- 1Leibniz Institute for Applied Geophysics, Hannover, Germany
- 2BayCEER and Chair of Geomorphology, University of Bayreuth, Bayreuth, Germany
At the Willendorf site Upper Paleolithic archeological layers associated to early Aurignacian cultures were found. The environmental conditions of the associated society, potentially co-existing with Neanderthal groups, is still not fully understood. Here, we report on environmental magnetic analyses including anisotropy of the magnetic susceptibility (AMS) carried out on loessic aeolian sediments at the Willendorf site. Data on lineation, foliation and the degree of anisotropy were used to assess depositional and post-depositional magnetic fabric properties and to deduce site-specific environmental processes. Overall, the loess is of aeolian origin and shows magnetic enhancement and magnetic fabric properties similar to those of other European loess geoarchives, but the magnetic mineralogy differs from many ‘dry’ loess sites, insofar as it shows a higher susceptibility during heating. We infer an enhanced neoformation of magnetite during heating due to the presence of organic matter. While at face value the AMS properties are indicative of pure aeolian loess consistent with previous studies, imbrication suggests post-depositional slope movement toward the Danube, which obscures inferences on palaeo-wind direction. It is well possible that these post-depositional magnetic fabric alterations occur at other localities with similar geomorphological settings.
Introduction
Loess deposits provide the longest continuous terrestrial record of paleoclimatic change still accumulating today, and cover large parts of Eurasia. These sediments originate from settled dust of dominantly silty composition. Generally, fine and predominantly silty material can be derived directly from vast drylands or high mountain ranges, and is in the latter case transported first by river systems, and later on by wind (e.g., Cowie, 1964; Smalley, 1972; Mason, 2001; Smalley et al., 2009; Lancaster, 2020). In western Eurasia and especially in the surroundings of glaciated areas and river floodplains of (braided) rivers, silt-size mineral dust typically accumulates in sediment-traps close to the major rivers systems, where they contribute the substrate for loess-paleosol-sequences (LPS; e.g., Smalley, 1972; Smalley et al., 2009; Jipa, 2014; Lehmkuhl et al., 2016; Lehmkuhl et al., 2018). This may include the slopes of surrounding mountains. While aeolian sedimentation rates and pedogenetic overprinting of accumulated dust may differ with climatic conditions, LPS often constitute high-resolution geoarchives of past environmental conditions where other archives are sparse or even absent (e.g., Derbyshire, 2003; Shi et al., 2003; Rousseau et al., 2007; Yang and Ding, 2014; Haesaerts et al., 2019). Therefore, numerous loess sites in Europe are considered valuable archives for past climate and environments (e.g., Shi et al., 2003; Antoine et al., 2009; Krauß et al., 2016; Moine et al., 2017; Obreht et al., 2017; Zeeden et al., 2018). This includes specifically archaeological sites imbedded in loess in Austria (e.g., Hambach et al., 2008; Händel et al., 2009; Sprafke et al., 2013; Terhorst et al., 2013, Terhorst et al., 2015; Zeeden et al., 2015; Meyer-Heintze et al., 2018; Sprafke et al., 2020). Notably in western Eurasia, loess hosts and preserves a wealth of archaeological materials due to its naturally high carbonate content which ensures not only bone preservation but generally buffers pervasive chemical weathering (Händel et al., 2009; Neugebauer-Maresch et al., 2014).
Sedimentological and rock magnetic analyses are used in geoarchaeological research in order to investigate the intensity and type of deposition, pedogenesis and post-depositional alterations (e.g., Hambach et al., 2008; Antoine et al., 2009; Zeeden et al., 2009; Bösken et al., 2018). The homogeneity of a sediment source may be determined from grain size-, geochemical- and rock magnetic properties (e.g., Cheng et al., 2019; Obreht et al., 2019). The intensity of pedogenesis may be derived from geochemical weathering indices (e.g., Buggle et al., 2011; Újvári et al., 2014; Obreht et al., 2019), the neoformation of clay (Schulte and Lehmkuhl, 2018) and also magnetic enhancement (e.g., Heller and Tung-Sheng, 1986; Forster et al., 1994; Forster et al., 1996; Hambach et al., 2008; Bradák et al., 2011; Zeeden et al., 2016). Post-depositional alteration through water logging may be derived from the depletion of magnetic minerals if present (e.g., Bábek et al., 2011; Baumgart et al., 2013; Gocke et al., 2014; Taylor et al., 2014; Krauß et al., 2016). In addition, the translocation of fine magnetic particles may play a role (e.g., Oldknow et al., 2020).
At Willendorf, the sediment is described to be of local aeolian origin due to its grain size distribution properties (Haesaerts, 1990). The sediment exhibits clear features of slope movement (Haesaerts, 1990; Haesaerts and Teyssandier, 2003; Nigst, 2006; Nigst et al., 2008; Nigst et al., 2014), also Figure 1B. The loess shows stratigraphical consistency of bleaching, pedogenesis of different intensity, and charcoal indicative of (human?) burning. To assess in which manner the loess is suitable for paleoenvironmental reconstructions, we conducted an environmental magnetic study. Several rock magnetic parameters were measured to assess the type of magnetic enhancement or depletion, and to roughly characterize the minerals contributing to the magnetic susceptibility. Most relevant, the anisotropy of the magnetic susceptibility (AMS) was measured for a suite of samples to gain insight into magnetic fabric and either depositional or post-depositional processes. In order to account for possible spatial differences, several profiles were sampled. The AMS describes the spatial anisotropy of the magnetic fabric. In sediments, oblate fabrics often dominate due to the settling of, among others, clay minerals; the resulting oblateness is commonly called foliation (F). A preferential alignment, called lineation (L), may be interpreted in terms of transport direction, and/or may give insight into post-depositional processes, especially slope movement. Importantly, the AMS can give insight different depositional processes, such as aeolian loess and water-lain material (Liu et al., 1988; Bradák and Kovács, 2014). However, the values reported in Liu et al. (1988) are not ubiquitously applicable (Bradák and Kovács, 2014; Cheng et al., 2019). Further, preferential alignment directions of magnetic particles can be used to investigate the direction in which deposition took place (Jordanova et al., 2007b; Zeeden et al., 2015) and also the effects of post-depositional alteration (e.g., Bradák and Kovács, 2014; Taylor and Lagroix, 2015; Bradák et al., 2019a).
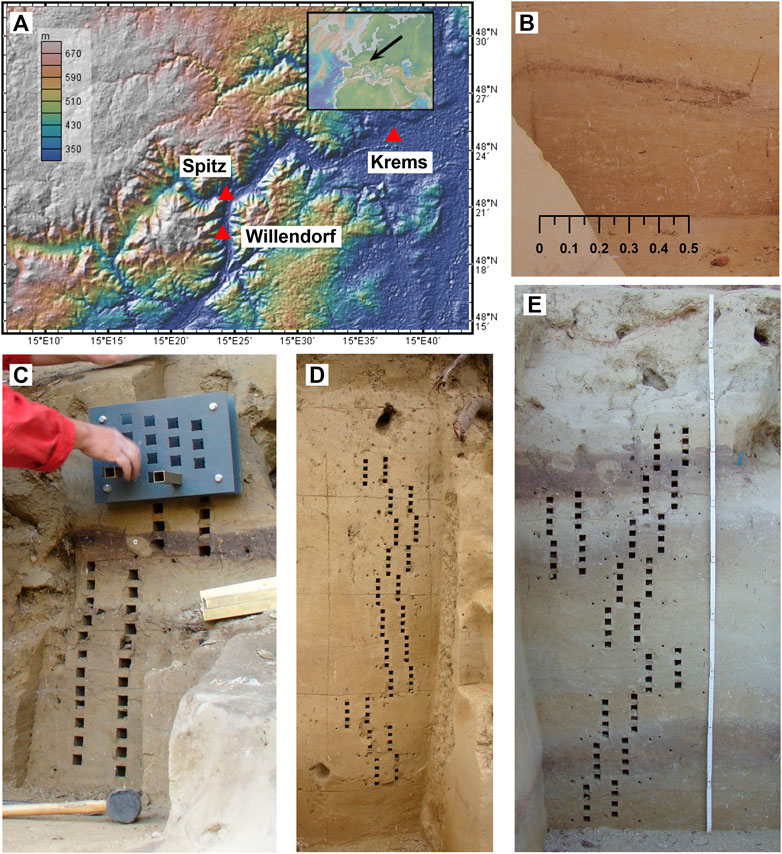
FIGURE 1. Location of the Willendorf Site in Lower Austria [(A); top] in the vicinity of the cities Spitz and Krems, and field photos of sediment movement (B), including a scale of 0.5 m, (C) the right profile #5, (D) the left profile #4, and (E) profile #1. Note that images (B–D) were taken into the wall, where (E) is taken perpendicular to the wall. Photographs were taken in daylight and partly under a tent, therefore illumination is imperfect.
Here we present a rock magnetic study on the Willendorf site in Austria, which is famous for reports on the oldest Aurignacian technology in Central Europe (Nigst et al., 2008; Nigst et al., 2014). The key aim is to understand the primary (post) depositional and pedogenic processes.
Setting
The archaeological site of Willendorf is famous for the “Venus of Willendorf” (Antl-Weiser, 2009). It is one of a suite of archaeological sites preserved in loess along the Danube in the area since 2006, systematic excavations were conducted by an interdisciplinary team (Nigst, 2006; Nigst et al., 2008; Nigst et al., 2014), from which one of the earliest modern human tool assemblages in western Europe was identified. The excavation studied here is commonly referred to as “Willendorf II.” The Willendorf II section is located in the Danube valley west of Vienna (Austria) in the Wachau area (Figure 1). The exposure is located on a terrace c. 15 above and c. 300 m west of the present Danube river at 48°19′23.5″ latitude and 15°24′15.2″ longitude.
Along with the archaeological excavations, sedimentological investigations and detailed 14C dating have been carried out. Several publications divide the site into sedimentological Units A (top) to E (bottom) (Haesaerts, 1990; Haesaerts et al., 1996; Haesaerts and Teyssandier, 2003, and references therein). We sampled the Units C and D in order to gain information on the site formation and post-depositional processes. Unit D is characterized as “thick stony heterogeneous brown loam” (Haesaerts and Teyssandier, 2003). Abundant molluscs, a polyedric structure and biogalleries filled with carbonates are reported from the top of Unit D. It was 14C dated to ∼41,700 and ∼39,500 BP. Layers (C9 to C2) represent a suite of yellow to gray loess intercalated with several humic horizons. The uppermost humic soil horizon (at the top of the interval C9 to C2; Figure 2) is expressed as strongest soil. Especially as the lower part is reported to be stretched by solifluction, and contains stripes of ash. Subunit C1 represents a tundra gley and represents a marker between the middle and late Pleniglacial at ∼28,000 BP (Haesaerts, 1990; Haesaerts and Teyssandier, 2003). The layers at the archaeological site are inclined toward the Danube valley (Haesaerts et al., 1996; their Figure 1) and show, at least in part, signs of solifluction (Haesaerts, 1990; Haesaerts et al., 1996; Haesaerts and Teyssandier, 2003; Nigst et al., 2008; Nigst et al., 2014). Grain size distributions of the Willendorf stratigraphy are reported in Haesaerts (1990), and show the highest contributions of silt in the ∼10–80 µm range. An additional coarse component (>150 µm) contributes up to ∼10% in Unit D, but less than 5% to samples from Unit C. Haesaerts (1990) interprets the grain size data (in context of field observations) as indicative aeolian transported fluvial sediments from the Danube River floodplain.
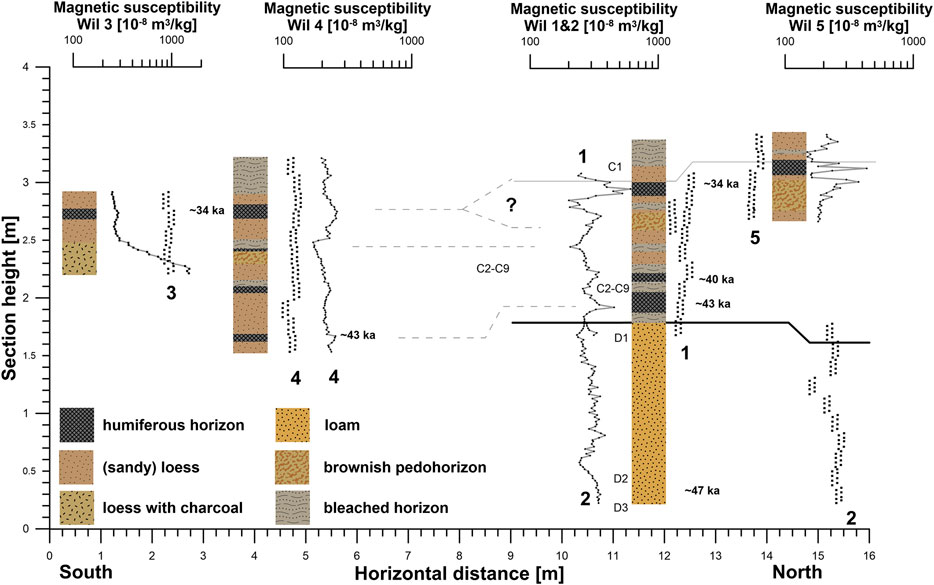
FIGURE 2. Sampling scheme for magnetic measurements at Willendorf as sampling spurs. The magnetic susceptibility profiles (one to five, where one and two are plotted together) are plotted with simplified stratigraphies. Several geological layer numbers (from Nigst et al., 2008) are included here (C1-D3). Ages are taken from the correlation to Greenland ice core proxy data by (Nigst et al., 2014) and have been applied to equivalent depths of our profiles. Lines correlate different profiles (own interpretation).
Here we report on the sedimentological findings from environmental magnetic investigations, and contextualize these regarding selected loess geoarchives. Sedimentological descriptions of Willendorf were done by Haesaerts and Teyssandier (2003) and the references therein. Especially the paper by Haesaerts and Teyssandier (2003) provides comprehensive sedimentological descriptions of loess, loam and different soil types. In addition, their work and the paper by Haesaerts (1990) place the Willendorf into the stratigraphic context of other archaeological sites from the Danube Valley and Moravia.
Methods
Oriented specimens were taken using brass tubes and an orientation holder. The samples are cubes with an edge length of 2 cm each, giving 8 cm3 sample volume. Full spatial orientation was provided by means of magnetic compass measurements. The vertical spacing of sample centers was ∼2.1 cm. Stratigraphic heights are given in cm as relative to the profile base.
Once in the laboratory, samples were stored in a µ-metal box until measurements began. Measurements were carried out in the Laboratory for Paleo- and Environmental Magnetism, Bayreuth. Anhysteric remanent magnetisations (ARMs) were produced along one spatial axis, and were induced with a 50 µT static field and 100 mT alternating field amplitude using a Magnon AFD 300 (Figure 3). Isothermal remanent magnetisations (IRMs) were induced with fields up to 2.5 T using a MAGNON PM II pulse magnetizer. Back-field IRMs were produced to determine the coercivity of remanence (Bcr). The magnetisations resulting from imprinting ARMs and IRMs were measured using an AGICO JR-6A spinner magnetometer.
The mass-specific magnetic low-field susceptibility (MS) and AMS measurements were made with an AGICO KLY-3S kappabridge at a frequency of 875 kHz and a magnetic field intensity of 300 A/m. The temperature dependent susceptibility (κ-T) was determined with this bridge and the CS-3 furnace under an Argon atmosphere. Data were corrected for a nine-point moving average smoothed dataset of the empty holder, and were scaled to the mass-normalized room temperature susceptibility using the CUREVAL software supplied by AGICO. The frequency dependent susceptibility (χfd%) was measured for profiles one to three. A MAGNON VFSM was used with frequencies of 300 Hz and 3 kHz and a field of 300 A/m. χfd% is defined as (χ300 Hz–χ3 kHz)/χ300 Hz × 100, Δχ represents the difference χ300 Hz–χ3 kHz (Figure 4).
The AMS was measured for every 8th/5th sample. The AMS is defined by a symmetric tensor with three principal axes (k1 > k2 > k3). k1 is the main MS axis of a 3-dimensional ellipsoid and represents the magnetic lineation direction. k3 is perpendicular to the magnetic foliation which represents a plain through k1 and k2 (e.g., Ferré et al., 2003). The principal susceptibility angles were corrected for the sampling angles, and were calculated together with AMS parameters using the AGICO software Anisoft 5.0. The determination and interpretation of AMS is a useful tool to determine the alignment of para- and ferromagnetic particles. Both the intensity of alignment and its direction can give insight into syn- and post-depositional sedimentary processes. However, a single sample may not be representative for a past environment, and commonly multiple samples are being investigated for a well-founded interpretation (Tarling and Hrouda, 1993). The AMS parameters lineation (L), foliation (F) and the degree of anisotropy (P) are plotted in Figure 3 with corresponding depths. These are defined as L = (k1–k2)/kmean, F = (k2–k3)/kmean and P = k1/k3 (Tarling and Hrouda, 1993, and references therein).
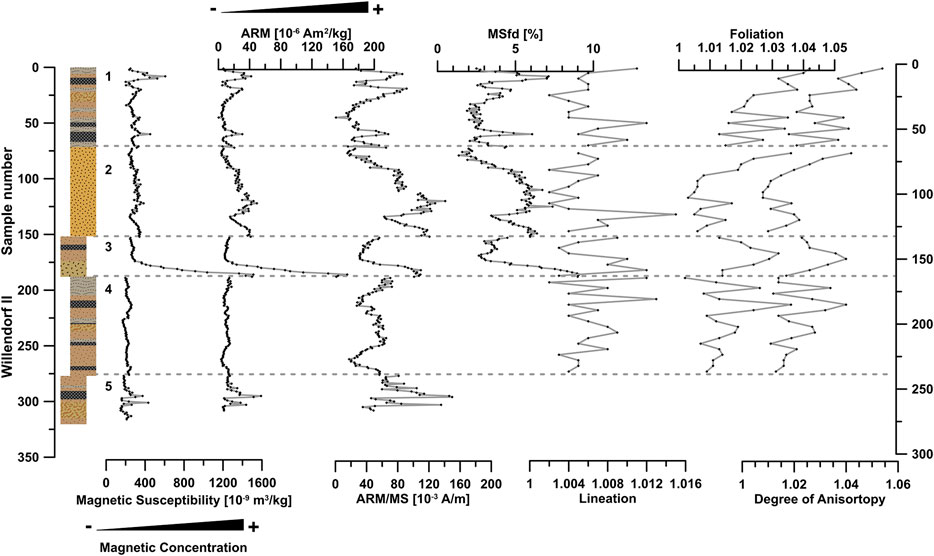
FIGURE 3. Rock magnetic parameters for the five profiles (from Figure 2; plotted on top of each other against lithology on the left), and their interpretation regarding magnetic concentration and grain size for the magnetic susceptibility (MS), anhysteretic remeanent magnetisation (ARM) and the ARM/MS ratio. The frequency dependent magnetic susceptibility (χfd%) may be regarded as representing a combination of magnetic concentration and grain size. Note that the ordinate is not representing a continuous stratigraphy.
Results and Discussion
Rock Magnetic Properties as Indicators of Lithology
The reported rock magnetic datasets, in depth, consist of the magnetic susceptibility, its frequency dependency, and the ARM. In addition, AMS, κ-T and IRM data have been measured for selected samples (Figures 3, 5‐7). An overview of the sampling scheme is given in Figure 2, with MS data plotted for all samples. Figure 3 depicts several rock magnetic properties (left) and also the AMS properties: Lineation and Foliation (right). The magnetic parameters ARM, ARM/MS and χfd%, indicative of magnetic concentration and grain size, roughly follow the lithology. Increased values of the MS, χfd%, and ARM occur in soil formation phases and cultural layers. The mean MS is 29.5 × 10–8 m3/kg, the mean χfd% is 4%, and the mean ARM is 18.63 × 10–8 Am/kg.
Mineral magnetic parameters have been recognized as relevant paleoclimate proxies in loess research. MS and χfd% provide highly sensitive proxies in particular for humidity during dust accumulation and loess formation (e.g., Buggle et al., 2014 and references therein). This is based on the assumption that the original loess can be regarded as homogenous, and the neoformation of magnetic minerals (mainly magnetite and maghemite) is driven by pedogenesis. Increasingly intense pedogenesis enhances the mineral magnetic signals. The MS of any mineral magnetic assemblage in loess-paleosol sequences is also determined by the physical grain size distribution and magnetic mineralogy. The highest values in MS and χfd% can occur in intervals with higher concentrations of ultra-fine magnetic particles, but may in theory also be dependent on dust source and wind intensity (Song et al., 2010). Such fine particles precipitate dominantly from weathering solutions, and their abundance is a sensitive sediment/soil humidity proxy (e.g., Balsam et al., 2011; Maher, 2011). The MS is mainly an indicator of bulk concentration of magnetic minerals, while the χfd% is mainly driven by magnetic grain size. Increased χfd% indicates fine-viscous (VF herein) magnetic grain sizes in the transition from Stable Single Domain (SSD) and Superparamagnetic (SP) states (e.g., Zheng et al., 1991; Yu and Oldfield, 1993; Deng et al., 2006).
The IRM acquisition curves show the dominance of magnetically soft minerals (Figure 7), here probably magnetite and maghemite. A hard component, which is not fully saturated at 2.5 T, is also present, and is interpreted to represent (goethite and/or) hematite. The ARM signal is considered to be driven by the concentration of ferromagnetic particles. Thereby, ARM reflects mainly Stable Single Domain (SSD) magnetite and maghemite, for which MS is relatively low. Consequently, the ARM/MS ratio is related to magnetic grain size, with higher ARM contributions (and thus a higher ARM/MS ratio) referring to magnetic grain sizes of SSD and small PSD range (c. 0.03–0.2 µm; Liu et al., 2012). This relationship between ARM contribution and grain size is highlighted by the pattern similarity of the ARM and the χfd%, which is also related to magnetic grain size. The information from both proxies characterize the enhancement of fine magnetic grain size populations at the SP-SSD boundary, which is increasing with pedogenetic overprinting of unaltered aeolian dust accumulation.
Although the frequency dependency of the magnetic susceptibility is relatively low on average, a magnetic enhancement similar to other Eurasian profiles can be observed (Figure 4). For example, the magnetic enhancement at Willendorf is similar to the enhancement at Semlac (eastern Middle Danube Basin; Zeeden et al., 2016) and Chaona (Chinese Loess Plateau; Song et al., 2007). The highest MS and Δχ data from Willendorf are likely related to a contribution of magnetic minerals created during burning in an archaeological and pedo-horizon in sub-profile three (vgl. Figure 2). In the enhancement plot (Figure 4), data from Willendorf show a higher increase of MS than of Δχ. This property suggests a higher proportion of SSD and MD particles with high MS but limited VF particles. This may also be an influence of higher hematite concentrations; its presence is indicated by the hard IRM component.
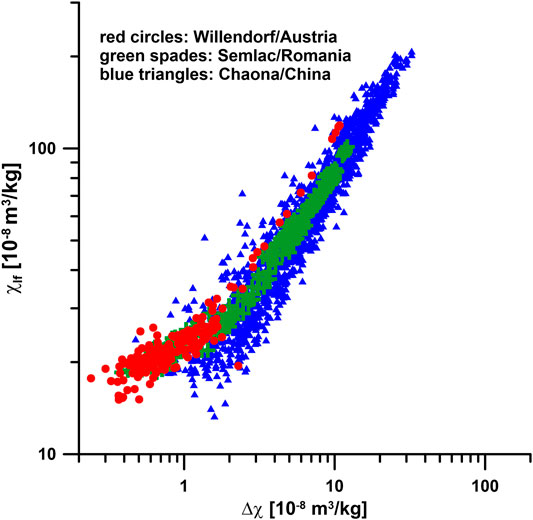
FIGURE 4. Magnetic enhancement at Willendorf, compared with Semlac in the Middle Danube Basin (Zeeden et al., 2016) and Chinese loess from Chaona (Song et al., 2007). Note that this is a double logarithmic plot.
Magnetic Mineralogy Derived from Temperature Dependent Magnetic Susceptibility
To gain insight into the magnetic mineralogy of the Willendorf site, high-temperature susceptibility experiments were carried out. Figure 5 shows the results of high-temperature susceptibility measurements (normalized to the initial susceptibility) from selected samples. The results are similar for the four investigated samples and are therefore discussed together.
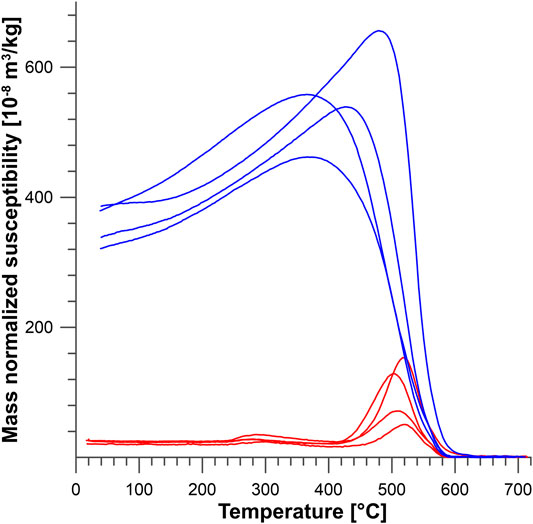
FIGURE 5. Temperature dependent magnetic susceptibility of four selected samples. Heating is plotted in red, cooling is depicted in blue. Note the increase at ∼300°C, and decrease at ∼580°C indicative of a magnetite contribution.
Little change can be observed in the magnetic susceptibility during heating up to ∼240°C, but an increase in the magnetic susceptibility is present from ∼250 to ∼280°C. This pattern is followed by an MS decrease up to ∼400°C, a strong increase to ∼500–520°C, and a final decrease in the susceptibilities at ∼580–600°C (Figure 5). Oches and Banerjee (1996) discuss an increase in the magnetic susceptibility at ∼300°C as related to a “transition of weakly-magnetic Fe-hydroxides (e.g., lepidocrocite) to maghemite or some other higher susceptibility phase”. The decrease between ∼300 and 400°C has been interpreted as the alteration of maghemite to hematite (Gao et al., 2019 and references therein). The increase from ∼400 up to ∼510°C is interpreted as formation of magnetite from several other iron bearing minerals (Hunt et al., 1995; Oches and Banerjee, 1996; Deng et al., 2001; Liu et al., 2005; Deng et al., 2006). The sample with the highest MS also shows an increase beyond this temperature, which may be a contribution of the Hopkinson effect (Dunlop, 2014). However, due to the decrease in MS before ∼550°C and a strong increase of the MS during heating we consider the Hopkinson effect a contributor, but not the dominant control on MS above ∼450°C (following Deng et al., 2000). Also the increased MS during cooling speaks for the neo-formation of magnetite, and against the dominance of the Hopkinson effect. Here, the final decrease of the susceptibility at ∼580°C is interpreted to represent the Curie temperature of magnetite. While it is clear from these data that magnetite dominates the magnetic susceptibility, the size of the potential contribution from hematite is uncertain in this case. The fact that magnetite did only partly oxidize to maghemite during seasonal dry phases may be interpreted as annually mostly wet soil and sediment conditions, possibly due to periglacial conditions. Note that our κ-T results are similar to the κ-T data obtained from loess from the last glacial at the Krems-Wachtberg archaeological site about 20 km north-east of Willendorf (Zeeden et al., 2015), a loess-paleosol sequence in the Czech Republic (Oches and Banerjee, 1996), and the Poiana Cireşului site in eastern Romania (Zeeden et al., 2009, Zeeden et al., 2011); and as such, indicates similarities in terms of magnetic mineralogy. However, in other studies of European loess distinctly different temperature dependent susceptibility patterns have been observed in the drier parts of the Middle and Lower Danube Basins (Jordanova et al., 2007a; Necula and Panaiotu, 2012; Bradák et al., 2019b). In particular, the strong increase in MS from ∼450 to 580°C is not present in loess preserved within arid and steppe environments. This increase in MS from ∼450 to 580°C may be related to a higher amount of organic matter in the sediment at Willendorf (Campbell et al., 1997).
A relevant contribution of water logging processes would lead to an increase in the amount of fine particles through grain size reduction. This would be visible as increasing Δχ and decreased MS in Figure 4 (Baumgart et al., 2013; Zeeden et al., 2018). However, this has not been observed in our results. Water logging can also increase magnetic grain size through preferential dissolution of small grains (e.g., Oldknow et al., 2020). This would lead to decreasing Δχ with decreasing MS, which does not seem a relevant factor either compared to reference data (Figure 4).
AMS Properties
Phyllosilicates (here probably micas and clay minerals), generally have oblate AMS ellipsoids (e.g., Borradaile and Werner, 1994; Martı́n-Hernández and Hirt, 2003). Under gravity these will settle preferentially in a horizontal plane, imprinting an oblate magnetic fabric in the bedding plane. Also compaction plays a role and increases F (Zhu et al., 2004). Since the AMS of most samples from Willendorf is oblate due to the higher foliation than lineation (Figure 3), and only few samples show strong lineations, the AMS may be dominated by phyllosilicates and potentially by super-fine ferrimagnetic particles incorporated in and attached to those minerals (Hyodo et al., 2020).
Here, the measures of anisotropy (P, L, F) show no obvious trends and relations to archaeological layers and pedo-horizons. Generally, the anisotropy of samples is low, mean values for P, L and F are 1.006, 1.017 and 1.023, respectively. The P and F data correlate with a correlation coefficient of 0.98, whereas the correlation between the L and F is only 0.01. The F and L are independent variables, and therefore may have different physical and genetic origins. Foliation is commonly found parallel to the bedding plane due to gravitational forces and compaction (Hus, 2003), and is generally interpreted to be the result of sedimentation of oblate particles. Wang and Løvlie (2010) suggest that the foliation is mainly acquired during (initial) wetting of freshly aeolian deposited loess.
The lineation of the magnetic fabric may be related to a preferential aeolian wind or deposition direction (e.g., Tarling and Hrouda, 1993; Lagroix and Banerjee, 2002; Zeeden et al., 2015), or can originate from post-depositional processes (e.g., slope movement processes; Reinders and Hambach, 1995). At Willendorf, slope movement toward the Danube valley is evident in the field (Figure 1B). The imbrication of the k3 axis in the north-east direction (Figure 6) may be related to either deposition on a slope, or to post-depositional changes due to down-slope sediment movement. Because the magnetic foliation is mainly acquired through gravitational forces during initial wetting (Wang and Løvlie, 2010), we consider the imbrication as originating from post-depositional slope movement in the direction of the Danube valley. The k1 direction is aligned in the downslope direction with the imbrication of the k3 inclination. We therefore interpret the k1 direction as indicative of the direction of the windblown loess, while the imbrication as representing post-depositional slope processes, the latter dominating the AMS at Willendorf.
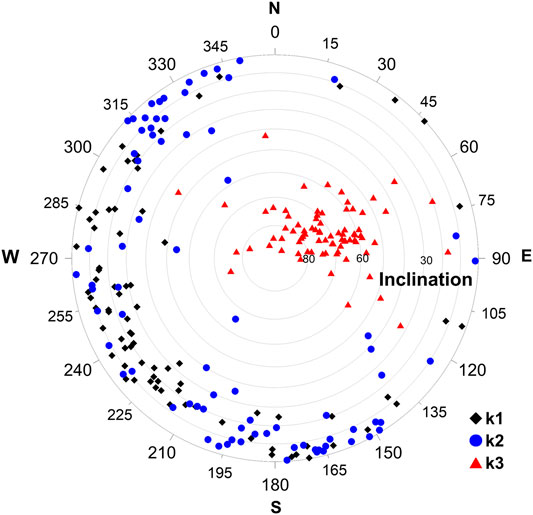
FIGURE 6. Directions of the anisotropy of the magnetic susceptibility, plotted as stereographic projection on the upper hemisphere. Red triangles represent Kmin directions pointing predominantly to the east (northeast), black spades stand for Kmax directions and blue circles represent Kint directions.
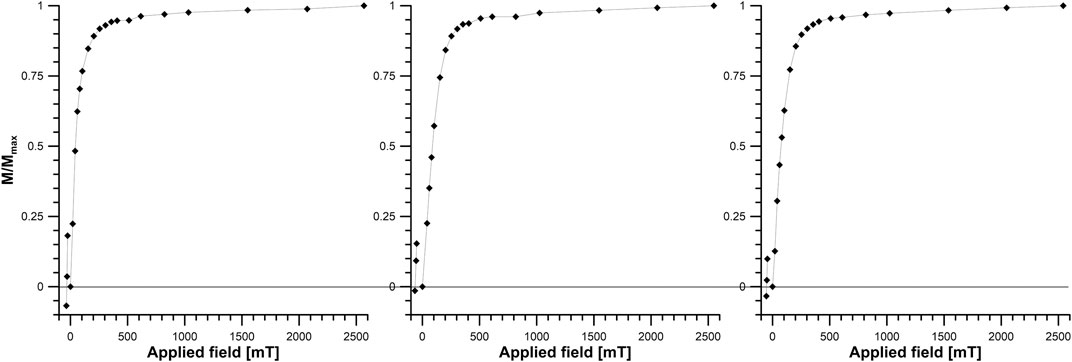
FIGURE 7. Acquisition and back-field curves of the Isotherman Remanent Magnetisation (IRM) for three samples. The ordinate denotes the magnetisation over the maximal magnetisation at the highest applied field. Note that magnetisation increases steeply with the applied field until ∼300 mT, and increases slowly thereafter. The field necessary to reduce the net remanence to zero lies between 32 and 64 mT.
The degree of anisotropy has been suggested to reflect the depositional environment of loess and loess-like deposits, where aeolian loess has been shown to obtain a lower foliation relative to loess redistributed by water or as water-saturated mud [mostly <1.02, (Liu et al., 1988; Bradák and Kovács, 2014); mostly <1.03 (Wang and Løvlie, 2010)]. Comparing the Willendorf data (mean Foliation F of 1.023 and mean Lineation L of 1.017) to the data of Bradák and Kovács (2014), Liu et al., (1988), and Wang and Løvlie (2010), the loess at Willendorf is of aeolian origin, and not re-deposited on the slope by periglacial or other slope processes. This contradicts our interpretation of a post-depositional imbrication. In addition, the loess was deposited on a slope which makes the absence of post-depositional relocation unlikely, especially also regarding potential sediment dynamics under a periglacial climate. Therefore, this study shows that the low foliations may not always be representative of “purely” aeolian loess. We hypothesize that the values of AMS properties are influenced by the grain size distribution, which is in this case rather coarse. Especially the addition of quartz sand can be expected to decrease the AMS and lead to a lower foliation than caused by finer silt- and clay-sized (and more often platy) particles.
While fine magnetic particles formed during in-situ pedogenesis may dominate the MS signal, the originally deposited carriers of MS are relevant for interpreting the AMS. This may be a large or small proportion, depending on the original contribution of grain sizes. Numerous studies have shown a relationship between particle size and magnetic grain size, with detrital magnetic grains being SSD or coarser. These tend to reside in coarser particle size fractions—and therefore, are less liable to translocation. Larger detrital (magnetic) grains are unlikely to be moved within the sediment, while small grains may move downward in the sediment column. In addition, illuviation of fine particles (as fine clay), which may be relevant carriers of fine magnetic particles, can be excluded here because the sediment has a high carbonate content throughout, preventing any illuviation processes (e.g., Kühn et al., 2018).
Conclusion
Based on the magnetic properties of the loess sediment at Willendorf, we draw the following conclusions on the sedimentation and post-depositional alteration.
Grain size data demonstrated the loess to be of aeolian origin. The loess is partly overprinted by pedogenesis, but interstadial soils are much more weakly developed than interglacial soils from the reference data, in agreement with chronological information. The magnetic sediment properties do not show magnetic depletion due to waterlogging and/or permafrost action which is surprising in light of the presence of leached horizons, but may have resulted from the presence of high carbonate content which could have buffered dissolution reactions.
Despite evidence for colluvial slope processes in the field, these do not result in enhanced foliation values. Rather, the relatively low foliation values observed are controlled by the grain size of the loess, provenanced from deflation of the nearby Danube floodplain. We imply that AMS is to be seen in the context of grain size of the investigated material, and that general distinctions between aeolian and water-lain fabric can only be made for specific grain size distributions. The lineation in the southwest/northeast direction is interpreted as indicative for deposition on a gentle slope inclined toward the Danube. In particular, we interpret the clear imbrication in the same direction as the result of post-depositional processes moving the sediment toward the valley, presumably under a periglacial climate with seasonal freezing and thawing cycles.
Data Availability Statement
The original data presented in the study are included in the article/Supplementary Material, further inquiries can be directed to the corresponding author.
Author Contributions
UH designed the study; CZ and UH took the samples; CZ carried out measurements and carried out the study. Both authors jointly interpreted data. CZ wrote the manuscript with input from UH.
Funding
Funding for measurements were provided by the DFG under grant number 5370964.
Conflict of Interest
The authors declare that the research was conducted in the absence of any commercial or financial relationships that could be construed as a potential conflict of interest.
Acknowledgments
We thank the archaeological team under P. Nigst and B. Violá for access to the Site and for providing local assistance. We appreciate the helpful and constructive feedback from three reviewers.
Supplementary Material
The Supplementary Material for this article can be found online at: https://www.frontiersin.org/articles/10.3389/feart.2020.599491/full#supplementary-material.
References
Antl-Weiser, W. (2009). The time of the Willendorf figurines and new results of Palaeolithic research in lower Austria. Anthropologie. 47, 131–141.
Antoine, P., Rousseau, D.-D., Fuchs, M., Hatté, C., Gauthier, C., Marković, S. B., et al. (2009). High-resolution record of the last climatic cycle in the southern Carpathian Basin (Surduk, Vojvodina, Serbia). Quat. Int. 198, 19–36. doi:10.1016/j.quaint.2008.12.008
Bábek, O., Chlachula, J., and Grygar, T. M. (2011). Non-magnetic indicators of pedogenesis related to loess magnetic enhancement and depletion: examples from the Czech Republic and southern Siberia. Quat. Sci. Rev. 30, 967–979. doi:10.1016/j.quascirev.2011.01.009
Balsam, W. L., Ellwood, B. B., Ji, J., Williams, E. R., Long, X., and El Hassani, A. (2011). Magnetic susceptibility as a proxy for rainfall: worldwide data from tropical and temperate climate. Quat. Sci. Rev. 30, 2732–2744. doi:10.1016/j.quascirev.2011.06.002
Baumgart, P., Hambach, U., Meszner, S., and Faust, D. (2013). An environmental magnetic fingerprint of periglacial loess: records of Late Pleistocene loess–palaeosol sequences from Eastern Germany. Quat. Int. 296, 82–93. doi:10.1016/j.quaint.2012.12.021
Borradaile, G. J., and Werner, T. (1994). Magnetic anisotropy of some phyllosilicates. Tectonophysics 235, 223–248. doi:10.1016/0040-1951(94)90196-1
Bösken, J., Sümegi, P., Zeeden, C., Klasen, N., Gulyás, S., and Lehmkuhl, F. (2018). Investigating the last glacial Gravettian site ‘Ságvár Lyukas Hill’ (Hungary) and its paleoenvironmental and geochronological context using a multi-proxy approach. Palaeogeogr. Palaeoclimatol. Palaeoecol. 509, 77–90. doi:10.1016/j.palaeo.2017.08.010
Bradák, B., and Kovács, J. (2014). Quaternary surface processes indicated by the magnetic fabric of undisturbed, reworked and fine-layered loess in Hungary. Quat. Int. 319, 76–87. doi:10.1016/j.quaint.2013.02.009
Bradák, B., Kovács, J., and Magyari, Á. (2019a). The origin and significance of some ‘irregular’ loess magnetic fabric found in the Paks succession (Hungary). Geophys. J. Int. 217, 1742–1754. doi:10.1093/gji/ggz117
Bradák, B., Seto, Y., Csonka, D., Végh, T., and Szeberényi, J. (2019b). The hematite–goethite enhancement model of loess and an ‘irregular’ case from Paks, Hungary. J. Quat. Sci. 34, 299. doi:10.1002/jqs.3101
Bradák, B., Thamó-Bozsó, E., Kovács, J., Márton, E., Csillag, G., and Horváth, E. (2011). Characteristics of Pleistocene climate cycles identified in Cérna Valley loess–paleosol section (Vértesacsa, Hungary). Quat. Int. 234, 86–97. doi:10.1016/j.quaint.2010.05.002
Buggle, B., Glaser, B., Hambach, U., Gerasimenko, N., and Marković, S. (2011). An evaluation of geochemical weathering indices in loess–paleosol studies. Quat. Int. 240, 12–21. doi:10.1016/j.quaint.2010.07.019
Buggle, B., Hambach, U., Müller, K., Zöller, L., Marković, S. B., and Glaser, B. (2014). Iron mineralogical proxies and quaternary climate change in SE-European loess–paleosol sequences. Catena. 117, 4–22. doi:10.1016/j.catena.2013.06.012
Campbell, A. S., Schwertmann, U., and Campbell, P. A. (1997). Formation of cubic phases on heating ferrihydrite. Clay Miner. 32, 615–622. doi:10.1180/claymin.1997.032.4.11
Cheng, L., Song, Y., Sun, H., Bradák, B., Rustam, O., Zong, X., et al. (2019). Pronounced changes in paleo-wind direction and dust sources during MIS3b recorded in the Tacheng loess, northwest China. Quat. Int. 552, 122–134. doi:10.1016/j.quaint.2019.05.002
Cowie, J. D. (1964). Loess in the Manawatu district, New Zealand. N. Z. J. Geol. Geophys. 7, 389–396. doi:10.1080/00288306.1964.10420185
Deng, C., Shaw, J., Liu, Q., Pan, Y., and Zhu, R. (2006). Mineral magnetic variation of the Jingbian loess/paleosol sequence in the northern Loess Plateau of China: implications for Quaternary development of Asian aridification and cooling. Earth Planet Sci. Lett. 241, 248–259. doi:10.1016/j.epsl.2005.10.020
Deng, C., Zhu, R., Jackson, M. J., Verosub, K. L., and Singer, M. J. (2001). Variability of the temperature-dependent susceptibility of the Holocene eolian deposits in the Chinese loess plateau: a pedogenesis indicator. Phys. Chem. Earth Part Solid Earth Geod. 26, 873–878. doi:10.1016/S1464-1895(01)00135-1
Deng, C., Zhu, R., Verosub, K. L., Singer, M. J., and Yuan, B. (2000). Paleoclimatic significance of the temperature-dependent susceptibility of Holocene loess along a NW-SE transect in the Chinese loess plateau. Geophys. Res. Lett. 27, 3715–3718. doi:10.1029/2000GL008462
Derbyshire, E. (2003). Loess, and the dust indicators and records of terrestrial and marine palaeoenvironments (DIRTMAP) database. Quat. Sci. Rev. 22, 1813–1819. doi:10.1016/S0277-3791(03)00209-9
Dunlop, D. J. (2014). High-temperature susceptibility of magnetite: a new pseudo-single-domain effect. Geophys. J. Int. 199, 707–716. doi:10.1093/gji/ggu247
Ferré, E. C., Teyssier, C., Jackson, M., Thill, J. W., and Rainey, E. S. G. (2003). Magnetic susceptibility anisotropy: a new petrofabric tool in migmatites. J. Geophys. Res. Solid Earth. 108, 2086. doi:10.1029/2002JB001790
Forster, T., Evans, M. E., and Heller, F. (1994). The frequency dependence of low field susceptibility in loess sediments. Geophys. J. Int. 118, 636–642. doi:10.1111/j.1365-246X.1994.tb03990.x
Forster, Th., Heller, F., Evans, M. E., and Havliček, P. (1996). Loess in the Czech Republic: magnetic properties and paleoclimate. Studia Geophys. Geod. 40, 243–261. doi:10.1007/BF02300741
Gao, X., Hao, Q., Oldfield, F., Bloemendal, J., Deng, C., Wang, L., et al. (2019). New high-temperature dependence of magnetic susceptibility-based climofunction for quantifying paleoprecipitation from Chinese loess. G-cubed. 20, 4273–4291. doi:10.1029/2019GC008401
Gocke, M., Hambach, U., Eckmeier, E., Schwark, L., Zöller, L., Fuchs, M., et al. (2014). Introducing an improved multi-proxy approach for paleoenvironmental reconstruction of loess–paleosol archives applied on the Late Pleistocene Nussloch sequence (SW Germany). Palaeogeogr. Palaeoclimatol. Palaeoecol. 410, 300–315. doi:10.1016/j.palaeo.2014.06.006
Haesaerts, P. (1990). Nouvelles recherches au gisement de Willendorf (Basse Autriche). Bull. L’Institut R. Sci. Nat. Belg. Sci. Terre. 60, 203–218.
Haesaerts, P., Damblon, F., Bachner, M., and Trnka, G. (1996). Revised stratigraphy and chronology of the Willendorf II sequence, Lower Austria. Archaeol. Austriaca. 80, 25–42.
Haesaerts, P., Gerasimenko, N., Damblon, F., Yurchenko, T., Kulakovska, L., Usik, V., et al. (2019). The Upper Palaeolithic site Doroshivtsi III: a new chronostratigraphic and environmental record of the Late Pleniglacial in the regional context of the Middle Dniester-Prut loess domain (Western Ukraine). Quat. Int. 546, 196. doi:10.1016/j.quaint.2019.12.018
Haesaerts, P., and Teyssandier, N. (2003). “The early Upper Paleolithic occupations of Willendorf II (Lower Austria): a contribution to the chronostratigraphic and cultural context of the beginning of the Upper Paleolithic in Central Europe.” in The chronology of the Aurignacian and of the transitional technocomplexes: dating, stratigraphies, cultural implications. proceedings of symposium, 2–8 September 2-8, 2001.
Hambach, U., Zeeden, C., Hark, M., and Zöller, L. (2008). Magnetic dating of an Upper Palaeolithic cultural layer bearing loess from the Krems-Wachtberg site (Lower Austria). Abh. Geol. Bundesanst. 62, 153–157.
Händel, M., Simon, U., Einwögerer, T., and Neugebauer-Maresch, C. (2009). Loess deposits and the conservation of the archaeological record—the Krems-Wachtberg example. Quat. Int. 198, 46–50. doi:10.1016/j.quaint.2008.07.005
Heller, F., and Tung-sheng, L. (1986). Palaeoclimatic and sedimentary history from magnetic susceptibility of loess in China. Geophys. Res. Lett. 13, 1169–1172. doi:10.1029/GL013i011p01169
Hunt, C. P., Banerjee, S. K., Han, J., Solheid, P. A., Oches, E., Sun, W., et al. (1995). Rock-magnetic proxies of climate change in the loess-palaeosol sequences of the western Loess Plateau of China. Geophys. J. Int. 123, 232–244. doi:10.1111/j.1365-246X.1995.tb06672.x
Hus, J. J. (2003). The magnetic fabric of some loess/palaeosol deposits. Phys. Chem. Earth, Parts A/B/C. 28, 689–699. doi:10.1016/S1474-7065(03)00128-1
Hyodo, M., Sano, T., Matsumoto, M., Seto, Y., Bradák, B., Suzuki, K., et al. (2020). Nanosized authigenic magnetite and hematite particles in mature-paleosol phyllosilicates: new evidence for a magnetic enhancement mechanism in loess sequences of China. J. Geophys. Res. Solid Earth. 125, e2019JB018705. doi:10.1029/2019JB018705
Jipa, D. C. (2014). The conceptual sedimentary model of the Lower Danube loess basin: sedimentogenetic implications. Quat. Int. 351, 14–24. doi:10.1016/j.quaint.2013.06.008
Jordanova, D., Hus, J., and Geeraerts, R. (2007a). Palaeoclimatic implications of the magnetic record from loess/palaeosol sequence Viatovo (NE Bulgaria): palaeoclimatic implications of the magnetic record. Geophys. J. Int. 171, 1036–1047. doi:10.1111/j.1365-246X.2007.03576.x
Jordanova, D., Jordanova, N., Henry, B., Hus, J., Bascou, J., Funaki, M., et al. (2007b). Changes in mean magnetic susceptibility and its anisotropy of rock samples as a result of alternating field demagnetization. Earth Planet Sci. Lett. 255, 390–401. doi:10.1016/j.epsl.2006.12.025
Krauß, L., Zens, J., Zeeden, C., Schulte, P., Eckmeier, E., and Lehmkuhl, F. (2016). A multi-proxy analysis of two loess-paleosol sequences in the northern Harz foreland, Germany. Palaeogeogr. Palaeoclimatol. Palaeoecol. 461, 401–417. doi:10.1016/j.palaeo.2016.09.001
Kühn, P., Aguilar, J., Miedema, R., and Bronnikova, M. (2018). “Chapter 14-textural pedofeatures and related horizons,” in Interpretation of micromorphological features of soils and regoliths. 2nd Edn, Editors F. Mees, G. Stoops, and V. Marcelino (Elsevier), 377–423.
Lagroix, F., and Banerjee, S. K. (2002). Paleowind directions from the magnetic fabric of loess profiles in central Alaska. Earth Planet Sci. Lett. 195, 99–112. doi:10.1016/S0012-821X(01)00564-7
Lancaster, N. (2020). On the formation of desert loess. Quat. Res. 96, 1–18. doi:10.1017/qua.2020.33
Lehmkuhl, F., Bösken, J., Hošek, J., Sprafke, T., Marković, S. B., Obreht, I., et al. (2018). Loess distribution and related quaternary sediments in the Carpathian Basin. J. Maps. 14, 673–682. doi:10.1080/17445647.2018.1526720
Lehmkuhl, F., Zens, J., Krauß, L., Schulte, P., and Kels, H. (2016). Loess-paleosol sequences at the northern European loess belt in Germany: distribution, geomorphology and stratigraphy. Quat. Sci. Rev. 153, 11–30. doi:10.1016/j.quascirev.2016.10.008
Liu, Q., Deng, C., Yu, Y., Torrent, J., Jackson, M. J., Banerjee, S. K., et al. (2005). Temperature dependence of magnetic susceptibility in an argon environment: implications for pedogenesis of Chinese loess/palaeosols. Geophys. J. Int. 161, 102–112. doi:10.1111/j.1365-246X.2005.02564.x
Liu, Q., Roberts, A. P., Larrasoaña, J. C., Banerjee, S. K., Guyodo, Y., Tauxe, L., et al. (2012). Environmental magnetism: principles and applications. Rev. Geophys. 50, 1–50. doi:10.1029/2012RG000393
Liu, X., Xu, T., and Liu, T. (1988). The Chinese loess in Xifeng, II. A study of anisotropy of magnetic susceptibility of loess from Xifeng. Geophys. J. Int. 92, 349–353. doi:10.1111/j.1365-246X.1988.tb01147.x
Maher, B. A. (2011). The magnetic properties of Quaternary aeolian dusts and sediments, and their palaeoclimatic significance. Aeolian Res. 3, 87–144. doi:10.1016/j.aeolia.2011.01.005
Martı́n-Hernández, F., and Hirt, A. M. (2003). The anisotropy of magnetic susceptibility in biotite, muscovite and chlorite single crystals. Tectonophysics 367, 13–28. doi:10.1016/S0040-1951(03)00127-6
Mason, J. A. (2001). Transport direction of peoria loess in Nebraska and implications for loess sources on the central great plains. Quat. Res. 56, 79–86. doi:10.1006/qres.2001.2250
Meyer-Heintze, S., Sprafke, T., Schulte, P., Terhorst, B., Lomax, J., Fuchs, M., et al. (2018). The MIS 3/2 transition in a new loess profile at Krems-Wachtberg East—a multi-methodological approach. Quat. Int. 464, 370–385. doi:10.1016/j.quaint.2017.11.048
Moine, O., Antoine, P., Hatté, C., Landais, A., Mathieu, J., Prud’homme, C., et al. (2017). The impact of Last Glacial climate variability in west-European loess revealed by radiocarbon dating of fossil earthworm granules. Proc. Natl. Acad. Sci. U.S.A. 114, 6209. doi:10.1073/pnas.1614751114 |
Necula, C., and Panaiotu, C. (2012). Rock magnetic properties of a loess-paleosols complex from Mircea Voda (Romania). Rom. Rep. Phys. 64, 516–527.
Neugebauer-Maresch, C., Hambach, U., and Anghelinu, M. (2014). Loess and the record of upper palaeolithic cultures in the Danube Basin. Quat. Int. 351, 1–4. doi:10.1016/j.quaint.2014.10.041
Nigst, P. R. (2006). “The first modern humans in the middle Danube area?: new evidence from Willendorf II (eastern Austria),” in When neanderthals and modern humans met. (Kerns), 269–304.
Nigst, P. R., Haesaerts, P., Damblon, F., Frank-Fellner, C., Mallol, C., Viola, B., et al. (2014). Early modern human settlement of Europe north of the Alps occurred 43,500 years ago in a cold steppe-type environment. Proc. Natl. Acad. Sci. U.S.A. 111, 14394–14399. doi:10.1073/pnas.1412201111 |
Nigst, P. R., Viola, T. B., Haesaerts, P., Blockley, S., Damblon, F., Frank, C., et al. (2008). New research on the Aurignacian of Central Europe: a first note on the 2006 fieldwork at Willendorf II. Quartar. 55, 9–15. doi:10.7485/QU55_01
Obreht, I., Hambach, U., Veres, D., Zeeden, C., Bösken, J., Stevens, T., et al. (2017). Shift of large-scale atmospheric systems over Europe during late MIS 3 and implications for Modern Human dispersal. Sci. Rep. 7, 5848. doi:10.1038/s41598-017-06285-x |
Obreht, I., Zeeden, C., Hambach, U., Veres, D., Marković, S. B., and Lehmkuhl, F. (2019). A critical reevaluation of palaeoclimate proxy records from loess in the Carpathian Basin. Earth Sci. Rev. 190, 498–520. doi:10.1016/j.earscirev.2019.01.020
Oches, E. A., and Banerjee, S. K. (1996). Rock-magnetic proxies of climate change from loess -paleosol sediments of the Czech Republic. Stud. Geophys. Geod. 40, 287–300. doi:10.1007/BF02300744
Oldknow, C. J., Oldfield, F., Carr, A. S., Hooke, J. M., Biggin, A., Boyle, J. F., et al. (2020). Palustrine wetland formation during the MIS 3 interstadial: implications for preserved alluvial records in the South African Karoo. Sediment. Geol. 405, 105698. doi:10.1016/j.sedgeo.2020.105698
Reinders, J., and Hambach, U. (1995). A geomagnetic event recorded in loess deposits of the Tönchesberg (Germany): identification of the Blake magnetic polarity episode. Geophys. J. Int. 122, 407–418. doi:10.1111/j.1365-246X.1995.tb07004.x
Rousseau, D.-D., Sima, A., Antoine, P., Hatté, C., Lang, A., and Zöller, L. (2007). Link between European and North Atlantic abrupt climate changes over the last glaciation. Geophys. Res. Lett. 34, L22713. doi:10.1029/2007GL031716
Schulte, P., and Lehmkuhl, F. (2018). The difference of two laser diffraction patterns as an indicator for post-depositional grain size reduction in loess-paleosol sequences. Palaeogeogr. Palaeoclimatol. Palaeoecol. 509, 126–136. doi:10.1016/j.palaeo.2017.02.022
Shi, C., Zhu, R., Glass, B. P., Liu, Q., Zeman, A., and Suchy, V. (2003). Climate variations since the last interglacial recorded in Czech loess. Geophys. Res. Lett. 30, 1562. doi:10.1029/2003GL017251
Smalley, I., O’Hara-Dhand, K., Wint, J., Machalett, B., Jary, Z., and Jefferson, I. (2009). Rivers and loess: the significance of long river transportation in the complex event-sequence approach to loess deposit formation. Quat. Int. 198, 7–18. doi:10.1016/j.quaint.2008.06.009
Smalley, I. J. (1972). The interaction of great rivers and large deposits of primary loess. Trans. N. Y. Acad. Sci. 34, 534–542.
Song, Y., Fang, X., Torii, M., Ishikawa, N., Li, J., and An, Z. (2007). Late Neogene rock magnetic record of climatic variation from Chinese eolian sediments related to uplift of the Tibetan Plateau. J. Asian Earth Sci. 30, 324–332. doi:10.1016/j.jseaes.2006.10.004
Song, Y., Shi, Z., Fang, X., Nie, J., Naoto, I., Qiang, X., et al. (2010). Loess magnetic properties in the ili basin and their correlation with the Chinese Loess Plateau. Sci. China Earth Sci. 53, 419–431. doi:10.1007/s11430-010-0011-5
Sprafke, T., Schulte, P., Meyer-Heintze, S., Händel, M., Einwögerer, T., Simon, U., et al. (2020). Paleoenvironments from robust loess stratigraphy using high-resolution color and grain-size data of the last glacial Krems-Wachtberg record (NE Austria). Quat. Sci. Rev. 248, 106602. doi:10.1016/j.quascirev.2020.106602
Sprafke, T., Terhorst, B., Peticzka, R., and Thiel, C. (2013). Paudorf locus typicus (Lower Austria) revisited–The potential of the classic loess outcrop for Middle to Late Pleistocene landscape reconstructions. Quat. Sci. J. EG. 62, 59–72. doi:10.3285/eg.62.1.06
Tarling, D., and Hrouda, F. (1993). Magnetic anisotropy of rocks. London, Glasgow, New York, Tokyo, Melbourne, Madras: Springer Science and Business Media.
Taylor, S. N., and Lagroix, F. (2015). Magnetic anisotropy reveals the depositional and postdepositional history of a loess-paleosol sequence at Nussloch (Germany). J. Geophys. Res. Solid Earth. 120, 2859–2876. doi:10.1002/2014JB011803
Taylor, S. N., Lagroix, F., Rousseau, D.-D., and Antoine, P. (2014). Mineral magnetic characterization of the Upper Pleniglacial Nussloch loess sequence (Germany): an insight into local environmental processes. Geophys. J. Int. 199, 1463–1480. doi:10.1093/gji/ggu331
Terhorst, B., Kühn, P., Damm, B., Hambach, U., Meyer-Heintze, S., and Sedov, S. (2013). Paleoenvironmental fluctuations as recorded in the loess-paleosol sequence of the Upper Paleolithic site Krems-Wachtberg. Quat. Int. 351, 67. doi:10.1016/j.quaint.2013.03.045
Terhorst, B., Sedov, S., Sprafke, T., Peticzka, R., Meyer-Heintze, S., Kühn, P., et al. (2015). Austrian MIS 3/2 loess–palaeosol records—key sites along a west–east transect. Palaeogeogr. Palaeoclimatol. Palaeoecol. 418, 43–56. doi:10.1016/j.palaeo.2014.10.020
Újvári, G., Varga, A., Raucsik, B., and Kovács, J. (2014). The Paks loess-paleosol sequence: a record of chemical weathering and provenance for the last 800ka in the mid-Carpathian Basin. Quat. Int. 319, 22–37. doi:10.1016/j.quaint.2012.04.004
Wang, R., and Løvlie, R. (2010). Subaerial and subaqueous deposition of loess: experimental assessment of detrital remanent magnetization in Chinese loess. Earth Planet Sci. Lett. 298, 394–404. doi:10.1016/j.epsl.2010.08.019
Yang, S., and Ding, Z. (2014). A 249 kyr stack of eight loess grain size records from northern China documenting millennial-scale climate variability. G-cubed. 15, 798–814. doi:10.1002/2013GC005113
Yu, L., and Oldfield, F. (1993). Quantitative sediment source ascription using magnetic measurements in a reservoir-catchment system near Nijar, S.E. Spain. Earth Surf. Process. Landforms. 18, 441–454. doi:10.1002/esp.3290180506
Zeeden, C., Hambach, U., and Händel, M. (2015). Loess magnetic fabric of the Krems-Wachtberg archaeological site. Quat. Int. 372, 188–194. doi:10.1016/j.quaint.2014.11.001
Zeeden, C., Hambach, U., Steguweit, L., and Anghelinu, M. (2011). Loess stratigraphy using palaeomagnetism: application to the Poiana Cireşului archaeological site (Romania). Quat. Int. 240, 100–107. doi:10.1016/j.quaint.2010.08.018
Zeeden, C., Hambach, U., Steguweit, L., Fülling, A., Anghelinu, M., and Zöller, L. (2009). Using the relative intensity variation of the Earth’s magnetic palaeofield as correlative dating technique: a case study from loess with Upper Palaeolithic cultural layers at Poiana Cireşului, Romania. Quartar. 56, 175–185. doi:10.7485/QU56_10
Zeeden, C., Hambach, U., Veres, D., Fitzsimmons, K., Obreht, I., Bösken, J., et al. (2018). Millennial scale climate oscillations recorded in the Lower Danube loess over the last glacial period. Palaeogeogr. Palaeoclimatol. Palaeoecol. 509, 164–181. doi:10.1016/j.palaeo.2016.12.029
Zeeden, C., Kels, H., Hambach, U., Schulte, P., Protze, J., Eckmeier, E., et al. (2016). Three climatic cycles recorded in a loess-palaeosol sequence at Semlac (Romania)—implications for dust accumulation in south-eastern Europe. Quat. Sci. Rev. 154, 130–142. doi:10.1016/j.quascirev.2016.11.002
Zheng, H., Oldfield, F., Yu, L., Shaw, J., and An, Z. (1991). The magnetic properties of particle-sized samples from the Luo Chuan loess section: evidence for pedogenesis. Phys. Earth Planet. In. 68, 250–258. doi:10.1016/0031-9201(91)90044-I
Keywords: environmental magnetism, geoarchaeology, sedimentology, loess, magnetic fabric (AMS), magnetic fabric and anisotropy, rock magnetic analyses
Citation: Zeeden C and Hambach U (2021) Magnetic Susceptibility Properties of Loess From the Willendorf Archaeological Site: Implications for the Syn/Post-Depositional Interpretation of Magnetic Fabric. Front. Earth Sci. 8:599491. doi: 10.3389/feart.2020.599491
Received: 27 August 2020; Accepted: 16 December 2020;
Published: 12 February 2021.
Edited by:
Barbara Mauz, University of Salzburg, AustriaReviewed by:
Chris Oldknow, University of Liverpool, United KingdomMaodu Yan, Institute of Tibetan Plateau Research (CAS), China
Copyright © 2021 Zeeden and Hambach. This is an open-access article distributed under the terms of the Creative Commons Attribution License (CC BY). The use, distribution or reproduction in other forums is permitted, provided the original author(s) and the copyright owner(s) are credited and that the original publication in this journal is cited, in accordance with accepted academic practice. No use, distribution or reproduction is permitted which does not comply with these terms.
*Correspondence: Christian Zeeden, Y2hyaXN0aWFuLnplZWRlbkBsZWlibml6LWxpYWcuZGU=