- 1Department of Geography, University of Utah, Salt Lake City, UT, United States
- 2Department of Geography, University of Wyoming, Laramie, WY, United States
In 1916 the time stamp for quantitative palynology was set with Lennart von Post's initial paper on pollen analysis and environmental change in the Scandinavian peat bogs. In the 1930s, Von Post provided a map of the known palynological reconstructions. This map showed many conspicuous gaps of geographic coverage that have endured to this day. In particular, environmental reconstruction in arid lands remain much less known, largely due to the paucity of depositional environments in these areas as well as challenges with preservation in regions either uniformly dry or subjected to strong wet/dry phases. Over the last decade we have examined linkages between desert wetland development and episodes of wet and arid conditions. Desert wetland, or ciénegas, are recharged by groundwater and appear to be sensitive to climate-driven groundwater fluctuations. These systems appear to “grow” during wet periods potentially associated with enhanced El Niño activity, suggesting an important linkage with groundwater dynamics and the quantity and frequency of winter precipitation delivery. Hydrologic conditions in ciénegas are also important controls on the preservation of pollen, where episodes of aridity coincide with periods of poor pollen preservation. We assess modern El Niño events, as analogs of past wet conditions, to provide context on the atmospheric controls for delivery of moisture into the desert southwest during winter. Our analysis shows that anomalously high and persistent moisture delivery into the region during El Niño events enables the growth of ciénegas, improves preservation of pollen and promotes the growth of fuels necessary to support wildfire. This paper examines ciénega sites located in the southwestern region of North America at the US/Mexico Border and discusses results that addresses a geographical gap identified by Von Post's original work in paleoenvironmental research.
Introduction
The legacy of Lennart Von Post on quantitative paleoecology and palynology cannot be overstated. While Von Post did not invent palynology, he helped to cement this field of study through his foundational research conducted in Sweden and Norway during the late 1800s and early 1900s. Von Post's approach to paleoenvironmental reconstruction made its way to the American Deserts via the geochronological work of Ernst Antevs and American researcher Kirk Bryan (Manten, 1967; Smiley, 1974). While Antevs did not focus on pollen analysis himself, he was interested enough in the technique to opine on the naming of the methodology (Antevs, 1944) and his work was critical to subsequent paleoenvironmental work in the desert landscapes of southwestern North America (hereinafter “southwestern deserts”).
Early pollen work in the southwestern deserts focused on woodland expansion (Clisby and Sears, 1956; Martin and Gray, 1962; Martin, 1963; Mehringer, 1965; Meyer, 1973). This initial research reported observations of periods where no pollen or low pollen abundances were recovered. These results altered the relative abundances of pollen types, which was interpreted to be caused by differential preservation and the individual researchers ability to identify degraded pollen grains. For example, through careful examination, the crumbled or degraded pollen grains of Pinus, Artemisia, Poaceae, and Amaranthaceae are easily distinguishable by the presence of distinct wall or annulated pore characteristic of the particular type. However, degradation and preservation issues reveal the difficult nature of arid land palynology. In short, the lack of perennial lacustrine environments results in discontinuous records of environmental change. Further, simple abundance in arid lands seems lower than one might experience in temperate environments. Subsequent research concentrated on macrobotanical records within woodrat middens (see Betancourt et al., 1990), instead of the ephemeral sedimentary nature of desert wetlands (however see Davis et al., 2002; Mensing et al., 2008).
In arid regions, environments suitable for pollen preservation are limited to riparian deposits, ciénegas or wetlands (Davis et al., 2002; Minckley and Brunelle, 2007), or on the deflated surfaces of former lake beds (Clisby and Sears, 1956; Martin, 1963; Meyer, 1973). Of these environments, ciénegas are proven archives of past environments and climate conditions as they collect charcoal, pollen, and macrofossils over thousands of years (Martin, 1963; Hall, 1985; Hendrickson and Minckley, 1985; Davis et al., 2002; Minckley and Brunelle, 2007; Brunelle et al., 2010; Pigati et al., 2014). The global distribution of desert wetland deposits has not been mapped, but they are considered to be widespread, though in other literature they are referred to as ground water deposits associated with hot or cold springs, seeps, or other wetland complexes (Pigati et al., 2014; Sáez et al., 2016). However, systematic studies of these complex systems are relatively recent, variously focusing on lithological, isotopic, or biological indicators (Minckley and Brunelle, 2007; Quade et al., 2008; Minckley et al., 2013a; Brunelle et al., 2014; Pigati et al., 2014 and references therein, Sáez et al., 2016).
Arid and semi-arid environments occupy 40% of the terrestrial land area on earth and in many cases represent areas where recent human population growth rates are high relative to more mesic regions (18.5% between 1990 and 2000; Hassan et al., 2005). The consumption of natural resources associated with rapid population growth places intense pressure on water resources, such as ciénegas. In addition to being a ready source of water, ciénegas provide crucial ecosystem services including erosion mitigation and prevention of the associated lowering of the water table. Ciénegas have also been identified as regions of high conservation priority for many reasons including endemism (Minckley, 1969, 1992; Hendrickson and Minckley, 1985; Abell, 2000). In Arizona, USA where wetland environments occupy ~2% of the land area, ciénegas are critical habitat for at least 19% of the threatened, candidate, or endangered species within the state (Baker et al., 2004). However, beyond species of concern, desert ciénegas and riparian corridors may increase regional biodiversity by up to 50% in some cases (Sabo et al., 2005).
Over the last decade, we examined sediment collected from ciénega systems located in the southwestern deserts to understand the hydrology, ecology, disturbance regimes, climatology, and environmental change from these critical systems. Our work builds on the legacies of scholars working in this region, including Antevs, Martin, and Mehringer (e.g., Antevs, 1962; Martin, 1963; Mehringer, 1967). Consideration of ciénegas as repositories of environmental history was largely untouched until Hendrickson and Minckley (1985) published “Ciénegas—Vanishing Climate Communities in the American Southwest.” Paleoecological research of these sedimentary environments was largely unexplored until Davis et al. (2002) published a synthesis of several sites that examined changes in burning regime with European settlement. However, a large-scale evaluation of ciénega form and function has not yet been undertaken (see Pigati et al., 2014).
In this paper, we present data from four ciénega sites in the Arizona/New Mexico-Mexico borderlands using multiproxy analyses. Herein, we present data from previously published research as well as new records to provide a regional perspective on vegetation and disturbance history from the borderlands region. We detail our approach to handling differential pollen preservation and explain how sedimentary indicators may also provide evidence for hydrology as a control on pollen preservation. Despite the analytical challenges associated with ciénega sediments, the environmental record we present here shows regional coherence of wet-dry episodes. We show that pollen records from ciénegas provide insight to past climatological states related to variations in winter precipitation. For the desert Southwest, this is currently associated with quasi-periodic El Niño Southern Oscillation (ENSO) variations. In the tradition of Von Post, our first objective is to identify conditions in the sedimentological proxy data (e.g., pollen and charcoal) that record changes in ciénega systems attributed to climate. For our second objective, we identify modern episodes that are analogous to conditions found in the paleoecological record (strong El Niño conditions) associated with ciénega growth (e.g., wet episodes) to assess the dominant atmospheric drivers related to enhanced winter precipitation in the arid southwest.
To address our first objective, we utilize indices of pollen preservation and charcoal abundance. We assess pollen preservation in ciénega systems of southwestern New Mexico and southeastern Arizona as well as the relationship to past ENSO variability based on our modern understanding of ENSO and southwestern North American hydroclimate teleconnections (Heyer et al., 2017). Further, we put forward past fire activity near the ciénegas was covariant with ENSO variability, similar to connections observed in historic southwestern forest fire research (Swetnam and Betancourt, 1990). Considering fuel availability produced during antecedent wet seasons is closely linked to wildfire in arid and semi-arid environments globally (Krawchuk and Moritz, 2011), we consider our linkage of past fire activity and ENSO variability viable. To address our second objective, on the impact that El Niño events have on Southwestern climate, we use high-resolution modern climate data to assess teleconnections between moisture delivery and southwestern precipitation anomalies during winter and spring of strong El Niño events as a mechanism for sustained wet conditions that are conducive for pollen preservation in ciénegas.
Regional Description
The southwestern deserts, particularly those in the Sonoran and Chihuahuan systems, have a high concentration of ciénegas (Hendrickson and Minckley, 1985; Minckley et al., 2013b; Cole and Cole, 2015). These wetlands are found in a variety of geomorphic contexts along riverine or stream channels, off-channel seeps, landslide backfill, and valley bottoms, to name a few. Often ciénegas are proximal to fault lines, which are common in the desert basin and range structures of western North America (Minckley, unpublished).
The modern climatic context of the arid southwest of North America is characterized as having bimodal precipitation (Mock, 1996; Shinker, 2010). Winter precipitation has broad spatial-scale patterns with persistent light precipitation delivered by midlatitude frontal systems (Mock, 1996; Comrie and Glenn, 1998; Shinker, 2010). In contrast, summer precipitation is highly variable spatially and typically short in duration associated with convective thunderstorms delivered via the North American Monsoon System (NAMS; Mock, 1996; Adams and Comrie, 1997; Sheppard et al., 2002; Diem and Brown, 2006; Shinker, 2010). The modern NAMS developed after climate started to warm and the Laurentide ice sheet began to collapse at the end of the last ice age. This warming led to the formation of summer thermal lows that provide a mechanism for drawing moisture from the Gulf of Mexico and southeastern Pacific (Spaulding, 1991; Adams and Comrie, 1997; Minckley et al., 2004). The bimodal nature of precipitation in the southwest, especially the extra precipitation in the summer associated with the NAMS, provides effective moisture at the surface related to water- and energy-balance components important for ecosystem and water resources related to vegetation and the ciénega systems of the southwest (Shinker and Bartlein, 2010).
In addition to the bimodal climate of the southwest, large-scale modes of variability such as ENSO impacts southwestern hydroclimate and ecosystems (Ropelewski and Halpert, 1986; Cole and Cook, 1998; Gershunov and Barnett, 1998a; Cayan et al., 1999; Dettinger et al., 2000). El Niño is the anomalous warming of the near-surface water off the western coast of South America that initiated about 5000 years ago (Enfield, 1992; Liu et al., 2014). Its atmospheric counterpart is the Southern Oscillation, which reflects changes in barometric pressure (Wallace and Gutzler, 1981; Enfield, 1992). Strong El Niño years in the modern record typically result in higher-than-normal winter precipitation in the southwestern United States; while strong La Niña typically causes lower-than-normal winter precipitation and is typically associated with regional drought (Shinker and Bartlein, 2009). Studies have identified a north-to-south precipitation dipole correlated with ENSO in the western U.S., showing a strong relationship in the southwest between cool season (Oct-Mar) precipitation and El Niño events (Dettinger et al., 1998; Wise, 2010). Further, many studies have shown statistically significant correlations between ENSO and western North America precipitation, specifically in the southwest where spatially coherent correlations are found during the cool season (Dettinger et al., 1998; Heyer et al., 2017). However, various modes of ENSO are associated with different impacts on western NA hydroclimate (Ashok et al., 2007), and teleconnections can vary on decadal-to-centennial time scales (Gershunov and Barnett, 1998b), suggesting that southwest hydroclimate does not always respond to ENSO variability in a linear manner based on our modern understanding of southwest hydroclimate and ENSO correlations (Heyer et al., 2017). However, despite teleconnection variability (Gershunov and Barnett, 1998b), dipole variability (Wise, 2010) and different modes of ENSO (Ashok et al., 2007), paleo-sedimentary records suggest teleconnections between ENSO and southwest climate are similar to those observed in the modern record, and have persisted at century–millennial time scales (Barron and Anderson, 2010; Antinao and McDonald, 2013; Kirby et al., 2014; Hart et al., 2015). Further, paleoecological records have revealed the ENSO phenomenon is correlated with fire regimes of the southwest (Swetnam and Betancourt, 1990). Therefore, our research on San Bernardino Ciénega suggests that over centuries-to-millenial scales fire frequency trends are consistent with ENSO variability, with fire frequency increasing (decreasing) when El Niño (La Niña) was active (Brunelle et al., 2010).
Our work has been focused on ciénegas near the US/Mexico borderlands region in southern Arizona and New Mexico (Figure 1) in the monsoon-dominated ecotone of the Sonoran and Chihuahuan deserts and in a region well correlated with ENSO activity (Wise, 2010; Heyer et al., 2017). Total annual precipitation is around 254 mm (Western Regional Climate Center, 2017). Summer temperatures are generally around 37°C while winter temperatures rarely fall below freezing.
Site Descriptions
San Bernardino Ciénega (31.3°N; 109.3°W, 1,160 m asl; Figure 1) is located in the drainage of Black Draw Wash/Rio de San Bernardino (RSB) of southeastern Arizona, USA and northeastern Sonora, MX near the ecotone of Chihuahuan and Sonoran grassland and desert scrub (Minckley and Brunelle, 2007; Minckley et al., 2009, 2011; Brunelle et al., 2010). Currently the ciénega surface is dry except for a few artificial impoundments and small perennial springs.
Cloverdale Ciénega is located in the Peloncillo Mountains, Coronado National Forest, NM (31°26.141′ N, 108°58.517 W, 1,640 m asl; Figure 1). Cloverdale Ciénega is situated at the ecotone between Madrean woodland and grassland (Brunelle et al., 2014), making this location sensitive to changes in community composition over time. Currently, the Madrean woodlands form a mosaic of clustered tree stands interspersed with patches of shrub and grass (Brown, 1982).
Canelo Hills Ciénega (31° 33.833′N, 110° 31.711′W, 1,506 m asl; Figure 1) is a Nature Conservancy preserve located southeast of Sonoita, Arizona on the east side of the Canelo Hills. The area is a Madrean grassland.
Babocamari Ciénega (31° 37.901′N, 110° 27.178′W, 1,391 m asl; Figure 1) is southeast of Sonoita, Arizona and located between the Whetstone and Huachuca Mountains. The site is considered Madrean grassland.
Methods
Field
San Bernardino
Sediments were collected from an active spring (Snail Spring), the incised channel wall of the Rio de San Bernardino arroyo (RSBA), and the ciénega surface of the San Bernardino National Wildlife Refuge (SBNWR). The RSBA samples were described and collected contiguously from a freshly exposed sediment face with a trowel. A 15-m long core was obtained from the SBNWR using a truck-mounted 5″ (12.7 mm) hollow barrel auger (Minckley et al., 2007). The Snail Springs samples were collected using a modified Livingstone piston corer.
Cloverdale, Babocamari, and Canelo Hills Ciénegas
Sediment cores were collected using a vibracore system where manual probes indicated maximum sediment accumulation. The vibracore apparatus uses a cement leveling motor attached to a 3-inch aluminum tube inserted into the sediments until refusal (bedrock). Sediment was retained in the aluminum tube by placing an airtight cap on the top of the tube to create a vacuum, allowing the sediment core to be removed from the ground without loss. The cores were transported in the aluminum tube to the University of Utah and split in the lab for description and sampling.
Laboratory and Analytical
Once the cores were removed from the aluminum tube, the color and texture of the sediments were described. Every contiguous centimeter of the core was examined for charcoal analysis. For pollen analysis, samples were collected to acquire a minimum sample resolution of approximately every 200 years. The sediments were weakly stratified, massive, and organic.
Age-depth relationships were determined for the sediments based on AMS (accelerator mass spectrometry) dates on pollen concentrates, lead-210 chronology (when available), and the surface (collection year; Table 1). Ages are presented in calibrated years before present (cal yr BP) where 1950 CE is equal to year 0 cal yr BP. The age-depth models were generated in CLAM (Blaauw, 2010) using smoothing splines (Figure 2). The age model for San Bernardino was created using multiple linear regressions and was not rerun due to the multiple publications already based on the existing chronology (Brunelle et al., 2010 and citations therein).
Pollen extraction methods followed Faegri et al. (1989). For pollen analysis, we attempted to identify at least 300 terrestrial pollen grains to complete the rarefaction curve associated with pollen diversity (Maher, 1963). Pollen grains were identified at a magnification of 500X to the lowest possible taxonomic classification using published atlases (e.g., Erdtman, 1969; Kapp et al., 2000). Counts were converted to percentages based on the sum of total terrestrial grains and influx using the sedimentation rate calculated using the age models. In desert sediments, a 300-grain terrestrial count is not always obtainable because of poor preservation (Minckley and Brunelle, 2007; Minckley et al., 2011). Our standard has been to attempt 300-grain terrestrial counts or 300–1000 Lycopodium spore (exotic spore added as a tracer) tallies for each depth. If pollen counts do not exceed at least 100 terrestrial pollen grains we plot pollen presence instead of abundance to avoid artificial inflation of identifiable pollen types as presenting ecological significance, an issue common in earlier studies as detailed in the Introduction.
A second way we avoid misinterpretation of desert pollen assemblages is by determining an index of pollen preservation (“preservation index”) where we consider the number of Lycopodium tracers to the total number of identified grains. Studying the way pollen has been preserved can help to determine changes in the deposition or preservation environment. This type of examination is a relatively underused technique (Tipping, 2000) that could be more widely used as an interpretive tool (Tweddle and Edwards, 2010). In our study, the preservation index is used as an indicator of a decrease in water table height that would expose deposited grains to conditions of wetting and drying which are known to be consistent with deterioration (Campbell and Campbell, 1994; Tweddle and Edwards, 2010). The depth to water table in ciénega settings controls the water level at the surface. In turn, the water table is largely controlled by the amount of winter precipitation. High preservation indices are used to indicate wet (active) ciénega surfaces, while low indices indicate dry (inactive) surfaces.
Volumetric samples were taken from the sediments for charcoal analysis to reconstruct fire history. Sample volumes ranged from 1 to 10 cc based on the amount of charcoal found at various depths throughout the sections. Sample volume was adjusted to maintain a significant number of charcoal particles, but to reduce excessive counts (>1,000 particles). Methods and rationale follow Clark (1988) and Long et al. (1998). While taphonomic studies of large particle deposition on fluvial wetland surfaces have not been conducted, isotopic analysis of ciénega sediments indicates pooling and stagnation of surface waters (Minckley et al., 2009), suggesting sedimentation of charcoal fragments would be similar to those of lake and bog surfaces. Charcoal concentrations (particles/cm3) were converted to influx (particles/cm2/yr) using the age models.
Statistical Analyses
To compare ciénega CHAR record to regional charcoal variability, regional charcoal for Arizona, New Mexico, Southern Utah, and Southern Colorado (i.e., latitude >30, latitude <38, longitude ≤105, longitude ≥113) were collected from the paleofire database (Blarquez et al., 2014), and used in correlative analyses. A total of 13 sites were included, of which all sites are from mid-upper elevations (i.e., >2,300 meters) with the exception of the Montezuma Well site (near 1,100 meters). Prior to comparing regional charcoal to ciénega charcoal, regional charcoal data were averaged (i.e., long-term average 10,000 cal yr BP—present) and standardized to z-scores. Charcoal data were then composited using a locally weighted scatterplot smoother and bootstrapping techniques (Blarquez et al., 2014).
Prior to correlation analyses, the CHAR and pollen preservation ratio data were decomposed into pseudoannual values and then reaggregated into 100-year bins to be comparable to the Moy et al. (2002) El Niño event frequency reconstruction. The time series of all the ciénega sites were then averaged to provide a comprehensive trend of the series. To quantitatively describe the relationships between the ENSO index (Moy et al., 2002) and the preservation ratio and CHAR time series, a Pearson product-moment correlation was run on the time series providing correlation coefficients and p-values.
Correlations were performed between data from 7,700 cal yr BP to present for mean-pollen preservation, and from 8,100 cal yr BP to present for mean-charcoal accumulation. Correlations were between ciénega sites mean-pollen preservation, ciénega sites mean-charcoal accumulation, regional standardized charcoal accumulation, and El Niño events/100 years (Moy et al., 2002). From 8,100 to 5,400 cal yr BP, only the San Bernardino ciénega site was active, with pollen preservation and charcoal accumulation at or near zero, as expected considering El Niño event inactivity. Therefore, correlation analyses from 8,100 to 5,400 cal yr BP is not based on an actual average. However, we assume a lack of pollen preservation and charcoal accumulation at the inactive ciénega sites was due to a lack groundwater recharge, which may represent a reduction of persistent winter and spring precipitation potentially associated with reduced El Niño events (Scanlon et al., 2006; Heyer et al., 2017). We suggest if the other three ciénega sites had been active, then pollen preservation and charcoal accumulation would have been close to zero, similar to the San Bernardino ciénega. Thus, assuming the other ciénega sites would have also had pollen preservation and charcoal accumulation near zero, we only use pollen preservation and charcoal accumulation data from the San Bernardino ciénega to calculate the long term mean-pollen preservation (i.e., 7,700 cal yr BP—present) and mean-charcoal accumulation (i.e., 8,100 cal yr BP—present). From 5,300 to 0 cal yr BP at least two ciénega sites recorded data, allowing for a mean to be calculated.
Modern Climate Analog Approach
While proxy data such as charcoal, pollen and macrofossils provide a record of past ciénega variability from an ecological perspective, paleoecological data does not provide a record of the climatic mechanisms that caused such variability (Shinker et al., 2006). Therefore, understanding modern climate mechanisms and processes that cause variability in ciénegas and the sensitivity and range of ecological responses to such changes are fundamental to ecosystem management. We incorporate a modern climate analog technique (Mock and Shinker, 2013) in order to understand the climate processes and mechanisms associated with ENSO conditions that are evident in the sedimentological record from ciénega sites across the United States/Mexico borderlands. The modern climate analog technique (Mock and Shinker, 2013) relies on the principle of uniformitarianism and assumes that modern synoptic and dynamic climate processes operated similarly today as they did in the past. The modern climate analog approach is an effective way to identify climate mechanisms associated with past environmental changes (e.g., as seen in reconstructed sedimentary pollen analyses; Mock and Brunelle-Daines, 1999; Shinker et al., 2006; Shinker, 2014). A modern climate analog is a conceptual model that uses modern extremes (e.g., wet or dry episodes) as analogs of past events (e.g., vegetation disturbance associated with changes in ciénega hydrology) to identify possible synoptic and dynamic patterns that may have caused past extremes (Edwards et al., 2001; Shinker, 2014). Such conceptual models of dynamic processes can provide examples of modern climatic mechanisms as analogs of historic and past hydroclimatic variability (Mock and Brunelle-Daines, 1999; Shinker et al., 2006; Mock and Shinker, 2013; Shinker, 2014).
The first step in the modern climate analog approach is to identify conditions in the sedimentological proxy data that record a change in vegetation and subsequently represents changes in the cienega system, which would have signaled a change in the hydrological cycle. The next step is to use an environment-to-circulation approach (Yarnal, 1993; Barry and Carleton, 2001; Yarnal et al., 2001; Mock and Shinker, 2013; Shinker, 2014), which considers the surface conditions based on information gained from the proxy data collected from cienega sediments. We use the Multivariate ENSO Index (MEI, Wolter and Timlin, 2011) to identify analogous periods (seasons, years) of anomalous conditions and treat the selected cases as the modern analog for past hydrologic variability seen in the sedimentary pollen record. By identifying extremes (i.e., El Niño conditions) in the modern record, the environment-to-circulation approach helps identify potential dominant synoptic processes that created the persistent conditions leading to the ecological changes seen in the sedimentary record.
We use five strong El Niño events from the MEI (Wolter and Timlin, 2011) for the period 1979–2017 (the common period for the atmospheric circulation data we use). The selected El Niño years (cases) represent similar conditions (e.g., El Niño modes consistent with anomalous wet winter and spring conditions in the southwest) to those found in the sedimentary analyses. Once selected, the case years are used to calculate and map composite-anomaly values to assess the spatial configuration of climate processes during the selected anomalous case years (the modern climate analogs). We use climate data from the North American Regional Reanalysis (NARR) dataset (Mesinger et al., 2006), which spans 1979–2017. Use of the NARR dataset is advantageous for two reasons: (1) it provides a variety of climate variables that represent atmospheric synoptic processes (e.g., atmospheric pressure; moisture availability, and vertical motion) as well as surface conditions (e.g., precipitation rate and soil moisture); and (2) The spatial resolution (32-km grids) of the NARR is at a finer scale than large-scale GCMs. The 32-km resolution of the NARR is valuable for our area of geographic study because it captures topographic and climatic diversity of the southwest (Heyer et al., 2017). For each climate variable in the NARR dataset we use, the seasonal value (e.g., DJF) of the selected modern analog case years are averaged together (composited) and compared to the long-term mean (1981–2010) to create composite-anomaly values.
Finally, we map composite-anomaly values for selected modern climate analog case years to analyze and assess the spatial and temporal variability of our selected modern climate analogs for surface and atmospheric conditions that would support ecological change identified in the paleoecological record. Atmospheric variables (e.g., atmospheric pressure and specific humidity) are mapped at a continental scale to illustrate the large spatial scales in which such variables operate. Similarly, the surface variables (e.g., precipitation rate and soil moisture) are mapped at the local or regional level to illustrate the spatial heterogeneity of such processes.
Results
Core Description
The sedimentary deposits were massive, organic rich clays with interbedded layers of silt and sand.
Age Models
San Bernardino Ciénega
The San Bernardino age model is presented in Minckley and Brunelle (2007); Blissett (2010), and Brunelle et al. (2010) and is based on a series of linear regressions using 8 radiocarbon dates.
Cloverdale Ciénega
The Cloverdale age model (Brunelle et al., 2014) is based on a smoothing spline (CLAM spar 0.1, goodness-of-fit = 22.4, Blaauw, 2010) based on the surface age, a lead-210 date and 4 radiocarbon dates (Figure 2).
Canelo Hills Ciénega
The Canelo Hills Ciénega age model is not previously published. It is based on a smoothing spline (CLAM spar 0.3, goodness-of-fit = 1.99, Blaauw, 2010) based on the surface age, and 3 radiocarbon dates (Figure 2).
Babocamari Ciénega
The Babocamari Ciénega age model is not previously published. It is based on a smoothing spline (CLAM spar 0.2, goodness-of-fit = 4.25, Blaauw, 2010) based on the surface age, and 3 radiocarbon dates (Figure 2).
Pollen
San Bernardino Ciénega
The pollen data represent changes in abundances of various taxa over the last ~8,000 cal yr BP (Figure 3). The Lycopodium curve indicates depths that have greater or lesser preservation. For example, the time period centered on 2,750 cal yr BP records a greater abundance of identified Lycopodium than pollen grains and reduced pollen preservation. Other interesting shifts in pollen types include the increase in Poaceae, Cyperaceae, and Typhaceae/Sparganium type after ~4,500 cal yr BP.
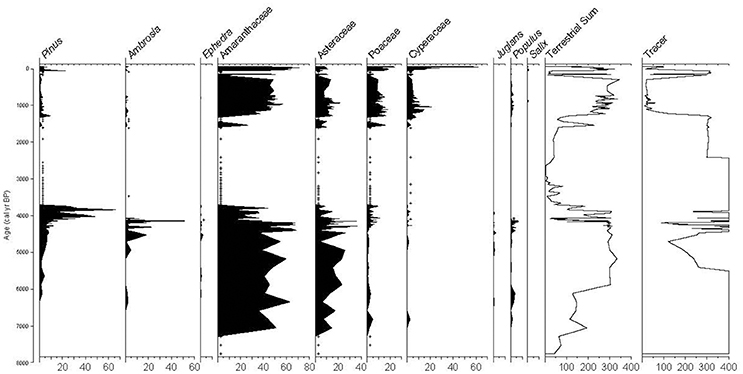
Figure 3. San Bernardino pollen diagram. “+” symbol indicates counts of <100 terrestrial grains. Counts <100 are plotted as presence or absence to avoid over interpretation of sparse data.
Cloverdale Ciénega
As with the SBNWR site, the amount of Lycopodium represents the preservation of the pollen grains for the last ~5,500 cal yr BP (Figure 4). At this site preservation is excellent from the beginning of the record until about 500 years ago. Other distinctive changes in the pollen include a decline and then subsequent increase in Cyperaceae centered on ~3,500 cal yr BP.
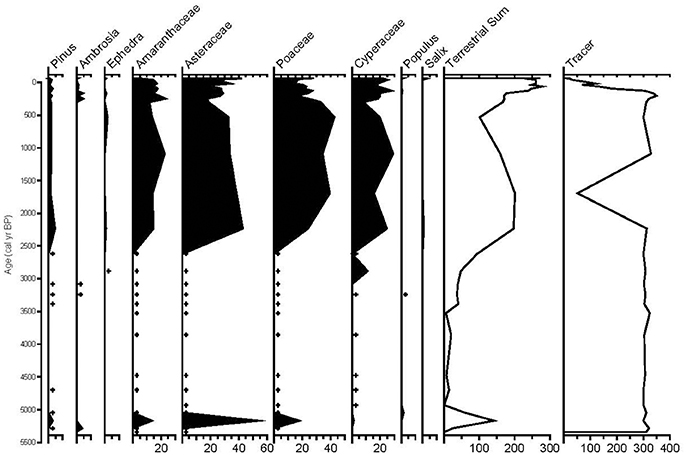
Figure 4. Cloverdale pollen diagram. “+” symbol indicates counts of <100 terrestrial grains. Counts <100 are plotted as presence or absence to avoid over interpretation of sparse data.
Canelo Hills Ciénega
The Canelo Hills record spans the last ~3,000 cal yr BP. Increased preservation as indicated by a decrease in Lycopodium improves from the beginning of the record toward present (Figure 5). Cyperaceae and grasses also increase toward present. Taxa extralocal to the ciénega remain relatively constant with slight fluctuations (e.g., Amaranthaceae).
Babocamari Ciénega
The Babocamari record represent the last ~1,800 cal yr BP (Figure 6). Preservation is variable over time as indicated by increases and decreases in Lycopodium. Cyperaceae shows a strong increase toward present starting at ~900 cal yr BP. All other taxa remain relatively constant over the record.
Charcoal
San Bernardino Ciénega
The San Bernardino Ciénega charcoal record is the longest published fire history from the desert southwest (Figure 7). The record spans the last ~8,000 cal yr BP. From ~8,000 to ~5,000 cal yr BP there is essentially no charcoal in the SBNWR record. After ~5,000 cal yr BP the charcoal influx (CHAR) increases to a sustained level from 5,000 to 4,000 cal yr BP. After 4,000 cal yr BP the CHAR stabilizes around a new mean, increasing again around 1,500 cal yr BP.
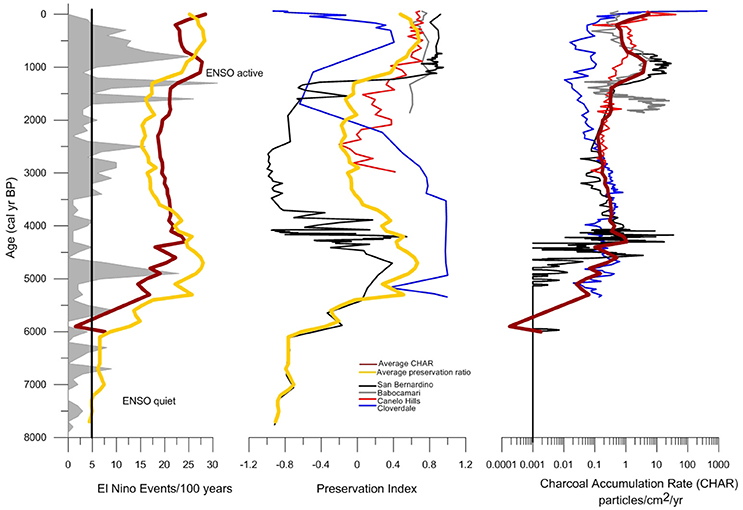
Figure 7. Synthesis figure which includes Moy et al. (2002) El Niño frequency curve, the pollen preservation index and charcoal influx for all four sites. An average of all the sites CHAR and pollen preservation indices are included to show broad trends.
Cloverdale Ciénega
The Cloverdale charcoal record initiates at ~5,000 cal yr BP and remains relatively constant for the entire record (Figure 7). There is a small decrease in CHAR centered on ~1,500 cal yr BP and it increases significantly in the last couple hundred years.
Canelo Ciénega
The Canelo Hills charcoal record represents the last ~3,000 cal yr BP (Figure 7). The CHAR slowly increases from the start of the record to present.
Babocamari Ciénega
The Babocamari record represents the last ~1,800 cal yr BP (Figure 7). The CHAR is relatively stable for the entire period and is of similar magnitude to the Canelo Hills and San Bernardino Ciénega records.
Modern Climate Analog
Our modern climate analog results illustrated in Figure 8 show composite-anomaly values based on the five strongest El Niño events from the Multivariate ENSO index (MEI) (Wolter and Timlin, 1993, 1998) and include 1982/1983; 1986/1987; 1991/1992; 1997/1998; and 2015/2016 compared to the long-term mean (1981–2010). We show winter (DJF) and spring (MAM) composite-anomaly values because these seasons represent the greatest impact of El Niño teleconnections in the region (Heyer et al., 2017). Additionally, winter and spring are important seasons for prolonged and persistent wet conditions that would support preservation of pollen in ciénegas.
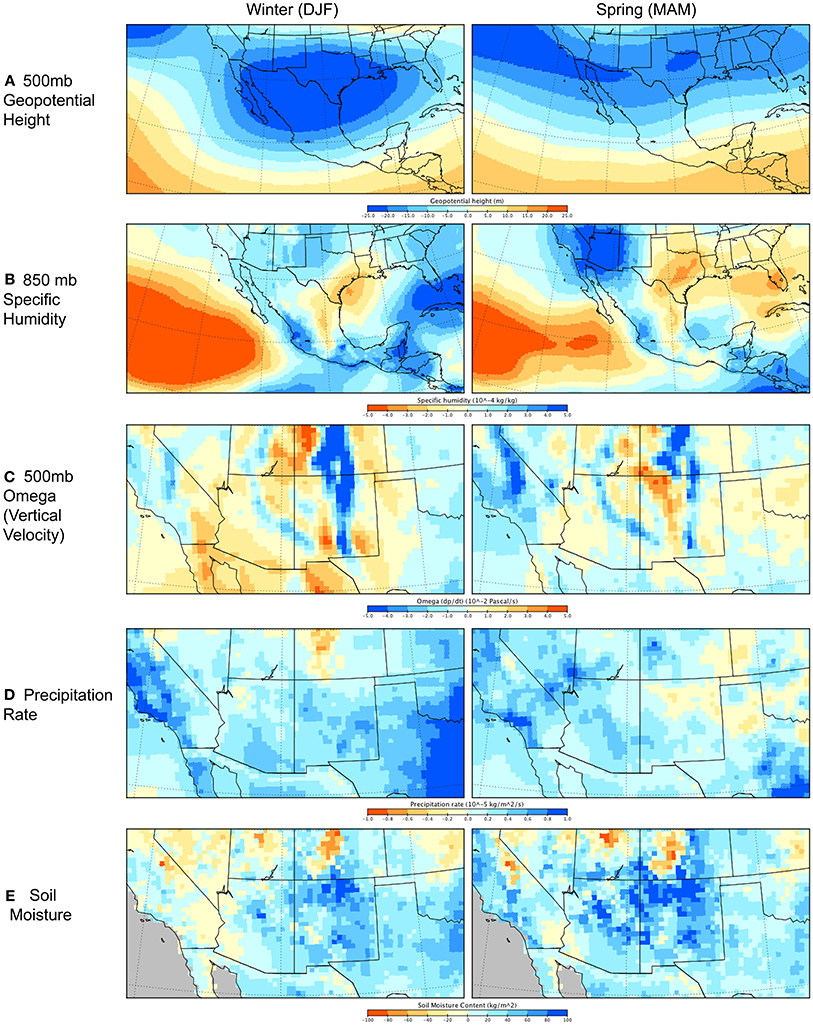
Figure 8. Composite-anomaly maps for strong El Niño events 1982/1983; 1986/1987; 1991/1992; 1997/1998; and 2015/2016. Each map contains composite-anomaly values for winter (DJF) and spring (MAM) from the selected strong El Niño events compared to the long-term mean (1981–2010). (A) 500 mb geopotential height indicating anomalous ridges (red) or troughs (blue); (B) 850 mb specific humidity indicating moisture availability; (C) 500 mb omega (vertical velocity) indicating rising (blue) or sinking (red) motions; (D) Precipitation rate at the surface; and (E) Soil moisture.
During both winter and spring seasons, the southwest region experienced lower-than-normal atmospheric pressure at the 500 mb level (Figure 8A) indicative of persistent low pressure troughs. Such conditions are associated with counterclockwise flow of air around the region that deliver greater-than-normal moisture from the south via 850 mb specific humidity (Figure 8B). While the 500 mb Omega (vertical velocity) composite-anomaly maps (Figure 8C) indicate a mixture of weaker-than-normal (winter) and stronger-than-normal (spring) rising motions these motions are still associated with greater-than-normal moisture availability via specific humidity at the 850mb level. Both winter and spring months exhibit greater-than-normal precipitation values (Figure 8D) in our region of interest indicating persistent wet conditions through multiple seasons. The greater-than-normal precipitation along with lower-than-normal temperatures (not shown) contribute to the persistence of greater-than-normal soil moisture (Figure 8E) during both winter and spring months.
Statistical Relationships
The correlations between El Niño frequency and mean-pollen preservation ratios and mean-charcoal accumulation (CHAR) were compelling. The results show a correlation between El Niño event frequency and CHAR and pollen preservation, however the correlation coefficient values strengthen when a lag in the data is considered. The optimal lag between El Niño frequency and CHAR is at 500 years while the optimal lag between El Niño frequency and pollen preservation ratios is at 300 years.
Discussion
In this paper we present a quantitative approach for the interpretation of arid-land pollen sequences and introduce two new Southwestern desert sites. By merging the traditional summation and proportioning of terrestrial and non-terrestrial pollen, first demonstrated by Vonpost (1916) with a Boolean (presence/absence) and preservation evaluation, interpretations of absence or degradation can be placed in an ecological context. Ciénegas have been shown to be highly sensitive to hydrologic change and might be one of the few terrestrial ecosystems where state-changes have been identified (Scheffer et al., 2001; Scheffer and Carpenter, 2003; Folke et al., 2004; Heffernan, 2008; Minckley et al., 2011, 2013b). We utilize modern climate analogs of strong El Niño years to provide mechanisms for winter and spring moisture delivery into the region that would support ecological conditions of ciénega growth and pollen preservation.
Our interpretive approach allows us to address potential mechanisms driving ciénega development and controls on their growth related to regional climate and land-use (Brunelle et al., 2014). Winter and subsequent spring precipitation appears important to ciénega form and function through recharging groundwater systems that feed ciénega complexes. Without critical inputs of winter and spring precipitation to support a water table emergence at the surface, ciénegas are intermittently dry or nonexistent. Groundwater lags have been shown to have long residence times of decades to centuries (Rodríguez et al., 2005; Wolaver et al., 2013) In the Southwest, winter and spring precipitation is enhanced during El Niño events (Heyer et al., 2017). Scanlon et al. (2006) indicated that periods of frequent El Niño events result in three times as much groundwater recharge in the desert Southwest as compared to periods of frequent La Niña events. Flint et al. (2004) demonstrated with their models that the southern part of the Great Basin gets 220% greater-than-mean recharge during El Niño years. These studies suggest a potential link between ENSO and ciénega state changes. Alternatively, groundwater discharge systems may also respond to local groundwater depletion via extraction in the present day (see Pigati et al., 2014; Sáez et al., 2016).
San Bernardino Ciénega
At San Bernardino Ciénega the pollen preservation ratio is low from the beginning of the record ~8,000 cal yr BP until ~5,000 cal yr BP (Minckley and Brunelle, 2007; Minckley et al., 2009, 2011). This transition in preservation coincides closely with our understanding of the initiation of ENSO active conditions (>5 El Niño events per 100 years; Moy et al., 2002). Prior to the onset of active ENSO, the site was an intermittent wetland based on the sediment characteristics and the presence of some aquatic-type pollen grains such as Cyperaceae (Figure 3). After ~5,000 cal yr BP preservation increases (Figure 7) as does the abundance of wetland pollen taxa (Figure 3). Not only does the pollen preservation ratio track the initiation of ENSO-active conditions at ~5,000 cal yr BP, but it also decreases during the “quiet active” ENSO period from ~4,500 to 2,000 cal yr BP (fewer than 5 El Niño events per 100 years), increasing again with the increase in El Niño activity after ~2,000 cal yr BP.
The fire history at San Bernardino Ciénega also correlates closely with the timing of the initiation of active ENSO conditions (Figure 7). There is essentially no charcoal in the San Bernardino Ciénega record until before 5,000 cal yr BP (Brunelle et al., 2010). Prior to 5,000 cal yr BP, the majority of rainfall in the region would have been from summer monsoons. In the Southwest, increased winter precipitation results in increases in the production of spring flowering annuals, which creates fine herbaceous fuels during the summer (Swetnam and Betancourt, 1990; Bowers, 2005). The production of these fuels creates a fuel continuity which would normally not be present in arid Southwestern desert ecosystems. Prior to the onset of El Niño events, there would have been ignition in the desert with summer convective storms, but the lack of fine fuel associated with enhanced winter precipitation would have prevented fire spread. The absence of charcoal prior to ~5,000 cal yr BP does suggest minimal fire activity prior to that time. In addition to the step-wise increase in charcoal at ~5,000 cal yr BP, there is a second increase in charcoal influx at ~1,500 cal yr BP, coincident with when El Niño activity enters its most active phase (Figure 7). This suggests that once winter precipitation provided the fine fuel source and fuel connectivity, fire activity and charcoal production increased, connecting charcoal records and El Niño activity.
Cloverdale Ciénega
The Cloverdale Ciénega initiates when ENSO enters the active phase ~5,000 cal yr BP (Figure 7). We propose that the increase in groundwater recharge associated with El Niño-enhanced winter and spring precipitation allowed the water table to rise to the point of surface saturation/standing water and the ciénega to form. Scanlon et al. (2006) assert that groundwater recharge in the desert southwest is controlled by the occurrence of wet winters and springs associated with El Niño conditions. The pollen preservation at Cloverdale Ciénega also improves with increased activity of El Niño events (Moy et al., 2002; Figure 7). Preservation decreases slightly with a small decrease in El Niño events ~2,000 cal yr BP, but then preservation increases with the subsequent increase in El Niño events. Wetland taxa like Cyperaceae decrease during the “quiet” El Niño period starting around 4,500 cal yr BP possibly indicating a lowering of the water table (Minckley et al., 2013b; Figure 4). While the pollen preservation signal remains high for the remainder of the record, Cyperaceae percentages drop with the introduction of cattle to the site ~1,600 CE (Brunelle et al., 2014). This extensive use of the region for cattle grazing at this time led to woody plant encroachment and a shift in the vegetation community clearly recorded in the sedimentary pollen record (Brunelle et al., 2014).
The charcoal accumulation rate from Cloverdale Ciénega follows the general trends of El Niño frequency with a slight decline in charcoal accumulation associated with the quieter ENSO period (Figure 7). The charcoal record suggests a fire regime controlled by the presence of fine fuels, which would be enhanced by anomalous winter and spring moisture typical of strong El Niño events in the region (Figure 8, also see Heyer et al., 2017). The large increase in charcoal accumulation in the latest part of the record appears to be a decoupling of the natural fire regime and is likely associated with the encroachment of woody plants at this time (Brunelle et al., 2014). The decrease in complete combustion associated with woody fuels would lead to an increase in the production of charcoal (Marlon et al., 2006).
Canelo Hills Ciénega
The preservation ratio at Canelo Hills increases from the onset of the record at ~3,000 cal yr BP to present. The wetland record from Canelo Hills doesn't initiate until ~3,000 cal yr BP which is when El Niño enters a consistently active phase. The initiation of Canelo Hills is likely related to the winter precipitation recharge of the aquifer which then allowed water at the surface of the ciénega. In addition to an increase of preservation, Cyperaceae grains become increasingly more abundant from about 500 cal yr BP to present, likely indicating the expansion of the cienega site. The charcoal accumulation rate slowly increases from the start of the record to present with dips coincident with decreases in the El Niño frequency curve. This again suggests a connection between El Niño years and fine fuel production and accumulation.
Babocamari Ciénega
Babocamari Ciénega is the youngest ciénega of the four sites, with a ~1,800 cal yr BP record. As with Cloverdale and Canelo Hills, there seems to be a strong connection between the state of ENSO and the initiation of the ciénega. Babocamari initiates after the very highest peaks in El Niño event frequency (Figure 7). There is strong support for the relationship between greater El Niño event frequency and increased groundwater recharge in the Southwestern deserts (e.g., Flint et al., 2004; Scanlon et al., 2006).
Also, like at Canelo Hills, we observe a relatively recent increase in Cyperaceae. At Babocamari the increase begins at about 900 cal yr BP, but then drops from ~ 150 to 50 cal yr BP (1,800–1,900 CE). This decrease is likely associated with EuroAmerican impacts to the landscape (e.g., grazing, water diversion, etc.), however we also see the recovery of the Cyperaceae wetland community in the most recent sample which likely reflects the restoration efforts underway at the ciénega.
Site Synthesis
Continuous records of environmental change reveal how environments respond to past natural perturbations, and provide insight into the trajectory and rate of change environments may experience in the future. Looking for a coherent pattern for the interpretation of desert wetland records is difficult, because unlike temperate lakes which can be produced from glaciation, ciénegas are controlled by local hydrologic conditions (Minckley et al., 2013b). In addition, during periods of disturbance or drought the sediments can be lost (Heffernan, 2008; Heffernan et al., 2008; Minckley et al., 2013b), so we lack a regional context that only multiple sites of similar age can provide. However, there are nested patterns in the data presented here. Wet-dry episodes seen in the longest record, San Bernardino Ciénega, are either repeated in the other records through temporal overlap of ecological histories recorded or the initiation of the younger records. This suggests that there is a regional climate linkage to the development, growth and senescence of these wetlands.
The relationship between fire and ENSO is well recorded in the regional upland forests records of the Southwest (Swetnam and Betancourt, 1990). To assess this relationship from the ciénega records, the average CHAR and pollen preservation ratios were calculated for the 4 sites. These averaged time series were then correlated with the El Niño frequency curve (Moy et al., 2002) to determine if statistically significant relationships exist [e.g., is the frequency of El Niño affecting the fire regime (CHAR) or state (wet vs. dry) of the wetland (as indicated by the pollen preservation ratio)]. When correlations were run on parallel time series (e.g., “no lag”), the correlation 95% confidence interval between El Niño frequency and CHAR was −0.04 to 0.38 with a p-value of 0.10, and the correlation 95% confidence interval between El Niño and pollen preservation was 0.10–0.50 with a p-value of 0.05 (Table 2). These values do suggest that some of the variability in CHAR and pollen preservation are controlled by the frequency of El Niño. However, when one examines the CHAR and pollen preservation curves in comparison with the El Niño frequency curve (Figure 8) a temporal offset is evident. It is ecologically consistent that if there was a period of increased El Niño frequency that the increase in ciénega CHAR might follow with some lag. To better understand ciénega system lags, correlation analyses were performed at 100, 200, 300, 400, and 500 years. The analyses demonstrated that average ciénega CHAR correlates best with El Niño frequency at a lag of 500 years with a correlation 95% confidence interval of 0.26–0.62 and p-value of 0.000026. The same exercise was conducted between El Niño and average ciénega pollen preservation data, with the optimal correlation 95% confidence interval of 0.25–0.61 and p-value of 0.00004 identified with a lag of 300 years (Table 2). Again, given the lag in groundwater recharge and emergence following a period of wet winters and springs (high El Niño frequency), and the lag in the drying of a ciénega system following a series of dry winters (low El Niño frequency), it is not surprising the best fit between the two time series included a several hundred year lag. Also, the fact that we are dealing with multiple (likely imperfectly) age-modeled systems should be considered in the evaluation of the statistical correlations between these time series. The compelling result is the relatively high correlation values given the comparison of climatic forcings and ecological responses.
Another observation using the averaged CHAR data comes from the analysis of the averaged ciénega CHAR with the regional standardized CHAR. A 95% confidence interval of −0.26 to 0.15, and a p-value of 0.57 (Table 2) indicate a lack of relationship between averaged ciénega CHAR and regional standardized CHAR. While ponderosa pine systems demonstrate a relationship with ENSO (Swetnam and Betancourt, 1990), many of the sites in the regional aggregate represent other forest types where fuel availability is less limited than the low elevation ciénega sites. In the American Southwest, upper elevation fire regimes are limited by moisture (low moisture and fire) whereas fire regimes in the deserts are limited by fuel availability (Krawchuk and Moritz, 2011). A Peason's correlation between average ciénega pollen preservation and average ciénega CHAR reveal a 95% confidence interval of 0.22–0.59 and p-value of 0.0001, suggesting fuel availability limited fire at our sites (Table 2). Further, the different controls on fire regime are strongly reflected in the lack of correlation between the average ciénega CHAR and the regional standardized CHAR (Table 2).
von Post (1946) identified the regions of the world where vegetation history was fairly well known. Conspicuously, temperate regions were well represented, while arid regions were largely blank. This map would be fairly similar today. Desert regions have limited depositional contexts where pollen data are preserved and, as such, quantifying past changes requires a degree of creativity. Our approach has been to take the traditional quantitative method as we have learned it and apply it as best we can in these contexts. However, palynology, as is necessarily practiced in arid environments, requires applying ecological knowledge to the variability within pollen counts (i.e., poor pollen preservation has meaning, and plotting percentages is not always appropriate). In doing so we can provide novel interpretations to the continuous history of place that sedimentary records provide us.
In addition to providing the general paleoenvironmental information for the sites presented here, there are four main interpretations that we make from these records. (1) The length of the sedimentary record provides information on groundwater levels (surface saturation via pollen preservation). (2) The pollen preservation index can be used to determine when sediments are continuously vs. intermittently wetted, which we propose as a proxy for winter precipitation. (3) Our evidence of fire history provides an indirect record of fuel connectivity away from the wetlands largely associated with fine fuel production. (4) Finally, our assessment of modern climate analogs during strong El Niño years provides a mechanism for consistent and persistent delivery of moisture into the region during winter and residual effective moisture during spring, which supports an active ciénega state, pollen preservation and production of fine fuels.
Conclusions
In the palynological tradition of Lennart Von Post, this paper utilized pollen data to understand the past environment in a poorly known region. We contribute two new ciénega reconstructions to the existing body of work of the relatively sparse paleoenvironmental record in the desert Southwest. Our objectives were to (1) identify conditions in the sedimentological proxy data (e.g., pollen and charcoal) that record changes in ciénega systems attributed to climate; and (2) to assess the dominant atmospheric drivers related to enhanced winter precipitation in ciénega systems. In addition to providing baseline information on the taxa present and fire regimes, we also detected connections between the frequency of El Niño events and sedimentary proxy.
We identified the pollen preservation index as a means to determine when sediments are continuously vs. intermittently wetted, which in this region is a proxy for winter precipitation. We also determined that the length of the sedimentary record provides information on groundwater levels (surface saturation) and the state and input of winter precipitation as well as persistence of effective moisture into spring months. Finally, fire history from ciénegas may be an indirect record of ENSO through the production of fine fuels. We propose that with continued analysis and the addition of more sites, desert ciénega records may be valuable in filling in the gaps of environmental reconstruction as an independent indicator of past El Niño activity.
By using modern climate analogs of strong El Niño events, we provide context for the teleconnections between such modes of variability and persistent wet conditions within desert Southwest ciénegas. Since pollen preservation in ciénegas is dependent upon wet conditions and winter season precipitation associated with El Niño events that persist into spring in the Southwest, our modern climate analog results provide mechanisms for moisture delivery into the region that would support active ciénegas. This delivery is a function of persistent lower-than-normal pressure and higher-than-normal moisture availability delivered into the region from the subtropics. Our results are specific for the prolonged wetter-than-normal conditions that persist into spring during strong El Niño events. In other words, wetter-than-normal winters alone are not enough to provide moisture availability through subsequent spring months. Thus, analyses of prolonged wet ciénega conditions should consider the impact of persistence associated with multiple season anomalies, such as those that occur during strong El Niño events. Our results suggest that increased El Niño intensity or persistent El Niño events support enhanced and prolonged wet conditions from winter into spring months within ciénega ecosystems. Such persistence of moisture from winter through spring supports both pollen preservation as well as providing conditions conducive for establishment of fine fuels and increased fire activity.
Author Contributions
AB and TM: field collection, lab analysis, data analysis, preliminary manuscript writing, editing, revisions; JS: modern climate analog analysis and writing, editing, revisions; JH: correlation analysis, regional charcoal analysis, writing, editing, revisions.
Funding
Funding for this research was provided by the EPA SCERP program.
Conflict of Interest Statement
The authors declare that the research was conducted in the absence of any commercial or financial relationships that could be construed as a potential conflict of interest.
The handling editor declared a shared affiliation, though no other collaboration, with one of the authors, AB.
Acknowledgements
The authors would like to thank The Nature Conservancy for access to the Canelo Hills Ciénega and the Brophy family for access to the Babocamari Ciénega. We appreciate Dale Turner for his support and facilitation of this research over the years. In addition, we express our gratitude to Shawn Blissett and Jessica Spencer for field assistance and lab analysis of the cores. Thanks to Drs. Alberto Saez and Michael-Shawn Fletcher and the reviewers for their thoughtful suggestions on the manuscript and our editor Jesse Morris for his assistance in the publishing process.
References
Abell, R. A. (2000). Freshwater Ecoregions of North America: A Conservation Assessment. Island Press.
Adams, D. K., and Comrie, A. C. (1997). The North American monsoon. Bull. Am. Meteorol. Soc. 78, 2197–2213. doi: 10.1175/1520-0477(1997)078<2197:TNAM>2.0.CO;2
Antinao, J. L., and McDonald, E. (2013). An enhanced role for the tropical pacific on the humid pleistocene–holocene transition in Southwestern North America. Q. Sci. 78, 319–341, doi: 10.1016/j.quascirev.2013.03.019
Ashok, K., Behera, S. K., Rao, S. A., Weng, H., and Yamagata, T. (2007). El Ni-o Modoki and its possible teleconnection. J. Geophys. Res. 112:C11007. doi: 10.1029/2006JC003798
Baker, M. B. Jr., Ffolliott, P. F., DeBano, L. R., and Neary, D. G. (2004). Riparian Areas of the Southwestern United States: Hydrology, Ecology, and Management. Boca Raton, FL: Lewis.
Barron, J. A., and Anderson, L. (2010). Enhanced Late Holocene ENSO/PDO expression along the margins of the eastern North Pacific. Q. Int. 235, 3–12, doi: 10.1016/j.quaint.2010.02.026
Barry, R. G., and Carleton, A. M. (2001). Synoptic and Dynamic Climatology. London; New York, NY: Routledge.
Betancourt, J. L., Vandevender, T. R., and Martin, P. S. (1990). Packrat Middens: The Last 40,000 Years of Biotic Change. Tucson, AZ: The University of Arizona Press.
Blaauw, M. (2010). Methods and code for “classical”age-modelling of radiocarbon sequences. Q. Geochronol. 5, 512–518. doi: 10.1016/j.quageo.2010.01.002
Blarquez, O., Vannière, B., Marlon J. R., Daniau, A. L., Power, M. J., Brewer, S., et al. (2014). Paleofire: an R package to analyse sedimentary charcoal records from the Global Charcoal Database to reconstruct past biomass burning. Comp. Geosci. 72, 255–261. doi: 10.1016/j.cageo.2014.07.020
Blissett, S. (2010). A Late-Holocene Record of Fire, Vegetation and Climate from a Cienega in Sonora, Mexico. Masters thesis, University of Utah.
Bowers, J. E. (2005). El Ni-o and displays of spring-flowering annuals in the Mojave and Sonoran deserts. J. Torrey Bot. Soc. 132, 38–49. doi: 10.3159/1095-5674(2005)132[38:ENADOS]2.0.CO;2
Brown, D. E. (ed). (1982). Biotic Communities: Southwestern United States and Northwestern Mexico. University of Utah Press.
Brunelle, A., Minckley, T. A., Blissett, S., Cobabe, S. K., and Guzman, B. L. (2010). A nearly 8000 year fire history from an arizona/sonora borderland cienega. J. Arid Environ. 74, 475–481. doi: 10.1016/j.jaridenv.2009.10.006
Brunelle, A., Minckley, T. A., Delgadillo, J., and Blissett, S. (2014). A long-term perspective on woody plant encroachment in the desert southwest, new mexico, U.S.A. J. Vegetat. Sci. 25, 829–838. doi: 10.1111/jvs.12125
Campbell, I. D., and Campbell, C. (1994). Pollen preservation: experimental wet–dry cycles in saline and desalinated sediments. Palynology 18, 5–10. doi: 10.1080/01916122.1994.9989434
Cayan, D. R., Redmond, K. T., and Riddle, L. G. (1999). ENSO and hydrologic extremes in the Western United States. J. Clim. 12, 2881–2893. doi: 10.1175/1520-0442(1999)012<2881:EAHEIT>2.0.CO;2
Clark, J. S. (1988). Particle motion and the theory of stratigraphic charcoal analysis: source area, transportation, deposition, and sampling. Q. Res. 30, 81–91. doi: 10.1016/0033-5894(88)90088-9
Clisby, K. H., and Sears, P. B. (1956). San augustin plains–pleistocene climatic changes. Science 124, 537–539. doi: 10.1126/science.124.3221.537
Cole, A., and Cole, C. (2015). An overview of aridland ciénagas, with proposals for their classification, restoration and preservation. New Mexico Botanist, 28–56.
Cole, J. E., and Cook, E. R. (1998). The changing relationship between ENSO variability and moisture balance in the continental United States. Geophys. Res. Lett. 25, 4529–4532. doi: 10.1029/1998GL900145
Comrie, A. C., and Glenn, E. C. (1998). Principal components-based regionalization of precipitation regimes across the southwest United States and northern Mexico, with an application to monsoon precipitation variability. Clim. Res. 10, 201–215. doi: 10.3354/cr010201
Davis, O. K., Minckley, T., Moutoux, T., Jull, T., and Kalin, B. (2002). The transformation of sonoran desert wetlands following the historic decrease of burning. J. Arid Environ. 50, 393–412. doi: 10.1006/jare.2001.0914
Dettinger, M. D., Cayan, D. R., Diaz, H. F., and Meko, D. M. (1998). North–south precipitation patterns in western North America on interannual-to-decadal timescales. J. Clim. 11, 3095–3111. doi: 10.1175/1520-0442(1998)011<3095:NSPPIW>2.0.CO;2
Dettinger, M. D., Cayan, D. R., Mccabe, G. M., and Marengo, J. A. (2000). “Multiscale stream?ow variability associated with El Ni-o/Southern Oscillation,” in El Ni-o and the Southern Oscillation-Multiscale Variability and Global and Regional Impacts, eds H. F. Diaz, and V. Markgraf (Cambridge University Press), 113–146.
Diem, J. E., and Brown, D. P. (2006). Tropospheric moisture and monsoonal rainfall over the southwestern United States. J. Geophys. Res. 111, 1–12. doi: 10.1029/2005JD006836
Edwards, M. E., Mock, C. J., Finney, B. P., Barber, V. A., and Bartlein, P. J. (2001). Potential analogues for paleoclimatic variations in eastern interior Alaska during the past 14,000 yr: atmospheric circulation controls of regional temperature and moisture responses. Q. Sci. Rev. 20, 189–202. doi: 10.1016/S0277-3791(00)00123-2
Enfield, D. B. (1992). “El nino historical and paleoclimatic aspects of the southern oscillation,” in El Nino: Historical and Paleoclimatic Aspects of the Southern Oscillation, eds H. F. Diaz, and V. Markgraf (Cambridge: Cambridge University Press), 95–117.
Faegri, K., Kaland, P. E., and Kzywinski, K. (1989). Textbook of Pollen Analysis. New York, NY: Wiley.
Flint, A. L., Flint, L. E., and Hevesi, J. A. (2004). “Fundamental concepts of recharge in the desert southwest: a regional modeling perspective,” in Groundwater Recharge in a Desert Environment: The Southwestern United States, eds J. F. Hogan, F. M. Phillips, and B. R. Scanlon (American Geophysical Union Water and Science Application), 9.
Folke, C., Carpenter, S., Walker, B., Scheffer, M., Elmqvist, T., Gunderson, L., and Holling, C. S. (2004). Regime shifts, resilience, and biodiversity in ecosystem management. Ann. Rev. Ecol. Evol. Syst. 35, 557–581. doi: 10.1146/annurev.ecolsys.35.021103.105711
Gershunov, A., and Barnett, T. P. (1998a). Interdecadal modulation of ENSO teleconnections. Bull. Am. Meteorol. Soc. 79, 2715–2725. doi: 10.1175/1520-0477(1998)079<2715:IMOET>2.0.CO;2
Gershunov, A., and Barnett, T. P. (1998b). ENSO influence on intraseasonal extreme rainfall and temperature frequencies in the contiguous united states: observations and model results. J. Clim. 11, 1575–1586. doi: 10.1175/1520-0442(1998)011<3192:EIOIER>2.0.CO;2
Hall, S. A. (1985). “Quaternary pollen analysis and vegetational history of the southwest,” in Pollen Records of Late-Quaternary North American Sediments, eds V. M. Bryant Jr, and R. G. Holloway (American Association of Stratigraphic Palynologists).
Hart, I. A., Broughton, J. M. and R. Gruhn (2015). El Ni-o controls Holocene rabbit and hare populations in Baja California. Q. Res. 84, 46–56. doi: 10.1016/j.yqres.2015.04.005
Hassan et al. (2005). Available online at: http://www.millenniumassessment.org/en/index.html
Heffernan, J. B. (2008). Wetlands as an alternative stable state in desert streams. Ecology 89, 1261–1271. doi: 10.1890/07-0915.1
Heffernan, J. B., Sponseller, R. A., and Fisher, S. G. (2008). Consequences of a biogeomorphic regime shift for the hyporheic zone of a sonoran desert stream. Freshw. Biol. 53, 1954–1968. doi: 10.1111/j.1365-2427.2008.02019.x
Hendrickson, D., and Minckley, W. L. (1985). Ciénegas-vanishing climax communities of the american southwest. Desert Plants 6, 130–176.
Heyer, J. P., Brewer, S. C., and Shinker, J. J. (2017). Using high-resolution reanalysis data to explore localized Western North America hydroclimate relationships with ENSO. J. Clim. 30, 5395–5417. doi: 10.1175/JCLI-D-16-0476.1
Kapp, R. O., Davis, O. K., and King, J. E. (2000). Guide to Pollen and Spores, 2nd Edn. American Association of Stratigraphic Palynologists, 279.
Kirby, M. E., Feakins, S. J., Hiner, C. A., Fantozzi, J., Zimmerman, S. R. H., Dingemans, T., and Mensing, S. A. (2014). Tropical Pacific forcing of Late-Holocene hydrologic variability in the coastal southwestern United States. Q. Sci. Rev. 102, 27–38, doi: 10.1016/j.quascirev.2014.08.005
Krawchuk, M. A., and Moritz, M. A. (2011). Constraints on global fire activity vary across a resource gradient. Ecology 92, 121–132. doi: 10.1890/09-1843.1
Liu, Z., Lu, Z., Wen, X., Otto-Bliesner, B. L., Timmermann, A., and Cobb, K. M. (2014). Evolution and forcing mechanisms of El Ni-o over the past 21,000 years. Nature 515, 550–553, doi: 10.1038/nature13963
Long, C. J., Whitlock, C., Bartlein, P. J., and Millspaugh, S. H. (1998). A 9000-year fire history from the Oregon Coast Range, based on a high-resolution charcoal study. Can. J. For. 28, 774–787. doi: 10.1139/x98-051
Maher, L. J. (1963). Pollen analyses of surface materials from the southern san-juan mountains, colorado. Geol. Soci. Am. Bull. 74, 1485–1503. doi: 10.1130/0016-7606(1963)74[1485:PAOSMF]2.0.CO;2
Manten, A. A. (1967). Lennart von post and the foundation of modern palynology. Rev. Palaeobot. Palynol. 1, 11–22. doi: 10.1016/0034-6667(67)90105-4
Marlon, J., Bartlein, P. J., and Whitlock, C. (2006). Fire-fuel-climate linkages in the northwestern USA during the Holocene. Holocene 16, 1059–1071. doi: 10.1177/0959683606069396
Martin, P. S. (1963). The Last 10,000 Years: A Fossil Pollen Record of the American Southwest. Tucson, AZ: The University of Arizona Press.
Martin, P. S. and Gray, J. (1962). Pollen analysis and the cenozoic. Science 137, 103–111. doi: 10.1126/science.137.3524.103
Mehringer, P. J. (1965). Late pleistocene vegetation in the mohave desert of southern nevada. J. Arizona Acad. Sci. 3, 172–188. doi: 10.2307/40022772
Mehringer, P. J. (1967). “The enviornment of extinction of the late-Pleistocene megafauna,” in The Arid Southwestern United States, eds P. S. Martin and H. E. Wright Jr. (New Haven: Pleistocene Extinctions, Yale Univ. Press), 247–266.
Mensing, S., Smith, J., Burkle Norman, K., and Allan, M. (2008). Extended drought in the great basin of western north america in the last two millennia reconstructed from pollen records. Q. Int. 188, 79–89. doi: 10.1016/j.quaint.2007.06.009
Mesinger, F., DiMego, G., Kalnay, E., Mitchell, K., Shafran, P. C., Ebisuzaki, W., et al. (2006). North American regional analysis. Bull. Am. Meteorol. Soc. 87, 343–360. doi: 10.1175/BAMS-87-3-343
Meyer, E. R. (1973). Late-quaternary paleoecology of cuatro cienegas basin, coahuila, mexico. Ecology 54, 983–995. doi: 10.2307/1935565
Minckley, T. A., and Brunelle, A. (2007). Paleohydrology and growth of a desert cienega. J. Arid Environ. 69, 420–431. doi: 10.1016/j.jaridenv.2006.10.014
Minckley, T. A., Bartlein, P. J., and Shinker, J. J. (2004). “Paleoecological response to climate change in the Great Basin since the Last Glacial Maximum,” in Early and Middle Holocene Archaeology of the Northern Great Basin, eds D. L. Jenkins, T. J. Connolly, and C. M. Aikens (University of Oregon Anthropological Papers), 21–30.
Minckley, T. A., Brunelle, A., and Blissett, S. (2011). Holocene sedimentary and environmental history of an in-channel wetland along the ecotone of the sonoran and chihuahuan desert grasslands. Q. Int. 235, 40–47. doi: 10.1016/j.quaint.2010.06.031
Minckley, T. A., Brunelle, A., and Turner, D. (2013a). “A paleoenvironmental framework for understanding the development, stability and state-changes of ciénegas in the american deserts,” in Merging science and management in a rapidly changing world: biodiversity and management of the Madrean Archipelago III, 2012 May 1-5, eds G. J. Gottfried, P. F. Ffolliott, B. S. Gebow, and L. G. Eskew (Fort Collins Colorado, CO; Tucson, AZ: U.S. Department of Agriculture, Forest Service, Rocky Mountain Research Station), 77–83.
Minckley, T. A., Clementz, M., Brunelle, A., and Klopfenstein, G. A. (2009). Isotopic analysis of wetland development in the american southwest. Holocene 19, 737–744. doi: 10.1177/0959683609105297
Minckley, T., Turner, D., and Weinstein, S. (2013b). The relevance of wetland conservation in arid regions: a re-examination of vanishing communities in the american southwest. J. Arid Environ. 88, 213–221. doi: 10.1016/j.jaridenv.2012.09.001
Minckley, W. L. (1969). Environments of the Bolson of Cuatro Cienegas, Coahuila, Mexico. Texas Western Press; The University of Texas at El Paso.
Minckley, W. L. (1992). Three Decades near Cuatro Ciénegas, México: photographic documentation and a plea for area conservation. J. Arizona-Nevada Acad. Sci. 26, 89–118.
Minckley, T. A., Whitlock, C., and Bartlein, P. J. (2007). Vegetation, fire, and climate history of the northwestern Great Basin during the last 14,000 years, Q. Sci. Rev. 26, 2167–2184. doi: 10.1016/j.quascirev.2007.04.009.
Mock, C. J. (1996). Climatic controls and spatial variations of precipitation in the western United States. J. Clim. 9, 1111–1125. doi: 10.1175/1520-0442(1996)009<1111:CCASVO>2.0.CO;2
Mock, C. J., and Brunelle-Daines, A. R. (1999). A modern analog of western United States summer palaeoclimate 6,000 years before present. Holocene 9, 541–545. doi: 10.1191/095968399668724603
Mock, C. J., and Shinker, J. J. (2013). “Modern analog approaches in paleoclimatology,” in The Encyclopedia of Quaternary Science, Vol. 3, ed S. A. Elias (London: Elsevier), 102–112.
Moy, C. M., Seltzer, G. O., Rodbell, D. T., and Anderson, D. M. (2002). Variability of el nino/southern oscillation activity at millennial timescales during the holocene epoch. Nature, 420, 162–165. doi: 10.1038/nature01194
Pigati, J. S., Rech, J. A., Quade, J., and Bright, J. (2014), Desert wetlands in the geologic record. Earth Sci. Rev. 132, 67–81. doi: 10.1016/j.earscirev.2014.02.001
Quade, J., Rech, J. A., Betancourt, J. L., Latorre, C., Quade, B., Rylander, K. A., and Fisher, T (2008). Paleowetlands and regional climate change in the central Atacama Desert, northern Chile. Q. Res. 69, 343–360. doi: 10.1016/j.yqres.2008.01.003
Rodríguez, A. A. A., Mijares, F. J. A., Ojeda, C. G., Morales, M. M., Hita, L. G., Zamarrón, G. H., et al. (2005). Estudio Hidrogeológico de los Acuíferos el Hundido y Cuatrociénegas. Secretaría de Medio Ambiente y Recursos Naturales, Instituto Mexicano de Tecnología del Agua, Comisión Nacional del Agua, Instituto Nacional de Ecología.
Ropelewski, C. F., and Halpert, M. S. (1986). North American precipitation and temperature patterns associated with the El Ni-o/ Southern Oscillation (ENSO). Month. Weat. Rev. 114, 2352–2362. doi: 10.1175/1520-0493(1986)114<2352:NAPATP>2.0.CO;2
Sabo, J. L., Sponseller, R., Dixon, M., Gade, K., Harms, T., Heffernan, J., and Welter, J. (2005). Riparian zones increase regional species richness by harboring different, not more, species. Ecology 86, 56–62. doi: 10.1890/04-0668
Sáez, A., Godfrey, L. V., Herrera, C., Chong, G., and Pueyo, J. J. (2016). Timing of wet episodes in Atacama Desert over the last 15 ka. The Groundwater Discharge Deposits (GWD) from Domeyko Range at 25°S. Q. Sci. Rev. 145, 82–93. doi: 10.1016/j.quascirev.2016.05.036
Scanlon, B. R., Keese, K. E., Flint, A. L., Flint, L. E., Gaye, C. B., Edmunds, M., et al. (2006). Global synthesis of groundwater recharge in semiarid and arid regions. Hydrol. Process. 20, 3335–3370. doi: 10.1002/hyp.6335
Scheffer, M., and Carpenter, S. R. (2003). Catastrophic regime shifts in ecosystems: linking theory to observation. Trends Ecol. Evol. 18, 648–656. doi: 10.1016/j.tree.2003.09.002
Scheffer, M., Carpenter, S., Foley, J. A., Folke, C., and Walker, B. (2001). Catastrophic shifts in ecosystems. Nature 413, 591–596. doi: 10.1038/35098000
Sheppard, P. R., Comrie, A. C., Packin, G. D., Angersbach, K., and Hughes, M. K. (2002). The climate of the US Southwest. Clim. Res. 21, 219-−238. doi: 10.3354/cr021219
Shinker, J. J. (2010). Visualizing spatial heterogeneity of western U.S. Clim. Variab. Earth Interact. 14, 1–15. doi: 10.1175/2010EI323.1
Shinker, J. J. (2014). Climatic controls of hydrologic extremes in south-interior intermountain west of Colorado, U.S.A. Rocky Mount. Geol. 49, 51–60. doi: 10.2113/gsrocky.49.1.51
Shinker, J. J., and Bartlein, P. J. (2009). Visualizing the large-scale patterns of ENSO-related climate anomalies in North America. Earth Interactions 13, 1–50. doi: 10.1175/2008EI244.1
Shinker, J. J., and Bartlein, P. J. (2010). Spatial variations of effective moisture in the western United States. J. Geophys. Res. Lett. 37:L02701. doi: 10.1029/2009GL041387
Shinker, J. J., Bartlein, P. J., and Shuman, B. (2006). Synoptic and dynamic climate controls of North American mid-continental aridity. Q. Sci. Rev. 25, 1401–1417. doi: 10.1016/j.quascirev.2005.12.012
Spaulding, W. G. (1991). Pluvial climatic episodes in North America and North Africa: types andcorrelation with global climate. Palaeogeogr. Palaeoclimatol. Palaeoecol. 84, 217–227. doi: 10.1016/0031-0182(91)90045-S
Swetnam, T. W., and Betancourt, J. L. (1990). Fire - southern oscillation relations in the southwestern united states. Science 249, 1017–1020. doi: 10.1126/science.249.4972.1017
Tipping, R. (2000). “Pollen preservation analysis as a necessity in Holocene palynology,” in Taphonomy and Interpretation: Symposia of the Association of Environmental Archaeologists, Vol. 14, Oxbow Books, J. P. Huntley and S. Stallibrass (Oxford), 23–33.
Tweddle, J. C., and Edwards, K. J. (2010). POllen preservation zones as an interpretative tool in Holocene palynology. Rev. Palaeobot. Palynol. 161, 56–76. doi: 10.1016/j.revpalbo.2010.03.004
Vonpost, L. (1916). Forest tree pollen in south swedish peat bog deposits, lecture to the 16th convention of scandinavian naturalists, kristiana (oslo). Pollen et Spores 9, 375–401.
von Post, L. (1946). The prospect for pollen analysis in the study of the earth's climatic history. New Phytol. 45, 193–217. doi: 10.1111/j.1469-8137.1946.tb05056.x
Wallace, J. M., and Gutzler, D. S. (1981). Teleconnections in the geopotential height field during the northern hemisphere winter. Month. Weat. Rev. 109, 784–812. doi: 10.1175/1520-0493(1981)109<784:TITGHF>2.0.CO;2
Western Regional Climate Center, Monthly Climate Summary Data (2017). Available online at: http://wrcc.dri.edu/climatedata/climsum/ (Accessed October 24, 2017).
Wise, E. K. (2010). Spatiotemporal variability of the precipitation dipole transition zone in the northwestern United States. Geophys. Res. Lett. 37:L07706. doi: 10.1029/2009GL042193.
Wolaver, B. D., Crossey, L. J., Karlstrom, K. E., Banner, J. L., Cardenas, M. B., GutiérrezOjeda, C., et al. (2013). Identifying origins of pathways for spring waters in a semiarid basin using He, Sr, and C isotopes: Cuatrociénegas Basin, Mexico. Geosphere 9, 113–125. doi: 10.1130/GES00849.1
Wolter, K., and M. S., Timlin (1993). “Monitoring ENSO in COADS with a seasonally adjusted principal component index,” in Proceedings of the 17th climate diagnostics workshop (Norman, OK, NOAA/NMC/CAC, NSSL, Oklahoma Clim. Survey, CIMMS and the School of Meteor, University of Oklahoma), 52–57.
Wolter, K., and M. S., Timlin (1998). Measuring the strength of ENSO events - how does 1997/98 rank? Weather 53, 315–324. doi: 10.1002/j.1477-8696.1998.tb06408.x
Wolter, K., and Timlin, M. S. (2011). El Ni-o/Southern Oscillation behaviour since 1871 as diagnosed in an extended multivariate ENSO index (MEI.ext). Int. J. Climatol. 31, 1074–1087. doi: 10.1002/joc.2336
Yarnal, B. (1993). Synoptic Climatology in Environmental Analysis: A Primer. London: Belhaven Press, 195.
Keywords: pollen, charcoal, ciénega, desert wetland, ENSO, El Niño
Citation: Brunelle A, Minckley TA, Shinker JJ and Heyer J (2018) Filling a Geographical Gap: New Paleoecological Reconstructions From the Desert Southwest, USA. Front. Earth Sci. 6:106. doi: 10.3389/feart.2018.00106
Received: 12 December 2017; Accepted: 05 July 2018;
Published: 31 July 2018.
Edited by:
Jesse L. Morris, Weber State University, United StatesReviewed by:
Michael-Shawn Fletcher, University of Melbourne, AustraliaAlberto Saez, University of Barcelona, Spain
Copyright © 2018 Brunelle, Minckley, Shinker and Heyer. This is an open-access article distributed under the terms of the Creative Commons Attribution License (CC BY). The use, distribution or reproduction in other forums is permitted, provided the original author(s) and the copyright owner(s) are credited and that the original publication in this journal is cited, in accordance with accepted academic practice. No use, distribution or reproduction is permitted which does not comply with these terms.
*Correspondence: Andrea Brunelle, YW5kcmVhLmJydW5lbGxlQGdlb2cudXRhaC5lZHU=