arctatus, Rhinesuchus whaitsi, Rhinesuchoides tenuiceps) and taxa with single occurrences of uncertain position (Nycteroleters indet., Australothyris smithi, Hipposaurus brinki, Agnosaurus pienaari, Moschognathus whaitsi, Galepus jouberti). Abbreviations: Ho, Hoedemaker; Karelsk, Karelskraal; Mo, Moordenaars.
The increasing resolution of fossil occurrences across the Abrahamskraal/Teekloof Formation transition and taxonomic clarification of many tetrapod groups have demonstrated that the highest occurrences of taxa associated with the main extinction pulse are staggered between the base of the Karelskraal Member and the lower Poortjie Member (Day et al., 2015a; Day and Rubidge, 2020; Figure 4B). This pattern can be explained by the Signor-Lipps effect (Signor and Lipps, 1982), especially given the relatively small proportion of fossils of most taxa that can be identified to genus or species level (only around 20% for dinocephalians). Quantitative analysis using both constrained optimization (CONOP) and gap-filler (GF) methods on occurrence data at the resolution of approximately 50 m intervals has suggested that the extinction rates peaked in the uppermost Abrahamskraal Formation, within the Karelskraal Member (Day et al., 2015a; Day et al., 2018a). A slightly drawn-out extinction may therefore have been the case, with the extinction of the last surviving dinocephalians occurring as extinction rates were returning to normal. Nevertheless, the actual extinction interval is likely more restricted than that depicted in Figure 4B.
Above the last occurrence of dinocephalians, there is an interval of varying thickness in which the only taxa present are a few survivors of the main extinction pulse (Figure 3). This interval corresponds best with the uppermost Tapinocephalus AZ as presented by Boonstra (1969). Although the number of therocephalian species is reduced, the abundance of fossils attributable to therocephalians does not decline rapidly, suggesting that at least one of the surviving taxa (Glanosuchus, Lycosuchus or Pristerognathus) may in fact increase in abundance compared to before the extinction event (Figure 4). The dicynodont Diictodon feliceps is particularly abundant in this interval, having become increasingly so from the onset of the extinctions in the lower Karelskraal Member. The first appearance of a new genus after this is Endothiodon, although an indeterminate burnetiamorph (BP/1/7555) was recovered from this interval at Muggefontein (Figure 3). This marks the beginning of the recovery and base of the overlying Endothiodon AZ (Day and Rubidge, 2020).
The upper part of the Poortjie Member produces mostly fossils of dicynodonts, primarily Diictodon and Endothiodon with lesser numbers of the survivor taxa Pristerodon and Emydops, but some typically Lopingian taxa also occur. These are mostly carnivores such as the small therocephalians (Ictidosuchoides and Ictidostoma) and the moderately large gorgonopsian Gorgonops. The appearance near the base of the overlying Hoedemaker Member of the cryptodont dicynodont Tropidostoma coincides with the appearance of a cohort of early Lopingian taxa and the extinction of the surviving large therocephalians, the reptile Eunotosaurus and the small gorgonopsian Eriphostoma.
The sequence of events comprising the Capitanian mass extinction in the Karoo can be summarized as a series of phases:
(1) Primary extinction phase. The main clustering of extinctions eliminating the majority of species present in the upper Tapinocephalus AZ occurs through the Karelskraal Member of the Abrahamskraal Formation and into the lowermost part of the Poortjie Member. Although most clades are affected, the biggest losses are among those that achieved large body size (>2.5 m total body length): dinocephalians became altogether extinct and pareiasaurs were extirpated from the basin with the extinction of the three genera of the Bradysauria (Bradysaurus, Embrithosaurs and Nochelesaurus; see Van Den Brandt et al., 2020). Varanopids and basal anomodonts also disappeared. Throughout this phase, the dicynodont Diictodon became increasingly common (Figure 4A).
(2) Low diversity interval. This is characterized by only by a few survivor taxa. The assemblage primarily comprises small herbivorous dicynodonts, with Emydops arctatus and Pristerodon mackayi being greatly outnumbered by Diictodon feliceps. The carnivore guild consists of three genera of medium-large basal therocephalians and the small gorgonopsian Eriphostoma. This interval varies between 20 m at Puntkraal in the west and 90 m at Vanvuurenskop in the east (Figure 3), though at Muggefontein and Vanvuurenskop this may be exaggerated by low sampling.
(3) Primary recovery phase. The first new genus to appear in the primary recovery phase is Endothiodon, followed by a several small carnivorous therocephalians, the first large gorgonopsian and the kingoriid dicynodont Dicynodontoides. This phase remains low in taxic diversity and like the preceding interval displays high unevenness in the relative distribution of taxa (Day et al., 2018a).
(4) Secondary recovery phase. The appearance of cryptodont dicynodonts characterizes the beginning of the secondary recovery, along with at least two species of burnetiamorph and several more species of therocephalians and gorgonopsians. Pareiasaurs also reappear in the basin at this time. These appearances coincide closely with the extinction of the survivor taxa: the reptile Eunotosaurus and the basal therocephalian families Scylacosauridae and Lycosuchidae. The dicynodont Brachyprosopus may also have survived to this point based on two specimens from the very top of the Poortjie Member at two separate localities (BP/1/7803, a small skull and lower jaw from Spinnekopkraal, Fraserburg, and BP/1/7590, a small skull mixed in with an assemblage on medium-sized postcranial bones belonging to a different animal); however, for the time being it has not been ruled out that they are in fact juvenile specimens of Endothiodon. Diictodon becomes even more abundant and overwhelmingly dominates the assemblage. Three species of dicynodont (Diictodon feliceps, Pristerodon mackayi, Emydops arctatus) and two species of temnospondyl (Rhinesuchus whaitsi, Rhinesuchoides tenuiceps) range through to this assemblage from before the primary extinction phase.
The above pattern of faunal turnover during the Capitanian mass extinction displays several similarities to the phased extinction proposed by Smith and Botha-Brink (2014) for the end-Permian mass extinction higher in the Karoo sequence. In both cases, an initial period of extinction removes the majority of species from the pre-existing ecosystem (Figure 4). This is followed by a recovery period in which the first taxa characteristic of the next assemblage appear, including opportunistic taxa that capitalized on vacant ecospace and thus became at least initially common. For the Capitanian extinction, this is the dicynodont Endothiodon, whereas for the end-Permian extinction, these are two species of the dicynodont Lystrosaurus: L. murrayi and L. declivis.
Both extinction events are also characterized by the survival of a few species that became extinct at the same time shortly after the recovery had begun. The secondary extinction of these ‘dead clades walking’ (Jablonski, 2002) define the end of the initial recovery phase and, in the case of the end-Permian mass extinction, represent the final extirpation of species-level taxa present prior to the extinction event. This contrasts with the Capitanian extinction, where several dicynodont genera and two temnospondyl genera range through. One of these species, Diictodon feliceps, increases in abundance during the primary extinction phase to become the dominant taxon of the ecosystem (Figure 4A; see also Day et al., 2018a) and remains so throughout the recovery. Diictodon is therefore more comparable with Lystrosaurus after the end-Permian mass extinction than is Endothiodon.
Because several taxa range through the Capitanian mass extinction in the Karoo, the relative species extinction rate is lower than that for the end-Permian mass extinction (perhaps 84% as opposed to 100% across both primary and secondary extinctions). Yet it is worth noting that species richness may have been greater in the upper Tapinocephalus AZ than it was in the upper Daptocephalus AZ at the onset of the end-Permian mass extinction, given the uncertain presence of many species in the latter biozone (17 out of 33; Viglietti, 2020).
Ecological Change
In the Karoo Basin, the Capitanian mass extinction is characterized by a change from a dinocephalian-to a dicynodont-dominated tetrapod fauna. The primary extinction phase reduces diversity across most tetrapod clades except rhinesuchid temnospondyls but it is the larger components of the ecosystem that are worst affected: the only taxa that became completely extinct were dinocephalians and bradysaurian pareiasaurs, of which all species from the Tapinocephalus AZ grew to over 2.5 m and may have weighed between 700 to 1100 Kg (Lee, 1997; Romano and Rubidge, 2019). The vastly different dental adaptations of pareiasaurs and herbivorous dinocephalians suggests they had different diets, so body size remains the main common attribute. The fauna immediately succeeding the primary extinction phase displays the characteristics of low diversity, small mean body size, and high unevenness (species abundance distribution) when compared with that preceding the extinction phase, which are consistent with a post-extinction fauna experiencing one form of the Lilliput effect (Urbanek, 1993; Harries and Knorr, 2009).
Within the carnivore guild of the Tapinocephalus AZ, the only gorgonopsian species currently recognised is the relatively small-bodied Eriphostoma microdon (Kammerer et al., 2015), compared to eight therocephalian species (Day and Rubidge, 2020). During the primary recovery, large species of gorgonopsians appeared for the first time in the form of Gorgonops torvus, and by the upper part of the Tropidostoma-Gorgonops Subzone there were seven gorgonopsian genera of which several were large, namely the rubidgeines Aelurognathus and Smilesaurus (Day and Smith, 2020). At the same time, the therocephalians that appear within the Endothiodon AZ are all relatively small, especially compared to the lycosuchids. The taxic diversity of therocephalians is initially higher than gorgonopsians during the recovery up to the mid-Tropidostoma-Gorgonops Subzone, but thereafter gorgonopsians are the more speciose clade (Day and Smith, 2020). The Capitanian mass extinction thus caused a shift in the body size distributions and relative diversity of the predatory theriodonts, whereby therocephalians came to dominate the small carnivore niches and gorgonopsians diversified into the ecospace vacated by the large/medium-sized predators Anteosaurus and basal therocephalians.
Prior to the to the mass extinction, dicynodonts in the Tapinocephalus AZ all have relatively small body sizes. Endothiodon bathystoma could achieve moderately large size (e.g., in excess of 100 Kg with skull length over 50 cm; Araújo et al., 2018; Maharaj et al., 2019) and it remained the only large dicynodont until the appearance of Rhachiocephalus in the Tropidostoma-Gorgonops Subzone; the other dicynodonts that appear as part of the secondary recovery in the lower part of this subzone are mostly medium-sized. The genus Endothiodon possessed a complex masticatory system (see Cox and Angielczyk, 2015) that has been linked to various forms of specialised feeding and that may have played a role in its success in the aftermath of the Capitanian mass extinction. This was discussed by Rey et al. (2020), who determined that Endothiodon bathystoma probably did not consume significant quantities of seeds or roots, but rather fed on riparian vegetation. They postulated Endothiodon bathystoma may have colonised the Karoo Basin as riparian woodland was re-established there following a period of aridity, which was associated with a reduction in water-dependent plant species and the tetrapod mass extinction event (Barbolini, 2014; Rey et al., 2018). Current evidence is consistent with Endothiodon bathystoma capitalizing on ecospace vacated by large herbivorous dinocephalians and pareiasaurs, though it remains tempting to ascribe its success, at least partially, to the specifics of its masticatory adaptations.
A short-lived increase in aridity at the time of the primary extinction interval (Rey et al., 2018) may have played a role in the early success of Diictodon, and to a lesser extent Pristerodon, if, for instance, riparian woodland was replaced by an expansion of groundcover vegetation along watercourses. Diictodon survived up until shortly before the P-T boundary, where its extinction has been linked to the loss of such vegetation during the severe droughts associated with that mass extinction (Smith and Botha-Brink, 2014). Although Diictodon has been found associated with vertebrate burrows, no such burrows have yet been found stratigraphically lower than the base of the Lycosuchus-Eunotosaurus Subzone of the Endothiodon AZ (Figure 3). This suggests that such behaviour was a novel adaptation to the new landscape rather than a characteristic that enabled it to survive the Capitanian mass extinction.
A predator-prey relationship between the predatory scylacosaurid and lycosuchid therocephalians and the gorgonopsian Eriphostoma on the one hand, and small dicynodonts like Diictodon and Pristerodon on the other, is implied by their survival through the primary extinction phase. Lycosuchids and the larger scylacosaurids were large enough to prey on animals such as juvenile dinocephalians and Endothiodon. The continued abundance of Diictodon, Pristerodon and Endothiodon into the Tropidostoma-Gorgonops Subzone suggests that a reduction in food availability was not a likely contributor to the disappearance of large predatory therocephalians during the secondary extinction.
Dating
The application of U-Pb geochronology in the south African Karoo sequence over the last decade has greatly improved age constraints on the tetrapod biostratigraphy. U-Pb CA-TIMS analysis of zircon from tuffaceous horizons has consistently supported a Guadalupian-Lopingian age for the lower Beaufort Group, incorporating the Eodicynodon to Daptocephalus assemblage zones (Rubidge et al., 2013; Day et al., 2015a; Gastaldo et al., 2015; Gastaldo et al., 2020). An age of 260.259 ± 0.081 Ma was reported by Day et al. (2015a) from the base of the Teekloof Formation at Puntkraal in the Northern Cape Province, close to the top of the Tapinocephalus AZ. This age was better constrained biostratigraphically and clarified the position of the oldest three ages reported by Rubidge et al. (2013) from the Eastern Cape Province, where such constraints were poorer due to a paucity of index fossils surrounding the lower horizons. At Puntkraal, the dated horizon occurs 29 m below the highest occurrence of a dinocephalian and 49 m below the lowest occurrence (LO) of Endothiodon (Figure 3).
The age of the Guadalupian/Lopingian boundary currently accepted by the International Commission for Stratigraphy is 259.1 ± 0.5 Ma (Zhong et al., 2014; Schneider et al., 2020), thus being approximately 1 m.y. younger than the dated horizon at Puntkraal. This is slightly younger than an age of 259.26 Ma for a tuffaceous horizon in the lower Middleton Formation (=Teekloof Formation) in the Eastern Cape (Rubidge et al., 2013). This horizon is constrained only by the close occurrence of Endothiodon, which is most abundant in the Lycosuchus-Eunotosaurus Subzone of the Endothiodon Assemblage Zone (formerly the Pristerognathus AZ; Day and Smith, 2020), and led Day et al. (2015a) to conclude that the Guadalupian/Lopingian boundary most likely lay in the upper part of this assemblage zone. The mass extinction at the top of the Tapinocephalus AZ is thus a late Capitanian mass event.
Capitanian Tetrapod Extinctions Outside South Africa
Laurasia
Tetrapod body fossils of late Guadalupian or early Lopingian age are known from France, Germany, Kazakhstan, and possibly China (Olroyd and Sidor, 2017; Lucas, 2018) but these are either too depauperate, isolated or poorly constrained temporally to provide much information on nature of the Capitanian mass extinction. The exception is the west Urals region of Russia, where three tetrapod assemblages (Isheevo, Sundyr, and Kotelnich) record faunal changes surrounding the dinocephalian mass extinction (Sennikov and Golubev, 2017). The recently discovered Sundyr assemblage partially fills a stratigraphic gap between the Isheevo and Kotelnich assemblages that had been postulated in light of discrepancies in independent dating (Day et al., 2015a; Lucas, 2017; Lucas, 2018), and this elucidates the progression of the dinocephalian extinctions in Russia; however, the distinction between these faunas and the absence of well-sampled localities of more than one within the same section means that the sequence of events is not as clear as in South Africa. Absolute age constraints on this part of the Russian sequence are also less secure.
In Russia, the Isheevo fauna has been widely correlated with the Tapinocephalus AZ because of the shared presence of tapinocephalid and anteosaurid dinocephalians, biarmosuchians, basal anomodonts and the possible scylacosaurid therocephalian Porosteognathus (Rubidge, 2005; Lucas, 2006; Lucas, 2018; Kammerer and Masyutin, 2018a), although the oldest part of the Isheevo assemblage appears to be Wordian and may therefore slightly older than the estimated base of the Tapinocephalus AZ (Schneider et al., 2020). Significant turnover occurred between the Isheevo assemblage and the succeeding (until recently) Kotelnich subassemblage with the loss of numerous clades including dinocephalians, lanthanosuchid and bolosaurid parareptiles, archegosaurid temnospondyls, and enosuchid anthracosaurs (Sennikov and Golubev, 2017). The intervening Sundyr assemblage indicates that extinctions and recovery began in aquatic communities first, with the replacement of typically Guadalupian genera of temnospondyls and anthracosaurs by late Permian forms, while terrestrial faunas still resembled Guadalupian ecosystems and included dinocephalians (Golubev, 2015; Sennikov and Golubev, 2017).
The true diversity represented by collections from the Sundyr assemblage is far from established. So far two large therocephalians assigned to the genera Julognathus and Gorynychus have been described (Suchkova and Golubev, 2019a; Suchkova and Golubev, 2019b). The descriptions suggest these genera belong to the two Guadalupian families of therocephalians, Lycosuchidae and Scylacosauridae, respectively, although Gorynychus was originally considered eutherocephalian by Kammerer and Masyutin (2018a). The diversity of other taxa potentially comparable with South Africa remains unclear, especially the dinocephalians.
The Kotelnich subassemblage is the next major assemblage and is devoid of dinocephalians, being instead most clearly characterized by the appearance of Gondwanan clades such as pareiasaurs, dicynodonts and possibly gorgonopsians (depending on the identity of Kamagorgon ulanovi;Kammerer et al., 2015), as well as the diversification of theriodontian therapsids (Sennikov and Golubev, 2017). Among the latter, the presence of small gorgonopsians and a number of eutherocephalian taxa, including baurioids, suggests similarities with the early recovery faunas in the Karoo (Kammerer and Masyutin, 2018a; Kammerer and Masyutin, 2018b). Guadalupian clades such as nycteroleter parareptiles and basal anomodonts of the Russian clade Venyukovioidea are still present but became extinct within this or the succeeding Ilinskoe subassemblage (Benton et al., 2012; Tsuji et al., 2012; Sennikov and Golubev, 2017), perhaps representing ‘dead clades walking’ in the Russian post-extinction fauna similar to the scylacosaurid and lycosuchid therocephalians or Eunotosaurus in the Karoo. These similarities support the correlation between the Kotelnich subassemblage (as it occurs in the lower Vanyushonki Member) and the early recovery fauna of the lower Endothiodon AZ (previously the Pristerognathus AZ) (Golubev, 2005; Benton et al., 2012; Day et al., 2018b; Kammerer and Masyutin, 2018b). This is further reinforced by the appearance of cryptodont dicynodonts (Australobarbarus) in the upper part of the assemblage at Port Kotelnich, which suggests a correlation with the Tropidostoma-Gorgonops Subzone. The Russian record thus indicates a stepped extinction pattern, but without better temporal constraints it is not possible to tell if this occurred over a comparable period of time to that in the Karoo.
In the low latitude basins of Europe the tetrapod body fossil record is poor around the Capitanian-Lopingian boundary but the ichnological record suggests the presence of dinocephalians in the Guadalupian of France (La Lieude Formation) and their absence in early Lopingian formations of Scotland and Germany (Cornockle and Cornberg formations; Marchetti et al., 2019). The Cornockle and Cornberg formations contain the earliest record of tracks attributed to dicynodonts and pareiasaurs, which matches the appearance of these clades in the assemblages immediately succeeding the last occurrence of dinocephalians in European Russia (Sennikov and Golubev, 2017; Marchetti et al., 2019). This appearance of Gondwanan taxa may have been related to low eustatic sea levels opening dispersal routes along coastlines (e.g., Kemp, 2006). Although Diictodon also penetrated into Laurasia, it is currently only known from the late Permian Junggur Basin of China (Lucas, 2005) and so its dispersal does not appear to have been closely related to the Capitanian mass extinction.
Gondwana
Outside of South Africa, tetrapod assemblages surrounding the dinocephalian extinction are known from Brazil, Tanzania, Zambia, Zimbabwe, and possibly Niger (Olroyd and Sidor, 2017; Lucas, 2018). The Rio do Rasto Formation of Brazil has produced fossils of typically Guadalupian taxa, such as archegosaurid temnospondyls and tapinocephalid and anteosaurid dinocephalians, but also Endothiodon. The fossils come from localities across the outcrop of the Rio do Rasto Formation but primarily from three areas: Posto Queimado, Aceguà, and Serra do Cadeado. The latter site, from which Endothiodon is known, was for some time thought to expose a younger horizon than those that produced dinocephalians further south (see Boos et al., 2013); however, the discovery of a tapinocephalid dinocephalian in the Serra do Cadeado area suggests that either the dinocephalians and Endothiodon co-occur or that stratigraphic horizons are so thin that the assemblages from each of the localities may comprise non-contemporaneous taxa (Boos et al., 2015).
Closer to the Karoo, both the Madumabisa Mudstone Formation of Zambia and the Ruhuhu Formation of Tanzania record older assemblages that include dinocephalians and younger assemblages that lack dinocephalians and include Endothiodon (Olroyd et al., 2017; Olroyd and Sidor, 2017). The temporal gap between these assemblages is not well understood, but in the Ruhuhu Formation two assemblages within the calcareous beds that were informally referred to as R1 and R2 by Angielczyk et al. (2014) may lie close to the extinction. The lowest horizon (R1) contains tapinocephalid dinocephalians, fragmentary temnospondyls, and at least one basal endothiodont dicynodont, Abajudon kaayai, and was thus correlated broadly with the Tapinocephalus AZ (Simon et al., 2010; Angielczyk et al., 2014; Sidor et al., 2014; Olroyd et al., 2018). The middle horizon (R2) contains a tusked species of Endothiodon, E. tolani, and at least one other indeterminate small dicynodont but no dinocephalians, which led Angielczyk et al. (2014) to propose it that it was likely late Capitanian and correlated with the former Pristerognathus AZ (lower subzone of the new Endothiodon AZ), post-dating the extinction of dinocephalians. The third and youngest horizon of the Ruhuhu Formation contains occurrences of Dicynodont huenei, Rhachiocephalus and Endothodon cf. bathystoma and is posited to correlate with the Cistecephalus AZ in the Karoo (Angielczyk et al., 2014; Cox and Angielczyk, 2015), thus postdating the recovery discussed here.
Without independent means of constraining the age of the East African tetrapod faunas, it is difficult to comment on the patterns of extinction there and to disentangle them from biogeographic shifts in the range of individual species or genera (Olroyd and Sidor, 2017). Nevertheless, at least one species of Endothiodon co-occurs with dinocephalians in Tanzania and possibly Brazil, whereas at least one other is present in post-dinocephalian faunas from across Gondwana, indicating that the genus expanded its range sometime after the extinction of dinocephalians. The occurrence of the endothiodont Abajudon with dinocephalians in both Tanzania and Zambia, and of E. tolani in Tanzania suggests that Endothiodon had evolved in lower latitude and dispersed from there (Olroyd et al., 2017). The data from South Africa shows that Endothiodon arrived in the Karoo not long after the primary extinction phase there.
The most successful benefactor of the mass extinction in the Karoo, Diictodon feliceps, is by contrast rare outside of South Africa. We have mentioned that it occurs in the latest Permian of China but in Gondwana (outside of South Africa) it is known only from the Luangwa Basin of Zambia, where it is found in the upper Madumabisa Mudstone Formation (Angielczyk and Sullivan, 2008). Angielczyk et al. (2014) have suggested that the fauna known from this formation correlates best with the Cistecephalus AZ, implying a gap in the Zambian record that includes the recovery phase. Thus, there is no evidence that Diictodon was able to enlarge its range outside of the Karoo Basin in the immediate aftermath of the Capitanian mass extinction.
Causes
What were the proximal causes of vertebrate extinctions in the late Capitanian?. Most work on potential mechanisms has come from studies of marine sections, where ocean acidification, anoxia, cooling, particularly low eustatic sea level, and photosynthetic shutdown from volcanic activity have all been implicated (Bond et al., 2010; McGhee et al., 2013; Bond et al., 2015; Bond and Grasby, 2017). The coincidence of volcanism in the Emeishan Large Igneous Province (ELIP) of southern China with the marine extinctions in nearby sections, as well as correlated sections elsewhere, has led to suspicion that this was a likely ultimate cause (e.g., Wignall et al., 2009; Bond and Wignall, 2014). The timing of marine extinctions varies depending on the section and taxa in question (e.g., Shen and Shi, 2009; Bond et al., 2010) but new U-Pb geochronology from the base of the Yinping Formation near Chaohu in the Yangtze region of south China, which lies outside the ELIP, suggests that most marine extinctions occurred before 261.6 ± 1.6 Ma (Zhang et al., 2019). The ELIP itself is thought to have been active between ∼263 Ma and 259.1 ± 0.5 Ma (Sun et al., 2010; Zhong et al., 2014), which would constrain most marine extinctions to the earlier part of its activity. There is thus the possibility that the marine extinctions occurred earlier than the tetrapod extinctions, of which at least some post-date 260.26 Ma (Day et al., 2015a), although the age from Chaohu does fall within error of the date from the base of the Poortjie Member. Both marine and terrestrial extinctions nevertheless occur within the window of ELIP volcanism.
How volcanism can lead to extinction is mostly discussed in terms of global temperature changes related to the release of CO2, that causes warming, and SO2, that forms sulfate aerosols that cause cooling (Bond and Grasby, 2017). The ratio of stable isotopes of carbon through the geological past record changes in the carbon cycle and can thus provide evidence for large-scale phenomena such as ELIP volcanism. A positive shift in δ13C has been reported in the mid Capitanian, which was initially associated with a period of global cooling and subsequent eutrophication called the ‘Kamura event’ (Isozaki et al., 2007; Bond et al., 2015), though recently this has become controversial and may in fact be a result of global warning (Cao et al., 2018; Zhang et al., 2020). Small positive δ13C shifts have been recorded in the latest Capitanian (Chen et al., 2011; Nishikane et al., 2014; Wei et al., 2018) but the marine extinctions, at least in some sections, are associated with a negative excursion in the mid-late Capitanian (e.g., Bond et al., 2010; Bond et al., 2015). Although volcanic outgassing of CO2 would be expected to introduce light carbon into the atmosphere, the long duration and the inconsistency in the magnitude, or indeed, presence of this negative excursion in some sections, including the Capitanian-Wuchiapingian boundary GSSP at Penglaitan, suggests that it was not related to volcanogenic CO2 (Jost et al., 2014; Zhang et al., 2020). Instead, the negative excursion may more likely record the local influence of an increased input of terrestrial organic material resulting from regression (Zhang et al., 2020).
Another geochemical variable that has become increasingly used as a proxy for volcanic activity is the concentration of mercury in geological sections (e.g., Sial et al., 2016; Grasby et al., 2016; Grasby et al., 2017; Percival et al., 2017; Thibodeau and Bergquist, 2017; Kwon et al., 2019). This also provides an additional extinction mechanism for several mass extinctions including the Capitanian (Grasby et al., 2016). However, mercury is also found in organic material and so its increased abundance in association with negative carbon may simply reflect the increased terrestrial weathering (Bergquist, 2017; Grasby et al., 2017; Thibodeau and Bergquist, 2017). Although these same authors show that Hg isotopes can be useful in disentangling organic from volcanogenic mercury, this has not yet been done for the Capitanian. Direct evidence for the role of ELIP volcanism in the marine Capitanian mass extinction outside of the ELIP itself is thus lacking at present.
In terrestrial environments, volcanism could drive extinctions through temperature change, or defoliation resulting from acid rain, toxicity, or suppressed photosynthesis due to particulate matter in the atmosphere (Bond and Grasby, 2017). However, evidence linking ELIP volcanism to the terrestrial extinctions is similarly lacking for this as in the marine realm. In South Africa, stable isotope geochemistry of vertebrate tissues has demonstrated a positive shift in δ13C across the extinction interval in lowest Poortjie Member (uppermost Tapinocephalus AZ), which was interpreted to represent an increase in aridity resulting from regional tectonics (Rey et al., 2018). Importantly, Rey et al. (2018) did not find a noticeable change in relative abundance of oxygen isotopes, suggesting that there was no significant change in temperature during the extinction interval. A period of increased aridity in the uppermost Karelskraal Member and lowermost Poortjie may be supported by a particularly low (<1) chemical index of alteration (CIA) value from the upper Karelskraal Member compared to surrounding horizons sampled by Paiva (2016), although this author concludes only that weathering rates and semi-arid conditions appear mostly consistent between the upper Abrahamskraal Formation and lower Teekloof Formation in the southwestern Karoo. It is also uncertain if the Karoo mudstones sample meet the criteria for the reliable use of CIA presented by Goldberg and Humayun (2010).
In terms of sedimentology, the deposition of the Poortjie Member has been linked to aridification and the reduction of vegetation cover in the source area for the southwestern Karoo, resulting in greater erosion and a consequent influx of arenaceous sediment to the basin (Turner, 1985; Cole and Wipplinger, 2001), or alternatively to tectonic activity in the source area (Paiva, 2016). In the southeastern Karoo, Catuneanu and Bowker (2001) determined tectonic influences were predominantly responsible for the arenaceous packages in the Koonap (=Abrahamskraal) and overlying Middleton formations in that area, and that sedimentological evidence suggested a consistent palaeoclimatic through the whole sequence that was temperate to humid, in contrast to the southwest.
Palynology from the Abrahamskraal and Teekloof formations in the southwestern Karoo suggests the extinction of some hydrophytic and mesophytic plant species (Barbolini, 2014) but the plant macrofossil record through the Abrahamskraal-Teekloof transition is poor. Extinctions of plant species are reported from the mid-late Permian of northern China, one of which may be contemporaneous with the Capitanian mass extinction in the Karoo (Stevens et al., 2011) but little other data is available on palaeobotanical change during this time.
In summary, the causes of the Capitanian mass extinction of vertebrates remain unclear. In the Main Karoo Basin of South Africa, vertebrate extinctions may have been partly driven by aridification resulting from regional tectonics; however, evidence for a tectonic cause for this aridification is mostly circumstantial and does not provide an explanation for terrestrial extinctions outside of southern Gondwana. Volcanism in the ELIP is still a candidate, and chemical signals of volcanism such as mercury that can be traced to that province may yet be found. Another cause could be a changed weather patterns associated with the late Capitanian eustatic lowstand, which was the most pronounced of the late Palaeozoic (Haq and Schutter, 2008; Zhang et al., 2019). Assessing the role of climate change in the Capitanian tetrapod extinctions will require higher resolution sampling for geochemical climate proxies across the extinction interval. Biotic factors such as competition can be ruled out on the basis that new taxa do not begin to appear in the Karoo until after the primary extinction phase.
Conclusion
(1) The Capitanian mass extinction was significant global event in tetrapod evolutionary history with global effects, most clearly evident in high latitude basins like the Karoo Basin of South Africa.
(2) In the Karoo, it displays a similar phased pattern to the end-Permian mass extinction, with a primary extinction phase through the Karelskraal Member and lower Poortjie Member, followed by a low diversity interval, then an early recovery phase in the upper Poortjie Member, and ending with a second set of extinctions associated with the appearance of more recovery taxa at the base of the Hoedemaker Member. Despite potentially greater absolute numbers of species extinctions, the Capitanian mass extinction did not lead to complete species-level turnover and had less of an impact at higher taxonomic levels.
(3) The primary extinction phase can be tightly constrained to around 260.26 Ma, although the age of the secondary extinctions remains uncertain.
(4) Dinocephalian therapsids, varanopids, the Gondwanan basal anomodonts and bradysaurian pareiasaurs became extinct during the main phase of extinctions, along with many species of dicynodonts and therocephalians. The therocephalian families Scylacosauridae and Lycosuchidae, and the reptile Eunotosaurus africanus became extinct during the secondary extinction, along with the small gorgonopsian genus Eriphostoma. The recovery begins before the secondary extinction pulse.
(5) In Russia, a phased extinction is also evident but appears more complex. Lanthanosuchid and bolosaurid parareptiles, archegosaurid temnospondyls, and enosuchid anthracosaurs became extinct first, followed by dinocephalians. The recovery began first in the aquatic part of the ecosystem. Venyukovioid anomodonts and nycteroleter parareptiles survived for a short time into the terrestrial recovery fauna, typified by gorgonopsians, therocephalians and derived pareiasaurs.
(6) The biogeographic effects of the Capitanian mass extinction are partly obscured by uneven and geographically limited sampling, particularly at lower latitudes, but it is clear that following the dinocephalian extinction in Laurasia there was an influx of tetrapod taxa previously limited to Gondwana (e.g. pareiasaurs and dicynodonts). In Gondwana, the dicynodont genus Endothiodon became widespread.
(7) A mechanism driving the tetrapod extinctions at a global level has not yet been clearly identified. The temporal coincidence of volcanism in the Emeishan LIP and global regression may suggest a causal relationship, but further work is required to identify climate change or the direct volcanic influences, such as mercury, in the extinction interval. Currently, geological data from the Karoo suggests only minor climatic differences between the time of deposition of the Abrahamskraal and Teekloof formations, though a short-lived period of aridification may have played a role in driving the tetrapod extinctions locally.
Data Availability Statement
The original contributions presented in the study are included in the article/Supplementary Material, further inquiries can be directed to the corresponding author.
Author Contributions
MD analysed the data and produced the figures; MD and BR collected the data, wrote the manuscript, and acquired funding.
Conflict of Interest
The authors declare that the research was conducted in the absence of any commercial or financial relationships that could be construed as a potential conflict of interest.
The handling editor declared a past co-authorship with one of the authors (MD).
Acknowledgments
We gratefully acknowledge the financial support of the Palaeontological Scientific Trust (PAST) and its Scatterlings of Africa programmes, the National Research Foundation (NRF), the DSI/NRF Center of Excellence for Palaeosciences (CoE-Pal), and the University of the Witwatersrand. We would also like to thank the many people who have contributed to fieldwork over the last decade. NRF/DST Centre of Excellence in Palaeoscience and National Research Foundation (South Africa) are thanked for contributing funds towards fieldwork and project support. The Palaeontological Scientific Trust (PAST) is thanked for contributing funds towards fieldwork. The Natural History Museum covered publishing costs. We are grateful to Sean Modesto, Christian Sidor, and Cesar Schultz for their constructive reviews.
Supplementary Material
The Supplementary Material for this article can be found online at: https://www.frontiersin.org/articles/10.3389/feart.2021.631198/full#supplementary-material.
References
Angielczyk, K. D., Huertas, S., Smith, R. M. H., Tabor, N. J., Sidor, C. A., Steyer, J.-S., et al. (2014). New dicynodonts (Therapsida, Anomodontia) and updated tetrapod stratigraphy of the Permian Ruhuhu Formation (Songea Group, Ruhuhu basin) of southern Tanzania. J. Vertebr. Paleontol. 34 (6), 1408–1426. doi:10.1080/02724634.2014.880448
Angielczyk, K. D., and Sullivan, C. (2008). Diictodon feliceps (owen, 1876), a dicynodont (therapsida, anomodontia) species with a pangaean distribution. J. Vertebr. Paleontol. 28 (3), 788–802. doi:10.1671/0272-4634(2008)28[788:dfoadt]2.0.co;2
Araújo, R., Fernandez, V., Rabbitt, R. D., Ekdale, E. G., Antunes, M. T., Castanhinha, R., et al. (2018). Endothiodon cf. bathystoma (Synapsida: dicynodontia) bony labyrinth anatomy, variation and body mass estimates. PLoS One 13 (3), e0189883. doi:10.1371/journal.pone.0189883 |
Barbolini, N. (2014). Palynostratigraphy of the South African Karoo supergroup and correlations with coeval gondwanan successions. PhD thesis. Johannesburg, South Africa: University of the Witwatersrand, 386.
Benton, M. J., Newell, A. J., Khlyupin, A. b. Y., Shumov, I. y. S., Price, G. D., and Kurkin, A. A. 2012). Preservation of exceptional vertebrate assemblages in Middle Permian fluviolacustrine mudstones of Kotel'nich, Russia: stratigraphy, sedimentology, and taphonomy. Palaeogeogr. Palaeoclimatol. Palaeoecol. 319-320, 58–83. doi:10.1016/j.palaeo.2012.01.005
Bergquist, B. A. (2017). Mercury, volcanism, and mass extinctions. Proc. Natl. Acad. Sci. U.S.A. 114 (33), 8675–8677. doi:10.1073/pnas.1709070114 |
Bond, D. P. G., and Grasby, S. E. (2017). On the causes of mass extinctions. Palaeogeogr. Palaeoclimatol. Palaeoecol. 478, 3–29. doi:10.1016/j.palaeo.2016.11.005
Bond, D. P. G., Wignall, P. B., Joachimski, M. M., Sun, Y., Savov, I., Grasby, S. E., et al. (2015). An abrupt extinction in the middle Permian (Capitanian) of the boreal realm (Spitsbergen) and its link to anoxia and acidification. GSA Bulletin. 127 (9–10), 1411–1421. doi:10.1130/b31216.1
Bond, D. P. G., and Wignall, P. B. (2014). Large igneous provinces and mass extinctions: an update. Geol. Soc. Am. Spec. Pap. 505, 29–55. doi:10.1130/SPE505
Bond, D. P. G., Wignall, P. B., Wang, W., Izon, G., Jiang, H. S., Lai, X. L., et al. (2010). The mid-Capitanian (Middle Permian) mass extinction and carbon isotope record of South China. Palaeogeogr. Palaeoclimatol. Palaeoecol. 292 (1-2), 282–294. doi:10.1016/j.palaeo.2010.03.056
Bond, D. P., Wignall, P. B., and Grasby, S. E. (2020). The Capitanian (Guadalupian, Middle Permian) mass extinction in NW Pangea (Borup Fiord, Arctic Canada): a global crisis driven by volcanism and anoxia. GSA Bull. 132 (5-6), 931–942. doi:10.1130/b35281.1
Boonstra, L. D. (1969). The fauna of the Tapinocephalus zone (Beaufort beds of the Karoo). Ann. S. Afr. Mus. 56, 1–73.
Boos, A. D. S., Schultz, C. L., Vega, C. S., and Aumond, J. J. (2013). On the presence of the Late Permian dicynodont Endothiodon in Brazil. Palaeontol. 56 (4), 837–848. doi:10.1111/pala.12020
Boos, A. D. S., Kammerer, C. F., Schultz, C. L., and Paes Neto, V. D. (2015). A tapinocephalid dinocephalian (Synapsida, Therapsida) from the Rio do Rasto Formation (Paraná Basin, Brazil): taxonomic, ontogenetic and biostratigraphic considerations. J. S. Am. Earth Sci. 63, 375–384. doi:10.1016/j.jsames.2015.09.003
Botha, J., Huttenlocker, A. K., Smith, R. M. H., Prevec, R., Viglietti, P., and Modesto, S. P. (2020). New geochemical and palaeontological data from the Permian-Triassic boundary in the South African Karoo Basin test the synchronicity of terrestrial and marine extinctions. Palaeogeogr. Palaeoclimatol. Palaeoecol. 540, 109467. doi:10.1016/j.palaeo.2019.109467
Cao, C., Cui, C., Chen, J., Summons, R. E., Shen, S., and Zhang, H. (2018). A positive C-isotope excursion induced by sea-level fall in the middle Capitanian of South China. Palaeogeogr. Palaeoclimatol. Palaeoecol. 505, 305–316. doi:10.1016/j.palaeo.2018.06.010
Catuneanu, O., and Bowker, D. (2001). Sequence stratigraphy of the Koonap and Middleton fluvial formations in the Karoo foredeep South Africa. J. Afr. Earth Sci. 33 (3-4), 579–595. doi:10.1016/s0899-5362(01)00095-1
Chen, B., Joachimski, M. M., Sun, Y., Shen, S., and Lai, X. (2011). Carbon and conodont apatite oxygen isotope records of Guadalupian-Lopingian boundary sections: climatic or sea-level signal?. Palaeogeogr. Palaeoclimatol. Palaeoecol. 311 (3-4), 145–153. doi:10.1016/j.palaeo.2011.08.016
Cole, D. I., and Wipplinger, P. E. (2001). Sedimentology and molybdenum potential of the Beaufort Group in the main Karoo Basin, South Africa. Memoir Counc. GeoSci. 80, 225.
Cox, C. B., and Angielczyk, K. D. (2015). A new endothiodont dicynodont (therapsida, anomodontia) from the permian Ruhuhu Formation (Songea Group) of Tanzania and its feeding system. J. Vertebr. Paleontol. 35 (4), e935388. doi:10.1080/02724634.2014.935388
Day, M. O., Ramezani, J., Bowring, S. A., Sadler, P. M., Erwin, D. H., Abdala, F., et al. (2015a). When and how did the terrestrial mid-Permian mass extinction occur? Evidence from the tetrapod record of the Karoo Basin, South Africa. Proc. Biol. Sci. 282 (1811), 20150834. doi:10.1098/rspb.2015.0834 |
Day, M. O., Güven, S., Abdala, F., Jirah, S., Rubidge, B., and Almond, J. (2015b). Youngest dinocephalian fossils extend the Tapinocephalus Zone, Karoo Basin, South Africa. S. Afr. J. Sci. 111 (3-4), 1–5. doi:10.17159/sajs.2015/20140309
Day, M. O., Benson, R. B. J., Kammerer, C. F., and Rubidge, B. S. (2018a). Evolutionary rates of mid-Permian tetrapods from South Africa and the role of temporal resolution in turnover reconstruction. Paleobiology 44 (3), 347–367. doi:10.1017/pab.2018.17
Day, M. O., Smith, R. M. H., Benoit, J., Fernandez, V., and Rubidge, B. S. (2018b). A new species of burnetiid (Therapsida, Burnetiamorpha) from the early Wuchiapingian of South Africa and implications for the evolutionary ecology of the family Burnetiidae. Pap Palaeontol. 4 (3), 453–475. doi:10.1002/spp2.1114
Day, M. O., and Rubidge, B. S. (2014). A brief lithostratigraphic review of the Abrahamskraal and Koonap formations of the Beaufort Group, South Africa: towards a basin-wide stratigraphic scheme for the Middle Permian Karoo. J. Afr. Earth Sci. 100, 227–242. doi:10.1016/j.jafrearsci.2014.07.001
Day, M. O., and Rubidge, B. S. (2019). Biesiespoort revisited: a case study on the relationship between tetrapod assemblage zones and Beaufort lithostratigraphy south of Victoria West. Palaeontol. Afr. 53, 51–65. https://hdl.handle.net/10539/26240.
Day, M. O., and Rubidge, B. S. (2020). Biostratigraphy of the Tapinocephalus Assemblage Zone (Beaufort Group, Karoo Supergroup), South Africa. S. Afr. J. Geol. 123 (2), 149–164. doi:10.25131/sajg.123.0012
Day, M. O., and Smith, R. M. H. (2020). Biostratigraphy of the Endothiodon Assemblage Zone (Beaufort Group, Karoo Supergroup), South Africa. S. Afr. J. Geol. 123 (2), 165–180. doi:10.25131/sajg.123.0011
Gastaldo, R. A., Kamo, S. L., Neveling, J., Geissman, J. W., Bamford, M., and Looy, C. V. (2015). Is the vertebrate-defined Permian-Triassic boundary in the Karoo Basin, South Africa, the terrestrial expression of the end-Permian marine event? Geol. 43 (10), 939–942. doi:10.1130/g37040.1
Gastaldo, R. A., Kamo, S. L., Neveling, J., Geissman, J. W., Looy, C. V., and Martini, A. M. (2020). The base of the Lystrosaurus Assemblage Zone, Karoo Basin, predates the end-Permian marine extinction. Nat. Commun. 11 (1), 1428–8. doi:10.1038/s41467-020-15243-7 |
Geological Survey (1979). “Geological map sheet 3222 Beaufort West (scale 1:250 000),” in Publication, geological Survey of South Africa. Editors A. Marsh, P. W. Leaman, and M. R. Johnson (Pretoria: Government Printer).
Geological Survey (1997). “Metallogenic map sheet 3220 Sutherland (scale 1:250 000),” in Publication, geological Survey of South Africa. Editors D. I. Cole and Vorster, C. J. (Pretoria: Government Printer).
Goldberg, K., and Humayun, M. (2010). The applicability of the chemical index of alteration as a paleoclimatic indicator: an example from the permian of the paraná basin. Braz. Palaeogeogr. Palaeoclimatol. Palaeoecol. 293 (1-2), 175–183. doi:10.1016/j.palaeo.2010.05.015
Golubev, V. K. (2005). Permian tetrapod stratigraphy. New Mexico Mus. Nat. Hist. Sci. Bull. 30, 95–99. https://www.google.co.uk/books/edition/The_Nonmarine_Permian/7r75CQAAQBAJ?hl=en&gbpv=0.
Golubev, V. K. (2015). Dinocephalian stage in the history of the Permian tetrapod fauna of Eastern Europe. Paleontol. J. 49 (12), 1346–1352. doi:10.1134/s0031030115120059
Grasby, S. E., Beauchamp, B., Bond, D. P. G., Wignall, P. B., and Sanei, H. (2016). Mercury anomalies associated with three extinction events (Capitanian crisis, latest Permian extinction and the Smithian/Spathian extinction) in NW Pangea. Geol. Mag. 153 (2), 285–297. doi:10.1017/s0016756815000436
Grasby, S. E., Shen, W., Yin, R., Gleason, J. D., Blum, J. D., Lepak, R. F., et al. (2017). Isotopic signatures of mercury contamination in latest Permian oceans. Geology 45 (1), 55–58. doi:10.1130/g38487.1
Haq, B. U., and Schutter, S. R. (2008). A chronology of Paleozoic sea-level changes. Sci. 322 (5898), 64–68. doi:10.1126/science.1161648
Harries, P. J., and Knorr, P. O. (2009). What does the ‘Lilliput Effect’mean?. Palaeogeogr. Palaeoclimatol. Palaeoecol. 284 (1-2), 4–10. doi:10.1016/j.palaeo.2009.08.021
Isozaki, Y., Kawahata, H., and Minoshima, K. (2007). The Capitanian (Permian) Kamura cooling event: the beginning of the Paleozoic–Mesozoic transition. Palaeoworld 16 (1-3), 16–30. doi:10.1016/j.palwor.2007.05.011
Jablonski, D. (2002). Survival without recovery after mass extinctions. Proc. Natl. Acad. Sci. U.S.A. 99 (12), 8139–8144. doi:10.1073/pnas.102163299 |
Jost, A. B., Mundil, R., He, B., Brown, S. T., Altiner, D., Sun, Y., et al. (2014). Constraining the cause of the end-Guadalupian extinction with coupled records of carbon and calcium isotopes. Earth Planet Sci. Lett. 396, 201–212. doi:10.1016/j.epsl.2014.04.014
Kammerer, C. F., and Masyutin, V. (2018a). A new therocephalian (Gorynychus masyutinae gen. et sp. nov.) from the Permian Kotelnich locality, Kirov Region, Russia. PeerJ 6, e4933. doi:10.7717/peerj.4933 |
Kammerer, C. F., and Masyutin, V. (2018b). Gorgonopsian therapsids (Nochnitsa gen. Nov. and Viatkogorgon) from the permian Kotelnich locality of Russia. PeerJ 6, e4954. doi:10.7717/peerj.4954 |
Kammerer, C. F., Smith, R. M. H., Day, M. O., and Rubidge, B. S. (2015). New information on the morphology and stratigraphic range of the mid-Permian gorgonopsian Eriphostoma microdon Broom, 1911. Pap. Palaeontology 1 (2), 201–221. doi:10.1002/spp2.1012
Kemp, T. S. (2006). The origin and early radiation of the therapsid mammal-like reptiles: a palaeobiological hypothesis. J. Evol. Biol. 19, 1231–1247. doi:10.1111/j.1420-9101.2005.01076.x |
Keyser, A. W., and Smith, R. H. M. (1978). Vertebrate biozonation of the Beaufort Group with special reference to the western Karoo Basin. Ann. Geol. Surv. S. Afr. 12, 1–36.
Kwon, H., Kim, M. G., and Lee, Y. I. (2019). Mercury evidence from the Sino-Korean block for Emeishan volcanism during the Capitanian mass extinction. Geol. Mag. 156 (6), 1105–1110. doi:10.1017/s0016756818000481
Lee, M. S. (1997). A taxonomic revision of pareiasaurian reptiles: implications for Permian terrestrial palaeoecology. Mod. Geol. 21 (3), 231–298. doi:10.1213/00000539-199707000-00051
Lucas, S. G. (2005). Age and correlation of Permian tetrapod assemblages from China. New Mexico Mus. Nat. Hist. Sci. Bull. 30, 187–191. https://www.google.co.uk/books/edition/The_Nonmarine_Permian/7r75CQAAQBAJ?hl=en&gbpv=0.
Lucas, S. G. (2006). Global Permian tetrapod biostratigraphy and biochronology. Geol. Soc. London Spec. Publ. 265 (1), 65–93. doi:10.1144/gsl.sp.2006.265.01.04
Lucas, S. G. (2009). Timing and magnitude of tetrapod extinctions across the Permo-Triassic boundary. J. Asian Earth Sci. 36 (6), 491–502. doi:10.1016/j.jseaes.2008.11.016
Lucas, S. G. (2017). Permian tetrapod extinction events. Earth Sci. Rev. 170, 31–60. doi:10.1016/j.earscirev.2017.04.008
Lucas, S. G. (2018). Permian tetrapod biochronology, correlation and evolutionary events. Geol. Soc. London Spec. Publ. 450 (1), 405–444. doi:10.1144/sp450.12
Maharaj, I. E. M., Chinsamy, A., and Smith, R. M. H. (2019). The postcranial anatomy of Endothiodon bathystoma (Anomodontia, Therapsida). Hist. Biol., 1–23. doi:10.1080/08912963.2019.1679128
Marchetti, L., Voigt, S., and Lucas, S. G. (2019). An anatomy-consistent study of the Lopingian eolian tracks of Germany and Scotland reveals the first evidence of the end-Guadalupian mass extinction at low paleolatitudes of Pangea. Gondwana Res. 73, 32–53. doi:10.1016/j.gr.2019.03.013
McGhee, G. R., Clapham, M. E., Sheehan, P. M., Bottjer, D. J., and Droser, M. L. (2013). A new ecological-severity ranking of major Phanerozoic biodiversity crises. Palaeogeogr. Palaeoclimatol. Palaeoecol. 370, 260–270. doi:10.1016/j.palaeo.2012.12.019
Nishikane, Y., Kaiho, K., Henderson, C. M., Takahashi, S., and Suzuki, N. (2014). Guadalupian-Lopingian conodont and carbon isotope stratigraphies of a deep chert sequence in Japan. Palaeogeogr. Palaeoclimatol. Palaeoecol. 403, 16–29. doi:10.1016/j.palaeo.2014.02.033
Olroyd, S. L., and Sidor, C. A. (2017). A review of the Guadalupian (middle Permian) global tetrapod fossil record. Earth Sci. Rev. 171, 583–597. doi:10.1016/j.earscirev.2017.07.001
Olroyd, S. L., Sidor, C. A., and Angielczyk, K. D. (2017). New materials of the enigmatic dicynodont Abajudon kaayai (Therapsida, Anomodontia) from the lower Madumabisa Mudstone Formation, middle Permian of Zambia. J. Vertebr. Paleontol. 37 (6), e1403442. doi:10.1080/02724634.2017.1403442
Olroyd, S. L., Sidor, C. A., and Angielczyk, K. D. (2018). New materials of the enigmatic dicynodont Abajudon kaayai (Therapsida, Anomodontia) from the lower Madumabisa Mudstone Formation, middle Permian of Zambia. J. Vertebr. Paleontol. 37, e1403442. doi:10.1080/02724634.2017.1403442
Paiva, F. (2016). Fluvial facies architecture and provenance history of the Abrahamskraal-Teekloof Formation transition (lower Beaufort Group) in the main Karoo Basin. PhD thesis. University of Cape Town, 98. http://hdl.handle.net/11427/20615
Percival, L. M. E., Ruhl, M., Hesselbo, S. P., Jenkyns, H. C., Mather, T. A., and Whiteside, J. H. (2017). Mercury evidence for pulsed volcanism during the end-Triassic mass extinction. Proc. Natl. Acad. Sci. U.S.A. 114 (30), 7929–7934. doi:10.1073/pnas.1705378114 |
Raup, D. M., and Sepkoski, J. J. (1982). Mass extinctions in the marine fossil record. Science 215 (4539), 1501–1503. doi:10.1126/science.215.4539.1501 |
Retallack, G. J., Metzger, C. A., Greaver, T., Jahren, A. H., Smith, R. M., and Sheldon, N. D. (2006). Middle-Late Permian mass extinction on land. GSA Bull. 118 (11-12), 1398–1411. doi:10.1130/b26011.1
Rey, K., Day, M. O., Amiot, R., Goedert, J., Lécuyer, C., Sealy, J., et al. (2018). Stable isotope record implicates aridification without warming during the late Capitanian mass extinction. Gondwana Res. 59, 1–8. doi:10.1016/j.gr.2018.02.017
Rey, K., Day, M. O., Amiot, R., Fourel, F., Luyt, J., Lécuyer, C., et al. (2020). Stable isotopes (δ18O and δ13C) give new perspective on the ecology and diet of Endothiodon bathystoma (Therapsida, Dicynodontia) from the late Permian of the South African Karoo Basin. Palaeogeogr. Palaeoclimatol. Palaeoecol. 556, 109882. doi:10.1016/j.palaeo.2020.109882
Romano, M., and Rubidge, B. (2019). First 3D reconstruction and volumetric body mass estimate of the tapinocephalid dinocephalian Tapinocaninus pamelae (Synapsida: Therapsida). Hist. Biol., 1–8. doi:10.1080/08912963.2019.1640219
Rossouw, P. J., and De Villiers, J. (1953). The geology of the Merweville area, Cape province: an explanation of sheet 198 (Merweville). Pretoria: Geological Survey of South Africa, 80.
Rubidge, B. S. (2005). 27th Du Toit Memorial Lecture: Re-uniting lost continents - fossil reptiles from the ancient Karoo and their wanderlust. S. Afr. J. Geol. 108 (1), 135–172. doi:10.2113/108.1.135
Rubidge, B. S., Erwin, D. H., Ramezani, J., Bowring, S. A., and de Klerk, W. J. (2013). High-precision temporal calibration of Late Permian vertebrate biostratigraphy: U-Pb zircon constraints from the Karoo Supergroup, South Africa. Geology 41 (3), 363–366. doi:10.1130/g33622.1
Schneider, J. W., Lucas, S. G., Scholze, F., Voigt, S., Marchetti, L., Klein, H., et al. (2020). Late paleozoic-early mesozoic continental biostratigraphy - links to the standard global chronostratigraphic scale. Palaeoworld 29 (2), 186–238. doi:10.1016/j.palwor.2019.09.001
Sennikov, A. G., and Golubev, V. K. (2017). Sequence of permian tetrapod faunas of Eastern Europe and the Permian-Triassic ecological crisis. Paleontol. J. 51 (6), 600–611. doi:10.1134/s0031030117060077
Shen, S.-Z., and Shi, G. R. (2009). Latest Guadalupian brachiopods from the Guadalupian/Lopingian boundary GSSP section at Penglaitan in Laibin, Guangxi, South China and implications for the timing of the pre-Lopingian crisis. Palaeoworld 18 (2–3), 152–161. doi:10.1016/j.palwor.2009.04.010
Sial, A. N., Chen, J., Lacerda, L. D., Frei, R., Tewari, V. C., Pandit, M. K., et al. (2016). Mercury enrichment and Hg isotopes in Cretaceous-Paleogene boundary successions: links to volcanism and palaeoenvironmental impacts. Cretaceous Res. 66, 60–81. doi:10.1016/j.cretres.2016.05.006
Sidor, C. A., Angielczyk, K. D., Smith, R. M. H., Goulding, A. K., Nesbitt, S. J., Peecook, B. R., et al. (2014). Tapinocephalids (Therapsida, Dinocephalia) from the Permian Madumabisa Mudstone Formation (lower Karoo, mid-Zambezi Basin) of southern Zambia. J. Vertebr. Paleontol. 34, 980–986. doi:10.1080/02724634.2013.826669
Signor, P. W., and Lipps, J. H. (1982). “Sampling bias, gradual extinction patterns, and catastrophes in the fossil record,” in Geological implications of impacts of large asteroids and comets on the Earth. Editors L. T. Silver, and P. H. Schultz (Boulder, CO: Geological Society of America Special Publication), Vol. 190, 291–296.
Simon, R. V., Sidor, C. A., Angielczyk, K. D., and Smith, R. M. H. (2010). First record of a tapinocephalid (Therapsida: Dinocephalia) from the Ruhuhu Formation (Songea Group) of southern Tanzania. J. Vertebr. Paleontol. 30 (4), 1289–1293. doi:10.1080/02724634.2010.483549
Smith, R. M. H. (1987). Morphology and depositional history of exhumed Permian point bars in the southwestern Karoo, South Africa. J. Sediment. Res. 57 (1), 19–29. doi:10.1306/212F8A8F-2B24-11D7-8648000102C1865D
Smith, R. M. H., and Botha-Brink, J. (2014). Anatomy of a mass extinction: sedimentological and taphonomic evidence for drought-induced die-offs at the Permo-Triassic boundary in the main Karoo Basin, South Africa. Palaeogeogr. Palaeoclimatol. Palaeoecol. 396, 99–118. doi:10.1016/j.palaeo.2014.01.002
Smith, R. M. H., and Keyser, A. W. (1995). “Biostratigraphy of the Tapinocephalus Assemblage Zone, 8‒12,” in Biostratigraphy of the Beaufort Group. Editor B. Rubidge (Pretoria, South Africa: SACS Biostratigraphic Series No. 1, Council for Geoscience), 46
Smith, R. M. H., Rubidge, B. S., Day, M. O., and Botha, J. (2020). Introduction to the tetrapod biozonation of the Karoo Supergroup. S. Afr. J. Geol. 123 (2), 131–140. doi:10.25131/sajg.123.0009
Stanley, S. M. (2016). Estimates of the magnitudes of major marine mass extinctions in Earth history. Proc. Natl. Acad. Sci. U.S.A. 113 (42), E6325–E6334. doi:10.1073/pnas.1613094113 |
Stanley, S. M., and Yang, X. (1994). A double mass extinction at the end of the Paleozoic Era. Science 266 (5189), 1340–1344. doi:10.1126/science.266.5189.1340 |
Stevens, L. G., Hilton, J., Bond, D. P. G., Glasspool, I. J., and Jardine, P. E. (2011). Radiation and extinction patterns in Permian floras from North China as indicators for environmental and climate change. J. Geol. Soc. 168 (2), 607–619. doi:10.1144/0016-76492010-042
Suchkova, Y. A., and Golubev, V. K. (2019a). A new primitive therocephalian (Theromorpha) from the Middle Permian of Eastern Europe. Paleontol. J. 53 (3), 305–314. doi:10.1134/s0031030119030158
Suchkova, Y. A., and Golubev, V. K. (2019b). A new Permian therocephalian (Therocephalia, Theromorpha) from the Sundyr Assemblage of Eastern Europe. Paleontol. J. 53 (4), 411–417. doi:10.1134/s0031030119040117
Sun, Y., Lai, X., Wignall, P. B., Widdowson, M., Ali, J. R., Jiang, H., et al. (2010). Dating the onset and nature of the Middle Permian Emeishan Large Igneous Province eruptions in SW China using conodont biostratigraphy and its bearing on mantle plume uplift models. Lithos 119 (1-2), 20–33. doi:10.1016/j.lithos.2010.05.012
Thibodeau, A. M., and Bergquist, B. A. (2017). Do mercury isotopes record the signature of massive volcanism in marine sedimentary records?. Geol. 45 (1), 95–96. doi:10.1130/focus012017.1
Tsuji, L. A., Müller, J., and Reisz, R. R. (2012). Anatomy of SGCFGTET Emeroleter levis and the phylogeny of the nycteroleter parareptiles. J. Vertebr. Paleontol. 32 (1), 45–67. doi:10.1080/02724634.2012.626004
Turner, B. R. (1985). Uranium mineralization in the Karoo basin, South Africa. Econ. Geol. 80 (2), 256–269. doi:10.2113/gsecongeo.80.2.256
Urbanek, A. (1993). Biotic crises in the history of Upper Silurian graptoloids: a Palaeobiological model. Hist. Biol. 7 (1), 29–50. doi:10.1080/10292389309380442
Van Den Brandt, M. J., Abdala, F., and Rubidge, B. S. (2020). Cranial morphology and phylogenetic relationships of the middle Permian pareiasaur Embrithosaurus schwarzi Watson, 1914 from the Karoo Basin of South Africa. Zool. J. Linnean Soc. doi:10.1093/zoolinne-an/zlz064
Viglietti, P. A. (2020). Biostratigraphy of the Daptocephalus Assemblage Zone (Beaufort Group, Karoo Supergroup), South Africa. S. Afr. J. Geol. 123 (2), 191–206. doi:10.25131/sajg.123.0014
Ward, P. D., Botha, J., Buick, R., De Kock, M. O., Erwin, D. H., Garrison, G. H., et al. (2005). Abrupt and gradual extinction among Late Permian land vertebrates in the Karoo Basin, South Africa. Science 307 (5710), 709–714. doi:10.1126/science.1107068 |
Watson, D. M. S. (1914). II.-The zones of the Beaufort Beds of the Karroo System in South Africa. Geol. Mag. New Ser. (Decade IV) 1 (5), 203–208. doi:10.1017/s001675680019675x
Wei, H., Baima, Q., Qiu, Z., and Dai, C. (2018). Carbon isotope perturbations and faunal changeovers during the Guadalupian mass extinction in the middle Yangtze Platform, South China. Geol. Mag. 155 (8), 1667–1683. doi:10.1017/s0016756817000462
Wignall, P. B., Sun, Y., Bond, D. P., Izon, G., Newton, R. J., Védrine, S., et al. (2009). Volcanism, mass extinction, and carbon isotope fluctuations in the Middle Permian of China. Science 324 (5931), 1179–1182. doi:10.1126/science.1171956 |
Wilson, A., Flint, S., Payenberg, T., Tohver, E., and Lanci, L. (2014). Architectural styles and sedimentology of the fluvial lower Beaufort Group, Karoo Basin, South Africa. J. Sediment. Res. 84 (4), 326–348. doi:10.2110/jsr.2014.28
Zhang, B., Yao, S., Wignall, P. B., Hu, W., Liu, B., and Ren, Y. (2019). New timing and geochemical constraints on the Capitanian (Middle Permian) extinction and environmental changes in deep-water settings: evidence from the Lower Yangtze region of South China. J. Geol. Soc. 176 (3), 588–608. doi:10.1144/jgs2018-137
Zhang, B., Yao, S., Mills, B. J. W., Wignall, P. B., Hu, W., Liu, B., et al. (2020). Middle Permian organic carbon isotope stratigraphy and the origin of the Kamura Event. Gondwana Res. 79, 217–232. doi:10.1016/j.gr.2019.09.013
Keywords: Tapinocephalus Assemblage Zone, Endothiodon Assemblage Zone, Permian, Abrahamskraal, Teekloof, dinocephalian
Citation: Day MO and Rubidge BS (2021) The Late Capitanian Mass Extinction of Terrestrial Vertebrates in the Karoo Basin of South Africa. Front. Earths Sci. 9:631198. doi: 10.3389/feart.2021.631198
Received: 19 November 2020; Accepted: 07 January 2021;
Published: 18 February 2021.
Edited by:
Spencer G. Lucas, New Mexico Museum of Natural History and Science, United StatesReviewed by:
Christian Sidor, University of Washington, United StatesSean P. Modesto, Cape Breton University, Canada
Cesar Schultz, Federal University of Rio Grande do Sul, Brazil
Copyright © 2021 Day and Rubidge. This is an open-access article distributed under the terms of the Creative Commons Attribution License (CC BY). The use, distribution or reproduction in other forums is permitted, provided the original author(s) and the copyright owner(s) are credited and that the original publication in this journal is cited, in accordance with accepted academic practice. No use, distribution or reproduction is permitted which does not comply with these terms.
*Correspondence: Michael O. Day, michael.day@nhm.ac.uk