- 1Department of Earth Sciences, University of Firenze, Firenze, Italy
- 2Department of Earth Sciences, University of Pisa, Pisa, Italy
- 3Geohazards InSAR Laboratory and Modelling Group (InSARlab), Geoscience Research Department, Geological Survey of Spain (IGME), Madrid, Spain
The use of InSAR (Interferometric Synthetic Aperture Radar) products has greatly increased in the last years because of the technological advances in terms of both acquisition sensors and processing algorithms. The development of multi-interferogram techniques and the availability of free SAR analysis tools has significantly increased the number of worldwide applications of satellite measurements for mapping and monitoring geohazards. InSAR techniques excel in determining ground deformation in urban areas, where the coherence of the radar images is high, and the obtainable results are particularly reliable. Thus, measuring urban subsidence has always been one of the main targets of the InSAR analysis. In this paper, we present a brief review on the applications, in the last decades, of both single and multi-interferogram techniques to monitor ground lowering in urban areas along the Italian Peninsula. Because of its geological context, Italy is prone to slow natural subsidence phenomena sometimes aggravated and accelerated, especially along the coasts and in urbanized areas, by anthropogenic factors (i.e., groundwater overexploitation, consolidation in recent urban expansion, geothermal activities). The review will show how the interferometric data allowed the scientific community to increase the knowledge of the phenomena, map their spatial distribution, and reconstruct their temporal evolution. The final goal of the review is to demonstrate the added value of InSAR data in supporting groundwater management and urban development in Italy.
Introduction
Land subsidence is commonly referred to a gentle and graduate lowering or to a sudden sinking of the ground surface (Galloway and Burbey, 2011). Active subsidence can be due to natural and anthropogenic processes or to a combination of both (Galloway et al., 1999). It is defined as endogenic, if associated to geologically-related motions (i.e., volcanism, isostatic adjustments), or as exogenic, when an anthropogenic or natural cause leads to the removal of underground materials that trigger ground subsidence (Prokopovich, 1979). In this case, several causes can generate ground lowering, such as fluid withdrawal (water, petroleum, or gas), mining, tunneling, dissolution of carbonate rocks, organic matter oxidation processes or thawing of ground ice (Galloway et al., 1999).
These phenomena are a major threat in urban areas, where the continuous lowering over time can seriously damage linear infrastructures (bridges, roads, or railways), creating also serious problems to buildings stability when differential settlements occur. In this case, the derived economic loss is not negligible. For example, Hu et al. (2004) determined that in China 45 cities recorded severe subsidence, 11 of them reaching an accumulated lowering of more than 1 m of cumulative lowering, generating a total economic loss of around $100 million/year. In the city of Bangkok (Thailand) the cost of floods, worsen by intense land subsidence, has been estimated in $12 million/year (Phien-Wej et al., 2006). Faunt et al. (2016) reported that, between 1955 and 1972, the total cost of subsidence due to water withdrawal reached $1.3 billion only for California (United States). When catastrophic subsidence, i.e., sinkhole, is the active hazard, it may unfortunately result in human losses (Tomás et al., 2014).
Traditionally, urban subsidence has been monitored using leveling or GPS (Global Positioning System) techniques that allow precise measurements on selected benchmarks composing local or regional networks (for example Baldi et al., 2009). Although being reliable and accurate instruments, GPS-based monitoring networks lack in measurement point density and have a high cost of installation and maintenance, especially when regional networks are involved. For this reason, in the last 20 years the use of Differential Interferometric Synthetic Aperture Radar (D-InSAR) has been widely recognized as a valuable tool to monitor, with a relative low cost, urban deformation, sometimes integrating into preexistent leveling networks (Teatini et al., 2005).
The D-InSAR (Differential Synthetic Aperture Interferometry) technique, whose first application is dated back to late 1980’s (Gabriel et al., 1989), allows obtaining the cumulated deformation occurred between two acquisitions of a satellite SAR (Synthetic Aperture Radar) platform thanks to the phase changes registered by the radar images. This approach, known as radar interferometry, has rapidly growth and became a well-established Earth observation technique (Zhou et al., 2009). Single interferogram (D-InSAR) and multi-interferogram (A-DinSAR, Advanced D-InSAR) approaches become increasingly exploited for detecting and mapping different types of geohazards, such as subsidence, landslides, volcanic, and tectonic activities (Tomás and Li, 2017).
This paper reviews single and multi-interferogram data exploitation for subsidence monitoring along the Italian Peninsula. An overview of the applications proposed by several authors in the last 20 years will be presented, focusing on how the monitoring and mapping techniques of subsidence evolved with the advancements of the InSAR techniques.
A Brief Overview on Radar Interferometry
Synthetic Aperture Radar (SAR) systems measure distance variations between ground targets and the antenna of the sensor by repeatedly emitting a radar pulse along the orbit of the satellite and by measuring the echoes received from each emitted signal (Zhou et al., 2009). The return echoes received by the sensor contain two quantities: phase and amplitude. The first, fundamental for estimating ground motions, records the travel of the signal from its emission to its return and it is measured as a 2π function (Zhou et al., 2009). Amplitude represents the backscattering capability of ground surface, i.e., how much of the emitted signal can be returned to the sensing antenna. The higher the backscatter, the larger the amplitude value in the SAR image, resulting in a “bright” pixel.
The Differential Interferometric Synthetic Aperture Radar (D-InSAR) technique exploits the phase difference, computed as an interferogram, between two coregistered SAR images acquired above the same area at different times (Massonnet and Feigl, 1998). Ideally, each interferogram should contain only the phase difference due to ground displacement. Actually, phase differences can be generated by external factors not related to ground motion. Thus, phase is a complex signal that must be cleaned up from not-deformation-related components. Usually, phase is expressed as the sum of five main components (Hanssen, 2001): flat earth component (range distance difference due to surface displacement assuming a flat surface), topographic phase, displacement phase (phase difference due to ground motion), atmospheric phase, and noise component. The final goal of an interferometric approach is to correctly estimate the displacement phase by totally removing or limiting as much as possible the other components. Moreover, considering the wrapped nature of the phase signal (2π function), an unwrapping procedure must be applied to correctly reconstruct ground deformation. Considering this, the major limitations for D-InSAR are: temporal and geometrical decorrelation that enhance the noise component (Hanssen, 2001), phase unwrapping errors (Ghiglia and Pritt, 1998) and incorrect atmospheric phase estimation (Zebker et al., 1997).
To overcome these limitations, MTInSAR (Multi Temporal InSAR)techniques have been developed to systematically process stacks of differential interferograms with a common master image (Zhou et al., 2009). These techniques, by using a stack of redundant interferograms, allow a proper estimation of all the noise components, so better deriving the real displacement component. A MTInSAR analysis of a SAR data stack produces a certain number of measurement points, characterized by estimated deformation velocity values and by time series of deformation, the latter extremely useful to highlight acceleration or slowdown of ground motion.
In the last decade, many different interferometric algorithms have been developed, starting from the PSInSAR (Permanent Scatterers Interferometry) approach designed by a spin-off company of the Politecnico of Milano (Ferretti et al., 2001). In the following years, several different MTInSAR techniques were then proposed, such as the Small Baseline Subset (SBAS – Berardino et al., 2002), the Coherent Pixel Technique (CPT – Mora et al., 2003) or the Interferometric Point Target Analysis (IPTA – Werner et al., 2003). In the light of these examples, many other research groups focused their efforts for realizing new specific PSI techniques. For a wider review on PSI algorithms we refer to (Crosetto et al., 2016).
PSI products guarantee both wide area coverage, for example a single Sentinel-1 frame is 250 by 250 km, and high measurement points density suitable for detail/building scale analyses. Depending on the working scale, a PSI technique can produce thousands to millions measurement points with millimeter precision on the estimation of the single ground deformation measurement and on the average displacement rates (Ferretti et al., 2011b). At present PSI product can reconstruct the “history” of a moving point back to June 1992 (first acquisition of ERS-1 sensor), providing a unique opportunity for back-monitoring phenomena (Casagli et al., 2017; Del Soldato et al., 2018). Another important feature of PSI products is the capability of providing useful information for environmental, security and civil protection authorities regarding the presence of moving areas on which to focus ground-based observations and logistic operations, reducing costs, and personnel time (Solari et al., 2018).
Subsidence in Italy
Italy is affected by different geohazards, such as landslides, subsidence, earthquakes, and volcanic activities. Of these, subsidence usually does not directly produce casualties but triggers long-term economic losses and water resources depletion. It is a well-known phenomenon in coastal and agricultural areas, where a combination of ground water overexploitation, natural consolidation of sediments and rapid urbanization cause ground lowering at different scales. Below we present a brief overview on the main subsidence areas along the Italian peninsula will be presented, focusing on those of particular interest for their impact on people, economy and environment.
The Po Delta and the Po Plain are the most studied subsidence areas in Italy because of the presence of large cities (with important cultural heritages), industrial activities, and intensive farming. The Po Plain hosts a sedimentary Quaternary sequence with maximum thickness of 2,000 m, composed of normal-consolidated sand and clay layers (Dondi et al., 1985). As reported by Carminati and Martinelli (2002) the maximum subsidence occurs where long-term geological phenomena and intense water withdrawal are found. On the other hand, low subsidence rates coincide with the top of buried active anticlines, where the thickness of the sedimentary succession is lower. The natural subsidence of the Po Plain, estimated in few mm/yr (Baldi et al., 2009), is related to tectonic and sediment loading, sediment compaction, and post-glacial rebound (Carminati and Martinelli, 2002). The anthropogenic subsidence of the Po Delta Plain, especially along the Adriatic coast, began after the Second World War reconstruction, during the economic boom of Italy, when the multi-aquifer system of the Plain began to be extensively exploited for agricultural, and industrial use (Teatini et al., 2006). A second and subordinate anthropic cause is the onshore and offshore gas production (Gambolati et al., 1991).
The Venice lagoon, North-East of the Po Delta, is another example of long-term natural subsidence accelerated by anthropogenic factors. The city of Venice, at the center of the lagoon, is the emblem of how long-term subsidence interacts with an urban area. Although the magnitude of the motion, in the last 20 years, is extremely low (less than 2 mm/yr as reported by Tosi et al., 2002), the effect of this long-term deformation is irrecoverable and compromised the safety of the city in relation to the sea level rise (Bonardi et al., 1999). The human activity accelerated the natural evolution of subsidence in the area, especially during the industrial boom of the fifties and the sixties (Tosi et al., 2002).
Tuscany is another Italian region affected by subsidence along coastal areas and inland plains. Subsidence in Tuscany is mainly related to human activities, being water overexploitation, geothermal production and recent urban development (Rosi et al., 2016). Three areas are well-known for ground lowering problems: the Lower (LV) and Medium Valdarno (MV) plains and the Larderello geothermal district. The LV is an alluvial plain composed of alluvial and marine deposits, sometimes with the presence of highly compressible organic layers (Zanchetta et al., 1998). The MV plain is a lacustrine basin formed by a succession of alluvial and lacustrine sand, clay and silt with a maximum thickness reaching up to 100 m (Briganti et al., 2003). Both areas host important cities from the industrial and cultural point of view (Florence, Pisa, Prato and Pistoia). Dating back to 1905, the Larderello geothermal activity district is the first worldwide example of geothermal fluids extraction for electricity production. Thirty-four power plants are operating in the area, producing a high environmental impact and triggering subsidence phenomena (Manzella et al., 2018).
In the Campania Region subsidence phenomena, mainly related to water overexploitation and to volcanic/hydrothermal activity, are found (Vilardo et al., 2009). The Campi Flegrei caldera is a unique example of volcanic-related subsidence with unrest cycles characterized by intense ground uplift (D’Auria et al., 2015). The dynamics of the caldera system are a challenging issue not only by a scientific point of view but also for the potential risk induced by the ground motions in a densely populated area (Walter et al., 2014). One of the last episodes of major ground uplift, between 1982 and 1984, was accompanied by more than 16000 earthquakes and led to the evacuation of around 40000 people during the emergency (Barberi et al., 1984). The Sele and Volturno coastal plains are affected by severe subsidence due to water overexploitation for agricultural use that induce coastal inundation and thus a future increase of marine and fluvial flooding (Pappone et al., 2012; Aucelli et al., 2017). Another well-known subsidence area is the city of Sarno where the intense water pumping since the forties triggered ground lowering that seriously damaged several buildings (Cascini et al., 2006).
Rome (Lazio Region) registers several localized subsidence phenomena related to the long-term urban variations of the city that rises on the Tiber riverbanks. The original hydrographic network connected to the Tiber has been obliterated along the centuries with entire districts placed on top of buried lateral valleys filled by extremely heterogeneous deposits made by alluvial sediments and anthropogenic debris (Campolunghi et al., 2007). As a result of this chaotic stratigraphical assets, entire building block built after the fifties, along these tributaries valleys, register tilting, and differential settlements quantified in several millimeters (Arangio et al., 2014). Along the coast, in the Tiber Delta area, severe subsidence has been recorded in correspondence of two reclaimed coastal ponds (“Maccarese” and “Ostia”). In these areas ground lowering results as the combination between long-term consolidation of thick peat and organic clay layers and urbanization in the last 40 years (Del Ventisette et al., 2015).
For the whole Italian territory, a database, developed and managed by ISPRA (Istituto Superiore per la Protezione e la Ricerca Ambientale – Institute for Enviromental Protection and Research), reporting all the municipalities affected by land subsidence is available online1. This database, dated back to 2016, has been built up starting from information provided by regional authorities, provinces and municipalities, environmental agencies and other research centers. The results of the investigation made by ISPRA are shown in Figure 1.
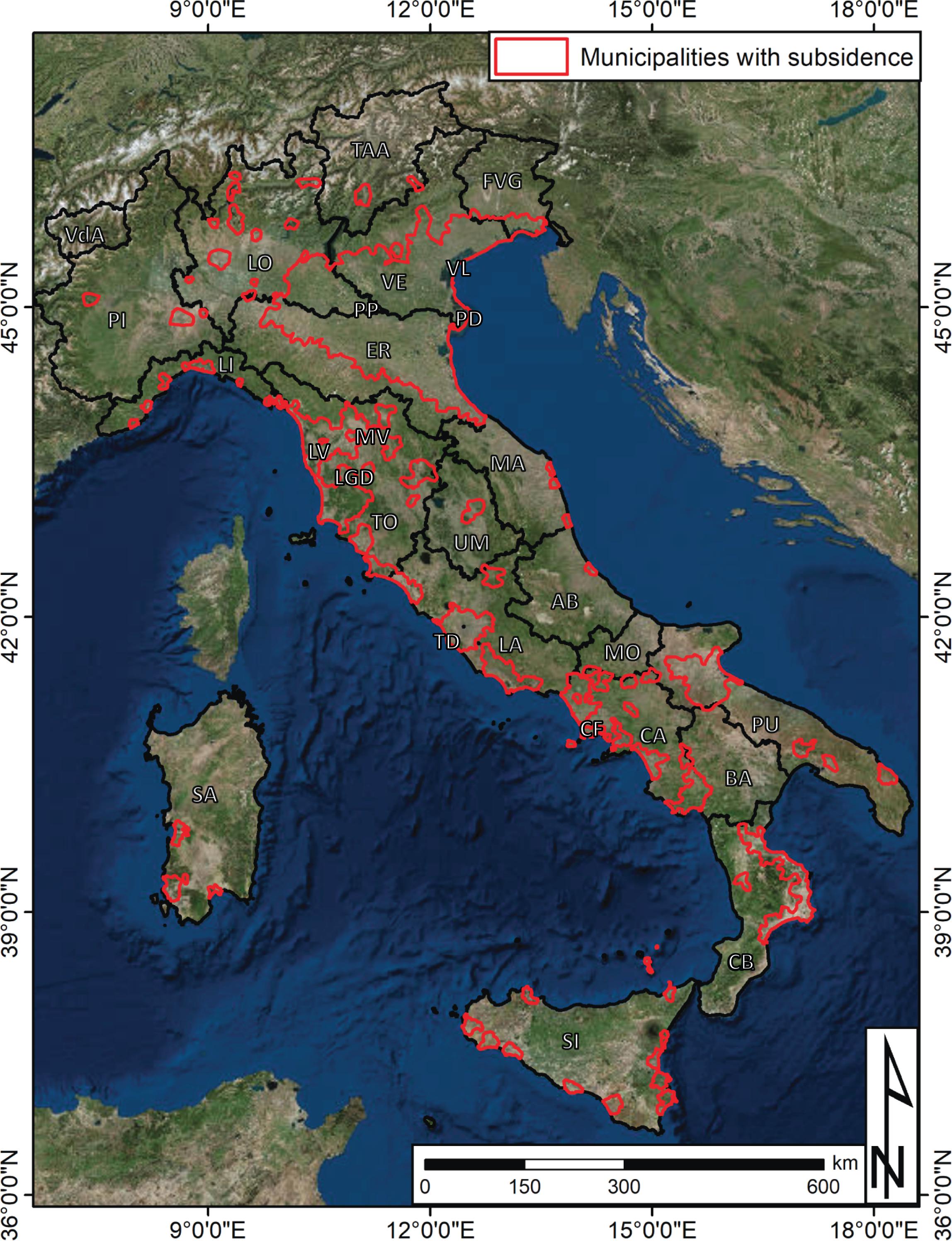
FIGURE 1. Municipalities affected by land subsidence in Italy derived from the ISPRA database. The acronyms in the map are referred to the Italian Regions and to geographical references used in the text. AB, Abruzzo; BA, Basilicata; CA, Campania; CB, Calabria; CF, Campi Flegrei; ER, Emilia Romagna; FVG, Friuli Venezia Giulia; LA, Lazio; LGD, Larderello geothermal district; LI, Liguria; LO, Lombardia; LV, Lower Valdarno; MO, Molise; MV, Medium Valdarno; PD, Po Delta; PI, Piemonte; PP, Po Plain; PU, Puglia; SA, Sardegna; SI, Sicilia; TAA, Trentino Alto Adige; TD, Tiber Delta; TO, Toscana; UM, Umbria; VdA, Valle d’Aosta; VL, Venice lagoon. The subsidence layer is overlaid on a Bing Aerial digital orthophoto.
The regions with the highest number of municipalities affected by subsidence are Veneto (52% of the total), Emilia Romagna (50% of the total), and Tuscany (28% of the total). On the other hand, Valle d’Aosta, Molise and Basilicata recorded no municipalities affected by subsidence. Less than 5% of the municipalities of 6 regions (Abruzzo, Marche, Umbria, Liguria, Sardegna, and Trentino Alto Adige) reported ground lowering problems. These numbers are estimates dependent on the quality and number of information collected and reported by the entities involved. Considering the total surface of the municipalities affected by subsidence, the 19% of the Italian territory is characterized by ground lowering phenomena; the 9% considering only Emilia Romagna and Veneto regions. This information is strongly qualitative; surface affected by subsidence is, in reality, significantly smaller. The percentage showed here are in fact referred to the total surface covered by the municipalities with subsidence and not to the real development of the phenomena that, without local and detailed analysis, cannot be derived.
InSAR Analyses for Subsidence Monitoring in Italy
A total of 109 scientific papers have been analyzed for this review (Figure 2). Three selection criteria were adopted: (i) the manuscript must be written in English and published in national or international peer-reviewed journal or in international conference proceedings; (ii) InSAR data have to be the core of the methodologies and results presented; (iii) all the D-InSAR and A-DInSAR methodologies and all the SAR satellite were considered.
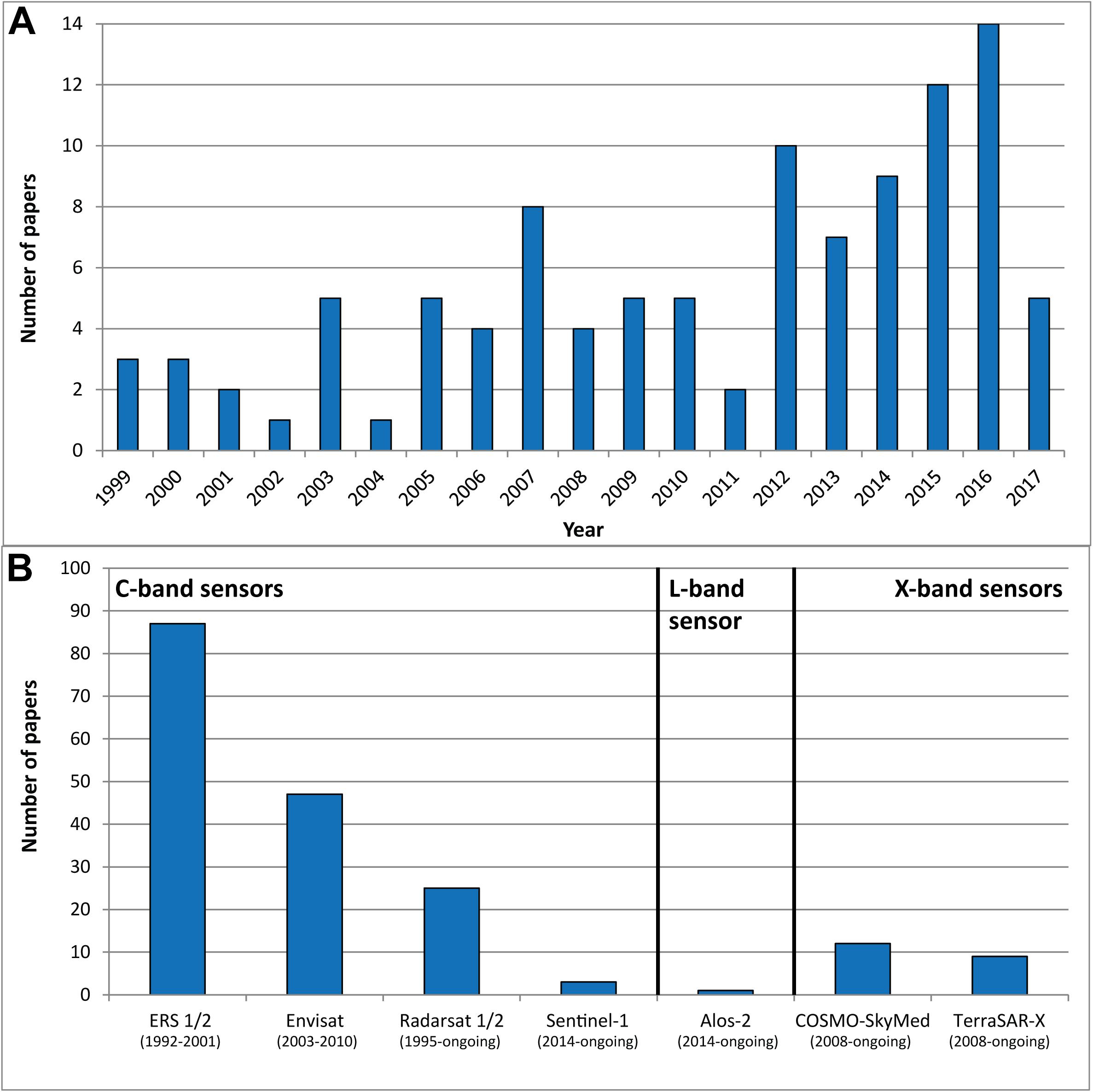
FIGURE 2. (A) Number of published scientific papers regarding interferometric techniques for subsidence monitoring since 1999. (B) Satellite exploited for subsidence monitoring in Italy. Multiple satellites can be used in the same scientific paper. The dates below satellite names are referred to their operational time-span.
The first applications of interferometric data for subsidence detection in urban areas go back to 1999 when, for the first time, the potentialities of InSAR data were assessed. In particular, Wegmuller et al. (1999) produced three long-time interferograms (period 1992–1996) using ERS data in the city of Bologna (Emilia Romagna region), showing the agreement between InSAR-derived information and leveling surveys. The obtained results were confirmed by Costantini et al. (2000) who applied an unwrapping approach based on sparse data networks. Avallone et al. (1999) presented the first InSAR result for the Campi Flegrei deformation during an inflation sequence, displaying the relationship between InSAR results, leveling and source modeling. Lundgren et al. (2001) confirmed the outcomes of Avallone et al. (1999) by using shorter time spans interferograms, combined into time series of deformation. Tosi et al. (2002) exploited D-InSAR data to verify and demonstrate the stability of the Venice historical city center. This study compared tide gage, leveling, and D-InSAR measurements to point out that the city of Venice, after the huge subsidence acceleration due to industrial exploitation of ground water in the sixties and seventies, registers extremely low deformation rates (below 2 mm/yr) related only to the natural evolution of the lagoon.
These few examples already shown the capability of InSAR techniques to create multi-temporal maps of deformation over wide areas with precision that can be compared to leveling data. Figure 3 presents a map showing the mean velocity values for all the cities and subsidence areas in Italy. This information has been derived from the analysis of all the scientific papers selected for the aims of this review.
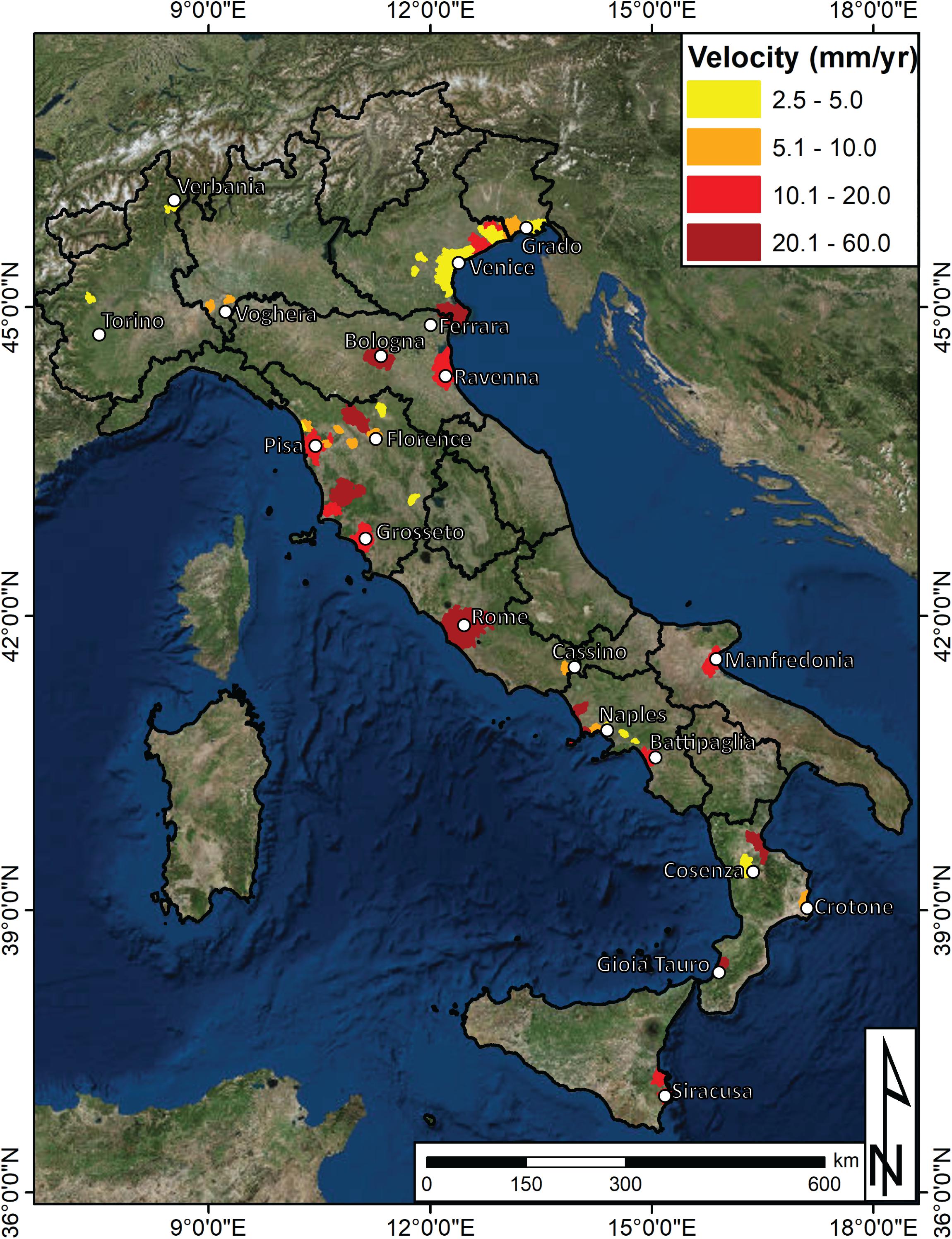
FIGURE 3. Subsidence areas in Italy monitored by means of D-InSAR or PSI techniques in the last 20 years. Local or building-scale velocity outliers are not considered. The velocity map is overlaid on a Bing Aerial digital orthophoto.
The technological evolution of the interferometric approach that led to the development of PSI techniques granted the opening of new scenarios for subsidence satellite monitoring. In fact, the availability of point-like data, connected to time series of displacement, allowed researchers to derive not only ground velocity maps above large areas but also to reconstruct the behavior of single buildings. An effect of the arrival of these new techniques can be seen in the number of scientific papers related to satellite subsidence monitoring in the last 6 years (Figure 2A). This is mainly due to (i) scientific acknowledgment of the InSAR data as reliable monitoring tools, (ii) increase in the number of research groups that work with InSAR-derived products, (iii) release of non-commercial software tools (e.g., StaMPS/MTI – Hooper, 2008) or web platforms (Geohazard Exploitation Platform, GEP – Galve et al., 2017) for PSI processing. Figure 2B shows the distribution of different satellite data within the stack of reviewed papers. The highest number of authors exploited ERS 1/2 or Envisat data (Figure 2B). On one side, this is due to a simple temporal matter; on the other side, some researchers exploited these satellite data thanks to the PST-A Project (Special Plan of Remote Sensing of the Environment) that allowed everyone to have access to already processed PSI product for the whole Italian territory (Costantini et al., 2017).
Applications of PSI approaches for subsidence monitoring in Italy date back to 2003. Colombo et al. (2003) presented the first application of the PSInSAR (Permanent Scatterers Interferometry) algorithm for urban subsidence monitoring in the Medium Valdarno (Firenze-Prato-Pistoia basin). Strozzi et al. (2003) analyzed ground deformation in the Venice Lagoon by means of the IPTA (Interferometric Point Target Analysis) algorithm, integrating the results obtained with a preexistent GPS network.
In the following sections, the main outcomes obtained by the research community using PSI data for subsidence monitoring will be presented. The sections are subdivided depending on the specific use of the PSI datasets and on subsidence driving force. For some selected and representative example, PSI data, derived from the PST-A project (Costantini et al., 2017) will be presented. These open data are used to better present to the reader the subsidence areas; so are not intended as a comparison with the results obtained by the different authors.
Subsidence Due to Consolidation Processes
PSI data, being able to extract reliable information from urbanized areas, are ideally suited for monitoring land subsidence due to the natural consolidation of sedimentary sequences, processes sometimes accelerated by anthropogenic factors, such as load imposition or land reclamation. This Section will illustrate Italian case studies affected by these type of phenomena, not considering subsidence due to water overexploitation that will be presented separately in “Subsidence Due to Groundwater Extraction”.
Rome, due to a subsurface heterogeneity generated by its millenary history, is strongly affected by local subsidence phenomena related to soil consolidation, producing differential settlements, and tilting that damage buildings and infrastructures. Manunta et al. (2008), using the SBAS approach, and Stramondo et al. (2008), exploiting the IPTA algorithm, performed similar and consistent interferometric analysis over the city center of Rome. Both authors verified the presence of subsidence trends over 10 mm/yr along the tributaries valleys of the Tiber river, nowadays filled by heterogeneous materials. Stramondo et al. (2008) compared the time series of deformation obtained in some areas of the city with the classical consolidation curve theorized by Terzaghi and Peck (1967). They concluded that high subsidence rates (above 15 mm/yr) are recorded in recent urbanization (not older than 15 years); these motions are related to primary consolidation that quickly slow-down in few years. If highly compressible or organic layers are present, this process can last longer, up to 50 years (as shown by the authors for the Grotta Perfetta case study). The results obtained were confirmed by a recent work presented by Scifoni et al. (2016), who also locally estimated differential settlement, comparing PSI results with geotechnical and hydrogeological information, for selected buildings of considerable cultural value (Palazzo Braschi, Palazzo Altieri and Palazzo Doria-Pamphili).
Comerci et al. (2015), Del Ventisette et al. (2015), and Raspini et al. (2016) measured ground deformation in the Tiber River Delta, 30 km western than Rome. The authors detected high deformation rates (up to 25 mm/yr) along the runways of the Leonardo Da Vinci International Airport and in the inland of the city of Ostia, located along the coast at the Tiber river mouth. These subsiding areas coincide with the perimeter of two reclaimed ponds in which highly compressible organic clay layers are found. Del Ventisette et al. (2015) exploited the PSI data as a proxy for mapping the spatial distribution of the organic clay layers and correlated the registered velocities with the thickness of the compressible sequence.
PSI data allowed scientists to obtain new information about the potential instability of Venice, the Venice Lagoon and the general evolution of this sector of the Adriatic coast. (Tosi et al., 2010) presented a review about the results obtained by combining satellite and GPS data for subsidence monitoring along the Adriatic coast, between the Po Delta and the Tagliamento river mouth (100 km northeastern than Venice). Tosi et al., 2009 proposed an interesting explanation for the subsidence rates recorded within the Venice Lagoon and outside of it, between the cities of Rovigo (80 km southwestern than Venice) and Treviso (40 km northeastern than Venice). Three main components of the motion, measured by means of PSI analysis, where defined: (i) deep component related to neotectonics fault systems, responsible for land uplift (around 2 mm/yr) in the plain between the Euganean Hills and the lagoon; (ii) medium depth component related to residual consolidation processes in the lagoon or to water overexploitation; (iii) shallow component that trigger the highest subsidence rates (up to 10 mm/yr) and that is related to peat oxidation and salinization processes in marshy and reclamation areas or to recent urbanization. One important outcome of these work is the definition of the city of Venice as “stable”, considering the extremely low deformation rates registered (lower than 2 mm/yr). Teatini et al. (2011) measured subsidence rated of the whole Po Delta by means of IPTA interferometry, discovering that the “recent compressible low-permeable deposits in the Po Delta is far from complete”. They estimated that, considering the current deformation rates in the youngest portion of the delta (last three centuries), high subsidence rates will continue to be observed. This is an extremely important information for land use planning and management, considering also that some sectors of the delta are already few meters below the sea level.
Recently, Tosi et al. (2016) and Da Lio et al. (2018) focused their attention on the estimation of subsidence rates not only in urban areas but also in the salt marsh that characterize this lagoonal environment. Tosi et al. (2016) combined the capabilities offered by high resolution X-band data (COSMO-SkyMed) with L-band ALOS-2 images that maximize the density of measurement points in vegetated areas. On the other hand, Da Lio et al. (2018) jointed high resolution TerraSAR-X data with a network of corner reflectors that helped in estimating subsidence rates in marshy areas. Da Lio and Tosi (2018) derived similar conclusion and deformation patterns for the Grado-Marano Lagoon, highlighting also a strong NE-SW regional subsidence gradient related to the depth of the bedrock and thus of the Holocene compressible sequence.
Another example of land subsidence due to soil consolidation, accelerated by recent urbanization, can be found in the Tuscany Region. (Solari et al., 2016a,b, 2017) characterized the temporal evolution of ground lowering in the urban area of Pisa at building-scale. The authors collected PSI results from 1992 to 2014, creating a 20 years-long time series of deformation for selected sectors of the city that registered average subsidence rates higher than 5 mm/yr, with maximum values of 35 mm/yr. The authors performed an integrated analysis between subsurface geotechnical data and PSI velocity to estimate the role of urbanization in determining the high deformation rates measured. They discovered that, excepting the historical city center that is almost stable, the outer sector of the city had a natural subsidence due to consolidation with rates between 2.5 and 5 mm/yr. All the velocity outliers were related to the urban evolution of the city, using an approach similar to the one presented by Stramondo et al. (2008) for the city of Rome.
The availability of Envisat PSI products (2003–2010) is shown in Figure 4 for the three aforementioned areas: Rome and the Tiber Delta (Figure 4A), Pisa (Figure 4B) and the Venice Lagoon (Figure 4C).
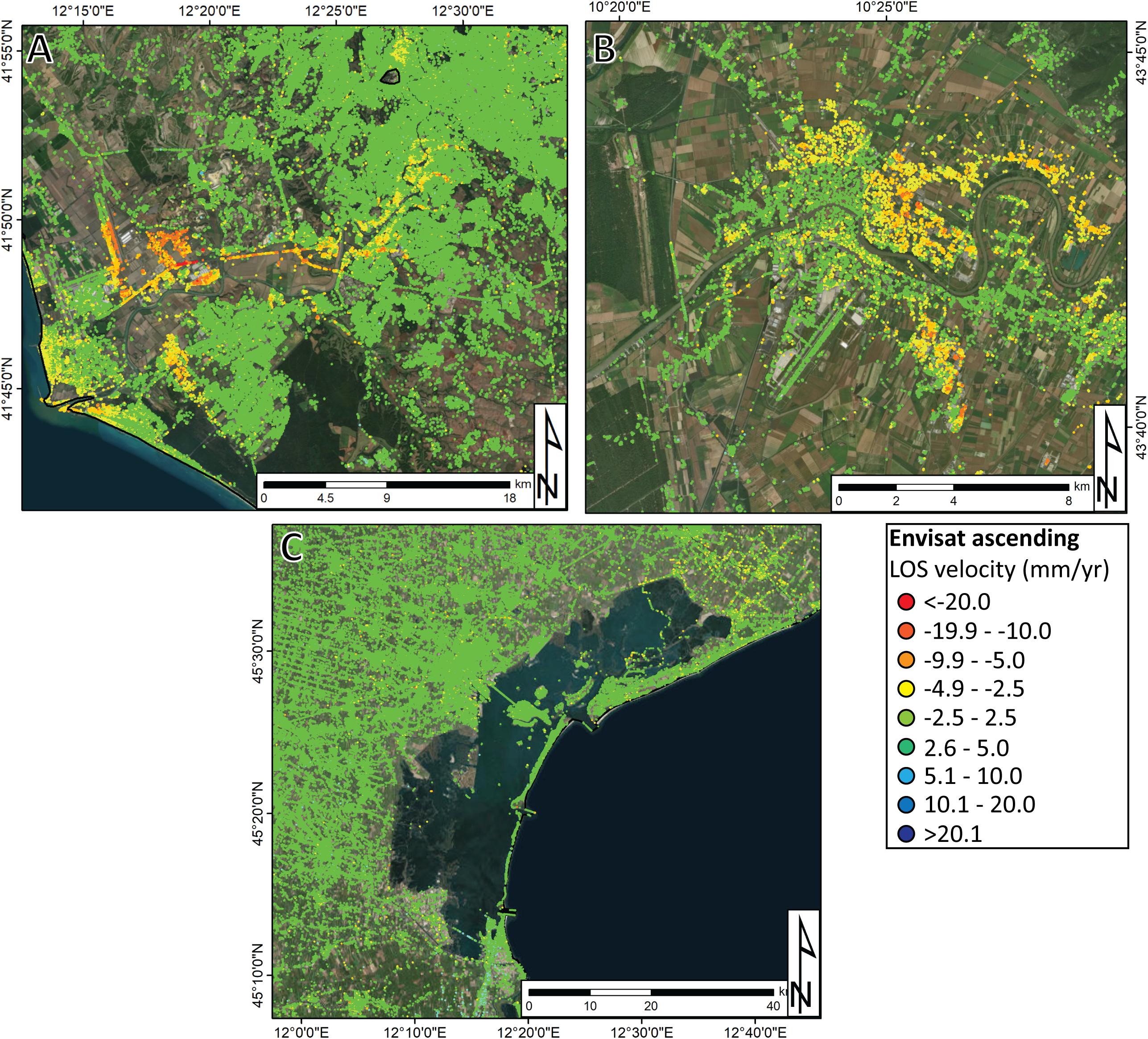
FIGURE 4. Examples of land subsidence due to soil consolidation along the Italian Peninsula. (A) Rome and the Tiber Delta; (B) Pisa; (C) the Venice Lagoon. The Envisat PSI dataset are part of the PST-A project (Costantini et al., 2017). The deformation maps are overlaid on a Bing Aerial digital orthophoto.
Subsidence Due to Groundwater Extraction
Ground lowering related to water overexploitation is quite common in Italy, especially along the coast and in agricultural/industrial areas. Thus, PSI data have been widely used to investigate the temporal evolution of ground subsidence triggered by intense water extraction. Several areas along the Italian peninsula have been characterized using PSI data cross-compared with groundwater level information.
In the Tuscany Region, Canuti et al. (2005) applied the PSInSAR algorithm to ERS radar images for detecting ground lowering in the Firenze-Prato-Pistoia plain. The recorded subsidence rates (up to 30 mm/yr) were in accordance with the exploitation of the water resources for industrial, agricultural and forestry plantation (mainly in the city of Prato and Pistoia). Lu et al. (2010) updated the results obtained by Canuti et al. (2005) using a new method named as PSI-HSR (PSI Hue–Saturation Representation) that represented PSI-derived velocity values with a different color (Hue-Saturation-Intensity, HSI, scheme) depending on the displacement rate and on the moving direction recorded. The authors highlighted that, in the monitored period (2003–2006), subsidence in Prato strongly decelerated, even registering ground uplift in some sectors of the city. This fact was related to a gradual termination of water extraction activities in early 2000, thus not registered with the ERS 1/2-derived results presented by Canuti et al. (2005).
Stramondo et al. (2007) performed a PSI analysis over an ERS image stack to retrieve ground deformation in the city of Bologna in a 9 years-long time period (1992–1999). Subsidence in this part of the Emilia Romagna Region is well known and mainly related to a long-term natural effect (tectonics, sedimentary loading and post glacial rebound) strongly accelerated by water overexploitation since the fifties. The authors presented one of the first application of the SBAS algorithm for urban monitoring; the results obtained were compared with leveling data and included in the geological and hydrogeological setting of this portion of the Po Plain.
In the Campania Region, Cascini et al. (2006, 2007) investigated subsidence-related building damage with SBAS interferometric data, correlating piezometric level decrease, ground lowering, and spatial distribution of fractures in the urban area of Sarno. In the same region, Aucelli et al. (2017) and Matano et al. (2018) assessed subsidence rates (up to 25 mm/yr) in the Volturno coastal plain, characterized by strong farming activities that cover the 80% of the territory. In addition, Aucelli et al. (2017) predicted long-term effects of the present subsidence by forecasting the future topography (on the basis of the current vertical deformation rates). This prediction has been compared with sea level rise models, using the data to build up multi-temporal coastal inundation hazard maps. This interesting approach has been applied by Di Paola et al. (2017) in the case of the Sele coastal plain.
Another example comes from the Calabria Region, where Righini et al. (2011) and Raspini et al. (2012) exploited ERS and Envisat archives to investigate the temporal and spatial evolution of pumping-induced subsidence in the Gioia Tauro plain. Since the seventies, several industrial activities and intensive farming (3% of the Mediterranean production of citrus) developed, leading to intense water withdrawal that triggered the current land subsidence (maximum subsidence rates of 23 mm/yr). In the same region, Bianchini and Moretti (2015) and Cianflone et al. (2015) presented similar results for the Sibari plain, where subsidence triggered by both natural (Holocene tectonic, only accounted by Cianflone et al. (2015), and consolidation processes) and anthropogenic factors (ground water extraction and urbanization) is present.
Canova et al. (2012) detected land subsidence along the Ionian coast of the Sicily Region, specifically in the city of Augusta and Siracusa. In this area, a confined aquifer is strongly depleted (maximum difference with the original static level of 100 m) for urban use and industrial supply (average pumping of 13000 m3/day), triggering ground lowering phenomena (up to 18 mm/yr as measured by means of PSI analysis). The authors reported that, where Holocene deposits with poor geotechnical characteristics are present, recent urbanization (after the eighties) has to be taken into account to explain the subsidence rates recorded.
In the Lazio Region, Bozzano et al. (2015) combined geological and hydrogeological modeling with PSI processing to evaluate the effects of ground water exploitation in the Acque Albule plain, in the hinterland of Rome. In this area, because of travertine quarrying activities, intense water pumping take place with an estimated flow rate of 1 m3/s in the seventies, producing a maximum decrease of the piezometric level up to 30 m. By comparing PSI-derived subsidence rates, that reached 15–20 mm/yr, with subsurface reconstructions the authors were able to assess that the groundwater level variations drive the timing of subsidence and the geological conditions the magnitude of ground lowering.
Bonì et al. (2016) detected and characterized land subsidence in the Oltrepò Pavese area (Piemonte Region), where groundwater extraction accelerated a natural consolidation process due to swelling/shrinkage of clayey soils, reported for the first time by Meisina et al. (2006). Moreover, the authors proposed a novel methodology to post-process PSI data by analyzing the principal components of the time series of deformation to derive over time the deformation pattern and kinematic model for each radar target.
Figure 5 shows four example of deformation maps extracted from Envisat radar images in areas affected by land subsidence induced by ground water extraction.
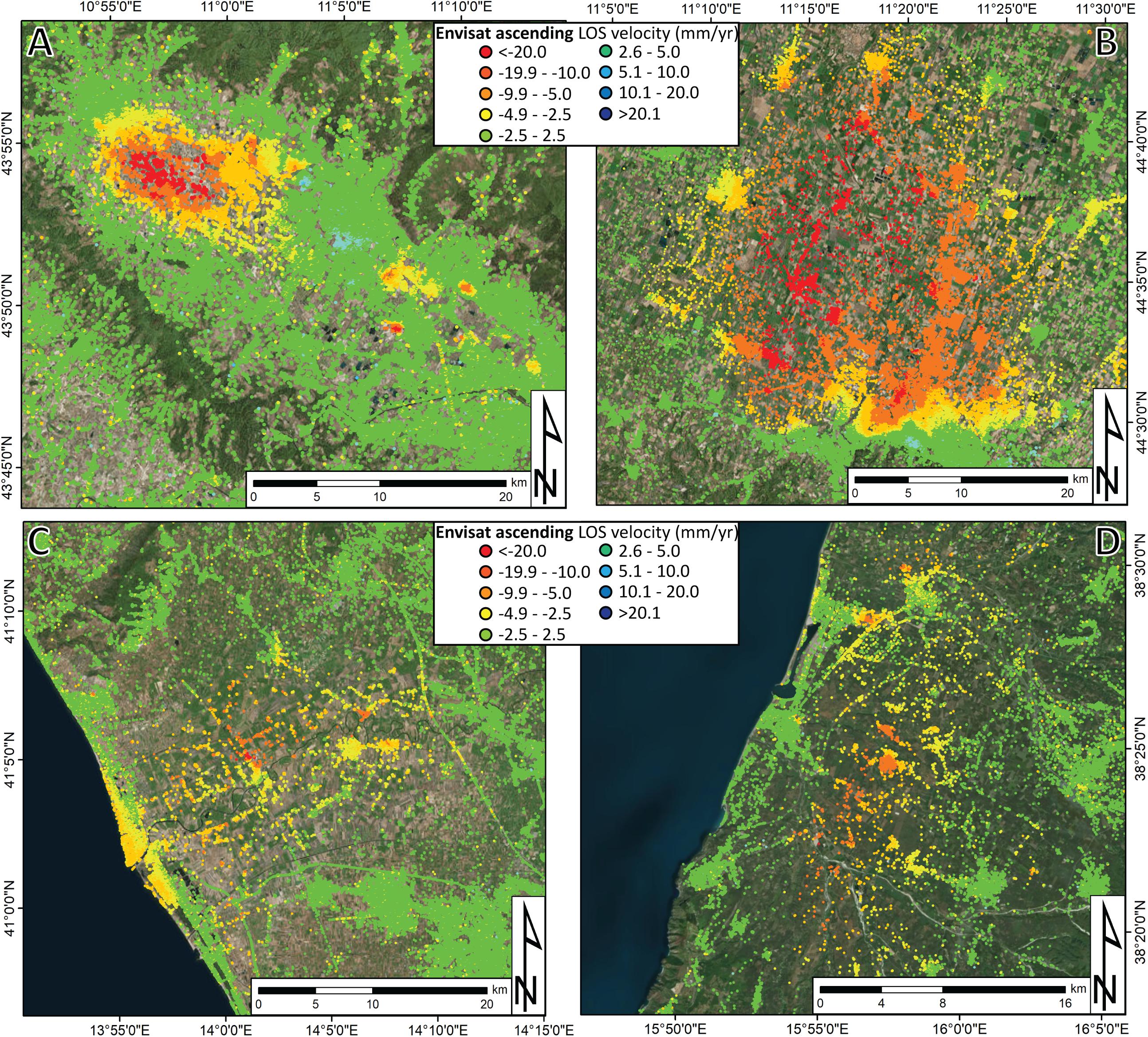
FIGURE 5. Examples of water overexploitation effects monitoring in Italy. (A) Firenze-Prato-Pistoia plain; (B) city of Bologna; (C) Volturno plain; (D) Gioia Tauro plain. The Envisat PSI dataset are part of the PST-A project (Costantini et al., 2017). The deformation maps are overlaid on a Bing Aerial digital orthophoto.
Campi Flegrei Deformation
Because of its peculiar characteristics and triggering cause, the subsidence/uplift of the Campi Flegrei caldera deserves a section alone to be debated.
The location of the Campi Flegrei caldera, within a densely populated urban area, offers an ideally suited scenario for PSI analyses that allowed several scientists to study the temporal evolution of this volcanic system.
Since 1985 the Campi Flegrei caldera has undergone a long deflation/subsidence period interrupted in early 2000 by a strong inflation episode related to an unrest of the volcanic system (Lanari et al., 2004). Several authors deeply characterize the dynamics of the caldera system thanks to the availability of PSI data derived from different radar sensors (i.e., Trasatti et al., 2008; Ventura et al., 2009; Vilardo et al., 2010; Samsonov et al., 2014; Walter et al., 2014; D’Auria et al., 2015).
The PSI products gave information not only on the extension of the phenomenon but, mostly important, on its temporal evolution. Samsonov et al. (2014) analyzed ERS 1/2, Envisat and Radarsat-2 radar images to evaluate the evolution of the caldera for a period of 20 years. The authors highlighted that, after a subsidence phase with maximum velocities of 30 mm/yr located in the center of the caldera, the area registered a slow uplift rate until 2005 when a strong positive acceleration of the ground motion took place. At the end of their monitoring period (2011–2013), Samsonov et al. (2014) estimated uplift rates of about 50 mm/yr. The same temporal evolution of the PSI data over the caldera area has been verified by Walter et al. (2014) using ERS 1/2 and Envisat images, spanning between 1992 and 2008. An example of Envisat PSI products for the Campi Flegrei caldera is shown in Figure 6.
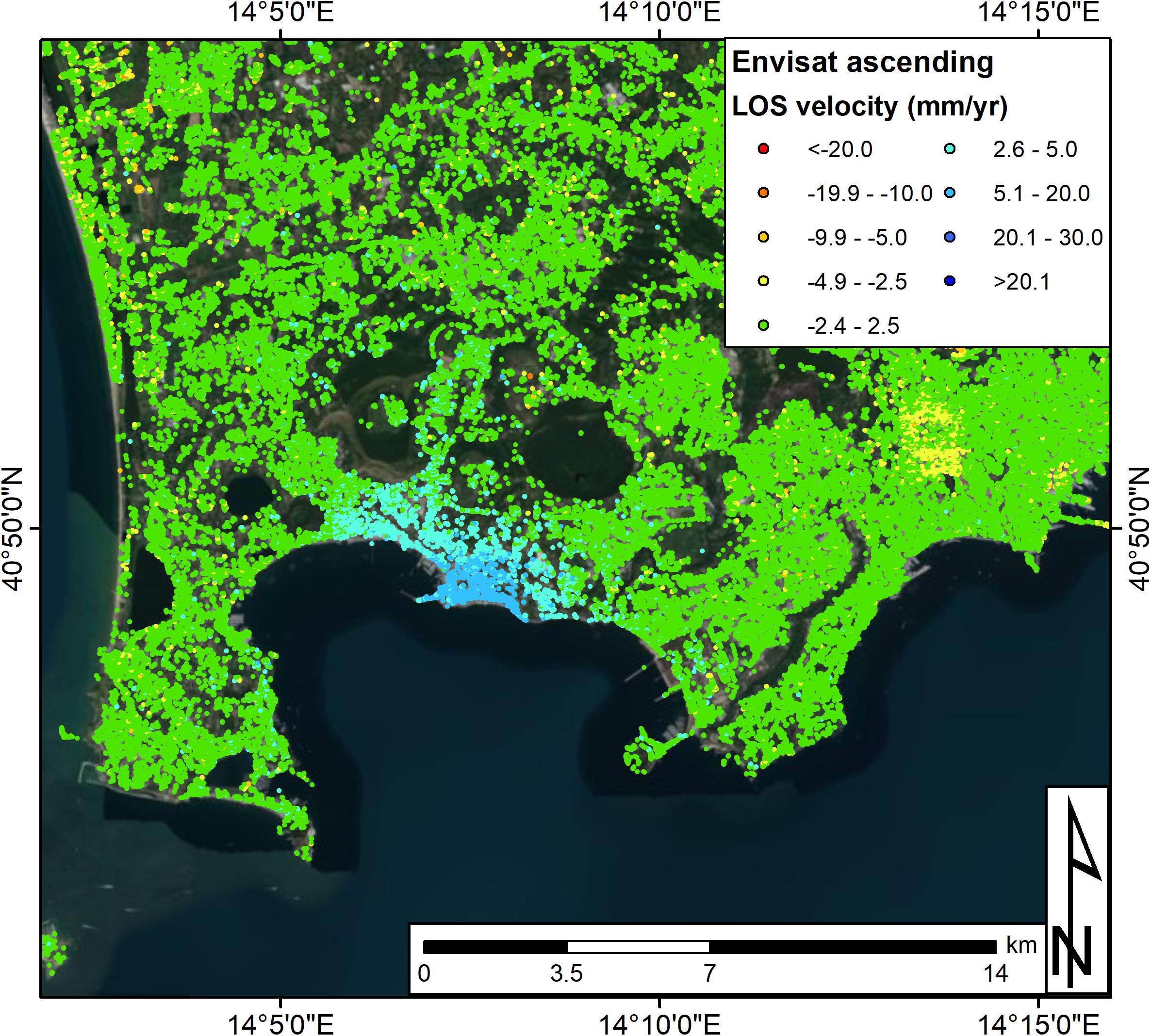
FIGURE 6. Deformation map for the Campi Flegrei caldera in the time span between 2003 and 2010. The Envisat PSI dataset is part of the PST-A project (Costantini et al., 2017). The deformation map is overlaid on a Bing Aerial digital orthophoto.
PSI data where used not only to derive the temporal evolution of the phenomenon but also as input data for modeling the deep source of the deformation using inversion approaches. For example, Tiampo et al. (2017) applied the Principal Component Analysis on ERS 1/2, Envisat and Radarsat-2 data, obtaining input data for inversion models. Two deformation sources were identified, the first and shallower located between 2750 and 3400 m below surface, the second at a depth between 7600 and 8000 m. D’Auria et al. (2015) presented, for the first time in the last 30 years, the evidence of a renewed magmatic activity at the Campi Flegrei Caldera. For this purpose, ground deformation measurements derived from PSI product and GPS data were integrated into a source model to demonstrate that the 2012–2013 inflation was the effect of the intrusion of magma within a sill at a depth of around 3000 m.
PSI Data for Buildings, Infrastructures and Cultural Heritages Investigations in Subsidence Areas
Interferometric data have been exploited for analyzing buildings and infrastructures affected by land subsidence, focusing on the evaluation of possible differential displacements that could compromise their stability. Nowadays, only a few comparisons between PSI products and structural damage have been published.
Two examples come from the city of Rome, where Arangio et al. (2014) and Cerchiello et al. (2017) quantitatively assessed the structural health of selected buildings in the southern part of Rome. Both authors integrated PSI results with a semi-empirical schematization of buildings (laminated beam model – Finno model, Finno et al., 2005) to evaluate the structural integrity of the analyzed edifices. The PSI data acted as input displacement values for the models, producing a damage classification for each, later compared with ground evidences.
Strozzi et al. (2009) evaluated, by means of X-band TerraSAR-X data, the possible effects triggered by the construction of mobile barriers at the three inlets of the Venice Lagoon. The authors evaluated that the construction of the barriers did not modify the deformation pattern of the lagoon, producing only high subsidence rates (up to 50 mm/yr) in correspondence of the new structures and in recently drained areas.
Pratesi et al. (2015, 2016) used a holistic approach to characterize single buildings affected by ground motions (mainly subsidence) in the city of Florence (Tuscany Region) by comparing PSI velocities, local stratigraphical asset, ground evidences and building structural features. The authors produced for each building a hazard rating calculated as a product between all the ancillary, ground and PSI information collected.
Monitoring cultural heritages in ground lowering areas by means of PSI technique is another application that challenged some authors. Cigna et al. (2014) derived an updated snapshot of the structural health of some heritages within the historic center of Rome exploiting high resolution COSMO-SkyMed radar images. The Palatine and Oppian Hills, Colosseum, Baths of Caracalla and the Aurelian Walls have been characterized in detail by means of interferometric analysis. Cigna et al. (2014) stated that satellite data highlighted the “overall stability of the main monuments and archeological sites of the historic center and the absence of clear deformation patterns at local-scale.” Tapete and Cigna (2012) and Cigna et al. (2016) performed an interferometric analysis over the archeological site of Capo Colonna in Calabria Region, located on top of a promontory affected by both rockfalls, due to marine erosion, and land subsidence, related to gas extraction in nearby wells. Cigna et al. (2016) exploited 40 artificial corner reflectors to increase the radar coherence of the area, obtaining a highest number of measurement points.
Geothermal Activity and Gas Extraction Monitoring
Geothermal resources are quite widely distributed in Italy, sometimes exploited for electricity production. The overexploitation of this renewable energy sometimes creates environmental problems and ground lowering. This is the case of the Larderello geothermal field in southern Tuscany. Rosi and Agostini (2013) and Botteghi et al. (2015) investigated the deformation measured between 1992 and 2000 in the area, relating the registered subsidence rates with the geothermal activity. Both authors, considering ERS and Envisat C-band data, registered concentric subsidence rates decreasing from a maximum of 35 mm/yr in correspondence of the deepest geothermal well (Sasso Pisano field) to a minimum of 5 mm/yr. The same dataset used by these authors is presented in Figure 7A.
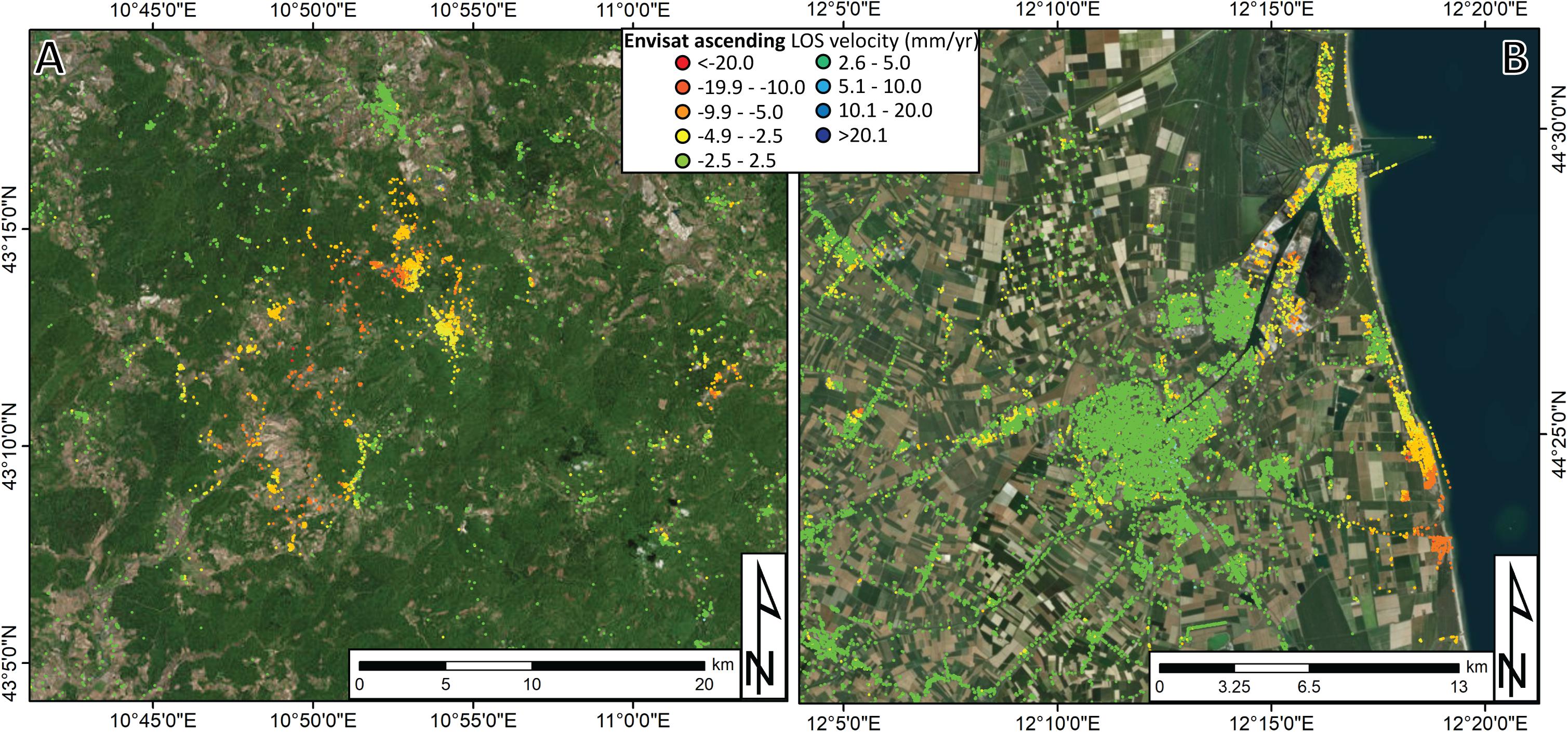
FIGURE 7. (A) Geothermal area of Larderello (Southern Tuscany). (B) Ravenna coast. The Envisat PSI dataset are part of the PST-A project Costantini et al. (2017). The deformation maps are overlaid on a Bing Aerial digital orthophoto.
Along the Adriatic coast, in the Ravenna area, offshore and onshore gas fields for methane extraction are present. Because of the high productivity of the fields and their proximity to the coastline, the gas exploitation produces reservoir compaction with maximum influence range of 6.5 km from the center of the gas field (Gambolati and Teatini, 1998). Fiaschi et al. (2017) reconstructed the last 25 years deformation scenario of the area, exploiting ERS, Envisat, TerraSAR-X and Sentinel-1 radar images. Maximum subsidence rates up to 35 mm/yr, registered in all the datasets, affect the coastline of Ravenna. The authors stated that the main driving force of the subsidence is the gas extraction from the offshore and inshore gas fields and considered that there is no evident relationship between detected velocities and water extraction. An example of Envisat PSI products for the Ravenna coast is shown in Figure 7B.
Regional/Wide Scale Applications
More recently, several authors have presented regional applications for subsidence mapping, exploiting the wide are coverage potential and great cost/benefits ratio of the PSI products.
Meisina et al. (2008) proposed a methodology to interpret PSI results at regional scale (Piemonte Region), considering different types of geohazards including subsidence. This approach is based on the definition of clusters of active motion PS automatically derived in a GIS environment. The authors provided also a list of guidelines to be followed for correctly interpreting the PSI results. The output of this work showed that the 14% of the moving areas (from a total of 2288) in the Piemonte Region could be related to land subsidence.
In the Campania Region, Vilardo et al. (2009) mapped the main moving areas in the region (considering hydrogeological, tectonic, and anthropic processes) exploiting ERS 1/2 data projected into the vertical and east-west planes. Cascini et al. (2013) developed a medium scale regional approach over the Campania plain (northern portion of the Campania Region) aimed at detecting facilities exposed to subsidence phenomena. This methodology relies on the use of PSI data in double geometry that are converted into a 100 by 100 m cell grid. Every cell has its own mean velocity value, weighted on the coherence values of the PS. Thus, a grid of moving and not moving cells is created. For all the moving cells the LOS velocities of the two orbits are converted into vertical/horizontal components. The final result is thus superimposed over a map of urbanized areas of the Campania plain, obtaining an evaluation of all the municipalities affected or potentially affected by ground subsidence. Peduto et al. (2015) presented an evolution, specifically designed to operate at different scales, of this approach. Considering the work flow proposed by Peduto et al. (2015), at regional scale only moving areas and vertical components maps are derivable; on the other hand, at detailed scale it is possible to derive building damage classification and multi-temporal displacement components profiles for single buildings.
Rosi et al. (2014) presented a procedure to map subsidence phenomena at regional scale, applying it to the Tuscany Region. The methodology is based on the calculation of the real displacement vector of the ground motion derived from the combination of the E-W and vertical components of the PSI velocities. Rosi et al. (2016) showed the results obtainable with this methodology. Within the Tuscany Region, the highest subsidence rates were detected in three areas: the Lower and Middle Valdarno and the Larderello geothermal district. The first two areas are related to water overexploitation or soil consolidation processes (see “Subsidence Due to Consolidation Processes” and “Subsidence Due to Groundwater Extraction”), whereas the third area registered ground lowering as an effect of geothermal activity (see “Geothermal Activity and Gas Extraction Monitoring”).
Bitelli et al. (2015) used Radarsat-2 data to map subsidence bowls in the Emilia Romagna Region, specifically along the Po plain. For this aim, SqueeSAR (Ferretti et al., 2011a) data have been calibrated and validated with GPS time series in order to create a vertical motion map for the period 2006–2011. The highest deformation rates were recorded in correspondence of the city of Bologna and along the Ravenna coast.
Raspini et al. (2018) designed an innovative monitoring system based on Sentinel-1 images for ground deformation monitoring at regional scale (Tuscany Region). The radar data are continuously analyzed (at every new acquisition of the sensor) with the combination of SqueeSAR and time series data mining algorithms. The authors presented the results obtained during 1 year of the activity of the system, showing how land subsidence in the city of Pistoia accelerated during the monitored period.
Final Remarks
Italy is one of the first European nations in which PSI data have been widely used for monitoring, mapping and potentially forecasting hydrogeological events. It is also the first worldwide example of a nation in which a systematic coverage of PSI products has been proposed (Costantini et al., 2017) and in which, for an entire region, a near real time monitoring service based on satellite interferometric data has been implemented and currently working (Raspini et al., 2018). Starting from early 2000, several authors exploited interferometric data for subsidence characterization along the Italian peninsula, producing more than 100 scientific papers.
The applications presented in this review illustrated the potential of PSI data for subsidence monitoring and mapping in urban and peri-urban areas. Firstly, the multi-scale capabilities of interferometric products allow working at different scales, from national or regional scale to single building. A representative example is the Tuscany region where Rosi et al. (2014) and Raspini et al. (2018) proposed small scale mapping and monitoring approaches for subsidence detection. Canuti et al. (2005) and Lu et al. (2010) characterized ground lowering at basin scale along the Firenze-Prato-Pistoia plain. Solari et al. (2016b, 2017) presented building scale analysis in the city of Pisa. Secondly, the multi-temporal capabilities of radar data permit reconstructing a time series of deformation for the areas of interest for a period of 25 years. An example here proposed is the Campi Flegrei caldera, in which it is possible to follow the recent evolution of the deformation starting from 1992, discovering the way in which the ground reacts to the subsurface modifications of this peculiar volcanic system (e.g., Samsonov et al., 2014; Walter et al., 2014). Finally, the availability of multi-band satellites allows scientists to perform different types of analyses depending on the sensor selected. This is the case of the Venice Lagoon where Tosi et al. (2016) and Da Lio et al. (2018) exploited X and L-band interferometric products to evaluate the current deformation in the non-urban portion of the lagoon.
PSI data represent a valuable support for urban planning activities. In fact, using local/building scale approaches (e.g., Stramondo et al., 2008; Solari et al., 2016b) it is possible to predict, assuming or knowing the stratigraphical asset, the possible deformation that will occur after the construction of a building. Moreover, PSI data can support structural evaluation of single buildings, as presented by Arangio et al. (2014) and Cerchiello et al. (2017).
As illustrated by several authors (see “Subsidence Due to Groundwater Extraction”), PSI products are ideally suited for monitoring activities in urban areas where water overexploitation-related subsidence occurs. This fact has important environmental implications; knowing the effects on the ground of the subsurface water extraction it is possible to better manage this activity, reducing the effects induced and the depletion of the resource.
Validation is an important aspect to be taken into account for a correct interpretation of the PSI products. Considering that subsidence has a main vertical component, GPS data confirmed the sensitivity, accuracy and precision of the interferometric products. Some examples have been proposed in this review, such as Tosi et al. (2010) for the Venice Lagoon or D’Auria et al. (2015) for the Campi Flegrei caldera.
In summary, this review illustrated the evolution of the interferometric application in Italy in the last 20 years, passing from local applications to regional scale approaches. Considering the limitations of the PSI techniques, the results obtained by the different authors confirm the reliability of the satellite interferometric data for mapping and monitoring subsidence, induced by natural and/or anthropogenic causes and in different environments.
Author Contributions
LS wrote the manuscript, designed its structure, and analyzed the data collected. MDS, SB, RM, and PE helped in collecting and analyzing the data. AC, FR, and SM critically revised the manuscript.
Conflict of Interest Statement
The authors declare that the research was conducted in the absence of any commercial or financial relationships that could be construed as a potential conflict of interest.
Acknowledgments
The authors want to thank the associate editor Victoria Miller and the reviewers for their valuable suggestions and comments which improved the quality of the manuscript.
Footnotes
References
Arangio, S., Calò, F., Di Mauro, M., Bonano, M., Marsella, M., and Manunta, M. (2014). An application of the SBAS-DInSAR technique for the assessment of structural damage in the city of Rome. Struct. Infrastruct. Eng. 10, 1469–1483. doi: 10.1080/15732479.2013.833949
Aucelli, P. P. C., Di Paola, G., Incontri, P., Rizzo, A., Vilardo, G., Benassai, G., et al. (2017). Coastal inundation risk assessment due to subsidence and sea level rise in a Mediterranean alluvial plain (Volturno coastal plain–southern Italy), estuarine. Coastal Shelf Sci. 198, 597–609. doi: 10.1016/j.ecss.2016.06.017
Avallone, A., Zollo, A., Briole, P., Delacourt, C., and Beauducel, F. (1999). Subsidence of campi flegrei (Italy) detected by SAR interferometry. Geophys. Res. Lett. 26, 2303–2306. doi: 10.1029/1999GL900497
Baldi, P., Casula, G., Cenni, N., Loddo, F., and Pesci, A. (2009). GPS-based monitoring of land subsidence in the Po Plain (Northern Italy). Earth Planet. Sci. Lett. 288, 204–212. doi: 10.1016/j.epsl.2009.09.023
Barberi, F., Corrado, G., Innocenti, F., and Luongo, G. (1984). Phlegraean fields 1982–1984: brief chronicle of a volcano emergency in a densely populated area. Bull. Volcanol. 47, 175–185. doi: 10.1007/BF01961547
Berardino, P., Fornaro, G., Lanari, R., and Sansosti, E. (2002). A new algorithm for surface deformation monitoring based on small baseline differential SAR interferograms. Geosci. Remote Sens. IEEE Trans. 40, 2375–2383. doi: 10.1109/TGRS.2002.803792
Bianchini, S., and Moretti, S. (2015). Analysis of recent ground subsidence in the Sibari plain (Italy) by means of satellite SAR interferometry-based methods. Int. J. Remote Sens. 36, 4550–4569. doi: 10.1080/01431161.2015.1084433
Bitelli, G., Bonsignore, F., Del Conte, S., Novali, F., Pellegrino, I., and Vittuari, L. (2015). “Integrated use of advanced InSAR and GPS data for subsidence monitoring,” in Engineering Geology for Society and Territory, Vol. 5, eds G. Lollino, A. Manconi, J. Clague, W. Shan, and M. Chiarle (Berlin: Springer), 147–150.
Bonardi, M., Canal, E., Cavazzoni, S., Barbero, R., Tosi, L., and Enzi, S. (1999). Impact of paleoclimatic fluctuations on depositional environments and human habitats in the Lagoon of Venice (Italy). World Resour. Rev. 11, 247–257.
Bonì, R., Pilla, G., and Meisina, C. (2016). Methodology for detection and interpretation of ground motion areas with the A-DInSAR time series analysis. Remote Sens. 8:686. doi: 10.3390/rs8080686
Botteghi, S., Montanari, D., Del Ventisette, C., and Moretti, S. (2015). Persistent scatterer interferometry (PSI) to detect surface deformation in the larderello-travale geothermal area (Tuscany, Italy). Rendicont. Online Soc. Geol. Ital. 35:398.
Bozzano, F., Esposito, C., Franchi, S., Mazzanti, P., Perissin, D., Rocca, A., et al. (2015). Understanding the subsidence process of a quaternary plain by combining geological and hydrogeological modelling with satellite InSAR data: the acque albule plain case study. Remote Sens. Environ. 168, 219–238. doi: 10.1016/j.rse.2015.07.010
Briganti, R., Ciufegni, S., Coli, M., Polimeni, S., and Pranzini, G. (2003). Underground florence; plio-quaternary geological evolution of the Florence area. Bollett. Soc. Geol. Ital. 122, 435–445.
Campolunghi, M. P., Capelli, G., Funiciello, R., and Lanzini, M. (2007). Geotechnical studies for foundation settlement in Holocenic alluvial deposits in the City of Rome (Italy). Eng. Geol. 89, 9–35. doi: 10.1016/j.enggeo.2006.08.003
Canova, F., Tolomei, C., Salvi, S., Toscani, G., and Seno, S. (2012). Land subsidence along the ionian coast of SE Sicily (Italy), detection and analysis via Small Baseline Subset (SBAS) multitemporal differential SAR interferometry. Earth Surf. Process. Landforms 37, 273–286. doi: 10.1002/esp.2238
Canuti, P., Casagli, N., Farina, P., Marks, F., Ferretti, A., and Menduni, G. (2005). “Land subsidence in the arno river basin studied through SAR interferometry,” in Proceedings of the 7th International Symposium on Land Subsidence, eds A. Zhang, S. Gong, L. Carbognin, and A. I. Johnson (Shanghai: Shanghai Institute of Geological Survey), 407–416.
Carminati, E., and Martinelli, G. (2002). Subsidence rates in the Po Plain, northern Italy: the relative impact of natural and anthropogenic causation. Eng. Geol. 66, 241–255. doi: 10.1016/S0013-7952(02)00031-5
Casagli, N., Frodella, W., Morelli, S., Tofani, V., Ciampalini, A., Intrieri, E., et al. (2017). Spaceborne, UAV and ground-based remote sensing techniques for landslide mapping, monitoring and early warning. Geoenviron. Disasters 4:9. doi: 10.1186/s40677-017-0073-1
Cascini, L., Ferlisi, S., Fornaro, G., Lanari, R., Peduto, D., and Zeni, G. (2006). Subsidence monitoring in Sarno urban area via multi-temporal DInSAR technique. Int. J. Remote Sens. 27, 1709–1716. doi: 10.1080/01431160500296024
Cascini, L., Ferlisi, S., Peduto, D., Fornaro, G., and Manunta, M. (2007). Analysis of a subsidence phenomenon via DInSAR data and geotechnical criteria. Ital. Geotech. J. 41, 50–67.
Cascini, L., Peduto, D., Pisciotta, G., Arena, L., Ferlisi, S., and Fornaro, G. (2013). The combination of DInSAR and facility damage data for the updating of slow-moving landslide inventory maps at medium scale. Nat. Hazards Earth Syst. Sci. 13, 1527–1549. doi: 10.5194/nhess-13-1527-2013
Cerchiello, V., Tessari, G., Velterop, E., Riccardi, P., Defilippi, M., and Pasquali, P. (2017). Building damage risk by modeling interferometric time series. IEEE Geosci. Remote Sens. Lett. 14, 509–513. doi: 10.1109/LGRS.2017.2651938
Cianflone, G., Tolomei, C., Brunori, C. A., and Dominici, R. (2015). InSAR time series analysis of natural and anthropogenic coastal plain subsidence: the case of Sibari (Southern Italy). Remote Sens. 7, 16004–16023. doi: 10.3390/rs71215812
Cigna, F., Bateson, L. B., Jordan, C. J., and Dashwood, C. (2014). Simulating SAR geometric distortions and predicting Persistent Scatterer densities for ERS-1/2 and ENVISAT C-band SAR and InSAR applications: nationwide feasibility assessment to monitor the landmass of Great Britain with SAR imagery. Remote Sens. Environ. 152, 441–466. doi: 10.1016/j.rse.2014.06.025
Cigna, F., Confuorto, P., Novellino, A., Tapete, D., Di Martire, D., Ramondini, M., et al. (2016). “25 years of satellite InSAR monitoring of ground instability and coastal geohazards in the archaeological site of Capo Colonna, Italy,” in Proceedings of the SAR Image Analysis, Modeling, and Techniques XVI: International Society for Optics and Photonics, Vol. 100030, eds C. Notarnicola, S. Paloscia, N. Pierdicca, and E. Mitchard (Warrington: SPIE).
Colombo, D., Farina, P., Moretti, S., Nico, G., and Prati, C. (2003). “Land subsidence in the Firenze-Prato-Pistoia basin measured by means of spaceborne SAR interferometry,” in Proceedings of the Geoscience and Remote Sensing Symposium, 2003: IGARSS’03 (Piscataway, NJ: IEEE International), 2927–2929. doi: 10.1109/IGARSS.2003.1294634
Comerci, V., Vittori, E., Cipolloni, C., Di Manna, P., Guerrieri, L., Nisio, S., et al. (2015). Geohazards monitoring in Roma from InSAR and in situ data: outcomes of the pangeo project. Pure Appl. Geophys. 172, 2997–3028. doi: 10.1007/s00024-015-1066-1
Costantini, M., Ferretti, A., Minati, F., Falco, S., Trillo, F., Colombo, D., et al. (2017). Analysis of surface deformations over the whole Italian territory by interferometric processing of ERS, envisat and COSMO-SkyMed radar data. Remote Sens. Environ. 202, 250–275. doi: 10.1016/j.rse.2017.07.017
Costantini, M., Iodice, A., Magnapane, L., and Pietranera, L. (2000). “Monitoring terrain movements by means of sparse SAR differential interferometric measurements,” in Proceedings of the Geoscience and Remote Sensing Symposium, 2000: IGARSS (Piscataway, NJ: IEEE International), 3225–3227.
Crosetto, M., Monserrat, O., Cuevas-González, M., Devanthéry, N., and Crippa, B. (2016). Persistent scatterer interferometry: a review. ISPRS J. Photogramm. Remote Sens. 115, 78–89. doi: 10.1016/j.isprsjprs.2015.10.011
Da Lio, C., Teatini, P., Strozzi, T., and Tosi, L. (2018). Understanding land subsidence in salt marshes of the Venice Lagoon from SAR Interferometry and ground-based investigations. Remote Sens. Environ. 205, 56–70. doi: 10.1016/j.rse.2017.11.016
Da Lio, C., and Tosi, L. (2018). Land subsidence in the Friuli Venezia Giulia coastal plain, Italy: 1992–2010 results from SAR-based interferometry. Sci. Total Environ. 633, 752–764. doi: 10.1016/j.scitotenv.2018.03.244
D’Auria, L., Pepe, S., Castaldo, R., Giudicepietro, F., Macedonio, G., Ricciolino, P., et al. (2015). Magma injection beneath the urban area of Naples: a new mechanism for the 2012–2013 volcanic unrest at Campi Flegrei caldera. Sci. Rep. 5:13100. doi: 10.1038/srep13100
Del Soldato, M., Riquelme, A., Bianchini, S., Tomàs, R., Di Martire, D., De Vita, P., et al. (2018). Multisource data integration to investigate one century of evolution for the Agnone landslide (Molise, southern Italy). Landslides 15, 1–16. doi: 10.1007/s10346-018-1015-z
Del Ventisette, C., Raspini, F., Ciampalini, A., Di Traglia, F., Moscatelli, M., Pagliaroli, A., et al. (2015). Use of PSInSAR data to map highly compressible soil layers. Geol. Acta Int. Earth Sci. J. 13, 309–323.
Di Paola, G., Alberico, I., Aucelli, P., Matano, F., Rizzo, A., and Vilardo, G. (2017). Coastal subsidence detected by Synthetic Aperture Radar interferometry and its effects coupled with future sea-level rise: the case of the Sele Plain (Southern Italy). J. Flood Risk Manage. 11, 191–206. doi: 10.1111/jfr3.12308
Dondi, L., Rizzini, A., and Rossi, P. (1985). “Recent geological evolution of the Adriatic Sea,” in Geological Evolution of the Mediterranean Basin, eds D. J. Stanley and F.C. Wezel (Berlin: Springer), 195–214. doi: 10.1007/978-1-4613-8572-1_9
Faunt, C. C., Sneed, M., Traum, J., and Brandt, J. T. (2016). Water availability and land subsidence in the Central Valley, California. Hydrogeol. J. 24, 675–684. doi: 10.1007/s10040-015-1339-x
Ferretti, A., Fumagalli, A., Novali, F., Prati, C., Rocca, F., and Rucci, A. (2011a). A new algorithm for processing interferometric data-stacks: SqueeSAR. IEEE Trans. Geosci. Remote Sens. 49, 3460–3470. doi: 10.1109/TGRS.2011.2124465
Ferretti, A., Tamburini, A., Novali, F., Fumagalli, A., Falorni, G., and Rucci, A. (2011b). Impact of high resolution radar imagery on reservoir monitoring. Energy Proc. 4, 3465–3471. doi: 10.1016/j.egypro.2011.02.272
Ferretti, A., Prati, C., and Rocca, F. (2001). Permanent scatterers in SAR interferometry, Geosci. Remote Sens. IEEE Trans. 39, 8–20. doi: 10.1109/36.898661
Fiaschi, S., Tessitore, S., Bonì, R., Di Martire, D., Achilli, V., Borgstrom, S., et al. (2017). From ERS-1/2 to Sentinel-1: two decades of subsidence monitored through A-DInSAR techniques in the Ravenna area (Italy). GISci. Remote Sens. 54, 305–328. doi: 10.1080/15481603.2016.1269404
Finno, R. J., Voss, F. T., Rossow, E., and Blackburn, J. T. (2005). Evaluating damage potential in building affected by excavations. ASCE J. Geotech. Geoenviron. Eng. 131, 1199–1210. doi: 10.1061/(ASCE)1090-0241(2005)131:10(1199)
Gabriel, A. K., Goldstein, R. M., and Zebker, H. A. (1989). Mapping small elevation changes over large areas: differential radar interferometry. J. Geophys. Res. Solid Earth 94(B7), 9183–9191. doi: 10.1029/JB094iB07p09183
Galloway, D. L., and Burbey, T. J. (2011). Regional land subsidence accompanying groundwater extraction. Hydrogeol. J. 19, 1459–1486. doi: 10.1007/s10040-011-0775-5
Galloway, D. L., Jones, D. R., and Ingebritsen, S. E. (1999). Land Subsidence in the United States. Reston, VR: US Geological Survey.
Galve, J. P., Pérez-Peña, J. V., Azañón, J. M., Closson, D., Caló, F., Reyes-Carmona, C., et al. (2017). Evaluation of the SBAS InSAR service of the european space agency’s geohazard exploitation platform (GEP). Remote Sens. 9:1291. doi: 10.3390/rs9121291
Gambolati, G., Ricceri, G., Bertoni, W., Brighenti, G., and Vuillermin, E. (1991). Mathematical simulation of the subsidence of Ravenna. Water Resour. Res. 27, 2899–2918. doi: 10.1029/91WR01567
Gambolati, G., and Teatini, P. (1998). Numerical analysis of land subsidence due to natural compaction of the Upper Adriatic Sea basin. CENAS 28, 103–131. doi: 10.1007/978-94-011-5147-4_5
Ghiglia, D. C., and Pritt, M. D. (1998). Two-Dimensional Phase Unwrapping: Theory, Algorithms, and Software. New York, NY: Wiley.
Hanssen, R. F. (2001). Radar Interferometry: Data Interpretation and Error Analysis. Berlin: Springer Science & Business Media. doi: 10.1007/0-306-47633-9
Hooper, A. (2008). A multi-temporal InSAR method incorporating both persistent scatterer and small baseline approaches. Geophys. Res. Lett. 35:L16302. doi: 10.1029/2008GL034654
Hu, R. L., Yue, Z. Q., Wang, L. C., and Wang, S. J. (2004). Review on current status and challenging issues of land subsidence in China. Eng. Geol. 76, 65–77. doi: 10.1016/j.enggeo.2004.06.006
Lanari, R., Berardino, P., Borgström, S., Del Gaudio, C., De Martino, P., Fornaro, G., et al. (2004). The use of IFSAR and classical geodetic techniques for caldera unrest episodes: application to the Campi Flegrei uplift event of 2000. J. Volcanol. Geotherm. Res. 133, 247–260. doi: 10.1016/S0377-0273(03)00401-3
Lu, P., Casagli, N., and Catani, F. (2010). PSI-HSR: a new approach for representing Persistent Scatterer Interferometry (PSI) point targets using the hue and saturation scale. Int. J. Remote Sens. 31, 2189–2196. doi: 10.1080/01431161003636716
Lundgren, P., Usai, S., Sansosti, E., Lanari, R., Tesauro, M., Fornaro, G., et al. (2001). Modeling surface deformation observed with synthetic aperture radar interferometry at Campi Flegrei caldera. J. Geophys. Res. Solid Earth 106(B9), 19355–19366. doi: 10.1029/2001JB000194
Manunta, M., Marsella, M., Zeni, G., Sciotti, M., Atzori, S., and Lanari, R. (2008). Two-scale surface deformation analysis using the SBAS-DInSAR technique: a case study of the city of Rome, Italy. Int. J. Remote Sens. 29, 1665–1684. doi: 10.1080/01431160701395278
Manzella, A., Bonciani, R., Allansdottir, A., Botteghi, S., Donato, A., Giamberini, S., et al. (2018). Environmental and social aspects of geothermal energy in Italy. Geothermics 72, 232–248. doi: 10.1016/j.geothermics.2017.11.015
Massonnet, D., and Feigl, K. L. (1998). Radar interferometry and its application to changes in the Earth’s surface. Rev. Geophys. 36, 441–500. doi: 10.1029/97RG03139
Matano, F., Sacchi, M., Vigliotti, M., and Ruberti, D. (2018). Subsidence trends of volturno river coastal plain (northern Campania, southern Italy) inferred by SAR interferometry data. Geosciences 8:8. doi: 10.3390/geosciences8010008
Meisina, C., Zucca, F., Fossati, D., Ceriani, M., and Allievi, J. (2006). Ground deformation monitoring by using the permanent scatterers technique: the example of the oltrepo pavese (Lombardia, Italy). Eng. Geol. 88, 240–259. doi: 10.1016/j.enggeo.2006.09.010
Meisina, C., Zucca, F., Notti, D., Colombo, A., Cucchi, A., Savio, G., et al. (2008). Geological interpretation of PSInSAR data at regional scale. Sensors 8, 7469–7492. doi: 10.3390/s8117469
Mora, O., Mallorqui, J. J., and Broquetas, A. (2003). Linear and nonlinear terrain deformation maps from a reduced set of interferometric SAR images. IEEE Trans. Geosci. Remote Sens. 41, 2243–2253. doi: 10.1109/TGRS.2003.814657
Pappone, G., Aucelli, P. P. C., Aberico, I., Amato, V., Antonioli, F., Cesarano, M., et al. (2012). Relative sea-level rise and marine erosion and inundation in the Sele river coastal plain (Southern Italy): scenarios for the next century. Rendiconti Lincei 23, 121–129. doi: 10.1007/s12210-012-0166-4
Peduto, D., Cascini, L., Arena, L., Ferlisi, S., Fornaro, G., and Reale, D. (2015). A general framework and related procedures for multiscale analyses of DInSAR data in subsiding urban areas. ISPRS J. Photogramm. Remote Sens. 105, 186–210. doi: 10.1016/j.isprsjprs.2015.04.001
Phien-Wej, N., Giao, P., and Nutalaya, P. (2006). Land subsidence in Bangkok, Thailand. Eng. Geol. 82, 187–201. doi: 10.1016/j.enggeo.2005.10.004
Pratesi, F., Tapete, D., Del Ventisette, C., and Moretti, S. (2016). Mapping interactions between geology, subsurface resource exploitation and urban development in transforming cities using InSAR persistent scatterers: two decades of change in Florence, Italy. Appl. Geogr. 77, 20–37. doi: 10.1016/j.apgeog.2016.09.017
Pratesi, F., Tapete, D., Terenzi, G., Del Ventisette, C., and Moretti, S. (2015). Rating health and stability of engineering structures via classification indexes of InSAR Persistent Scatterers. Int. J. Appl. Earth Observ. Geoinform. 40, 81–90. doi: 10.1016/j.jag.2015.04.012
Prokopovich, N. P. (1979). “Genetic classification of land subsidence,” in Evaluation and Prediction of Subsidence, ed. S. K. Saxena (New York, NY: ASCE), 389–399.
Raspini, F., Bianchini, S., Ciampalini, A., Del Soldato, M., Solari, L., Novali, F., et al. (2018). Continuous, semi-automatic monitoring of ground deformation using Sentinel-1 satellites. Sci. Rep. 8, 1–10. doi: 10.1038/s41598-018-25369-w
Raspini, F., Bianchini, S., Moretti, S., Loupasakis, C., Rozos, D., Duro, J., et al. (2016). Advanced interpretation of interferometric SAR data to detect, monitor and model ground subsidence: outcomes from the ESA-GMES Terrafirma project. Nat. Hazards 83, 155–181. doi: 10.1007/s11069-016-2341-x
Raspini, F., Cigna, F., and Moretti, S. (2012). Multi-temporal mapping of land subsidence at basin scale exploiting Persistent Scatterer Interferometry: case study of Gioia Tauro plain (Italy). J. Maps 8, 514–524. doi: 10.1080/17445647.2012.743440
Righini, G., Raspini, F., Moretti, S., and Cigna, F. (2011). Unsustainable use of groundwater resources in agricultural and urban areas: a persistent scatterer study of land subsidence at the basin scale. WIT Trans. Ecol. Environ. 144, 81–92. doi: 10.2495/ECO110071
Rosi, A., and Agostini, A. (2013). Subsidence analysis in the Cornia river basin (Southern Tuscany, Italy) by using PSInSAR technique. Rend. Online Soc. Geol. Ital 24, 276–278.
Rosi, A., Agostini, A., Tofani, V., and Casagli, N. (2014). A procedure to map subsidence at the regional scale using the persistent scatterer interferometry (PSI) technique. Remote Sens. 6, 10510–10522. doi: 10.3390/rs61110510
Rosi, A., Tofani, V., Agostini, A., Tanteri, L., Stefanelli, C. T., Catani, F., et al. (2016). Subsidence mapping at regional scale using persistent scatters interferometry (PSI): the case of Tuscany region (Italy). Int. J. Appl. Earth Observ. Geoinform. 52, 328–337. doi: 10.1016/j.jag.2016.07.003
Samsonov, S. V., Tiampo, K. F., Camacho, A. G., Fernández, J., and González, P. J. (2014). Spatiotemporal analysis and interpretation of 1993–2013 ground deformation at Campi Flegrei, Italy, observed by advanced DInSAR. Geophys. Res. Lett. 41, 6101–6108. doi: 10.1002/2014GL060595
Scifoni, S., Bonano, M., Marsella, M., Sonnessa, A., Tagliafierro, V., Manunta, M., et al. (2016). On the joint exploitation of long-term DInSAR time series and geological information for the investigation of ground settlements in the town of Roma (Italy). Remote Sens. Environ. 182, 113–127. doi: 10.1016/j.rse.2016.04.017
Solari, L., Barra, A., Herrera, G., Bianchini, S., Monserrat, O., Béjar-Pizarro, M., et al. (2018). Fast detection of ground motions on vulnerable elements using Sentinel-1 InSAR data. Geomat. Natural Hazards Risk 9, 152–174. doi: 10.1080/19475705.2017.1413013
Solari, L., Ciampalini, A., Bianchini, S., and Moretti, S. (2016a). PSInSAR analysis in urban areas: a case study in the Arno coastal plain (Italy). Rendiconti Online Soc. Geol. Ital. 41, 255–258. doi: 10.3301/ROL.2016.142
Solari, L., Ciampalini, A., Raspini, F., Bianchini, S., and Moretti, S. (2016b). PSInSAR analysis in the pisa urban area (Italy): a case study of subsidence related to stratigraphical factors and urbanization. Remote Sens. 8:120. doi: 10.3390/rs8020120
Solari, L., Ciampalini, A., Raspini, F., Bianchini, S., Zinno, I., Bonano, M., et al. (2017). Combined use of C-and X-Band SAR data for subsidence monitoring in an urban area. Geosciences 7:21. doi: 10.3390/geosciences7020021
Stramondo, S., Bozzano, F., Marra, F., Wegmuller, U., Cinti, F., Moro, M., et al. (2008). Subsidence induced by urbanisation in the city of Rome detected by advanced InSAR technique and geotechnical investigations. Remote Sens. Environ. 112, 3160–3172. doi: 10.1016/j.rse.2008.03.008
Stramondo, S., Saroli, M., Tolomei, C., Moro, M., Doumaz, F., Pesci, A., et al. (2007). Surface movements in bologna (Po Plain—Italy) detected by multitemporal DInSAR. Remote Sens. Environ. 110, 304–316. doi: 10.1016/j.rse.2007.02.023
Strozzi, T., Teatini, P., and Tosi, L. (2009). TerraSAR-X reveals the impact of the mobile barrier works on Venice coastland stability. Remote Sens. Environ. 113, 2682–2688. doi: 10.1016/j.rse.2009.08.001
Strozzi, T., Tosi, L., Wegmuller, U., Werner, C., Teatini, P., and Carbognin, L. (2003). “Land subsidence monitoring service in the Lagoon of Venice,” in Proceedings of the Geoscience and Remote Sensing Symposium, 2003: IGARSS’03 (Piscataway, NJ: IEEE International), 212–214. doi: 10.1109/IGARSS.2003.1293727
Tapete, D., and Cigna, F. (2012). Satellite-Based Preventive Diagnosis: Use of Persistent Scatterer Interferometry on Cultural Heritage Sites in Italy. London: RSPSoc.
Teatini, P., Ferronato, M., Gambolati, G., and Gonella, M. (2006). Groundwater pumping and land subsidence in the Emilia-Romagna coastland, Italy: modeling the past occurrence and the future trend. Water Resour. Res. 42:W01406. doi: 10.1029/2005WR004242
Teatini, P., Tosi, L., and Strozzi, T. (2011). Quantitative evidence that compaction of Holocene sediments drives the present land subsidence of the Po Delta, Italy. J. Geophys. Res. Solid Earth 116:B08407. doi: 10.1029/2010JB008122
Teatini, P., Tosi, L., Strozzi, T., Carbognin, L., Wegmüller, U., and Rizzetto, F. (2005). Mapping regional land displacements in the Venice coastland by an integrated monitoring system. Remote Sens. Environ. 98, 403–413. doi: 10.1016/j.rse.2005.08.002
Terzaghi, K., and Peck, R. B. (1967). Soil Mechanics in Engineering Practice. Hoboken, NJ: John Wiley & Sons.
Tiampo, K. F., González, P. J., Samsonov, S., Fernández, J., and Camacho, A. (2017). Principal component analysis of msbas dinsar time series from Campi flegrei, Italy. J. Volcanol. Geother. Res. 344, 139–153. doi: 10.1016/j.jvolgeores.2017.03.004
Tomás, R., and Li, Z. (2017). Earth Observations for Geohazards: Present and Future Challenges. Basel: Multidisciplinary Digital Publishing Institute.
Tomás, R., Romero, R., Mulas, J., Marturià, J. J., Mallorquí, J., Lopez-Sanchez, J. M., et al. (2014). Radar interferometry techniques for the study of ground subsidence phenomena: a review of practical issues through cases in Spain. Environ. Earth Sci. 71, 163–181. doi: 10.1007/s12665-013-2422-z
Tosi, L., Carbognin, L., Teatini, P., Strozzi, T., and Wegmüller, U. (2002). Evidence of the present relative land stability of Venice, Italy, from land, sea, and space observations. Geophys. Res. Lett. 29, 3-1–3-4.
Tosi, L., Da Lio, C., Strozzi, T., and Teatini, P. (2016). Combining L-and X-band SAR interferometry to assess ground displacements in heterogeneous coastal environments: the Po River Delta and Venice Lagoon, Italy. Remote Sensing 8:308. doi: 10.3390/rs8040308
Tosi, L., Teatini, P., Carbognin, L., and Brancolini, G. (2009). Using high resolution data to reveal depth-dependent mechanisms that drive land subsidence: the Venice coast, Italy. Tectonophysics 474, 271–284. doi: 10.1016/j.tecto.2009.02.026
Tosi, L., Teatini, P., Strozzi, T., Carbognin, L., Brancolini, G., and Rizzetto, F. (2010). Ground surface dynamics in the northern Adriatic coastland over the last two decades. Rendiconti Lincei 21, 115–129. doi: 10.1007/s12210-010-0084-2
Trasatti, E., Casu, F., Giunchi, C., Pepe, S., Solaro, G., Tagliaventi, S., et al. (2008). The 2004-2006 uplift episode at Campi Flegrei caldera (Italy): constraints from SBAS-DInSAR ENVISAT data and Bayesian source inference. Geophys. Res. Lett. 35:L07308. doi: 10.1029/2007GL033091
Ventura, G., Vilardo, G., and Sepe, V. (2009). “Monitoring and structural significance of ground deformations at Campi Flegrei supervolcano (Italy) from the combined 2D and 3D analysis of PS-InSAR, geophysical, geological and structural data,” in Proceedings of the 6th International Symposium on Digital Earth, Beijing.
Vilardo, G., Isaia, R., Ventura, G., De Martino, P., and Terranova, C. (2010). InSAR permanent scatterer analysis reveals fault re-activation during inflation and deflation episodes at Campi Flegrei caldera. Remote Sens. Environ. 114, 2373–2383. doi: 10.1016/j.rse.2010.05.014
Vilardo, G., Ventura, G., Terranova, C., Matano, F., and Nardò, S. (2009). Ground deformation due to tectonic, hydrothermal, gravity, hydrogeological, and anthropic processes in the Campania Region (Southern Italy) from Permanent Scatterers Synthetic Aperture Radar Interferometry. Remote Sens. Environ. 113, 197–212. doi: 10.1016/j.rse.2008.09.007
Walter, T., Shirzaei, M., Manconi, A., Solaro, G., Pepe, A., Manzo, M., et al. (2014). Possible coupling of Campi Flegrei and Vesuvius as revealed by InSAR time series, correlation analysis and time dependent modeling. J. Volcanol. Geother. Res. 280, 104–110. doi: 10.1016/j.jvolgeores.2014.05.006
Wegmuller, U., Strozzi, T., and Bitelli, G. (1999). “Validation of ERS differential SAR interferometry for land subsidence mapping: the Bologna case study,” in Proceedings of the Geoscience and Remote Sensing Symposium, 1999: IGARSS’99 (Piscataway, NJ: IEEE International), 1131–1133. doi: 10.1109/IGARSS.1999.774555
Werner, C., Wegmuller, U., Strozzi, T., and Wiesmann, A. (2003). “Interferometric point target analysis for deformation mapping,” in Geoscience and Remote Sensing Symposium, 2003: IGARSS’03 (Piscataway, NJ: IEEE International), 4362–4364.
Zanchetta, G., Bonadonna, F., Esu, D., Grassi, R., Leone, G., and Mazza, P. (1998). Stratigraphic and paleontologic aspects of middle Pleistocene continental deposits from lower Valdarno (Tuscany). Boll. Soc. Geol. Ital. 117, 113–132.
Zebker, H. A., Rosen, P. A., and Hensley, S. (1997). Atmospheric effects in interferometric synthetic aperture radar surface deformation and topographic maps. J. Geophys. Res. Solid Earth 102, 7547–7563. doi: 10.1029/96JB03804
Keywords: subsidence, DInSAR, MTInSAR, urban monitoring, local and regional scale applications, satellite monitoring
Citation: Solari L, Del Soldato M, Bianchini S, Ciampalini A, Ezquerro P, Montalti R, Raspini F and Moretti S (2018) From ERS 1/2 to Sentinel-1: Subsidence Monitoring in Italy in the Last Two Decades. Front. Earth Sci. 6:149. doi: 10.3389/feart.2018.00149
Received: 01 July 2018; Accepted: 18 September 2018;
Published: 04 October 2018.
Edited by:
Victoria Miller, The University of the West Indies St. Augustine, Trinidad and TobagoReviewed by:
Yuri Fialko, University of California, San Diego, United StatesJoern Lauterjung, Helmholtz-Zentrum Potsdam Deutsches Geoforschungszentrum, Helmholtz-Gemeinschaft Deutscher Forschungszentren (HZ), Germany
Copyright © 2018 Solari, Del Soldato, Bianchini, Ciampalini, Ezquerro, Montalti, Raspini and Moretti. This is an open-access article distributed under the terms of the Creative Commons Attribution License (CC BY). The use, distribution or reproduction in other forums is permitted, provided the original author(s) and the copyright owner(s) are credited and that the original publication in this journal is cited, in accordance with accepted academic practice. No use, distribution or reproduction is permitted which does not comply with these terms.
*Correspondence: Lorenzo Solari, bG9yZW56by5zb2xhcmlAdW5pZmkuaXQ=