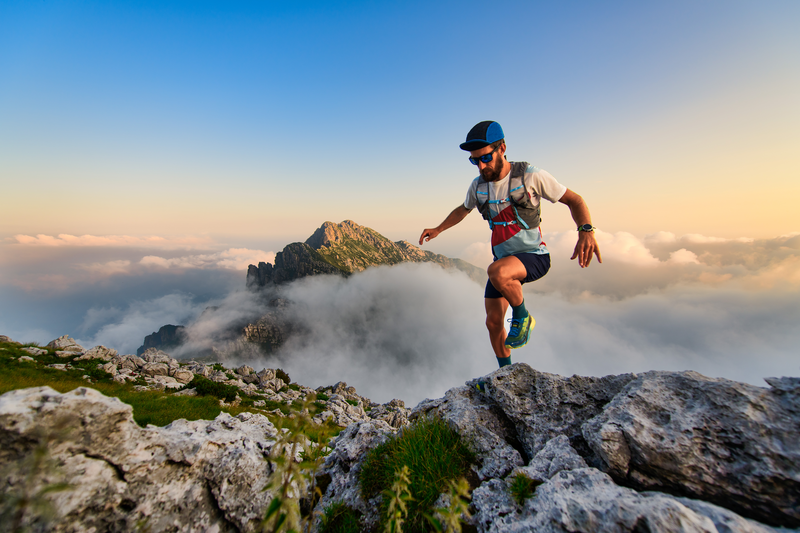
94% of researchers rate our articles as excellent or good
Learn more about the work of our research integrity team to safeguard the quality of each article we publish.
Find out more
ORIGINAL RESEARCH article
Front. Earth Sci. , 07 June 2018
Sec. Biogeoscience
Volume 6 - 2018 | https://doi.org/10.3389/feart.2018.00070
To establish the environmental status of Rufiji coastal waters in Tanzania, it is necessary to document the different contaminants as major entry points into the lower estuarine areas. Because there is no data on polycyclic aromatic hydrocarbon (PAH) contamination in this estuarine delta, the current study measured the concentrations of 19 PAHs in suspended particulate matter (SPM), surface sediments and marine organisms that are part of a telescoping food chain (gastropod Terebralia sp., clams Crassostrea cucullata, crabs Uca sp., panaeid shrimps Panaeus monodon, teleost Hilsa kelee, Trichiurus lepturus, and Arius thalassinus). Total PAH concentrations in SPM were low to moderate (18.7–223 ng/g) and varied between the sites; phenanthrene and chrysene were the dominant PAHs (2.40–47.2 and 4.20–28.1 ng/g, respectively). Significant variation between the sites indicates the influence of fuel spills and contribution from terrestrial sources resulting from different land use practices, such as agriculture, fishing, and harvesting firewood, charcoal, and mangroves poles. PAH concentrations in surface sediments were higher (127–376 ng/g) than SPM samples, and high molecular weight PAHs were the dominant fraction. Animal tissues indicated low PAH levels (9.20–158 ng/g). Only low molecular weight PAHs were dominant in the muscle tissues of pelagic and filter feeders (C. cucullata, P. monodon, and H. kelee). Bioaccumulation factor (BAF) ranged between 0.20 and 69.5 and it suggests 1) PAH accumulation in the marine organisms has so far been limited, and 2) distribution of PAHs in the Rufiji estuary poses limited risks.
Estuarine areas are of substantial ecological, environmental, and economic value to the coastal and inland communities. Freshwater and sediment inputs from fluvial systems draining the upper reaches of the catchment play an important role in defining the physical and chemical characteristics in estuarine areas. The different habitats and high rates of primary production in estuaries allow them to be home to a diversity of plant and animal life that support fishing, aquaculture, tourism and recreation (Sørensen, 1998; Elliott and Whitfield, 2011). These activities constitute the main sources of livelihood and income to the local communities. Estuaries are also a sink for fine-grain sediments and contaminants, and thereby potentially serve as secondary sources of organic and inorganic pollutants in marine environments (Elliott and Whitfield, 2011; Ranjan et al., 2012). Climate which plays an important role in precipitation, flooding events, and driving various human activities is an important driver of both natural and anthropogenic changes in estuaries. These changes for example influence the distribution of various organic and inorganic pollutants that impact the flora and fauna in these sensitive and dynamic ecosystems.
Polycyclic aromatic hydrocarbons (PAHs) are organic pollutants that are persistent and ubiquitously distributed in the environment. These compounds can be easily traced in air, water, soil, sediments, plants, animals, and human beings. Their presence in the aquatic environment is closely related to anthropogenic sources (Soclo et al., 2000). The distribution and fate of PAHs in aquatic ecosystems are influenced by numerous factors such as salinity, pH, suspended particulate matter (SPM) load, tidal currents and seasonal variability (McVeety and Hites, 1988; Countway et al., 2003). In general, PAHs are mostly of anthropogenic origin (Yong and Cerniglia, 1995; Harvey, 1996; Yunker et al., 2002) except perylene, which forms naturally during sediment diagenesis (Silliman et al., 1999). Environmental distribution of PAHs is mostly associated with anthropogenic activities such as fossil fuel combustion, waste incineration, coal gasification, liquefaction processes, petroleum cracking, and production of coke, black carbon, coal tar, pitch and asphalt (Yunker et al., 2002). These compounds are released into the aquatic environment through industrial wastewater, sewage, runoff from roads, street dust, oil spills and vehicular/maritime traffic (Bouloubassi and Saliot, 1993; Soclo et al., 2000; Yunker et al., 2002; Ranjan et al., 2012).
PAHs are very hazardous compounds. They are carcinogenic and mutagenic to both terrestrial and aquatic organisms (Yang and Silverman, 1988; Phillips and Grover, 1994; Denissenko et al., 1996; Yunker et al., 2002). The low molecular weight (LMW) PAHs (i.e., two or three ring compounds) are highly toxic for algal growth (Pulich et al., 1974). Therefore, it is important to understanding the processes which govern the behavior of these contaminants in aquatic systems to establish their potential toxic effects on water and biota. Previous studies have indicated that the pathways and fate of PAHs were largely determined by the behavior and dynamics of particulate matter in estuarine systems (McGroddy and Farrington, 1995; McGroddy et al., 1996; Countway et al., 2003; Shilla, 2016). A notable exception is the behavior of LMW PAHs, which are more susceptible to microbial degradation and volatilization (Readman et al., 1982; van Zoest and van Eck, 1990).
Several studies have been carried out along the Tanzanian coast, which include pristine mangrove stands in river mouths, estuaries, and sheltered bays. This dynamic ecosystem is very sensitive to the regional climate, flooding events, hydrological changes, and human interactions in both the upstream and downstream riparian areas of the Rufiji river system. Previous studies in the Rufiji ecosystem indicate high concentrations of pesticides, metals and combustion related contaminants (Mwevura et al., 2002; Mremi and Machiwa, 2003; Gaspare et al., 2009). However, there is no data on PAH distribution in the Rufiji estuary located 100 km south of Dar es Salaam. Although at present the Rufiji estuary and its delta can be considered as one of the less polluted coastal areas in Tanzania, the presence of small scale industries, agricultural activities, commercial fishing and other socioeconomic activities associated with fossil fuel combustion and its discharge suggest that PAHs might also be released in significant amounts to this coastal environment.
The present study provides the first overview on PAH composition, distribution, and its sources in the Rufiji estuary. The study establishes PAH levels in suspended particulate matter (SPM), surface sediments, invertebrates and fish with different feeding modes (i.e., benthic, pelagic and filter feeders). These organisms are part of a telescoping food chain (gastropod Terebralia sp., clams Crassostrea cucullata, crabs Uca sp., panaeid shrimps Panaeus monodon, teleost Hilsa kelee, Trichiurus lepturus, and Arius thalassinus). Since, fish and some invertebrates collected for this study include those routinely consumed by the local population, PAH bioaccumulation and the likelihood of health risks posed to humans because of biomagnification will also be investigated. This approach will possibly contribute to the identification of potential biomonitors and provide helpful information to support major management choices in the ecologically sensitive Rufiji estuary.
The study sites are in the Rufiji estuary, in Rufiji River delta (Figure 1). Rufiji river is one of the largest rivers in East Africa and drains ca. 20% of the mainland in Tanzania (Erftemeijer and Hamerlynck, 2005). The Rufiji delta complex has a catchment area of 177,400 km2 and is over 640 km long (UNEP, 2001). The lower Rufiji River branches out into a series of channels and forms a large delta covering ca. 1,200 km2; nearly 530 km2 of the delta is covered by mangrove forests (Sørensen, 1998). It is by far, the largest delta in east Africa, and contains the largest continuous block of mangrove forests on the eastern seaboard of the African continent. The Rufiji estuary is an integral part of the largely saline swamps, tidal marshes, woodlands and mangrove forests that dominates eastern Tanzania. The four northern tributaries carry the bulk discharge of freshwater and sediments into the Rufiji River. The southern part of the delta, which is sheltered, receives little freshwater, and the water in front of this part of the delta is clear and saline, and feature seagrass meadows and scattered reefs. Tidal influence in the delta is considerable (REMP., 2003). The upstream section of the Rufiji River is dotted with small towns that depend on agriculture and small industries as means of livelihood for the local population. The downstream sections are mostly free of industries, but within the delta, mangrove sections have partially been cleared for cultivation of rice (Taylor et al., 2003).
Figure 1. Map of study site in the Rufiji estuary, Tanzania. Three sampling locations are indicated in Muhoro stream (site 1), Msomeni (site 2), and Kalale stream (site 3).
The present study was carried out in three locations (Muhoro – site 1, Msomeni – site 2, and Kalale – site 3), representing the south, middle and northern parts of the Rufiji delta, respectively (Figure 1). These locations were chosen because they are representative of the entire estuary, and include a variety of habitats (e.g., mangrove stands, mud flats, and seagrass meadows) with sufficient diversity and densities of telescoping consumer organisms.
Fauna, sediment and water samples were collected from three locations at the Rufiji estuary in June and July 2014 as part of a reconnaissance survey. Enough material was collected for three replicate analyses. A range of animals, including gastropods (Terebralia sp.), clams (Crassostrea cucullata), and crabs (Uca sp.), were collected from all three locations; Panaeid shrimps (Panaeus monodon) were collected from sites 1 and 3. The animals under investigation are the dominant species at respective stations in the Rufiji delta (REMP., 2003). Ten to twenty individual gastropods, bivalves, crabs and shrimps were dissected to remove their muscle tissues and used for preparing the dry tissue pool of individual animals. Dissected tissues were frozen (20°C) and stored for further analysis.
Teleost (Trichiurus lepturus and Arius thalassinus) were collected from sites 2 and 3, whereas Hilsa kelee were collected from sites 1 and 3, where they were found in sufficient numbers. Six individuals of each species of fish were randomly selected from the total catch, and they were euthanized in an ice slurry. Muscle tissues (skin removed) were collected from both sides of each fish, for biochemical analyses within 24 h of sample collection. All samples were frozen (−20°C) until further analysis and conducted within 3 months of sample collection.
The sediment samples were obtained by scraping the top 1–2 cm off the surface sediment at each location. Water samples (4 L) were collected from each sampling site and were transported back to the laboratory for filtration. Filtration was performed through pre-combusted (4 h at 450°C) glass fiber filters (Whatman GF/F, 0.7 μm). Each replicate had three filter papers which were stored at −20°C until further extraction and conducted within 3 months of sampling.
For lipid extraction, 10 g dry weight (dw) of sediments, 3 g dw of filtrates, and between 3 and 5 g dw of animal tissues, including three replicates for each type of sample at each location were performed, using the modified extraction protocol in marine particles and sediment samples (Wakeham et al., 2002, 2006; Routh et al., 2014). The samples were freeze-dried in batches and homogenized; their dry weight was estimated by weighing the samples before and after freeze-drying. Samples were extracted with a mixture of CH2Cl2 and CH3OH (9:1 v/v ratio) in an automated solvent extractor (Dionex ASE 300; 3 cycles at 1,500 psi and 100°C). 50 mg/l n-hexatriacontane—d50 was used as a recovery standard and added prior to extraction. A Büchi Syncore SPE extractor was used to reduce the extracts to ca. 0.5 ml in volume; later these extracts were reduced to dryness under a gentle stream of N2. The total lipid extract (TLE) was separated into two fractions using 6 ml glass columns packed with 500 mg of Supelco Superclean LC-NH2 (Kim and Salem, 1990). The columns were eluted with CHCl3/isopropanol (15 ml of 2:1 v/v, neutral fraction) and 2% acetic acid in diethyl ether (15 ml; acid fraction). The neutral fraction was separated into alkane and PAH fractions using the low bleed bilayer cartridges (Agilent Bilayer cartridge, Alumina-silica gel column) with hexane (5 ml, alkanes fraction) and hexane/dichloromethane (1:1 v/v, 5 ml, for PAH fraction). PAH fraction was concentrated to about 3 ml on a Büchi Syncore SPE, and further concentrated to 150 μl under N2 after the addition of an internal standard (d-12 phenanthrene, d-12 chrysene, and d-12 perylene).
PAHs were quantified relative to the deuterated surrogate PAHs using an Agilent 6890N GC interfaced to an Agilent 5973 MSD mass spectrometer. The mass spectrometer operated at 70 eV scanning from m/z 40–600 (at 2.62 scans/s); the run-time was 68.42 min. The extracts were injected in splitless mode (10 μl; inlet pressure of 10 psi with a flow rate 54.3 ml/min) and separated on a HP-5 MS capillary column (5% diphenyl dimethyl polysiloxane; 30 m length × 0.25 mm i.d. × 0.25 μm film thickness). The samples were run at constant flow (1.3 ml/min) with helium as carrier gas. The interface temperature was set at 300°C, the source temperature at 230°C and the MS quadrupole was maintained at 150°C, respectively. The samples were injected at 35°C and the oven was programmed to 130°C at 20°C/min and then to 320°C at 6°C/min, where it was held isothermally for 15 min. Based on the retention time and mass spectra of different compounds, the PAHs were identified by comparing our results with the published literature (Peters et al., 2005; Zhao et al., 2014) and online libraries (Lipid library, 2011). Nineteen PAHs were analyzed: naphthalene (Na), acenaphthylene (Ac), acenaphthene (Ace), fluorene (Flu), anthracene (An), phenanthrene (Ph), fluoranthene (Fln), pyrene (Pyr), benzo[a]anthracene (BaA), chrysene (Chr), benzo[a]pyrene (BaP), benzo[e]pyrene (BeP), benzo[b]fluoranthene (BbF), benzo[j]fluoranthene (BjF), benzo[k]fluoranthene (BkF), benzo[g,h,i]perylene (ghi), Indeno[1,2,3-cd]pyrene (IP), perylene (Per), and dibenzo[a,h]anthracene (DahA).
To ensure that no contaminants were introduced during the extraction and separation procedures, blanks were prepared following the same extraction procedure. Furthermore, some samples were extracted twice under identical conditions (duplicates). No PAHs were detected in the second set of extracts. The surrogate recovery ranged from 89 to 92%. PAH concentrations were not corrected for recovery and expressed on dry weight basis.
Filters that contain particulate organic carbon (POC) were washed with distilled water to eliminate salt and dried for 24 h at 60°C. The dry weight was used to calculate the total amount of suspended solid (TSS). Filters were placed in a desiccator in contact with HCl fumes overnight to decompose inorganic carbon (e.g., CaCO3) and again dried at 60°C. POC quantification was done by using a CHN analyzer (NC-80 Model, Sumika Chemical Analysis, Tokyo, Japan) with a precision of <5%.
Comparison of PAH concentrations between different sites and sample types was made using the software programs ANOVA and Statistica 6.0. Linear regression and Pearson's correlation coefficient test were run using the SPSS Statistics v 20 software, to explore the relationships between PAHs and different environmental variables (POC, TSS, and source specific lipid biomarkers). Cluster analysis and non-metric multi-dimensional scaling (NMDS) (Kruskal and Wish, 1978; Clarke and Gorley, 2006; Anderson et al., 2008) were also run using PRIMER v6 software to obtain the different PAH trends at these sites.
The concentrations of 19 PAHs were analyzed and the sum of all compounds for SPM and estuarine sediments are shown in Table 1. SPM samples had low levels of total PAHs ranging from 18.7 to 224 ng/g. Sampling site 1 showed significantly (p < 0.05) low concentration of total PAHs compared to sites 2 and 3, but there were no significant differences between sites 2 and 3. Phenanthrene was the dominant PAH with concentrations ranging between 2.40 and 47.2 ng/g, followed by chrysene with concentration between 4.20 and 28.1 ng/g. Furthermore, concentrations of LMW PAHs (2 and 3 ring compounds) represented 32.6 to 59.5% of total PAHs. The total concentration of potentially carcinogenic PAHs (cPAH) which include benzo[a]anthracene, chrysene, benzo[b]fluoranthene, benzo[k]fluoranthene, benzo[a]pyrene, indeno[1,2,3-c,d]pyrene, and dibenzo[a,h]anthracene (International Agency for Research on Cancer, 1987) ranged from 5.70 to 59.4 ng/g, with site 3 showing significantly (p < 0.005) higher concentration compared to site 1.
Table 1. Concentration (in ng/g) of 19 individual PAHs, total PAHs, total cPAHs (representing the total concentration of carcinogenic PAHs including BaA, Chr, BbF, BkF, BaP, IP, and DahA), LMW (low molecular weight PAHs with 2 and 3 rings), HMW PAH (high molecular weight PAHs with 4, 5, and 6 rings), % LMW PAHs in suspended particulate matter (SPM) and surface sediments (Sd) from three sites representing the southern, central and northern parts of the Rufiji estuary.
In sediment samples, total PAH concentrations were slightly higher than those in SPM, ranging from 128 to 377 ng/g. Like the SPM samples, site 1 had statistically lower concentrations of total PAH (p < 0.05) than sites 2 and 3. The dominant PAHs were chrysene (20.7–69.4 ng/g), pyrene (11.2–79.9 ng/g), perylene (24.6–69.3 ng/g), and phenanthrene (7.90–45.9 ng/g). The proportion of LMW PAHs represented 9.90–20.8% of total PAH concentration and was significantly (p < 0.05) lower than SPM samples. The concentrations of cPAH in sediments were significantly (p < 0.05) higher (58.3–153 ng/g) than SPM samples.
The concentrations of 19 PAHs and the sum of all PAHs in animal tissue are shown in Table 2. Total PAH concentrations in the animals ranged between 9.20 and 158 ng/g. The lowest concentrations of total PAHs were recorded at site 1 for Terebralia sp. (9.20 ng/g), Panaeus monodon (27.0 ng/g), and Crassostrea cucullata (32.4 ng/g), whereas significantly (p < 0.005) higher concentrations of total PAHs were recorded at site 3 for Terebralia sp. (158 ng/g), Crassostrea cucullata (131 ng/g), and Uca sp. (133 ng/g). Total concentrations of cPAH were higher in Terebralia sp. at sites 2 and 3 (51.9 ng/g and 94.5 ng/g, respectively) and in Uca sp. at sites 2 (52.3 ng/g) and 3 (73.0 ng/g). Proportion of LMW PAHs was significantly (p < 0.05) high for Crassostrea cucullata at all sites (64.1–70.3%) and for Panaeus monodon at sites 1 and 3 (65.2–74.4%).
Table 2. Concentration (in ng/g) of the 19 individual PAHs, total PAHs, total cPAHs (which represents the total concentration of carcinogenic PAHs including BaA, Chr, BbF, BkF, BaP, IP, and DahA), LMW (low molecular weight PAH with2 and 3 rings), HMW PAH (high molecular weight PAH with 4, 5, and 6 rings), and % LMW PAH in samples of dominant fauna from their respective collection sites in the Rufiji estuary.
PAH distribution in fish (teleost) is presented in Table 3. Total PAHs ranged from 35.1 to 137 ng/g. High PAH concentrations were recorded in Arius thalassinus which is a benthic fish. In contrast, Hilsa kelee, a pelagic feeder, indicated low PAH concentrations (Tables 1, 3). The proportion of LMW PAHs was statistically higher (p < 0.05) for H. kelee at both sites, whereas total cPAH concentration was significantly higher (p < 0.0) for Trichrirus leptrus (benthopelagic feeder) at site 2 and for A. thalassinus at site 3.
Table 3. Concentration (in ng/g) of the 19 individual PAHs, total PAHs, total cPAHs (which represents the total concentration of carcinogenic PAHs +including BaA, Chr, BbF, BkF, BaP, IP, and DahA), LMW PAHs with 2 and 3 rings, HMW PAHs with 4, 5, and 6 rings), and % LMW PAH in samples of three dominant teleost from their respective collection sites in the Rufiji estuary.
Compositional patterns of both SPM and sediments varied across the sampling sites as revealed by a plot of non-multidimensional scaling (MDS) of all samples (Figures 2A,B). Sediment samples from site 1 grouped separately from sites 2 and 3 (Figure 2A). However, sediment samples from site 1 (Sd1) grouped with SPM samples from sites 1 (Sp1) and 2 (Sp2), which suggest that total PAH levels, as well as the pattern of individual PAHs in these samples are similar, and probably share the same source(s) or processes responsible for their distribution in the estuary. SPM samples from site 3 (Sp3) grouped separately from rest of the sample types and sites (Figure 2A). This feature suggests a different pattern, with a higher predominance of LMW PAHs other than phenanthrene (Table 1). This site is located close to the area where several fishing boats are stationed, which are reloaded and refueled regularly. Consistent with this observation, PAHs in SPM consist mostly of LMW volatile components suggesting a strong contribution of fuel spilled by boats and exhaust fumes released by engines. These results indicate that strong guidelines should be enforced for refueling, engines used in the motorized boats, and effluents released to reduce the environmental effects.
Figure 2. Non-Metric Multidimensional Scaling analysis (NMDS) plots. (A) Ordination of sampled sites in the Rufiji estuary. (B) Non-Metric Multidimensional Scaling analysis (NMDS) plots. Ordination of PAH groupings along the sampled sites. Stress factor = 0.13. Na, naphthalene; Ac, acenaphthylene; Ace, acenaphthene; Flu, fluorine; An, anthracene; Ph, phenanthrene; Fln, fluoranthene; Pyr, pyrene; BaA, benzo[a]anthracene; Chr, chrysene; BaP, benzo[a]pyrene; BeP, benzo[e]pyrene; BbF, benzo[b]fluoranthene; BjF, benzo[j]fluoranthene; BkF, benzo[k]fluoranthene; Bghi, benzo[hgi]perylene; IP, Indeno[1,2,3-cd]pyrene; Per, perylene; DahA, dibenzo[a,h]anthracene. Stress factor = 0.01.
Reduced amount of total PAH in both SPM and sediment samples from site 1 indicates that quantitatively, terrigenous inputs were not a significant contributor of PAHs within this part of the estuary. High PAH levels at sites 2 and 3 however suggest contribution of terrestrial inputs of these compounds, perhaps due to land use undertakings, such as rice cultivation, fishing, and harvesting firewood, charcoal, and mangroves poles. The large fraction of fine particles brought into these sites by enhanced freshwater outflow (Erftemeijer and Hamerlynck, 2005) as well as the high proportion of terrestrial organic matter present in SPM and surface sediments from these parts of the Rufiji estuary (Shilla and Routh, 2017) favor adsorption of PAHs. This reasoning is also most likely responsible for greater accumulation of PAHs at both sites.
Figure 2B showed three distinct groups of PAHs. Group I consisted of LMW PAHs (acenaphthylene, anthracene, and phenanthrene), whereas group II had HMW PAHs (fluoranthene, pyrene, benzo[a]anthracene, chrysene, benzo[a]pyrene, benzo[b]fluoranthene, benzo[g,h,i]perylene, perylene, Indeno[1,2,3-cd]pyrene, dibenzo[a,h]anthracene). Group III had a mixture of LMW PAHs (naphthalene and acenaphthene) and HMW PAHs (benzo[e]pyrene, benzo[j]fluoranthene, and benzo[k]fluoranthene. These PAHs did not have a trend, and they were often below the detection limit (Table 1). If we exclude the third group of PAHs as indicated in Figure 2B, similar trend and PAH groupings have been reported in other estuaries (e.g., Yong and Carniglie, 1995; Countway et al., 2003; Ranjan et al., 2012; Shilla, 2016).
The LMW PAHs (particularly phenathrene) were abundant in SPM samples, ranging from 32.6 to 59.5%. In contrast, the HMW PAHs dominated in sediment samples. This trend is related to differences in physical and chemical properties between the LMW and HMW PAHs. According to van Zoest and van Eck (1990), the solubilities and vapor pressure of LMW PAHs are high, while their hydrophobicity, as reflected by the octanol-water partition coefficients, are low, causing a profound influence on their environmental behavior. The high proportion of LMW PAHs in SPM suggests adsorption to SPM and less sequestration of these PAHs to bottom sediments. On the other hand, HMW PAHs are largely sequestrated and deposited on bottom sediments, and this has been reported in other studies also (Brion and Pelletier, 2005; Shilla, 2016).
The relationship between PAH and POC is presented in Table 4. POC content is one of the important factors that controls the concentration, transport and fate of PAHs in aquatic environment (Zhou et al., 1998; Countway et al., 2003; Shilla, 2016). Sorption of organic compounds is dominated by hydrophobic interactions and organic matter is one of the primary surfaces for sorption in aquatic environments (Zhou et al., 1998). Assessment of the relationships between PAH and POC in the Rufiji estuary shows that both LMW and HMW PAHs had a very weak positive correlation with POC, except for acenaphthylene and phenanthrene (r = 0.60 and 0.64, respectively). Lack of correlation could mean that the type, quality and quantity of POC pool in the Rufiji estuary played an important role in transport and fate of these hydrophobic contaminants. This possibility led to further investigation of the relationship between individual PAHs and fatty acid biomarkers as discussed below.
Table 4. Regression coefficients for relationship between SPM and sediment PAHs and environmental variables.
Details of fatty acid biomarker distribution and abundance in the Rufiji estuary were reported by us earlier (Shilla and Routh, 2017). The postulated relationship between PAH and specific POC pools are presented in Table 5. Polyunsaturated fatty acids (PUFA) trace fresh and labile organic matter derived from macroalgae, diatom, and dinoflagellate markers in aquatic environments (Countway et al., 2003). PUFAs correlated positively with anthracene and phenathrene in SPM samples (r = 0.71 and 0.76, respectively), whereas a negative correlation was observed between them and HMW PAHs such as chrysene, benzo[a]anthracene, and perylene (Table 4). A similar trend was observed in the sediment samples too. The strong relationship between biomarkers for autochthonous particulate organic matter and group I PAHs could result from the growing pool of phytoplankton available for preferential partitioning of volatile PAHs. Comparable observations have been made for PAHs in the York River estuary (Countway et al., 2003), Manko estuary in Okinawa (Shilla, 2016), and PCBs in lakes located in northwestern Ontario (Dachs et al., 1999).
Most of the HMW PAHs (pyrene, chrysene, benzo[a]anthracene, and perylene) strongly correlated with saturated fatty acids (SAFA; r = 0.58 to 0.72) and long chain-chain fatty acids (LCFA; r = 0.59 to 0.60; Table 4). However, these PAHs correlated negatively with PUFAs (r = −0.58 to −0.64). SAFAs were largely composed of 16:0 and 18:0 carbon chains and attributed to a mixed planktonic source (i.e., phytoplankton, zooplankton, and bacteria). Nevertheless, these FAs, in addition to the PUFA 18:2ω6, have also been associated with agricultural products. Likewise, 16:0, 18:0, and 18:1ω9 have been recorded as a dominant component in areas exposed to agricultural and residential runoff wastes (Shilla et al., 2011). These relationships suggest that a considerable amount of HMW PAHs are derived from surface runoff and they are attached to terrestrially-derived detrital organic matter in surface water and sediments. Other alternative could be, these PAHs and terrestrially-derived organic matter are unassociated, but they have similar ways of entry into the estuarine environment. Further investigations on processes/factors which influence the spatial and temporal variability of pollutant sources will help in understanding the fate of these pollutants.
PAH concentrations in the Rufiji samples when compared to other estuarine sites are less contaminated. According to the classification system suggested previously by Baumard et al. (1998a), SPM and sediment samples from the Rufiji estuary have low to moderate contamination with total PAH concentration of 18.7–376 ng/g. These values are lower than those found in sites severely impacted by human activities, such as a value of 100 to > 5,000 ng/g measured in sediments from the intertidal areas of Dar es Salaam, Tanzania (Gaspare et al., 2009) or values as high as 20,500 ng/g in the Masan Bay, Korea (Yim et al., 2005). Thus, results from the current study indicate that environmental samples and aquatic organisms in the Rufiji estuary have PAH levels that pose a low risk to the environment (ATSDR, 1995).
In previous section, the distributional composition of PAHs was used to examine PAH sources, along with MDS plots that indicated distinct compositional patterns of PAHs, resulting into two major groups of LMW and HMW PAHs. Except for perylene, other group II PAHs (i.e., fluoranthene, pyrene, benzo[a]anthracene, chrysene, benzo[a]pyrene, benzo[b]fluoranthene, benzo[k]fluoranthene, benzo[g,h,i]perylene, dibenzo[a,h]anthracene) are usually products of high temperature combustion or pyrolytic processes (Yunker et al., 2002). Nonetheless, the association between perylene and other PAHs in this study may suggest that these PAHs were probably derived from similar sources or input processes. As mentioned earlier, freshwater inflow into the estuary constitutes a main input pathway for these PAHs.
PAH isomer ratios, such as An/128, Fl/Fl+Py and BaA/228, are commonly used to apportion PAH sources (Yunker et al., 2002). According to the measurements of PAH isomer ratios compiled by Yunker et al. (2002), an An/178 ratio of 0.10 is defined as the petroleum/combustion transition point. Fl/Fl+Py < 0.40 suggests petroleum, 0.40–0.50 suggests petroleum combustion, and >0.50 suggests combustion of coal, grass and wood. Ratio of BaA/228 < 0.20 suggests petroleum, values from 0.20 to 0.34 suggest either petroleum or combustion, and >0.35 implies combustion.
The isomer ratios calculated for SPM samples from three sites in the Rufiji estuary ranged from 0.10 to 0.40 for An/128; 0.20 to 0.50 for Fl/Fl+Py; and 0.20 to 0.42 for BaA/228. This indicates a mixed contribution from petroleum and combustion derived inputs of PAHs. The ratios calculated in sediment samples for An/128 (0.20–0.30) and BaA/228 (0.20–0.42) suggest combustion-derived sources. Likewise, the ratio of Fl/Fl+Py (0.40–0.50) suggests a strong input from biomass combustion. Consistent with this idea, as suggested earlier, sites 2 and 3 receive freshwater inflow from the surrounding areas where harvesting of firewood and charcoal and burn and slash agriculture practice potentially increases the HMW PAH input into the Rufiji estuary. The PAH ratios however did not vary significantly between the sites.
PAH contents in organisms varied according to the different species, their location in the water column and their feeding habits (i.e., pelagic and benthic organisms). A close comparison with other studies indicate differences in extraction protocols and quantification of LMW and HMW PAHs. However, total PAH levels in invertebrates and fish from non-polluted environments reported by several authors as reviewed by Hussein et al. (2016) are in the same range as those found in this study. Although no data about PAH distribution in the Rufiji estuary is available, studies performed from other places could be compared with our results. For example, Gaspare et al. (2009) analyzed PAHs in oysters from the inter-tidal areas of Dar es Salaam, a site that is more affected by human activities than the Rufiji estuary. The authors reported PAH levels exceeding > 500 ng/g, whereas in the current study comparable organisms such as clams, never exceeded 130 ng/g of total PAHs. Another study by Deb et al. (2000) analyzed PAHs in 11 different fish species from the Hiroshima Bay and reported levels of some individual PAHs exceeding 1,000 ng/g.
PAH distribution patterns in pelagic feeders (C. cucullate, P. monodon, and H. kelee) are characterized by the abundance of LMW PAHs. The results indicate that these organisms are exposed to less hydrophobic compounds, and they accumulate these PAHs adsorbed on floating particles, such as fine SPM and phytoplankton, that are also the principal food sources for these organisms. Similar observations have been reported in previous studies (Hellou et al., 1993; Soclo et al., 2008; Gaspare et al., 2009). In contrast, the semi-pelagic and benthic feeders (Terebralia sp., Uca sp., T. lepturus, and A. thalassinus) have relatively higher proportions of HMW PAHs (Tables 2, 3), reflecting the presence of these PAHs in the feed consumed by these animals in the estuary.
Bioaccumulation factors [BAF; (Soclo et al., 2008)] that were obtained by dividing PAH concentration in tissue by PAH concentration in sediments, ranged between 0.20 and 69.5. The low values of BAF clearly indicate that PAHs have not accumulated in the animals investigated in this study. Naphthalene and phenathrene had the highest values for BAF (29.5 and 69.5, respectively) in the muscular tissues of pelagic and filter feeding animals (H. kelee and C. cucullata) at site 3. Although sediment samples at this site contained high concentrations of HMW PAHs (Table 1), very low values for BAF were observed in the animals. This confirms that pelagic and filter feeders exhibit high affinity for LMW PAHs compared to HMW PAHs. Furthermore, the results indicate that low values of BAF for HMW PAHs even for benthic feeders such as Terebralia sp. and A. thalassinus, are not adequate as guard species to detect the risks of PAH contamination in the Rufiji estuary. Hence, additional comparative studies, whereby PAH levels in animals, sediments, and SPM samples from the same sites need to be correlated and compared with other sites in the Rufiji estuary, and during different seasons to confirm this hypothesis.
The results of this investigation show that all samples (SPM, surface sediments and specific animals in a telescoping food chain) from the Rufiji estuary have PAH levels that are currently of limited environmental concern. The distribution of PAHs in this estuary suggest their origin as petrogenic and combustion products derived from burning of coal and biomass (grass and wood). Moreover, the geochemical trends match well with human activities in the upstream and downstream riparian areas in the catchment. It is our belief that improvement and management of land use practices and stricter environmental regulations will result in reducing the terrestrial contaminant inputs that will decrease PAH levels in the Rufiji estuary.
Ethical clearance was sought before beginning the study. Clearance for experiments involving animals was obtained from the Directorate of Research and Consultancy at the University of Dar es Salaam. This was forwarded to the Regional and District Administrative Secretaries, respectively in the localities where sampling was conducted. Finally, all animals used in this study were euthanized prior to laboratory testing according to the pre-approved protocol.
Fieldwork was led by DS. Laboratory work was led by DS and JR. The paper was written by DS with contributions from JR.
The authors declare that the research was conducted in the absence of any commercial or financial relationships that could be construed as a potential conflict of interest.
DS is grateful to the Swedish Institute (SI), which provided financial support for this work during her post-doctoral training. The following persons are kindly thanked for their help in this research: Mr. Richard Masinde and Amos Lugata, for sampling and initial processing of samples, Dr. Daniel Shilla for organizing the sampling campaign, Susanne Karlsson and Lena Lundman for valuable practical assistance in the lab. We thank the reviewers for their suggestions. The study was supported from funds provided by Vetenskapsrådet (Grant 348-211-7408) to JR.
Anderson, M. J., Gorley, R. N., and Clarke, K. R. (2008). PERMANOVA+ for PRIMER: Guide to Software and Statistical Methods. Plymouth: PRIMER-E.
ATSDR (1995). (Agency for Toxic Substances and Disease Registry) Toxicological Profile for Polycyclic Aromatic Hydrocarbons (PAHs). Atlanta, GA: Public Health Service, U.S. Department of Health and Human Services.
Baumard, P., Budzinski, H., and Garrigues, P. (1998a). Polycyclic aromatic hydrocarbons in sediments and mussels of the western Mediterranean Sea. Environ. Toxicol. Chem. 17, 765–776.
Bouloubassi, I., and Saliot, A. (1993). Dissolved, particulate and sedimentary naturally derived polycyclic aromatic hydrocarbons in a coastal environment: geochemical significance. Mar. Chem. 42, 127–143. doi: 10.1016/0304-4203(93)90242-G
Brion, D., and Pelletier, E. (2005). Modelling PAHs adsorption and sequestration in freshwater and marine sediments. Chemosphere 61, 867–876. doi: 10.1016/j.chemosphere.2005.04.097
Countway, R. E., Dickhut, R. M., and Canuel, E. A. (2003). Polycyclic aromatic hydrocarbon (PAH) distributions and associations with organic matter in surface waters of the York River, VA Estuary. Org. Geochem. 34, 209–224. doi: 10.1016/S0146-6380(02)00162-6
Deb, S. C., Araki, T., and Fukushima, T. (2000). Polycyclic aromatic hydrocarbons in fish organs. Mar. Pollut. Bull. 40, 882–885. doi: 10.1016/S0025-326X(00)00090-4
Dachs, J., Eisenreich, S. J., Baker, J. E., Ko, F. C., and Jeremiason, J. D. (1999). Coupling of phytoplankton uptake and air-water exchange of persistent organic pollutants. Environ. Sci. Tech. 33, 3653–3660. doi: 10.1021/es990168o
Denissenko, M. F., Pao, A., Tang, M., and Pfeifer, G. P. (1996). Preferential formation of benzo[a]pyrene adducts at lung cancer mutational hotspots in P53. Science 274, 430–432. doi: 10.1126/science.274.5286.430
Elliott, M., and Whitfield, A. K. (2011). Challenging paradigms in estuarine ecology and management. Est. Coast. Shelf Sci. 94, 306–314. doi: 10.1016/j.ecss.2011.06.016
Erftemeijer, P. L. A., and Hamerlynck, O. (2005). Die-back of the mangrove Heritiera littoralis dryland, in the Rufiji Delta (Tanzania) following El Nino floods. J. Coast. Res. 42, 228–235. Available online at: http://www.jstor.org/stable/25736988
Gaspare, L., Machiwa, J. F., Mdachi, S. J., Streck, G., and Brack, W. (2009). Polycyclic aromatic hydrocarbon (PAH) contamination of surface sediments and oysters from the inter-tidal areas of Dar es Salaam, Tanzania. Environ. Pollut. 157, 24–34. doi: 10.1016/j.envpol.2008.08.002
Hellou, J., Upshall, C., Payne, J. F., Naidu, S., and Paranjape, M. A. (1993). Total unsaturated compounds and polycyclic aromatic-hydrocarbons in mollusks collected from waters around Newfoundland. Arch. Environ. Contamin. Toxicol. 24, 249–257. doi: 10.1007/BF01141355
Hussein, I., and Abdel-Shafy Mansour, M. S. M. (2016). A review on polycyclic aromatic hydrocarbons: source, environmental impact, effect on human health and remediation. Egypt. J. Petrol. 25, 107–123. doi: 10.1016/j.ejpe.2015.03.011
International Agency for Research on Cancer (1987). “IARC monographs on the evaluation of the carcinogenic risk of chemicals to humans,” in Overall Evaluation of Carcinogenicity: An updating of IAPC Monographs, Vols. 1–42. (Lyon: International Agency for Research on Cancer).
Kim, H. Y., and Salem, N. Jr. (1990). Separation of lipid classes by solid phase extraction. J. Lipid Res. 31, 2285–2289.
Lipid library (2011). NIST online library, and Archives of Mass Spectrometry. Available online at: http://lipidlibrary.aocs.org/ms/arch_xyz/index1.htm
McGroddy, S. E., and Farrington, J. W. (1995). Sediment porewater partitioning of polycyclic aromatic hydrocarbons in three cores from Boston Harbor, Massachusetts. Environ. Sci. Tech. 29, 1542–1550. doi: 10.1021/es00006a016
McGroddy, S. E., Farrington, J. W., and Gschwend, P. M. (1996). Comparison of in situ and desorption sediment-water partitioning of polycyclic aromatic hydrocarbons and polychlorinated biphenyls. Environ. Sci. Tech. 30, 172–177. doi: 10.1021/es950218z
McVeety, B. D., and Hites, R. A. (1988). Atmospheric deposition of polycyclic aromatic hydrocarbons to water surfaces: a mass balance approach. Atmos. Environ. 22, 511–536. doi: 10.1016/0004-6981(88)90196-5
Mremi, S. D., and Machiwa, J. F. (2003). Heavy metal contamination of mangrove sediments and the associated biota in Dar es Salaam, Tanzania. Tanz. J. Sci. 29, 69–76. doi: 10.4314/tjs.v29i1.18367
Mwevura, H., Othman, O. C., and Mhehe, G. L. (2002). Organochlorine pesticides residue in edible biota from the coastal area of Dar es Salaam city. West. Indian Ocean J. Mar. Sci. 1, 91–96.
Peters, K. E., Walters, C. C., and Moldowan, J. M. (2005). The Biomarker Guide, 2nd Edn. Cambridge, UK: Cambridge University Press.
Phillips, D. H., and Grover, P. L. (1994). Polycyclic hydrocarbon activation: Bay regions and beyond. Drug Metab. Rev. 26, 443–467. doi: 10.3109/03602539409029808
Pulich, W. M. Jr., Winters, K., and Baalen, C. (1974). The effects of a No. 2 fuel oil and two crude oils on the growth and photosynthesis of microalgae. Mar. Biol. 28, 87–94. doi: 10.1007/BF00396299
Ranjan, R. K., Routh, J., Ramanathan, A. L., and Klump, J. V. (2012). Polycyclic aromatic hydrocarbon fingerprints in the Pichavaram mangrove-estuarine sediments, southeastern India. Org. Geochem. 53, 88–94. doi: 10.1016/j.orggeochem.2012.08.007
Readman, J. W., Mantoura, R. F. C., Rhead, M. M., and Brown, L. (1982). Aquatic distribution and heterotrophic degradation of polycyclic aromatic hydrocarbons (PAH) in the Tamar Estuary. Est. Coast. Shelf Sci. 14, 369–389. doi: 10.1016/S0272-7714(82)80009-7
REMP. (2003). A summary Report on biodiversity of Rufiji District –. Rufiji Environmental Management Project Technical Report No. 44.
Routh, J., Gustaf, H., and Peter, K. (2014). Multi-proxy study of soil organic matter dynamics in permafrost peat deposits reveal vulnerability to climate change in the European Russian Arctic. Chem. Geol. 368, 104–117. doi: 10.1016/j.chemgeo.2013.12.022
Shilla, D. J. (2016). Distribution and Behavior of Polycyclic Aromatic Hydrocarbon (PAH) In Sub-tropical River-Estuary, Okinawa Japan. Chem. Ecol. 32, 472–491. doi: 10.1080/02757540.2016.1152945
Shilla, D. J., and Routh, J. (2017). Using biochemical and isotopic tracers to characterize organic matter sources and their incorporation into estuarine food webs (Rufiji delta, Tanzania). Chem. Ecol. 33, 893–917. doi: 10.1080/02757540.2017.1391796
Shilla, D. J., Tsuchiya, M., and Abel, D. S. (2011). Terrigenous nutrient and organic matter in a subtropical river estuary, Okinawa, Japan: Origin, distribution and pattern across the estuarine salinity gradient. Chem. Ecol. 27, 523–554. doi: 10.1080/02757540.2011.600831
Silliman, J. E., Meyers, P., and Eadie, J. B. (1999). Perylene: an indicator of alteration processor precursor material? Org. Geochem. 29, 1737–1744.
Soclo, H. H., Budzinski, H., Garrigues, P., and Matsuzawa, S. (2008). Biota accumulation of polycyclic aromatic hydrocarbons in Benin coastal waters. Polycycl. Arom. Comp. 28, 112–127. doi: 10.1080/10406630801940530
Soclo, H. H., Garrigues, P., and Ewald, M. (2000). Origin of polycyclic aromatic hydrocarbons (PAHs) in coastal marine sediments: case studies in Cotonou (Benin) and Aquitaine (France) areas. Mar. Pollut. Bull. 40, 387–396. doi: 10.1016/S0025-326X(99)00200-3
Sørensen, C. (1998). Fisher, Farmer, Forester: Smallholder Management in the Rufiji Delta of Tanzania. Danish Council for Development Research Field Report.
Taylor, M., Green, E., and Ravilious, C. (2003). Mangroves of East Africa. UNEP – World Conservation Monitoring Centre, Biodiversity Series No 13, 25.
UNEP (2001). Eastern Africa Atlas of coastal resources: Tanzania. Nairobi: United Nations Environment Programme.
van Zoest, R., and van Eck, G. T. M. (1990). Behavior of particulate polychlorinated biphenyls and polycyclic aromatic hydrocarbons in the Scheldt Estuary. Nether. J. Sea Res. 26, 89–96. doi: 10.1016/0077-7579(90)90059-P
Wakeham, S. G., McNichol, A. P., and Kostka, J. E. (2006). Natural abundance radiocarbon as a tracer of assimilation of petroleum carbon by bacteria in salt marsh sediments. Geochim. Cosmochim. Acta 70, 1761–1771. doi: 10.1016/j.gca.2005.12.020
Wakeham, S. G., Peterson, M. L., and Hedges, J. I. (2002). Lipid biomarker fluxes in the Arabian Sea, with a comparison to the equatorial Pacific Ocean. Deep Res. II Top. Stud. Oceanogr. 49, 2265–2301. doi: 10.1016/S0967-0645(02)00037-1
Yang, S. K., and Silverman, B. D. (1988). Polycyclic Aromatic Hydrocarbon Carcinogenesis: Structure–Activity Relationships. Boca Raton, FL: CRC Press.
Yim, U. H., Hong, S. H., Shim, W. J., Oh, J. R., and Chang, M. (2005). Spatio-temporal distribution and characteristics of PAHs in sediments from Masan Bay, Korea. Mar. Pollut. Bull. 50, 319–326. doi: 10.1016/j.marpolbul.2004.11.003
Yong, L. Y., and Cerniglia, C. E. (1995). Microbial Transformation and Degradation of Toxic Organic Chemicals. New York, NY: Wiley.
Yunker, M. B., Macdonald, R. W., Vingarzan, R., Mitchell, R. H., Goyette, D., and Sylvestre, S. (2002). PAHs in the Fraser River basin: a critical appraisal of PAH ratios as indicators of PAH source and composition. Org. Geochem. 33, 489–515. doi: 10.1016/S0146-6380(02)00002-5
Zhao, S. M., Wang, D. W., Li, X. M., Huang, B., Hu, P., Zhang, L. W., et al. (2014). Environmental behavior of PAHs in Dianchi lake: distributions, sources and risk assessment of polycyclic aromatic hydrocarbons in surface sediments from Dianchi Lake, China. Int. J. Environ. Res. 8, 317–328. doi: 10.22059/ijer.2014.722
Keywords: PAHs, suspended matter, sediment, animal tissues, bioaccumulation, Rufiji estuary
Citation: Shilla DJ and Routh J (2018) Distribution, Behavior, and Sources of Polycyclic Aromatic Hydrocarbon in the Water Column, Sediments and Biota of the Rufiji Estuary, Tanzania. Front. Earth Sci. 6:70. doi: 10.3389/feart.2018.00070
Received: 20 February 2018; Accepted: 17 May 2018;
Published: 07 June 2018.
Edited by:
Shiny Mathews, North Dakota State University, United StatesReviewed by:
Neal Michelutti, Queen's University, CanadaCopyright © 2018 Shilla and Routh. This is an open-access article distributed under the terms of the Creative Commons Attribution License (CC BY). The use, distribution or reproduction in other forums is permitted, provided the original author(s) and the copyright owner are credited and that the original publication in this journal is cited, in accordance with accepted academic practice. No use, distribution or reproduction is permitted which does not comply with these terms.
*Correspondence: Joyanto Routh, am95YW50by5yb3V0aEBsaXUuc2U=
Disclaimer: All claims expressed in this article are solely those of the authors and do not necessarily represent those of their affiliated organizations, or those of the publisher, the editors and the reviewers. Any product that may be evaluated in this article or claim that may be made by its manufacturer is not guaranteed or endorsed by the publisher.
Research integrity at Frontiers
Learn more about the work of our research integrity team to safeguard the quality of each article we publish.