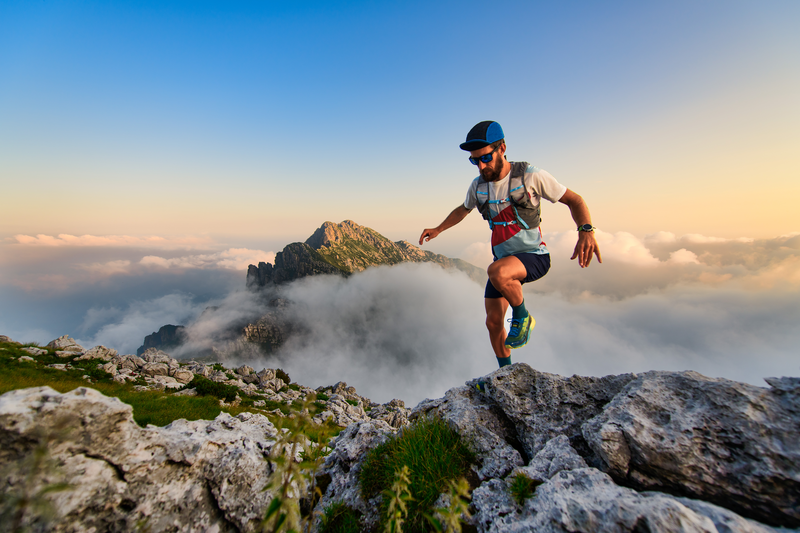
95% of researchers rate our articles as excellent or good
Learn more about the work of our research integrity team to safeguard the quality of each article we publish.
Find out more
ORIGINAL RESEARCH article
Front. Earth Sci. , 21 December 2020
Sec. Hydrosphere
Volume 8 - 2020 | https://doi.org/10.3389/feart.2020.528632
Gully Consolidation and Highland Protection (GCHP) Project is a major soil and water conservation and land remediation project implemented in the Chinese Loess Plateau (CLP). As the connection between the mechanisms of erosion and practical applications for addressing it is not clear, the implementation of engineering measures to combat the problem has been insufficient to date. This study used field investigation and descriptive statistics, together with hydrological analysis modeling to gain an understanding of the impact of the Loess Plateau Highland Control Schemes on the evolution of the Dongzhi Plateau as the largest, most well-preserved, and the thickest loess deposit region in China. A remote sensing image was introduced to hydrological modeling to prove the analysis results of the Dongzhi Plateau. According to these investigations and analysis, four major schemes of gully head retrogressive erosion control were summarized and a comprehensive theory and technology based on a watershed were proposed. After hydrological analysis, the Dongzhi Plateau was divided into 1225 watersheds. It was found that GCHP should be implemented in the catchment area based on hydrological analysis to solve the problem of retrogressive erosion, and it is recommended that a scientific and rational drainage system should be designed based on the roads and pipe networks in the whole watershed area. Findings from this paper provide insights into the evolution of CLP and it can give a good suggestion on the future implementation of GHCP.
The Loess Plateaus are rich in coals, petroleum, bauxite and other resources which are thus a major base for energy and chemical engineering (Li et al., 2020). Loess is widely distributed all over the world, including in Europe (Jefferson et al., 2003a; Haase et al., 2007), North America (Bettis et al., 2003; Muhs et al., 2014), Asia (Liu, 1965; Murton et al., 2015; Mu et al., 2020), South America (Zárate, 2003), North Africa (Crouvi et al.,2010), and other locations (Eden and Hammond, 2003). Loess, which accounts for one tenth of the global land area, is intermittently distributed in forest grasslands, grasslands, and desert grasslands in the mid-latitudes of the northern and southern hemispheres. Loess systems are a key component of the delivery of many ecosystems, including those that are crucial to water and nutrient cycling. However, Loess systems are facing threats from soil erosion as a result of climate change and human engineering, which is a cause of worldwide concern (e.g., Van Andel et al., 1990; Butzer, 2005; Dreibrodt et al., 2010a; Starkloff and Stolte, 2014; Zuffetti et al., 2018; Mirzaee et al., 2020). Soil erosion brings disastrous consequences to local agricultural production and socio-economic development, affecting people's lives and property, and posing a significant threat to safety.
Loess erosion is a major environmental issue that has been addressed in many studies (e.g., Berhe et al., 2018; Jiang et al., 2019; Juang et al., 2019). Using two GIS-based machine-learning approaches, the analytical hierarchy process and fuzzy logic modeling, Saha et al. (2019) mapped the susceptibility of soil erosion in the Kunur River Basin in West Bengal, India. Through field investigations and laboratory experiments, Zhuang et al. (2020) revealed the process of internal erosion and the effects of water infiltration on the undisturbed loess. Shi et al. (2020) conducted a series of rainfall combined scouring experiments on experimental plots to clarify the influence of gully heads and the erosion process on hydraulic parameters under different flow discharges. Amir and Jonathan (2020) analyzed the characteristics of the gully area, examining the pattern of soil, biological activity, agricultural utilization, topography, and geomorphology based on five separate geomorphological profiles from nearby landforms, and determined the impact of the gully erosion process on the ecosystem. As a result of soil erosion, a large number of soil resources are eroded and destroyed (Kayet et al., 2018) and large areas of land are being cut to pieces, resulting in a decline in productivity (Sanchez, 2002) with a significant impact on crop yields (Baptista et al., 2015) and ecosystem quality (Fujaco et al., 2016). In addition, this process causes the silting of dams and reservoirs, which affects their use and development (Balthazar et al., 2013). Borrelli et al. (2017) outline that the impact of soil erosion is expected to worsen over the next few decades due to rapid population growth, rapid deforestation, agricultural intensification, and climate change.
The Chinese Loess Plateau (CLP) is the largest concentrated area of loess in China. Because fine and soft loess are abundant in mineral nutrients and favorable for farming, the Loess Plateau was the cradle of ancient Chinese culture and civilization (Peng et al., 2014). The CLP is highly prone to natural hazards, such as floods, bank collapses, runoff, and sometimes landslides, due to the fragile geological environment and extreme rainfall events, as well as intense human activities (e.g., Luo et al., 2018; Peng et al., 2018a; Guo et al., 2020a; Guo et al., 2020b). For example, the Dongzhi Plateau is the largest and most well-preserved loess highland, with the thickest loess deposition (about 200 m thickness) in the Loess Plateau, but it is also the political, economic, and cultural center of Qingyang, located to the east of Qingyang City in the Gansu Province (Peng et al., 2018a). As the largest residual loess highland, over the past few decades there has been geomorphologic evolution and soil erosion in the area (Xiong et al., 2014; Goldberg et al., 2019). However, Dongzhi Plateau has been prone to severe soil erosion since ancient times (Qiu et al., 2014; Peng et al., 2017), and some eroded gullies have spread to urban areas. Investigation shows that up to 23,292 km2 of Qingyang, about 86% of its total area, is experiencing the soil erosion. The average soil erosion modulus is above 7200 tons and locally up to 10,000 tons. The annual loess amount flowing into the Yellow River is 0.1684 billion tons, which is approximately one tenth of the total soil amount flowing into the Yellow River and one third of the soil amount flowing into the Yellow River in Gansu (Qiu et al., 2014; Feng et al., 2019). It is thus imperative to protect the highland.
Efforts to control soil erosion in the CLP have been progressing for a long time (Kukla, 1987; Zhang et al.,2020; Ulrich et al., 1980). Early control strategies were targeted at gully slopes and gully valleys by building terraces and planting trees in slopes and constructing checking dams at gully valleys, known as the gully-slope combined prevention and control technique. This philosophy of small-watershed comprehensive control was promoted in the 1990s, when the slope surface and gully valley combined control method was more comprehensively and profoundly applied (Thomas et al., 2009; Tsoar and Pye, 2010; Reigstad et al., 2011; Qiu et al., 2014). In the past 60 years, owing to long-term research and exploration by the Department of Water Resources of the Ministry of Agriculture and Rural Affairs, and other relevant research departments, the concept of “Gully solidification–slope protection–highland conservation” became clearer, and a series of comprehensive soil–water conservation control strategies were put forward (Ulrich et al., 1980; Cui et al., 2008; Williams, 2008; Peng et al., 2018). For instance, after over 60 years of exploration, the Xifeng Soil and Water Conservation Experimental Station proposed a three-line defense control scheme involving “highland surface runoff regulation and control, slope vegetation restoration, and gully channel water-sand accumulation”, which showed good preliminary results as part of the Gully Consolidation and Highland Protection (GCHP) project (Myers, 1948; Schreiber, 1987; Feng et al., 2019). In 2008, targeting the Yanwachuan watershed, the Yanwachuan Station put forward a polyatomic comprehensive control scheme based on the runoff regulation and control. This scheme was centered on highland surface runoff regulation, control, and utilization, and involved gully channel runoff and soil regulation and control, and it was supplemented by gully–slope runoff regulation, and constructed an efficient runoff soil regulation and use system (Heller and Tung-Sheng, 1986; Guo et al., 2020c). In order to implement the scheme, the Qingyang Municipal Government had organized a themed deployment on GCHP in 2014. This key soil-water conservation and land renovation project targeted the exclusive geomorphology and severe soil erosion in the loess highlands and had no precedent, starting with early-stage soil and water conservation (Nordmeyer and Richter, 1985; Pathak et al., 2016). Although it offers useful data and experience accumulation for GCHP projects, it lacks loess erosion/invasion mechanisms and engineering data on geology; as a result, this scheme is still insufficient for the ongoing GCHP projects. At present, the project of Gully Consolidation and Highland Protection (GCHP) is speeding up in the CLP. The government mainly uses the methods of the GCHP to prevent the gully head from advancing. This is a strong method of artificial intervention that consolidates the gullies and protects the highlands. However, not enough attention has been paid to the problems of GCHP, for example, most measures of the current GCHP projects have only focused on small-scale control (e.g., only gully head), and ignored the large- or medium-scale (e.g., watershed) feedback effects. On the temporal scale, these are short-term engineering control effects that ignore medium- or long-term effects. For instance, the retrogressive eroded gully heads that are sheltered are replaced by new gully heads (Blankespoor et al., 2012; Merten et al., 2015; Leng et al., 2017). After the gully heads are under control, new gully heads have tended to emerge after several rainfall events. Therefore, further studies on the spatial and temporal distribution, characteristics, dynamic processes, and control factors of soil and water disasters under different measures are urgently required to protect the tableland in gullies from a historical, regular, and systematic perspective to elucidate understanding of the mechanisms and process of loess erosion, especially headcut erosion and its potential to induce disasters (Guo et al., 2020d).
As a result of the aforementioned issues of soil erosion in the CLP, GCHP projects are critically important. The is study summarizes GCHP engineering schemes and analyzes these existing problems in detail, aiming to enhance the GCHP by using a hydrological analysis method that will: 1) investigate the capability of GCHP engineering schemes along with drainage systems to forecast discharge and find improvements; 2) reconstruct a catchment and drainage system based on reasonable road and pipe networks, and propose comprehensive and scientific schemes according to prevention/control theories and techniques; and, 3) undertake multivariate prediction of GCHP via hydrological Analysis and enhance its capability by the proposed pre-processing approach. The CLP encompasses cities along the route of the area covered under the “One Belt One Road” (OBOR) initiative of the Chinese government, a global infrastructure development strategy along ancient trade routes in Central Asia. When soil erosion occurs in the CLP it affects the development of cities and can even cause their ruins. The results of this study will provide technological support for the major project of GCHP and contribute to the safety of “One Belt One Road” and other important national strategies.
The Xifeng District of Qingyang, Gansu province, China, is located at the hinterland of the Dongzhi Plateau (E 107°27′42″-107°52′48″, N 35°25′55″-35°51′11″; Figure 1). It is 47.7 km long and 34.8 km wide from east to west, and the total area is 996.35 km2. The Dongzhi Plateau is located in the CLP and is a typical large loess highland. Xifeng is the political, economic, and cultural center of Qingyang. The Dongzhi Plateau is a natural green agricultural production base and is often referred to as the “barn of east Gansu” and the “pearl of Gansu”. The total area covered by the Dongzhi Plateau is 812 km2, about 3.3% of the total area of Qingyang, and the total cultivated land area is 71,520 ha. The highlands are flat and slightly inclined southeastward. The north-south length is 110 km, and the east-west width varies from 50 km to 50 m. It is located inland in an arid and semiarid zone distant from oceans. It enjoys a temperature of 8.6°C and precipitation of 480–660 mm on average annually. The precipitation varies greatly from region to region and is generally larger in the southeast than in the northwest. Precipitation also varies unevenly throughout the year occurring largely from May to September.
FIGURE 1. Study areas. Map showing the surrounded hydro-geomorphology and the general situation of the stream network. DEM: digital elevation model; RS: remote sensing.
Due to the special geographical location and topographic conditions of the Dongzhi Plateau, a hydrogeological unit with independent supply and drainage conditions was formed in Dongzhi Plateau, and the only supply source of phreatic water was atmospheric precipitation. The groundwater in Dongzhi Plateau generally flows from the highland center with a high water level to the edges, but the runoff rate is very slow. The horizontal penetration velocity of the aquifer layer is between 0.02 and 2.01 m/d, and the drainage of phreatic water is divided into spring water natural drainage, and artificial exploitation (Raynaud et al., 2015; Huo et al., 2016; Xu et al., 2017).
The vegetation in the highland is dominated by crops, and the vegetation distribution is consistent with the spatial precipitation quantity distribution, as the vegetation grows well in the southern wet zone but is sparse in the northern arid zone (Meserve and Glanz, 1978). The soils in the Dongzhi Plateau are dominated by dark loessial soil, which is basically consistent and insignificantly different from south to north. No river has developed there, but the east, south, west, and north sides are cut by the Malian River, Jing River, Pu River, and Caijiamiao gully, and multiple branches of the Jiaozi River, respectively. The relative height difference between the rivers and the highland surface varies between 250 and 300 m. The area surrounding the Dongzhi Plateau is cut and eroded by a number of small branch rivers. The Malian River, located in the east of Dongzhi Plateau, is the largest in Qingyang. The Pu River, located in the west of Dongzhi Plateau, is a first-level branch of the Jing River.
We conducted field visits and surveys for about 2 months between July 13 and September 30, 2018, and the surveyed areas included Xifeng District, Zhengning, Heshui, and Qingcheng counties. We surveyed the advancing gully heads in detail, considering behaviors in recent years together with the Qingyang GCHP projects that have been implemented and offered by the local Water Conservation Bureau as well as statistics such as local meteorology, runoffs, social economy, water, and soil loss areas (Figure 1). Through remote sensing, unmanned aerial vehicles (UAVs), field surveys, and other methods, the topography, geomorphology, and incoming water conditions in the areas surrounding gully heads were investigated and analyzed in detail (Dc 1–13 in Figure 1). From these investigations, we clarified the types and rules of GCHP in the Dongzhi Plateau as well as the possible influence of human engineering. Moreover, the confluence at the gully heads was observed during precipitation, and the runoffs at the catchments were analyzed (Li et al., 1995). The sampling frequency for the runoff was once every 5 min, and finally, daily averages were used.
A hydrological analysis is a method of geoscience statistical analysis (Huo et al., 2012). Here, the geographic information system (ArcGIS 9.3) and regional hydrological analysis were combined (Huo et al., 2011) using QuickBird image, spatial resolution was as high as 0.61 m and temporal resolution was from 1 to 6 days. Based on the image processing function of ArcGIS, a regional digital elevation model (DEM) was producted. Aster satellite used (STER GDEM V2: http://www.gdem.aster.ersdac.or.jp/search.js) this spatial resolution and the final DEM resolution was 30 m. Firstly, its depressions were filled in. Then, based on its surface analysis function, the slopes, aspects, streamlines, and watershed identification were analyzed to divide the different catchment areas. The processing chain is summarized in Figure 2. After the extraction of these basic hydrological factors and the basic hydrological analysis, we reproduced the water flowing processes on the DEM surfaces, and analyzed the origin and flow directions of the surface streams. Then, more hydrological information (including slope and watershed unit division) was acquired from the DEM, and finally, the regional hydrological analytical process was finished.
The hydrological analysis was mainly conducted by the D8 algorithm (O'Callaghan and David, 1984), which is the most widely used watershed feature extraction technique based on flow analysis and confluence analysis (Peng et al., 2018; Huo et al., 2019a; Luo et al., 2020). This algorithm first assumes that each rainfall event falls into one cell on the topography, and among the 8 surrounding cells, the water in this cell flows to the cell with the lowest terrain. If the maximum dropping directions are the same among several cells, the adjacent cell extent is enlarged until the steepest dropping direction is found. This algorithm consists of 3 procedures: 1) flow analysis: the flow direction of each cell is expressed by a numerical value, which varies from 1 to 255 (where 1: east; 2: southeast; 4: south; 8: southwest; 16: west; 32: northwest; 64: north; 128: northeast); 2) computation of water flow accumulation, which mainly aims to clarify flow routes (confluence grids are generated based on flow cells); and, (3) extraction of watershed features, with the watershed confluence cells, the different characteristic parameters of watersheds can be easily extracted (e.g., the watershed water system can be simulated to determine different characteristic parameters, including river length, slopes, watershed area, and watershed ratio). Although the D8 algorithm is a common method, 30 m resolution DEM was used for hydrological analysis in this paper. We mainly use the method of rolling-over DEM. The catchment area generated twice was overlaid and analyzed in ArcGIS 9.3, which can further generate typical catchment units with the same geomorphological features. In this study, the scope, area, and watershed of every erosion of the gully were located and calculated by watershed hydrological analysis (Figure 3). Then, through hydrological analysis, the Xifeng District was divided into 1225 catchment zones, and the largest zone with an area of 6.063 km2 was numbered 509, and No. 365 was the minimum (0.101 km2).
A hydrological analysis is a major method of geostatistical analysis (Huo et al., 2019b; Luo et al., 2019; Guo et al., 2020e). Based on the hydrological analysis function of GIS, the slopes, aspects, streamlines, and watershed identification in the DEM of the Dongzhi Plateau were analyzed and divided into different catchment areas (Figure 3). From the aspect of slope distribution, the slope of the Dongzhi Plateau is gentle, and the slope of both gully sides is steep. Erosion activity is the strongest in the direction of the gully head. After hydrological analysis, the Dongzhi Plateau was divided into 1225 catchment zones (or sub-watersheds), and each zone had a runoff outlet. Table 1 shows the geostatistical variables of 10 larger sub-watersheds. For instance, catchment zone No. 509 was the largest (6.063 km2). Thus, the runoffs generated in catchment zone No. 509 would flow to the erosion gullies in this zone, causing highland surface erosion and gully channel retrogressive erosion. Therefore, the control measures that only targeted gully heads could not also solve the runoff confluence in the catchment zones.
The red line in Figure 3 shows the boundary range of the catchment area, and the whole Xifeng District is separated by multiple catchment areas of different sizes. The blue line shows the flow path, and each flow path is surrounded by corresponding catchment areas with a total of 488 channels. The terrain of the study area is characterized by high in the middle and low around. The highest value is 1445.74 m, which is shown in red and the lowest value is 984.575 m, which is shown in blue. Therefore, the results of hydrological analysis results accurately show the flow path and the size and scope of the catchment area in the study area.
The water runoff and confluence process after precipitation can be reconstructed on the surface of the DEM through hydrological analysis, and the catchment boundary of each gully can be distinguished. If the catchment area is superimposed with the remote sensing (RS) image, the precise boundary on the RS image of the catchment area can be obtained, which provides technical support for GCHP. This process also requires high-precision DEM, which means that the higher the DEM precision, the more accurate the boundary of the catchment. Figure 3 shows the Huoxiang and Fengjiapan gullies, which are two typical catchment areas. It can be seen that the boundary of the catchment area at the gully head is calculated through hydrological analysis. Therefore, the focus of governance should be on catchment areas rather than being limited to the gully head region.
The gully head images were hydrologically analyzed in ArcGIS. The resulting catchment zones are illustrated in Figure 4. The hydrological analysis showed that Huoxiang gully (Figure 4A) was located in catchment zone No. 648, with an area of 4868 m2, and Fengjiapan gully (Figure 4B) was located in catchment zone No. 1165, with an area of 528,604 m2. The runoffs generated in the catchment zones converged and flowed to Huoxiang gully or Fengjiapan gully, washing highland surfaces and invading gully heads.
The overlying map of remote sensing image and the shape of hydrological analysis reflects the degree of coincidence between the scope of the catchment area and the flow path, especially whether the boundary of the catchment area is consistent with the actual landform. The field investigation and remote sensing image interpretation found that the two results were consistent.
It can be seen from Figure 4A that the Huoxiang gully head invades the urban area and is close to the center of the urban area. The gully catchment area is almost made up of urban buildings. Road hardening accelerates the concentration process of rainwater, causing the gully head to retreat. This puts higher requirements on the scientific planning of the urban drainage network. As can be seen in Figure 4B, the catchment area of the gully head of Fengjiapan is mainly composed of farmland, and the hydraulic conductivity is larger than that of urban areas; the confluence process in the catchment area is relatively slow. However, with long-term development, the farmland land area will be reduced; thus, the gully erosion will continue to develop and will gradually reach villages and towns.
On-site surveys showed that 80% of the current GCHP measures were delaying the retreat of the gully channel and protecting the highland surfaces. However, during the GCHP measurements in the gully heads taken after precipitation, signs of new erosion appeared. Due to the wet collapsibility of loess, new gully heads developed at the sides of the filled gully heads (Figure 5), a predictable outcome for these GCHP engineering measures, as it was likely that they would be damaged by runoff sooner or later.
Figure 5 shows the catchment phenomena affecting different gully head areas after a GCHP precipitation event. In Figure 5A shows the phenomenon of accumulated water and submerged erosion at the outlet of the catchment area, where a large reservoir accumulates at the gully head when it rains. In Figure 5B, the gully head backfill project is shown however, once new water accumulation and submerged erosion phenomena appear on its side after precipitation, it forms a deep undercut, i.e., a new gully head expansion process occurs. Figure 5C shows the new gully head expansion process after precipitation. It is important not to underestimate these small gully heads, as they will grow into huge gully heads after a hundred years of frequent heavy rain events. Figure 5D presents the comparison of runoff processes in hardened and non-hardened areas, indicating that the runoff process on a hardened land surface is much faster than that of a non-hardened land surface under the same precipitation conditions. The blue part in Figure 5D is the loess area, and the rest is the road hardening area. Precipitation easily infiltrates in the loess area, and it is not easy to form runoff. After the road hardens, it is not easy to infiltrate, and the rainwater accumulates on the surface (known as the deposit process), meaning it easily forms runoff. The larger the runoff, the greater the erosion of loess in the gully.
In conclusion, after the implementation of the GCHP project, once there is rain, the rainwater will form runoff, which will be collected in the gully head area. Therefore, the implementation of GCHP cannot be confined to the gully head only and should be implemented in the catchment area based on hydrological analysis to solve the fundamental problem of retrogressive erosion.
This study proposes an alternative approach, known as the “natural GCHP project management method”, which is based on the natural runoff path, channel cascade closure, and the reasonable construction of a reservoir on the highland surface to effectively reduce erosion and prevent runoff from flowing into the gullies. This method builds upon a long history of experiences and knowledge of this location. Breaking up the whole process into parts and focusing on the use of natural processes in the catchment area, the project proposes the construction of a “thousand pools and hundred lakes”. The lakes and pools of this CLP conservation project should be located at the points where the rainwater accumulates, taking the reconstruction and expansion of Beihu, Xihu, Dongjiaohu, and Donghu Park in Qingyang City as the main axis (the detailed location of the lakes was shown in Figure 3), the landscape of “Pinghu Plateau” can be utilized. At the same time, the ultimate goal is to create harmony between the ecological conservation plateau and human settlement. By gathering rainwater deposits, the plateau lakes can reduce runoffs into the gully heads, which will avoid the erosion of Loess by runoff, turning harm into benefit and waste to our advantage.
Retrogressive erosion means that the valley stretches towards the source direction and is one of the main causes of soil erosion in loess areas. If the gully head is not protected, it will continue to move forward, which could destroy traffic infrastructure, industry and factories, and residential areas, causing the city to shrink and possibly even divide into two parts.
According to investigations and analysis, there are four major schemes for gully head retrogressive erosion control, including gully head simple landfill (the first scheme); drainage+landfill (the second scheme); drainage+landfill+engineering slope protection (the third scheme); and drainage+landfill+engineering slope protection+ecological slope protection (the last scheme). A representative picture of each scheme is shown in Figure 6. Figure 6A is a simple landfill type. Figure 6B has drainage blind pipes under the landfill body and a drainage channel on the surface. Figure 6C adds engineering slope protection measures onto the surface of the slope. Figure 6D adds ecological slope protection measures on the surface of the slope body. The characteristics and problems of each scheme are listed in Table 2.
The differences between these schemes are as follows: the first gully head scheme is distributed in the widest area. These areas are mainly distributed outside the city and cover sparsely populated areas, accounting for about 80% of the total area of the region. Because of its simple structure and low cost, it is mostly distributed in ecological space, far away from towns and agricultural areas (Wang et al., 2019). Based on the first scheme, the second scheme builds a horizontal drainage channel and discharges the runoff at the rear of the confluence into the ditch, which can prevent and control the erosion of the surface by runoff to a certain extent, but the cost is higher than the first scheme. The third scheme is based on the second scheme, adding engineering measures for slope protection, which can intercept rainfall, weaken splash erosion, and better prevent the loss of landfill soil, and the cost is higher (Monsieurs et al., 2015). The cost of the last scheme is higher than that of the other three schemes because of its high level, long cycle of ecological measures, and slow effect, along with the fact that it is mostly distributed in urban space, and mainly protects residential areas from the threat of natural hazards caused by hydraulic erosion (Li et al., 2015; Guo et al., 2020). The interaction between plants and soil can protect and reinforce the surface of slopes, which effectively reduces splashes, wind erosion, soil stability, and degradation of organic pollutants (Valentin et al., 2005; Li et al., 2015).
In recent decades, GCHP has been explored and developed continuously, and proposed approaches include edge-protection of the plateau, waterlogging ponds, planting forest and grass, warping dams, and checking dams, all of which are important measures in controlling retrogressive erosion and reducing soil and water loss in the CLP area. However, due to lack of the systematic understanding of the overall ecological environment of the CLP and lack of comprehensive understanding of soil and water dynamics, especially the erosion mechanism of the CLP under the synergistic action of soil and water dynamics and human activities, the erosion problems that affect the plateau area have not been comprehensively controlled and solved, and retrogressive erosion in some areas has further intensified. For example, the hydrological analysis of Dongzhi Plateau highland surfaces clearly shows that landfilling and drainage measures that focus only on the gully heads cannot block runoff convergence at the catchment zones. After precipitation, water accumulation phenomenon will appear at the different positions of the gully heads where the GCHP project interventions have been implemented, as shown in Figure 5. If there are no drainage measures, when there is heavy rain a large amount of rainwater will gather there because this is generally the lowest part of the watershed), forming new ponding pools (Figure 5A), drop holes (Figure 5B), and erosion gullies (Figure 5C).
Despite the large investment and long duration of GCHP modes, it is important to address that they are short term solutions and that these methods have slow effects in terms of soil and water conservation, and that there are still serious threats to safety in residential areas and cities. To alleviate the above problems, the government has mobilized communities and resources in the construction of physical soil and water conservation structures (stone bunds, terraces) in almost all land uses. However, any improvement was mainly concentrated within the vicinity of the structures, and runoff continued overtop structures, as no measures for in situ soil conservation were taken (Tewodros et al., 2008). In addition, the Chinese local government has mainly adopted the method of gully head land backfilling to control and prevent gullies from advancing. However, the problem of strong manual ditch protection for the backfilling of the gully head has not attracted enough attention, such as the potential engineering catastrophes caused by a change of the soil and water environment on the artificial backfilling surface. Therefore, there is an urgent need for research into the characteristics of the temporal and spatial distribution, dynamic processes, and control factors of water and soil catastrophes that have affected different gully conservation measures from the perspectives of history, regularity, and the system, and to clarify the mechanism and process of loess erosion, especially retrogressive and suffusion erosion.
It is a good measure for GCHP to use the hydrologic analysis method. The hydrological analysis method based on DEM uses hydrological analysis tools to extract the flow direction, the flow accumulation, flow length, and the river network (including the classification of the river network, the hydrologic analysis tool based on DEM can automatically extract about 1175 tributaries.) of the surface water runoff model and watershed segmentation of the study area (Figure 3). The advantage of the hydrological analysis method is that, through the extraction of basic hydrological factors and analysis, the flow process of water can be reproduced on the surface of DEM, which provides technical support for the engineering measures of GCHP. For example, the path of surface runoff and the boundary of the catchment can be determined by hydrological analysis.
Based on the hydrological analysis, the retrogressive erosion control schemes developed traditional control schemes which are limited in erosion areas, such as the gully head and erosion slope, expanding the spatial scale of control areas i.e., extending the scope of the control region to the watershed. Therefore, the control schemes of the CLP based on hydrological analysis is necessary to be fully understood and deeply applied to fundamentally solve the problem of retrogression erosion in the plateau surface, solve the problem of soil and water loss in the CLP, and finally achieve the ultimate goal of GCHP.
We here put forward some recommendations and key points for controlling erosion.
(1) Based on the hydrological analysis, systematic and science-based drainage/catchment systems should be established at catchment zones. For instance, the roads and pipe networks can be effectively utilized to build terraces, which would prevent the surface water erosion from washing away from highland surfaces and avoid the development of erosion gullies (Figure 7).
(2) The “thousand pools and hundred lakes” engineering construction project should be considered and implemented, as efficient drainage systems are needed and should ideally be combined with catchment systems. The “thousand pools and hundred lakes project” will enable the collection and use of catchment water and comprehensive drainage measures should be carried out in urban, rural-urban, and rural-residential areas.
(3) A natural rain and flood runoff collection/use project should be established to shift approaches to water collection from drainage to collection. The scientific and reasonable implementation of this project would alleviate the erosive damage of rain and flooding in the gully heads around Qingyang and reduce urban geohazards.
(4) Gully head protection systems, dominated by flood discharge pipes, should be built at suburban gully heads. To address issues related to eroded gully heads at urban flood drainage outlets on highland edges, the catchment area and drainage quantities should be scientifically measured and estimated to enable a subsequent unified and planned design. In addition, the standards of prevention and control should be raised. Engineering measures should be dominant and assist through biological prevention and control measures. From a long-term and overall perspective, urban soil/water conservation, and protection systems should be built, GCHP measures need to be strengthened, and the suburban eco-environment should be improved.
A weakness of this study is that it lacked data on the long-term and large-scale prediction of the project. These engineering recommendations based on hydrological analysis have not been implemented on a large scale, lack practical experience, and it is uncertain whether there may be potential harm in large-scale implementation, which needs to be further examined. The GCHP project was proposed in China relatively late (and officially started in 2018), and there is no international precedent. However, at present, the GCHP ecological management project is one of the fastest and most effective solutions to secondary disasters. It is, therefore, necessary to test and explore the project implementation as soon as possible, which will provide practical solutions through problem-solving and enable researchers to accumulate experience and further information. We think the future research should carry the longterm (50-100 years) and watershed hydrological analysis of GCHP.
Through field investigation and hydrological analysis, this study has established that the GCHP project is effective on a small space-time scale, but that its effectiveness over a longer space-time scale needs further study. Based on the information discussed here, the present study has several main conclusions:
(1) This study proposes four common control models of gully head retrogressive erosion. The four models are: gully head landfill; drainage+landfill; drainage+landfill+engineering slope protection; and drainage+landfill+engineering slope protection+ecological slope protection. Among them, the effect of the fourth governance mode was relatively good.
(2) Aiming to solve the existing problems of gully head treatment, this study observed that hydrological analysis should be carried out from the perspective of the river basin, and the treatment scheme should be determined based on hydrological analysis. The road and pipe network can be used to managing cascade drainage, and potentially reduce the erosion of surface water on the highland, and prevent the development of erosion gullies.
(3) This paper puts forward the natural GCHP. The core content of the project is to establish a natural rainwater and flood runoff collection and utilization project to realize a transformation in approach from discharge to storage. The scientific and reasonable implementation of this project could reduce the damage of rainfall and flooding to the surrounding area of the city and the erosion damage of the gully heads, reducing the occurrence of urban geological disasters.
All datasets generated for this study are included in the article/Supplementary Material.
AH wrote the original draft of this manuscript. JP and PL designed the manuscript. YC and CZ undertook the analysis of data. PL and ZZ revised the manuscript.
This work was supported by the National Natural Science Foundation of China (Grant Nos. 41672255, 41877232, 41402255, and 41790444); the National Key R&D Program of China (2018YFE0103800); and through Fundamental Research Funds for the Central Universities, CHD (Grant Nos. 300102268202, 300102299302, and 300102299201),International Collaborative Research of Disaster Prevention Research Institute of Kyoto University(2019W-02), Excellent projects for science and technology activities of overseas staff in Shaanxi Province (2018038).
The authors declare that the research was conducted in the absence of any commercial or financial relationships that could be construed as a potential conflict of interest.
Thanks to the Soil and Water Conservation Bureau of Qingyang, Gansu Province for their great help and support in our outdoor field inspections and the installation and monitoring of instruments.
Amir, M.-M., and Jonathan, B. L. (2020). The effects of gully erosion on the ecology of arid loessial agro-ecosystems, the northern Negev, Israel. Catena 194, 104712. doi:10.1016/j.catena.2020.104712
Balthazar, V., Vanacker, V., Girma, A., Poesen, J., and Golla, G. (2013). Human impact on sediment fluxes within the blue nile and atbara river basins. Geomorphology 180–181, 231–241. doi:10.1016/j.geomorph.2012.10.013
Baptista, I., Ritsema, C., and Geissen, V. (2015). Effect of integrated water-nutrient management strategies on soil erosion mediated nutrient loss and crop productivity in cabo verde drylands. PloS One 10, e0134244. doi:10.1371/journal.pone.0134244
Berhe, A. A., Barnes, R. T., Six, J., and Marín-Spiotta, E. (2018). Role of soil erosion in biogeochemical cycling of essential elements: carbon, nitrogen, and phosphorus. Annu. Rev. Earth Planet. Sci. 46, 521–548. doi:10.1146/annurev-earth-082517-010018
Bettis, E. A., Muhs, D. R., Roberts, H. M., and Wintle, A. G. (2003). Last glacial loess in the conterminous USA. Quat. Sci. Rev. 22 (18–19), 1907–1946. doi:10.1016/s0277-3791(03)00169-0
Blankespoor, B., Basist, A., Dinar, A., and Dinar, S. (2012). Assessing economic and political impacts of hydrological variability on treaties: case studies on the zambezi and mekong basins. Policy Res. Working Pap. 34 (224), 259–282. doi:10.1596/1813-9450-5996
Borrelli, P., Robinson, D. A., Fleischer, L. R., Lugato, E., Ballabio, C., Alewell, C., et al. (2017). An assessment of the global impact of 21st century land use change on soil erosion. Nat. Commun. 8, 2013. doi:10.1038/s41467-017-02142-7
Butzer, K. W. (2005). Environmental history in the Mediterranean world: cross-disciplinary investigation of cause-and-effect for degradation and soil erosion. J. Archaeol. Sci. 32, 1773–1800. doi:10.1016/j.jas.2005.06.001
Crouvi, O., Amit, R., Enzel, Y., and Gillespie, A. R. (2010). Active sand seas and the formation of desert loess. Quat. Sci. Rev. 29, 2087–2098. doi:10.1016/j.quascirev.2010.04.026
Cui, P., Wang, D. J., Fan, J. R., Wang, Y. K., He, X. B., Zhu, B., et al. (2008). Current status and comprehensive control strategies of soil erosion for the upper Yangtze and other rivers in the Southwestern China. Sci. Soil Water Conserv. 6, 43–50. doi:10.16843/j.sswc.2008.01.008
Dreibrodt, S., Lubos, C., Terhorst, B., Damm, B., and Bork, H. R. (2010a). Historical soil erosion by water in Germany: scales and archives, chronology, research perspectives. Quat. Int. 222, 80–95. doi:10.1016/j.quaint.2009.06.014
Eden, D. N., and Hammond, A. P. (2003). Dust accumulation in the New Zealand region since the last glacial maximum. Quat. Sci. Rev. 22, 2037–2052. doi:10.1016/s0277-3791(03)00168-9
Feng, T., Wei, W., Chen, L., Cerdà, A., Yang, L., and Yu, Y. (2019). Combining land preparation and vegetation restoration for optimal soil eco-hydrological services in the Loess Plateau, China. Sci. Total Environ. 657, 535–547. doi:10.1016/j.scitotenv.2018.11.476
Fujaco, M. A. G., Leite, M. G. P., and Neves, A. H. C. J. (2016). A gis-based tool for estimating soil loss in agricultural river basins. Geociências. Int. Eng. J. 69, 417–424. doi:10.1590/0370-44672015690197
Goldberg, J. A., Bildsten, L., and Paxton, B. (2019). Inferring explosion properties from type II-plateau supernova light curves. Astrophys. J. 879, 3. doi:10.3847/1538-4357/ab22b6
Guo, B., Su, Y., Pei, L., Wang, X., Wei, X., Zhang, B., et al. (2020e). Contamination, distribution and health risk assessment of risk elements in topsoil for amusement parks in Xi’an, China. Pol. J. Environ. Stud. 30, 1–17. doi:10.15244/pjoes/123606
Guo, B., Su, Y., Pei, L., Wang, X., Zhang, B., Zhang, D., et al. (2020b). Ecological risk evaluation and source apportionment of heavy metals in park playgrounds: a case study in Xi'an, Shaanxi province, a northwest city of China. Environ. Sci. Pollut. Res. 27 (19), 24400–24412. doi:10.1007/s11356-020-08744-x
Guo, B., Wang, X., Pei, L., Su, Y., Zhang, D., and Wang, Y. (2020d). Identifying the spatiotemporal dynamic of PM2.5 concentrations at multiple scales using geographically and temporally weighted regression model across China during 2015–2018. Sci. Total Environ. 751, 141765. doi:10.1016/j.scitotenv.2020.141765
Guo, B., Wang, X., Zhang, D., Pei, L., Zhang, D., and Wang, X. (2020c). A land use regression application into simulating spatial distribution characteristics of particulate matter (PM2.5) concentration in city of Xi’an, China. Pol. J. Environ. Stud. 29 (6), 4065–4076. doi:10.15244/pjoes/118426
Guo, B., Zhang, D., Zhang, D., Su, Y., Wang, X., and Bian, Y. (2020a). Detecting spatiotemporal dynamic of regional electric consumption using NPP-VIIRS nighttime stable light data case study of Xi’an, China. IEEE Access 8, 171694–171702. doi:10.1109/ACCESS.2020.3025013
Guo, M. M., Wang, W. L., Wang, T. C., Wang, W. X., and Kang, H. L. (2020). Impacts of different vegetation restoration options on gully head soil resistance and soil erosion in loess tablelands. Earth Surf. Processes Landforms 45, 1038–1050. doi:10.1002/esp.4798
Haase, D., Fink, J., Haase, G., Ruske, R., Pécsi, M., Richter, H., et al. (2007). Loess in Europe—its spatial distribution based on a European Loess Map, scale 1:2,500,000. Quat. Sci. Rev. 26, 1301–1312. doi:10.1016/j.quascirev.2007.02.003
Heller, F., and Tung-Sheng, L. (1986). Palaeoclimatic and sedimentary history from magnetic susceptibility of loess in China. Geophys. Res. Lett. 13, 1169–1172. doi:10.1029/gl013i011p01169
Huo, A., Chen, X., Li, H., Hou, M., and Hou, X. (2011). Development and testing of a remote sensing-based model for estimating groundwater levels in aeolian desert areas of China. Can. J. Soil Sci. 91, 29–37. doi:10.4141/cjss10044
Huo, A., Huang, Z., Cheng, Y., and Van Liew, M. W. (2019a). Comparison of two different approaches for sensitivity analysis in Heihe River basin (China). Water Sci. Technol. Supply 20 (1), 319–327. doi:10.2166/ws.2019.159
Huo, A., Peng, J., Chen, X., Lin, D., Wang, G., and Cheng, Y. (2016). Groundwater storage and depletion trends in the Loess areas of China. Environ. Earth Sci. 75, 1167. doi:10.1007/s12665-016-5951-4
Huo, A., Wang, X., Liang, Y., Jiang, C., and Zheng, X. (2019b). Integrated numerical model for irrigated area water resources management. J. Water Clim. Change. doi:10.2166/wcc.2019.042
Huo, A., Zhang, H., Li, Z., Hua, L., and Ming, H. (2012). A sampled method of classification of susceptibility evaluation unit for geological hazards based on GIS. Appl. Math. Inf. Sci. 6, 19S–23S.
Jefferson, I. F., Evstatiev, D., Karastanev, D., Mavlyanova, N. G., and Smalley, I. J. (2003a). Engineering geology of loess and loess-like deposits: a commentary on the Russian literature. Eng. Geol. 68, 333–351. doi:10.1016/s0013-7952(02)00236-3
Jiang, C., Zhang, H. Y., Wang, X. C., Feng, Y. Q., and Labzovskii, L. (2019). Challenging the land degradation in China's Loess Plateau: benefits, limitations, sustainability, and adaptive strategies of soil and water conservation. Ecol. Eng. 127, 135–150. doi:10.1016/j.ecoleng.2018.11.018
Juang, C. H., Dijkstra, T., Wasowski, J., and Meng, X. M. (2019). Loess geohazards research in China: advances and challenges for mega engineering projects. Eng. Geol. 251, 1–10. doi:10.1016/j.enggeo.2019.01.019
Kayet, N., Pathak, K., Chakrabarty, A., and Sahoo, S. (2018). Evaluation of soil loss estimation using the RUSLE model and SCS-CN method in hillslope mining areas. Int. Soil Water Conserv. Res. 6, 31–42. doi:10.1016/j.iswcr.2017.11.002
Kukla, G. (1987). Loess stratigraphy in central China. Palaeogeogr. Palaeoclimatol. Palaeoecol. 72, 203–225. 10.1016/0031-0182(89)90143-0
CrossRef Full Text |Leng, Y., Peng, J., Wang, Q., Meng, Z., and Huang, W. (2017). A fluidized landslide occurred in the Loess Plateau: a study on loess landslide in South Jingyang tableland. Eng. Geol. 236, 129–136. doi:10.1016/j.enggeo.2017.05.006
Li, F., Zhao, S., Duan, S., University, T., and Lanzhou, (1995). Preliminary study on limited irrigation for spring wheat field in semi-arid region of loess plateau. Chin. J. Appl. Ecol. 6, 259–264. doi:10.13287/j.1001-9332.1995.005
Li, Y., Shi, W., Aydin, A., Beroya-Eitner, M. A., and Gao, G. (2020). Loess genesis and worldwide distribution. Earth-Sci. Rev. 201, 102947. doi:10.1016/j.earscirev.2019.102947
Li, Z., Zhang, Y., Zhu, Q. K., He, Y. M., and Yao, W. (2015). Assessment of bank gully development and vegetation coverage on the Chinese Loess Plateau. Geomorphology 228, 462–469. doi:10.1016/j.geomorph.2014.10.005
Luo, H., Wu, F., Chang, J., and Xu, J. (2018). Microstructural constraints on geotechnical properties of Malan Loess: a case study from Zhaojiaan landslide in Shaanxi province, China. Eng. Geol. 236, 60–69. doi:10.1016/j.enggeo.2017.11.002
Luo, P., Kang, S., Apip, M. Z., Lyu, J., Aisyah, S., Binaya, M., et al. (2019). Water quality trend assessment in Jakarta: a rapidly growing Asian megacity. PloS One 14 (7), e0219009. doi:10.1371/journal.pone.0219009
Luo, P., Sun, Y., Wang, S., Wang, S., Lyu, J., Zhou, M., et al. (2020). Historical assessment and future sustainability challenges of Egyptian water resources management. J. Cleaner Prod. 263, 121154. doi:10.1016/j.jclepro.2020.121154
Merten, G. H., Araújo, A. G., Biscaia, R. C. M., Barbosa, G. M. C., and Conte, O. (2015). No-till surface runoff and soil losses in southern Brazil. Soil Tillage Res. 152, 85–93. doi:10.1016/j.still.2015.03.014
Meserve, P. L., and Glanz, W. E. (1978). Geographical ecology of small mammals in the northern Chilean arid zone. J. Biogeogr. 5, 135–148. doi:10.2307/3038168
Mirzaee, S., Ghorbani-Dashtaki, S., and Kerry, R. (2020). Comparison of a spatial, spatial and hybrid methods for predicting inter-rill and rill soil sensitivity to erosion at the field scale. Catena 188, 104439. doi:10.1016/j.catena.2019.104439
Monsieurs, E., Poesen, J., Dessie, M., Adgo, E., Verhoest, N. E. C., Deckers, J., et al. (2015). Effects of drainage ditches and stone bunds on topographical thresholds for gully head development in North Ethiopia. Geomorphology 234, 193–203. doi:10.1016/j.geomorph.2015.01.011
Mu, D., Luo, P., Lyu, J., Zhou, M., Huo, A., Duan, W., et al. (2020). Impact of temporal rainfall patterns on flash floods in Hue City, Vietnam. J. Flood Risk Manage. 20, e12668. doi:10.1111/jfr3.12668
Muhs, D. R., Cattle, S. R., Crouvi, O., Rousseau, D. D., Sun, J. M., and Zárate, M. A. (2014). “Loess records,” in Mineral dust: a key player in the earth system. Editors P. Knippertz, and J. W. Stuut (Berlin: Springer Verlag), 411–441.
Murton, J. B., Goslar, T., Edwards, M. E., Bateman, M. D., Danilov, P. P., Savvinov, G. N., et al. (2015). Palaeoenvironmental interpretation of yedoma silt (ice complex) deposition as cold-climate loess, Duvanny Yar, northeast Siberia. Permafr. Periglac. Process. 26, 208–288. doi:10.1002/ppp.1843
Myers, O. H. (1948). The consolidation and protection OF ancient monuments OF the Sudan. Sudan Notes and Records 29 (2), 206–217.
Nordmeyer, H., and Richter, J. (1985). Incubation experiments on nitrogen mineralization in loess and sandy soils. Plant. Soil 83, 433–445. doi:10.1007/bf02184455
O'Callaghan, J. F., and David, M. M. (1984). The extraction of drainage networks from digital elevation data. Comput. Vis. Graph. Image Process 28, 323–344. doi:10.1016/s0734-189x(84)80011-0
Pathak, P., Chandrasekhar, K., Wani, S. P., Sudi, R. R., and Budama, N. (2016). Integrated runoff and soil loss monitoring unit for small agricultural watersheds. Comput. Electron. Agric. 128, 50–57. doi:10.1016/j.compag.2016.08.011
Peng, J., Huo, A., Cheng, Y., Dang, J., Wei, H., Wang, X., et al. (2017). Submersion simulation in a typical debris flow watershed of Jianzhuangchuan catchment, Loess Plateau. Environ. Earth Sci. 76, 462. doi:10.1007/s12665-017-6797-0
Peng, J., Lin, H., Wang, Q., Zhuang, J., Cheng, Y., and Zhu, X. (2014). The critical issues and creative concepts in mitigation research of loess geological hazards. J. Eng Geol. 22, 684–691
Peng, J., Ping, S., Igwe, O., and Li, X. A. (2018). Loess caves, a special kind of geo-hazard on loess plateau, northwestern China. Eng. Geol. 236, 79–88. doi:10.1016/j.enggeo.2017.08.012
Peng, J., Tong, X., Wang, S., and Ma, P. (2018a). Three-dimensional geological structures and sliding factors and modes of loess landslides. Environ. Earth Sci. 77, 675. doi:10.1007/s12665-018-7863-y
Qiu, H. J., Cui, P., Cao, M. M., Liu, W., and Wang, Y. M. (2014). Distribution of frequency-size of geological disaster base on principle of maximum entropy in loess plateau. Rock. Mech. 35, 3541–3549. doi:10.16285/j.rsm.2014.12.003
Raynaud, D., Thielen, J., Salamon, P., Burek, P., Anquetin, S., and Alfieri, L. (2015). A dynamic runoff co‐efficient to improve flash flood early warning in Europe: evaluation on the 2013 central European floods in Germany. Meteorol. Appl. 22, 410–418. doi:10.1002/met.1469
Reigstad, L. J., Jorgensen, S. L., Lauritzen, S. E., Schleper, C., and Urich, T. (2011). Sulfur-oxidizing chemolithotrophic proteobacteria dominate the microbiota in high arctic thermal springs on Svalbard. Astrobiology 11, 665. doi:10.1089/ast.2010.0551
Saha, S., Gayen, A., Pourghasemi, H. R., and Tiefenbacher, J. P. (2019). Identification of soil erosion-susceptible areas using fuzzy logic and analytical hierarchy process modeling in an agricultural watershed of Burdwan district, India. Environ. Earth Sci. 78, 649. doi:10.1007/s12665-019-8658-5
Sanchez, P. A. (2002). Soil fertility and hunger in Africa. Science 295, 2019–2020. doi:10.1126/science.1065256
Schreiber, K. J. (1987). Conquest and consolidation: a comparison of the wari and inka occupations of a highland Peruvian valley. American Antiquity 52, 266. doi:10.2307/281780
Shi, Q., Wang, W., Guo, M., Chen, Z., Feng, L., Zhao, M., et al. (2020). The impact of flow discharge on the hydraulic characteristics of headcut erosion processes in the gully region of the Loess Plateau. Hydrol. Processes 34, 718–729. doi:10.1002/hyp.13620
Starkloff, T., and Stolte, J. (2014). Applied comparison of the erosion risk models EROSION 3D and LISEM for a small catchment in Norway. Catena 118, 154–167. doi:10.1016/j.catena.2014.02.004
Tewodros, G., Jan, N., Bram, G., Fekadu, G., Mintesinot, B., Haile, M., et al. (2008). Contour furrows for in situ soil and water conservation, Tigray, Northern Ethiopia. Soil Tillage Res. 103, 257–264. 10.1016/j.still.2008.05.021
Thomas, J. T., Iverson, N. R., and Burkart, M. R. (2009). Bank‐collapse processes in a valley‐bottom gully, western Iowa. Earth Surf. Process. Landf. 34, 109–122. doi:10.1002/esp.1699
Tsoar, H., and Pye, K. (2010). Dust transport and the question of desert loess formation. Sedimentology 34, 139–153. doi:10.1111/j.1365-3091.1987.tb00566.x
Ulrich, B., Mayer, R., and Khanna, P. K. (1980). Chemical changes due to acid precipitation in a loess-derived soil in central Europe. Soil Sci. 130, 193–199. doi:10.1097/00010694-198010000-00005
Valentin, C., Poesen, J., and Li, Y. (2005). Gully erosion: impacts, factors and control. Catena 63, 132–153. doi:10.1016/j.catena.2005.06.001
Van Andel, T., Zangger, E., and Demitrack, A. (1990). Land use and soil erosion in Prehistoric and Historical Greece. J. Field Archaeol 17, 379–396. doi:10.1179/009346990791548628
Wang, X. F., Huo, A. D., Zhu, X. H., Zhao, Y. B., Jiang, C., and Zheng, X. L. (2019). Study on governance mode of Gully Consolidation and Highland Protection Project in east Gansu. Yellow River 41, 106–109. doi:10.3969/j.issn.1000-1379.2019.09.022
Williams, M. A. (2008). Regional environmental agreements and initiatives in the americas. Revista Geologica De Chile 18, 65–95. doi:10.1093/yiel/18.1.65
Xiong, L. Y., Tang, G. A., Li, F. Y., Yuan, B. Y., and Lu, Z. C. (2014). Modeling the evolution of loess-covered landforms in the Loess Plateauof China using a DEM of underground bedrock surface. Geomorphology 209, 18–26. doi:10.1016/j.geomorph.2013.12.009
Xu, L., Coop, M. R., Zhang, M., and Wang, G. (2017). The mechanics of a saturated silty loess and implications for landslides. Eng. Geol. 236, 29–42. doi:10.1016/j.enggeo.2017.02.021
Zárate, M. A. (2003). Loess of southern south America. Quat. Sci. Rev. 22. 1987–2006. doi:10.1016/s0277-3791(03)00165-3
Zhang, Y., Luo, P., Zhao, S., Kang, S., Wang, P., Zhou, M., et al. (2020). Control and remediation methods for eutrophic lakes in recent 30 years. Water Sci. and Technol. 81, 1099–1113. doi:10.2166/wst.2020.218
Zhuang, J., Peng, J., Zhu, Y., Leng, Y., Zhu, X., and Huang, W. (2020). The internal erosion process and effects of undisturbed loess due to water infiltration. Landslides 240, 1–10. doi:10.1007/s10346-020-01518-z
Keywords: hydrological analysis, control schemes, drainage system, dongzhi plateau, gully consolidation and highland protection
Citation: Huo A, Peng J, Cheng Y, Luo P, Zhao Z and Zheng C (2020) Hydrological Analysis of Loess Plateau Highland Control Schemes in Dongzhi Plateau. Front. Earth Sci. 8:528632. doi: 10.3389/feart.2020.528632
Received: 21 January 2020; Accepted: 19 November 2020;
Published: 21 December 2020.
Edited by:
Frédéric Frappart, Laboratoire d'études en géophysique et océanographie spatiales (LEGOS), FranceReviewed by:
Artemi Cerdà, University of Valencia, SpainCopyright © 2020 Huo, Peng, Cheng, Luo Zhao and Zheng. This is an open-access article distributed under the terms of the Creative Commons Attribution License (CC BY). The use, distribution or reproduction in other forums is permitted, provided the original author(s) and the copyright owner(s) are credited and that the original publication in this journal is cited, in accordance with accepted academic practice. No use, distribution or reproduction is permitted which does not comply with these terms.
*Correspondence: Jianbing Peng, ZGljZXh5XzJAY2hkLmVkdS5jbg==; Pingping Luo, bHBwQGNoZC5lZHUuY24=;
Disclaimer: All claims expressed in this article are solely those of the authors and do not necessarily represent those of their affiliated organizations, or those of the publisher, the editors and the reviewers. Any product that may be evaluated in this article or claim that may be made by its manufacturer is not guaranteed or endorsed by the publisher.
Research integrity at Frontiers
Learn more about the work of our research integrity team to safeguard the quality of each article we publish.