- Department of Geosciences and Environment, Facultad de Minas, Universidad Nacional de Colombia, Sede Medellín, Medellín, Colombia
The spatiotemporal variability of precipitation in regions of complex mountainous terrains constitutes one of the most challenging research topics of geosciences. This paper explores hourly precipitation data from a set of 25 stations spanning the period 1998 to 2005 within northwestern Colombia, in the Aburrá Valley and the neighboring San Nicolás plateau (75.16°W−6°N and 75.66°W−6.6°N) which accounts for a land area of ~4,000 km2. Our aim is to identify the main features of the diurnal cycle of precipitation over this complex terrain. We found that the average diurnal cycle of rainfall in the study region is bimodal at regional scale although it results from the superposition of two unimodal diurnal cycles shifting its phase throughout the seasons of the year. From October to April, average diurnal rainfall peaks in the afternoon hours (13:00–16:00 LST) but from May to September, the phase of the diurnal cycle changes to midnight hours (22:00–02:00 LST). Three low-level jets (LLJs), namely Caribbean, CHOCO, and the so-called Corriente de los Andes Orientales (CAO), are relevant to explain the seasonal shift of the diurnal cycle given their modulation of the seasonal variation of moisture sources and transport over this region. During June-July-August, moisture from afternoon evaporation processes at the bottom of the inter-Andean Magdalena Valley, located at the east of the study region, is transported by anabatic and easterly trade winds and contribute to explain the midnight and early morning peak. The life cycle of convective processes influences the orographic nature of rainfall distribution and timing in the region since deep convective cores are related with the afternoon peak, whereas wide convective cores with the early morning peak.
Introduction
Colombia is located in the northwestern corner of Tropical South America and presents some particular geomorphologic and hydro-climate features including: (i) steep slopes across the three Cordilleras (ranges) of the Andes crossing from southwest to northeast, (ii) seasonal activity of three atmospheric low-level jets, (iii) hydro-climate systems of the Amazon and Orinoco River basins, (iv) synoptic-scale atmospheric patterns associated with the tropical Pacific and Atlantic Oceans and the Caribbean Sea, and (v) land-atmosphere feedback processes. Regarding the spatial pattern of long-term mean precipitation, Snow (1976) refers to the Colombian Andes as “a dry island in a sea of rain.” Diverse studies have tackled the spatio-temporal variability of rainfall over the Colombian Andes (Schmidt, 1952; Trojer, 1959; López and Howell, 1967; Snow, 1976; Oster, 1979; Martínez, 1993; Poveda and Mesa, 1997; Mejía et al., 1999; León and Narávez, 2001; Poveda et al., 2001, 2005, 2007, 2011; Mapes et al., 2003a; Poveda, 2004, 2011; Zuluaga and Poveda, 2004; Pabón and Dorado, 2008; Hurtado and Poveda, 2009; Alvarez-Villa et al., 2011; Hurtado-Montoya and Mesa-Sánchez, 2014; Poveda and Salas, 2015; Salas and Poveda, 2015; Jaramillo et al., 2017; Bedoya-Soto et al., 2018). In spite of such large body of literature, the dynamical and thermodynamical processes associated with rainfall over the Andes of Colombia are largely unknown, more so at high spatial and temporal resolutions.
The scientific literature regarding the diurnal cycle of rainfall in the Andes of Colombia is limited to small data sets (Trojer, 1958; Pita Suárez-Cobián., 1959; Snow, 1976; Trewartha, 1981; Mapes et al., 2003a; Warner et al., 2003). Trojer (1959) describes the effect of the altitude on the diurnal cycle of precipitation maxima over the inter-Andean Cauca River valley, with a mid-night (afternoon) peak for the lowest (higher) elevations of the valley. The study of Poveda et al. (2005) regarding the diurnal cycle of rainfall over the Andes of Colombia found: (i) seasonal variations of the diurnal (24 h) and semi-diurnal (12 h) cycles, with highly variable phase and amplitude even at nearby raingauges; (ii) driest hours of the diurnal cycle during the late-morning (09:00–11:00 LST) throughout the year; (iii) a general afternoon peak over northeastern and western regions of Colombia; (iv) a bimodal diurnal cycle over the western side slope of the Central Cordillera with midnight and afternoon hours peaks; and (v) a midnight peak over the eastern side slope of the Central Cordillera. The mentioned study did not find a clear-cut relation between extremes of the diurnal cycle with elevation, branch, and aspect on the Andes, except for some small regions within the intra-Andean valleys.
The strong spatiotemporal variability of the diurnal cycle of rainfall along the Andes of Colombia results from dynamical combinations of local and synoptic patterns and thermodynamic features. The complex topography is essential to determine the observed diurnal cycles but is not the single factor to explain the seasonal variations of the diurnal rainfall peaks. The seasonal variations could be explained by the latitudinal migration of the Intertropical Convergence Zone (ITCZ), and also by the connection of the ITCZ and the dynamics of three Low-Level Jets in the region namely CHOCO, Caribbean, and Corriente de los Llanos Orientales (CAO) (Torrealba and Amador, 2010). Particularly, the ITCZ is the most important control of the annual cycle of Colombia's hydro-climatology (Trojer, 1959; Eslava, 1994; Mejía et al., 1999; León and Narávez, 2001; Hastenrath, 2002; Poveda et al., 2006; Killeen et al., 2007; Espinoza-Villar et al., 2009; Alvarez-Villa et al., 2011; Sierra et al., 2015). A bimodal annual cycle of rainfall is mostly confined to the Andean region (Poveda et al., 2005) with two distinct wet seasons (March to May, M-A-M, and September to November, S-O-N), and two drier (less rainy) seasons (December to February, D-J-F, and June to August, J-J-A). Furthermore, the activity of the CHOCO low-level jet explains the world-record breaking precipitation area over the western Pacific coast of Colombia (Poveda and Mesa, 2000; Mapes et al., 2003a; Warner et al., 2003; Rueda and Poveda, 2006; Sakamoto et al., 2011; Poveda et al., 2014; Sierra et al., 2015; Hoyos et al., 2017; Yepes et al., 2019). The CHOCO jet originates and develops over the far eastern Pacific, and it can be traced back as 30°S, off the Chilean coast (Sakamoto et al., 2011). Upon entering the continent, the CHOCO jet is lifted by the orography of the Western Cordillera of the Colombian Andes, and interacts with the easterly trade winds to focus and enhance deep convection (López and Howell, 1967; Poveda and Mesa, 2000; Mapes et al., 2003a; Warner et al., 2003; Poveda et al., 2006; Jaramillo et al., 2017). The easterly Caribbean low-level jet (CLLJ), independently identified by two Latin American groups (Amador and Magaña, 1999; Poveda and Mesa, 1999), is active in a region covering from northern South America to the Greater Antilles, mostly on the Intra-Americas Sea (IAS) and the Western Hemisphere Warm Pool (WHWP) (Wang and Enfield, 2001, 2003; Wang et al., 2006; Wang, 2007). Spatially, the CLLJ is strong over Colombia's Caribbean Sea (70–80°W and 12–15°N). The annual cycle of the CLLJ is bimodal showing maximum (minimum) velocities during June-July and December to January (October-November and April-May). The highest (lowest) velocities of the CLLJ along the year reach values of 11–13 ms−1 (5–6 ms−1) (Poveda et al., 2014).
The work by Poveda et al. (2005) evidenced that the diurnal cycle of rainfall in the Colombian Andes may exhibit sharp differences even among nearby raingauges. They found that both the phase and amplitude of the diurnal cycle of rainfall over this region exhibit an extraordinary spatially (incoherent) behavior, even for rain gauges separated 5 km away from each other, albeit located over the same slope and altitude over the valley floor. The main objective of this study is to study whether such strong spatial variability in the phase and amplitude in the diurnal cycle of rainfall keeps showing up in other unexplored regions of the Colombian Andes. We study the linkages between the diurnal cycles of rainfall along a longitudinal transect covering two neighboring regions located on the Central range of the Colombian Andes: the San Nicolás Plateau (elevation between 2,220 and 2,600 m) and the Aburrá Valley, containing the city of Medellín (elevation 1,500 m). The study of Bedoya-Soto and Poveda (2008) found a spatially coherent behavior in some features of the seasonal march of the diurnal cycle over both regions, and highly significant lagged correlation between hourly rainfall in the San Nicolás Plateau and that over the Aburrá Valley. We are interested in studying those diurnal cycle changes throughout the year (with the phase of the annual cycle) and the spatial coherence of such variability among the studied raingauges. Basically, we use the term “coherence” to describe a common spatial behavior of rainfall intensities at different time scales for the set of studied rain gauges located over the complex terrain of the Colombian Andes. Defining such coherence will contribute to advance our understanding of the spatiotemporal dynamics of rainfall over the Andes of Colombia and its predictability. This is particularly important and relevant in a region where the TRMM satellite rainfall does a very poor job in capturing in-situ measurements (Bedoya-Soto et al., in preparation; Vallejo-Bernal et al., submitted).
Toward those aims, the study is organized as follows. Section 2 describes the data sets and methods. Section 3 contains the results, and the conclusions are drawn in the final section.
Data and Methodology
Data
Our dataset consists of hourly precipitation records covering the period from 1998 to 2005 at 25 raingauges, 18 (7) of which are located along the Aburrá Valley (San Nicolás Plateau). The data set was provided by Empresas Públicas de Medellín (EPM). The Aburrá Valley is located among the inter-Andean valleys of the Cauca and Magdalena Rivers, in the northern Central range of the Andes of Colombia between 75.66°W−6.6°N and 75.16°W−6°N and houses the city of Medellín and its metropolitan area. The San Nicolas Plateau is located just to the east of the Aburrá Valley. Figure 1 shows the study region and the location of the set of raingauges. Terrain elevations range from 1,500 to 1,700 m a.s.l (2,300 to 2,500 m a.s.l.) in the Aburrá Valley (San Nicolás Plateau). Both regions are separated by an orographic barrier reaching 2,600 m. Figure 1 shows that Vasconia is the highest raingauge (2,510 m) in the San Nicolas Plateau, whereas the lowest raingauge is Miguel Aguinaga (1,549 m) in the Aburrá Valley. The separation between these two stations is around 20 km, which denotes the strong altitudinal gradients of the study region.
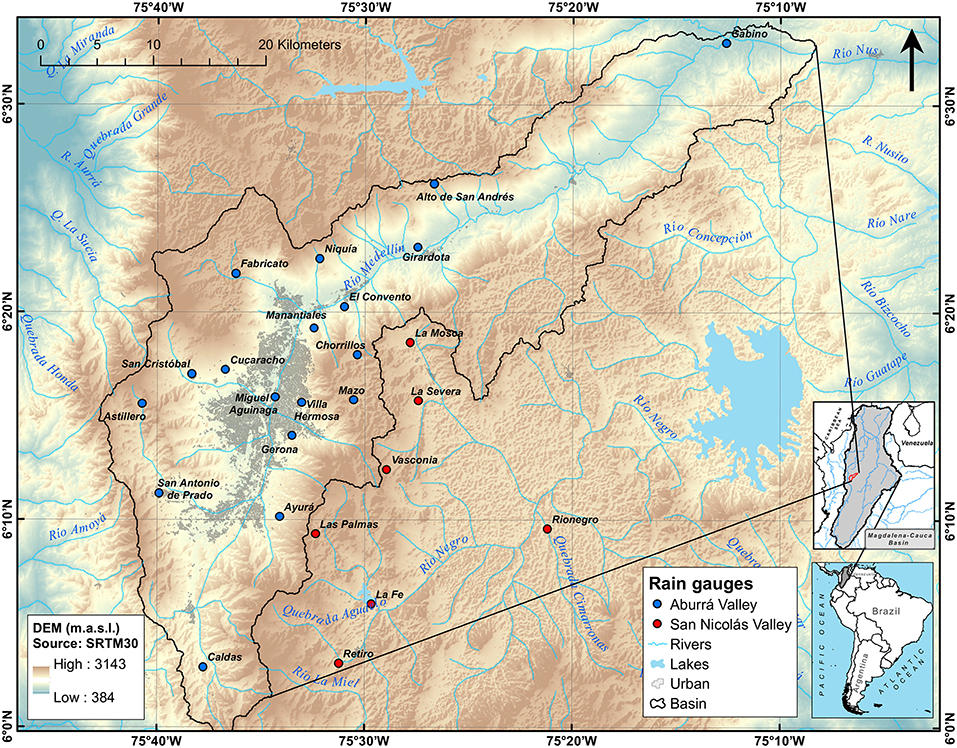
Figure 1. Location of the region and rain gauges included in the study. The middle inset depicts the location of the Magdalena-Cauca River basin.
With the purpose of investigating the driving mechanisms explaining the features of the diurnal cycle through the annual cycle, we also use precipitation products from TRMM 3B42/3B43, and climatic data (zonal winds, precipitable water, and specific humidity at several pressure levels) from the ERA-Interim, NCEP-NCAR, and MERRA-2 reanalyses. Satellite imagery from GOES (http://rammb-slider.cira.colostate.edu) is also evaluated to understand the local sources of evaporation influencing rainfall in the study region (Schmit et al., 2017). We specifically used the “cirrus” near-infrared band at 1.37 μm with a nominal sub satellite pixel spacing of 2 km which identify thin cirrus clouds. We also use reflectivity data from a meteorological radar located over the region and operated by Sistema de Alerta Temprana de Medellín y el Valle de Aburrá (SIATA, https://siata.gov.co/). The data set from this radar consists of three storm events developed during August, October and November of 2018.
Methods
From the existing data sets, hourly and monthly rainfall averages were estimated considering both the presence and absence of zeroes in hourly rainfall. Hourly resolution rainfall is a very rare event. Up to the ninety percentiles, hourly records are in the 0–0.5 mm/hour intensity range. Estimating the different cycles excluding zeros is meaningful insofar captures the dynamics whenever actually rain does occur. On the other hand, estimating the average diurnal cycles including zeros aims at capturing the importance of dry hours in the estimations. Obviously, estimates including zeros are smoother and slighter less in magnitude with respect to estimates excluding zeros.
As we already mentioned, the diurnal cycle was estimated for the 24 h period starting and ending at 07:00 LST (Poveda et al., 2005). Values of hourly rainfall were estimated as a percent of total daily rainfall, but also in terms of average intensity, as explained next.
Let Ph,m,y be the precipitation rate (mm h−1) for the hour h of the day (h = 1, …, 24) from a certain month m (m = 1, …, 12), and a certain year y (y = 1, …, ny). The mean diurnal cycle of rainfall is estimated as the long-term average intensity for each hour (Dh), as follows:
where nh,m,y is the total number of data for a specific hour, taking into account all the months and years of the entire sample.
The annual average of hourly percent rainfall DPh(%) indicates the relative amount of rainfall concentrated at each hour as a percent of accumulated daily values, estimated as:
We also estimate the monthly variation of the diurnal cycle of rainfall. The long-term average rainfall intensity for each hour, h = 1, …, 24, at month m (m = 1, …, 12), is estimated as:
Likewise, we estimate the percent diurnal cycle of rainfall distributed through the annual cycle. The percentage of rainfall for each hour at each month is estimated using (Equation 3), scaled by the accumulated daily rainfall for each month, as:
We use i to denote that the denominator in Equation (4) is the daily rainfall accumulated at each month m.
Results
Annual Cycle
The mean annual cycle of precipitation over the study region is shown in Figure 2. Consistently with previous research, all raingauges exhibit the bimodal character of precipitation in the Andean mountains of Colombia (Mejía et al., 1999; Poveda, 2004; Poveda et al., 2005). Two periods of higher rainfall are seen during April and May (A-M) and from September to October (S-O), and two periods of lower rainfall during D-J-F and J-J-A, owing to the meridional displacement of the ITCZ and other physical mechanisms. Specifically, the S-O rainy season coincides with the coupling of the ITCZ with the dynamics of the CHOCO Jet, which exhibits maximum winds and moisture advection during S-O-N (Poveda and Mesa, 2000; Poveda et al., 2006, 2014; Yepes et al., 2019), and with the passage of tropical easterly waves over the region (Poveda, 2004). Also, minimum values appear during D-J-F and July-August, with similar values over both regions, on the order of 80 mm per month. It is shown that the intensity is higher in the northernmost raingauge station (Gabino) of the Aburrá Valley. However, we cannot generalize this behavior because of the lack of rainfall stations in this area.
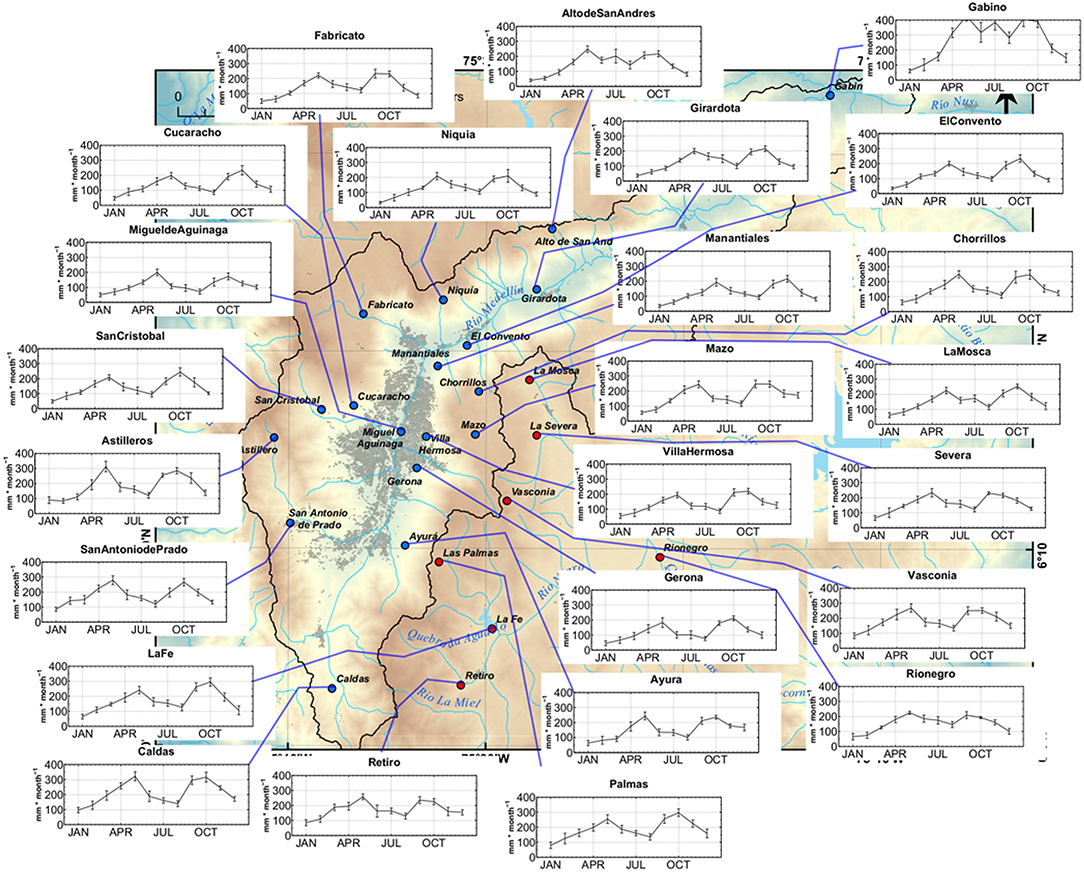
Figure 2. Long-term mean annual cycle of rainfall at rain-gauges included in the study. Error bars on all panels represent the standard error of the mean. Blue (red) points in the map represent the raingauges located on the Aburrá Valley (San Nicolás Plateau).
Diurnal Cycle
The mean diurnal cycle of rainfall (excluding zeroes from calculations) is shown in Figure 3. Values are given as percent (provided it rains) of the total daily rainfall. At first glance, a bimodal diurnal cycle is observed, with two precipitation maxima during the afternoon (15:00–17:00 LST), and a second peak around midnight (23:00–02:00 LST).
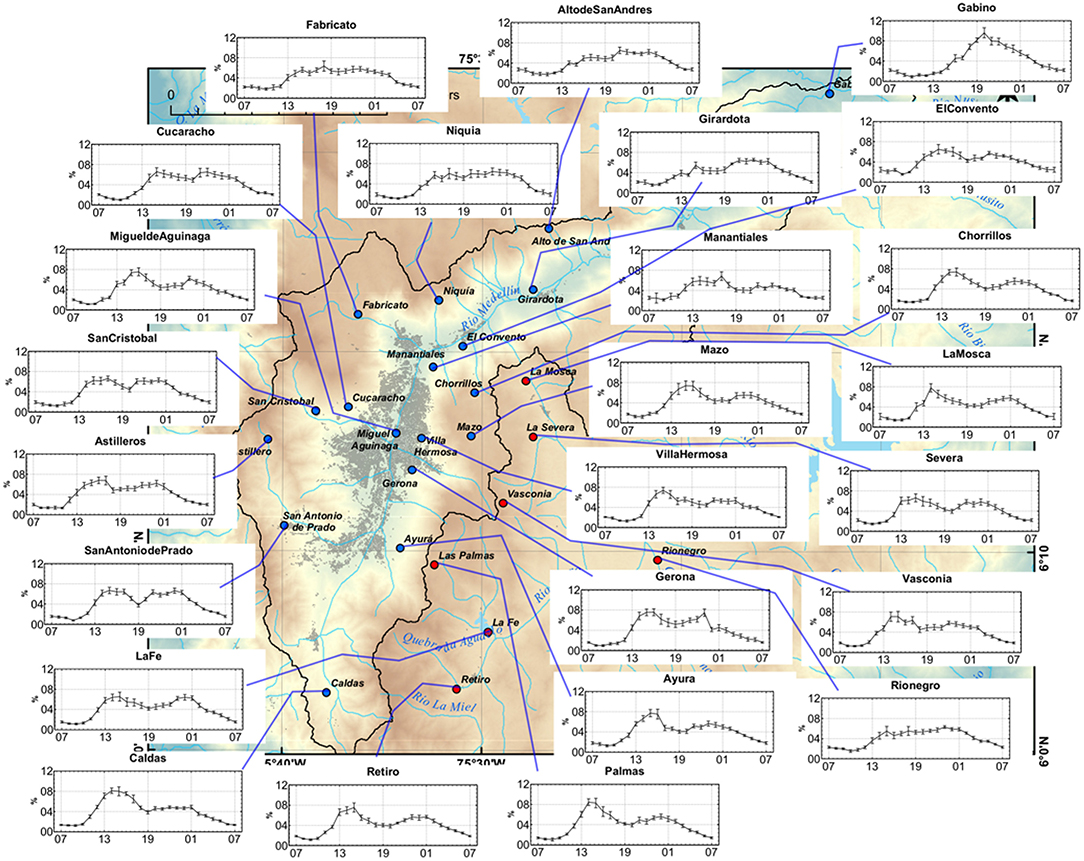
Figure 3. Long-term mean (1998–2005) diurnal cycle of percent rainfall (with respect to daily total) of raingauges in the study region (Equation 2). Error bars on all panels represent the standard error of the mean. Computations based on hourly series excluding zeros. Blue (red) points in the map represent the raingauges located on the Aburrá Valley (San Nicolás Plateau).
Regarding the identified two maxima, Houze (2012) argues that convective life cycle influences diurnal variability; as small (afternoon peak) and large (early-morning peak) convective systems contribute to local precipitation, this fact may explain the bimodality of the average diurnal cycle. Higher hourly average intensities are observed in the Gabino station located at the northernmost part of the region. However, we cannot generalize this result since this is the only station in this tip of the Aburrá Valley. Rainfall minima occur between 07:00 and 11:00 LST, with lowest values from 08:00 to 10:00 LST. The afternoon peak is mainly explained by the effect of direct solar insolation that triggers convective cells during the day on high terrain at lower latitudes (Houze, 2012).
Figure 4 shows the diurnal cycle of average rainfall intensity (zeroes excluded). Most stations witness average intensity values ranging from 0.3 to 2 mm h−1. The latter limit is only exceeded at Gabino, the northernmost raingauge. There is a predominant bimodality (afternoon-midnight) excepting the northernmost and the easternmost raingauges (Rionegro). Some features of the mean diurnal cycle are determined by the mountains aspect and slope. Along the Aburrá Valley eastern slope, the afternoon peak predominates over the early-morning peak (e.g., Ayurá and Palmas) while on the western slope both peaks are better balanced (e.g., San Antonio de Prado and San Cristobal). A spatial pattern of maximum intensities is also observed in association with the afternoon mode over the southern region (i.e., Caldas and El Retiro stations), while the maximum is associated with the midnight and early morning peak in the northern raingauges (i.e., San Andrés and Gabino Stations).
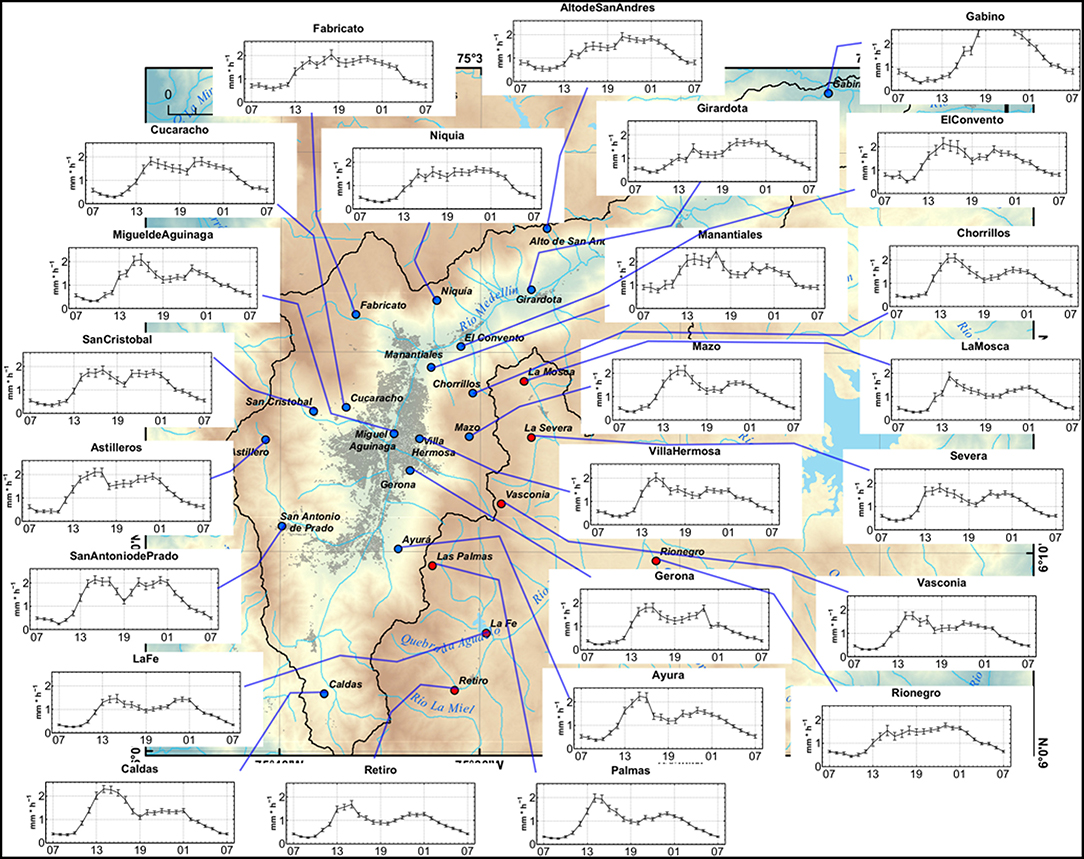
Figure 4. Long-term mean (1998–2005) diurnal cycle of rainfall intensity at rain gauges over the study region (Equation 1). Error bars represent the standard error of the mean. Computations based on hourly series excluding zeros. Blue (Red) points in the map represent the raingauges located on the Aburrá Valley (San Nicolás Plateau).
The highest diurnal intensity of rainfall occurs pole-ward in the Aburrá Valley. Caldas (southernmost) shows the highest afternoon peak, and Gabino (northernmost) the highest night peak. Interestingly, both poles show different climatic and physical conditions, since Caldas is characterized by higher terrain elevations and steeper slopes, and Gabino is in the flattest and lowest terrain of the study area.
Variability of the Diurnal Cycle Through the Annual Cycle
As we mentioned earlier, the study of Poveda et al. (2005) provided evidence that the phase and amplitude of the diurnal cycle of Andean rainfall exhibit strong variability through the annual cycle even for the nearest neighbor rain gauges (see their Figure 2). We aim to investigate whether this is also the case for the present study region. Figure 5 shows the march of the diurnal cycle of hourly rainfall throughout the annual cycle as a percent of total daily rainfall. A remarkably coherent pattern can be seen for the whole set of raingauges: the diurnal cycle is predominantly uni-modal throughout the year, but the phase does shift with the phase of the annual cycle (season). That is why the apparent bimodality of the diurnal cycle shown in Figure 4 arises from the superposition of two different uni-modal peaks occurring at different epochs of the year. As a matter of fact, all raingauges exhibit maximum rainfall around 22:00–02:00 LST from May to September, and 13:00–16:00 LST from October to April. During some short transition periods, a slightly bimodal diurnal cycle shows up particularly during May and September. From these results we conclude two important facts: (i) there is clear-cut coherent behavior of rainfall at diurnal and annual cycles, and (ii) unlike other regions located on the Andes of Colombia (Poveda et al., 2005), the neighboring regions studied in the present work exhibit a coherent behavior in the diurnal cycle of rainfall (percent and intensity) throughout the annual cycle. This observation suggests that similar physical mechanisms are at play to control the spatial dynamics of rainfall in the region at the timescales studied.
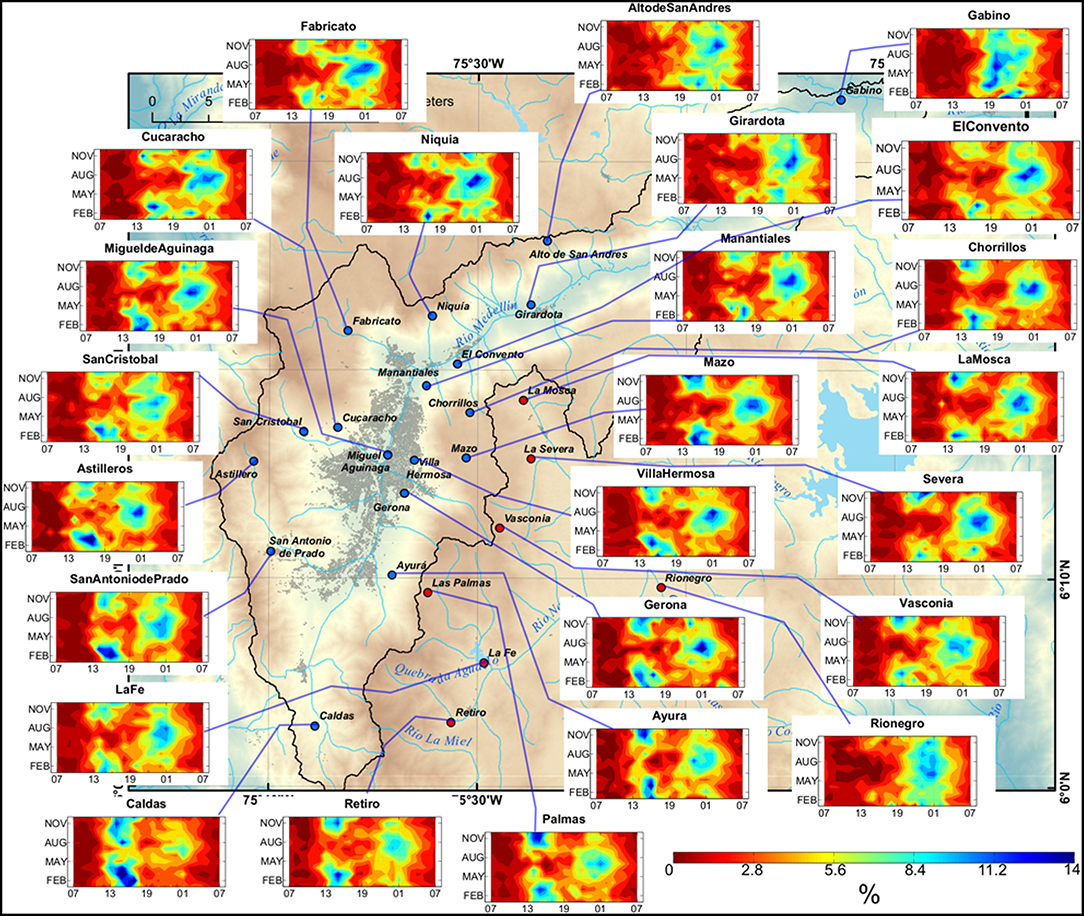
Figure 5. Average diurnal cycle of hourly rainfall through the annual cycle, as a percent of total daily rainfall (Equation 4). Computations based on hourly series including zeros. Blue (Red) points in the map represent the raingauges located on the Aburrá Valley (San Nicolás Plateau).
To explain the seasonal march of the diurnal cycle, we first analyzed the seasonal source moistures over the study region. Figure 6 shows seasonal air parcel backward trajectories ending at the Aburrá Valley, extracted every 15 days based on the TRAJ3D Model (https://www.esrl.noaa.gov/psd/cgi-bin/data/trajtool/traj.pl) based on a Lagrangian approach. The analysis included several pressure levels during a representative Neutral (2013), El Niño (1997–1998) and La Niña (1998–1999) years. These results confirm that during a Neutral year (Non-ENSO), the humidity sources vary seasonally in the Aburrá Valley mainly between the Caribbean and Pacific Oceans. Moreover, the trajectories plotted at 925 and 850 hPa are representative of the dynamics of the LLJs acting in the region (CHOCO, Caribe and CAO). During J-J-A and S-O-N, air parcels predominantly travel from the eastern Pacific Ocean as for D-J-F and M-A-M the main source is the Caribbean Sea. Durán-Quesada et al. (2012) describe the same seasonal variability of moisture sources over northwestern Colombia and Central America (see their Figure 3) for the neutral conditions of ENSO. Those authors also remark the importance of local conditions to control the transport of moisture entering into these regions; and, in the case of northwestern Colombia, they confirmed that local evaporation is an important source of humidity at seasonal scales. In summary, the variability of the humidity sources through the year in the Aburrá Valley is the main driver of the phase change of the diurnal cycle along the year.
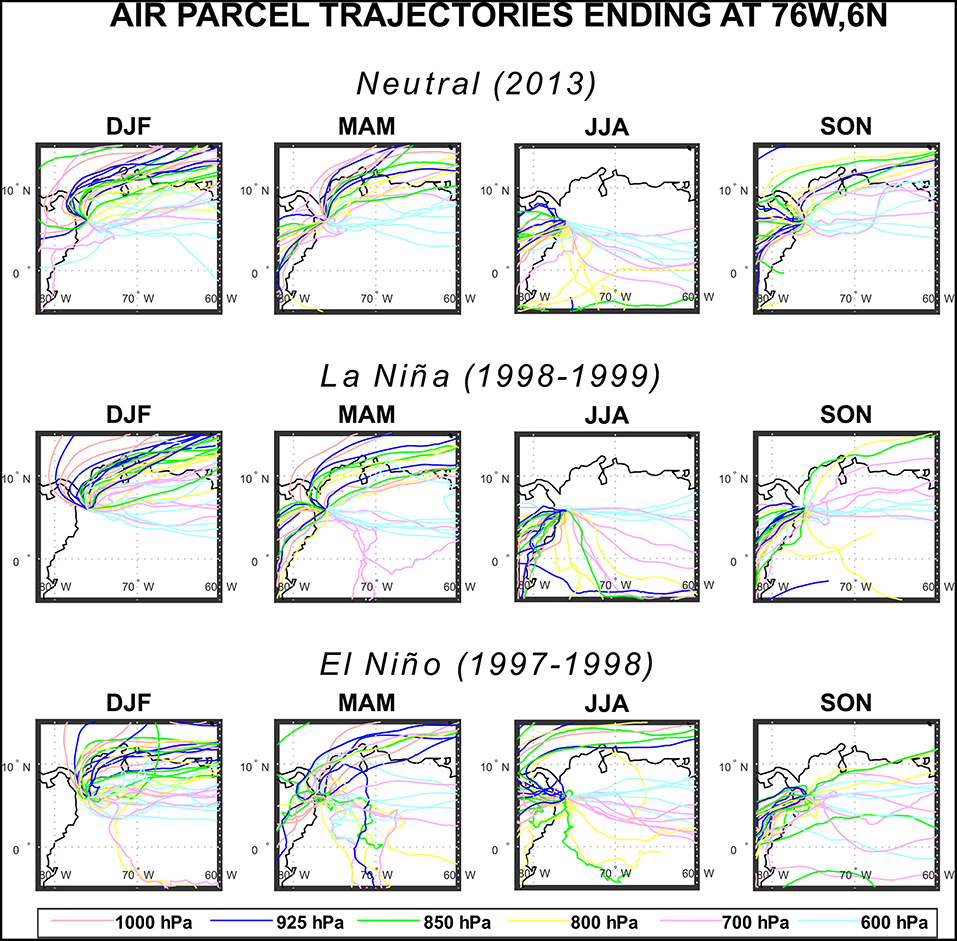
Figure 6. Seasonal air parcel trajectories ending at 76W, 6N (Aburrá Valley) for a Neutral year (2013), La Niña (1998–1999) and El Niño (1997–1998) along 1,000, 925, 850, 800, 700 and 600 hPa. Data source: https://www.esrl.noaa.gov/psd/cgi-bin/data/trajtool/traj.pl.
Moreover, the timing of daily maximum convection in tropical South America responds to location and highly localized orographic effects (Mapes et al., 2003b; Zuluaga and Poveda, 2004; Vera et al., 2006b). Previous studies evidence a general late afternoon peak in the diurnal cycle of convective rainfall over the continent (Taylor et al., 2012; Folkins et al., 2014). Specifically, the afternoon rainfall maximum in the Aburrá Valley during October-April (Figure 5) is related to convective dynamics over the Andean slopes (Houze, 2012). Moisture and heat availability during the morning and noon hours favor buoyancy, atmospheric instability, and convective processes during the afternoon. In this regard, Zuluaga and Houze (2015) evidence that the occurrence of Deep Convective Cores (DCC) are related to topographic features over the mountain ranges of the northern Andes and that DCCs are produced by strong updrafts maximized by diurnal daytime heating at local spatial scales.
Otherwise, the midnight and early-morning peak of rainfall during J-J-A in the Aburrá Valley (one of the two drier seasons) coincides with a cluster of Mesoscale Convective Systems (MCS) developed over the Gulf of Panamá, Colombia's Pacific Coast and the western slope of the Colombian Andes (Zuluaga and Houze, 2015; Jaramillo et al., 2017). Those MCSs are developed during the evening and reaching maximum sizes during the midnight and early-morning hours (Mapes et al., 2003a; Mejia and Poveda, 2005; Vera et al., 2006a; Zuluaga and Houze, 2015; Jaramillo et al., 2017). Storms of this type are contained in Wide Convective Cores (WCC) (Houze, 2012; Zuluaga and Houze, 2015) covering regional precipitation areas wider than those comprised by DCC. This fact contributes to explain the frequency of convective clouds during the J-J-A season in the study area.
Another causal factor to the midnight-early morning peak during the J-J-A season is the transport of moisture from the Magdalena River Valley, located to the east of the study region. Evaporation from the bottom of the valley (300 m a.s.l.) during the afternoon hours, along with anabatic circulation from the bottom of the valley to the top of the Central Cordillera, and the generalized transport of moisture by the easterly trade winds over the western slope of the Magdalena River Valley which, upon ascent, contribute to condensation and rainfall during the midnight hours over the study region. Using a regional model to represent the local atmospheric circulation patterns in the intra Andean topography of Colombia, the study of Posada-Marín et al. (2018) provides evidence about the existence of such anabatic circulation from the bottom to the top of the Magdalena Valley that contributes with the formation of mid-night storms in the San Nicolás Plateau and the Aburrá Valley.
We also aim to provide further evidence regarding the influence of afternoon processes taking place on the Magdalena River valley on nightly rainfall events over the study regions. Figure 7 shows a set of nine variables presented as averaged Hovmöller diagrams over 5°N to 6°N at 3-hourly timesteps highlighting the location of the Magdalena Valley (around 74°W to 75°W), the San Nicolás Plateau and the Aburrá Valley (around 75.5°W) for specific events during 15–21 July of 1998. First, the diurnal cycle of surface skin temperature shows maximum values at afternoon hours and this also delineates the latitudinal extension of the Magdalena Valley (74 to 75°W) which shows the highest temperatures in its bottom (Figure 7A). Intense heating at afternoon hours is connected with the highest evaporative rates in the region; notice the strong diurnal cycle of ET, particularly over the Magdalena Valley. Soil moisture content is lower at afternoon hours due to surface evaporation over Magdalena Valleys and is higher at early-morning hours over the Aburrá Valley (Figures 7B–C). Also, soil moisture availability spatially increases from eastern to western in the selected domain. Interestingly, the surface eastward wind shows two spatial modes along the diurnal cycle over the Magdalena Valley, during the night and early-morning these winds are strong over the western slope of the Magdalena Valley and during the afternoon over the eastern slope (Figure 7D). Indeed, the surface specific humidity shows a strong connection between both valleys (Magdalena and Aburrá) between 0z (18:00 LST) and 6z (0:00 LST) as shown in Figure 7E. Transport of moisture is comparatively stronger along the lower atmospheric pressure levels (700 hPa) than over surface revealing the action of the easterly trade winds in the high atmosphere allowing the uphill humidity movement along the slopes of the Magdalena Valley (Figure 7F). The transport of moisture between both valleys is particularly strong from the afternoon up to midnight. This also coincides with the backward trajectories at 800, 700, and 600 hPa ending in the Aburrá Valley for J-J-A (Figure 6) which are predominantly forced by the easterly trade winds allowing the moisture-wave connection between the two valleys. Total precipitable water vapor and total water vapor instantaneous show the high availability of moisture over the Magdalena Valley and its important role as a source of humidity in the region (Figures 7G–H). These variables show the highest values at midnight and early-morning hours. Finally, the Hovmöller diagram for hourly precipitation from TRMM-3B42 illustrates the connected storms coming from the Magdalena Valley to the Aburrá Valley in the nights and early-morning of every day between 16 and 20 July 1998 (Figure 7I).
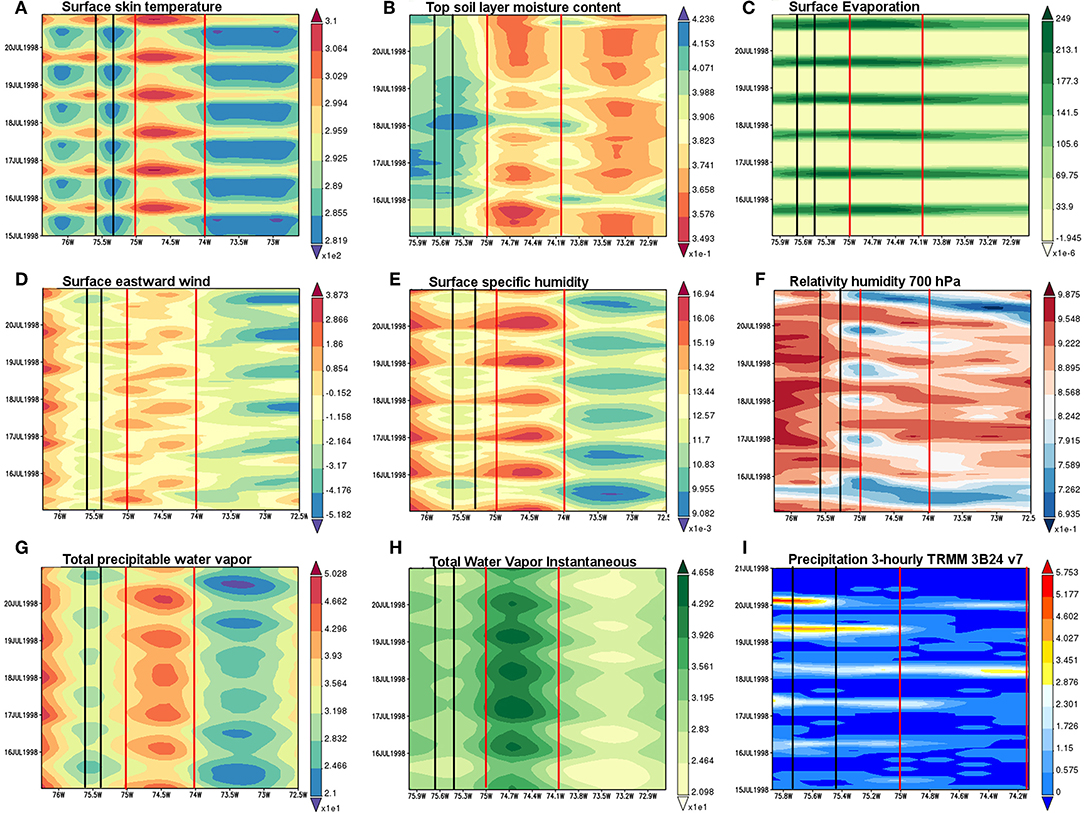
Figure 7. Hovmöller time-latitude diagrams averaged over 5N to 6N for the 6-day period spanning between 15 and 21 July of 1998 with 3-h timesteps (A) Surface Skin temperature, (B) Top soil layer moisture, (C) Surface Evaporation, (D) Surface eastward wind, (E) Surface Specific Humidity, (F) Relative humidity at 700 hPa after moist (MERRA 2), (G) Total precipitable water, (H) Total water vapor, and (I) Precipitation (TRMM 3B42 v.7). These diagrams were produced with the Giovanni online data system, developed and maintained by the NASA GES DISC (Acker and Leptoukh, 2007). The Magdalena Valley (Aburrá Valley) location is enclosed in red (black) lines.
Hovmöller diagrams corresponding to other specific events are presented in the Supplementary Material No. 4 for the same variables and the complete June-July-August season of 1998. Those results reinforce the evidence that evaporation from the bottom of the Magdalena River valley during the afternoon provides the moisture and the easterly anabatic circulation transport it to the San Nicolás Plateau and the Aburrá Valley during the night hours to produce midnight and early morning (mostly cirrus type and shallow convective) storms.
A complete sequence of 3-hourly TRMM 3B42 precipitation over Colombia during July 1998 also supports the hypothesis of the described phenomena connecting both valleys is shown in the animation included in Figure S1, evidencing the alternation of these events with the incoming convective precipitation from the Magdalena River Valley and the eastern Pacific into the Aburrá Valley.
Similarly, satellite imagery from GOES-R shows the influence of local evaporative sources coming from the bottom of the Magdalena Valley in the midnight and early-morning rainfall over to the Aburrá Valley during the J-J-A season. We present this evidence in Figure S2 in form of animations of the band 4 (1.37 μm) for the hourly time spanning the period 24-27 of August 2017. We clearly identify the formation of clouds over the lowlands of the Magdalena Valley, which move later on to the San Nicolás Plateau and the Aburrá Valley in the early-morning peak of the 24, 26 and 27 of August. In Figures S3A–C, we also include three animations of the radar reflectivity operated by SIATA (https://siata.gov.co) of three storms corresponding to August 4/2018 (Figure S3A), October 3/2018 (Figure S3B), and November 25/2018 (Figure S3C). The August's storm over the Aburrá Valley occurred in the early morning and the humidity source located in the Magdalena Valley is easily tracked. This storm traveled from east to west forced by the trade winds, usually stronger during this season (J-J-A). Again, this fact points out the connection between precipitation events occurring over the Magdalena and Aburrá Valleys. The October storm is a typical example of the afternoon peak of the season October-November with the moisture mainly transported from the Pacific Ocean. Finally, the November storm is also representative of the afternoon peak but the moisture source is local possibly related to soil moisture excesses at the end of the rainy season, associated with strong isolation at morning hours and convective mechanisms to produce the precipitation.
All the previous evidences confirm the important role of diverse afternoon and evening dynamic and thermodynamic processes that take place in the Magdalena River Valley on the development of nightly storms over the San Nicolás Plateau and the Aburrá Valley, particularly during the J-J-A season. This conclusion also confirms that precipitation recycling is a very important rainfall-generating mechanism along the tropical Andes, reaching values of 70–80% (Zemp et al., 2014).
Regarding longer time scales, Durán-Quesada et al. (2017) emphasized the key role of the CHOCO and Caribbean LLJs to determine the seasonal patterns of precipitation over northwestern South America. They argued that the connection between the ITCZ dynamics and the interaction between these LLJs could provide a better understanding of the regional precipitation patterns, although the importance of the dynamics of MCSs over the region cannot be overstated. Also, we showed that the trajectories of air parcels ending at the Aburrá Valley over the 925 and 850 hPa are connected with the moisture coming from the CLLJ (Caribbean Sea) and the CHOCO Jet (Eastern Pacific). The relation between precipitation and the aforementioned LLJs in northwestern South America and over the study region is also revealed through correlation analysis between precipitation and zonal winds at 925 and 850 hPa from ERA-Interim (Figure 8). Precipitation over the entire region shows very high statistically significant correlations between the zonal winds around the CLLJ zone during M-A-M, J-J-A, and S-O-N, although the effect over the Aburra's Valley rainfall is stronger for J-J-A. Moreover, the connection is more evident between the CHOCO Jet and rainfall during J-J-A and S-O-N (Figure 8). Figure 9 shows monthly lagged correlations between the specific humidity at 925 hPa in the Choco Jet region with precipitation rate over western Colombia. The simultaneous correlation reaches a value of 0.6 (p > 0.05) and 0.4 (p > 0.05) up to 4 months-lag. This fact confirms the general influence of the CHOCO Jet on precipitation over Colombia. Also, Poveda et al. (2006) studied the seasonal effects of El Niño and La Niña on the transport of moisture by the CHOCO Jet. They found that La Niña (El Niño) enhances (diminishes) the advection of moisture of the CHOCO Jet. Higher (Lower) value of the moisture transport carrying for this LLJ is ~0.04 m/s*kg/kg (0.03 m/s*kg/kg) in October for La Niña (Niño). Durán-Quesada et al. (2017) also highlighted the interannual modulation of ENSO on the CHOCO Jet and the CLLJ as main sources of humidity over Southern Central America and Northwestern Colombia.
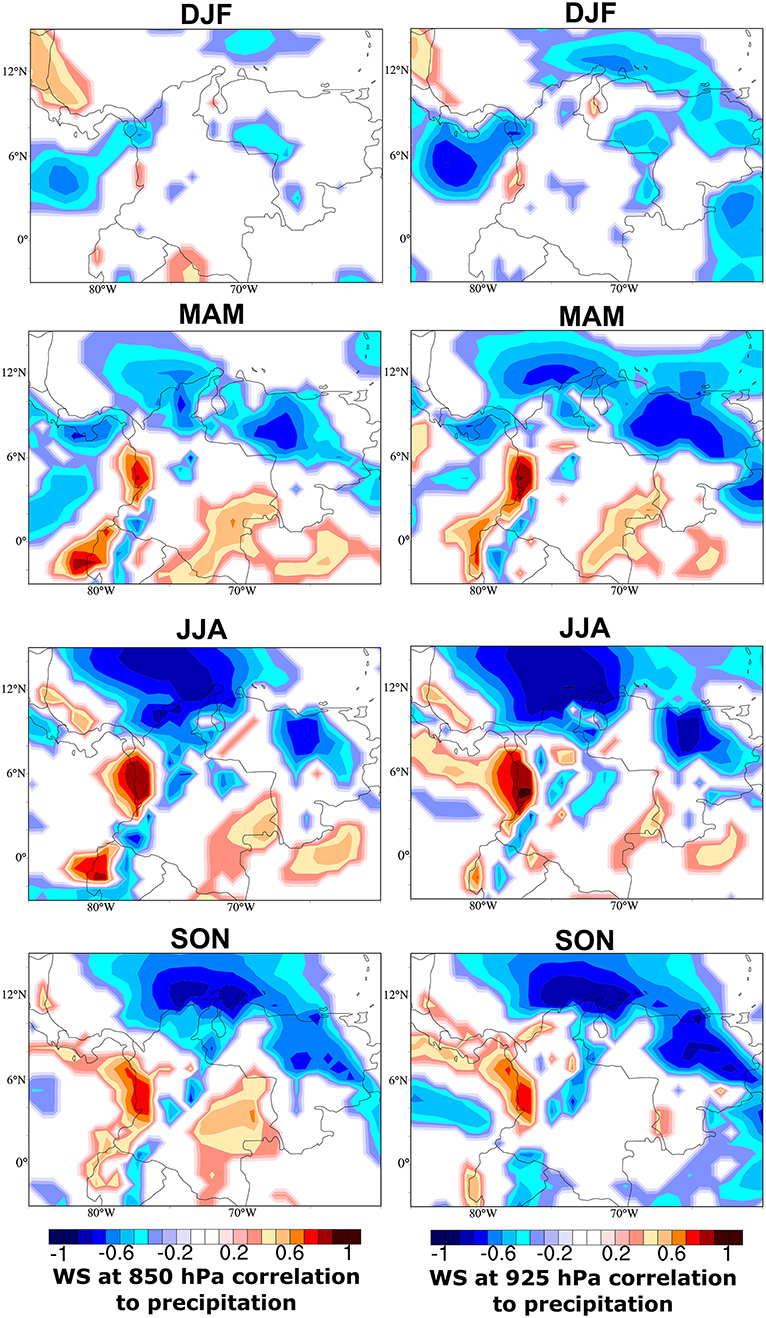
Figure 8. Seasonal iso-correlations between wind speeds at 850 hPa (left)/ 925 hPa (right) and precipitation rates over Colombia for the 1979-2017 period. Data source: ERA-Interim Reanalysis, prepared using the Climate Reanalyzer (https://ClimateReanalyzer.org), Climate Change Institute, University of Maine, USA.
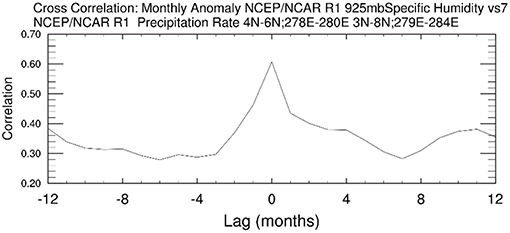
Figure 9. Monthly lagged correlations between anomalies of specific humidity at 925 hPa in Choco LLJ region and precipitation rates over western Colombia. Data source: NCEP/NCAR R1.
At this point, it is also necessary to discuss the possible role of the three low-level jets discussed in the introduction on the seasonal variability of rainfall over the Aburrá Valley. The annual cycle of average wind velocities of the CHOCO, Caribbean, and CAO LLJs, and their variability through the diurnal cycle, during the study period, are shown in Figure 10. The three LLJs show a distinctive annual cycle, with maxima occurring during different seasons of the year: D-J-F for the CAO, J-J-A for the Caribbean, and S-O-N for the CHOCO LLJ (Figure 10). Wind velocities of the CHOCO and CAO LLJs are complementary (out-of-phase) through the year. Diurnal cycles of the LLJs show that the CAO exhibits highest wind velocities during the early morning hours throughout the year. This behavior agrees well with the highest activity of the Caribbean LLJ (Figure 3). The CHOCO LLJ exhibits higher velocities in the afternoon and nightly hours. In this context, the midnight-early morning peak of the diurnal cycle of rainfall during J-J-A in the Aburrá Valley is concurrent with the highest activity of the Caribbean LLJ (coinciding with stronger easterly trade winds); while during those months the CHOCO LLJ is moderately strong at nightly hours (Figure 10). The afternoon rainfall peak in the study region during S-O-N coincides with a stronger CHOCO Jet and a weaker Caribbean LLJ at these months and hours. During the first months of the year (January to May/afternoon peak rainfall) the zonal winds activity comes mainly from the CAO and Caribbean LLJs (Figure 10). Thus, the activity of the three LLJs, varying at seasonal and diurnal timescales, contribute to the regional rainfall-generating mechanisms by transporting moisture from neighboring (continental and oceanic) regions to the study region.
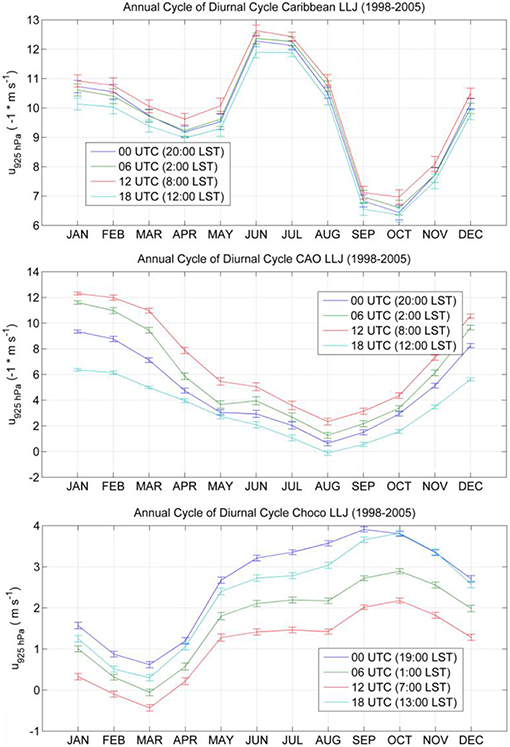
Figure 10. Average diurnal cycle of the zonal winds at 925 hPa through the annual cycle for the period 1998–2005, at the core of the Caribbean (top), CAO (middle), and CHOCO (bottom) LLJs. Data source: ERA-Interim Reanalysis. Error bars denote the standard error of the mean.
Conclusions
We studied the coherence of rainfall intensities over 25 raingagues located along two neighboring regions of the Central range of the Colombian Andes during the 1998–2005 period, at diurnal, seasonal, and annual timescales. Our results indicate that the annual cycle is bimodal at the regional scale, with higher rainfall occurring during April-May (A-M) and September-October (S-O), while lower rainfall occurs during D-J-F and J-J-A. An indiscriminate analysis of the average diurnal cycle shows a general false bimodal diurnal cycle with two precipitation maxima occurring between 15:00–17:00 LST, and 23:00–02:00 LST, and minima during early morning hours (07:00–10:00 LST) and noon. A more careful analysis allowed us to find that such apparent bimodal diurnal cycle results from the superposition of different uni-modal diurnal cycles with a shifting phase during the seasonal march, with rainfall peaking around 13:00–16:00 h during October-April, and around 22:00–02:00 h during May-September.
The physical mechanisms driving this phase shift during the annual cycle can be synthesized as follows. The life cycle of diverse convective processes influences orographic rainfall in the study zone. Small (deep) and large (wide) convective systems approximately correspond with afternoon and early-morning peaks. This convective life-cycle is modulated at diurnal and annual timescales by regional mechanisms such as (i) the position of the ITCZ, (ii) transport moisture by the Caribbean, CHOCO, and CAO LLJs, (iii) a cluster of MCSs developed over the neighboring maritime (Pacific Coast of Colombia and Panamá Gulf); during J-J-A, the early morning peak of rainfall is due to highest activity of this MCSs cluster, (iv) moisture from afternoon evaporation processes at the bottom of the inter-Andean Magdalena Valley transported by anabatic and easterly trade winds that specifically influences the early morning peak over the study area, and (v) moisture and heat availability during the morning and noon hours that trigger instabilities and convective processes related to the afternoon peak during the wetter months of the mean annual cycle.
We confirm a spatial coherence among the diurnal and annual rainfall cycles over the Aburrá Valley. Previously, this type of coherence could not be identified in the work of Poveda et al. (2005). Those authors found strong changes in small distances among the rainfall cycles over large Andean regions. In the Aburrá Valley, this coherence is consistent through the slopes (western-eastern) and over the main south (highest terrain elevations) to north (lowest terrain elevations) predominant location of the Andes. The spatial coherence is stronger at the center of the Aburrá valley with some differences in the north and south extremes: at the southernmost region of the valley the average diurnal rainfall cycle is mostly concentrated in the afternoon peak (deep convective cores) while the northernmost diurnal rainfall cycle is concentrated in the midnight and early morning peak.
Author Contributions
GP and JB-S designed, proposed, and conducted the research. GP, JB-S, EA, and AC wrote the paper. JB-S, AC, and EA performed the experiments. GP reviewed the paper.
Funding
The work of JB-S was funded by The Canadian Queen Elizabeth II Diamond Jubilee Scholarships (QES), a partnership among Universities in Canada, the Rideau Hall Foundation (RHF), Community Foundations of Canada (CFC). The QES-AS is made possible with financial support from IDRC and SSHRC. The work of GP and EA was supported by Universidad Nacional de Colombia at Medellin. We thank Empresas Públicas de Medellin for providing access to data.
Conflict of Interest Statement
The authors declare that the research was conducted in the absence of any commercial or financial relationships that could be construed as a potential conflict of interest.
Acknowledgments
We also acknowledge to provide data to this research to ERA-Interim, MERRA and NCEP Reanalysis as well as TRMM and GOES. Thanks to the providers of the analysis tools Giovanni (NASA), Climate Explorer, Climate Reanalyzer, and RAMSDIS CIRA (GOES). Thanks to SIATA for providing the animations of storms over the Aburrá Valley.
Supplementary Material
The Supplementary Material for this article can be found online at: https://www.frontiersin.org/articles/10.3389/feart.2019.00092/full#supplementary-material
References
Acker, J. G., and Leptoukh, G. (2007). Online analysis enhances use of NASA earth science data. Eos. Trans. AGU. 88, 14–17. doi: 10.1029/2007EO020003
Alvarez-Villa, O. D., Vélez, J. I., and Poveda, G. (2011). Improved long-term mean annual rainfall fields for Colombia. Int. J. Climatol. 31, 2194–2212. doi: 10.1002/joc.2232
Amador, J. A., and Magaña, V. O. (1999). “Dynamics of the low-level jet over the Caribbean, ” in 23rd Conference on Hurricanes and Tropical Meteorology. Amercan Meteorological Socity (Dallas, TX), 868869.
Bedoya-Soto, J. M., Poveda, G., Trenberth, K. E., and Vélez-Upegui, J. J. (2018). Interannual hydroclimatic variability and the 2009-2011 extreme ENSO phases in Colombia: from Andean glaciers to Caribbean lowlands. Theor. Appl. Climatol. 135, 1531–1544. doi: 10.1007/s00704-018-2452-2t
Bedoya-Soto, M., and Poveda, G. (2008). “Sobre una posible influencia de la precipitación del Valle de San Nicolás en eventos de precipitación sobre el Valle de Aburrá,” in VIII CCMCI - Cambio Climático, Recursos Naturales y Desarrollo Sostenible, Quibdó.
Durán-Quesada, A. M., Gimeno, L., and Amador, J. (2017). Role of moisture transport for Central American precipitation. Earth Syst. Dynam. 8, 147–161. doi: 10.5194/esd-8-147-2017
Durán-Quesada, A. M., Reboita, M., and Gimeno, L. (2012). Precipitation in tropical America and the associated sources of moisture: a short review. Hydrol. Sci. J. 57, 612–624. doi: 10.1080/02626667.2012.673723
Eslava, J. A. (1994). Acerca de la distribución espacio-temporal de la precipitación en la región del Pacífico Colombiano. Atmósfera. 22, 71–80.
Espinoza-Villar, J. C., Ronchail, J., Guyot, J. L., Cochonneau, G., Naziano, F., Lavado, W., et al. (2009). Spatio-temporal rainfall variability in the Amazon basin countries (Brazil, Peru, Bolivia, Colombia, and Ecuador). Int. J. Climatol. 29:1574–1594. doi: 10.1002/joc.1791
Folkins, I., Mitovski, T., and Pierce, J. R. (2014). A simple way to improve the diurnal cycle in convective rainfall over land in climate models. J. Geophys. Res. Atm. 5, 2113–2140. doi: 10.1002/2013JD020149
Hastenrath, S. (2002). The intertropical convergence zone of the eastern Pacific revisited. Int. J. Climatol. 22, 347–356. doi: 10.1002/joc.739
Houze, R. A. (2012). Orographic effects on precipitating clouds. Rev. Geophys. 50:1001. doi: 10.1029/2011RG000365
Hoyos, I., Dominguez, F., Ca-on-Barriga, J., Martínez, J. A., Nieto, R., Gimeno, L., et al. (2017). Moisture origin and transport processes in Colombia, northern South America. Clim. Dyn. 50, 971–990. doi: 10.1007/s00382-017-3653-6t
Hurtado, A. F., and Poveda, G. (2009). Linear and global space-time dependence and Taylor hypotheses for rainfall in the tropical Andes. J. Geophys. Res. 114:D10105. doi: 10.1029/2008JD011074
Hurtado-Montoya, A. F., and Mesa-Sánchez, O. J. (2014). Reconstrucción de los campos de precipitación mensual en Colombia. DYNA. 81, 251–258. doi: 10.15446/dyna.v81n186.40419
Jaramillo, L., Poveda, G., and Mejía, J. F. (2017). Mesoscale convective systems and other precipitation features over the tropical Americas and surrounding seas as seen by TRMM. Int. J. Climatol. 37, 380–397. doi: 10.1002/joc.5009
Killeen, T. J., Douglas, M., Consiglio, T., Jorgensen, P. M., and Mejía, J. (2007). Dry spots and wet spots in the Andean hotspot. J. Biogeograp. 34, 1357–1373. doi: 10.1111/j.1365-2699.2006.01682.x
León, G. E., and Narávez, G. (2001). Caracterización y zonificación climática de la región Andina. Meteorología Colombiana 4, 121–126.
López, M. E., and Howell, W. E. (1967). Katabatic winds in the equatorial andes. J. Atmos. Sci. 24, 29–35. doi: 10.1175/1520-0469(1967)024<0029:KWITEA>2.0.CO;2
Mapes, B. E., Warner, T. T., Xu, M., and Negri, A. J. (2003a), Diurnal patterns of rainfall in northwestern South America. part I: observations context. Mon. Wea. Rev. 131, 799–812. doi: 10.1175/1520-0493(2003)131<0799:DPORIN>2.0.CO;2
Mapes, B. E., Warner, T. T., Xu, M., and Negri, A. J. (2003b), Diurnal patterns of rainfall in northwestern South America. part III: diurnal gravity waves nocturnal convection off-shore. Mon. Wea. Rev. 131, 830–844. doi: 10.1175/1520-0493(2003)131<0830:DPORIN>2.0.CO;2
Martínez, M. T. (1993). Principales sistemas sinópticos en Colombia y su influencia en el comportamiento del tiempo. Atmósfera 16, 1–10.
Mejía, F., Mesa, O., Poveda, G., Vélez, J., Hoyos, C., Mantilla, R., et al. (1999). Distribución espacial y ciclos anual y semianual de la precipitación en Colombia. Dyna, 127, 7–24. doi: 10.1029/JD093iD09p11022
Mejia, J. F., and Poveda, G. (2005). Atmospheric environments of mesoscale convective systems over Colombia during 1999 using TRMM radar and microwave products and NCEP/NCAR reanalysis [in Spanish]. Rev. Acad. Colomb. Cienc. 29, 495–514.
Oster, R. (1979). Las precipitaciones en Colombia. Revista Colombia Geográfica Instituto Geográfico Agustín Codazzi. Bogotá. 6, 5–147.
Pabón, J. D., and Dorado, J. (2008). Intraseasonal variability of rainfall over northern South America and Caribbean region. Earth Sci. Res. J. 12, 194–212.
Pita Suárez-Cobián, P. (1959). El período diurno en las lluvias de los Andes ecuatoriales. Rev. Acad. Colomb. Cienc. X, 327–335.
Posada-Marín, J. A., Rendón, A. M., Salazar, J. F., Mejía, J. F., and Villegas, J. C. (2018). WRF downscaling improves ERA-Interim representation of precipitation around a tropical Andean valley during El Niño: implications for GCM-scale simulation of precipitation over complex terrain. Clim.Dyn. 52, 3609–3629. doi: 10.1007/s00382-018-4403-0
Poveda, G. (2004). The hydro-climatology of Colombia: a synthesis from inter-decadal to diurnal timescales [in Spanish]. Rev. Acad. Colomb. Cienc. 28, 201–222.
Poveda, G. (2011). Mixed memory, (non) Hurst effect, and maximum entropy of rainfall in the tropical Andes. Adv. Water Resour. 34, 243–256. doi: 10.1016/j.advwatres.2010.11.007
Poveda, G., Alvarez, D. M., and Rueda, O. (2011). Hydro-climatic variability over the Andes of Colombia associated with ENSO: a review of climatic processes and their impact on one of the Earth's most important biodiversity hotspots. Clim. Dyn. 36, 2233–2249. doi: 10.1007/s00382-010-0931-y
Poveda, G., Jaramillo, A., Gil, M. M., Quiceno, N., and Mantilla, R. (2001). Seasonality in ENSO related precipitation, river discharges, soil moisture, and vegetation index (NDVI) in Colombia. Water Resour. Res. 37, 2169–2178. doi: 10.1029/2000WR900395
Poveda, G., Jaramillo, L., and Vallejo, L. F. (2014). Seasonal precipitation patterns along pathways of South American low-level jets and aerial rivers. Water Resour. Res. 50, 98–118. doi: 10.1002/2013WR014087
Poveda, G., and Mesa, O. J. (1997). Feedbacks between hydrological processes in tropical South America and large scale oceanic atmospheric phenomena. J. Climate. 10, 2690–2702. doi: 10.1175/1520-0442(1997)010<2690:FBHPIT>2.0.CO;2
Poveda, G., and Mesa, O. J. (1999). The CHOCO low-level jet and two others jets over Colombia: climatology and variability during ENSO [in Spanish]. Rev. Acad. Colomb. Cienc. 23, 517–528.
Poveda, G., and Mesa, O. J. (2000). On the existence of Lloró (the rainiest locality on Earth): enhanced ocean-atmosphere-land interaction by a low-level jet. Geophys. Res. Lett. 27, 1675–1678. doi: 10.1029/1999GL006091
Poveda, G., and Salas, H. D. (2015). Statistical scaling, shannon entropy, and generalized space-time q-entropy of rainfall fields in tropical South America. Chaos. 25:075409. doi: 10.1063/1.4922595
Poveda, G., Vélez, J. I., Mesa, O. J., Cuartas, A., Barco, J., Mantilla, R. I., et al. (2007). Linking long-term water balances and statistical scaling to estimate river flows along the drainage network of Colombia. J. Hydrol. Eng. 12, 4–13. doi: 10.1061/(ASCE)1084-0699(2007)12:1(4)
Poveda, G., Waylen, P. R., and Pulwarty, R. (2006). Modern climate variability in northern South America and southern Mesoamerica. Palaeogeogr. Palaeoclimatol. Palaeoecol. 234, 3–27. doi: 10.1016/j.palaeo.2005.10.031
Poveda, G. O. J., Mesa, L. F., Salazar, P. A., Arias, H. A., Moreno, S. C., Vieira, P. A., et al. (2005). The diurnal cycle of precipitation in the Tropical Andes of Colombia. Mon. Wea. Rev. 133, 228–240. doi: 10.1175/MWR-2853.1
Rueda, O. A., and Poveda, G. (2006). Variabilidad espacial y temporal del Chorro del Chocó y su efecto en la hidroclimatología del Pacífico Colombiano. Meteorología Colombiana. 10, 132–145.
Sakamoto, M., Ambrizzi, T., and Poveda, G. (2011). Moisture sources and life cycle of convective systems over western Colombia. Adv. Meteorol. 11:890759. doi: 10.1155/2011/890759
Salas, H. D., and Poveda, G. (2015). Scaling of entropy and multi-scaling of the time generalized q-entropy in rainfall and streamflows. Physica A. 423, 11–26. doi: 10.1016/j.physa.2014.12.015
Schmidt, R. (1952). Die Niederschlagsverteilung im andinen Kolumbien, Bonner Geogr, Abhandlungen. Heft 9:1952.
Schmit, T. J., Griffith, P., Gunshor, M. M., Daniels, J. M., Goodman, S. J., and Lebair, W. J. (2017). A closer look at the ABI on the GOES-R series. Bull. Am. Meteorol. Soc. 98, 681–698. doi: 10.1175/BAMS-D-15-00230.1
Sierra, J. P., Arias, P. A., and Vieira, S. C. (2015). Precipitation over Northern South America and its seasonal variability as simulated by the CMIP5 models. Adv. Meteorol. 2015:22. doi: 10.1155/2015/634720
Snow, J. W. (1976). “The climate of northern South America,” in Climates of Central and South America, eds W. Schwerdtfeger (Amsterdam: Elsevier), 295–403.
Taylor, C. M., de Jeu, R. A., Guichard, F., Harris, P. P., and Dorigo, W. A. (2012). Afternoon rain more likely over drier soils. Nature. 489, 423–426. doi: 10.1038/nature11377
Torrealba, E. R., and Amador, J. A. (2010). La corriente en chorro de bajo nivel sobre los Llanos Venezolanos de Sur America. Revista de Climatologia. 10, 1–20.
Trewartha, G. T. (1981). The earth's problem climates. Madison, WI: University of Wisconsin Press, 371.
Trojer, H. (1958). Meteorología y climatología de la vertiente del Pacífico Colombiano. Rev. Acad. Colomb. Cienc. 10, 199–219.
Trojer, H. (1959). Fundamentos para una zonificación meteorológica y climatológica del trópico y especialmente de Colombia. Seminarios Cenicafé. 10, 289–373.
Vera, C., Baez, J., Douglas, M., Emmanuel, C., Marengo, J., Meitin, J., et al. (2006a). The South American low-level jet experiment. BAMS 87, 63–77. doi: 10.1175/BAMS-87-1-63
Vera, C., Higgins, W., Amador, J., Ambrizzi, T., Garreaud, R., Gochis, D., et al. (2006b). Toward a unified view of the American monsoon systems. J. Climate 19, 4977–5000. doi: 10.1175/JCLI3896.1
Wang, C. (2007). Variability of the Caribbean low-level jet and its relations to climate. Clim. Dyn. 29, 411–422. doi: 10.1007/s00382-007-0243-z
Wang, C., and Enfield, D. B. (2001). The tropical western hemisphere warm pool. Geophys. Res. Lett. 28, 1635–1638. doi: 10.1029/2000GL011763
Wang, C., and Enfield, D. B. (2003). A further study of the tropical western hemisphere warm pool. J. Clim. 16, 1476–1493. doi: 10.1175/1520-0442-16.10.1476
Wang, C., Enfield, D. B., Lee, S.-K., and Landsea, C. W. (2006). Influences of the Atlantic warm pool on western hemisphere summer rainfall and Atlantic hurricanes. J. Clim. 19, 3011–3028. doi: 10.1175/JCLI3770.1
Warner, T. T., Mapes, B. E., and Xu, M. (2003). Diurnal patterns of rainfall in northwestern South America. part II: model simulations. Mon. Wea. Rev. 131, 813–829. doi: 10.1175/1520-0493(2003)131<0813:DPORIN>2.0.CO;2
Yepes, J., Poveda, G., Mejía, J. F., Moreno, L., and Rueda, C. (2019). CHOCO-JEX: a research experiment focused on the CHOCO low-leveljet over the Far Eastern Pacific and Western Colombia. Bull. Am. Meteorol. Soc. (2019). doi: 10.1175/BAMS-D-18-0045.1. [Epub ahead of print].
Zemp, D. C., Schleussner, C.-F., Barbosa, H. M. J., van der Ent, R. J., Donges, J. F., Heinke, J., et al. (2014). On the importance of cascading moisture recycling in South America. Atmos. Chem. Phys. 14, 13337–13359. doi: 10.5194/acp-14-13337-2014
Zuluaga, M. D., and Houze, R. A. (2015). Extreme convection of the near-equatorial Americas, Africa, and adjoining oceans as seen by TRMM. Mon. Wea. Rev. 143, 298–316. doi: 10.1175/MWR-D-14-00109.1
Keywords: tropical andes, mountain rainfall, annual cycle, diurnal cycle, Colombia
Citation: Bedoya-Soto JM, Aristizábal E, Carmona AM and Poveda G (2019) Seasonal Shift of the Diurnal Cycle of Rainfall Over Medellin's Valley, Central Andes of Colombia (1998–2005). Front. Earth Sci. 7:92. doi: 10.3389/feart.2019.00092
Received: 29 August 2018; Accepted: 15 April 2019;
Published: 16 May 2019.
Edited by:
Jing-Jia Luo, Bureau of Meteorology, AustraliaReviewed by:
Silvina A. Solman, Sea Research Center and Atmospheric Administration (CIMA), ArgentinaEduardo Zorita, Helmholtz Centre for Materials and Coastal Research (HZG), Germany
Rosane Rodrigues Chaves, Federal University of Rio Grande do Norte, Brazil
Copyright © 2019 Bedoya-Soto, Aristizábal, Carmona and Poveda. This is an open-access article distributed under the terms of the Creative Commons Attribution License (CC BY). The use, distribution or reproduction in other forums is permitted, provided the original author(s) and the copyright owner(s) are credited and that the original publication in this journal is cited, in accordance with accepted academic practice. No use, distribution or reproduction is permitted which does not comply with these terms.
*Correspondence: Juan Mauricio Bedoya-Soto, am1iZWRveWFAdW5hbC5lZHUuY28=