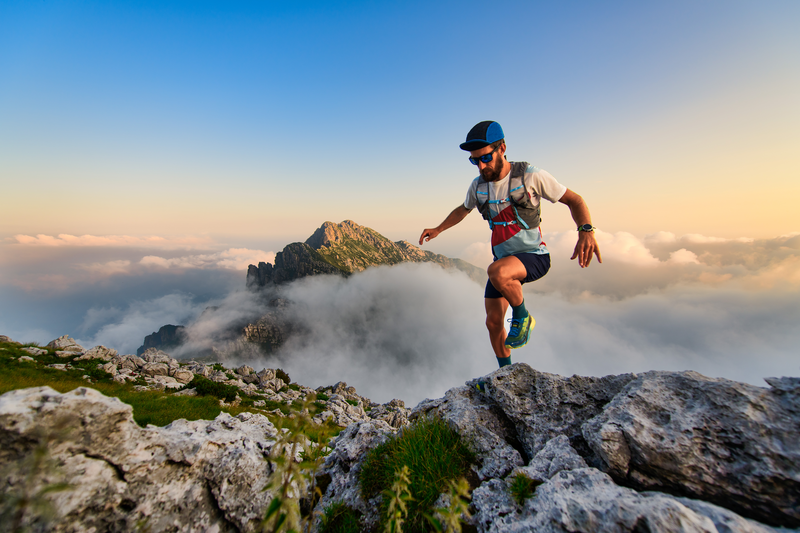
94% of researchers rate our articles as excellent or good
Learn more about the work of our research integrity team to safeguard the quality of each article we publish.
Find out more
ORIGINAL RESEARCH article
Front. Earth Sci. , 14 April 2021
Sec. Hydrosphere
Volume 9 - 2021 | https://doi.org/10.3389/feart.2021.554588
This article is part of the Research Topic Smart Approaches to Predict Urban Flooding: Current Advances and Challenges View all 13 articles
Stormwater runoff is identified as urban nonpoint source pollution that increasingly introduces contaminants to urban water bodies and impedes sustainable development. The pollution load of runoff varies due to the interception of different land cover types during the urban hydrological process. During the rainy season (June–August) in 2018, five different underlying surfaces (green roof, parking lot, urban road, parkway, and grassland) were selected in Guangzhou to analyze the migration characteristics of stormwater runoff pollutants. The concentrations of heavy metals, such as chromium (Cr), cadmium (Cd), lead (Pb), and mercury (Hg), as well as total nitrogen (TN), total phosphorus (TP), and polycyclic aromatic hydrocarbons (PAHs) were collected and analyzed on different underlying surfaces with the rainfall data at the beginning of a stormwater runoff event. The results showed that PAHs, heavy metals, and TP existed mainly in the form of particles; nitrogen was mainly present as ammonia and nitrate; and the TN, TP, PAHs, and heavy metal were significantly different in the stormwater runoff on each underlying surface. The pollutant concentration in urban road runoff was the highest, accounting for 40–70% of the total pollutant concentration in the stormwater runoff, and the pollutant concentration in green roof runoff was the lowest, accounting for 10–40% of the total pollutant concentration in the runoff. An obvious effect of initial rainfall erosion was observed during stormwater runoff from urban roads and parking lots, and the scouring effect on grasslands and green roofs was mainly due to the many factors affecting the underlying surface during the middle and late stages. The rates of reduction of heavy metals were the most significant. The effect of water purification was positively correlated with stormwater runoff duration. The rates of reduction of TN, heavy metals (Cr, Cd, and Hg), and PAHs in the grassland areas were 35.20 ± 26.28, 0.24 ± 10.13, 71.77 ± 10.97,32.62 ± 110.48, and 41.30 ± 8.78%, respectively. This study could provide a theoretical basis for preventing and managing pollutants in urban stormwater runoff.
Population growth, industrial development, land use, waste emissions, and automobile exhaust have significantly increased the urban environmental load with the development of urbanization (Gimeno et al., 2014). Environmental problems have become a major issue that we must face, and urban water pollution is serious (Xu and Haung, 2004; Wu, 2018; Iuliana et al., 2020; Zeng et al., 2020). Urban stormwater has been identified as a major nonpoint source of pollution containing heavy metals, polycyclic aromatic hydrocarbons (PAHs), and nutrients (nitrogen and phosphorus) that cause urban flooding, accelerate the migration of pollutants, and introduce secondary pollution into water and have toxic effects on human health. Urban underlying surfaces conditions are key drivers of urban runoff quality. Numerous studies on stormwater management strategies have focused on the characteristics of stormwater runoff occurring on different urban underlying surfaces (Gilbert and clausen, 2006; Ou et al., 2011; Ouyang et al., 2010), including the initial runoff erosion effect (Soller et al., 2005; Ouyang et al., 2010; Zeng et al., 2019) and runoff models and other aspects (Deletic, 1998; Yu et al., 2016; Andrzejc et al., 2020).
The US Environment Protection (EPA) reported that approximately 90% of surface pollutants are carried by the first 2.5–4 cm of rainfall (Shang and Sun 2019), and there are significant cumulative toxic effects of various stormwater contaminants that threaten public health. Therefore, mitigating contaminants from stormwater runoff has attracted attention. Stormwater can be managed by green infrastructures, such as green roofs, wells, or bioswales (Walaszek et al., 2018). Previous studies have concluded that the initial scour of different underlying surfaces was different, the initial scour of pavement is less than that of roofs, and the pollutant characteristics of runoff from roofs and pavement are different. The degree of pollution in pavement runoff is higher than that from roofs. Different types of land cover generally have different functional areas and pollution sources (Buytaert et al., 2014). However, the effects of different land cover types on runoff pollution in the same environment are not well understood.
Most scholars believe that the sources of water pollution can be divided into point sources and areal sources (Diebel et al., 2009; Shi, 2011). As national and local governments have paid more attention to the control of pointsource, pollution has basically been controlled. (Guo et al., 2006). However, the features of areal sources, such as fuzzy temporal and spatial distributions and complex influencing factors, are difficult to control, and areal source pollution is relatively serious (Donigian and Huber, 1991; Wang and wang, 2002). Urban areal source pollution, also known as urban stormwater runoff pollution, refers to precipitation and runoff in different cities, such as industrial areas, residential areas (Lusk et al., 2020), commercial areas, roofs, parking lots, green belts, and roads. The process of leaching and scouring of the pollutants deposited on the underlying surface allows the pollutants to be collected in the runoff and discharged into the receiving body of water, which pollutes the aquatic environment (Camorani et al., 2005; Chang et al., 2006; Huang and Nie, 2012; Ren et al., 2013).
Urban areal source pollution includes both impermeable ground leaching and erosion from parking lots, roofs, and roads, as well as permeable ground leaching of green belts, grasslands, and other permeable grounds (Ou et al., 2011; Bao, 2016). Areal source pollution caused by erosion includes a wide range of pollutant sources and complex components (Gupta and Saul, 1996; Sansalone and Cristina, 2004; Jair et al., 2020). One of the main effects of urban green infrastructure on runoff water quality is the overall reduction in runoff quantity, thereby reducing the quantity of pollutants reaching urban waterways and the sewer infrastructure (Gooré Bi et al., 2015). Research on urban rainfall and runoff pollution mainly includes the following aspects: the spatial and temporal distribution of urban stormwater runoff pollutants (Brezonik and Stadlemann, 2002; Jeffrey et al., 2005; Geonha et al., 2007); the pollution characteristics of stormwater runoff produced by different underlying surfaces such as urban asphalt pavement (Ellis, 2000) and green pavement (Huang et al., 2006); the controlling effect of urban greening on pollutants (Ren et al., 2005; Chen et al., 2009; Ren et al., 2020; Xu et al., 2020); and the erosive effect of stormwater runoff and the development on urban rainfall-runoff models (Murakami et al., 2008; Ouyang et al., 2010;; Jaiswal et al., 2020). Among them, studying the characteristics of urban rainfall and runoff products is the basis for in-depth research on urban areal source pollution.
Guangzhou is located in the south subtropical zone of China, with sufficient light and heat resources and abundant rainfall. This region has the fastest urbanization and economic developmental rate in China. The Guangdong-Hong Kong-Macao Greater Bay Area, where Guangzhou is located, is one of China’s three regional economic centers and one of the most dynamic economic zones in China and the world (Ye et al., 2017). The surface vegetation structure in Guangzhou is undergoing major changes with economic development, rapid population growth, and construction leading to local environmental disturbances in land development (Zhu et al., 2016). The status of hydrology and water resources is also becoming increasingly severe. At the same time, chemical, power, mineral, printing, and other industries are developing rapidly in Guangzhou, with many factories and enterprises beginning production. Rainfall carries pollutants into the river system and pollutes the water (Gong et al., 2017). Therefore, comprehensive water pollution treatment is necessary in Guangzhou to control the pollutant output of precipitation, particularly the transport of pollutants on different underlying surfaces.
Low Impact Development (LID) had been imposed for increasing rainwater infiltration into the soil or vegetation in urban areas in Guangzhou (Zeng et al., 2019). LID infrastructure was widely used, mainly by ecological tree pools, green roofs, rain gardens, grasslands, and permeable pavements (Tang et al., 2021). Many of the traditional roofs and green spaces were transformed based on the corresponding LID designing (Zeng et al., 2019). The main aim of LID was to reduce initial rainwater pollution caused by the quick wash-off of pollutants accumulated on the watershed surfaces. Deep tunnel drainage systems had been constructed at the final step among the measures that reduced combined sewer overflow (Wu et al., 2016). To investigate the effects of LID practices on purifying water quality, it is quite important to study the characteristics of pollutant concentrations in urban rainfall-runoff from different surfaces.
The objectives of this study were to develop an accurate understanding of pollutants loads of urban surface types and to investigate the pollutant hydrologic dynamics processes that occur during rain events. The study encompasses untreated stormwater runoff quality from five surfaces during three heavy rain events in different catchments within LuHu, Guangzhou. This research focused on heavy metals and PAHs primarily originating from automobile exhaust gases, which are the most common toxic chemical compounds present in urban stormwater. The filtration and storage efficiency of stormwater runoff pollutants under the different underlying surfaces were estimated based on the temporal and spatial patterns of the output of stormwater runoff pollution. The results elaborated the water quality characteristic for surface-specific runoff within a single climatic location to ensure an appropriate treatment solution is selected in urban water management.
This study was carried out in Guangzhou, located in southern China in central and southern Guangdong Province and on the north-central border of the Pearl River Delta. The study area included the confluence of the Xijiang, Beijiang, and Dongjiang Rivers. The southern subtropical monsoon climate is characterized by warm and rainy conditions, sufficient light and heat, long summers, and short frost periods. With an annual average temperature of 21.9°C, Guangzhou is one of the largest cities in China with the smallest annual average temperature difference. The hottest month of the year is July, with an average monthly temperature of 28.7°C. The coldest month is January, with an average monthly temperature of 13.5°C. The average relative humidity is 77%, and the annual rainfall is approximately 1,800 mm, which is mostly concentrated in April–September. Rainfall during the rainy season accounts for more than 70% of the annual rainfall.
The sampling area was a unit compound (Guangzhou Institute of Forestry and Landscape Architecture, GIFLA) located in LuHu, Guangzhou urban area (23°9′46.25″N, 113°17′22.4″E), also in the Guangzhou National Field Station for Scientific Observation and Research of Urban Ecosystem established in 2017 (Figure 1). It covers a total area of 8.9 × 104 m2 and is primarily used for green space with some land set aside for construction and parkway. The drainage system uses a rainwater and sewage separation system.
FIGURE 1. Sampling site locations for the three sampling programs. (A) Urban road, (B) parking lot, (C) grassland, (D) green roof, (E) parkway, (F) CK, (G) weather station, (H) Guangzhou urban field station.
Five underlying surfaces’ runoff samples were collected from the grassland catchment, urban road water collection wellhead, parkway water collection wellhead, parking lot drain, green roof rainwater vertical drain, and precipitation (as control, CK) during three rainfall events from June to August in 2018. Water collection wellhead is formed by a rainspout connecting urban stormwater drainage system when runoff occurs on the road. These sampling points were distributed within a range of 100 m and exposed to the same single climatic conditions.
For each effective stormwater runoff event, surface runoff samples were collected manually with a time interval of 5 min, that is, at 5, 10, 15, 20, 25, 30, 35, and 40 min from the start of runoff. Each water sampling was repeated three times and water samples were stored in 1 L brown glass bottles. The presence and characteristics of the first flush effect were detected by samples collected at 5 and 10 min after the first runoff outflow. The second stage of samples is followed by the first stage. All sampling was completed after approximately 45 min of rainfall event. All the samples were held at 4°C and delivered to the laboratory within 24 h of collection (HJ 494, 2009).
Rainfall data were recorded by a Campbell weather station at the neighboring Guangzhou National Field Station for Scientific Observation and Research of Urban Ecosystem at a frequency of 1 min. The annual rainfall is 1,723.9 mm, of which 21 rainfall events greater than 50 mm occurred within 24 h. However, for the sake of ensuring the safety of the experimental process, water samples were collected during the daytime, without lightning strikes, and the required rainfall time exceeded 1 h. Three effective stormwater events were finally successfully sampled on 1st June and 2nd and 28th August in 2018 (Table 1). The total rainfall was between 34.7 and 102.5 mm, the rainfall duration was from 90 to 420 min, the maximum rainfall intensity was 1.4–2.0 mm/min, the average rainfall intensity was from 0.355 to 0.486 mm/min, and the number of antecedent dry days was more than 24 h.
Seven water quality indexes were selected for analysis, including TN, TP, heavy metals involving Cr, Cd, Hg, and Pb, and 16 PAHs, including naphthalene (NAP), acenaphthene (ACE), acenaphthylene (ACY), fluorene (FLU), phenanthrene (PHE), anthracene (ANT), fluoranthene (FLa), pyrene (Pyr), benzo[a]anthracene (BaA), chrysene (Chr), benzo[b]fluoranthene (BbF), benzo[k]fluoranthene (BkF), benzo[a]pyrene (BaP), indeno[1, 2, 3-cd]pyrene (IcdP), dibenzo[a, h]anthracene (DahA), and benzo[g, h, i]perylene (BghiP) (Environmental Protection Agency and USEPA, 1983). Water quality measurements followed the standard methods (environmental quality standards for surface water, GB3838-2002) specified by the national standards of the P.R.C.
PAHs were determined by the EPA3510C-1996 and EPA8270D-2007 methods, and gas chromatography-mass spectrometry (GM-MS, QP2010plus) was used for the analysis with the selective ion detection method (SIM) to measure the samples. A DB5-MS GC-MS column (30 m × 0.25 mm × 0.25 μm) was used.
Pollutant loading was estimated using the event mean concentration (EMC), which is used to represent the average concentration of a pollutant discharged during an entire stormwater event (Chow et al., 2013). In this study, the EMC was expressed as follows:
where M is the total content of a certain pollutant (g) during the entire rainfall event; V is the corresponding total net flow (m3); t is total runoff time (min); Ct is the pollutant content that changes with time (μg/L); Qt is the runoff rate (m3/min) that changes with time; and △t is the discontinuous time interval. Because the continuous concentration data of a pollutant cannot be monitored with actual measurements, the concentration of the pollutant at a certain point in the actual calculation process was used to replace the concentration in the time period.
As shown in Figure 2, the heavy metal Cd concentration at the beginning of the stormwater runoff ranked as the parking lot, urban road, grassland, parkway, and green roof. The concentrations of grassland and green roof were higher than those of other surface runoff at the end of the stormwater lasting 40 min. This result shows that the heavy metal Cd content collected by stormwater runoff on the impermeable surface was higher. According to the national environmental quality standards for surface water (The Ministry of Environmental Protection of the People's Republic of China, 2002), the Cd concentration in the stormwater runoff from 40 min rainfall event on urban roads and parkways is beyond the Class II standards. During the 40 min rainfall event, the average Cd concentrations of roads, parkways, and green roofs met the minimum emission standard V.
FIGURE 2. The change in the heavy metal average concentration index over time. The smoothing blue curve was obtained by loess method, and the corresponding color shadow part was its 95% confidence interval.
The higher Cr concentration was also detected on the urban road first flush and the lower in grassland. Unexpectedly, high levels of Cr were produced on the green roof runoff at the end of the storm lasting 40 min. The green roof runoff produced Cr concentrations up to 28.1 μg/L. During the 40 min rainfall, the average Cr concentrations on the grasslands, urban roads, parkways, and green roofs met the minimum emission standard V.
The Hg concentrations in the parking lot and parkway were very low later during the rain event and could not be detected. During the 40 min rainfall, the average Hg concentrations in grassland, urban road, parkway, and green roof runoff met the Class I emission standards.
The Pb concentration was substantially higher in the urban road runoff than other underlying surfaces at the beginning of the rain event. The higher concentrations were seen from higher trafficked urban roads in comparison with the lower trafficked roads. The average Pb concentration in the grassland, urban road, parkway, and green roof runoff reached Class I emission standards. The Pb concentrations due to the natural crude oil sources were excessive in the road and grassland runoff compared with that of the other land cover types.
During the 40 min stormwater runoff, the average concentration of TN ranged from 1160.8 μg/L in the parkways runoff to 4989.6 μg/L in the urban road runoff (Figure 3). The average TN concentration in grassland, urban road, parkway, and green roof runoff exceeded China's surface water Class V standard in the environmental quality standards. Among them, the concentration on the urban road reached a maximum of 10,460 μg/L and exceeded the Class V standard by four times during the 10 min stormwater runoff, but the concentration decreased by 86.63% after 35 min, which is within the Class IV standard.
The average concentration of TP in the urban road and green roof runoff fluctuated with stormwater duration. TP concentration in green roof runoff during the whole rainfall process was much greater than that in the parking lots, grasslands, and parkways (Figure3). TP concentration in green roofs was 40.78 times that on parking lots. At the beginning of the stormwater runoff, TP concentration was highest in the runoff water samples collected from urban road, followed by green roof, and the lowest in parking lot. TP concentration was highest in the green roof at the end of the stormwater runoff lasting 40 min. TP concentration almost met the Class IV standard except in the green roof runoff.
The mean concentration of PAHs ranged from 0.67 μg/L in the grassland runoff to 1.88 μg/L in the urban road runoff (Figure 4). The urban road has a peak of 4.039 μg/L at 10 min of stormwater runoff and a minimum concentration of 0.927 μg/L at 30 min. The ranking of the concentrations of the PAHs at the beginning of the stormwater runoff was parking lot, followed by urban road, parkway, green roof, and grassland. The PAH concentration was the highest in the parkway and the lowest in grassland runoff at the end of the stormwater runoff lasting 40 min.
FIGURE 4. PAH average concentration index changes over time in stormwater and runoff events. The smoothing blue curve was obtained by loess method, and the corresponding color shadow part was its 95% confidence interval.
Urban land cover type and spatial pattern are the key factors affecting urban stormwater runoff contaminants. The average concentration index of pollutants in the runoff events changed over time. The contribution rates of the five types of underlying pollutants to the same pollutant and the changes in pollutant concentrations with time are quite different, but the overall initial pollutant concentration is relatively high after the erosion effect, and the concentration later decreased. Urban road runoff produced higher heavy metal concentrations (Cd, Pb, and Hg) than any other sampled surface except the higher Cr concentrations in green roof during the stormwater runoff process.
The smoothing blue curve was obtained by the loess method, and the corresponding color shadow part was its 95% confidence interval.
Asphalt pavement has higher pollution intensity, among which the main urban roads are the most polluted. The EMCs of TN and TP increased up to 4988.3 ± 249.3 and 318.2 ± 43.2 μg/L, respectively (Table 2). The TP concentration exceeds 1.5 times higher than the Class V standard in China’s environmental quality standards for surface water. TN of the parking lot and green roof is lower than that of the urban road but still does not meet the Class V standard. The concentrations of Hg and Cd were mostly on the order of 0.002∼0.005 and 0.028∼0.115 μg/L. The EMC values of the heavy metals were not greater than the standard V.
The pollutant EMC values of the various underlying surfaces were different. The higher loads of TN, Pb, and PAHs were detected in urban road runoff. The higher loads of TP and Cr were tested in green roof runoff (Table 2). Asphalt pavement was most polluted by TN and TP, the most prominent heavy metals were Cr and Pb, and the EMC values of Cd and Hg pollutants were lower than the concentrations of PAHs.
The same pollutant showed differences on the various underlying surfaces. The TN, Hg, Pb, and PAHs pollution loads were higher in urban road runoff. Heavy metals and PAHs can easily enter surface runoff and drainage system and pollute urban water during rainfall events. The highest TP and Cr pollution loads occurred in the green roof runoff (Table 2).
The results of the principal component analysis of the pollutants in the storm runoff are shown in Table 3. The results divided the pollutants into three main components. The cumulative contribution rate of the first and second principal components reached 80.118%, which accounted for the largest proportion. These two main components contained more than 80% of the information content of the entire pollutant index, which met the requirement of main component extraction.
The pollutants in the Guangzhou stormwater runoff were divided into two groups. The first group included Pb, Cd, Hg, and PAHs which had a large positive correlation with the first principal component, TN had a weak positive correlation with the first principal component, and TP and Cr and the first principal component were weakly negatively correlated, so principal component 1 mainly represented the principal component of heavy metal pollution in the stormwater runoff. The second group of TN, TP, and Cr had a large positive correlation with the second principal component, while Pb, Cd, Hg, and PAHs were poorly correlated. Principal component 2 was the principal component that described the nutrients in Guangzhou stormwater runoff. The main component scores of the seven pollutants in the storm runoff were Cd (0.237)> Pb (0.226)> PAHs (0.213)> Hg (0.205)> Cr (−0.187)> TP (-0.167)> TN (0.163), suggesting that Pb, Cd, Hg, and the PAHs may have originated from the same pollution source. TN, TP, and Cr came from the same pollution source and played an important role in the concentrations of the stormwater runoff pollutants.
Table 4 shows the differences in the reduced rate of pollutants in stormwater runoff between the different underlying surfaces. When the reduction rate is negative, the underlying surface plays a “sink” role in releasing pollutants; otherwise, the surface plays a “source” role.
TABLE 4. The rates of decrease in the seven pollutants in stormwater runoff on the underlying surfaces.
The grassland played a role in purifying the seven kinds of pollutants, except TP and Pb, and its rank for the rate of reduction was Cd > PAHs > TN > Hg > Cr (Table 4). After the rain event, the rate of increase in TP was greater than that of Pb in the grassland runoff; the urban road was the main “source” for pollutants, and the ranking for the rate of increase in runoff pollutants was TP > Pb > TN > PAHs > Cr. The rate of reduction in Cd was greater than that of Hg. The parkway had a purifying effect on the seven pollutants, except TP. The ranking in the rates of reduction was Hg > Cd > Pb > TN > Cr > PAHs. The rate of increase in TP was 67.72%. The green roof played a role in purifying the heavy metals and PAHs, and the ranking of the rate of decrease was Cd > Hg > Pb > PAHs. The green roof increased nutrients in the stormwater runoff; in particular, TP increased 4070.58%.
The water quality of urban stormwater runoff varies by orders of magnitude between different land surfaces, and stormwater monitoring is important to design reasonable treatments to protect urban bodies of water. Heavy metals, PAHs, and pesticides were the main pollutants of concern in stormwater runoff and of concern in the urban catchment. However, Pb, Cr, Cd, and Hg were detected in all stormwater runoff sampling points, and their concentrations met the EPA worst-case-based water quality criteria. The Hg concentrations in urban stormwater runoff were too low to be detected. These results indicate that heavy metals in urban residential areas and road stormwater runoff are present in nontoxic forms to aquatic life in urban bodies of water.
Urban stormwater runoff contains elevated concentrations of various nutrients, such as nitrogen and phosphorus compounds, which can lead to eutrophication of urban water. It has been reported that the algae available P in urban stormwater runoff, grassland, and green roof areas is derived from the leaching of tree leaves and flowers (Lee and Jones-Lee 2005). The pollution from the urban road was the most serious. The TN and TP concentrations exceeded the Class V standard stipulated by the national surface water environmental quality standard by 1.5 times. The TN concentrations in green roof and parking lot runoff were lower than that in the urban road runoff but still failed to meet the standard V.
PAHs are adsorbed on particles with small aerodynamic diameters in the molecular state in the atmosphere, and some fall to the ground or water surfaces in raindrops. Stormwater runoff has become the main source of urban nonpoint source pollution. PAHs are persistent toxic organic pollutants of potential concern in urban stormwater runoff (Pilcher et al., 2018). China stipulates that the discharge standard for benzo[a]pyrene (BaP) in sewage is 30 ng/L (GB 18918-2002), but no regulations exist for discharge of other monomers or total PAHs. The BaP geometric means for the grassland, main road, parking lot, green roof, and parkway were 11.69, 339.57, 253.04, 99.75, and 237.61 ng/L, respectively. These results show that the grassland runoff met the national emission standard. Urban roads, parking lots, and parkways exceeded the standard by 11.32, 8.43, and 7.92 times, respectively, which should be taken seriously by relevant departments.
The feature ratio method is a commonly used method to analyze the source of PAHs. The principle is to judge the main source according to the ratio of the PAH concentrations of each isomer, and it is mostly used for qualitative analysis. The feature ratio method often uses the Fla/(Fla + Pyr), BaA/(BaA + Chr), and IcdP/(IcdP + BghiP) ratios to explain the possible sources of PAHs (Wu et al., 2019) (Table 5). We calculated the characteristic ratios of PAHs in the different underlying surfaces (Table 6), to show that the typical PAHs in Guangzhou mainly originated from coal/biomass fuel combustion and the petroleum sources originated from PAHs in the grassland, petroleum combustion source, and coal/biomass fuel combustion.
This study found that TN, Cd, Hg, Pb, and the PAHs exhibited first flush effects in the urban road stormwater runoff, while the P elements were adsorbed on the surface by dry sedimentation. Urban road surfaces are important impervious surfaces and an essential platform for PAHs and heavy metal ions during antecedent dry days (Ma et al., 2017). When stormwater runoff was generated, PAHs and heavy metals were washed away and dissolved. After the TP concentration increased, it remained flat, and Cr and TP changed in similar ways. The green roof Cr and TP concentrations exhibited the same pattern as that of the urban road runoff, and the other pollutants decreased-increased and then decreased again.
The pollutant concentrations after filtration through the soil and vegetation decrease, the soil and vegetation pollutants leach into the runoff, the concentration increases, and then the pollutant concentrations decrease due to dissolution by stormwater runoff. The TP, Cd, and Hg concentrations in the grassland runoff suddenly increased, mainly because grassland absorbs each element into the soil through dry deposition, and after long-term runoff leaching, each element reenter the water, causing secondary pollution (Wu, 2018). There were obvious initial erosive effects on the urban road and parking lot, whereas the grassland and green roof mitigated runoff. The initial erosion effects were not obvious on residential areas and roads, which agrees with the results of Huang and Nie (2012).
The underlying surfaces of parkways and urban roads are asphalt, but the pollutant EMC value of the parkways runoff was much lower than that of urban roads, mainly because litter and dust are cleaned up on parkways, and the roads are washed. When pollutants are adsorbed on dust and ground, they enter other areas. Thus, the total amount of pollutants can be effectively controlled by urban environmental sanitation, intercepting, precipitating, and filtering stormwater runoff, which is similar to the report by Ren et al. (2005).
The TN and TP EMC values of the green roof were much larger than those of the other underlying surfaces and had also been detected in Shengzhen (Tang et al., 2021). This result may be attributed to the fact that the artificial application of compound fertilizers causes an abnormal increase in the concentrations of TN and TP in the runoff. Especially during storm events, stormwater runoff transports massive amounts of P from urban greening soil to the surrounding water systems. The Cr content also increased, indicating that the green roof substrate may have a certain concentration of Cr. Their green roof design and maintenance may neglect nutrient pollution.
The parking lot had a high TN content, mainly because the exhaust gas of motor vehicles contains a large amount of incomplete combustion products and combustion reaction intermediate products such as NOX. After a rain event, NOX dissolves in the water resulting in high TN content (Ren et al., 2013).
The five underlying surfaces had different effects on reducing/increasing pollutants. Among them, the rates of reduction by grassland and green roof were higher, and the reduction in heavy metals was the most significant. Grassland and green roof play a blocking role and reduce rain intensity so that the soil structure is not damaged. When rainwater enters the soil, some pollutants are adsorbed on the soil, the nutrient elements are absorbed by plants at a later stage, and most of the heavy metals form complexes. The five underlying surfaces all played roles in reducing Cd concentrations because Cd is a water-soluble heavy metal, which is leached in large amounts during the stormwater runoff (Chen and Duan, 2013). The different underlying surfaces in cities intercept the rainfall at different rates and change the pollution load of the runoff.
Urban surface runoff emissions affect the quality of the receiving water, and heavy metals and PAHs are the main pollutants in urban runoff pollution and play a major toxic role (Zhao et al., 2015). Many nonpoint sources of heavy metals are detectable in the urban environment, particularly the traffic pollution caused by motor vehicles. The heavy metal pollution load is detected in the main roads and parking lots runoff (Yu et al., 2016). PAHs adhere to the surface of particulate matter, and it becomes the main persistent organic pollutant in the urban water environment as rainwater enters the surface runoff, which is mainly caused by asphalt roads and gasoline combustion (Chen and Adams, 2006). Therefore, PAHs and heavy metal pollution loads are high in urban road stormwater runoff and become an important source of urban water pollution. Strengthening the diversion of rain and sewage urban runoff is an important way to alleviate urban water environmental pollution.
The LID design in Guangzhou was required to develop stormwater runoff water pollution control programs to control pollution to the maximum extent practicable using the best ecologically designed stormwater management practices. Ponds, grassy swales, etc., cannot be considered sufficient to treat urban stormwater runoff to achieve compliance with water quality standards.
This study had some limitations. The rainfall spatial heterogeneity in Guangzhou is high, industrial development in each region is different, and the composition of the rainfall pollutants differed. The sampling point for this study was in Baiyun District, so the sampling range was relatively small. In the future, sampling points should be set up in each administrative area to expand the scope of the study. For the variability in accumulative and wash effect in the hydrological processes, we should make it accurate that the flush amount of pollutants is only a partial indication of the buildup. However, the relationship was far too complex to ignore. Sampling points can be set up in each administrative area to expand the scope in future study. The characteristics of heavy rain in Guangzhou are that the rain duration is short and the rain intensity is strong. Therefore, the research results represent the load of pollutants due to short-term heavy rainfall. On the other hand, pollutants cannot be tracked for long-term rainfall loads, and there was uncertainty associated with the temporal resolution of rainfall. However, the influence of rainfall intensity and runoff cannot be neglected. More factors can be considered in future including the impacts from land use in each catchment, dry deposition pollutant concentration, and the seasonal variations of rainfall. As basic research, we should also strengthen the research on the impact of surface runoff on urban water bodies and provide a theoretical and practical basis for risk assessment by specific pollutants in urban surface runoff.
In this study, the stormwater pollution mitigation performance of five urban underlying surfaces was quantitatively characterized with natural stormwater runoff event-based field monitoring. Some key findings are in the following:
1) The first flush effect of impermeable pavement system was most prone, while urban grasslands and green roofs reduced the pollutions because the first flush contributed to rainwater purification.
2) The pollutant abatement of green roofs is not significant or even storage effects. The substrate material, nutrient pollution, and dry deposition cannot be neglected in green roof and green spaces design.
3) The grassland purified the seven pollutants, except TP and Pb. The urban road was the main “source” of pollutants. The parking lot had a purifying effect on TP, Cd, Hg, and Pb and the parkway had a purifying effect on the seven pollutants except TP, whereas the green roof purified the heavy metals and PAHs.
4) The preliminary treatment of stormwater runoff should be strengthened due to the integration of ecological concerns and urban green infrastructures, such as green spaces, green roofs, vertical greening, grasslands, and forestry.
The original contributions presented in the study are included in the article/Supplementary Material; further inquiries can be directed to the corresponding author.
YP, YG, YT, and CZ conceptualized the study and were responsible for resources; YP, ZL, YG, and YX were responsible for methodology; YQ and QW were responsible for software; YP, YG, LR, SD and CZ validated the study, prepared the original draft, reviewed and edited the manuscript, and supervised the study; WL, YQ, and JL performed formal analysis; YP, ZL, YG, YX, YQ, and QW investigated the study; YP, YG, CZ, ZL, YT, and YX were responsible for data curation; LR, SD, XL, and CZ were responsible for visualization; YP and CZ involved in project administration.
This research was supported by the Guangdong Natural Science Foundation Project (2019A1515011627), National Natural Science Foundation of China (31660233; 31770492), and the project of Guangdong provincial innovation platform of forestry science and technology “Construction of network platform for forestry and ecological monitoring” (No. 2020-KYXM-09). The authors acknowledge the financial special project of Guangzhou City in 2020: the long-term scientific research base operation fee project, Guangzhou urban ecosystem national field station, for our support.
The authors declare that the research was conducted in the absence of any commercial or financial relationships that could be construed as a potential conflict of interest.
Andrzej, W., Devendra, A., Peter, C., Dan, M., and Sudhanshu, P. (2020). Assessment of storm direct runoff and peak flow rates using improved SCS‐CN models for selected forested watersheds in the Southeastern United States. J. Hydrol. Reg. Stud. 27. doi:10.1016/j.ejrh.2019.100645
Bao, X. (2016). Characteristics analysis and load calculation of urban storm runoff pollution for harbin city example. Harbin, China: Harbin Normal University.
Brezonik, P. L., and Stadelmann, T. H. (2002). Analysis and predictive models of stormwater runoff volumes, loads, and pollutant concentrations from watersheds in the Twin Cities metropolitan area, Minnesota, USA. Water Res. 36 (7), 1743–1757. doi:10.1016/s0043-1354(01)00375-x
Buytaert, W., Zulkafli, Z., Grainger, S., and Acosta, L., Alemie Alemie, T. C., Bastiaensen, J., et al. (2014). Citizen science in hydrology and water resources: opportunities for knowledge generation, ecosystem service management, and sustainable development. Front. Earth Sci. 2, 26. doi:10.3389/feart.2014.00026
Camorani, G., Castellarin, A., and Brath, A. (2005). Effects of landuse changes on the hydrologic response of reclamation systems. Phys. Chem. Earth 30 (8/10), 561–574. doi:10.1016/j.pce.2005.07.010
Chang, J., Liu, M., Xu, S., Hou, L., and Wang, H. (2006). Temporal-spatial distribution and first flush effect of urban stormwater runoff pollution in Shanghai City. Geographical Res. 25 (6), 994–1002. doi:10.11821/yj2006060006
Chen, J., and Adams, B. J. (2006). Analytical urban storm water quality models based on pollutant buildup and washoff processes. J. Environ. Eng. 132 (10), 1314–1330. doi:10.1061/(asce)0733-9372(2006)132:10(1314)
Chen, T., Tie, B., Duan, Z., Yang, Y., and Deng, G. (2013). Impact of rainfall and micro-fertilizers on the leaching migration of heavy metals under planting rape condition. J. Soil Water Conservation 1 (27), 142–145. doi:10.13870/j.cnki.stbcxb.2013.01.003
Chen, Z., Yang, K., Huang, M., Xie, B., and Li, X. (2009). Reduction effect of sunken green space on urban rainfall-runoff pollution. China Environ. Sci. 29 (6), 611–616. 10.CNKI:SUN:ZGHJ.0.2009-06-014
Chow, M. F., Yusop, Z., and Shirazi, S. M. (2013). Storm runoff quality and pollutant loading from commercial, residential, and industrial catchments in the tropic. Environ. Monit. Assess. 185, 8321–8331. doi:10.1007/s10661-013-3175-6
Deletic, A. (1998). The first flush load of urban surface runoff. Water Res. 32 (8), 2462–2470. doi:10.1016/S0043-1354(97)00470-3
Diebel, M. W., Maxted, J. T., Robertson, D. M., Han, S., and Vander Zanden, M. J. (2009). Land-scape planning for agricultural nonpoint source pollution reduction III: assessing phosphorus and sediment reduction potential. Environ. Manage. 43 (1), 69–83. doi:10.1007/s00267-008-9139-x
Donigian, A. S., and Huber, W. C. (1991). Modeling of nonpoint source water quality in urban and non-urban areas. United States: EPA600/3-91/039.
Ellis, J. B. (2000). Risk assessment approaches for ecosystem responses to transient pollution events in urban receiving waters. Chemosphere 40, 85–91. doi:10.1016/S0045-6535(99)00393-8
Environmental Protection Agency(USEPA) (1983). Results of the nationwide urban runoff program. Washington, DC: Water Planning Division.
Geonha, K., Joonghyun, Y., and Jeongkon, K. (2007). Diffuse pollution loading from urban stormwater runoff in Daejeon City, Korea. J. Environ. Manage. 85, 9–16. doi:10.1016/j.jenvman.2006.07.009
Gilbert, J. K., and Clausen, J. C. (2006). Stormwater runoff quality and quantity from asphalt, paver, and crushed stone driveways in Connecticut. Water Res. 40, 826–832. doi:10.1016/j.watres.2005.12.006
Gimeno, L., Nieto, R., Vázquez, M., and Lavers, D. A. (2014). Atmospheric rivers: a mini-review. Front. Earth Sci. 2, 2. doi:10.3389/feart.2014.00002
Gong, J., Li, Y., and Huang, J. (2017). Heavy metal pollution and its spatial distribution in the Guangzhou-Dongguan reach of the Pearl River. J. Guangzhou Univ. (Nat. Sci. Edition) 4 (16), 78–81.
Gooré Bi, E., Monette, F., and Gasperi, J. (2015). Analysis of the influence of rainfall variables on urban effluents concentrations and fluxes in wet weather. J. Hydrol. 523, 320–332. doi:10.1016/j.jhydrol.2015.01.017
Guo, Q., Ma, K., and Yang, L. (2006). Main sources of urban non-point source pollution and control measures for classified catchments. Environ. Sci. 27 (11), 2170–2175. doi:10.13227/j.hjkx.2006.11.006
Gupta, K., and Saul, A. J. (1996). Specific relationships for the first flush load in combined sewer flows. Water Res. 30 (5), 1244–1252. doi:10.1016/0043-1354(95)00282-0
Huang, G., and Nie, T. (2012). Characteristics and load of non-point source pollution of urban rainfall runoff in Guangzhou China. J. South China Univ. Tech. (Natural Sci. Edition) 40 (2), 142–147. doi:10.3969/j.issn.1000-565X.2012.02.025
Huang, J., Du, P., AO, C. T., Lei, M. H., Zhao, D. Q., Ho, M. H., et al. (2006). Characterization of urban roadway runoff in Macau. China Environ. Sci. 26 (4), 469–473. 10.CNKI:SUN:ZGHJ.0.2006-04-019
Jair, A., Nerilde, F., Jeferson, D., and Gabriel, B. (2020). Manure application at long-term in no-till: effects on runoff, sediment and nutrients losses in high rainfall events. Agric. Water Management 228, 105908. doi:10.1016/j.agwat.2019.105908
Jaiswal, R., Ali, S., and Bharti, B. (2020). Comparative evaluation of conceptual and physical rainfall–runoff models. Appl. Water Sci. doi:10.1007/s13201-019-1122-6
Jeffrey, S., Julie, S., Kendra, O., James, D., and Adam, W. O. (2005). Evaluation of seasonal scale first flush pollutant loading and implications for urban runoff management. J. Environ. Manage. 76, 309–318. doi:10.1016/j.jenvman.2004.12.007
Lee, G. F., and Jones-Lee, A. (2005). “Urban stormwater runoff water quality issues,” in Water encyclopedia: surface and agricultual water. Hoboken, NJ: Wiley, 432–437. doi:10.1002/047147844X.sw1602
Iuliana, C., Alexandru, C., Felicia, I., and Silviu, G. (2020). City water pollution by soot-surface-active agents revealed by FTIR spectroscopy. Appl. Surf. Sci. 499, 142487. doi:10.1016/j.apsusc.2019.04.179
Lusk, M. G., Toor, G. S., and Inglett, P. W. (2020). Organic nitrogen in residential stormwater runoff: implications for stormwater management in urban watersheds. Sci. total Environ. 707, 135962. doi:10.1016/j.scitotenv.2019.135962
Ma, Y., McGree, J., and Liu, A., Deilami Deilami, K., Egodawatta Egodawatta, P., and Goonetilleke Goonetilleke, A. (2017). Catchment scale assessment of risk posed by traffic generated heavy metals and polycyclic aromatic hydrocarbons. Ecotoxicol Environ. Saf. 144, 593–600. doi:10.1016/j.ecoenv.2017.06.073
Murakami, M., Nakajima, F., and Furumai, H. (2008). The sorption of heavy metal species by sediments in soakaways receiving urban road runoff. Chemosphere 70, 2099–2109. doi:10.1016/j.chemosphere.2007.08.073
Ou, L., Hu, D., and Huang, Y., Cui Cui, S., Guo Guo, T., Zhang Zhang, W., et al. (2011). First flush analysis of PAHs in roof runoff in beijing. Environ. Sci. 32 (10), 2896–2902. doi:10.13227/j.hjkx.2011.10.043
Ouyang, W., Wang, W., Hao, F., Song, K. Y., and Wang, Y. H. (2010). Pollution characterization of urban stormwater runoff on different underlying surface conditions. China Environ. Sci. 30 (9), 1249–1256.
Pilcher, D. J., Siedlecki, S. A., Hermann, A. J., Coyle, K. O., Mathis, J. T., and Evans, W. (2018). Simulated impact of glacial runoff on CO2 uptake in the Gulf of Alaska. Geophys. Res. Lett. 45 (7), 880–890. doi:10.1002/2017GL075910
Ren, X., Hong, N., Li, L., Kang, J., and Li, J. (2020). Effect of infiltration rate changes in urban soils on stormwater runoff process. Geoderma 363. doi:10.1016/j.geoderma.2019.114158
Ren, Y., Wang, X., Han, B., Ouyang, Z., and Miao, H. (2005). Chemical analysis on stormwater-runoff pollution of different underlying urban Surfaces. Acta Ecologica sinica 25 (12), 3225–3230.
Ren, Y., Wang, X., Ouyang, Z., and Hou, P. Q. (2013). Analysis of first flush effect of typical underlying surface runoff in beijing urban city. Environ. Sci. 34 (1), 373–377. doi:10.13227/j.hjkx.2013.01.046
Sansalone, J. J., and Cristina, C. M. (2004). First flush impervious concepts for suspended and dissolved solids in small watershed. Jouranal Environ. Eng. ASCE 130 (11), 1301–1314. doi:10.1061/(ASCE)0733-9372(2004)130:11(1301)
Shang, H., and Sun, Z. (2019). PAHs (naphthalene) removal from stormwater runoff by organoclay amended pervious concrete. Construction Building Mater. 200, 170–180. doi:10.1016/j.conbuildmat.2018.12.096
Shi, R. (2011). Ecological environment problems of the three gorges reservoir area and countermeasures. Proced. Environ. Sci. 10, 1431–1434. doi:10.1016/j.proenv.2011.09.228
Soller, J., Stephenson, J., Olivieri, K., Downing, J., and Olivieri, A. W. (2005). Evaluation of seasonal scale first flush pollutant loading and implications for urban runoff management. J. Environ. Manage. 76, 309–318. doi:10.1016/j.jenvman.2004.12.007
Tang, S., Jiang, J., Zheng, Y., Hong, Y., Chung, E. S., Shamseldine, A. Y., et al. (2021). Robustness analysis of storm water quality modelling with LID infrastructures from natural event-based field monitoring. Science of the Total Environment (753), 142007. doi:10.1016/j.scitotenv.2020.142007
The Ministry of Environmental Protection of the People's Republic of China (2002). Environmental quality standards for surface water, GB3838-2002, Beijing (in Chinese).
Walaszek, M., Bois, P., Laurent, J. E., and Wanko, A. (2018). Micropollutants removal and storage efficiencies in urban stormwater constructed wetland. Sci. Total Environ. 645, 854–864. doi:10.1016/j.scitotenv.2018.07.156
Wang, X., and Wang, X. (2002). Recent progress of diffuse pollution on loading, control and management. J. capital normal Univ. 023 (001), 91–96. doi:10.19789/j.1004-9398.2002.01.020.101
Wu, H., Huang, G., Meng, Q., Zhang, M., and Li, L. (2016). Deep tunnel for regulating combined sewer overflow pollution and flood disaster: a case study in Guangzhou city, China. Water (8), 329. doi:10.3390/w8080329
Wu, J., Xiong, L., Wu, J., Sha, C. Y., Tang, H., Lin, K. F., et al. (2019). Comparison and source apportionment of PAHs pollution of runoff from roads in suburb and urban areas of shanghai. Environ. Sci. 05 (40), 2240–2248. doi:10.13227/j.hjkx.201810055
Wu, Q. (2018). The phyto immobilizing characteristics of the polycyclic aromatic hydrocarbons (PAHs) contents of rainstorm runoff from forest and different surfaces in Guangzhou city. Beijing, China: Chinese Academy of Forestry.
Xu, D., Lee, L., Lim, F., Lyu, Z., Zhu, H., Ong, S. L., et al. (2020). Water treatment residual: a critical review of its applications on pollutant removal from stormwater runoff and future perspectives. J. Environ. Manage. 259. doi:10.1016/j.jenvman.2019.109649
Xu, S., and Huang, Y. (2004). Study of atlas of shanghai urban physical geography. Beijing: China Cartographic Publishing House.
Ye, Y., Li, S., Zhang, H., Su, Y., Wu, Q., and Wang, C. (2017). Spatial-temporal dynamics of the economic efficiency of construction land in the Pearl River delta megalopolis from 1998 to 2012. Sustainability 10 (1), 63. doi:10.3390/su10010063
Yu, G., Choi, J., Kang, H, M., and Kim, L. H. (2016). Evaluation of the volume and pollutant reduction in an infiltration and filtration facility with varying rainfall conditions. J. Korean Soc. Water Environ. 32 (1), 30–35. doi:10.15681/KSWE.2016.32.1.30
Zeng, J., Huang, G., Luo, H., Mai, Y., and Wu, H. (2019). First flush of non-point source pollution and hydrological effects of LID in a Guangzhou community. Scientific Rep. 9, 13865. doi:10.1038/s41598-019-50467-8
Zeng, Y., Cai, Y., Tan, Q., and Dai, C. (2020). An integrated modeling approach for identifying cost-effective strategies in controlling water pollution of urban watersheds. J. Hydrol. 581, 124373. doi:10.1016/j.jhydrol.2019.124373
Zhao, D., Tan, C., and Peng, X. (2015). Preliminary study on the pollution of rainwater in the initial stage of shijing river basin in Guangzhou. J. Guangdong Tech. Coll. Water Resour. Electric Eng. 3 (17), 5–8.
Zhu, Z., Fu, Y., Woodcock, C. E., Olofsson, P., Vogelmann, J. E., Holden, C., et al. (2016). Including land cover change in analysis of greenness trends using all available Landsat 5, 7, and 8 images: a case study from Guangzhou, China (2000–2014). Remote Sensing Environ. S0034425716301407 185, 243–257. doi:10.1016/j.rse.2016.03.036
Keywords: urban rainstorm runoff, Guangzhou city, urban pollutant migration, urban hydrology and hydraulics, polycyclic aromatic hydrocarbons
Citation: Pan Y, Li Z, Gao Y, Xiong Y, Qiao Y, Tao Y, Wu Q, Lin W, Qi Y, Long J, Ruan L, Dai S and Zang C (2021) Analysis of the Migration Characteristics of Stormwater Runoff Pollutants on Different Underlying Surfaces in Guangzhou, China. Front. Earth Sci. 9:554588. doi: 10.3389/feart.2021.554588
Received: 22 April 2020; Accepted: 08 February 2021;
Published: 14 April 2021.
Edited by:
Mingfu Guan, The University of Hong Kong, Hong KongReviewed by:
An Liu, Shenzhen University, ChinaCopyright © 2021 Pan, Li, Gao, Xiong, Qiao, Tao, Wu, Lin, Qi, Long, Ruan, Dai and Zang. This is an open-access article distributed under the terms of the Creative Commons Attribution License (CC BY). The use, distribution or reproduction in other forums is permitted, provided the original author(s) and the copyright owner(s) are credited and that the original publication in this journal is cited, in accordance with accepted academic practice. No use, distribution or reproduction is permitted which does not comply with these terms.
*Correspondence: Chuanfu Zang, Y2h1YW5mdXphbmdAMTYzLmNvbQ==
Disclaimer: All claims expressed in this article are solely those of the authors and do not necessarily represent those of their affiliated organizations, or those of the publisher, the editors and the reviewers. Any product that may be evaluated in this article or claim that may be made by its manufacturer is not guaranteed or endorsed by the publisher.
Research integrity at Frontiers
Learn more about the work of our research integrity team to safeguard the quality of each article we publish.