- 1NASA Goddard Space Flight Center, Greenbelt, MD, United States
- 2Universities Space Research Association, Columbia, MD, United States
- 3The City College of New York, New York, NY, United States
The Plankton, Aerosol, Cloud, ocean Ecosystem (PACE) mission will include a hyperspectral imaging radiometer to advance ecosystem monitoring beyond heritage retrievals of the concentration of surface chlorophyll and other traditional ocean color variables, offering potential for novel science and applications. PACE is the first NASA ocean color mission to occur under the agency’s new and evolving effort to directly engage practical end users prior to satellite launch to increase adoption of this freely available data toward societal challenges. Here we describe early efforts to engage a community of practice around marine food-related resource management, business decisions, and policy analysis. Obviously one satellite cannot meet diverse end user needs at all scales and locations, but understanding downstream needs helps in the assessment of information gaps and planning how to optimize the unique strengths of PACE data in combination with the strengths of other satellite retrievals, in situ measurements, and models. Higher spectral resolution data from PACE can be fused with information from satellites with higher spatial or temporal resolution, plus other information, to enable identification and tracking of new marine biological indicators to guide sustainable management. Accounting for the needs of applied researchers as well as non-traditional users of satellite data early in the PACE mission process will ultimately serve to broaden the base of informed users and facilitate faster adoption of the most advanced science and technology toward the challenge of mitigating food insecurity.
Introduction
Seafood is an important source of protein for much of the world’s population. Wild catches cannot match growing demand and their sustainability is in question. Aquaculture is an increasingly important industry to feed the world’s population. According to the Food and Agriculture Organization of the United Nations (FAO), as of 2017 more than 200 nations and $152 Billion per year are involved in the international trade of fish, shellfish, and fish products, as the most traded food product for developing countries at twice the value of the coffee trade (FAO, 2018). However, fisheries face significant threats from environmental and human pressures. There is a need for sustainable and local seafood. Aquaculture has been increasing to supply this demand, yet coastal eutrophication and harmful algal blooms imperil the safety of this food source. Warming, acidification, deoxygenation, and pollution are a few of the environmental stressors that cause habitat shift or loss and undermine the sustainability of the fishing industry. Around the world, illegal, unreported, and unregulated fishing also jeopardizes food security, economic security, and human security. The latest global assessment by the Food and Agriculture Organization of the United Nations indicates that the percentage of fisheries that are unsustainably overfished has increased to one third (World Bank, 2017; FAO, 2018). Fragile states with limited capabilities to enforce their Exclusive Economic Zones need actionable information to evaluate their natural resources. Freely available remotely sensed data from satellites combined with in situ sensors provide environmental insights that can help resource managers and other operators respond to these challenges.
Global aquatic ecosystems have been regularly monitored from space during the past four decades by polar-orbiting, multispectral ocean color radiometers, transforming our understanding of processes that support life in the ocean (McClain, 2009). U.S. examples include the NASA Coastal Zone Color Scanner (CZCS; 1978–1986), Sea-viewing Wide Field-of-view Sensor (SeaWiFS; 1997–2010), Moderate Resolution Imaging Spectroradiometer (MODIS; 1999-present on Terra and 2002-present on Aqua), and Visible Infrared Imaging Radiometer System (VIIRS; 2012-present on Suomi NPP and 2018-present on NOAA-20). These satellite-borne sensors were designed to provide daily, global views of the open ocean where sampling opportunities are infrequent and expensive. In Europe, the Ocean and Land Color Imager (OLCI) was launched onboard Sentinel 3A and 3B in 2016 and 2018, respectively, with another two Sentinel 3 satellites planned to ensure continuity and consistency of services (Donlon et al., 2012; Groom et al., 2019). Additional ocean color sensors have been successfully launched into polar orbit by India, Japan, and China, and into geostationary orbit by South Korea (Groom et al., 2019).
NASA is currently developing the Plankton, Aerosol, Cloud, ocean Ecosystem (PACE) mission, with its launch scheduled for late 2022. PACE has several important technological advancements that will expand its use beyond the heritage instruments listed above, enabling it to enhance and extend current biological and water quality parameters measured from space with new spectral information (Werdell et al., 2019). The primary instrument on the observatory, the Ocean Color Instrument (OCI), consists of two spectrometers that continuously span the ultraviolet (UV) and orange and orange to near-infrared (NIR) spectral regions, as well as seven additional detectors to collect measurements at discrete NIR and shortwave infrared (SWIR) bands (Figure 1). Although not the focus of this manuscript, note that OCI is complemented on the observatory by two multi-angle polarimeters, the Spectropolarimeter for Planetary Exploration (SPEXone) (Hasekamp et al., 2019) and the Hyper Angular Rainbow Polarimeter (HARP2) (Martins et al., 2018). These instruments will provide additional aerosol and cloud data products relative to what is possible with OCI alone. Table 1 provides additional details on characteristics of OCI and the PACE observatory. SPEXone and HARP2 are scheduled for delivery to NASA in the 3rd and 4th quarter of 2020 when integration and testing of the spacecraft begins. Testing and integration of the OCI flight unit will begin in 2020 and continue until delivery in the 3rd quarter of 2021 when integration and testing with the observatory begins. The PACE observatory has a scheduled launch window between November 2022 and March 2023. Public distribution of PACE science data products will begin immediately following official commissioning of the observatory, which is scheduled to occur 60 days after launch. As with most scientific satellites, delays in the delivery of the instruments or in the observatory integration and testing are possible and may result in postponement of the launch, which would in turn delay the availability of PACE science data.
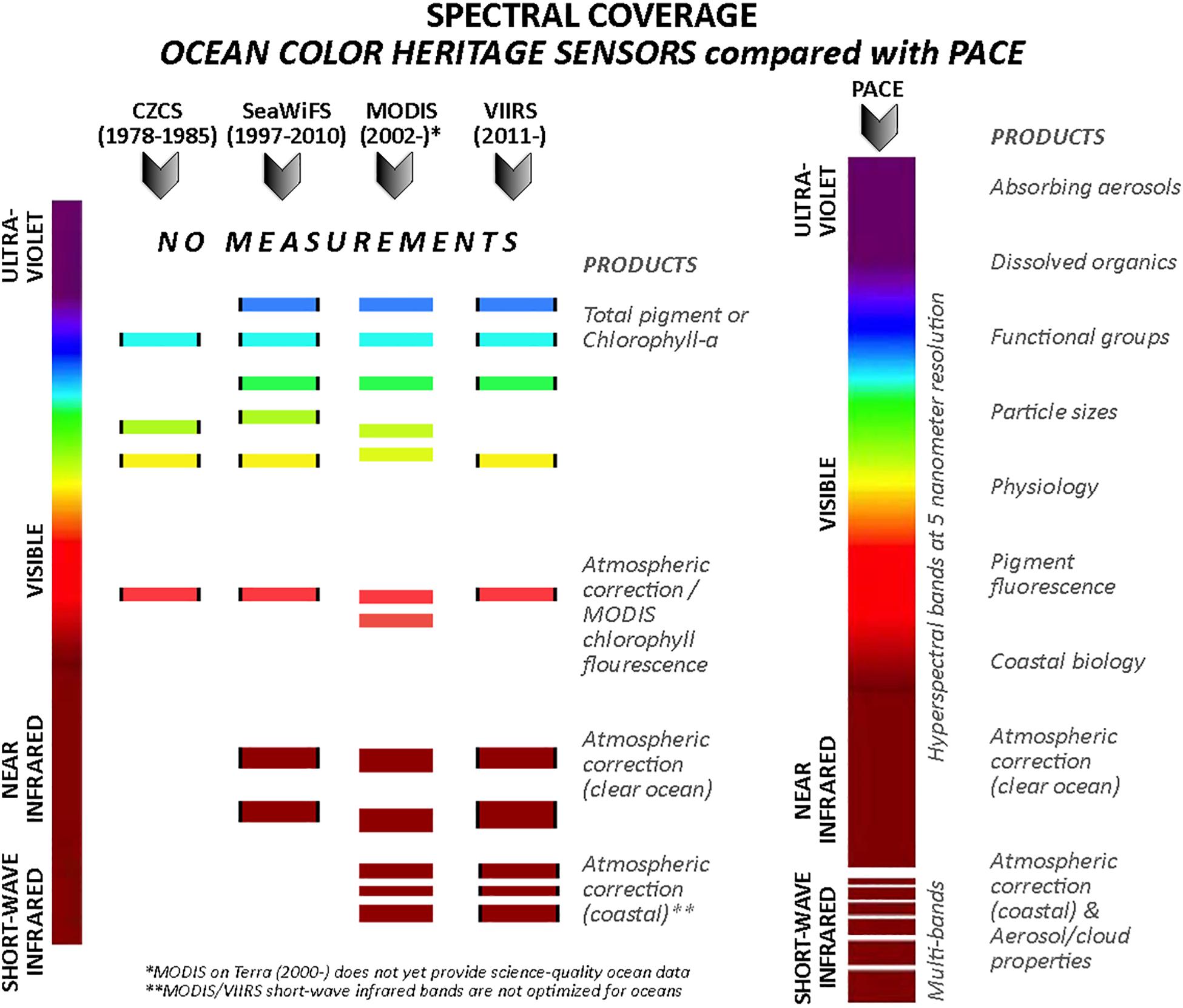
Figure 1. The spectral coverage of ocean color heritage sensors compared to PACE. The hyperspectral capabilities of PACE OCI will provide new information about phytoplankton functional groups and metrics regarding the health of the ecosystem such as particle sizes and phytoplankton physiology. Source: https://pace.gsfc.nasa.gov.
The mission of NASA is to explore through a synthesis of science and technology. Traditionally, NASA Earth Science designs new Earth observing satellites based upon science research questions and then NOAA and the private sector adopt the results of the evolving technology and apply it to practical applications around fisheries and aquaculture. NOAA resource managers, researchers and private businesses have been using satellite data to assist with finding fish for decades, and especially since the launch of SeaWiFS in 1997 (e.g., Maul et al., 1984; Friedl et al., 2006; Wilson et al., 2008, 2009; Wall et al., 2009; Wilson, 2011). NASA is currently undergoing a paradigm shift to consider such practical applications of its satellite data in coordination with science research questions during the initial concept and pre-formulation of a mission before it becomes formalized (National Academies of Sciences Engineering and Medicine [NASEM], 2018). Although the international community has already been considering practical applications in their satellite design process (e.g., ESA’s Copernicus Program), PACE is the first NASA ocean color mission that endeavors to engage operational stakeholders during the design-build phases of a mission prior to launch (Figure 2). Our objective is to prepare a community of practice that can immediately apply the advanced data from PACE as soon as it is available to maximize the nation’s return on investments of this space-based asset. In this manuscript, we describe several early efforts to survey the fisheries and aquaculture communities, among others, about their current uses of ocean color data and how future PACE science data streams could help fill gaps. In addition to regularly attending international meetings to discuss capabilities and needs with scientists and users from around the world, we have undertaken a few targeted activities to foster the exchange of ideas and requirements between satellite data providers and stakeholders. The end goal is to increase access to satellite products that can guide more informed decisions by resource managers, the fishing industry, as well as artisanal and recreational fishers.
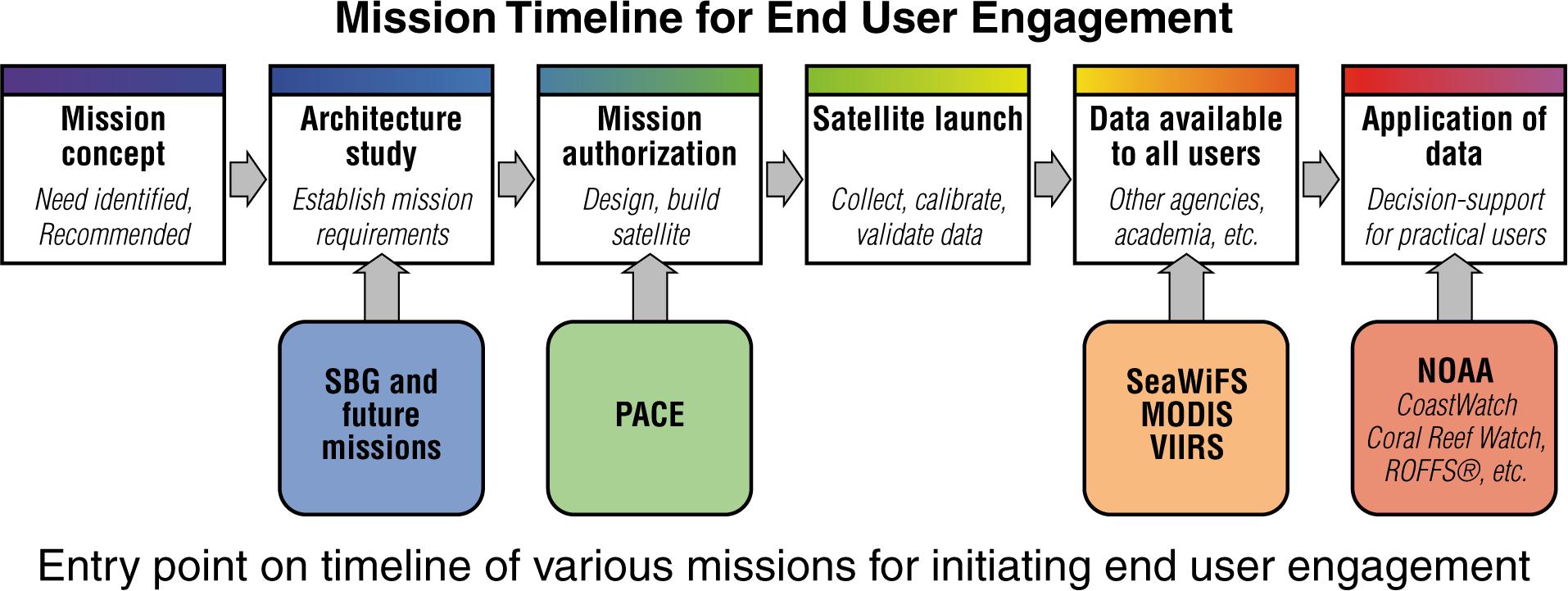
Figure 2. A comparison of the entry point in the timeline for engaging end users in practical applications of satellite data by various NASA ocean color missions and operational activities by NOAA and the private sector. With PACE and future missions, such as Surface Biology and Geology (SBG), NASA now engages operational agencies and end users earlier in the satellite development process.
The activities and outcomes we describe in this manuscript are complementary to previous efforts to ensure the continuity of high quality ocean color satellite data for basic and applied research uses. For example, in 2011 an international committee of experts outlined minimum requirements to sustain global ocean color measurements for research and operational applications, and options to minimize the risk of a data gap (National Research Council [NRC], 2011). NOAA routinely conducts needs assessments targeted to various thematic and regional communities, including fisheries and water quality issues, and looks for ways to connect satellite data providers to practical end users. In 2007, the International Ocean Colour Coordinating Group (IOCCG) established a working group on the operational use of ocean color (IOCCG, 2008). In 2014, the IOCCG established a working group to develop a strategy for incorporating current and future satellite missions into global near-coastal and inland water quality monitoring efforts. Through breakout sessions at international conferences and their 2018 report (IOCCG, 2018), these working groups identified user needs and requirements in the context of existing observing capabilities, and offered suggestions for improving links between data providers and end users, as have other efforts (e.g., Nahorniak et al., 2013). We aim to accomplish similar objectives with an eye toward the role of new spectral capabilities by PACE through the activities described here.
Satellite Ocean Color Provides Applications-Relevant Data Records
Heritage satellite OCIs observe the spectral radiance emanating from the top-of-the-atmosphere at discrete visible, NIR, and SWIR wavelengths. Atmospheric correction algorithms are applied to remove the contribution of the atmosphere and reflection off of the sea surface (e.g., foam and whitecaps) from the total signal to produce estimates of remote sensing reflectances [Rrs(λ); sr–1], the light exiting the water normalized to the downwelling irradiance incident on the sea surface (Mobley et al., 2016). Bio-optical algorithms are then applied to the Rrs(λ) to derive geophysical properties of interest, such as surface concentrations of chlorophyll-a (Chl-a; mg m–3) (O’Reilly et al., 1998). SeaWiFS, for example, collected data at six visible and two NIR wavelengths, where both NIR wavelengths were used for atmospheric correction and four of the six visible wavelengths were used to derive Chl-a. Other applications-relevant geophysical parameters derived from satellite ocean color include indicators of harmful algal blooms (HABs), water clarity, turbidity, sediments and detritus, shallow submerged and floating aquatic vegetation, surface oil slicks, and other variables estimated or inferred through regional correlations between field measurements and remotely sensed proxies (e.g., Stumpf et al., 2003; Hu et al., 2004; Stumpf and Tomlinson, 2005; Hu et al., 2009).
With respect to food security, the most relevant capability of a satellite OCI is its ability to monitor phytoplankton community structure (IOCCG, 2014). Understanding spatial and temporal changes in such structure provides insight into aquatic ecosystem health, water quality and related human health issues, as in the case of HABs, and ecological forecasting of aquatic resources including fisheries production. While ocean color methods for identifying different phytoplankton groups exist (e.g., Bracher et al., 2017; Mouw et al., 2017), most remain confounded by the limited number of wavelengths available on heritage instruments. Increased spectral resolution in the visible region offers improved characterization of spectral phytoplankton pigment absorption and particulate backscattering, both of which provide highly useful metrics for discriminating between phytoplankton groups and plankton size composition (Lubac et al., 2008; Bracher et al., 2009; Torrecilla et al., 2011; Catlett and Siegel, 2018). Several studies identify needs for hyperspectral measurements of at least 5 nm resolution to support classification of phytoplankton community structure (Lee et al., 2007; Vandermeulen et al., 2017). One promise of PACE is the utility of OCI’s hyperspectral capability for the improved assessment of phytoplankton biomass, composition, and production (Table 1). Advances in such routine, global assessments will benefit ecosystem-based models, which aid in managing fisheries and coastal resources as well as policy-making related to monitoring of water clarity and detection and identification of the spatial extent and durations of HABs.
End User Engagement Activities Around Fisheries and Aquaculture
In this section, we describe preliminary efforts to engage a diverse group of potential PACE data users from ocean color experts to novices and non-traditional user groups. The following sections explore these themes for attendees at our workshops, with a few specific case studies of current end users, the ways they utilize remote sensing technologies now, and their data needs related to remote sensing. All workshops were hosted in Washington, DC or Greenbelt, MD through the Applied Sciences activities of the Earth Science Division at NASA Goddard. The workshops were promoted through routine communication channels (e.g., websites, monthly e-mailed newsletter, social media) with free and open registration by anyone interested in attending. The majority of participants in these activities and those who responded to our pre- and post-workshop surveys described their line of work as research, resource management, and public health. Other fields represented included policy/regulatory, remote sensing product development, commercial business, and applied remote sensing training and education. Casting a wide net of data users and potential users serves to deepen our awareness of broader needs beyond the traditional ocean color research community and also informs new groups about the possible applications of this freely available, public information resource.
A workshop on the societal applications of satellite data for ocean health and fisheries was co-hosted by NASA and World Resources Institute (WRI) with assistance from NOAA in November, 2017, garnering 152 registrants from multiple agencies from around the world, non-governmental organizations, and private businesses included research, education, resource management, policy, national security, public health and the fishing industry (Ward and Schollaert Uz, 2018). The meeting was live-streamed to enable remote participation, thus about 80 people participated in person with the rest online. The interactive program brought together multiple perspectives and diverse viewpoints (i.e., fishing businesses, resource managers, lawmakers). The format fostered dialogue between data providers, users, and potential users who could benefit from using satellite products. Four panels focused around the themes of ocean health in the context of climate change, fisheries and ecosystem health, links between fisheries and human security, and resources and tools for accessing ocean satellite data products. Agency managers and panelists shared science and products developed from publicly available ocean satellite data. A keynote presentation described how Global Fishing Watch monitors fishing activity from space to improve understanding, regulation, and protection. Notes taken during the workshop led to an extensive accounting of all presentations and discussions (Ward and Schollaert Uz, 2018).
Nearby NASA Goddard Space Flight Center, the Chesapeake Bay is the largest estuary in North America (Figure 3) and benefits the growing population of the region through its ecosystem services, fishing, and recreation. According to the Maryland Department of Natural Resources (DNR), the primary commercially harvested species in the Bay are oysters, blue crabs, and striped bass. Studies indicate the health of the Bay has seen some improvement in recent years, yet threats to its health persist (e.g., warming, oxygen minimum zones, pollution nutrient run-off). Increasing human presence in such a densely populated coastal region requires constant vigilance by agencies managing water quality, especially with a growing oyster aquaculture industry. In April, 2018 we began monthly meetings between NASA and NOAA satellite data providers with local resource managers around the Chesapeake Bay who routinely monitor shellfish beds and are interested in how remote sensing could help them improve the efficiency of their in situ sampling. In addition to meeting monthly, we held a day-long interagency workshop in August, 2018 attended by Chesapeake Bay science and applications stakeholders with the overall theme of monitoring water quality from space. The 95 participants included federal, state, and local agencies, non-governmental organizations, and universities. Future hyperspectral capabilities by PACE were highlighted, as they will provide the opportunity to measure additional indicators of ecological health and water quality. Workshop discussions focused on environmental variables needed by managers and whether and how remote sensing could address those needs through current or future capabilities, including water quality impacts to human health, shellfish poisoning, and quantifying the economic value of information needed to support decisions by resource managers and fishers (Schollaert Uz et al., 2018).
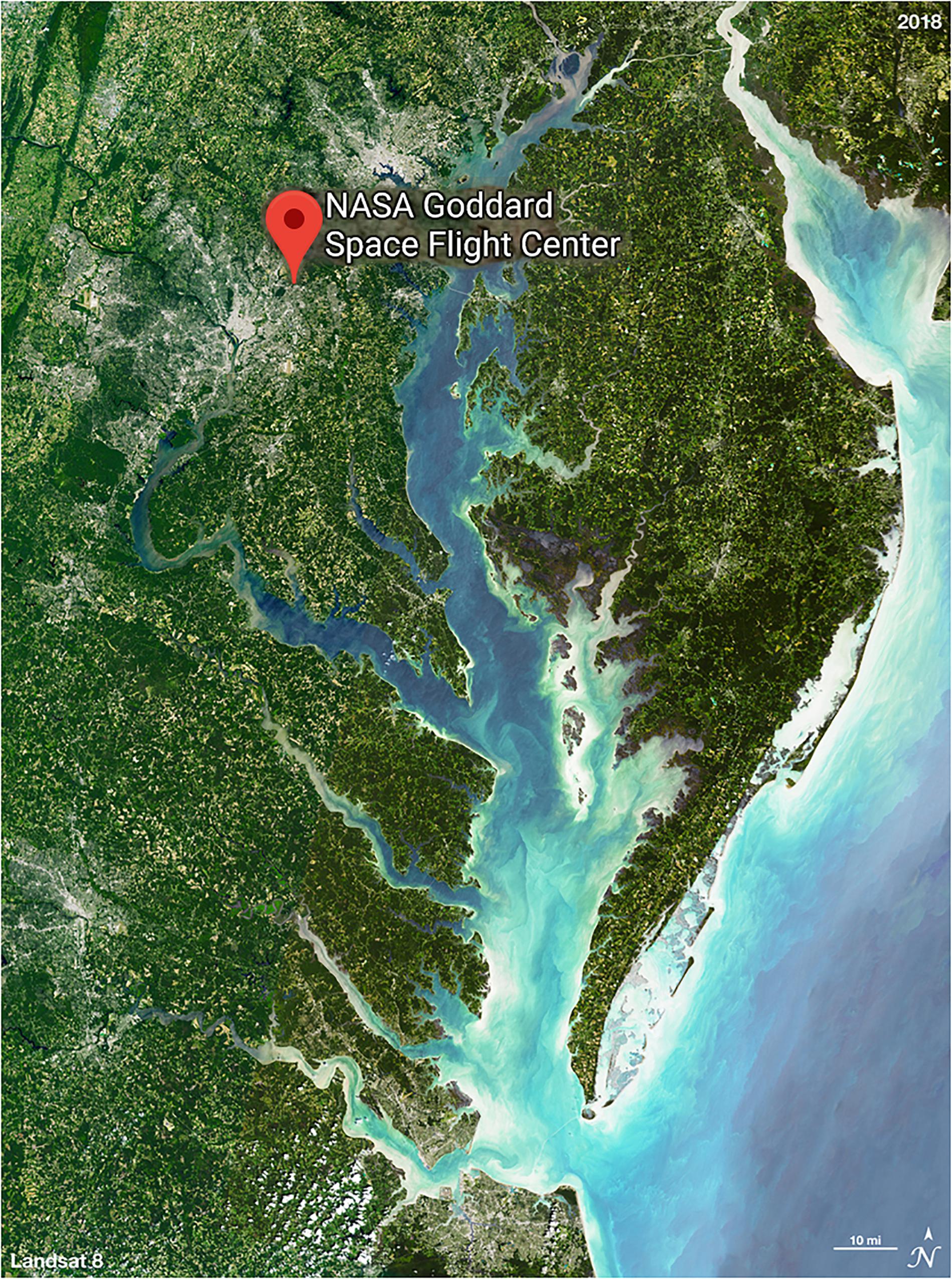
Figure 3. Landsat 8 image of the Chesapeake Bay, tributaries, estuaries, sediment plumes and proximity to NASA Goddard. Measurements from space are used to guide in situ sampling by water quality resource managers, especially around oyster beds within the growing aquaculture industry. Credit: NASA/Michael Taylor and Ginger Butcher.
Discussions with these diverse international and regional groups drew increased attention to broader societal issues that could be informed by ocean color data and also identified applied research themes that need to be explored for development. Continuous engagement with these diverse groups informs NASA about their current challenges that satellite data could potentially address and keeps these groups informed about the status of PACE in its design cycle and the way its information will augment the international satellite fleet.
Current Uses of Remote Sensing Data for Fisheries and Aquaculture
Many examples of ocean color use toward marine food security have been previously described by efforts such as the Societal Applications in Fisheries and Aquaculture using Remotely Sensed Imagery (SAFARI) initiative (IOCCG, 2009). Here we provide updates on uses of remote sensing technologies through several examples, highlighting a few case studies shared during our workshops in which PACE data could help improve operations.
Oceanic management and conservation tools such as Coral Reef Watch and Ocean Health Index plus newer dynamic ecosystem models (e.g., Dynamic Seascapes within the Marine Biodiversity Observation Network) (Strong et al., 2006; Lewison et al., 2015; Hughes et al., 2017) rely on a combination of environmental and biological observations. These products merge in situ data, satellite sensors and big data techniques to quantify the effects of human impacts and natural variability on the marine ecosystem into useable indicators. The need for multiple data types is driven by the scientific understanding that there are multiple stressors acting on the marine ecosystem at the same time, which complicates the interpretation of trends in individual environmental variables.
Fisheries forecasting and management rely on multiple data streams to account for the dynamic nature of the aquatic environment. EcoCast, a fisheries sustainability tool, uses in situ and satellite data for environmental and species-specific information to maximize productive catch while minimizing bycatch (Hazen et al., 2018). This tool is one implementation of a new dynamic approach in fisheries management that accounts for spatial and temporal variations in oceanic processes, as opposed to the traditional static approach with catch limits defined over a fixed area. From a commercial perspective, fisheries forecasting also utilizes a combination of remotely sensed physical (microwave SST) and biological (ocean color) indicators.
Aside from the technical challenges of fisheries management, regulatory agencies are tasked with the enforcement of lawful fishing. Global Fishing Watch tracks real-time commercial fishing activity to combat illegal, unreported and unregulated fishing and seafood fraud. This tool utilizes Automatic Identification System (AIS) data, a collison avoidance system for precise vessel location tracking. Combined with Sentinel-1 satellite radar data, Global Fishing Watch has been able to identify non-broadcasting vessels that attempt to go undetected in illegal fishing activities. In the year after its launch in 2016, this tool acquired over 5,000 core users from 189 countries, engaging a broad, international audience.
Harmful Algal Blooms
NOAA routinely monitors harmful algal blooms in coastal U.S. waters and the Great Lakes based on species’ associations with environmental conditions (e.g., salinity fronts) and measured optical properties (Stumpf et al., 2003; Hu et al., 2004; Stumpf and Tomlinson, 2005). MERIS data is used to distinguish cyanobacteria blooms in U.S. inland waters (Wynne et al., 2008). During our Ocean Health and Fisheries workshop, a shellfish aquaculture farmer who has been operating in coastal ocean waters off Santa Barbara, California since 2002, Bernard Friedman described his business as intermittently plagued by harmful algal blooms. He provides ground-truth data to scientists developing the California-Harmful Algal Risk Mapping model to more precisely predict toxic algae events several days in advance (Anderson et al., 2016; Schollaert Uz, 2018), but the model’s current resolution of 3 km2 is too coarse to be reliable for him. Applying synoptic-scale satellite data to his 0.1 km2 farm will require refining models in combination with additional observations, just as farmers on land use local weather forecasts from sophisticated models that assimilate many sources of observations.
Turbidity
For aquaculture planning, water clarity data products from MODIS at 250 m and Landsat-8 at 30 m are used in coastal waters (e.g., Snyder et al., 2017). The Shellfish Monitoring unit of the Maryland Department of the Environment (MDE) routinely patrols the Chesapeake Bay looking for signs of failing septic systems. MDE collects and analyzes water samples near oyster beds and closes the beds to harvesting whenever fecal coliform levels exceed a safe threshold. Scouting for failing septic systems is labor-intensive and time-consuming. DNR and MDE use available satellite products, e.g., water clarity (Figure 4), but see an opportunity to increase the efficiency of field sampling through a combination of more advanced remote sensing and other assets (e.g., models, autonomous observations).
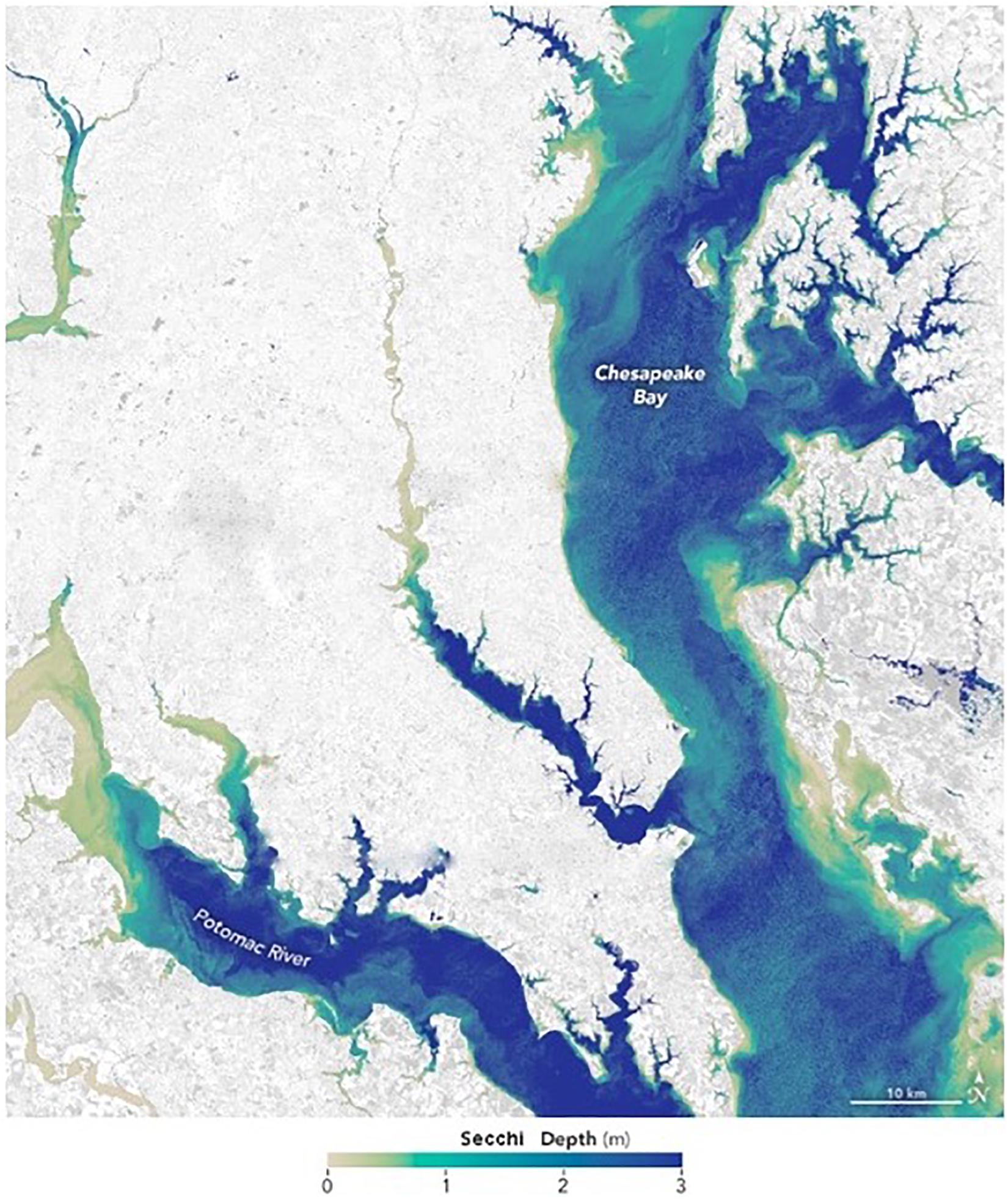
Figure 4. Secchi depth indicates water clarity of the upper Chesapeake Bay and several tributaries derived from the Landsat OLI April 13, 2016 scene. Credit: NASA Earth Observatory and Lachlan McKinna.
Economic Valuation of Ecosystem Services
Economic benefits of improved water quality on commercial fisheries in the Chesapeake Bay have been evaluated by incorporating the effect of water quality changes on production and growth of the stock and producer and consumer surplus. Benefit assessments are complicated by environmental, regulatory, and business factors, such as new firms entering a commercial fishery once water quality improves and stocks grow. An estimate of the benefit of improved water quality in the Chesapeake Bay to striped bass sport fishing alone was valued at $15M in 1996 dollars (Morgan and Owens, 2001; Moore and Griffiths, 2017). Efforts to specifically address the benefit of incorporating Earth observations into decisions is currently being undertaken by the NASA-funded Consortium for the Valuation of Applications Benefits Linked with Earth Science (VALUABLES). This consortium has begun conducting rigorous, quantitative studies about how people use improved information to make decisions and quantify how these decisions improve socioeconomically meaningful outcomes such as lives saved or resources conserved. Within the next few years, they plan to evaluate the use of satellite data applied to water quality decisions, hopefully including PACE data.
Aspirational Uses of Satellite Data and Barriers to Use
In this section, we present survey results from the two workshops described in Section “End User Engagement Activities Around Fisheries and Aquaculture.” Links to the surveys are provided below, along with links to other material about each workshop. The post-workshop surveys were completed by 45 respondents, with an additional 37 responses collected during the second workshop around specific water quality needs of resource managers around the Chesapeake Bay. Common survey results are graphed in Figure 5. Respondents expressed an overall need for satellite data to support decision making in fisheries management and water quality generally (Figure 5A), with smaller percentages of responses for more specific aims such as management of a specific water body or species (under “other management”). Attendees expressed a need for both physical and biological remotely sensed parameters, such as sea surface temperature and salinity, along with ocean color products such as chlorophyll-a concentration and water clarity parameters. Attendees at the Ocean Health workshop uniquely indicated sea surface height as a data need, while Chesapeake Bay workshop attendees require bottom characteristics, submerged aquatic vegetation, precipitation, and terrestrial products for their work. The most desired derived products are harmful algal bloom detection and water safety indices, such as potability and specific bacterial indices (Figure 5B).
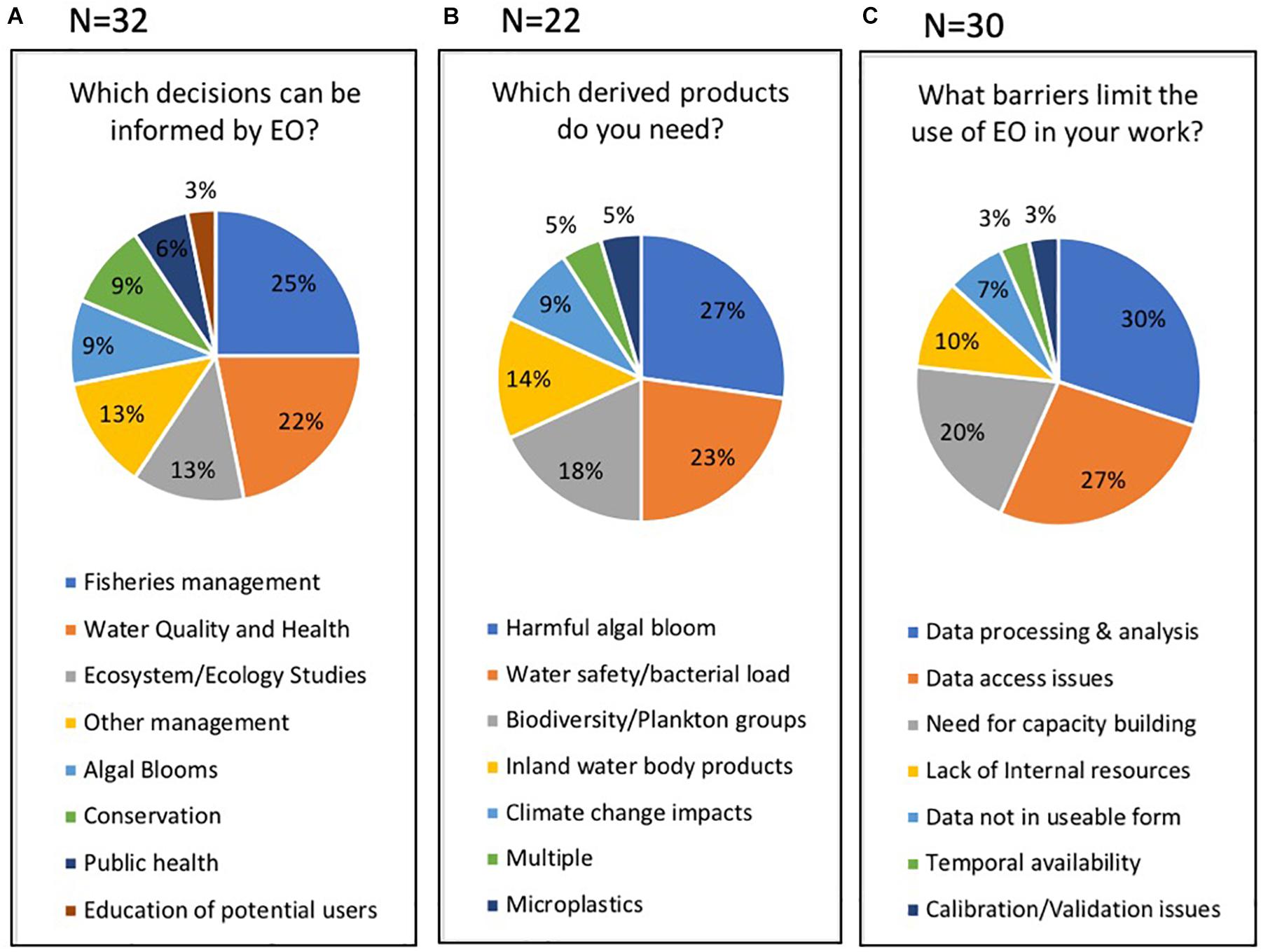
Figure 5. Survey responses from the workshops quantifying (A) end user decision categories, (B) product needs and (C) barriers to the use of Earth Observations (EO).
The main barriers to using satellite and Earth Observation products in their work by most attendees (77%) is a lack of data processing and analysis skills, data access issues and the need for training (Figure 5C). Filling this need is an on-going challenge for data providers and end users alike. IOCCG (2018) discusses a new efforts in Earth Observation data delivery, shifting from the old model of downloading and processing data locally to a new model of moving the processing software to the data source. This is motivated by the ever-increasing volume of data available and the challenges it presents to users for data processing. For example, with Platform as a Service cloud computing services, the provider hosts the data, hardware and/or software on its own infrastructure (e.g., Google Earth Engine, international Open Data Cube, ESA’s Thematic Exploitation Platform and Copernicus Data and Information Access Service, DIAS). Cloud computing services will be even more important for PACE data, as the volume of its hyperspectral data will greatly exceed those of existing polar-orbiting multi-spectral ocean color satellites.
Spectral, spatial, frequency, and latency needs vary widely by community. Attendees primarily seek high spatial resolution for their applications, with 58% stating their ideal spatial scale for data products is finer than 100 m, and a further 21% indicating multiple scales of measurement are important depending on the application. The need for data at <10 m resolution was unique to responses from the Chesapeake Bay workshop, which include users who require this fine scale for land and/or aquatic products. Temporally, 54% indicated their ideal scale for data products is monthly to seasonal, while 35% seek weekly or more frequent temporal resolution. Needs for near real-time data were expressed by the commercial sector and fisheries management applications.
Next Steps
Trade-offs in coverage, resolution, or frequency present barriers for practical use and have limited the local application of existing resources. With global coverage, polar-orbiting satellites capture slow-moving, mesoscale and larger biological features as often as daily, but cannot resolve transient, features on the order of 1km or less. Ocean ecosystems move and change - more similar to weather systems in the atmosphere than vegetation on land. Higher resolution Landsat 8 and Sentinel-2a&b data (10–30 m) are now used to intermittently monitor inland and coastal water quality albeit with a 5–16 days repeat cycle (Franz et al., 2015; Claverie et al., 2018; Keith et al., 2018). PACE will provide higher spectral resolution more frequently with which to distinguish phytoplankton communities (Figure 6), but still not as frequently as a geostationary platform such as GOCI that can capture diurnal changes (Ryu et al., 2012). Biogeochemical-ecosystem models integrated with radiative transfer models yield the absorption and scattering of light by optical constituents and spectrally resolved irradiance and been verified against in situ observations and satellite-derived products (e.g., Dutkiewicz et al., 2015). Models that further combine optical satellite data with fisheries demographic information have recently been developed to predict the location of an endangered species in order to guide resource manager in protection efforts (Breece et al., 2018). Future models that integrate the benefits of each satellite platform (e.g., high spectral resolution from PACE, high temporal frequency from geostationary, and higher spatial resolution from commercial or international sensors) with in situ observations at depth will enable the routine derivation of locally actionable three-dimensional information that a single satellite alone cannot provide.
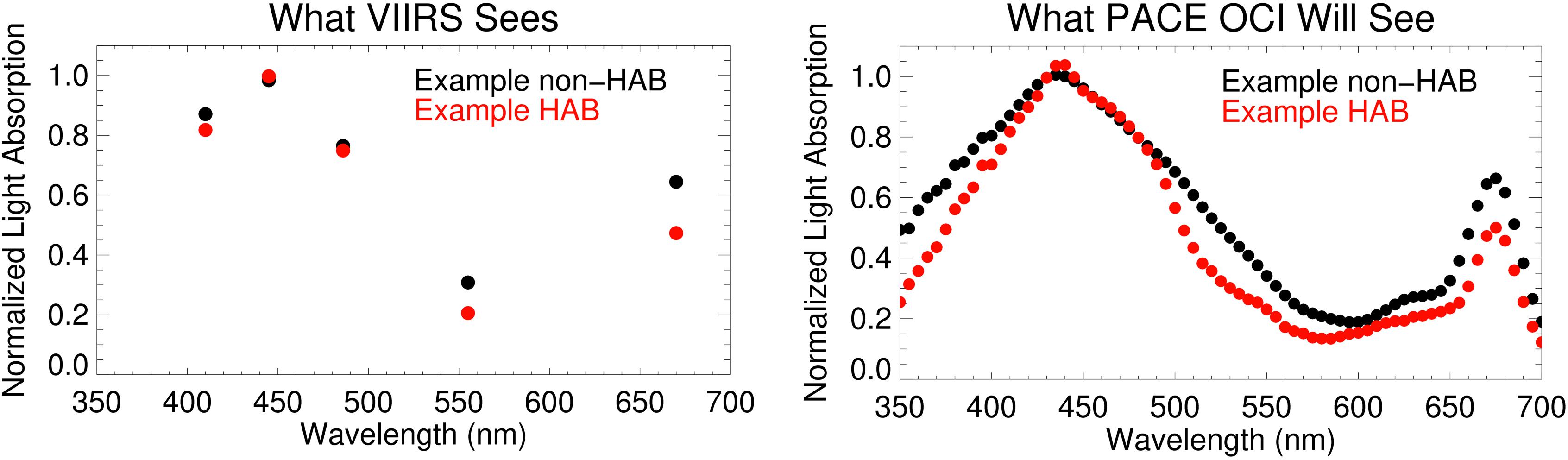
Figure 6. A comparison of the spectral absorption signature of an algal bloom that’s not harmful (non-HAB, black dots) compared to a harmful algal bloom (HAB, red dots) as detected at VIIRS wavelengths (left) compared to future retrievals at PACE OCI wavelengths. In this example, the non-HAB and HAB refer to the background diatom signature and that of Noctiluca scintillans, respectively. A phytoplankton community dominated by one versus the other has significant consequences for the marine food web and fisheries, as has been observed in the Arabian Sea (Gomes et al., 2014).
Assessing the needs of the fisheries and aquaculture community around the Chesapeake Bay through collaboration and regular meetings with resource managers informs applied science priorities for PACE. Many variables that factor into resource manager decisions cannot currently be retrieved from satellite measurements (e.g., toxins, fecal coliform, pH, O2). We need a multi-faceted monitoring system that exploits ecological associations with satellite-derived products. Aquatic features move and change faster than polar-orbiting satellites can observe. Clouds and the intervening atmosphere obstruct a satellite view of the water at visible and infrared wavelengths. Despite challenges, a synoptic view of key variables (e.g., SST, Chl-a, salinity) improves the efficiency of in situ sampling around aquaculture sites. Sustained satellite measurements also provide a long-term average from which anomalies may be detected. Through our regional effort in the Chesapeake Bay, we collaborate with MDE, NOAA, UMD and others to sample the optical, biological, physical, and chemical properties of water flowing into the Bay from land with a goal of finding unique hyperspectral signatures associated with various water quality indicators. Any progress to apply space-based assets through this and other such campaigns will assist NASA to prioritize PACE satellite data products and data distribution in support of fisheries and aquaculture. No single observing system will meet all needs by resource managers and others, but assimilation could exploit the strengths of each asset (i.e., high spectral resolution by PACE, high spatial resolution by harmonized Landsat/Sentinel, high temporal resolution by GOES). We are planning future interagency workshops around integrating satellite and in situ observations with modeling efforts to increase the utility of satellite data products.
The NASA Applied Sciences Program works together with NASA satellite missions prior to launch to engage user communities, solicit data and accuracy requirements for their operations and prepare them for the upcoming mission (Brown and Escobar, 2014). Now referred to as the Early Adopter program, this was discussed at both workshops as one way to engage end users early in the mission development process and have them test their applications with synthetic data. The European Space Agency conducted user needs assessments to make engineering choices prior to the Copernicus mission. By the time a NASA mission becomes official, it has historically been too late to make substantial hardware trade-offs but taking into account input from Early Adopters is still feasible for setting software priorities. As a cost-capped mission, the PACE Project did not receive funding to formally establish an Early Adopter program until recently. Initial efforts to recruit future early adopters have begun and the PACE Mission expects to begin executing a dedicated effort in 2020 to foster a community of practice specifically around the data that will be collected by OCI and the two multi-angle polarimeters and work directly with those implementing these observing systems.
Professional Review of the Pace User Community
One of the main objectives of the PACE Applied Science Program is to engage potential data users during the early stages of mission development to ensure the PACE product suite and delivery mechanisms meet the most urgent demands of users and have the broadest impact. As part of these outreach activities, we have recently designed a professional review of Earth science data end users and experts who have been using, or are interested in using, observations relevant to PACE data products to address a broad range of applied science questions, from food and water security to fisheries, water quality assessments, air quality monitoring and improved pollution forecasts (PACE Mission Survey, 2019). Conducted via an online questionnaire of 17 questions, the professional review will be used to characterize the PACE mission user community in terms of its composition, activities, remote sensing needs, and research interests. The review questions are structured around three themes: user information, data use and requirements, and data access and outreach. We used experiences from satellite data user community evaluations conducted previously for other NASA missions, including SMAP (Soil Moisture Active Passive) and ICESat-2 (Ice, Cloud and land Elevation Satellite-2) (Brown and Escobar, 2014). The main goal of the PACE survey is to solicit data product, data accuracy and access needs, and apply results to plan outreach and applications before PACE launches in order to tailor them to user needs and enhance the societal value of the mission.
The target audience for the professional review is users from a wide range of sectors from around the world, including non-government organizations, non-profit organizations, private industry, universities, local, state and federal agencies. The subject community includes over 1,000 members, representing multiple disciplines including ocean biogeochemistry, oceanography, ecological forecasting, hazards, water resources and quality, terrestrial resources, weather, climate, air quality and human health. To characterize the community in terms of its composition, the survey includes questions on experience and training, and whether the responder is involved in policy making that involves hazard mitigation, increasing public awareness of emergencies, planning for environmental contingencies such as air quality, water quality, and marine resources, monitoring of environmental parameters, or conducts environmental research.
The need to bridge the gap between the increasing amount of satellite data available and end user requirements for data products, tools, and user training, has been widely highlighted by the international Earth Observations community (World Meteorological Organization [WMO], 2017). The Earth Science Division Directive on Project Applications Program presents guidance to all future directed NASA missions, emphasizing the need to characterize potential satellite data application uses and user needs during the early stages of a satellite mission development to increase the relevance and usefulness of their data products (i.e., latency, direct downloads, spatial resolution, data format, repeat frequency). Information from community surveys and professional reviews can help improve understanding on the use of satellite data for environmental applications by users globally, and to identify current gaps in data products, limitations in data access, and areas for improvement (Brown and Escobar, 2014). For example, a professional review conducted for the Famine EarlyWarning System Network (FEWS NET) created by the United States Agency for International Development (USAID) to improve their emergency response capabilities in Africa, had a significant influence on FEWS NET operations, including increased investment in higher resolution and better quality rainfall and vegetation data (Ross et al., 2009).
Conclusion
The recent Decadal Survey serves as a guiding document for NASA programs of Earth observations from space, placing a new emphasis on use-inspired science, whereby end-user needs explicitly inspire research scientists with the hope of improved outcomes for those applications. This is complementary to the curiosity-inspired science that has historically guided work in this field, in which societal benefits are considered after the scientific research has been completed. In response to this new direction, NASA is initiating direct interaction with the satellite data user community. The early efforts described here have begun to engage a community that could use future PACE data in support of fisheries and aquaculture. Through these activities, we are increasing access to satellite products that can give resource managers, lawmakers, fishing and aquaculture businesses the best available remotely sensed information. This ensures that satellite data providers and program managers understand the impact of various choices they make about PACE (e.g., data processing, latency, product development, and applied research efforts) and it will prepare future users of PACE data and engage them around the potential strengths, limitations, and uncertainties. Data gaps and barriers and a need for more training and dialogue have been identified, particularly around preparing the operational community to assimilate OCI data into early warning systems and dynamic ecosystem models.
Technical limitations aside, there are also practical limits to the satellite data products that PACE will be able to provide. The needs of end-users are as diverse as the data requirements and delivery mechanisms to address those needs. While the role of NASA is to explore new frontiers and foster innovative uses of its satellite data for societal benefit, massaging the data into just the right product and format for each user is not. Likewise, NOAA provides operational products, but has to balance the government’s interest in providing timely, open access to global environmental data to promote and protect the safety and security of the American public while not competing with private businesses. Other organizations have developed the ability to take NASA and NOAA satellite data products and get them into the hands of new users. For example, in its Aquaduct water risk platform WRI provides satellite products to the business and investment community via Bloomberg’s mapping tool where it is accessed by more than 400K users around the world. Regularly exchanging ideas with diverse end-user groups informs the PACE Mission about potential downstream uses of its data while preparing user communities for the advancements that are coming so that they can align resources to be ready to take full advantage of the data as soon as it flows. Engaging stakeholders on these and future follow-up activities with NOAA and other federal and state agencies and businesses (e.g., fishing, tourism, big data), we will be able to increase the return on investment of PACE data by providing useful satellite products that give managers, fishers, and aquaculture businesses the best available information. Synergy with operational partners and end-users of satellite data products is essential to realizing the full potential of this and future NASA missions. Interagency collaboration on stakeholder engagement through working groups, workshops, and applied research projects will help us understand how current and future satellite data can be optimized in new ways to solve societal problems around marine food security.
Notes
Workshop agendas and presentations are available at the following sites: NASA/WRI Ocean Health and Fisheries workshop, 2017, Washington, DC: https://www.wri.org/events/2017/11/nasa-wri-ocean-health-and-fisheries-applications-work shop. Post-workshop-Survey: https://www.surveymonkey.com/r/MF2VSHJ. Interagency Chesapeake Bay workshop, 2018, Greenbelt, MD: https://science.gsfc.nasa.gov/610/applied-sciences/chesapeake_bay_workshop.html. Post-workshop Survey: https://docs.google.com/forms/d/1y4hA7JA-eDrKwZbjKUkgEtwakyxrcR0v-_oVhBeil7E/viewform?edit_requested=true.
Author Contributions
SS led the workshops, surveys, and working group, and wrote and revised the manuscript. GK analyzed the survey data and helped to revise the manuscript. AM presented at the workshops, participated in the working group, and provided project content to the manuscript. PW presented at the workshop, attended the working group, and edited the manuscript. MT attended the working group and provided content on the PACE Mission Applications survey.
Funding
This study was supported by the NASA funding at NASA Goddard through the PACE Project and Applied Sciences Ecological Forecasting Program.
Conflict of Interest
The authors declare that the research was conducted in the absence of any commercial or financial relationships that could be construed as a potential conflict of interest.
Acknowledgments
Workshop planning and hosting assistance from Woody Turner of the NASA Applied Sciences Program on Ecological Forecasting, Cara Wilson of NOAA, and Janet Ranganathan of WRI, respectively, as well as meaningful discussions with all panelists and participants of both workshops are gratefully recognized. Motivation to form the interagency Chesapeake Bay working group began with Mark Trice of Maryland DNR and has sustained through collaboration with Rusty McKay of MDE, Shelly Tomlinson and Mike Ondrusek of NOAA, Rossana Del Vecchio of UMD, and many others. NASA Goddard Applied Sciences team members Brendan McAndrew and Jamie Dulaney assist with the monthly meetings and workshops. Encouragement from Jim Irons, Earth Sciences Division Director at NASA Goddard, fosters these activities. Finally, we are grateful to Jim Yoder, Professor Emeritus at URI, and the reviewers for helpful comments on earlier versions of this manuscript.
References
Anderson, C. R., Kudela, R. M., Kahru, M., Chao, Y., Rosenfeld, L. K., Bahr, F. L., et al. (2016). Initial skill assessment of the california - harmful algae risk mapping (C-HARM) system. Harmful Algae 59, 1–18. doi: 10.1016/j.hal.2016.08.006
Bracher, A., Bouman, H. A., Brewin, R. J. W., Bricaud, A., Brotas, V., Ciotti, A. M., et al. (2017). Obtaining phytoplankton diversity from ocean color: a 697 scientific roadmap for future development. Front. Mar. Sci. 4:698. doi: 10.3389/fmars.2017.00055
Bracher, A., Vountas, M., Dinter, T., Burrows, J. P., Röttgers, R., and Peeken, I. (2009). Quantitative observation of cyanobacteria and diatoms from space using PhytoDOAS on SCIAMACHY data. Biogeosciences 6, 751–764. doi: 10.5194/bg-6-751-2009
Breece, M. W., Fox, D. A., Haulsee, D. E., Wirgin, I. I., and Oliver, M. J. (2018). Satellite driven distribution models of endangered atlantic sturgeon occurrence in the mid-atlantic bight. ICES J. Mar. Sci. 75, 562–571. doi: 10.1093/icesjms/fsx187
Brown, M. E., and Escobar, V. M. (2014). Assessment of soil moisture data requirements by the potential smap data user community: review of smap mission user community. IEEE J. Sel. Top. Appl. Earth Obs. Remote Sens. 7, 277–283. doi: 10.1109/JSTARS.2013.2261473
Catlett, D., and Siegel, D. A. (2018). Phytoplankton pigment communities can be modeled using unique relationships with spectral absorption signatures in a dynamic coastal environment. J. Geophys. Res. 123, 246–264. doi: 10.1002/2017jc013195
Claverie, M., Ju, J., Masek, J. G., Dungan, J. L., Vermote, E. F., Roger, J.-C., et al. (2018). The harmonized landsat and sentinel-2 surface reflectance data set. Remote Sens. Environ. 219, 145–161. doi: 10.1016/j.rse.2018.09.002
Donlon, C., Berruti, B., Buongiorno, A., Ferreira, M. H., Femenias, P., Frerick, J., et al. (2012). The global monitoring for environment and security (GMES) Sentinel-3 mission. Remote Sens. Environ. 120, 37–57.
Dutkiewicz, S., Hickman, A. E., Jahn, O., Gregg, W. W., Mouw, C. B., and Follows, M. J. (2015). Capturing optically important consituents and propoerites in a marine biogeochemical and ecosystem model. Biogeosciences 12, 4447–4481. doi: 10.5194/bg-12-4447-2015
FAO (2018). The State of World Fisheries and Aquaculture 2018 - Meeting the Sustainable Development Goals. Rome: FAO.
Franz, B. A., Bailey, S. W., Kuring, N., and Werdell, P. J. (2015). Ocean color measurements with the operational land imager on landsat-8: implementation and evaluation in Seadas. Appl. Remote Sens. 2:096070. doi: 10.1117/I.JRS.9.096070
Friedl, L., Wilson, C., Chao, Y., Bograd, S., and Turner, W. (2006). Using satellite data products to manage living marine resources. EOS 87:437. doi: 10.1111/brv.12359
Gomes, H. D. R., Goes, J. I., Matondkar, S. G. P., Buskey, E. J., Basu, S., Parab, S., et al. (2014). Massive outbreaks of Noctiluca scintillans blooms in the Arabian Sea due to spread of hypoxia. Nat. Commun. 5:4862. doi: 10.1038/ncomms5862
Groom, S., Sathyendranath, S., Ban, Y., Bernard, S., Brewin, R., Brotas, V., et al. (2019). Satellite ocean colour: current status and future perspective. Front. Mar. Sci. 6:485. doi: 10.3389/fmars.2019.00485
Hasekamp, O. P., Fu, G., Rusli, S. P., Wu, L., Di Noia, A., Brugh, J. A. D., et al. (2019). Aerosol measurements by SPEXone on the NASA PACE mission: expected retrieval capabilities. J. Quant. Spectrosc. Radiat. Transf. 227, 170–184. doi: 10.1016/j.jqsrt.2019.02.006
Hazen, E. L., Scales, K. L., Maxwell, S. M., Briscoe, D. K., Welch, H., Bograd, S. J., et al. (2018). A dynamic ocean management tool to reduce bycatch and support sustainable fisheries. Sci. Adv. 4:eaar3001. doi: 10.1126/sciadv.aar3001
Hu, C., Chen, Z., Clayton, T. D., Swarzenski, P., Brock, J. C., and Muller-Karger, F. E. (2004). Assessment of estuarine water-quality indicators using MODIS medium-resolution bands: initial results from Tampa Bay, FL. Remote Sens. Environ. 93, 423–441. doi: 10.1016/j.rse.2004.08.007
Hu, C., Li, X., Pichel, W. G., and Muller-Karger, F. E. (2009). Detection of natural oil slicks in the NW Gulf of Mexico using MODIS imagery. Geophys. Res. Lett. 36:1. doi: 10.1029/2008GL036119
Hughes, T. P., Kerry, J. T., Álvarez-Noriega, M., Álvarez-Romero, J. G., Anderson, K. D., Baird, A. H., et al. (2017). Global warming and recurrent mass bleaching of corals. Nature 543, 373–377. doi: 10.1038/nature21707
IOCCG, (2008). “Why ocean colour? the societal benefits of ocean-colour technology,” in Reports of the International Ocean-Colour Coordinating Group, eds T. Platt, N. Hoepffner, V. Stuart, and C. Brown, (Dartmouth: IOCCG).
IOCCG, (2009). “Remote sensing in fisheries and aquaculture,” in Reports of the International Ocean-Colour Coordinating Group, No. 8, eds M.-H. Forget, V. Stuart, and T. Platt, (Dartmouth: IOCCG).
IOCCG, (2018). “earth observations in support of global water quality monitoring,” in IOCCG Report Series, No. 17, International Ocean Colour Coordinating Group, eds S. Greb, A. Dekker, and C. Binding, (Dartmouth: IOCCG).
Keith, D., Rover, J., Green, J., Zalewsky, B., Carpenter, M., Thursby, G., et al. (2018). Monitoring algal blooms in drinking water reservoirs using the landsat-8 operational land imager. Int. J. Remote Sens. 39, 2818–2846. doi: 10.1080/01431161.2018.1430912
Lee, Z. P., Carder, K., Arnone, R., and He, M. X. (2007). Determination of primary spectral bands for remote sensing of aquatic environments. Sensors 7, 3428–3441. doi: 10.3390/s7123428
Lewison, R. L., Hobday, A. J., Maxwell, S. M., Hazen, E. L., Hartog, J. R., Dunn, D. C., et al. (2015). Dynamic ocean management: identifying the critical ingredients of dynamic approaches to ocean resource management. Bioscience 65, 486–498. doi: 10.1093/biosci/biv018
Lubac, B., Loisel, H., Guiselin, N., Astoreca, R., Artigas, L. F., and Mériaux, X. (2008). Hyperspectral and multispectral ocean color inversions to detect Phaeocystis globosa blooms in coastal waters. J. Geophys. Res. 113:C06026. doi: 10.1029/2007JC004451
Martins , J. V., Fernandez-Borda, R., McBride, B., Remer, L. A., and Barbosa, H. M. J. (2018). “The HARP hyperangular imaging polarimeter and the need for small satellite payloads with high science payoff for earth science remote sensing,” in Proceedings of IEEE International Geoscience and Remote Sensing Symposium, IGARSS 2018, Valencia.
Maul, G. A., Williams, F., Roffer, M., and Sousa, F. M. (1984). Remotely sensed oceanographic patterns and variability of blue fin tuna catch in the Gulf of Mexico. Oceanol. Acta 7, 469–479.
McClain, C. R. (2009). A decade of satellite ocean color observations. Annu. Rev. Mar. Sci. 1, 19–42. doi: 10.1146/annurev.marine.010908.163650
Mobley, C. D., Werdell, J., Franz, B., Ahmad, Z., and Bailey, S. (2016). Atmospheric Correction for Satellite Ocean Color Radiometry. Washington, D.C: NASA.
Moore, C., and Griffiths, C. (2017). Welfare analysis in a two-stage inverse demand model: an application to harvest changes in the chesapeake Bay. Empir. Econ. 55, 1181–1206. doi: 10.1007/s00181-017-1309-3
Morgan, C., and Owens, N. (2001). Benefits of water quality policies: the Chesapeake Bay. Ecol. Econ. 39, 271–284. doi: 10.1016/s0921-8009(01)00212-9
Mouw, C. B., Hardman-Mountford, N. J., Alvain, S., Bracher, A., Brewin, R. J. W., Bricaud, A., et al. (2017). A consumer’s guide to satellite remote sensing of multiple phytoplankton groups in the global ocean. Front. Mar. Sci. 4:862. doi: 10.3389/fmars.2017.00041
Nahorniak, J., Letelier, R., Asanuma, I., Muller-Karger, F., King, E., and Colazo, M. (2013). Ocean Satellite Data: Requests from the Fishery and Aquaculture Community, Report from the OSU/NASA Dream Ocean Satellite Image Workshop. Newort, OR: NASA.
National Academies of Sciences Engineering and Medicine [NASEM], (2018). Thriving on Our Changing Planet: A Decadal Strategy for Earth Observation from Space. Washington, DC: The National Academies Press.
National Research Council [NRC], (2011). Assessing the Requirements for Sustained Ocean Color Research and Operations. Washington, DC: The National Academies Press.
O’Reilly, J. E., Maritorena, S., Mitchell, B. G., Siegel, D. A., Carder, K. L., Garver, S. A., et al. (1998). Ocean color chlorophyll algorithms for SeaWiFS. J. Geophys. Res. 103, 24937–24953. doi: 10.1029/98jc02160
PACE Mission Survey (2019). PACE Mission Survey. Available at: https://www.surveymonkey.com/r/7S2B7NF (accessed May 2, 2019).
Ross, K. W., Brown, M. E., Verdin, J. P., and Underwood, L. W. (2009). Review of FEWS NET biophysical monitoring requirements. Environ. Res. Lett. 4:024009. doi: 10.1088/1748-9326/4/2/024009
Ryu, J. H., Han, H. J., Cho, S., Park, Y. J., and Ahn, Y. H. (2012). Overview of geostationary ocean color imager (GOCI) and GOCI data processing system (GDPS). Ocean Sci. J. 47, 223–233. doi: 10.1007/s12601-012-0024-4
Schollaert Uz, S. (2018). Using Scientific Muscle to Grow Safer Mussels. NASA Earth Observatory. Available at: https://earthobservatory.nasa.gov/IOTD/view.php?id=91595 (accessed January 18, 2018).
Schollaert Uz, S., Turpie, K., Tomlinson, M., and Brown, C. W. (2018). Prioritizing Aquatic Science And Applications Needs in the Cheseapeake Bay for a Space-Borne Hyperspectral Mission, Abstract GC13F-1092 Presented at the 2018 Fall Meeting, AGU, Washington, DC. Available at: https://ntrs.nasa.gov/archive/nasa/casi.ntrs.nasa.gov/20190000495.pdf (accessed December 10, 2018).
Snyder, J., Boss, E., Weatherbee, R., Thomas, A. C., Brady, D., and Newell, C. (2017). Oyster aquaculture site selection using landsat 8-derived sea surface temperature, turbidity, and chlorophyll a. Front. Mar. Sci. 4. doi: 10.3389/fmars.2017.00190
Strong, A. E., Arzayus, F., Skirving, W., and Heron, S. F. (2006). “identifying coral bleaching remotely via coral reef watch - improved integration and implications for changing climate,” in Coral Reefs and Climate Change: Science and Management. Coastal and Estuarine Studies, eds J. T. Phinney, et al. (Washington, DC: American Geophysical Union), 163–180.
Stumpf, R. P., Culver, M. E., Tester, P. A., Tomlinson, M., Kirkpatrick, G. J., Pederson, B. A., et al., (2003). Monitoring Karenia brevis blooms in the Gulf of Mexico using satellite ocean color imagery and other data. Harmful Algae 2, 147–160. doi: 10.1016/S1568-9883(02)00083-5
Stumpf, R. P., and Tomlinson, M. C. (2005). “Remote sensing of harmful algal blooms,” in Remote Sensing of Coastal Aquatic Environments, eds R. L. Miller, C. E. DelCastillo, and B. A. McKee (Dordrecht: Springer), 277–296. doi: 10.1007/1-4020-3100-9_12
Torrecilla, E., Stramski, D., Reynolds, R. A., Millan-Nunez, E., and Piera, J. (2011). Cluster analysis 938 of hyperspectral optical data for discriminating phytoplankton pigment assemblages in the open 939 ocean. Remote Sens. Environ. 115, 2578–2593. doi: 10.1016/j.rse.2011.05.014
Vandermeulen, R. A., Mannino, A., Neeley, A., Werdell, J., and Arnone, R. (2017). Determining the optimal spectral sampling frequency and uncertainty thresholds for hyperspectral remote sensing of ocean color. Opt. Express 25:A785. doi: 10.1364/OE.25.00A785
Wall, C., Muller-Karger, F. E., and Roffer, M. (2009). Linkages between environmental conditions and recreational king mackerel (Scomberomorus cavalla) catch off west-central Florida. Fish. Oceanogr. 18, 185–199. doi: 10.1111/j.1365-2419.2009.00507.x
Ward, A., and Schollaert Uz, S. (2018). Interagency workshop on societal applications of satellite data for ocean health and fisheries. Earth Obs. 30, 9–20.
Werdell, P. J., Behrenfeld, M. J., Bontempi, P. S., Boss, E., Cairns, B., Davis, G. T., et al. (2019). The plankton, aerosol, cloud, ocean ecosystem (pace) mission: status, science, advances. Bull. Amer. Meteor. Soc. 100, 1775–1794. doi: 10.1175/BAMS-D-18-0056.1
Wilson, C. (2011). The rocky road from research to operations for satellite ocean-colour data in fishery management. ICES J. Mar. Sci. 68, 677–686. doi: 10.1093/icesjms/fsq168
Wilson, C., Chen, C., Clark, C., Fanning, P., Forget, M.-H., Friedland, K., et al. (2009). “Remote sensing applications to fisheries management,” in Remote Sensing in Fisheries and Aquaculture, IOCCG Report 8, eds M.-H. Forget, V. Stuart, and T. Platt, (Dartmouth, NS: IOCCG), 43–56.
Wilson, C., Morales, J., Nayak, S., Asanuma, I., and Feldman, G. (2008). “Ocean colour radiometry and fisheries. in why ocean colour?,” in The Societal Benefits of Ocean-Colour Technology, eds T. Platt, N. Hoepffner, V. Stuart, and C. Brown, (Dartmouth, NS: IOCCG), 47–58.
World Bank (2017). The Sunken Billions Revisited: Progress and Challenges in Global Marine Fisheries. Environment and Development. Washington, DC: World Bank.
World Meteorological Organization [WMO] (2017). Survey on the Use of Satellite Data. 2017. WMO Space Programme SP-13, World Meteorological Organization. Available at: http://www.wmo.int/pages/prog/sat/meetings/documents/IPET-SUP-3_Doc_09-01_WMOUserSurvey2016.pdf (accessed May 2, 2019).
Keywords: satellite ocean color applications, PACE mission user community, phytoplankton, fisheries, aquaculture, societal impact
Citation: Schollaert Uz S, Kim GE, Mannino A, Werdell PJ and Tzortziou M (2019) Developing a Community of Practice for Applied Uses of Future PACE Data to Address Marine Food Security Challenges. Front. Earth Sci. 7:283. doi: 10.3389/feart.2019.00283
Received: 09 December 2018; Accepted: 17 October 2019;
Published: 05 November 2019.
Edited by:
Irina Alexandrovna Mironova, Saint Petersburg State University, RussiaReviewed by:
Yoav Lehahn, University of Haifa, IsraelTim J. Malthus, CSIRO Oceans and Atmosphere (O&A), Australia
Copyright © 2019 Schollaert Uz, Kim, Mannino, Werdell and Tzortziou. This is an open-access article distributed under the terms of the Creative Commons Attribution License (CC BY). The use, distribution or reproduction in other forums is permitted, provided the original author(s) and the copyright owner(s) are credited and that the original publication in this journal is cited, in accordance with accepted academic practice. No use, distribution or reproduction is permitted which does not comply with these terms.
*Correspondence: Stephanie Schollaert Uz, stephanie.uz@nasa.gov