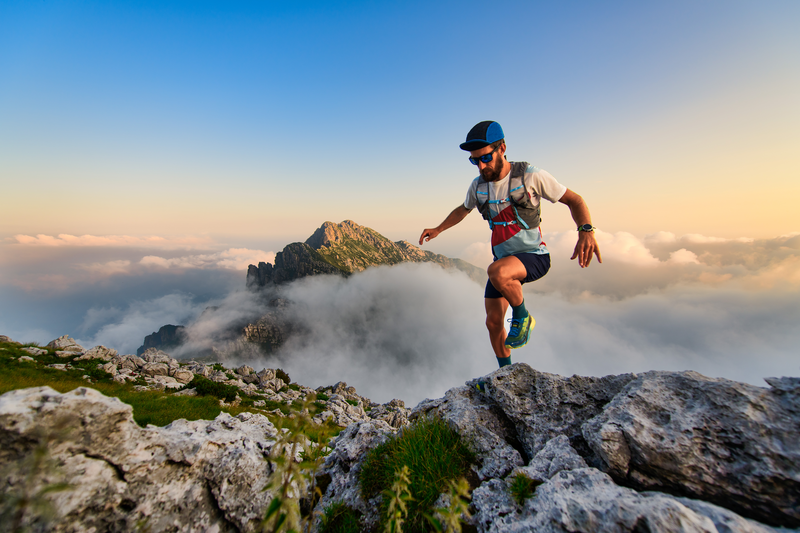
94% of researchers rate our articles as excellent or good
Learn more about the work of our research integrity team to safeguard the quality of each article we publish.
Find out more
OPINION article
Front. Earth Sci. , 21 February 2020
Sec. Quaternary Science, Geomorphology and Paleoenvironment
Volume 8 - 2020 | https://doi.org/10.3389/feart.2020.00038
Studies of marine sediments with a view to reconstruct ancient climates are based on multiple and complementary approaches. In particular, powerful thermometers of ancient oceanic waters have been developed based on micropalaeontological transfer functions, on the chemistry of compound-specific organic molecules produced by various unicellular organisms, and trace metals substituted to calcium in calcite microfossils. Despite the diversity of palaeoceanographic tools or proxies that have been developed, the geochemistry of planktonic foraminifera is arguably the most widely used. More than 60 years after the advent of the oxygen isotope (δ18O) proxy in palaeoceanography (Urey, 1947; Emiliani, 1955), the isotopic analyses of calcite produced by these single-celled organisms remain the most applied tool to constrain variations in ice volume and oceanic temperatures across geological times. The relative easiness to extract the shells of the foraminifera, to clean and to isotopically measure with a mass-spectrometer explains the dominance of foraminifera-based studies in the literature (Minoletti et al., 2009). Yet, other key information pertaining to physical and chemical properties of surface oceanic waters, and especially the carbonate chemistry, are inaccessible from the foraminiferal archive. This contribution will make the case that the combined study of foraminifera and coccoliths preserved in sedimentary successions –thus combining an animal and a vegetal archive– has the potential to substantially improve the proxies on which palaeoceanographic research relies.
Foraminifera consume particulate organic carbon present in seawater to sustain growth and replication. They build their shells under biological control from dissolved inorganic carbon (DIC) contained in vacuolized seawater (Erez, 2003). As a consequence, they primarily utilize bicarbonate ions –the dominant DIC species in seawater– to biomineralise. Most of the published literature based on the coccolith record relies on the use of palaeoecological transfer functions (McIntyre et al., 1970; CLIMAP Project Members, 1976; Giraudeau and Pujos, 1990; Kameo et al., 2004; Hernández-Almeida et al., 2019, among many others). Calcareous nannofossils have been, by far, less used as a source of palaeoenvironmental information than planktonic foraminifera. The coccoliths are more challenging to study. Indeed, coccoliths are micron-sized particles in the 2–20 microns range that are too small to be extracted from sediments through hand-picking under the binocular microscope. Coccolithophores are marine phytoplankton that thrive exclusively in sunlit oceanic waters. They source carbon from the external milieu in the forms of dissolved inorganic carbon, primarily under the form of aqueous CO2 through passive diffusion. The formation of their calcite biominerals, the coccoliths, occurs entirely intracellularly. This fundamental biogeochemical difference in the sourcing of carbon between foraminifera and coccolithophores lead to appreciable consequences in the isotopic ratios of their calcite biominerals. Most coccolith species are precipitated out of isotopic equilibrium and therefore exhibit large compositional departures from the inorganic reference, a concept referred to as the vital effect (Weber and Woodhead, 1972; Weiner and Dove, 2003; Ziveri et al., 2003; Hermoso, 2014).
Beyond oceanic temperatures, knowledge of the carbonate chemistry of seawater (including the concentration of DIC & pH) allows the reconstruction of aqueous CO2 levels, which can be subsequently converted into atmospheric pCO2 estimates, provided SST, salinity and the local air-sea CO2 exchange rate are constrained. The growth of coccolithophores and the isotopic composition of their biominerals, are largely dependent on the availability of ambient CO2. The mechanistic and causal link between CO2 availability and the magnitude of the vital effects of the coccoliths reflects several intracellular controls by: (i) cell size; (ii) cellular growth rate (μ); and (iii) the relative allocation of the internal DIC pool to calcification and photosynthesis that might be approximated by their particulate inorganic to organic carbon ratio (PIC/POC ratio). Culture studies have provided decisive constraints on the changing intensity of the vital effect with the environment (Rickaby et al., 2010; Hermoso et al., 2014, 2016; McClelland et al., 2017). These biogeochemical controls are detailed in Hermoso (2014) and McClelland et al. (2017), and overall explain that the coccolith intrinsically bear valuable environmental information in their geochemistry (carbon and oxygen isotope systems), and in particular for retracing the evolution of [CO2aq].
Sedimentary studies on these questions were made possible by the ability to separate coccoliths from the sediments, and measure size-restricted assemblages taxonomically, morphometrically, and isotopically [e.g., protocol by Minoletti et al. (2009) and subsequent refinements]. The coccolith data are all the more insightful that the study of the coccoliths can be implemented in parallel with that of the contemporaneous surface-dwelling foraminifera, whose signal can provide both a reliable temperature signal and a more or less vital effect-free reference, thus allowing quantification of palaeo-vital effects of fossil coccoliths. The vital effect has been long seen as a “black box” problem in palaeoceanography and can now be used as a source of environmental information (Hermoso, 2016). Indeed, it is possible to derive pCO2 levels from the magnitude of the vital effect using the coccolith–foraminifera offset (Figure 1). In the absence of reliable foraminiferal records, it is also possible to use the relative vital effect using coccoliths of distinct sizes, a parameter that scales with ambient CO2 concentration and cell size (Tremblin et al., 2016).
Figure 1. Reconstruction of atmospheric pCO2 using the δ13C coccolith vital effect over glacial-interglacial fluctuations of the last 280 kyrs in the Caribbean Sea. (a) Shows the δ13C record of the planktonic foraminifera Globigerinoides sacculifer, which allows an oxygen isotope stratigraphy (MIS stands for Marine Isotope Stages). (b) Corresponds to the pCO2 record from the Vostok record (Petit et al., 1999). (c) Shows the foraminifera (white dots) and coccoliths (black dots) δ13C record. The offset between the two curves is used to quantify the coccolith vital effect. More details on the procedure can be read in Hermoso (2016). (d) is an attempt to derive new pCO2 from the δ13C vital effect of the coccolith Gephyrocapsa oceanica using calibration detailed in Hermoso (2016). (e) Quantifies the offsets between reconstructed and measured pCO2 levels. The source of the foraminiferal and coccolith records for this site is Anderson and Steinmetz (1981).
For the past 800 ka, atmospheric pCO2 levels have been measured directly, notably from the Vostok ice core (Petit et al., 1999). Over this time slice, the intensity of the vital effect in coccoliths can be used to derive the evolution of aqueous CO2 by applying empirical calibrations achieved in culture studies. Figure 1c shows the various isotopic record from the Caribbean Sea over the last 280 kyrs, including the δ13C foraminiferal record from Globigerinoides sacculifer, and that of the coccoliths Gephyrocapsa oceanica. The isotopic offset between the two signals (hence, δ13C G.oceanica – δ13C G.sacculifer) can be used to quantify the magnitude of the vital effect affecting the coccoliths. Using the biogeochemical calibration in Hermoso (2016), it becomes possible to reconstruct a new pCO2 curve (Figure 1d). This coccolith-derived pCO2 curve appears to be in good agreement (absolute values and glacial-interglacial fluctuations) with the direct measurements of atmospheric CO2 from the Vostok ice core (Figure 1b), with in a mean offset of ±40 ppm. This approach can be extended for periods predating the oldest Vostok measurement. It is further possible to quantify the offset between the atmospheric and seawater CO2 levels () during a time interval, which can offer the invaluable potential to constrain the dynamics of air-sea CO2 exchanges during a time interval. This disequilibrium has to be related with the biogeochemistry of the mesopelagic zone, and particularly the intensity of pelagic calcification with consequence on the alkalinity of the oceans. These latter concepts are crucial to understand the mechanisms behind the inception of the deglacial periods and cannot be constrained directly by other existing approaches. This chemical proxy can be complemented by the evolution in morphological parameters of the coccoliths, as outlined below.
For older geological periods, the coccolith isotopic composition can also provide new pCO2 estimates, taking advantage of the good preservation of these nannofossils in the sedimentary record. In addition, the coccolith record can complement and refine more classical approaches to reconstruct atmospheric pCO2 from CO2aq concentrations. Existing proxies include boron isotopes from foraminiferal shells and the alkenone-derived CO2 palaeobarometry (Pagani, 2014; Foster and Rae, 2016). In both cases, the cascade of calculations between measured carbon isotope composition of alkenones (δ13Calk) and final pCO2 estimates requires constraints on the δ13C of the DIC, and arguably that of the internal carbon pool of the coccolithophores. Existing alkenone-based studies currently make use of the foraminiferal record to this aim and it naturally emerges that the proxy will greatly benefit from the use of the coccolith δ13C records since both the organic and inorganic tracers derive from the same organism. Future culture studies of coccolithophores will have to establish new calibrations combining paired δ13Calk/δ13Ccalcite signals in response to changing ambient CO2 concentration to reduce uncertainties in the application of the proxy. Likewise, developing an organic–inorganic study of ancient coccolithophores can resolve uncertainties induced by underconstrained palaeo-growth rates (assembled within the b coefficient—see Zhang et al., 2013; Pagani, 2014), as well as the effect of possible carbon concentrating mechanisms on the isotopic composition of the intracellular DIC pool and eventually organic and inorganic substrates produced inside the cell (Stoll et al., 2019).
Several lines of evidence indicate that the expression of the vital effect affecting the isotopic composition of coccolith calcite is not species-specific or constant for a given species (Rickaby et al., 2010; Bolton and Stoll, 2013; Hermoso, 2014, 2015; Hermoso et al., 2015, 2016; McClelland et al., 2017; Jin et al., 2018). This feature is apparent in the data by Bolton et al. (2012), Bolton and Stoll (2013), Bolton et al. (2016), Tremblin et al. (2016) dealing with long term intervals in the Cenozoic, but also on more restricted time intervals in the Quaternary (Hermoso, 2016; Jin et al., 2018). A rather exploratory work was conducted from ancient published data from the Quaternary in the Caribbean Sea (Hermoso, 2016). It was shown that the magnitude of the carbon and oxygen vital effects follow the atmospheric CO2 fluctuations during glacial/interglacial periods, suggesting a causal link. A more comprehensive study was published subsequently by Jin et al. (2018). In this latter study, the authors tied coccolith morphometrics to the isotopic compositions across the glacial/interglacial cycles of the last 400 kyr, and reported strong covariations between coccolith size and the isotopic vital effects that they tentatively discussed in relation with the palaeo growth cellular rates.
A study by Beaufort et al. (2011) provided quantitative constraints on the environmental forcing on the calcification by the coccolithophores. Using the computational software called SYRACO (acronym in French standing for SYstème de Reconnaissance Automatique des COccolithes; Beaufort and Dollfus, 2004; Beaufort et al., 2014), it was possible to measure 2D (in the plane of the coccolith) parameters, such as the length, width and surface area, and 3D biometrics integrating coccolith thickness assessed from the birefringence color of calcite. From this 3D approach, it becomes possible to derive average calcite masses of individual coccoliths and estimate fluxes of coccolith calcite to the seafloor. The ambient carbonate chemistry ([]) was found to correlate with average mass of the coccoliths along a transect off the Peru Margin. Two subsequent SYRACO-based studies of Quaternary sediments have further established the potential of the morphometrics of the coccoliths (McClelland et al., 2016; Duchamp-Alphonse et al., 2018). To account for allometric changes in the aspect ratios of the coccoliths, the average mass and the coccolith 3D aspect ratio, representing the ratio between its thickness and the square root of its surface area, are the parameters of interest. Culture studies have established for various taxonomic ranks a correlation between the coccolith 3D aspect ratios, which can be measured on the fossil coccoliths, and cellular PIC/POC ratios, a parameter measured in culture studies, but resolvable from the fossil record. Overall, higher CO2 concentrations were registered with more heavily calcified coccoliths belonging to the Noëlaerhabdaceae family, and especially the species Gephyrocapsa oceanica (McClelland et al., 2016). A causal link between ambient carbon availability, growth rates, and cellular calcite quota was suggested. On longer time scales, the environmental forcing of declining pCO2 on less calcified coccoliths was also reported (Bolton et al., 2016). Increased coccosphere calcite quota was found during Heinrich Stadial 1 and the Younger Dryas and attributed to more elevated aqueous CO2 concentrations, also in the context of coeval changes of oceanic circulation and the fertilization of the biological pump by enhanced Southern Ocean upwelling (Duchamp-Alphonse et al., 2018). Overall, it clearly appears that a change in the production of pelagic calcite during these transitional periods had fundamental consequences on the CO2 partitioning between the atmosphere and the surface oceans, potentially lending support to a causality between the intensity of calcification and the levels of CO2 in the atmosphere (Omta et al., 2013).
We hope that the concepts of the morphometry and geochemistry of the coccoliths and new possibilities to extract them from the sediments exposed in this short communication will encourage and stimulate the use of the “vegetal oceanic archive” in downcore palaeoceanographic studies. A powerful research avenue will consist of coupling foraminifera and coccolith measurements, as the former archive has the potential to provide us with vital effect-free signals, which in turn can serve to quantify and exploit the magnitude of the coccolith vital effects in terms of new proxies. Last, we wish to point out that most of the published datasets show a correlation between δ13C and δ18O coccolith compositions. Such a correlation has been repeatedly reported from cultures and the geological record measuring bulk isotopic composition of the sediments (Hermoso et al., 2015; Hermoso, 2016; Jin et al., 2018). It clearly appears that a primary (biogeochemical) origin has to be considered for this geochemical feature, which has long been regarded as a diagenetic overprinting (Marshall, 1992). A common control on these two distinct isotopic systems remains largely elusive given the very contrasting dynamics of these two isotope systems between DIC assimilation by the cell and eventually its biomineralisation. Understanding mechanistically and in fine exploiting such relationships in culture, modern and Meso-Cenozoic periods can potentially represent a valuable biogeochemical and palaeoclimatic research avenue.
All Authors have discussed and shared views contained in this opinion paper. MH wrote the paper with input from all co-Authors.
We acknowledge with thanks the financial support from the French Agence Nationale de la Recherche (ANR) – Project CARCLIM under reference ANR-17-CE01-0004-01, and to French Centre National de la Recherche Scientifique (CNRS) and particularly the Mission pour les Initiatives Transverses et l'Interdisciplinarité for the project COCCOTOPE funded within the scheme Défi ISOTOP.
The authors declare that the research was conducted in the absence of any commercial or financial relationships that could be construed as a potential conflict of interest.
We thank several colleagues from the ISTeP lab and elsewhere for continuous support and insightful discussions.
Anderson, T. F., and Steinmetz, J. C. (1981). Isotopic and biostratigraphical records of calcareous nannofossils in a Pleistocene core. Nature 294, 741–744. doi: 10.1038/294741a0
Beaufort, L., Barbarin, N., and Gally, Y. (2014). Optical measurements to determine the thickness of calcite crystals and the mass of thin carbonate particles such as coccoliths. Nat. Protoc. 9, 633–642. doi: 10.1038/nprot.2014.028
Beaufort, L., and Dollfus, D. (2004). Automatic recognition of coccoliths by dynamical neural networks. Mar. Micropaleontol. 51, 57–73. doi: 10.1016/j.marmicro.2003.09.003
Beaufort, L., Probert, I., de Garidel-Thoron, T., Bendif, E. M., Ruiz-Pino, D., Metzl, N., et al. (2011). Sensitivity of coccolithophores to carbonate chemistry and ocean acidification. Nature 476, 80–83. doi: 10.1038/nature10295
Bolton, C. T., Hernández-Sánchez, M. T., Fuertes, M.-Á., González-Lemos, S., Abrevaya, L., Mendez-Vicente, A., et al. (2016). Decrease in coccolithophore calcification and CO2 since the middle Miocene. Nat. Commun. 7:10284. doi: 10.1038/ncomms10284
Bolton, C. T., and Stoll, H. M. (2013). Late Miocene threshold response of marine algae to carbon dioxide limitation. Nature 500, 558–562. doi: 10.1038/nature12448
Bolton, C. T., Stoll, H. M., and Mendez-Vicente, A. (2012). Vital effects in coccolith calcite: Cenozoic climate- p CO2 drove the diversity of carbon acquisition strategies in coccolithophores? Paleoceanography 27:PA4204. doi: 10.1029/2012PA002339
CLIMAP Project Members (1976). The surface of the ice-age Earth. Science 191, 1131–1137. doi: 10.1126/science.191.4232.1131
Duchamp-Alphonse, S., Siani, G., Michel, E., Beaufort, L., Gally, Y., and Jaccard, S. L. (2018). Enhanced ocean-atmosphere carbon partitioning via the carbonate counter pump during the last deglacial. Nat. Commun. 9, 1–10. doi: 10.1038/s41467-018-04625-7
Erez, J. (2003). The source of ions for biomineralization in foraminifera and their implications for paleoceanographic proxies. Rev. Mineral. Geochem. 54, 115–149. doi: 10.2113/0540115
Foster, G. L., and Rae, J. W. B. (2016). Reconstructing ocean pH with boron isotopes in foraminifera. Annu. Rev. Earth Planet. Sci. 44, 207–237. doi: 10.1146/annurev-earth-060115-012226
Giraudeau, J., and Pujos, A. (1990). Calcareous nannofossil-based transfer function in Caribbean Pleistocene sediments. Oceanol. Acta 13, 453–469.
Hermoso, M. (2014). Coccolith-derived isotopic proxies in palaeoceanography: where geologists need biologists. Cryptogam. Algol. 35, 323–351. doi: 10.7872/crya.v35.iss4.2014.323
Hermoso, M. (2015). Control of ambient pH on growth and stable isotopes in phytoplanktonic calcifying algae. Paleoceanography 30:PA002844. doi: 10.1002/2015PA002844
Hermoso, M. (2016). Isotopic record of Pleistocene glacial/interglacial cycles in pelagic carbonates: revisiting historical data from the Caribbean Sea. Quat. Sci. Rev. 137, 69–78. doi: 10.1016/j.quascirev.2016.02.003
Hermoso, M., Candelier, Y., Browning, T. J. T. J., and Minoletti, F. (2015). Environmental control of the isotopic composition of subfossil coccolith calcite: Are laboratory culture data transferable to the natural environment? Geo. Res. J. 7, 35–42. doi: 10.1016/j.grj.2015.05.002
Hermoso, M., Chan, I. Z. X., McClelland, H. L. O., Heureux, A. M. C., and Rickaby, R. E. M. (2016). Vanishing coccolith vital effects with alleviated carbon limitation. Biogeosciences 13, 301–312. doi: 10.5194/bg-13-301-2016
Hermoso, M., Horner, T. J., Minoletti, F., and Rickaby, R. E. M. (2014). Constraints on the vital effect in coccolithophore and dinoflagellate calcite by oxygen isotopic modification of seawater. Geochim. Cosmochim. Acta 44, 612–627. doi: 10.1016/j.gca.2014.05.002
Hernández-Almeida, I., Ausín, B., Saavedra-Pellitero, M., Baumann, K.-H., and Stoll, H. M. (2019). Quantitative reconstruction of primary productivity in low latitudes during the last glacial maximum and the mid-to-late Holocene from a global Florisphaera profunda calibration dataset. Quat. Sci. Rev. 205, 166–181. doi: 10.1016/j.quascirev.2018.12.016
Jin, X., Liu, C., Zhang, H., Zhou, C., Jiang, X., Wu, Z., et al. (2018). Evolutionary driven of Gephyrocapsa coccolith isotopic vital effects over the past 400 ka. Earth Planet. Sci. Lett. 503, 236–247. doi: 10.1016/j.epsl.2018.09.010
Kameo, K., Shearer, M. C., Droxler, A. W., Mita, I., Watanabe, R., and Sato, T. (2004). Glacial-interglacial surface water variations in the Caribbean Sea during the last 300 ky based on calcareous nannofossil analysis. Palaeogeogr. Palaeoclimatol. Palaeoecol. 212, 65–76. doi: 10.1016/j.palaeo.2004.05.017
Marshall, J. D. (1992). Climatic and oceanographic isotopic signals from the carbonate rock record and their preservation. Geol. Mag. 129, 143. doi: 10.1017/S0016756800008244
McClelland, H. L. O., Bruggeman, J., Hermoso, M., and Rickaby, R. E. M. (2017). The origin of carbon isotope vital effects in coccolith calcite. Nat. Commun. 8:14511. doi: 10.1038/ncomms14511
McClelland, H. L. O. L. O., Barbarin, N., Beaufort, L., Hermoso, M., Ferretti, P., Greaves, M., et al. (2016). Calcification response of a key phytoplankton family to millennial-scale environmental change. Sci. Rep. 6:34263. doi: 10.1038/srep34263
McIntyre, A., Bé, A. W., and Roche, M. B. (1970). Modern Pacific Coccolithophorida: a paleontological thermometer. Trans. N. Y. Acad. Sci. 32, 720–731. doi: 10.1111/j.2164-0947.1970.tb02746.x
Minoletti, F., Hermoso, M., and Gressier, V. (2009). Separation of sedimentary micron-sized particles for palaeoceanography and calcareous nannoplankton biogeochemistry. Nat. Protoc. 4, 14–24. doi: 10.1038/nprot.2008.200
Omta, A. W., Van Voorn, G. A. K., Rickaby, R. E. M., and Follows, M. J. (2013). On the potential role of marine calcifiers in glacial-interglacial dynamics. Global Biogeochem. Cycles 27, 692–704. doi: 10.1002/gbc.20060
Pagani, M. (2014). Biomarker-Based Inferences of Past Climate: The Alkenone pCO2 Proxy, 2nd Edn. Oxford, UK: Elsevier Ltd. doi: 10.1016/B978-0-08-095975-7.01027-5
Petit, R. J., Raynaud, D., Basile, I., Chappellaz, J., Ritz, C., Delmotte, M., et al. (1999). Climate and atmospheric history of the past 420,000 years from the Vostok ice core, Antarctica. Nature 399, 429–413. doi: 10.1038/20859
Rickaby, R. E. M., Henderiks, J., and Young, J. N. (2010). Perturbing phytoplankton: response and isotopic fractionation with changing carbonate chemistry in two coccolithophore species. Clim. Past 6, 771–785. doi: 10.5194/cp-6-771-2010
Stoll, H. M., Guitian, J., Hernandez-Almeida, I., Mejia, L. M., Phelps, S., Polissar, P., et al. (2019). Upregulation of phytoplankton carbon concentrating mechanisms during low CO2 glacial periods and implications for the phytoplankton pCO2 proxy. Quat. Sci. Rev. 208, 1–20. doi: 10.1016/j.quascirev.2019.01.012
Tremblin, M., Hermoso, M., and Minoletti, F. (2016). Equatorial heat accumulation as a long-term trigger of permanent Antarctic ice-sheets during the Cenozoic. Proc. Natl. Acad. Sci. U.S.A. 113, 11782–11787. doi: 10.1073/pnas.1608100113
Weber, J. N., and Woodhead, P. M. J. (1972). Temperature dependence of oxygen-18 concentration in reef coral carbonates. J. Geophys. Res. 77, 463–473. doi: 10.1029/jc077i003p00463
Weiner, S., and Dove, P. M. (2003). An overview of biomineralization processes and the problem of the vital effect. Rev. Mineral. Geochem. 54, 1–29. doi: 10.2113/0540001
Zhang, Y. G., Pagani, M., Liu, Z., Bohaty, S. M., and Deconto, R. (2013). A 40-million-year history of atmospheric CO2. Philos. Trans. R. Soc. A 371:20130096. doi: 10.1098/rsta.2013.0096
Keywords: coccolith, foraminifera, palaeoceanography, isotopic proxies, vital effect, biomineralization and calcification, morphometrics
Citation: Hermoso M, Godbillot C and Minoletti F (2020) Enhancing Our Palaeoceanographic Toolbox Using Paired Foraminiferal and Coccolith Calcite Measurements From Pelagic Sequences. Front. Earth Sci. 8:38. doi: 10.3389/feart.2020.00038
Received: 06 September 2019; Accepted: 04 February 2020;
Published: 21 February 2020.
Edited and reviewed by: Steven L. Forman, Baylor University, United States
Reviewed by:
Renee Lee, University of Reading, United KingdomCopyright © 2020 Hermoso, Godbillot and Minoletti. This is an open-access article distributed under the terms of the Creative Commons Attribution License (CC BY). The use, distribution or reproduction in other forums is permitted, provided the original author(s) and the copyright owner(s) are credited and that the original publication in this journal is cited, in accordance with accepted academic practice. No use, distribution or reproduction is permitted which does not comply with these terms.
*Correspondence: Michaël Hermoso, TWljaGFlbC5IZXJtb3NvQHVuaXYtbGl0dG9yYWwuZnI=
Disclaimer: All claims expressed in this article are solely those of the authors and do not necessarily represent those of their affiliated organizations, or those of the publisher, the editors and the reviewers. Any product that may be evaluated in this article or claim that may be made by its manufacturer is not guaranteed or endorsed by the publisher.
Research integrity at Frontiers
Learn more about the work of our research integrity team to safeguard the quality of each article we publish.